- 1Department of Biochemistry, Dr. Rammanohar Lohia Avadh University, Ayodhya, India
- 2Biotechnology Programme, Dr. Rammanohar Lohia Avadh University, Ayodhya, India
- 3Department of Biosciences, Integral University, Lucknow, India
- 4Department of Biotechnology, Guru Ghasidas Vishwavidyalaya, Bilaspur, India
- 5Department of Microbiology, King George Medical University, Lucknow, India
Nowadays, effective cancer therapy is a global concern, and recent advances in nanomedicine are crucial. Cancer is one of the major fatal diseases and a leading cause of death globally. Nanotechnology provides rapidly evolving delivery systems in science for treating diseases in a site-specific manner using natural bioactive compounds, which are gaining widespread attention. Nanotechnology combined with bioactives is a very appealing and relatively new area in cancer treatment. Natural bioactive compounds have the potential to be employed as a chemotherapeutic agent in the treatment of cancer, in addition to their nutritional benefits. Alginate, pullulan, cellulose, polylactic acid, chitosan, and other biopolymers have been effectively used in the delivery of therapeutics to a specific site. Because of their biodegradability, biopolymeric nanoparticles (BNPs) have received a lot of attention in the development of new anticancer drug delivery systems. Biopolymer-based nanoparticle systems can be made in a variety of ways. These systems have developed as a cost-effective and environmentally friendly solution to boost treatment efficacy. Effective drug delivery systems with improved availability, increased selectivity, and lower toxicity are needed. Recent research findings and current knowledge on the use of BNPs in the administration of bioactive chemicals in cancer therapy are summarized in this review.
Introduction
Non–communicable diseases, such as cancer, are one of the leading causes of death worldwide. The most common type of cancer is lung cancer, which is followed by breast, prostate, and colon cancer (1). Within the next two decades, the number of new cases is expected to increase by almost 70% (2). Present treatment options for cancer include surgery, chemotherapy, and/or radiation therapy. But there are some challenges associated with the above treatment strategies like radiation therapy or chemotherapeutic drugs not only damage cancerous cells, but also harm healthy cells (3). The degree of side effects may vary from person to person depending on the type of cancer, and other variables. Chemotherapy hinders the DNA synthesis in fast–dividing cancer cells, leading to their death. It leads to various side effects as it affects healthy cells of the body (4). Additionally, high drug doses used during chemotherapy lead to increased toxicity to normal cells, with the risk of development of drug resistance (4). Thus, a major task is to strengthen the selectivity of anticancer drugs against cancerous cells while sparing normal cells and tissues. In order to treat cancer, efficient targeted delivery methods of chemotherapeutic drugs or anticancer bioactive molecules are also required. In view of the above facts, a potential approach was used to target tumor cells through nano–medicine–based preparations (5). These preparations consist of ultra–micrometer–sized carriers having the bioactive compound(s) that are capable of selectively treating tumors, thus, improving the therapeutic value of the anticancer drugs that are being used.
For the past decade, nanoparticles (NPs) have become tremendously appealing for use in biomedicine. Through enhanced permeability and retention (EPR) effects in tumor tissues, nanoparticle-based drug delivery techniques or nanocarriers can improve therapeutic efficacy and selectivity (6). Nanocarriers also have superior cellular uptake as compared to standard chemotherapeutic molecules. Among the nanocarriers, polymeric NPs, micelles, and liposomes have received significant attention (7).
The polymers utilized to prepare polymeric NPs such as poly (methyl methacrylate), polystyrene, polyacrylates, and polyacrylamide were not eco–friendly (8). The nanosystems prepared by these materials displayed chronic toxicity and inflammatory reactions. The use of new nanosystems might have huge interest to accomplish additional applications for the current large–scale characterized bioactive compounds. Nanoencapsulation, in actuality, is a low-cost technology that could allow for regulated bioactives release, which could be useful in cancer treatment. There are synthetic and natural biodegradable polymers. Polymeric nanoparticles have been created using naturally occurring biodegradable polymers, such as alginate, gelatin, chitosan, and others (9). Some researchers studied about targeted delivery systems of therapeutics through chitosan and other biopolymer based NPs for cancer therapy (10, 11). Therefore, this review focuses on recent progresses in the development of BNPs for the effective delivery of bioactive chemicals for targeted therapy of cancer.
Bioactive compounds
Bioactive substances are a natural occurrence as well as a part of our diet. Plants produce phytochemicals, which are natural bioactives capable of regulating metabolic processes and promoting good health (12). Amino acids, vitamins, enzymes, fibers, minerals, essential oils, and polyphenols are among the plant-based bioactive compounds (13). Natural biopolymers also consist of plant–derived or animal polysaccharides and proteins as well as polymers of microbial (bacteria, fungi, and yeast) sources. Several bioactive substances have the potential to be used as cancer chemotherapeutic drugs in past few years. Phytochemicals like vinca alkaloids, cephalotaxus, berberine, combretastatins, colchicine, ellipticine, and others are known for anticancerous activities against many cancer types (14). A flavonoid, chicoric acid isolated from Echinacea purpurea act as an immune stimulant by increasing the number of natural killer cells (15). A sulfur–containing compound called Ajoene isolated from Allium sativum suppresses the rate of cancer development. In a study, Shang et al. (16) reported the role of ajoene in the suppression of the growth of human breast cancer cells. Likewise, curcumin, an active chemical derived from the Curcuma longa plant, has anticancer properties. It caused the transcription factors AP1, NFkB, and STAT3 to be inhibited, halting the cell cycle in the G2/M phase (17). Similarly, another anticancer agent like Lappaol F has been obtained from Arctium lappa L. and has arrested the G2 phase of the cell cycle by promoting the G1 phase in which the p21 gene plays an important role for the same Lappaol F (18).
Citral is a bioactive ingredient found in citrus–based plant Cymbopogon citratus. Nanostructured lipid carrier–citral showed effective results against MDA–MB−231 breast cancer cells (19). Several researchers reported promising bioactive components from Cannabis sativa like delta 9-tetrahydrocannabinol (THC) and cannabinoids (CBD) which have also been investigated for their potential to treat cancer in both in vivo and in vitro studies (20–22).
A compound wedelolactone, isolated from Eclipta alba involve in the inhibition of T47D, MCF−7, and MDAMB−231 cells by exciting ER signaling (23). Curcumin is another important bioactive obtained from Curcuma longa which contains curcuminoids compounds (24). Curcumin is known to have low bioavailability because of its poor absorption and fast removal from the body. Basniwal et al. (25) have used NPs mediated delivery of curcumin to increase its bioavailability. Curcumin has been reported as a potent anticancer agent on various types of human cancers such as lung, pancreatic, prostate, breast, ovarian and others (26). Another bioactive myricetin is a bioflavonoid commonly present in food sources such as berries, red wine, vegetables, tea, and medicinal plants which is a promising anti–carcinogen with therapeutic potential reported in the breast (27), colon (28), liver (29), ovarian (30), and skin cancers (31). Geraniin is a dehydroellagitannin that is found in a variety of medicinal plants and is thought to be the most active natural chemical. Geraniin was discovered from the fruit of the Phyllanthus emblica L. plant, and found to have potent antioxidant, antimicrobial, and anticancer properties (32, 33). On MCF7 human breast cancer cells, geraniin was found to have an anticancer impact (34).
