- 1College of Pharmaceutical Sciences, Zhejiang University of Technology, Hangzhou, China
- 2Institute of Animal Husbandry and Veterinary Medicine, Zhejiang Academy of Agricultural Sciences, Hangzhou, China
- 3Xianju Breeding Chicken Farm, Taizhou, China
Fulvic acid (FA) is a mixture of polyphenolic acid compounds extracted from humus, peat, lignite, and aquatic environments; it is used in traditional medicine to treat digestive tract diseases. The purpose of the present study was to investigate the effect of FA on growth performance, inflammation, intestinal microbiota, and metabolites in Xianju yellow chicken. The 240 Xianju yellow chickens (age, 524 days) included were randomly categorized into 4 treatments with 6 replicates per treatment and 10 birds per replicate. Birds received a basal diet or a diet supplemented with 500, 1,000, or 1,500 mg/kg of FA, for a period of 42 days. Dietary supplementation of FA improved average daily gain (ADG) and feed conversion ratio (FCR) (P > 0.05). Compared with the control group, the serum level of TNF-α in birds supplemented with FA was significantly decreased (P < 0.05), and that of IL-2 was significantly increased after administration of 1,500 mg/kg FA (P < 0.05). Analysis of gut microbiota indicated that FA reduced the relative abundance of genus Mucispirillum, Anaerofustis, and Campylobacter, but enriched genus Lachnoclostridium, Subdoligranulum, Sphaerochaeta, Oscillibacter, and Catenibacillus among others. Untargeted metabolomic analyses revealed that FA increased 7-sulfocholic acid, but reduced the levels of Taurochenodeoxycholate-7-sulfate, LysoPC 20:4 (8Z, 11Z, 14Z, 17Z), LysoPC 18:2, Phosphocholine and other 13 metabolites in the cecum. The results demonstrated that FA may potentially have a significant positive effect on the growth performance and immune function of Xianju yellow chicken through the modulation of the gut microbiota.
Introduction
Using antibiotics in livestock and poultry farming could efficiently reduce disease frequency, improve growth performance, and enhance feeding efficacy. However, overuse of antibiotics in livestock and poultry promotes the development of antibiotic resistance, which has garnered considerable attention. Currently, several countries have banned the use of antibiotics as growth promoters in poultry farming. The general tendency of the reduction in the use of antibiotics in the breeding industry has created a need for alternative strategies. Chinese herbs, which have beneficial nutritional and medicinal properties, can potentially be used as feed additives to improve the performance of animal growth owing to their strong antimicrobial activity and absence of residues.
Fulvic acid (FA) is a mixture of polyphenolic acid compounds extracted from humus, peat, lignite, and aquatic environments; it is characterized by its small molecular weight and the abundance of biologically active molecules (1, 2). FA, confirmed as the main active component of Wujinshi, was considered an effective treatment for ulcerative carbuncle in the Compendium of Materia Medica. Modern pharmacological studies have revealed that FA has multiple effects, including antioxidant (3), anti-inflammatory (4), immunomodulatory (5), and antidiabetic (6). In recent years, studies have demonstrated that adding FA to the feed or drinking water could enhance the growth rate, improve feed conversion ratio, and increase immunity (7, 8). However, using FA as an alternative antimicrobial feed additive in animal production remains in its infancy.
Xianju yellow chicken is one of the local breeds in the Zhejiang province, China. This breed of chicken is characterized by its superior meat quality, high egg production, and strong adaptability. In the poultry industry, high stocking density and intensive feeding have resulted in a decline in immunity, decrease in productivity, and low feed conversion ratio. FA has been used as a traditional Chinese medicine owing to its immune regulatory activity. However, the effects of dietary supplementation with FA require thorough examination in terms of the poultry industry. Therefore, the purpose of this study was to investigate the suitable supplemental amount of FA in poultry feed. Furthermore, the influence of FA on the gut microbiota and potential mechanisms underlying the regulation of productive performance through FA supplementation in poultry farming has been explored.
Materials and Methods
The experimental procedures for this study were approved by the Ethics Committee of the Zhejiang Academy of Agricultural Sciences and the Ministry of Science and Technology of the People's Republic of China.
Animals, housing, and experimental treatments
A total of 240 (age, 524 days; sex, female) Xianju yellow chickens of similar egg production rates and weight (1.83 ± 0.33 g) were provided by Xianju Breeding Chicken Farm, Zhejiang province, China. All chickens were randomly divided into 4 groups with 6 replicates each, and each replicate included 10 birds. The chickens in the Control group were fed a basal diet, and the other 3 groups were, respectively, fed the basal diet supplemented with 500 mg/kg (FA-L), 1,000 mg/kg (FA-M), and 1,500 mg/kg FA (FA-H) for 42 days. The FA provided by Slona Biological Technology Co., Ltd (the content of FA was 19.28%, Supplementary Table 1). The basal diet had been formulated according to the nutritional requirements of yellow chickens, which has been presented in Table 1. All chickens were raised in three-story cages (two chickens per cage) under the recommended environmental conditions and were provided access to feed and water ad libitum. Feed consumption per cage was recorded every 10 days. The chickens were weighed at the end of the experiment. Egg number were recorded daily. Average daily gain (ADG), average daily feed intake (ADFI), feed conversion ratio (FCR), laying rates, and feed/egg were determined for the overall rearing period.
Sampling and measurements
At the end of the experiment (the 42nd day), two chickens were randomly selected from each replicate, individually weighed, and exsanguinated. Blood samples were collected from the jugular vein of each bird without the use of anticoagulants. One chicken was used to measure and calculate the slaughter performance. Furthermore, the lengths of the duodenum, jejunum, ileum, and ceca were measured. Digesta from the ceca of another chicken were mixed and divided into 2 subsamples; these subsamples were then placed in liquid nitrogen and stored at −80°C for analyses of gut microbiota and untargeted metabolomics.
Slaughter performance and meat quality traits determination
According to the poultry production performance noun terms and metric statistics method (NY/T823-2020), the live weight before slaughter, dressed weight, half-eviscerated weight with giblet, eviscerated weight, leg muscle weight, breast muscle weight, and abdominal fat weight were measured and the dressed percentage, percentage of half-eviscerated weight with giblet, percentage of eviscerated yield, percentage of leg muscle yield, percentage of breast muscle yield and percentage of abdominal fat yield were calculated. The formulae for calculating the abovementioned parameters have been described below.
After weighing, breast muscles were measured pH at 45 min in triplicate with a PH-STAR pH meter (Matthaus, German). And meat color was measured using a colorimeter (CE-410, Konica Minolta Sensing, Inc., Osaka, Japan).
Serum cytokines measurement
The collected blood samples, which were allowed to stand for 30 min to coagulate, were centrifuged at 3,500 rpm for 10 min at 4°C to obtain the serum. Serum levels of the cytokines such as IL-2, IL-6, IL-10, IFN-α, and TNF-α were measured using the ELISA kit (Shanghai Hepeng Biological Co., Ltd, China) according to the manufacturer's protocol.
