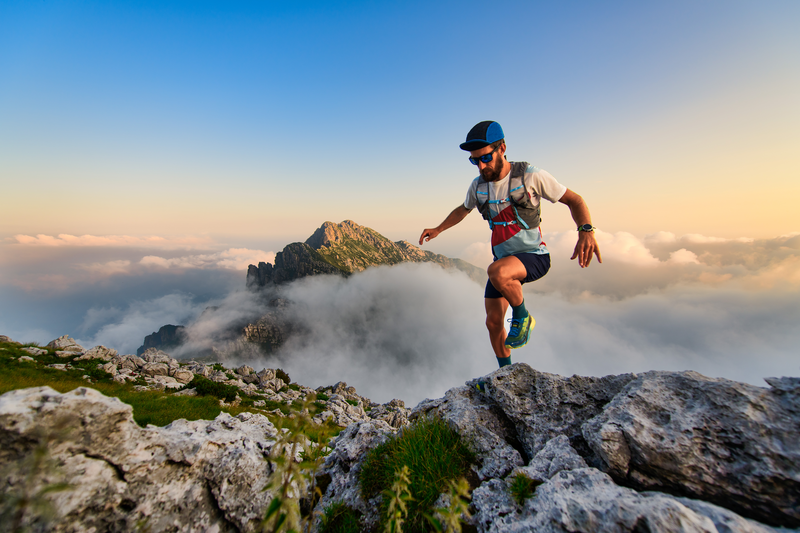
95% of researchers rate our articles as excellent or good
Learn more about the work of our research integrity team to safeguard the quality of each article we publish.
Find out more
ORIGINAL RESEARCH article
Front. Nutr. , 24 August 2022
Sec. Nutrition and Food Science Technology
Volume 9 - 2022 | https://doi.org/10.3389/fnut.2022.963655
Nine-processed tangerine peel (Jiuzhi Chenpi in Chinese) is a famous Chinese traditional snack. The composition and contents of volatile substances during its processing is unclear. Gas chromatography combined with ion mobility spectrometry (GC-IMS) was applied to determine the characteristic changes of volatile components throughout the production process. Four stages such as untreated dry tangerine peel (raw material), debittered tangerine peel, pickled tangerine peel, and final product were examined. A total of 110 flavor compounds including terpenes, alcohols, aldehydes, ketones, esters, acids, and two others were successfully detected in tangerine peel samples across the various production stages. There were abundant amounts of terpenes contributing to the flavor, including limonene, gamma-terpinene, alpha-pinene, myrcene, beta-pinene, and alpha-thujene which were reduced at the later stage of production. Large amounts of esters and alcohols such as methyl acetate, furfuryl acetate, ethyl acetate, benzyl propionate, 2-hexanol, linalool, and isopulegol, were diminished at the early stage of processing, i.e., soaking for debittering. One the other hand, the final product contained increased amount of aldehydes and ketones including pentanal, hexanal, 2-hexenal, 2-heptenal (E), 2-pentenal (E), 1-penten-3-one, 6-methyl-5-hepten-2-one, 2-methyl-2-propenal, and 2-cyclohexen-1-one, and very high level of acetic acid. Present findings help to understand the formation of the unique flavor of nine-processed tangerine peel and provide a scientific basis for the optimization of processing methods and quality control.
Nine-processed tangerine peel (Jiuzhi Chenpi in Chinese) is a famous traditional snack in China, originated from the Chaoshan region in Guangdong Province in China. The term “nine-process” describes the complicated and rigorous production process, which involves picking up (CP1), soaking (CP2), keeping fresh, peeling, pickling (CP3), draining, seasoning, repeated drying, and lastly storage (aging; CP4) of tangerine peels (1). With the addition of licorice, salt, and sugar though the manufacturing produces, this preserved food shows a sweet, salty, aromatic, and pungent flavor.
Nine-processed tangerine peel has become a famous snack in China due to its characteristic flavor. However, there have been limited studies on its volatile constituents, which possess potent efficacy and affect the flavor of nine-processed tangerine peel. In particular, the impact of different manufacturing procedures on the contents of volatile components and thereby the flavor of final product remains unclear. Flavor is not only an indicator for evaluating the food quality, but also a key factor affecting consumer satisfaction and market value of food products. The volatile components vary due to different processing and storage methods. Throughout the complicated series of manufacturing procedures, the volatile components will be changed and result in the unique flavor of nine-processed tangerine peel. Moreover, the volatile ingredients may possess pharmacological effects as suggested in previous studies (2, 3).