The two major classes of fat soluble antioxidants in Vitamin E are tocopherols and tocotrienols (T3) (35) that showed anticancerous properties against skin (36), stomach (37), liver (38), breast (39), colon (40), lung (41), and prostate cancer (42). Anticancer activity has been documented for a number of biologically active substances (Table 1).
Development of biopolymeric nanomaterials
Biopolymer composites integrate the necessary qualities of two or more acceptable materials to improve the physiological and mechanical needs of biomedical regions. Because of their long-term stability, chemical substances utilized in standard processes to produce polymeric nanomaterials may cause environmental impact. Biopolymers, on the other hand, are frequently created from non-toxic monomers and are carbon-neutral. It is easier to make nanomaterials from biopolymers since they are deposited at the nanoscale. Biopolymers have been found in plants, algae, fungi, bacteria, and animals. Macromolecules include starch, alginate, chitosan, dextran, and chitin (Figure 1). There are several compounds like pectin, cellulose, lignin, and hyaluronic acid, that are used to create nanomaterials composites. In the medical field, such as in the treatment of cancer, nano biocomposites have shown encouraging outcomes in recent years such as biodegradable substances, and chitosan nanoparticles are employed to deliver anticancer drugs (58). Biopolymers are made up of diverse components and have varied physiological properties. Biopolymeric nanomaterials can be made by attaching metals to biopolymers. To construct molecular capsules, biopolymers, in particular, utilize intramolecular hydrogen bonding. Starch, for example, can be used to create polymeric nanocomposites by combining metals or metal oxides. Chitosan is also used in nanotechnology applications because of its extensive compatibility (59). To make nanomaterials, silver (Ag) can be supramolecularly incorporated into starch (60). Nanomaterials can be incorporated into biopolymers by impregnation or absorption. Physical features of NPs can differ from macro-sized bulk materials due to their greater surface area and reactivity. Nanocapsules or nanospheres can be manufactured depending on the manufacturing approach (61). Spectroscopy, microscopy, and others are among the techniques used for NPs characterization.
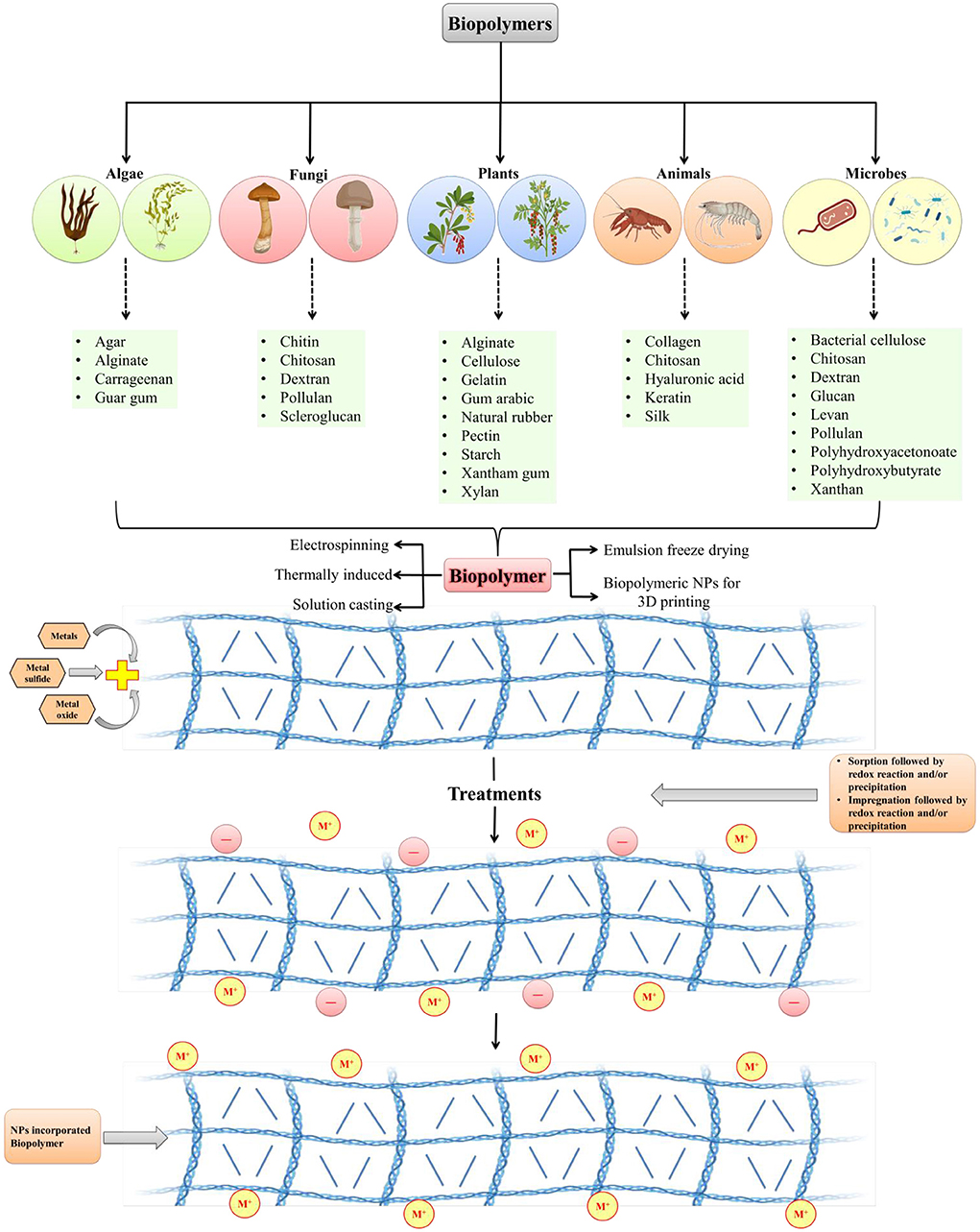
Figure 1. Development of biopolymeric nanoparticles from various sources (modified and adapted from Sarkar et al. (57).
Biopolymers and their derivatives are widely utilized, and studied in the creation of hydrogels. Chemical and physical methods of producing biomaterial-based hydrogels can be distinguished. The chemical process of incorporating substituent groups into biopolymer structures, strengthening and developing new functional bioactivities as a result. Covalent bonds between the utilized polymers are created via free radical polymerization, graft copolymerization, and other polymerization techniques (6, 62, 63). The chemical reaction within the hydrogel creates everlasting bonds in the polymer system. However, reversible physical interactions such as van der Waals attraction, hydrogen bonding, polymer chain entanglements, ionic interactions, and crystallite associations hold the hydrogels together during the physical process (64). There are several nanomaterials based biopolymers employed for effective delivery of bioactives for cancer therapy (Table 2).