Serum liver function indexes
The serum liver function indexes, including alanine aminotransferase (ALT), aspartate aminotransferase (AST), and total protein (TP) were measured using an automatic biochemical analyzer (Hitachi 7020, Tokyo, Japan).
Gut microbiota analysis
DNA from ceca digesta samples of the Control and FA-H group were extracted using the E.Z.N.A. ®Stool DNA Kit (D4015, Omega, Inc., USA) according to the manufacturer's instructions. The total DNA was eluted in 50 μL of elution buffer and stored at −80°C until measurement in the PCR by Majobio Bio-pharm Technology Co., Ltd (Shanghai, China). The V3–V4 regions of 16S rDNA were amplified using 341F (5′-CCTACGGGNGGCWGCAG-3′) and 806R (5′- GGACTACHVGGGTWTCTAAT-3′) (9). PCR amplification was performed in a total volume of 25 μL reaction mixture containing 25 ng of template DNA, 12.5 μL PCR Premix, 2.5 μL of each primer, and PCR-grade water to adjust the volume. The PCR reaction procedure was as follows: predenaturation at 98°C for 30 s; denaturation at 98°C for 10 s, annealing at 54°C for 30 s, extension at 72°C for 45 s, with 32 cycles; and then final extension at 72°C for 10 min.
The PCR products were confirmed with 2% agarose gel electrophoresis, purified by AMPure XT beads (Beckman Coulter Genomics, Danvers, MA, USA), and quantified through Qubit (Invitrogen, USA). The amplicon pools were prepared for sequencing; furthermore, the size and quantity of the amplicon library were measured using Agilent 2100 Bioanalyzer (Agilent, USA) and with the Library Quantification Kit for Illumina (Kapa Biosciences, Woburn, MA, USA), respectively. The libraries were sequenced on the Illumina NovaSeq PE250 platform. Paired-end reads were assigned to the relevant samples on the basis of their unique barcode and truncated by cutting off the barcode and primer sequence and merged using FLASH (v1.2.11). To obtain the high-quality clean tags based on Fastp (v0.19.6), the raw reads were filtered for quality using specific filtering conditions. Chimeric sequences were removed using the Vsearch software (v2.3.4). After dereplication using DADA2, we obtained the feature table and sequence. Subsequently, feature abundance was normalized using the relative abundance of each sample according to SILVA (v138) classifier. Alpha and Beta diversity were calculated using Mothur (v1.30.2) and QIIME (v1.9.1). Additionally, the graphs were prepared using the R package. Blast was used for sequence alignment, and the feature sequences were annotated with the SILVA database for each representative sequence. Furthermore, a linear discriminant analysis (LDA) effect size (LEfSe) analysis was performed to compare species with significant intergroup differences (10). Functional profiles of microbial communities were predicted using PICRUSt2 (v2.2.0) (11).
Metabolomic analysis
The sample preparation, metabolite identification and quantification, and primary quality control (QC) were performed at Majobio Bio-pharm Technology Co., Ltd (Shanghai, China) as described previously. After Ultra-high performance liquid chromatography–tandem mass spectrometry (HPLC-MS) analyses, the Progenesis QI 2.3 (Nonlinear Dynamics, Waters, USA) was used for peak detection and alignment of raw data. Subsequently, a data matrix was generated, which included retention time (RT), mass-to-charge ratio (m/z) values, and peak intensity. Metabolic features detected a minimum of 80% in any set of samples were retained. After filtering, quality control, normalization, and imputation, all data were log-transformed before statistical analysis. The metabolites were identified based on accurate mass, MS/MS fragments spectra, and isotope ratio difference in the human metabolome database (HMDB) (http://www.hmdb.ca/) and Metlin database (https://metlin.scripps.edu/).
The following composition analysis was implemented using the Majorbio cloud platform (https://cloud.majorbio.com). All multivariate statistical analyses, including principal component analysis (PCA) and partial least squares, discriminate analysis (PLS-DA), were performed using the “ropls” package for R software. Differentially expressed metabolites were identified using a combination of Variable importance in the projection (VIP) values of the PLS-DA model and Student's t-test. Metabolites with VIP > 2 and P < 0.05 indicated the presence of significant intergroup differences. The Kyoto Encyclopedia of Genes and Genomes (KEGG) pathway annotation and enrichment analysis were performed utilizing the KEGG pathway database (http://www.genome.jp/kegg). To test the co-linearity between metabolites, variance inflation factors (VIF) were used. The metabolites with VIF > 10 were removed before the redundancy analysis (RDA) analysis. The RDA and envfit analyses were performed using the vegan package in R software.
Statistical analysis
Statistical analyses were performed with SPSS 22.0. The continuous data were presented as means + SEM or medians and interquartile ranges (IQRs). The student's t-test or one-way analysis of variance was used for the normally distributed data. P < 0.05 indicated statistical significance.
Results
Effects of FA on growth and slaughter performance
Compared with the control group, the ADG and FCR in the FA-H group increased and decreased by 21.32 and 14.46%, respectively; however, no significant differences (P > 0.05) were observed (Table 2). Furthermore, the four groups showed no intergroup differences (P > 0.05) in terms of ADFI, laying rate and feed/egg ratio.

Table 2. Effects of dietary supplementation of FA on growth performance of Xianju yellow chickens (Mean ± SD).
As shown in Table 3, the general carcass characteristics such as dressed percentage, percentage of half-eviscerated yield with giblet, percentage of eviscerated yield, percentage of breast muscle yield, and percentage of leg muscle yield of chickens were similar regardless of supplement with varying concentration of FA (P > 0.05). However, the percentage of abdominal fat yields were different among FA supplement groups; FA-H vs. FA-L and FA-M (P < 0.05). In addition, the four groups showed no significant intergroup differences in terms of the length of the duodenum, jejunum, ileum, and ceca (P > 0.05) (Table 4). Breast muscle color and pH at 45 min after slaughter were no significant differences among the treatments (P > 0.05) (Table 5).
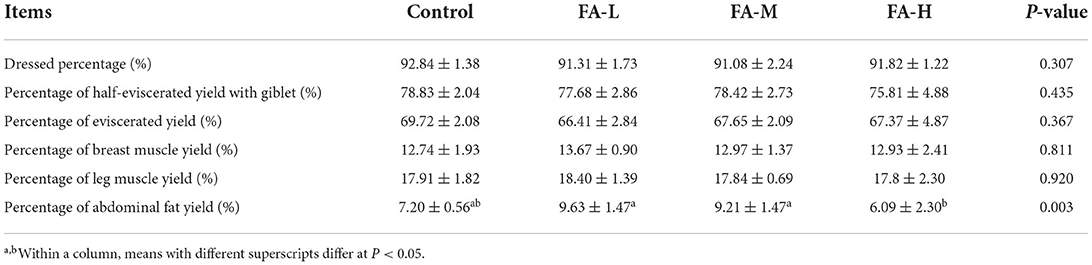
Table 3. Effects of dietary supplementation of FA on slaughter performance of Xianju yellow chickens (Mean ± SD).