New technique having gas chromatography combined with ion mobility spectrometry (GC-IMS) allows the separation and identification of flavor molecules based on their migration under an electric field at specific pressures and temperatures (4). GC-IMS has low detection limits and high sensitivity without any pre-treatment requirement, making it easy to use and advantageous for accurate characterization and quantification of the volatile components in the sample. GC-IMS can be used to classify and analyze samples according to the intensity of the signal peaks of each component in the sample through its accompanying software function, and to create fingerprint profiles. Displaying the results in color images, GC-IMS allows us to detect the differences among samples visually. With these aforementioned advantages, GC-IMS has widely been used in food quality analysis and volatile compound identification (5, 6).
A recent study by gas chromatography–olfactometry-mass spectrometry (GC-O-MS) has identified the presence of 58 volatile components and their changes in contents during the pickling and storage of nine-processed tangerine peel, among which D-limonene was the major volatile component, up to 81.84% for different storage time periods (7). However, the composition and contents of volatile substances at each stages of the processing of nine-processed tangerine peel is still unclear and need to be further investigated. In this study, we used GC-IMS to explore in depth the changes of volatile components during the processing of nine-processed tangerine peel. Four stages in the processing were selected for examination: untreated dry tangerine peel (raw material; CP1), debittered tangerine peel (CP2), pickled tangerine peel (CP3), and final product (CP4), as they are the most crucial stages affecting the flavor. This study explored the characteristic changes of the volatile components throughout the process to understand the formation of the unique flavor of nine-processed tangerine peel and provide a scientific basis for the optimization of processing methods and quality control.
In this study, all samples were provided by Guangdong Jiabao Group Co., Ltd. (Guangdong, China). In brief, the production process included picking up (CP1), soaking (CP2), keeping fresh, peeling, pickling (CP3), draining, seasoning, repeated drying, and lastly storage (aging; CP4). Picking up was to select thick dried tangerine peel obtained from ripened tangerine as raw material (CP1). Soaking in water could debitter and soften the tangerine peel whilst removing impurities (CP2). Then the tangerine peel were cut into filamentous or blocky shape. Pickling involved soaking with 8–10% salt solution to further remove bitter substances by high osmotic pressure and to break the fibers in tangerine peel (CP3), followed by draining of water and remaining salt solution. Licorice, cyclamate, vanillin, citric acid, and others were added for seasoning. The tangerine peel was dried to ∼38% moisture content using 45–50°C heat pump. Importantly, the nine-processed tangerine peel were stored at room temperature for 0.5–1 year so that various flavor substances could be transformed and released to form the unique flavor of final products, which were packaged for sale after passing the necessary food quality and safety tests (CP4). The tangerine peel samples were divided into four groups based on different stages in the processing of nine-processed tangerine peel: untreated dry tangerine peel (CP1), deastringent and debittered tangerine peel (CP2); pickled tangerine peel (CP3), and final product (CP4). Three independent batches of samples were obtained from each of these four stages of processing. The samples were powdered with a pulverizer (Qingdao Juchuang Environmental Protection Group Co., Ltd. JC-FW-200) and passed through a 100-mesh sieve for further GC-IMS analysis.
Gas chromatography combined with ion mobility spectrometry analysis of tangerine peel samples were performed by FlavourSpec® (GAS Dortmund Co., Dortmund, German), equipped with a PAL3 autosampler system (CTC Analytics AG, Zwingen, Switzerland). Each sample powder (1 g) was put in a 20 mL vial for analysis. Headspace sampling was selected as the injection method. After heating at an oscillatory rate of 500 rpm at 60°C for 15 min, a 200-μL sample headspace was automatically injected by a heated syringe (85 °C) into the GC-IMS system. The FS-SE-54-CB-1 capillary column (15 m × 0.53 mm i.d. × 1.0 μm) from CS-Chromatographie Service GmbH (Langerwehe, Germany) was heated at 60°C. Purified nitrogen gas (99.999% purity) was used as the carrier. The carrier gas flow rate was programmed as follows: the flow rate was 2 mL/min for the first 2 min, the internal linearity was increased to 10 mL/min within 8 min, followed by 100 mL/min within 10 min, and then reached 150 mL/min within the last 10 min. The drift tube was 9.8 cm long and operated at a 500 V/cm constant voltage with a 150 mL/min nitrogen flow at 45°C.
