- 1College of Pharmacy, Changchun University of Chinese Medicine, Changchun, China
- 2College of Life Sciences, Changchun Sci-Tech University, Changchun, China
- 3College of Food Science and Engineering, Jilin Agriculture University, Changchun, China
Numerous studies reported that betulinic acid (BA), a natural product extracted from birch bark, exhibited various beneficial effects in vitro. However, its pharmacological activities in aging are rarely understood. In this study, Caenorhabditis elegans was deployed as a whole animal model to investigate the impacts of BA on lifespan and stress resistance. Wild-type C. elegans were fed in the presence or absence of BA and tested for a series of phenotypes, including longevity, mobility, reproductive capacity, pharyngeal pumping, heat stress, and oxidative stress. BA at the optimal dose (50 μg/mL) extended the lifespan, improved the healthspan, and significantly evoked the increased oxidative stress resistance in C. elegans. Incorporating the genetic analysis with different types of longevity mutants, DAF-16, the downstream effector of the Insulin/IGF-1 receptor signaling, was revealed to mediate the protective effects of BA on lifespan and antioxidant activity. Together, these data showcased the potential of BA in promoting healthy aging, which shall facilitate its further development in the food and pharmaceutical industries.
Introduction
Aging is one of the most significant burdens on public health. It currently accounts for about two-thirds of global deaths daily (1). In humans, aging is accompanied by complex physiological and pathological changes in almost all the organs, thus, causing shorten lifespan, reduced stress resistance, and progressive dysfunction (2). Aging research has gained milestones on the underlying mechanisms; some molecules and signaling pathways play a pivotal role in aging processes, including target of rapamycin (TOR) proteins (3), nicotinamide adenine dinucleotide (NAD+) (4), insulin-like signaling pathway (5), Chronic inflammation (6), mitochondria and oxidative stress (7). Although aging is irreversible, these discoveries have also pointed out the possible ways for aging intervention to increase lifespan and achieve healthy aging (8). For example, natural compounds with antioxidant activity, such as broccoli-derived isothiocyanate sulforaphane (9) and resveratrol in red wine (10), have been proven to exhibit anti-aging effects via different mechanisms.
Betulinic acid (BA), the naturally occurring lupin-type pentacyclic triterpenoid, represents another type of potential drug lead compound with antioxidant activity. BA is widely distributed in plants and most abundant in birch bark. Birch bark and its derivatives have been used in traditional medicines to treat gastrointestinal ailments such as diarrhea and dysentery in China, Russia, the United States, and other countries (11). The previous works have consistently documented the beneficial effects of BA in antioxidant, anti-inflammatory, antibacterial, antiviral, antidiabetic, antimalarial, anti-HIV, and antitumor activities (12, 13). Notably, while numerous studies have showcased the great value of BA in alleviating oxidative damages in vitro, the in vivo evidence is rare, and there is no functional assessment at the whole animal level. As a result, it is still unknown whether BA has an anti-aging effect or not.
In the past three decades, Caenorhabditis elegans (C. elegans) has become a premier animal model for aging research. As an intact multicellular organism, the short lifecycle, tractable genetics, and behavioral phenotypes decline with aging, making C. elegans an excellent system for investigating the environmental and genetic basis of lifespan. Some unprecedented advances in understanding the aging process stem from seminal discoveries in C. elegans and then translated to higher organisms, such as the regulation of longevity by DAF-2/DAF-16/insulin/insulin-like growth factor signaling (5). Moreover, C. elegans also has profound implications for dissecting mitochondrial longevity pathways (14), reproductive aging (15), oxidative stress (16), and dietary restriction (17). In conclusion, C. elegans offers substantial advantages for studying the basic biology of aging and the translational applications of anti-aging.
In this study, C. elegans was implemented to investigate the pharmacological activities of BA in lifespan and stress resistance. BA exhibited dose-dependent lifespan extension. The healthy aging-related behavioral phenotypes, including locomotion, pharyngeal pumping, heat stress, and oxidative stress, were also improved at the optimal dose. After testing with the longevity mutants affecting mitochondria metabolism, dietary restriction, reproductive capacity, or insulin/IGF-1 receptor signaling, DAF-16, the downstream effector of the insulin/IGF-1 receptor signaling, was discovered to mediate the protective effects of BA on longevity and antioxidant activity. The findings showcased the potential of BA in promoting healthy aging, which will facilitate its further development in the food and pharmaceutical industries.