Table 4. Effects of dietary supplementation of FA on intestine length of Xianju yellow chickens (Mean ± SD).
FA supplement in Xianju yellow chicken alters IL-2 and TNF-α, without influencing liver function indexes
In serum, the level of IL-2 in the FA-H group was significantly higher than in the control and FA-M group (P < 0.05) (Figure 1A). Moreover, compared with the control group, supplementation of FA significantly decreased the serum levels of TNF-α (P < 0.05) (Figure 1D). Compared with the control, the levels of IL-6, IL-10, and IFN-α were relatively lower in chickens supplemented with FA (Figures 1B,C,E); however, the differences were not significant (P > 0.05).
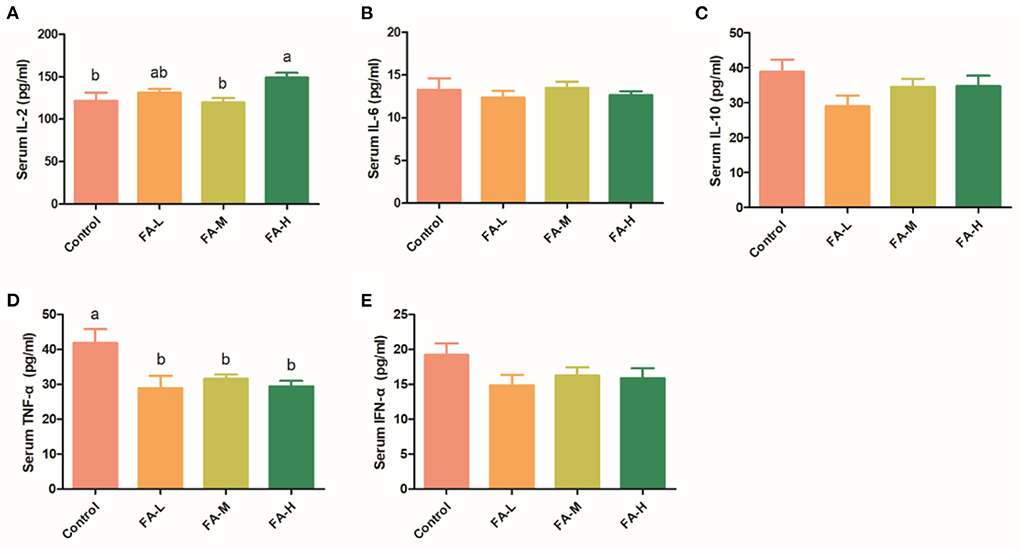
Figure 1. Effect of FA on serum concentration of IL-2 (A), IL-6 (B), IL-10 (C), TNF-α (D), and IFN-α (E). Data with different superscript letters are significantly different (P < 0.05).
The liver function indexes were assayed to further evaluate the effects of FA in poultry. There was no significant difference in AST, ALT, and TP (Figure 2).
FA supplement did not change Alpha diversity in the gut microbiota
For the control and FA-H samples, 1.198 million high-quality sequences (mean length, 419) were generated, representing 4,334 bacterial operational taxonomic units (OTUs) at 97% sequence similarity. As estimated by the Alpha diversity, no significant differences (P > 0.05) were observed in terms of the diversity, richness, and evenness between the control and FA-H groups (Supplementary Table 2).
Difference in gut bacterial community compositions between control and FA-H groups
Regarding the β-diversity, PCA and Hierarchical Clustering Analysis revealed a powerful effect of FA. The first and second principal components of PCA clarified 42.59 and 17.7% of the total variation, respectively (Figure 3A). Additionally, some degree of clustering in FA-H samples was observed in the hierarchical clustering (Figure 3B). The LEfSe analysis was used to identify significant differences in microbiota between the control and FA-H groups from phylum to genus levels. At the phylum level, the two major bacterial groups identified in the FA-H group were Verrucomicrobiota and WPS-2, whereas Deferribacterota was highly represented in the control group (Figure 3C). There were 16 relatively high abundance genera in the FA-H group, including Lachnoclostridium, Subdoligranulum, Sphaerochaeta, Oscillibacter, and Catenibacillus, among others. Additionally, Mucispirillum, Anaerofustis, and Campylobacter were abundant in the control group (LDA > 2, P < 0.05) (Figure 3E).
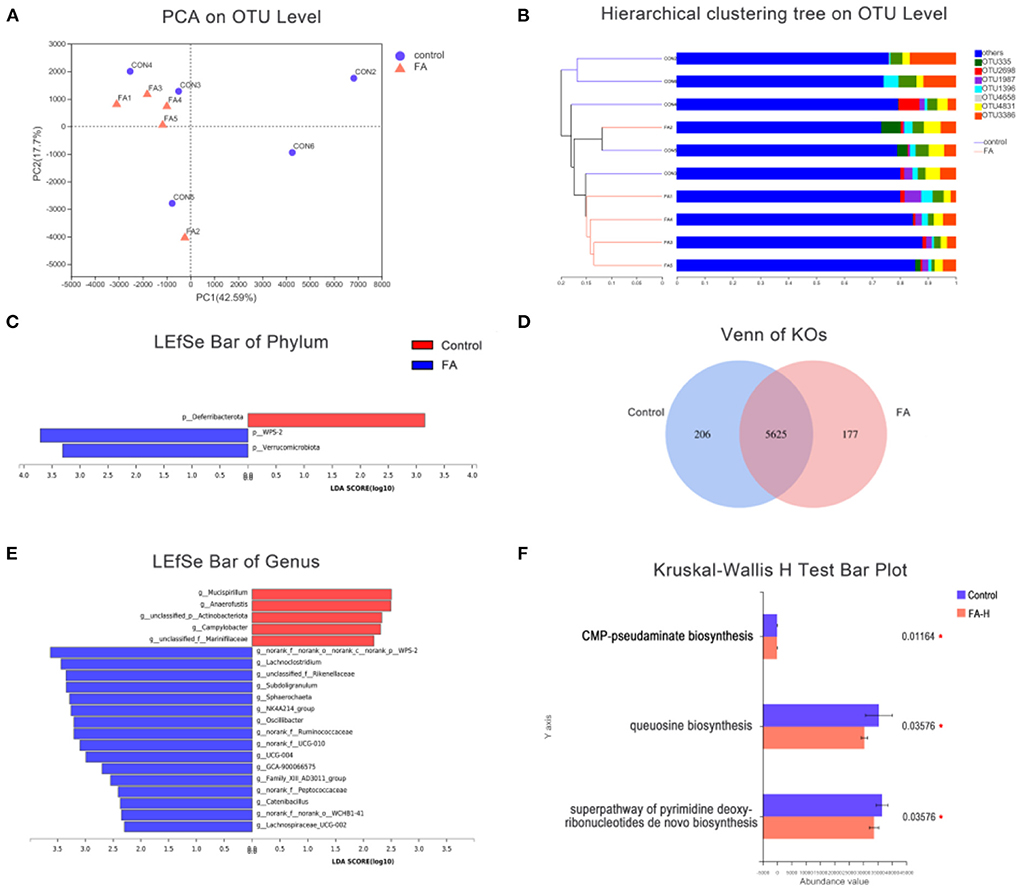
Figure 3. Effect of FA on gut microbiota. (A) PCA of gut microbiota communities based on OUT level. (B) Hierarchical sample clustering tree of the microbial community at OUT level based on Bray–Curtis distances. (C) Comparison of gut microbial variations at phylum level between control and FA-H group using LEfSe analysis. (D) Venn diagram indicated unique and shared KOs. (E) Comparison of gut microbial variations at genus level between control and FA-H group using LEfSe analysis. (F) Significantly enriched MetaCyc pathways of gut microbiota between the control and FA-H groups. *P < 0.05.