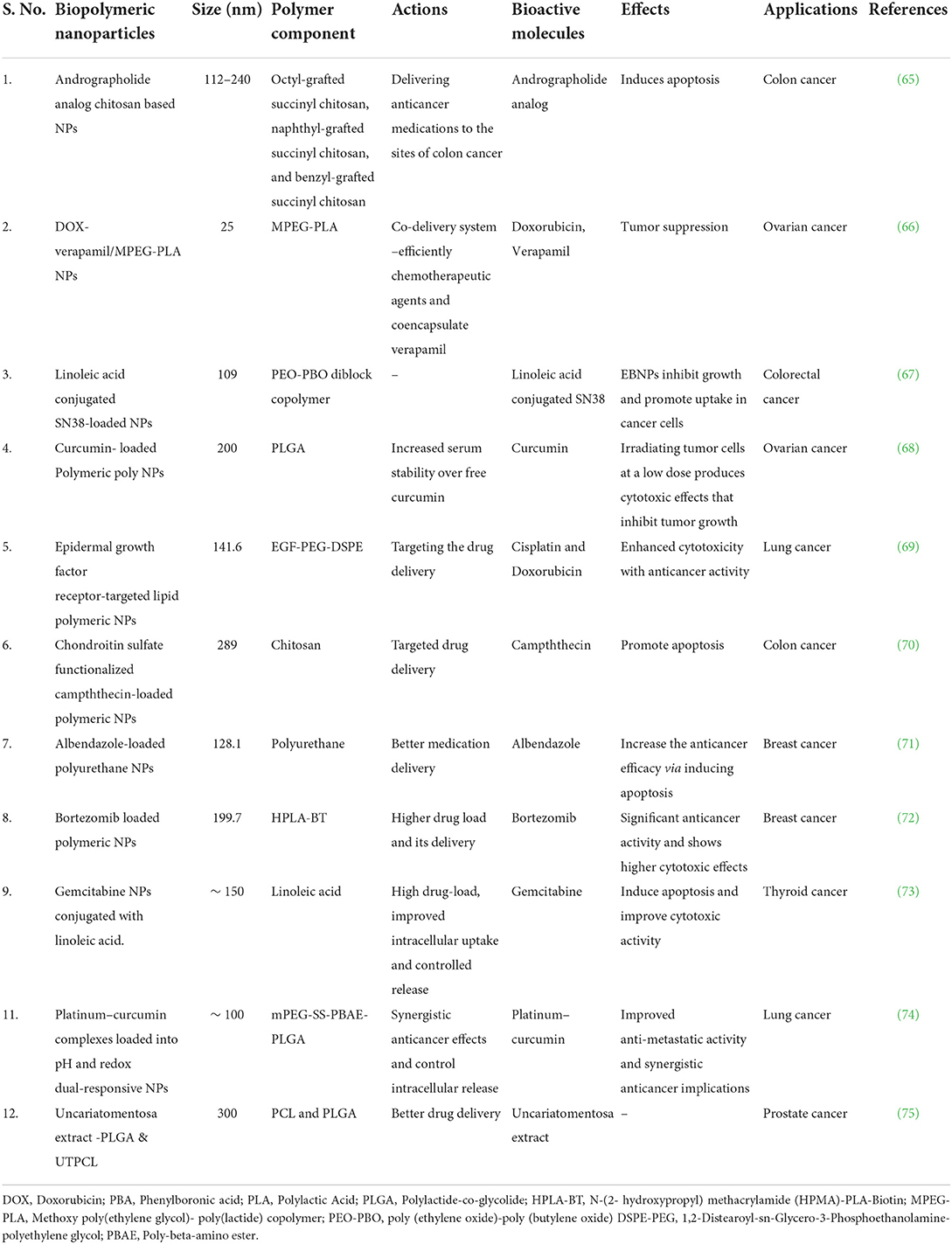
Table 2. Nanoparticles based numerous biopolymeric compounds and their action in various cancer treatments.
Targeted delivery of bioactives via biopolymeric nanoparticles for cancer therapy
According to the World Health Organization, cancer is one of the leading causes of mortality, with more than twenty million people expected to die by the year 2025 (1). Chemotherapeutic medicines work by interfering with DNA synthesis in rapidly dividing cancer cells, slowed DNA replication, reducing therapeutic efficacy and generating a significant mortality rate in cancer patients due to their large doses (4). Bioactive molecule sources and development, as well as their involvement in drug delivery systems and molecular mechanisms against different cancer cells, are illustrated in (Figures 2A–E). Natural bioactive chemicals have an impact on cellular metabolism and signaling pathways, and they can be used to cure cancer (Figure 2C). Natural bioactive chemicals have a favorable effect on cancer treatment, according to results from both in vivo and in vitro trials (10). A study reported in cancer reports showed that resveratrol NPs has enhanced anticancer activity both in vitro and in vivo (77). Biopolymers as NPs are increasingly being employed as a different way to deliver bioactive compounds/drugs or biological macromolecules to specific body regions. Polymer nanoparticles are a novel way of medication delivery. As a result, the primary goal of biopolymer-based nanomedicine is to deliver therapeutics just to cancer cells, increasing efficacy while reducing toxicity. Nanoparticles containing natural bioactives for targeted medication delivery in various tissues enhanced bioavailability, reduced side effects, improved in vivo stability, and improved target– specific activity of bioactive chemicals in cancer treatment. In the year 2020, Mousa et al. (78) reported that nanoformulation of 3, 3′-diindolylmethane (DIM) and ellagic acid showed more effective suppression of cell viability in pancreatic cancer, tumor weight, and tumor angiogenesis as compared to with these bioactive compounds alone. New formulation strategies on lipid–based delivery systems as nanocarriers also showed improved bio–efficacy under in vivo conditions (79).