Gas chromatography combined with ion mobility spectrometry technology was equipped with VOCal software for data collection and analysis, as well as NIST database and IMS database for quantitative analysis of volatile substances. The GC-IMS instrument also provided with three plugins: (a) Reporter plug-in for direct comparison among samples from 3D spectrum, 2D top view spectrum and differential map; (b) Gallery Plot plugin for fingerprint comparison; and (c) Dynamic principal component analysis (PCA) plugin for clustering samples into different types.
Four groups of samples represented different stages during the production of nine-processed tangerine peel. The changes of volatile substances were determined by GC-IMS analysis and clearly visualized in the 3D (Figure 1A) and 2D topographic spectra (Figure 1B). In the spectra, X-axis represents the drift time in the drift tube while Y-axis represents the retention time of gas chromatography. The red vertical line at abscissa 1.0 is reactive ion peak (RIP) after normalization of the data and blue color is set as background for the whole plot. Each volatile compound is present as a point on either side of RIP with color indication for its quantity, i.e., red indicates higher concentration, yellow and then white indicate lower concentration, and so on. The ionized molecules can be affected by factors including chemical properties, concentration of analyte, and temperature of drift tube (8).
Figure 1. GC-IMS spectra of different tangerine peel samples. (A) 3D-topographic spectra. (B) 2D-topographic spectra. CP1, untreated dry tangerine peel raw material; CP2, deastringent and debittered tangerine peel; CP3, pickled tangerine peel; CP4, finished nine-processed tangerine peel.
To better identify the differences among the four samples, we used CP1 as a reference for topographic plot deduction to get a differential plot (Figure 2). In terms of signal intensity compared to the reference, blue means a lower concentration than the reference and the darker the color, the lower the concentration; and vice versa, red means a higher concentration than the reference and darker the color, the higher the concentration. For volatile compounds at the same concentration, the background is white after the deduction. In total, 110 volatile substances were identified in each tangerine peel sample from four different processing stages by identification approach based on the retention index (RI) and drift time in the drift tube (Dt), and were listed in Table 1. The substances were grouped by types with 18 of them unidentified. There were 9 terpenes, 12 alcohols, 17 aldehydes, 13 ketones, 8 esters, 2 acids, and 2 others detected. Dimers and trimers of some compounds were also detected.
Table 1. Quantitative analysis result of volatile compounds found in different processing stages of nine-processed tangerine peel using GC-IMS.
Ion mobility spectrometry is a fast and sensitive way to detect molecules with low concentration (ppbv levels) (9). Combining the separation properties of GC with the fast response of IMS, GC-IMS can effectively employed for identification and differentiation of volatile compounds in foods. A great variety in the volatile compositions was observed across the processing of nine-processed tangerine peel. Some detected compounds gradually increased while other flavor compounds diminished with the depth of processing; such changes were further illustrated in details in the following analyses. Previous studies have determined the volatile profiles consisting of 51 compounds in varieties of dried tangerine peels by gas chromatography-mass spectrometry (GC-MS) analysis (10, 11). The components were predominantly terpenes such as D-limonene, γ-terpinene, α-pinene, and β-pinene. In addition, a recent report have detected a total of 58 volatile components in nine-processed tangerine peel during pickling and storage by gas chromatography–olfactometry-mass spectrometry (GC-O-MS) (7). Present findings were in line with the reported results and particularly, more flavor compounds were identified. These results supported the advantages of GC-IMS for trace gas analysis compared with GC-MS or GC-O-MS. GC-MS and GC-O-MS have drawbacks, as they require pre-treatments of the samples, such as solvent extraction, supercritical fluid extraction, water vapor distillation and solid phase microextraction (SPME) (12). These complicated treatments may affect the reflection of the true gaseous composition in its natural state. Besides, these techniques require a long detection interval, which is not conducive to the rapid analysis of volatile substances. Therefore, GC-IMS has been increasing applied for examining the volatile profile in foods, as for example, extra virgin olive oils (13), and Dezhou braised chicken (14). Recently, we have examined the impact of different drying methods on the volatile flavor components in nine-processed tangerine peel by GC-IMS, revealing that those prepared by baking has a richer aroma than those prepared by sun-drying (15). Currently, we did not determine the effects of drying methods but the effects of soaking, pickling, and aging to compare with the raw material. Similar but more volatile flavor components were detected in this study. Of note, compounds labeled with number 1–18 were unidentified in the tangerine peel samples, which should be studied in the future.