To investigate the significant features of gut microbiota between the control and FA-H groups, PICRUSt2 was used to predict KEGG orthologous groups (KOs), MetaCyc pathways, and the abundance of Cluster of Orthologous Group of Proteins (COG). A total of 304 KOs were altered between the control and FA-H groups (Supplementary Table 3). Venn diagrams showed that 206 and 177 KOs were unique to the control and FA-H groups, respectively (Figure 3D). Three MetaCyc functional pathways, including “Superpathway of pyrimidine deoxyribonucleotides de novo biosynthesis,” “Queuosine biosynthesis,” and “CMP-pseudaminate biosynthesis,” were significantly enriched (P < 0.05) in the control group (Figure 3F). In the COG classification, 4,126 OTUs were classified into 25 function classifications. The “Function unknown” represented the richest function category, followed by “Amino acid transport and metabolism,” “Translation, ribosomal structure, and biogenesis,” and “Cell wall/membrane/envelope biogenesis” (Supplementary Figure 1).
Alteration in metabolic signature between control and FA-H group
Using HPLC-MS, untargeted metabolite analysis was performed to investigate the alteration of the metabolome and examine the correlation between gut metabolites and microbiota. A total of 406 metabolites ions in both positive and negative ion modes were obtained from the extracted digesta samples of ceca. In addition, 14 annotated metabolites were significantly altered in the FA-H group compared to the control group (P < 0.05, PLS-DA VIP > 2) (Figure 4A; Supplementary Table 4). We performed receiver operating characteristic curve analysis on our data to evaluate the significance of metabolite discrimination. The area under the curve (AUC) was analyzed to assess the accuracy of potential biomarkers. Among the 14 differential metabolites, 12 showed an AUC of >0.85 with high sensitivity and specificity (Supplementary Figure 2). Therefore, 11β-Hydroxytestosterone, Asp-Ile, Biliverdin, Flurandrenolide, Gamma-Glu-Leu, Ganoderenic acid C, LysoPC 18:2, LysoPC 20:4 (8Z, 11Z, 14Z, 17Z), LysoPC 22:6 (4Z, 7Z, 10Z, 13Z, 16Z, 19Z), LysoPE 20:4 (5Z, 8Z, 11Z, 14Z)/0:0, Neocasomorphin, and Phosphocholine may be the key metabolites affected by FA.
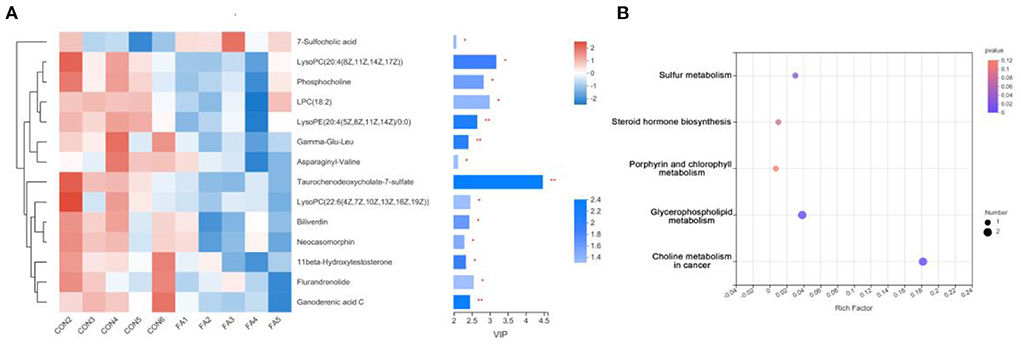
Figure 4. Effect of FA on gut metabolomic. (A) VIP scores with the expression of the heat map of intestinal metabolites in the FA-H and control groups. The selected metabolites were those with VIP ≥ 2 and P < 0.05. *P < 0.05, **P < 0.01. (B) Comparison of the KEGG enriched pathways of differential gut metabolites in the FA-H and in the control group. The size of the bubble represents the number of enriched metabolites, and the color indicates enrichment significance (P-value).
The functions of these differential metabolites were determined using KEGG pathway analysis (Figure 4B). According to the analysis, 3 KEGG pathways, including “Sulfur metabolism,” “Glycerophospholipid metabolism,” and “Choline metabolism in cancer,” were identified (P < 0.05). Additionally, four significantly decreased metabolites including LysoPC 22:6 (4Z, 7Z, 10Z, 13Z, 16Z, 19Z), Phosphocholine, LysoPC 20:4 (8Z, 11Z, 14Z, 17Z), and 11β-Hydroxytestosterone were involved in lipid metabolism. These results indicated that FA supplementation may primarily affect lipid metabolism.
Correlation analysis between intestinal microbiota and metabolites
To determine the association of changes in the gut microbiota and metabolites, we used the Spearman rank correlation analysis on the microbiota and metabolites that changed significantly after supplementation with FA (Figure 5A). Notably, nearly all differential metabolites showed a positive association with Campylobacter and Anaerofustis. After removing the redundant variables, seven metabolites were selected for RDA. The length of arrows in the RDA plot indicated the strength of the correlation between the environmental factor and sample distribution. RDA revealed that 11beta-Hydroxytestosterone, Flazine, and Taurochenodeoxycholate-7-sulfate exhibited the strongest correlation with microbiota composition (Figure 5B). However, no significant difference was found in the envfit analysis (Supplementary Table 5). To explore the key drivers involved in FA supplementation, we analyzed the co-occurrence analysis of microbiota and metabolites in the FA group. Base on the centralities of degree, closeness, and betweenness, the most important nodes of FA group were genus Lachnoclostridium and metabolite (3S, 5R, 6R, 6'S)-6,7-Didehydro-5,6-dihydro-3,5,6'-trihydroxy-13,14,20-trinor-3'-oxo-beta, epsilon-caroten-19',11'-olide 3-acetate (Figure 5C). Furthermore, the most important nodes of control were genus Olsenella, metabolites 5'-Deoxyadenosine and LysoPE 20:4 (5Z, 8Z, 11Z, 14Z)/0:0 (Figure 5D).