Materials and methods
Chemicals and reagents
Betulinic acid (BA, 98% purity, Aladdin, Shanghai, China), total superoxide dismutase (T-SOD; R22262), catalase (CAT; R30337), malondialdehyde (MDA; R21869), and glutathione peroxidase (GSH-Px; R21876) assay kits were purchased from Shanghai Yeyuan Bioengineering Research Institute (Shanghai, China). 2′, 7′-dichlorofluorescein diacetate (DCFH-DA), and 5-hydroxy-1,4-naphthalenedione (juglone) were purchased from Sigma-Aldrich Chemical Co. (St Louis, MO, United States). All chemicals and solvents were analytical grades or higher.
Nematode strains and maintenance
Caenorhabditis elegans strains were provided by Caenorhabditis Genetics Center (CGC, University of Minnesota, Minneapolis, MN, United States) and maintained using standard protocols at 20°C. The following mutant alleles and transgenes were used: wild-type Bristol N2 isolate, eat-2 (ad1116), daf-2 (e1368), clk-1 (qm30), glp-1 (e2141), daf-16 (mgDf50), skn-1 (zu67), sod-1 (tm776), sod-2 (gk257), sod-3 (tm760), sod-4 (gk101), sod-5 (tm1146), ctl-1 (ok1242), ctl-2 (ok1137), ctl-3 (ok2042), and GR1352 (daf-16 [mgDf47] I; xrIs87 [Pdaf–16GFP]).
Toxicity assay
Betulinic acid is readily soluble in dimethyl sulfoxide (DMSO) but not in water (18). Therefore, DMSO was used to dissolve BA to make the stock solution, which was then diluted in LB liquid medium containing Escherichia coli OP50. It was ensured that the concentration of DMSO in the medium did not exceed 0.3% since studies have shown that DMSO content lower than 0.3% does not affect the health and lifespan of C. elegans (19). The synchronized C. elegans at the L4 stage were transferred to NGM plates containing appropriate working doses of BA (10, 50, 100, 200, 500, and 1,000 μg/mL) (20). The 0.3% DMSO group was used as vehicle control, and the pure blank control was also set. The age-synchronized animals (30 animals/well and three wells/condition replicates) were transferred to 300 μL of liquid NGM medium in 24-well plates supplemented with BA. The experiment was performed at 20°C, and the survival rate was scored every hour. The experiment was repeated three times, and each experimental group included at least 90 worms.
Lifespan assay
According to the drug toxicity assay (Section “Toxicity assay”), the optimal dose of BA was set at 50 μg/mL. FUDR (12.5 mg/mL) was added to the medium to inhibit the egg-laying of worms. The synchronized animals were picked into the medium at the L4 stage, 60 animals/well and three wells/condition replicates (21). The day of transfer was recorded as Day 0, and the counts were scored daily. When it was observed that the animals no longer wriggled independently, a heated platinum wire hovered over the animals but without contact. Those animals that did not respond to stimuli were scored as dead. The experiment ended when all the animals died in each group.
Escherichia coli OP50 metabolism experiment
Escherichia coli OP50 bacterial solution (100 mL) was inactivated by incubation at 121°C for 20 min. The inactivated bacterial solution was dispensed into sterile centrifuge tubes and centrifuged at 4,000 rpm/min for 5 min. Two-thirds of the supernatant volume was removed under aseptic conditions. The remaining bacterial solution was sonicated for 5 min, thoroughly and evenly mixed, and then added dropwise to the surface of the NGM plate (22). The synchronized animals were grown into adults and transferred to the inactivated bacteria plates of the experimental group (50 μg/mL BA) and the control group. The lifespan assay was carried out according to Section “Lifespan assay.”
Reproductivity, mobility, pharyngeal pumping, and lipofuscin assays
For the reproductivity assay, the synchronized C. elegans were grown into gravid adults and then transferred to fresh medium daily in egg-laying. The total numbers of eggs were scored according to their hatching rates with or without BA application.