During the whole process of nine-processed tangerine peel production, flavor substances detected by GC-IMS consist of alcohols, aldehydes, carboxylic acids, esters, ketones, terpenes, and other compounds. The Gallery Plot analysis as fingerprinting technique was applied to provide overall and intuitive analysis of the composition changes in flavor substances during the processing (16). We selected the signal peaks of volatile substances in the spectrum of each sample to form the fingerprint spectrum (Figure 3A). Each row in the figure represents all signal peaks in one sample, and each column represents the same volatile substances in different samples. According to the whole spectrum analysis, the composition of volatile substances was different during the production stages of nine-processed tangerine peel. Furthermore, the peak intensities of volatile substances categorized by types for the four stages were summarized in Figure 3B.
Figure 3. Changes of volatile substances during processing of nine-processed tangerine peel. (A) Fingerprint spectrum of volatile compounds in four groups of samples. (B) Summarized data showing peak intensities of different types of volatile substances in different process stages.
Firstly, the concentrations of favor substances including limonene, gamma-terpinene, alpha-pinene, myrcene, beta-pinene, alpha-thujene, acetophenone, diethyl malonate, and heptanal marked in the red frame were the lowest in the final products. These substances are mainly terpenes, indicating that terpenes will lose during the processing of nine-processed tangerine peel and the loss mainly happened at the later stage of production. Terpenes are important aroma and flavor compounds with characteristic odors, flavors, and colors. In accordance with the previous reports, D-limonene, γ-terpinene, α-pinene, β-myrcene, β-pinene, and α-thujene were abundant in citrus peel (17, 18). Microorganims may play crucial roles in transformation of terpenes to other compounds (19).
A number of enzymatic reactions and metabolic pathways for transformation of terpenes have been identified. For instance, pinene with isomers α-pinene and β-pinene is the most abundant bicyclic monoterpene. In Pseudomonas rhodesiae and P. fluorescens, α-pinene can be oxidized to α-pinene oxide by a NADH-dependent α-pinene oxygenase and also form isonovalal or novalal by α-pinene oxide lyase (20). Alternatively, α-pinene can be transformed into limonene and pinocarveol but β-pinene can only form pinocarveol in Bacillus pallidus. Limonene is the most abundant monocyclic monoterpene, which transforms into limonene-1,2-diol through limonene-1,2-epoxide by limonene-1,2 monooxygenase and limonene-1,2-epoxide hydrolase in Rhodococcus erythropolis DCL14. Besides, limonene can be hydroxylated by a NADPH-dependent limonene 6-monooxygenase to trans-carveol, which is oxidized to carvone and dihydrocarvone by carveol dehydrogenase and carvone reductase respectively. The field of the microbial transformation of terpenes is not fully understood and remains to be explored.
Secondly, methyl acetate, furfuryl acetate, ethyl acetate, benzyl propionate, 2-hexanol, linalool, isopulegol, and decanal shown in the yellow frame mainly were present in the raw material CP1 samples. They are mostly esters and alcohols, suggesting that a large number of esters and alcohols in the tangerine peel will reduce rapidly at the early stage of processing.