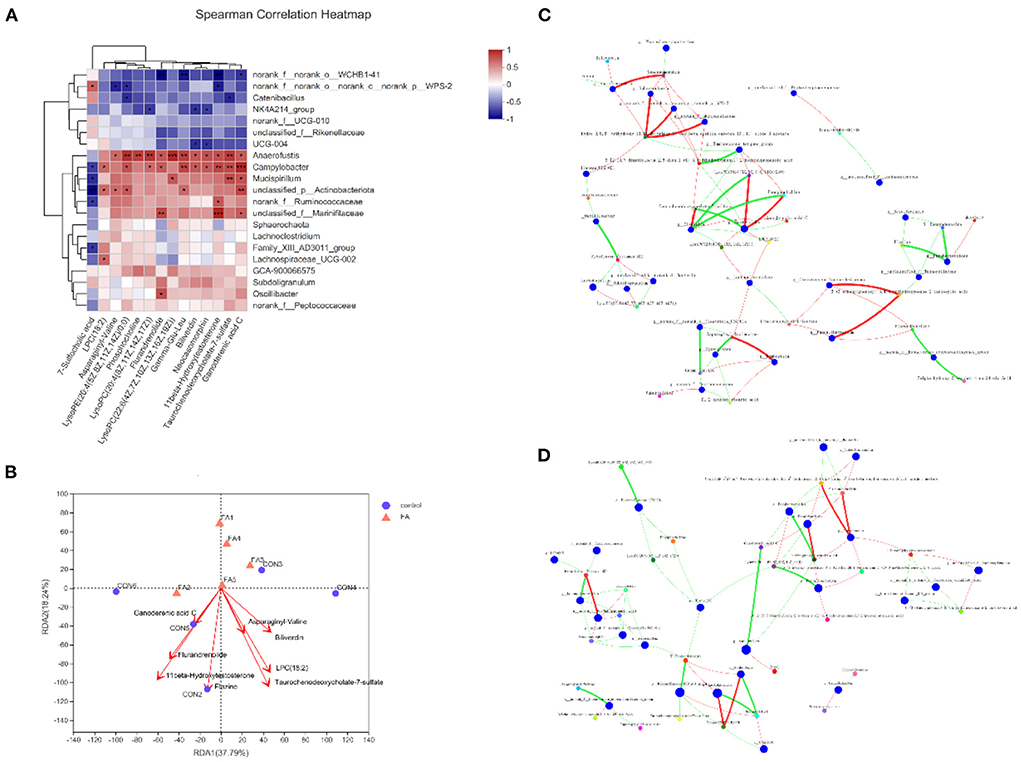
Figure 5. Correlation of gut metabolites and microbiota between FA-H and control group. (A) Heat map of correlation between differential metabolites and microbiota based on Spearman's correlation analysis. *P < 0.05, **P < 0.01, ***P < 0.001. (B) RDA plot showing the correlation between microbial community composition and metabolites. (C) Bivariate correlation analysis of microbiota and metabolites in FA-H group. (D) Bivariate correlation analysis of microbiota and metabolites in control. A connection represents a strong (Spearman's correlation coefficient [ρ] > 0.5) and significant (P < 0.01) correlation. Red indicates positive correlation, whereas green indicates negative correlation. The size of each node represents the relative abundance of the species.
Discussion
Effects of FA on growth and slaughter performance in Xianju yellow chicken
Feed cost is consistently the largest expense on poultry production, which represents ~65–75%. Therefore, the FCR is an important index to measure economic benefits. Several independent studies have reported the improvement of livestock and poultry growth performance after the dietary supplementation of FA. Chand et al. (7) indicated that diets supplemented with FA improved growth performance of pigs by increasing ADG and feed ratio. Additionally, Mao et al. (12) observed that the addition of FA in broiler diets increased body weight gain. In the present study, we supplemented the chickens with 1,500 mg/kg FA in diet, although it did not significantly improve the growth performance of Xianju yellow chicken, we observed an increase in the ADG and a decrease in the FCR. And supply of 1,500 mg/kg FA cost about $4 per ton, which may not cause additional burden.
Dressed percentage and eviscerated percentage are important indicators for judging the slaughter performance. Our results showed that dressed percentage and eviscerated percentage of all groups reached 91 and 66%, respectively, which were similar to previous studies (13). Fat is widely known as a by-product that has a relatively low commercial value. Moreover, excessive deposition of abdominal fats is not only unfavorable to the health of poultry animals but also associated with reproductive dysfunction (14). We noted that the addition of FA significantly decreased the percentage of abdominal fat, which suggested that FA may affect the deposition of abdominal fat.
Effects of FA on serum cytokine levels and liver function indexes in Xianju yellow chicken
The available literature contains little information on the immune-regulating effect of FA on the body. Our results have demonstrated that FA supplementation increased IL-2 levels and decreased TNF-α levels in serum, but did not affect IL-6, IL-10, and IFN-α levels. IL-2 is an important immune regulatory cytokine, and it can enhance T-cell proliferation, maintain T-cell function, and regulate the immune response function of T-cell, B-cell, NK cell, and monocytes (15). TNF-α is a well-known pro-inflammatory factor, and plays various roles in driving chronic inflammation and autoimmunity (16). TNF-α participates in vasodilation and edema formation, accelerates inflammation via increasing oxidative stress, and indirectly induces fever (17). Therefore, FA can regulate body immune response through activation of anti-inflammatory cytokines and suppression of pro-inflammatory cytokines.
The ALT and AST biochemical values were the main indicators for evaluating live health (18). As an enzyme, ALT is primarily present in cytosol, whereas AST is primarily present in mitochondria and cytoplasm in the liver. In cases of hepatocellular injury or alteration in the liver membrane permeability, ALT and AST are released into peripheral blood and elevate the serum levels of ALT and AST (19). Therefore, serum levels of ALT and AST are widely used as part of the routine diagnosis of liver disease. Serum TP may be elevated because of dehydration or diseases and reduced through impaired protein synthesis and increased protein loss (20). Owing to the fact that ALT, AST, and TP are reliable markers of liver function, our results revealed that the addition of FA at 1,500 mg/kg was safe for use and did not cause any disturbance to the liver functions of the chickens.
Effects of FA on gut microbiota in Xianju yellow chicken
Gut microbiota are essential in maintaining gastrointestinal homeostasis and host health (21, 22). In the present study, we noted that Lachnoclostridium and unclassified_f_Rikenellaceae have increased relative abundance in the FA-H group, which showed an association with inflammation. Lachnoclostridium is a genus of gram-positive, obligate anaerobic, spore-forming, motile bacteria, which ferment mono- and disaccharides to produce acetate (23). The relative abundance of Lachnoclostridium was reportedly reduced in DSS-induced mice (24, 25), and negatively related to IL-6 (26). Rikenellaceae, comprises two genera, Alistipes and Rikenella (27). The proportion of Rikenellaceae was positively associated with TLR3, TLR8, sIgA, and IgG (28, 29). Our results demonstrated that FA could influence the specific microbes, which in turn affected immune regulation and intestinal homeostasis.