For the mobility assay, the synchronized C. elegans were grown to the L4 stage and then transferred to the appropriate medium. The day of transfer was recorded as Day 0, and the locomotion of the C. elegans was quantified on the 4th, 8th, and 12th days. A sinusoidal motion was defined as a one-wavelength shift relative to the long axis of the body (23). The mobility was designated to three levels as following standards: Class A, animals move spontaneously and smoothly; Class B, animals don’t move unless stimulated; Class C, animals don’t move but wiggle their noses or tails in response to touch (24).
For the pharyngeal pumping assay, the synchronized C. elegans were visualized at the magnification of 50X under a stereomicroscope. The pumping frequency of the terminal pharyngeal bulb was counted for 30s at room temperature (25).
For the lipofuscin assay, C. elegans were anesthetized on agarose pads with 2% sodium azide. The autofluorescence of lipofuscin in vivo was detected with an inverted fluorescence microscope using 355/445 nm (Ex/Em) and 550/605 nm (Ex/Em). The fluorescence intensity was analyzed using ImageJ 8.5 software.
Stress resistance assays
Prior to stress exposure, the synchronized C. elegans were grown up to the L4 stage in the presence or absence of BA (50 μg/mL). FUDR (12.5 mg/mL) was added to inhibit egg-laying.
Thermal shock assay
Caenorhabditis elegans were put into incubators at 35, 38, or 40°C. Their viability was scored every hour until all the animals died (26). The experiment was repeated three times.
Juglone-induced oxidative stress assay
Wild-type C. elegans and the indicated mutants (clk-1, daf-16, eat-2, and glp-1) were put into the NGM plates containing 200 μM juglone. Their viability was scored every hour until all the animals died (27). The experiment was repeated three times.
Antioxidant enzyme activity and reactive oxygen species determination in Caenorhabditis elegans
The synchronized C. elegans were fed in the presence or absence of BA and grew up to the L4 stage. After three times washing with M9 buffer, the animals were collected by high-speed centrifuge and lysed for protein extraction. The protein concentrations were quantified to ensure consistency, and the supernatants of each sample were added to the 96-well plate. The kits for malondialdehyde (MDA), total superoxide dismutase (T-SOD), glutathione peroxide (GSH-Px) kit, and catalase (CAT) were used to measure antioxidant enzyme activities and lipid oxidation levels following the corresponding instructions.
To detect ROS with DCFH-DA staining, the L4-stage C. elegans were washed and collected into the centrifuge tube. A 200 μL aliquot of 10 mM DCFH-DA solution was added, and the tube was shaken gently to make animals in contact with the dye solution evenly. The staining lasted for 30 min at 37°C and was stopped by discarding the dye solution. The animals were washed with M9 buffer and anesthetized with levamisole. The animals were loaded onto agarose pads, and the images were captured with an inverted fluorescence microscope using 504/529 nm (Ex/Em). The fluorescence intensity was analyzed using ImageJ 8.5 software.
Effects of betulinic acid on the expression levels of related antioxidant genes in Caenorhabditis elegans
The synchronized C. elegans were fed in the presence or absence of BA and grew up to the L4 stage. After three times washing with M9 buffer, the animals were collected by high-speed centrifuge. The total RNA was extracted using the TransZol Up Plus RNA Kit and was reverse-transcribed into cDNA using the EasyScript One-Step gDNA Removal and cDNA Synthesis SuperMix Kit. The expression of antioxidant genes (ctl-1, ctl-2, ctl-3, sod-1, sod-2, sod-3, sod-4, and sod-5) was detected using the 2–ΔΔCt method. The primer sequences of the RT-qPCR reaction are shown in Table 1.
Gene knock-down experiment
Gene knock-down was achieved by RNAi strategy. The plasmid pL4440 [Tiangen Biotech (Beijing) Co., Ltd., Beijing, China] was used to construct the E. coli strain for the induction of RNAi by feeding in C. elegans. In brief, the cDNA of daf-16 was cloned into the L4440 plasmid and then transformed into the HT115 E. coli strain. The RNAi-feeding bacteria were cultured and harvested. C. elegans were fed with the bacteria to achieve RNAi of the daf-16 gene. The gene knock-down efficiency was confirmed by RT-qPCR of daf-16. To determine the lifespan of daf-16 RNAi strain, the experimental procedures were described in Section “Reproductivity, mobility, pharyngeal pumping, and lipofuscin assays.” To analyze the downstream genes (clk-1, ctl-2, isp-1, dod-17, and sod-3) affected by daf-16 RNAi, the RT-qPCR method was described in Section “Effects of betulinic acid on the expression levels of related antioxidant genes in Caenorhabditis elegans.”