Next, tangerine peel CP2 samples in the second processing stage after debittering contained a great number of volatile substances with dynamic composition. The contents of (E, E)-2,4-non-adienal, butanal, p-methylbenzaldehyde, isobutyl propanoate, ethyl butanoate, 2-butanol, borneol, 2-methyl-1-propanol, 3-methyl-2-butanol, cis-3-hexenol, 2-butanone, 2-pentanone, 2-heptanone, methyl isobutyl ketone, mesityl oxide, 2-methylbutyric acid, alpha-terpinene, and alpha-phellandrene marked in the green frame were more abundant in the second stage than other production stages. Among these flavor substances, p-methylbenzaldehyde, ethyl butanoate, mesityl oxide, propylsulfide, 2-heptanone, 3-methyl-2-butanol, cis-3-hexeno were only found in the samples of the second stage. On the other hand, substances in the purple frame consisting 2,3-butanedione, furfural, benzothiazole, n-non-anal, and terpinolene showed an opposite trend, being absent in the second stage.
Lastly, the contents of pentanal, hexanal, 2-hexenal, 2-heptenal (E), 2-pentenal (E), 1-penten-3-one, and 6-methyl-5-hepten-2-one detected were higher in samples of the last two production stages. Furthermore, volatile substances such as acetic acid, 2-methyl-2-propenal, methyl 2-furoate, 2-cyclohexen-1-one gradually increased and reached the highest concentration during the processing of nine-processed tangerine peel. Aldehydes may be formed from lipid oxidation and Maillard reactions, which are also known as non-enzymatic browning reactions in foods, resulting in browned food with characteristic flavor (21). Aldehydes are widely considered to be the critical contributors to the overall flavor among all categories due to the relatively low odor thresholds. For instance, hexanal can be derived from linoleic acid and exhibits a low odor threshold of 1.1 ng/L, affecting rice aroma (22).
To sum up, the final product of nine-processed tangerine peel contained reduced amount of terpenes, esters and alcohols, which were lost during processing; whereas the final product contained increased amount of aldehydes and very high level of acetic acid. The preserved food contained abundant terpenes and acids, which are particularly important contributors for the unique odors, flavors, and colors.
Principal component analysis (PCA) is a classification method that can effectively highlight the similarities or differences among datasets, commonly applied in chemometrics and bioinformatics (23, 24). Converting the original variables into clusters based on chemical properties, the PCA result of the volatile substances in different stages was shown in Figure 4A. All domains for four different stages were clearly separated among one another.
Figure 4. Similarity analysis of volatile substances in different process stages. (A) Score plot of principal component analysis (PCA) based on the GC-IMS signal intensity in samples from different process stages of nine-processed tangerine peel. (B) Euclidean distance analysis.
Euclidean distance analysis apart from PCA was applied further for similarity analysis and the results were shown in Figure 4B and Table 2. Euclidean distance is defined as the distance between two points or between the point and the origin (25). Euclidean distances of all samples were measured, indicating that the compositions of volatile substances between processing stages were significant different. The results of both PCA and Euclidean distances analysis indicated that there were apparent differences in the volatile substance profiles among four processing stages, and hence the processing stages can be distinguished according to the composition of volatile substances.
The present study determined the complexity of volatile compounds at key processing stages throughout the manufacturing of nine-processed tangerine peel. A total of 110 flavor compounds were successfully detected by HG-GC-IMS in tangerine peel samples across the various production stages. The distinctive flavor of nine-processed tangerine peel is attributed to the unique set of volatile compounds. The limitations of the present study are that we did not perform quantitative analysis for the various volatile flavor components and did not determine the transformation pathways of the compounds happened during the manufacturing processes, which should be explored in the future.
The raw data supporting the conclusions of this article will be made available by the authors, without undue reservation.
MF, YY, and JinW performed the experiments and analyzed the data. YW, MC, and WC prepared the manuscript. MF and JijW revised the manuscript. All authors read and approved the final manuscript.
This work was supported by the National Key Research and Development Program of China (No. 2021YFD1600104), Chinese National Natural Science Foundation (No. 31901713), Natural Science Foundation of Guangdong Province (No. 2021A1515011049), Talent Project of Guangdong Academy of Agricultural Sciences (No. R2020PY-JX011), Research Group Construction Project of Guangdong Academy of Agricultural Sciences (No. 202109TD), and the Department of Agriculture and Rural Areas of Guangdong Province (No. 2020KJ110).
The authors declare that the research was conducted in the absence of any commercial or financial relationships that could be construed as a potential conflict of interest.