Furthermore, an increased abundance of several SCFA-producing genera, including Subdoligranulum, Sphaerochaeta, and Catenibacillus, were observed in the FA-H group. Subdoligranulum, which was isolated from human feces, is strictly anaerobic, gram-negative, and negative in terms of the intestinal permeability of the host (30, 31). Different from other members of phylum Spirochaetes, Sphaerochaeta genomes lacked the genes about motility and associated signal transduction; however, they are highly enriched in genes related to fermentation and carbohydrate metabolism (32). Consequently, they grow as biofilms and by fermenting carbohydrates to formate, acetate, propionate, and iso-butyrate (33, 34). Catenibacillus is a genus of anaerobic gram-positive bacteria and typically appears in the form of chains (35). Previous studies have shown that Catenibacillus could deglycosylate by not only deglycosylating puerarin to daidzein but also other aromatic C-glucosides and flavonoid O-glucosides (36). Their catabolites, protocatechuic acid, 3,4-dihydroxyphenylacetic acid, and 3,4-dihydroxyphenylpropionic acid, have been verified to exert anti-inflammatory and antioxidant effects by decreasing the level of pro-inflammatory cytokines IL-1β, IL-6, and TNF-α and reducing the contents of NO, H2O2, and malondialdehyde (37–39). These results implied that FA facilitated the abundance of SCFA-producing bacteria, and in turn regulated body fat metabolism and energy production.
Mucispirillum, Anaerofustis, and Campylobacter were three bacteria that were found enriched in the control birds. Mucispirillum colonizes in the intestinal mucus layer and exhibits the ability to degrade mucin (40). Although Mucispirillum schaedleri can assist the host's defenses against gut inflammation induced by Salmonella Typhimurium infection (41), most studies have demonstrated that several disease conditions were associated with Mucispirillum, including Crohn's disease, pathogen infection, and Parkinson's disease (42). Multiple studies have demonstrated that Mucispirillum was enriched during active colitis, and it has been identified as an indicator for colitis in the model of colitis (43, 44). Anaerofustis is a strictly anaerobic and gram-positive genus belonging to the family of Eubacteriaceae (45, 46) reported that Anaerofustis was enriched in heat-stressed broilers. In addition, stress susceptibility was associated with the increase of Anaerofustis in rats (47). Campylobacter is one of the most common bacterial causes of diarrhea worldwide; poultry is known to be the major source of this organism. Previous research supported that Campylobacter usually develops as an asymptomatic infection in poultry (48). However, recent studies have challenged this point and argue that Campylobacter disturbs poultry performance by impairing intestinal integrity, increasing intestinal permeability and bacterial translocation, and enhancing inflammatory response (49). Our results indicated that FA might promote poultry health by reducing the abundance of pathogenic microorganisms.
Effects of FA on gut metabolome in Xianju yellow chicken
Phosphocholines are storage forms for choline within the cytosol. Studies have reported that phosphocholines were associated with cell and hepatic regeneration, and significantly elevated in acute hepatitis and liver injury (50, 51). Chenodeoxycholic acid, cholic acid, and b biliverdin are the main components of bile in poultry. The decreased amounts of taurochenodeoxycholate-7-sulfate and biliverdin in the intestinal tract may indicate that FA is involved in bile acid circulation. However, the underlying specific mechanisms are required for further investigation. Notably, the amount of some dipeptides and peptides, including γ-Glu-Leu, Asp-Val, and neocasomorphin, were significantly decreased in the FA group, which might be related to reduced microbial abundance.
Conclusion
The present study suggests the use of novel feed additives such as FA in poultry production. On the basis of our finding, FA at the level of 1,500 mg/kg into the diet of Xianju yellow chicken could enhance growth performance, improve immune function, and modulate intestinal microbiota community and metabolites.
Data availability statement
The data presented in the study are deposited in the NCBI SRA BioProject repository, and the accession number is PRJNA841423.
Ethics statement
The animal study was reviewed and approved by the Ethics Committee of the Zhejiang Academy of Agricultural Sciences.
Author contributions
PF: conceptualization, methodology, formal analysis, investigation, and writing-original draft. QL: software and formal analysis. HS and JG: investigation. XY: resources. YT: writing-review and editing and funding acquisition. PW: project administration and funding acquisition. All authors contributed to the article and approved the submitted version.
Funding
This study was financially supported by the Key Research and Development Program of Zhejiang Province (Nos. 2022C03050 and 2021C02034) and Zhejiang Provincial Special Commissioner Team Projects of Science & Technology (No. Xianju Chicken Industry, 2020-2024).
Acknowledgments
We thank Slona Biological Technology Co., Ltd. (Beijing, China) for supplying the fulvic acid. We also acknowledge the free online platform of Majorbio Cloud Platform (https://cloud.majorbio.com/page/tools/).
Conflict of interest
Author XY was employed by the company Xianju Breeding Chicken Farm, Taizhou, China.
The remaining authors declare that the research was conducted in the absence of any commercial or financial relationships that could be construed as a potential conflict of interest.
Publisher's note
All claims expressed in this article are solely those of the authors and do not necessarily represent those of their affiliated organizations, or those of the publisher, the editors and the reviewers. Any product that may be evaluated in this article, or claim that may be made by its manufacturer, is not guaranteed or endorsed by the publisher.
Supplementary Material
The Supplementary Material for this article can be found online at: https://www.frontiersin.org/articles/10.3389/fnut.2022.963271/full#supplementary-material
Supplementary Figure 1. COG function classification of the control and FA-H groups.
Supplementary Figure 2. The ROC analysis of differential metabolites between control and FA-H group.
References
1. Alvarez-Puebla RA, Valenzuela-Calahorro C, Garrido JJ. Theoretical study on fulvic acid structure, conformation and aggregation. Sci Total Environ. (2006) 358:243–54. doi: 10.1016/j.scitotenv.2004.11.026
2. Winkler J, Ghosh S. Therapeutic potential of fulvic acid in chronic inflammatory diseases and diabetes. J Diabetes Res. (2018) 2018:5391014. doi: 10.1155/2018/5391014
3. Wang C, Wang Z, Peng A, Hou J, Xin W. Interaction between fulvic acids of different origins and active oxygen radicals. Sci China C Life Sci. (1996) 39:267–75.