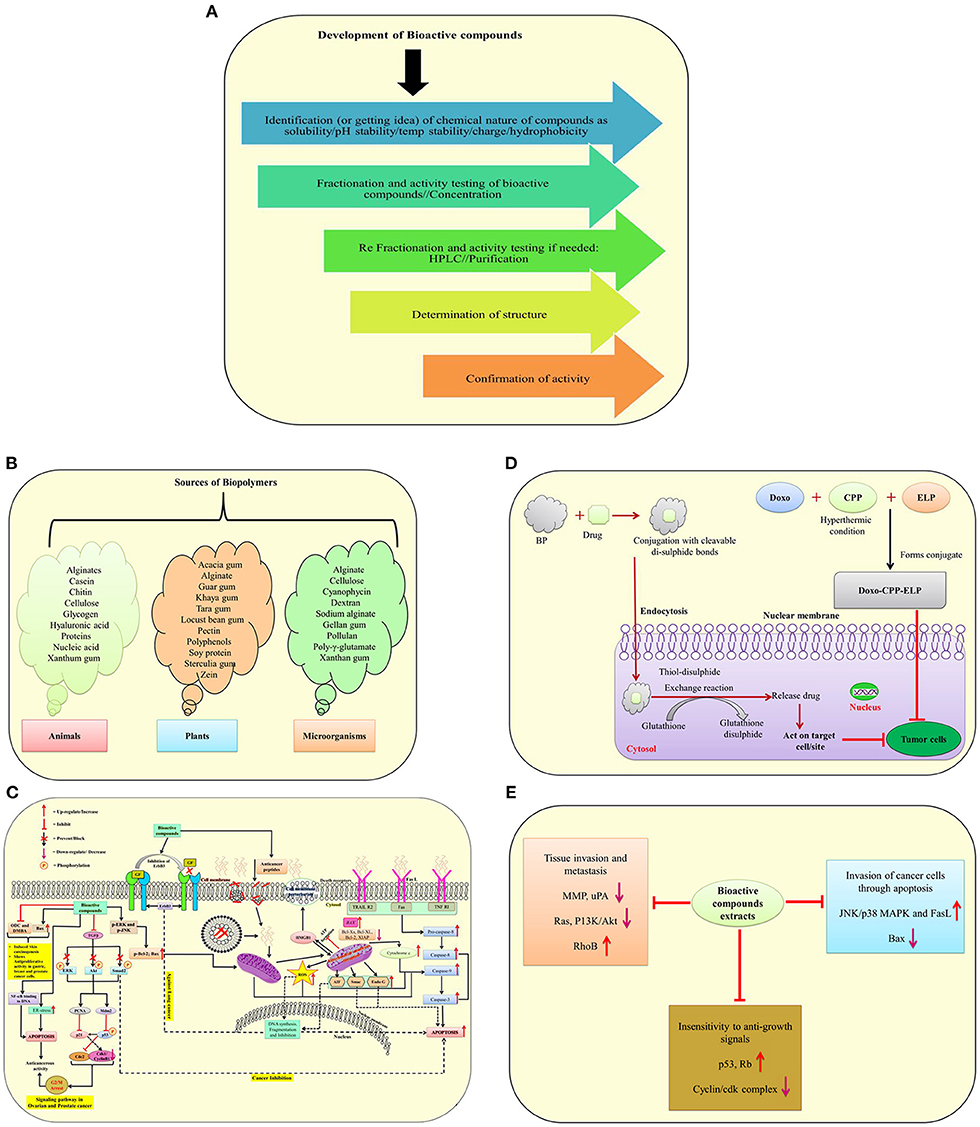
Figure 2. A schematic illustration of bioactive molecule sources and development, as well as their involvement in drug delivery systems and molecular mechanisms against different cancer cells. (A) Development of bioactive compounds; (B) Sources of biopolymers from plants, animals and microorganisms; (C) Mode of action of anticancerous molecules via TGF–β signaling pathway, death receptor, mitochondrial receptor–induced pathways against cancer cells; (D) A mechanism of suppressing tumor cells using biopolymers in conjunction with drugs; (E) The effect of bioactive molecules from plant extracts on the underlying mechanisms of cancer development [modified and adapted from Lin et al. (76)]. ODC, Ornithine decarboxylase; DMBA, 7,12-Dimethylbenz(α)anthracene; Bcl-2, B-cell lymphoma 2; Bax, Bcl-2-associated X protein; NF-Kb, Transcription factor; p-ERK, Protein kinase RNA-like ER kinase; ROS, Reactive oxygen species; ENK, c-Jun-N terminal kinase; AIF, Apoptosis-inducing factor; FasL, Fas Ligand; ErbB3, Receptor tyrosine kinase; TGF-β, Transforming growth factor beta; ERK, Extracellular-signal-regulated kinase; AKT1, Serine/threonine-protein kinase; SMAD2, SMAD family member 2; Mdm2, Mouse double minute 2 homolog; PCNA, Proliferating cell nuclear antigen; p53 and p21, Tumor protein; WAF1/CIP1, Wildtype activating factor-1/cyclin-dependent kinase inhibitory protein-1; cdc2, Cell division cycle 2; HMGB1, High mobility group box 1; Smac, Second mitochondria-derived activator of caspase; EndoG, Endonuclease G; ER, Endoplasmic reticulum; TRAIL, Tumor necrosis factor (TNF)-related apoptosis-inducing ligand; TNF RI, Tumor necrosis factor receptor 1; Rb, Retinoblastoma protein; PI3K, Phosphatidylinositol-3-kinase; uPA, Urokinase plasminogen activator; BP, Biopolymers.
Biopolymer-based nanocarriers, liposomes, dendrimers, lipid-based NPs, polymeric micelles, and other new drug delivery technologies have been discovered in the last two decades that allow bioactive chemicals to be delivered into the site of action (80). Proteins (albumin, gelatin, and milk proteins), polysaccharides (cellulose, starch, pectin, chitosan, dextran and sodium alginate), and nucleic acids are examples of biopolymers (81). Biopolymer NPs were found to be effective in delivering bioactive chemicals to malignant cells in both in vivo and in vitro investigations. Biopolymeric nanoparticles containing bioactive substances have several advantages over other current delivery technologies, including non water–soluble drug delivery, stability protection, and targeted medication delivery to specific tumor cells (82). Nanoparticles deliver the therapeutic chemical, which could be natural bioactive or synthetic, directly into the target tissue/organ. Nanoparticles have a lot of potential as molecular transporters since they can deliver treatments to malignant cells without harming healthy cells (83). Polymeric pharmaceuticals, polymeric micelles, polyplexes, polymer–drug conjugates, and polymer–protein conjugates are all examples of biopolymers utilized in therapy (84).
The ways NPs are administered, as well as their physicochemical properties, have a big impact on therapeutic efficacy and biological consequences (Figure 2C). Every mode of administration has advantages and disadvantages, but the oral route of delivery is the safest and most convenient way to treat cancer. The oral mode of administration, on the other hand, has drawbacks such as gastrointestinal degradation, insolubility, and reduced absorption. As a result, current anticancer compositions aren't suited for use as oral chemotherapy. The use of biopolymers for oral delivery platforms has the potential to overcome some of the problems associated with the delivery of chemotherapy drugs. Biopolymeric formulations prevent NPs from pH degradation, enhance water solubility and stability, and target particular binding sites for selective absorption and drug release (Figure 2D) (85). Future formulations from diverse plants with anticancer properties could significantly boost the potency of anticancer medications for cancer therapy (9). The potency of recognized naturally occurring bioactive chemicals has been increased through the use of nanomaterials. In the future, herbal compounds might play a bigger role in the delivery mechanism for nano biocomposites mediated therapeutic molecules. Further research is required in this area.