Statistical analysis
All the experiments were repeated at least three times. GraphPad Prism 8.0 (San Diego, CA, United States) was used for data analysis. The data of the lifespan assays and stress resistance assays were analyzed using the Kaplan-Meier method, and the p-values of survival differences were determined with the log-rank test. The data were presented as mean and SEM in the other groups. “*” indicates P < 0.05, i.e., the difference is statistically significant, “**” indicates P < 0.01, i.e., a significant difference, and “***” indicates P < 0.001, i.e., a very significant difference.
Results
Betulinic acid extends lifespan in Caenorhabditis elegans
The study began by performing an in vivo assay for drug toxicity screening. The wild-type C. elegans were treated with various doses of BA, and their survival rates were scored 24 h later. BA did not affect the viability of animals when at the dose range of 0∼100 μg/mL (Figure 1A). Accordingly, BA at the concentrations of 10, 50, and 100 μg/mL were selected for the lifespan assay. To avoid the potential effect caused by long-term exposure to DMSO, which was used to dissolve BA, the vehicle control was set to 0.3% DMSO. As shown in Figure 2A, all three doses of BA application significantly extended C. elegans life span, and there was no interference from DMSO (Table 2). Notably, BA at 50 μg/mL evoked the maximal effect in extending lifespan; therefore, this optimal dose was kept for all tests down the road.
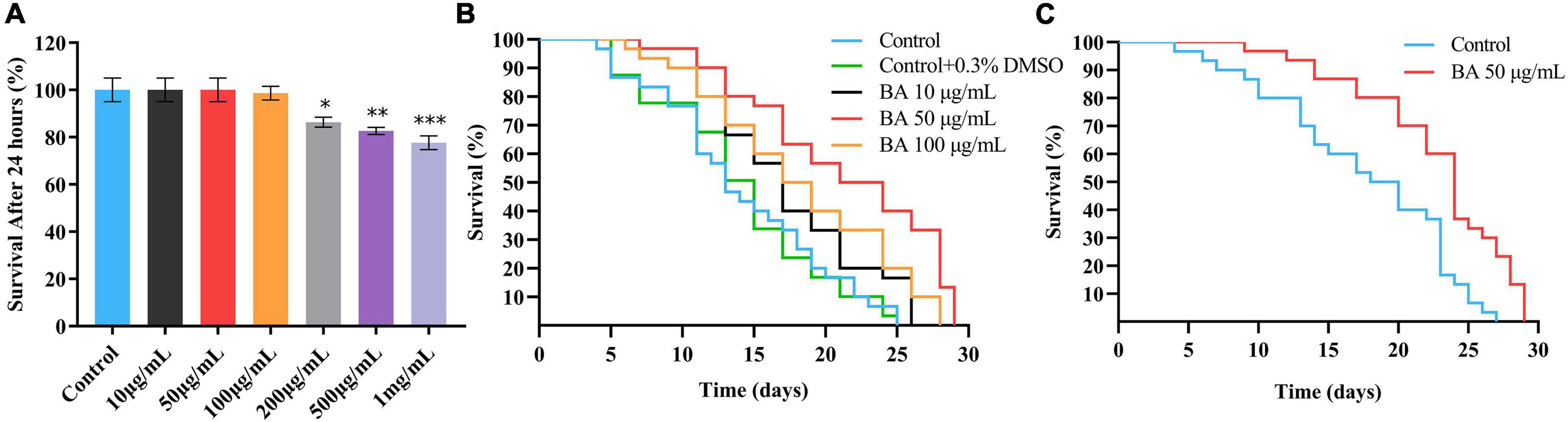
Figure 1. Optimal dose of betulinic acid (BA) extends lifespan of Caenorhabditis elegans. (A) Survival rates of C. elegans after 24 h treatment with different doses of BA (0, 10, 50, 100, 200, 500, and 1,000 μg/mL). (B) Lifespan analysis of C. elegans supplied with indicated BA (10, 50, and 100 μg/mL) or vehicle (0.3% DMSO). (C) Lifespan analysis of C. elegans fed with inactivated Escherichia coli OP50 and BA (50 μg/mL). For panel (A), comparisons represent the One-way ANOVA followed by post-hoc Bonferroni’s test. Data were displayed as mean ± SEM. *P < 0.05; **P < 0.01; ***P < 0.001.