All claims expressed in this article are solely those of the authors and do not necessarily represent those of their affiliated organizations, or those of the publisher, the editors and the reviewers. Any product that may be evaluated in this article, or claim that may be made by its manufacturer, is not guaranteed or endorsed by the publisher.
2. Hamdan D, El-Readi MZ, Nibret E, Sporer F, Farrag N, El-Shazly A, et al. Chemical composition of the essential oils of two Citrus species and their biological activities. Pharmazie. (2010) 65:141–7. doi: 10.1691/ph.2010.9731
3. Phillips CA, Gkatzionis K, Laird K, Score J, Kant A, Fielder MD. Identification and quantification of the antimicrobial components of a citrus essential oil vapor. Nat Prod Commun. (2012) 7:103–7.
4. Wang SQ, Chen HT, Sun BG. Recent progress in food flavor analysis using gas chromatography-ion mobility spectrometry (GC-IMS). Food Chem. (2020) 315:126158. doi: 10.1016/j.foodchem.2019.126158
5. Makinen M, Nousiainen M, Sillanpaa M. Ion spectrometric detection technologies for ultra-traces of explosives: a review. Mass Spectr Rev. (2011) 30:940–73. doi: 10.1002/mas.20308
6. Ge S, Chen YY, Ding SH, Zhou H, Jiang LW, Yi YJ, et al. Changes in volatile flavor compounds of peppers during hot air drying process based on headspace-gas chromatography-ion mobility spectrometry (HS-GC-IMS). J Sci Food Agric. (2020) 100:3087–98. doi: 10.1002/jsfa.10341
7. Ma RT, Wu Y, Cao Y, Hu H, Li XL, He LP, et al. Changes in aroma components of jiuzhi tangerine peel pericarpium Citri Reticulatae during pickling and storage determined by gas chromatography-mass spectrometry/olfactory. Mod Food Sci Technol. (2020) 36:244–54.
8. Gerhardt N, Schwolow S, Rohn S, Perez-Cacho PR, Galan-Soldevilla H, Arce L, et al. Quality assessment of olive oils based on temperature-ramped HS-GC-IMS and sensory evaluation: comparison of different processing approaches by LDA, kNN, and SVM. Food Chem. (2019) 278:720–8. doi: 10.1016/j.foodchem.2018.11.095
9. Karpas Z. Applications of ion mobility spectrometry (IMS) in the field of foodomics. Food Res Int. (2013) 54:1146–51. doi: 10.1016/j.foodres.2012.11.029
10. Yi L, Dong N, Liu S, Yi Z, Zhang Y. Chemical features of pericarpium Citri reticulatae and pericarpium Citri reticulatae viride revealed by GC-MS metabolomics analysis. Food Chem. (2015) 186:192–9. doi: 10.1016/j.foodchem.2014.07.067
11. Zheng YY, Zeng X, Peng W, Wu Z, Su WW. Study on the discrimination between Citri reticulatae pericarpium varieties based on HS-SPME-GC-MS combined with multivariate statistical analyses. Molecules. (2018) 23:1235. doi: 10.3390/molecules23051235
12. Xu CH, Chen GS, Xiong ZH, Fan YX, Wang XC, Liu Y. Applications of solid-phase microextraction in food analysis. Trac Trends Anal Chem. (2016) 80:12–29. doi: 10.1016/j.trac.2016.02.022
13. Gerhardt N, Birkenmeier M, Sanders D, Rohn S, Weller P. Resolution-optimized headspace gas chromatography-ion mobility spectrometry (HS-GC-IMS) for non-targeted olive oil profiling. Anal Bioanal Chem. (2017) 409:3933–42. doi: 10.1007/s00216-017-0338-2
14. Yao WS, Cai YX, Liu DY, Chen Y, Li JR, Zhang MC, et al. Analysis of flavor formation during production of Dezhou braised chicken using headspace-gas chromatography-ion mobility spec-trometry (HS-GC-IMS). Food Chem. (2022) 370:130989. doi: 10.1016/j.foodchem.2021.130989
15. Chen SP, Liu HC, Yang WY, Yu YS, Fu MQ, Bu ZB, et al. Effect of different drying methods on the volatile flavpr pf nine-process dried tangerine peel. Guangdong Agric Sci. (2022) 1:142–50.