Challenges and future perspectives
Every day, there are large number of patients who have advance tumors and most common concern regarding the therapy is the effectiveness and the expense of cancer medication. The effectiveness and cost of pharmaceuticals have not altered dramatically as a result of the development of new technology and drugs. Not only is the efficacy of new treatments a source of concern in India; but wealthier countries such as the United States and Europe are also experiencing issues with cancer drug delivery systems. Nanotechnology is one of the most exciting fields in the area of drug delivery systems to treat non–curable cancer disorders, especially in such a crucial situation. Nanoparticles are utilized to target and deliver drugs to cancer/tumor cells while preserving the physiology of non-tumorous cells. Researchers are working to make more consistent NPs so that the right amount of harmless therapeutics can be delivered to the right place. Metal–based (gold and silver) NPs production has progressed significantly for their applications in diagnostic and therapeutic purposes. The understanding of treatment mechanism at the molecular level will expand bioactive compounds applications for cancer therapy. Because of the regulated release of specific medications at targeted places, assessment of events during drug interaction at the tissue/cellular level, and prediction has not yet been found completely, and its application in cancer therapy/diagnosis is still limited. Animal research will provide important information on the possible use of nanoformulations as medicinal therapeutics. Acute and chronic studies are required to assess the possible harm of any type of anticancerous therapeutic molecule to humans and the environment, and their affordability is another area of research that requires further input.
Conclusions
Drugs have numerous obstacles, thus researchers are focusing on herbal medicine to reduce drug side effects and improve their effectiveness through the use of appropriate effective delivery systems. Preclinical and in vitro investigations have revealed that NPs are particularly effective at delivering chemotherapeutic medicines, molecules as well as natural components to specific tumor sites. Nanoparticles have significant advantages over alternative drug delivery systems in terms of high specificity, high stability, controlled drug release, and high drug–carrying capacity, and the ability to transport both hydrophobic and hydrophilic drug molecules. Because of their controlled release, subcellular size, and biocompatibility with cells, polymeric NPs are now widely used as drug/anticancer bioactives delivery systems in the pharmaceutical and medical areas for cancer therapy.
Author contributions
NP: conceptualization. PS, PKS, and AG: writing—original draft, reviewing and editing. SS, RS, AG, RM, and VM: reviewing, editing, and drawing figures and tables. MT and NP: reviewing, editing and finalize the manuscript. All authors have read and agreed to the published version of the manuscript.
Acknowledgments
The authors are grateful to the Biotechnology Program, Department of Biochemistry, Dr. Rammanohar Lohia Avadh University, Ayodhya, India, and Department of Bioscience, Integral University, Lucknow, India for providing us with the research environment.
Conflict of interest
The authors declare that the research was conducted in the absence of any commercial or financial relationships that could be construed as a potential conflict of interest.
Publisher's note
All claims expressed in this article are solely those of the authors and do not necessarily represent those of their affiliated organizations, or those of the publisher, the editors and the reviewers. Any product that may be evaluated in this article, or claim that may be made by its manufacturer, is not guaranteed or endorsed by the publisher.
References
1. Bray F, Ferlay J, Soerjomataram L, Siegel RL, Torre LA, Jemal A. Global cancer statistics 2018: GLOBACAN estimates of incidence and mortality worldwide for 36 cancers in 185 countries. CA Cancer J Clin. (2018) 68:394–424. doi: 10.3322/caac.21492
2. Siegel RL, Miller KD, Fedewa SA, Ahnen DJ, Meester RGS, Barzi A, et al. Colorectal cancer statistics. CA Cancer J Clin. (2017) 67:177–93. doi: 10.3322/caac.21395
3. Debela DT, Muzazu SG, Heraro KD, Ndalama MT, Mesele BW, Haile DC, Kitui SK, Manyazewal T. New approaches and procedures for cancer treatment: current perspectives. SAGE Open Med. (2021) 9:20503121211034366. doi: 10.1177/20503121211034366
4. D'Souza CA, Antony S, Thomas B, Murthy SG. Coping strategies used by cancer patients to deal with physical and psychological problems of chemotherapy. Int J Innov Res Dev. (2016) 5:36–41.
5. Oerlemans C, Bult W, Bos M, Storm G, Nijsen JFW, Hennink WE. Polymeric micelles in anticancer therapy: targeting, imaging and triggered release. Pharm Res (N Y). (2010) 27:2569–89. doi: 10.1007/s11095-010-0233-4
6. Zhao Y, Sun Q, Zhang X, Baeyens J, Su H. Self-assembled selenium nanoparticles and their application in the rapid diagnostic detection of small cell lung cancer biomarkers. Soft Matter. (2018) 14:481–9. doi: 10.1039/C7SM01687E
7. Conte C, Mastrotto F, Taresco V, Tchoryk A, Quaglia F, Stolnik S, et al. Enhanced uptake in 2D- and 3D- lung cancer cell models of redox responsive PEGylated nanoparticles with sensitivity to reducing extra and intracellular environments. J Control Release. (2018) 277:126–41. doi: 10.1016/j.jconrel.2018.03.011
8. Gagliardi A, Giuliano E, Venkateswararao E, Fresta M, Bulotta S, Awasthi V, et al. Biodegradable polymeric nanoparticles for drug delivery to solid tumors. Front Pharm. (2021) 12:601626. doi: 10.3389/fphar.2021.601626
9. Gagliardi A, Paolino D, Iannone M, Palma E, Fresta M, Cosco D. Sodium deoxycholate-decorated zein nanoparticles for a stable colloidal drug delivery system. Int J Nanomed. (2018) 13:601–14. doi: 10.2147/IJN.S156930
10. Chavda VP, Patel AB, Mistry KJ, Suthar SF, Wu ZX, Chen ZS, et al. Nano-drug delivery systems entrapping natural bioactive compounds for cancer: recent progress and future challenges. Front Oncol. (2022) 12:867655. doi: 10.3389/fonc.2022.867655
11. Sharifi-Rad J, Quispe C, Butnariu M, Rotariu LS, Sytar O, Sestito S, et al. Chitosan nanoparticles as a promising tool in nanomedicine with particular emphasis on oncological treatment. Cancer Cell Int. (2021) 21:318. doi: 10.1186/s12935-021-02025-4
12. Watkins R, Wu L, Zhang C, Davis RM, Xu B. Natural product-based nanomedicine: recent advances and issues. Int J Nanomedicine. (2015) 10:6055–74. doi: 10.2147/IJN.S92162