4. Zhao Y, Paderu P, Delmas G, Dolgov E, Lee MH, Senter M, et al. Carbohydrate-derived fulvic acid is a highly promising topical agent to enhance healing of wounds infected with drug-resistant pathogens. J Trauma Acute Care Surg. (2015) 79(4 Suppl 2):S121–29. doi: 10.1097/TA.0000000000000737
5. Schepetkin IA, Khlebnikov AI, Ah SY, Woo SB, Jeong C, Klubachuk ON, et al. Characterization and biological activities of humic substances from mumie. J Agr Food Chem. (2003) 51:5245–54. doi: 10.1021/jf021101e
6. Rahmani Barouji S, Saber A, Torbati M, Fazljou SMB, Yari Khosroushahi A. Health beneficial effects of moomiaii in traditional medicine. Galen. (2020) 9:e1743. doi: 10.31661/gmj.v9i0.1743
7. Chang Q, Lu Z, He M, Gao R, Bai H, Shi B, et al. Effects of dietary supplementation of fulvic acid on lipid metabolism of finishing pigs. J Anim Sci. (2014) 92:4921–26. doi: 10.2527/jas.2014-8137
8. Lieke T, Steinberg CEW, Pan B, Perminova IV, Meinelt T, Knopf K, et al. Phenol-rich fulvic acid as a water additive enhances growth, reduces stress, and stimulates the immune system of fish in aquaculture. Sci Rep UK. (2021) 11:174. doi: 10.1038/s41598-020-80449-0
9. Logue JB, Stedmon CA, Kellerman AM, Nielsen NJ, Andersson AF, Laudon H, et al. Experimental insights into the importance of aquatic bacterial community composition to the degradation of dissolved organic matter. Isme J. (2016) 10:533–45. doi: 10.1038/ismej.2015.131
10. Segata N, Izard J, Waldron L, Gevers D, Miropolsky L, Garrett WS, et al. Metagenomic biomarker discovery and explanation. Genome Biol. (2011) 12:R60. doi: 10.1186/gb-2011-12-6-r60
11. Douglas GM, Maffei VJ, Zaneveld JR, Yurgel SN, Brown JR, Taylor CM, et al. PICRUSt2 for prediction of metagenome functions. Nat Biotechnol. (2020) 38:685–88. doi: 10.1038/s41587-020-0548-6
12. Mao Y. Modulation of the growth performance, meat composition, oxidative status, and immunity of broilers by dietary fulvic acids. Poult Sci. (2019) 98:4509–13. doi: 10.3382/ps/pez281
13. Tang H, Gong YZ, Wu CX, Jiang J, Wang Y, Li K. Variation of meat quality traits among five genotypes of chicken. Poult Sci. (2009) 88:2212–18. doi: 10.3382/ps.2008-00036
14. Zhang XY, Wu MQ, Wang SZ, Zhang H, Du ZQ, Li YM, et al. Genetic selection on abdominal fat content alters the reproductive performance of broilers. Animal. (2018) 12:1232–41. doi: 10.1017/S1751731117002658
15. Ross SH, Cantrell DA. Signaling and function of interleukin-2 in t lymphocytes. Annu Rev Immunol. (2018) 36:411–33. doi: 10.1146/annurev-immunol-042617-053352
16. Wynn TA, Chawla A, Pollard JW. Macrophage biology in development, homeostasis and disease. Nature. (2013) 496:445–55. doi: 10.1038/nature12034
17. Zelová H, Hošek J. TNF-α signalling and inflammation: interactions between old acquaintances. Inflamm Res. (2013) 62:641–51. doi: 10.1007/s00011-013-0633-0
18. Bajaj JS. Alcohol, liver disease and the gut microbiota. Nat Rev Gastroenterol Hepatol. (2019) 16:235–46. doi: 10.1038/s41575-018-0099-1
19. Ramaiah SK. A toxicologist guide to the diagnostic interpretation of hepatic biochemical parameters. Food Chem Toxicol. (2007) 45:1551–57. doi: 10.1016/j.fct.2007.06.007
20. Dawnay AB, Hirst AD, Perry DE, Chambers RE. A critical assessment of current analytical methods for the routine assay of serum total protein and recommendations for their improvement. Ann Clin Biochem. (1991) 28:556–67. doi: 10.1177/000456329102800604
21. Huang P, Jiang A, Wang X, Zhou Y, Tang W, Ren C, et al. NMN maintains intestinal homeostasis by regulating the gut microbiota. Front Nutr. (2021) 8:714604. doi: 10.3389/fnut.2021.714604
22. Huang P, Zhou Y, Tang W, Ren C, Jiang A, Wang X, et al. Long-term treatment of nicotinamide mononucleotide improved age-related diminished ovary reserve through enhancing the mitophagy level of granulosa cells in mice. J Nutr Biochem. (2022) 101:108911. doi: 10.1016/j.jnutbio.2021.108911
23. Yutin N, Galperin MY. A genomic update on clostridial phylogeny: gram-negative spore formers and other misplaced clostridia. Environ Microbiol. (2013) 15:2631–41. doi: 10.1111/1462-2920.12173
24. Zhang W, Zou G, Li B, Du X, Sun Z, Sun Y, et al. Fecal microbiota transplantation (FMT) alleviates experimental colitis in mice by gut microbiota regulation. J Microbiol Biotechn. (2020) 30:1132–41. doi: 10.4014/jmb.2002.02044
25. Wang C, Li W, Wang H, Ma Y, Zhao X, Zhang X, et al. Saccharomyces boulardii alleviates ulcerative colitis carcinogenesis in mice by reducing TNF-α and IL-6 levels and functions and by rebalancing intestinal microbiota. BMC Microbiol. (2019) 19:246. doi: 10.1186/s12866-019-1610-8
26. Pagliai G, Russo E, Niccolai E, Dinu M, Di Pilato V, Magrini A, et al. Influence of a 3-month low-calorie mediterranean diet compared to the vegetarian diet on human gut microbiota and SCFA: the CARDIVEG study. Eur J Nutr. (2020) 59:2011–24. doi: 10.1007/s00394-019-02050-0
27. Graf J. The family rikenellaceae. In: Rosenberg E, DeLong EF, Lory S, Stackebrandt E, Thompson F, editors. The Prokaryotes: Other Major Lineages of Bacteria and the Archaea. Berlin: Springer Berlin Heidelberg (2014). p. 857–59.