16. Yang LZ, Liu J, Wang XY, Wang RR, Ren F, Zhang Q, et al. Characterization of volatile component changes in jujube fruits during cold storage by using headspace-gas chromatography-ion mobility spectrometry. Molecules. (2019) 24:3904. doi: 10.3390/molecules24213904
17. Qin KM, Zheng LJ, Cai H, Cao G, Lou YJ, Lu TL, et al. Characterization of chemical composition of pericarpium Citri reticulatae volatile oil by comprehensive two-dimensional gas chromatography with high-resolution time-of-flight mass spectrometry. Evidence-Based Complement Alternat Med. (2013) 2013:237541. doi: 10.1155/2013/237541
18. Gioffre G, Ursino D, Labate MLC, Giuffre AM. The peel essential oil composition of bergamot fruit (Citrus bergamia, Risso) of Reggio Calabria (Italy): a review. Emir J Food Agric. (2020) 32:835–45. doi: 10.9755/ejfa.2020.v32.i11.2197
19. Marmulla R, Harder J. Microbial monoterpene transformations-a review. Front Microbiol. (2014) 5:346. doi: 10.3389/fmicb.2014.00346
20. Bicas JL, Fontanille P, Pastore GM, Larroche C. Characterization of monoterpene biotransformation in two pseudomonads. J Appl Microbiol. (2008) 105:1991–2001. doi: 10.1111/j.1365-2672.2008.03923.x
21. Lund MN, Ray CA. Control of maillard reactions in foods: strategies and chemical mechanisms. J Agric Food Chem. (2017) 65:4537–52. doi: 10.1021/acs.jafc.7b00882
22. Hu XQ, Lu L, Guo ZL, Zhu ZW. Volatile compounds, affecting factors and evaluation methods for rice aroma: a review. Trends Food Sci Technol. (2020) 97:136–46. doi: 10.1016/j.tifs.2020.01.003
23. Chatterjee S, Singh B, Diwan A, Lee ZR, Engelhard MH, Terry J, et al. A perspective on two chemometrics tools: PCA and MCR, and introduction of a new one: pattern recognition entropy (PRE), as applied to XPS and ToF-SIMS depth profiles of organic and inorganic materials. Appl Surf Sci. (2018) 433:994–1017. doi: 10.1016/j.apsusc.2017.09.210
24. Li MQ, Yang RW, Zhang H, Wang SL, Chen D, Lin SY. Development of a flavor fingerprint by HS-GC-IMS with PCA for volatile compounds of Tricholoma matsutake Singer. Food Chem. (2019) 290:32–9. doi: 10.1016/j.foodchem.2019.03.124
Keywords: nine-processed tangerine peel, terpenes, volatile components, GC-IMS, food processing
Citation: Fu M, Wang Y, Yu Y, Wen J, Cheong MS, Cheang WS and Wu J (2022) Changes of volatile substance composition during processing of nine-processed tangerine peel (Jiuzhi Chenpi) determined by gas chromatography-ion mobility spectrometry. Front. Nutr. 9:963655. doi: 10.3389/fnut.2022.963655
Received: 07 June 2022; Accepted: 05 August 2022;
Published: 24 August 2022.
Edited by:
Sibel Uluata, İnönü University, TurkeyCopyright © 2022 Fu, Wang, Yu, Wen, Cheong, Cheang and Wu. This is an open-access article distributed under the terms of the Creative Commons Attribution License (CC BY). The use, distribution or reproduction in other forums is permitted, provided the original author(s) and the copyright owner(s) are credited and that the original publication in this journal is cited, in accordance with accepted academic practice. No use, distribution or reproduction is permitted which does not comply with these terms.
*Correspondence: Jijun Wu, d3VqaWp1bkAxMjYuY29t; Wai San Cheang, YW5uYWNoZWFuZ0B1bS5lZHUubW8=
Disclaimer: All claims expressed in this article are solely those of the authors and do not necessarily represent those of their affiliated organizations, or those of the publisher, the editors and the reviewers. Any product that may be evaluated in this article or claim that may be made by its manufacturer is not guaranteed or endorsed by the publisher.
Research integrity at Frontiers
Learn more about the work of our research integrity team to safeguard the quality of each article we publish.