13. Durazzo A, Lucarini M, Souto EB, Cicala C, Caiazzo E, Izzo AA, et al. Polyphenols: a concise overview on the chemistry, occurrence, and human health. Phyther Res. (2019) 13:2221–3. doi: 10.1002/ptr.6419
14. Bai LY, Chiu CF, Chu PC, Lin WY, Chiu SJ, Weng JR. A triterpenoid from wild bitter gourd inhibits breast cancer cells. Sci Rep. (2016) 6:22419. doi: 10.1038/srep22419
15. Kooti W, Servatyari K, Behzadifar M, Samani MA, Sadeghi F, Nouri B, et al. Effective medicinal plant in cancer treatment, Part 2: review study. J Evid-Based Complementary Altern Med. (2017) 22:982–95. doi: 10.1177/2156587217696927
16. Shang A, Cao SY, Xu XY, Gan RY, Tang GY, Corke H, et al. Bioactive compounds nad biological functions of Garlic (Allium sativum L). Foods. (2019) 8:246. doi: 10.3390/foods8070246
17. Lou C, Zhu Z, Zhao Y, Zhu R, Zhao H. Arctigenin, a liganan from Arctium lappa L, inhibits metastasis of human breast cancer cells through the downregulation of MMP-2/-9 and heparanase in MDA-MB-231 cells. Oncol Rep. (2016) 37:179–84. doi: 10.3892/or.2016.5269
18. Sun Q, Liu K, Shen X, Jin W, Jiang L, Sheikh SM, et al. A novel anticancer agent isolated from plant Arctium Lappa L. Mol Cancer Ther. (2014) 13:49–59. doi: 10.1158/1535-7163.MCT-13-0552
19. Nordin N, Yeap SK, Rahman HS, Zamberi NR, Abu N, Mohamad NE, et al. In vitro cytotoxicity and anticancer effects of citral nanostructured lipid carrier on MDA MBA-231 human breast cancer cell. Sci Rep. (2019) 9:1614. doi: 10.1038/s41598-018-38214-x
20. Caffarel MM, Andradas C, Mira E, Pérez-Gómez E, Cerutti C, Moreno-Bueno G, et al. Cannabinoids reduce ErbB2-driven breast cancer progression through Akt inhibition. Mol Cancer. (2010) 9:196. doi: 10.1186/1476-4598-9-196
21. Dariš B, Tancer VM, Knez Ž, Ferk P. Cannabinoids in cancer treatment: therapeutic potential and legislation. Bosn J Basic Med Sci. (2019) 19:14–23. doi: 10.17305/bjbms.2018.3532
22. Mangal N, Erridge S, Habib N, Sadanandam A, Reebye V, Sodergren MH. Cannabinoids in the landscape of cancer. J Cancer Res Clin Oncol. (2021) 147:2507–4. doi: 10.1007/s00432-021-03710-7
23. Xu D, Hua LT, Ren YC, Cheng MA, Min CL, Chang C, et al. The Wedelolactone derivative inhibits estrogen receptor-mediated breast, endometrial, and ovarian cancer cells growth. BioMed Res Int. (2014) 2014:713263. doi: 10.1155/2014/713263
24. Jurenka JS. Anti-inflammatory properties of curcumin, a major constituent of Curcuma longa: a review of preclinical and clinical research. Altern Med Rev. (2009) 14:277.
25. Basniwal RK, Buttar HS, Jain VK, Jain N. Curcumin nanoparticles: preparation, characterization, and antimicrobial study. J Agric Food Chem. (2011) 59:2056–61. doi: 10.1021/jf104402t
26. Patra S, Pradhan B, Nayak R, Behera C, Panda KC, Das S, et al. Apoptosis and autophagy modulating dietary phytochemicals in cancer therapeutics: current evidences and future perspectives. Phytother Res. (2021) 35:4194-214. doi: 10.1002/ptr.7082
27. Jayakumar JK, Nirmala P, Kumar BAP, Kumar AP. Evaluation of protective effect of myricetin, a bioflavonoid in dimethyl benzanthracene-induced breast cancer in female Wistar rats. South Asian J Cancer. (2014) 3:107. doi: 10.4103/2278-330X.130443
28. Kim ME, Ha TK, Yoon JH, Lee JS. Myricetin induces cell death of human colon cancer cells via BAX/BCL2-dependent pathway. Anticancer Res. (2014) 34:701–6.
29. Sangwan V, Banerjee S, Jensen K, Chen Z, Chugh R, Dudeja V. Primary and liver metastasis-derived cell lines from KrasG12D; Trp53R172H; Pdx-1 Cre animals undergo apoptosis in response to triptolide. Pancreas. (2015) 44:583doi: 10.1097/MPA.0000000000000317
30. Xu Y, Xie Q, Wu S, Yi D, Yu Y, Liu S. Myricetin induces apoptosis via endoplasmic reticulum stress and DNA double-strand breaks in human ovarian cancer cells. Mol Med Rep. (2016) 13:2094–100. doi: 10.3892/mmr.2016.4763
31. Cijo GV, Vijayakumaran VV, Inoka MAD. ALC, Anbarasu K, Ragupathi NKD. Mechanism of action of flavonoids in prevention of inflammation-associated skin cancer. Curr Med Chem. (2016) 23:3697–716. doi: 10.2174/0929867323666160627110342
32. Palanisamy UD, Ling LT, Manaharan T, Appleton D. Rapid isolation of geraniin from Nephelium lappaceum rind waste and its anti-hyperglycemic activity. Food Chem. (2011) 127:21–7. doi: 10.1016/j.foodchem.2010.12.070
33. Ren Z, Zou W, Cui J, Liu L, Qing Y, Li Y. Geraniin suppresses tumor cell growth and triggers apoptosis in human glioma via inhibition of STAT3 signaling. Cytotechnology. (2017) 69:765–73. doi: 10.1007/s10616-017-0085-4
34. Liu X, Zhao M, Wu K, Chai X, Yu H, Tao Z. Immunomodulatory and anticancer activities of phenolics from emblica fruit (Phyllanthus emblica L). Food Chem. (2012) 131:685–90. doi: 10.1016/j.foodchem.2011.09.063
35. Traber MG, Packer L. Vitamin E: beyond antioxidant function. Am J Clin Nutr. (1995) 62:1501S−9S. doi: 10.1093/ajcn/62.6.1501S
36. Korac RR, Khambholja KM. Potential of herbs in skin protection from ultraviolet radiation. Pharmacogn Rev. (2011) 5:164. doi: 10.4103/0973-7847.91114
37. Manu KA, Shanmugam MK, Ramachandran L, Li F, Fong CW, Kumar AP et al. First evidence that γ-tocotrienol inhibits the growth of human gastric cancer and chemosensitizes it to capecitabine in a xenograft mouse model through the modulation of NF-κB pathway- Tocotrienol Enhances the Effect of Capecitabine in Gastric Cancer. Clin Cancer Res. (2012) 18:2220–9. doi: 10.1158/1078-0432.CCR-11-2470
38. Chang C, Lee SO, Yeh S, Chang TM. Androgen receptor (AR) differential roles in hormone-related tumors including prostate, bladder, kidney, lung, breast and liver. Oncogene. (2014) 33:3225–34. doi: 10.1038/onc.2013.274
39. Tran AT, Ramalinga M, Kedir H, Clarke R, Kumar D. Autophagy inhibitor 3-methyladenine potentiates apoptosis induced by dietary tocotrienols in breast cancer cells. Eur J Nutr. (2015) 54:265–72. doi: 10.1007/s00394-014-0707-y
40. Zhang JS, Zhang SJ Li Q, Liu YH, He N, Zhang J, et al. Tocotrienol-rich fraction (TRF) suppresses the growth of human colon cancer xenografts in Balb/C nude mice by the Wnt pathway. PLoS ONE. (2015) 10:e0122175. doi: 10.1371/journal.pone.0122175
41. Rajasinghe L, Pindiprolu R, Razalli N, Wu Y, Gupta S. Delta tocotrienol inhibits MMP-9 dependent invasion and metastasis of non-small cell lung cancer (NSCLC) cell by suppressing Notch-1 mediated NF-_b and uPA pathways. FASEB J. (2015) 29:718–52. doi: 10.1096/fasebj.29.1_supplement.752.18
42. Huang Y, Wu R, Su ZY, Guo Y, Zheng X, Yang CS, et al. A naturally occurring mixture of tocotrienols inhibits the growth of human prostate tumor, associated with epigenetic modifications of cyclin-dependent kinase inhibitors p21 and p27. J Nutr Biochem. (2017) 40:155–63. doi: 10.1016/j.jnutbio.2016.10.019
43. Guo JS, Wang SX, Li X, Zhu TR. Studies on the antibacterial constituents of Geranium sibiricum L. Acta Pharm Sin. (1987) 22:28–32.