28. van der Eijk JAJ, de Vries H, Kjaer JB, Naguib M, Kemp B, Smidt H, et al. Differences in gut microbiota composition of laying hen lines divergently selected on feather pecking. Poultry Sci. (2019) 98:7009–21. doi: 10.3382/ps/pez336
29. Lu J, Zhu M, Cao H, Zhang X, Wang Z, Zhang X, et al. Impact of fermented corn–soybean meal on gene expression of immunity in the blood, level of secretory immunoglobulin a, and mucosa-associated bacterial community in the intestine of grower–finisher pigs. Front Ve Sci. (2020) 7:246. doi: 10.3389/fvets.2020.00246
30. Leclercq S, Matamoros S, Cani PD, Neyrinck AM, Jamar F, Stärkel P, et al. Intestinal permeability, gut-bacterial dysbiosis, and behavioral markers of alcohol-dependence severity. Proc Natl Acad Sci USA. (2014) 111:E4485–93. doi: 10.1073/pnas.1415174111
31. Holmstrøm K, Collins MD, Møller T, Falsen E, Lawson PA. Subdoligranulum variabile gen. nov., sp. nov. from human feces. Anaerobe. (2004) 10:197–203. doi: 10.1016/j.anaerobe.2004.01.004
32. Caro-Quintero A, Ritalahti KM, Cusick KD, Loffler FE, Konstantinidis KT. The chimeric genome of Sphaerochaeta: nonspiral spirochetes that break with the prevalent dogma in spirochete biology. Mbio. (2012) 3:e00025-12. doi: 10.1128/mBio.00025-12
33. Ritalahti KM, Justicia-Leon SD, Cusick KD, Ramos-Hernandez N, Rubin M, Dornbush J, et al. Sphaerochaeta globosa gen. nov., sp. nov. and Sphaerochaeta pleomorpha sp. nov., free-living, spherical spirochaetes. Int J Syst Evol Micr. (2012) 62:210–16. doi: 10.1099/ijs.0.023986-0
34. Bidzhieva SK, Sokolova DS, Grouzdev DS, Kostrikina NA, Poltaraus AB, Tourova TP, et al. Sphaerochaeta halotolerans sp. nov., a novel spherical halotolerant spirochete from a Russian heavy oil reservoir, emended description of the genus Sphaerochaeta, reclassification of Sphaerochaeta coccoides to a new genus Parasphaerochaeta gen. nov. as Parasphaerochaeta coccoides comb. nov. and proposal of Sphaerochaetaceae fam. nov. Int J Syst Evol Micr. (2020) 70:4748–59. doi: 10.1099/ijsem.0.004340
35. Braune A, Blaut M. Catenibacillus scindens gen. nov., sp. nov., a C-deglycosylating human intestinal representative of the Lachnospiraceae. Int J Syst Evol Micr. (2018) 68:3356–61. doi: 10.1099/ijsem.0.003001
36. Braune A, Blaut M. Deglycosylation of puerarin and other aromatic C-glucosides by a newly isolated human intestinal bacterium. Environ Microbiol. (2011) 13:482–94. doi: 10.1111/j.1462-2920.2010.02352.x
37. Bunt D, Minnaard A, El Aidy S. Potential modulatory microbiome therapies for prevention or treatment of inflammatory bowel diseases. Pharmaceuticals. (2021) 14:506. doi: 10.3390/ph14060506
38. Li M, Bao X, Zhang X, Ren H, Cai S, Hu X, et al. Exploring the phytochemicals and inhibitory effects against α-glucosidase and dipeptidyl peptidase-IV in Chinese pickled chili pepper: insights into mechanisms by molecular docking analysis. Lwt. (2022) 162:113467. doi: 10.1016/j.lwt.2022.113467
39. Shang Z, Li M, Zhang W, Cai S, Hu X, Yi J. Analysis of phenolic compounds in pickled chayote and their effects on antioxidant activities and cell protection. Food Res Int. (2022) 157:111325. doi: 10.1016/j.foodres.2022.111325
40. Robertson BR, O'Rourke JL, Neilan BA, Vandamme P, On S, Fox JG, et al. Mucispirillum schaedleri gen. nov., sp. nov., a spiral-shaped bacterium colonizing the mucus layer of the gastrointestinal tract of laboratory rodents. Int J Syst Evol Microbiol. (2005) 55:1199–204. doi: 10.1099/ijs.0.63472-0
41. Herp S, Brugiroux S, Garzetti D, Ring D, Jochum LM, Beutler M, et al. Mucispirillum schaedleri antagonizes salmonella virulence to protect mice against colitis. Cell Host Microbe. (2019) 25:681–94. doi: 10.1016/j.chom.2019.03.004
42. Herp S, Durai Raj AC, Salvado Silva M, Woelfel S, Stecher B. The human symbiont mucispirillum schaedleri: causality in health and disease. Med Microbiol Immun. (2021) 210:173–79. doi: 10.1007/s00430-021-00702-9
43. Berry D, Kuzyk O, Rauch I, Heider S, Schwab C, Hainzl E, et al. Intestinal microbiota signatures associated with inflammation history in mice experiencing recurring colitis. Front Microbiol. (2015) 6:1408. doi: 10.3389/fmicb.2015.01408
44. Berry D, Schwab C, Milinovich G, Reichert J, Ben Mahfoudh K, Decker T, et al. Phylotype-level 16S rRNA analysis reveals new bacterial indicators of health state in acute murine colitis. ISME J. (2012) 6:2091–106. doi: 10.1038/ismej.2012.39
45. Finegold SM, Lawson PA, Vaisanen ML, Molitoris DR, Song Y, Liu C, et al. Anaerofustis stercorihominis gen. nov., sp. nov., from human feces. Anaerobe. (2004) 10:41–5. doi: 10.1016/j.anaerobe.2003.10.002
46. Liu G, Zhu H, Ma T, Yan Z, Zhang Y, Geng Y, et al. Effect of chronic cyclic heat stress on the intestinal morphology, oxidative status and cecal bacterial communities in broilers. J Therm Biol. (2020) 91:102619. doi: 10.1016/j.jtherbio.2020.102619
47. Zhang K, Fujita Y, Chang L, Qu Y, Pu Y, Wang S, et al. Abnormal composition of gut microbiota is associated with resilience versus susceptibility to inescapable electric stress. Transl Psychait. (2019) 9:231. doi: 10.1038/s41398-019-0571-x
48. Massacci FR, Lovito C, Tofani S, Tentellini M, Genovese DA, De Leo AAP, et al. Dietary saccharomyces cerevisiae boulardii CNCM I-1079 positively affects performance and intestinal ecosystem in broilers during a campylobacter jejuni infection. Microorganisms. (2019) 7:596. doi: 10.3390/microorganisms7120596
49. Awad WA, Hess C, Hess M. Re-thinking the chicken-Campylobacter jejuni interaction: a review. Avian Pathol. (2018) 47:352–63. doi: 10.1080/03079457.2018.1475724
50. Oberhaensli R, Rajagopalan B, Galloway GJ, Taylor DJ, Radda GK. Study of human liver disease with P-31 magnetic resonance spectroscopy. Gut. (1990) 31:463–67. doi: 10.1136/gut.31.4.463
Keywords: gut microbiota, growth performance, inflammation, Xianju yellow chicken, fulvic acid
Citation: Feng P, Li Q, Sun H, Gao J, Ye X, Tao Y, Tian Y and Wang P (2022) Effects of fulvic acid on growth performance, serum index, gut microbiota, and metabolites of Xianju yellow chicken. Front. Nutr. 9:963271. doi: 10.3389/fnut.2022.963271
Received: 07 June 2022; Accepted: 11 July 2022;
Published: 05 August 2022.
Edited by:
Quancai Sun, National University of Singapore, SingaporeCopyright © 2022 Feng, Li, Sun, Gao, Ye, Tao, Tian and Wang. This is an open-access article distributed under the terms of the Creative Commons Attribution License (CC BY). The use, distribution or reproduction in other forums is permitted, provided the original author(s) and the copyright owner(s) are credited and that the original publication in this journal is cited, in accordance with accepted academic practice. No use, distribution or reproduction is permitted which does not comply with these terms.
*Correspondence: Yong Tian, MTAxNTA4MDE1MyYjeDAwMDQwO3FxLmNvbQ==; Ping Wang, d2FuZ3Bpbmc0NSYjeDAwMDQwO3pqdXQuZWR1LmNu
†These authors have contributed equally to this work and share first authorship