44. Smith CD, Zhang X, Mooberry SL, Patterson GM, Moore RE. Cryptophycin: a new antimicrotubule agent active against drug-resistant cells. Cancer Res. (1994) 54:3779–84.
45. Dirsch VM, Gerbes AL, Vollmar AM. Ajoene, a compound of garlic, induces apoptosis in human promyeloleukemic cells, accompanied by generation of reactive oxygen species and activation of nuclear factor kappaB. Mol Pharmacol. (1998) 53:402–07. doi: 10.1124/mol.53.3.402
46. Luesch H, Yoshida WY, Moore RE, Paul VJ, Corbett TH. Total structure determination of apratoxin A, a potent novel cytotoxin from the marine cyanobacterium Lyngbya majuscula. J Am Chem Soc. (2001) 123:54185423. doi: 10.1021/ja010453j
47. Chen HM, Wu YC, Chia YC, Chang FR, Hsu HK, Hsieh YC, et al. Gallic acid, a major component of Toona sinensis leaf extracts, contains a ROS-mediated anti-cancer activity in human prostate cancer cells. Cancer Lett. (2009) 286:161–71. doi: 10.1016/j.canlet.2009.05.040
48. Wang HM, Pan JL, Chen CY, Chiu CC, Yang MH, Chang HW, et al. Identification of anti-lung cancer extract from Chlorella vulgaris C-C by antioxidant property using supercritical carbon dioxide extraction. Process Biochem. (2010) 45:1865–72. doi: 10.1016/j.procbio.2010.05.023
49. Kounnis V, Chondrogiannis G, Mantzaris MD, Tzakos AG, Fokas D, Papanikolaou NA, et al. Microcystin LR shows cytotoxic activity against pancreatic cancer cells expressing the membrane OATP1B1 and OATP1B3 transporters. Anticancer Res. (2015) 35:5857–65.
50. Nazir T, Taha N, Islam A, Abraham S, Mahmood A, Mustafa M. Monocytopenia; Induction by vinorelbine, cisplatin and doxorubicin in breast, non-small cell lung and cervix cancer patients. Int J Health Sci. (2016) 10:542–7. doi: 10.12816/0048904
51. Sanna V, Singh CK, Jashari R, Adhami VM, Chamcheu JC, Rady I, et al. Targeted nanoparticles encapsulating epigallocatechin-3-gallate for prostate cancer prevention and therapy. Sci Rep. (2017) 7:1–15. doi: 10.1038/srep41573
52. Chou GL, Peng SF, Liao CL, Ho HC, Lu KW, Lien JC, et al. Casticin impairs cell growth and induces cell apoptosis via cell cycle arrest in human oral cancer SCC-4 cells. Environ Toxicol. (2018) 33:127–41. doi: 10.1002/tox.22497
53. Sharma A, Ghani A, Sak K, Tuli HS, Sharma AK, Setzer WN, et al. Probing into therapeutic anti-cancer potential of apigenin: recent trends and future directions. Recent Pat Inflamm Allergy Drug Discov. (2019) 13:124–33. doi: 10.2174/1872213X13666190816160240
54. Jang CH, Moon N, Oh J, Kim JS. Luteolin shifts Oxaliplatin-induced cell cycle arrest at G0/G1 to apoptosis in HCT116 human colorectal carcinoma cells. Nutrients. (2019) 11:770. doi: 10.3390/nu11040770
55. Cox-Georgian D, Ramadoss N, Dona C, Basu C. Therapeutic and medicinal uses of terpenes. Med Plants. (2019) 12:333–59. doi: 10.1007/978-3-030-31269-5_15
56. Farkhondeh T, Folgado SL, Pourbagher-Shahri AM, Ashrafizadeh M, Samarghandian S. The therapeutic effect of resveratrol: focusing on the Nrf2 signaling pathway. Biomed Pharmacother. (2020) 127:110234. doi: 10.1016/j.biopha.2020.110234
57. Sarkar S, Ponce NT, Banerjee A, Bandopadhyay R, Rajendran S, Lichtfouse E. Green polymeric nanomaterials for the photocatalytic degradation of dyes: a review. Env Chem Lett. (2020) 18:1569–80. doi: 10.1007/s10311-020-01021-w
58. Kanaoujiya R, Saroj SK, Srivastava S, Chaudhary MK. Renewable polysaccharide and biomedical application of nanomaterials. J Nanomat. (2022) 2022:1–16. doi: 10.1155/2022/1050211
59. Morin-Crini N, Lichtfouse E, Torri G, Crini G. Applications of chitosan in food, pharmaceuticals, medicine, cosmetics, agriculture, textiles, pulp and paper, biotechnology, and environmental chemistry. Environ Chem Lett. (2019) 17:1667–1692. doi: 10.1007/s10311-019-00904-x
60. Raveendran P, Fu J, Wallen SL. Completely “green” synthesis and stabilization of metal nanoparticles. J Am Chem Soc. (2003) 125:13940–41. 267j. doi: 10.1021/ja029267j
61. Sharma M. Transdermal and intravenous nano drug delivery systems: present and future. In: Applications of Targeted Nano Drugs and Delivery Systems. Amsterdam: Elsevier (2019). p. 499–550.
62. Bao Y, Ma J, Li N. Synthesis and swelling behaviors of sodium carboxymethyl cellulose-g-poly (AA-co-AM-co-AMPS)/MMT superabsorbent hydrogel. Carbohydr Polym. (2011) 84:76–82. doi: 10.1016/j.carbpol.2010.10.061
63. Rather RA, Bhat MA, Shalla AH. An insight into synthetic and physiological aspects of superabsorbent hydrogels based on carbohydrate type polymers for various applications: a review. Carbohydr Polym Technol Appl. (2022) 3:100202. doi: 10.1016/j.carpta.2022.100202
64. Li Z, Lin Z. Recent advances in polysaccharide-based hydrogels for synthesis and applications. Aggregate. (2021) 2:e21. doi: 10.1002/agt2.21
65. Kansom T, Sajomsang W, Saeeng R, Charoensuksai P, Opanasopit P, Tonglairoum P. Apoptosis induction and antimigratory activity of andrographolide analog (3a1)-incorporated self-assembled nanoparticles in cancer cells. AAPS Pharm Sci Tech. (2018) 19:3123–33. doi: 10.1208/s12249-018-1139-4
66. Zheng W, Li M, Lin Y, Zhan X. Encapsulation of verapamil and doxorubicin by MPEG-PLA to reverse drug resistance in ovarian cancer. Biomed Pharmacother. (2018) 108:565–73. doi: 10.1016/j.biopha.2018.09.039
67. Cheng G, Zhang X, Chen Y, Lee RJ, Wang J, Yao J, et al. Anticancer activity of polymeric nanoparticles containing linoleic acid-SN38 (LA-SN38) conjugate in a murine model of colorectal cancer. Colloids Surf B. (2019) 181:822–9. doi: 10.1016/j.colsurfb.2019.06.020
68. Duse L, Agel MR, Pinnapireddy SR, Schäfer J, Selo MA, Ehrhardt C, et al. Photodynamic therapy of ovarian carcinoma cells with curcumin-loaded biodegradable polymeric nanoparticles. Pharmaceutics. (2019) 11:282. doi: 10.3390/pharmaceutics11060282
69. Nan Y. Lung carcinoma therapy using epidermal growth factor receptor-targeted lipid polymeric nanoparticles co-loaded with cisplatin and doxorubicin. Oncol Rep. (2019) 42:2087–96. doi: 10.3892/or.2019.7323
70. Zu M, Ma L, Zhang X, Xie D, Kang Y, Xiao B. Chondroitin sulfate-functionalized polymeric nanoparticles for colon cancer-targeted chemotherapy. Colloids Surf B. (2019) 177:399–406. doi: 10.1016/j.colsurfb.2019.02.031
71. Racoviceanu R, Trandafirescu C, Voicu M, Ghiulai R, Borcan F, Dehelean C, et al. Solid polymeric nanoparticles of albendazole: synthesis, physico-chemical characterization and biological activity. Molecules. (2020) 25:5130. doi: 10.3390/molecules25215130
72. Rani S, Sahoo RK, Nakhate KT, Ajazuddin Gupta U. Biotinylated HPMA centered polymeric nanoparticles for Bortezomib delivery. Int J Pharm. (2020) 579:119173. doi: 10.1016/j.ijpharm.2020.119173
73. Liu C, Han Q, Liu H, Zhu C, Gui W, Yang X, et al. Precise engineering of Gemcitabine prodrug cocktails into single polymeric nanoparticles delivery for metastatic thyroid cancer cells. Drug Deliv. (2020) 27:1063–72. doi: 10.1080/10717544.2020.1790693
74. Chen Y, Chen C, Zhang X, He C, Zhao P, Li M, et al. Platinum complexes of curcumin delivered by dual-responsive polymeric nanoparticles improve chemotherapeutic efficacy based on the enhanced anti-metastasis activity and reduce side effects. Acta Pharm Sin B. (2020) 10:1106–21. doi: 10.1016/j.apsb.2019.10.011
75. Ribeiro AF, Santos JF, Mattos RR, Barros EGO, Nasciutti LE, Cabral LM, et al. Characterization and in vitro antitumor activity of polymeric nanoparticles loaded with uncariatomentosa extract. An Acad Bras Cienc. (2020) 92:1–16. doi: 10.1590/0001-3765202020190336
76. Lin H-H, Chen J-H, Wang C-J. Chemopreventive properties and molecular mechanisms of the bioactive compounds in Hibiscus sabdariffa Linne. Curr Med Chem. (2011) 18:1245–54. doi: 10.2174/092986711795029663
77. Annaji M, Poudel I, Boddu SHS, Arnold RD, Tiwari AK, Babu RJ. Resveratrol-loaded nanomedicines for cancer applications. Cancer Rep. (2021) 4:e1353. doi: 10.1002/cnr2.1353
78. Mousa DS, El-Far AH, Saddiq AA, Sudha T, Mousa SA. Nanoformulated bioactive compounds derived from different natural products combat pancreatic cancer cell proliferation. Int J Nanomed. (2020) 15:2259–68. doi: 10.2147/IJN.S238256
79. Mouhid L, Corzo-Martínez M, Torres C, Vázquez L, Reglero G, Fornari T, et al. Improving in vivo efficacy of bioactive molecules: an overview of potentially antitumor phytochemicals and currently available lipid-based delivery systems. J Oncol. (2017) 2017: 7351976. doi: 10.1155/2017/7351976
80. Yun YH, Lee BK, Park K. Controlled drug delivery: historical perspective for the next generation. J Control Release. (2015) 219:2–7. doi: 10.1016/j.jconrel.2015.10.005
81. Vasile C, Pamfil D, Stoleru E, Baican M. New developments in medical applications of hybrid hydrogels containing natural polymers. Molecules. (2020) 25:1539. doi: 10.3390/molecules25071539
82. Calzoni E, Cesaretti A, Polchi A, Michele AD, Tancini B, Emiliani C. Biocompatible polymer nanoparticles for drug delivery applications in cancer and neurodegenerative disorder therapies. J Funct Biomater. (2019) 10:1–15. doi: 10.3390/jfb10010004
83. Honey PJ, Rijo J, Anju A, Anoop KR. Smart polymers for the controlled delivery of drugs—A concise overview. Acta Pharm Sin B. (2014) 4:120–27. doi: 10.1016/j.apsb.2014.02.005
84. Duncan R. The dawning era of polymer therapeutics. Nat Rev Drug Discov. (2003) 2:347–60. doi: 10.1038/nrd1088
Keywords: biopolymeric nanoparticles, cancer treatment, drug delivery, nanomedicines, natural bioactives
Citation: Pathak N, Singh P, Singh PK, Sharma S, Singh RP, Gupta A, Mishra R, Mishra VK and Tripathi M (2022) Biopolymeric nanoparticles based effective delivery of bioactive compounds toward the sustainable development of anticancerous therapeutics. Front. Nutr. 9:963413. doi: 10.3389/fnut.2022.963413
Received: 07 June 2022; Accepted: 27 June 2022;
Published: 15 July 2022.
Edited by:
Kandi Sridhar, Agrocampus Ouest, FranceReviewed by:
Baskaran Stephen Inbaraj, Fu Jen Catholic University, TaiwanNavin Kumar, Agricultural Research Organization (ARO), Israel
Prateeksha, TTUHSC, United States
Copyright © 2022 Pathak, Singh, Singh, Sharma, Singh, Gupta, Mishra, Mishra and Tripathi. This is an open-access article distributed under the terms of the Creative Commons Attribution License (CC BY). The use, distribution or reproduction in other forums is permitted, provided the original author(s) and the copyright owner(s) are credited and that the original publication in this journal is cited, in accordance with accepted academic practice. No use, distribution or reproduction is permitted which does not comply with these terms.
*Correspondence: Manikant Tripathi, manikant.microbio@gmail.com
†These authors have contributed equally to this work and share first authorship
‡ORCID: Neelam Pathak https://orcid.org/0000-0003-2412-4756
Anmol Gupta https://orcid.org/0000-0003-4406-1684