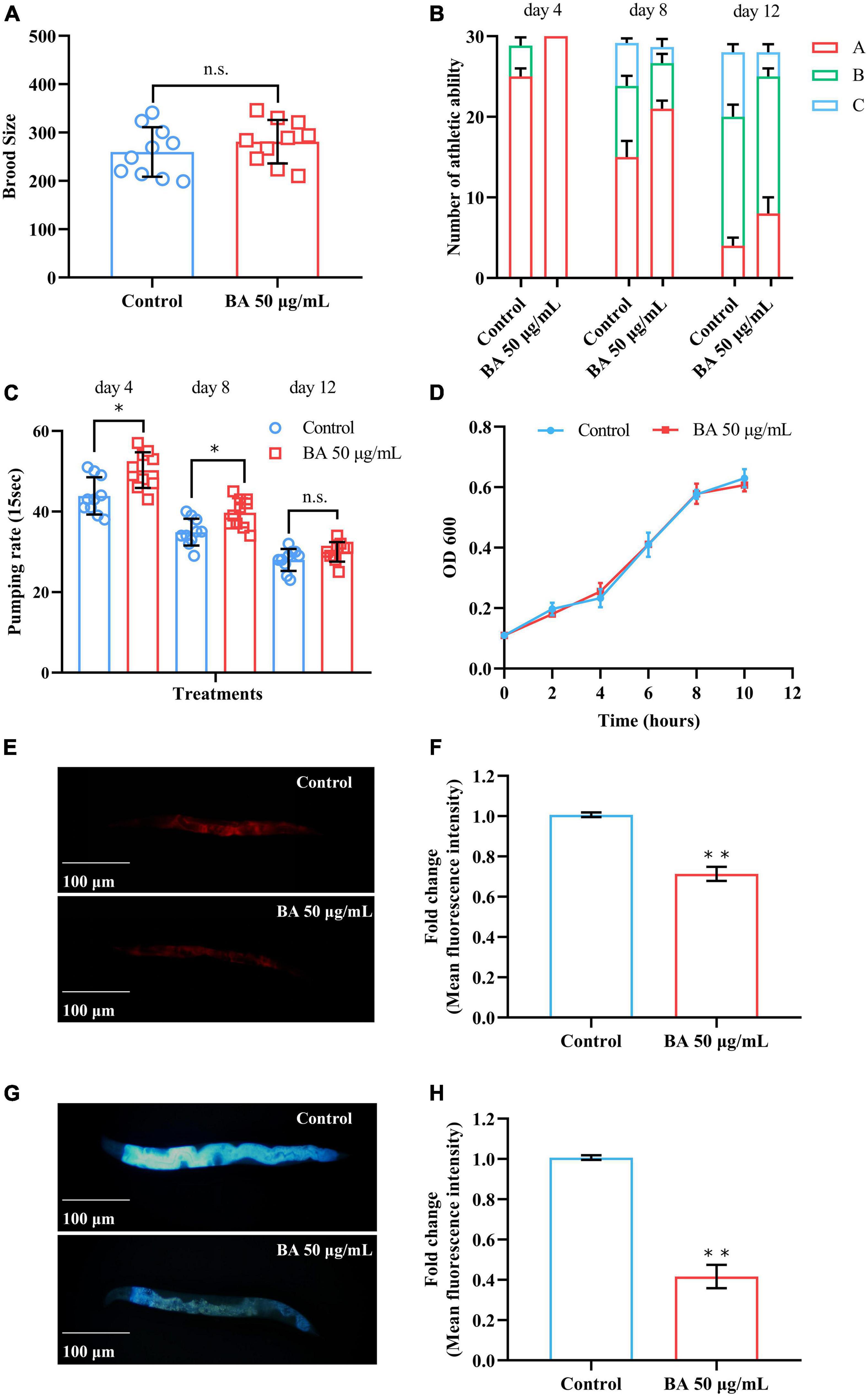
Figure 2. Betulinic acid (BA) delays age-associated physiological decline. (A) Reproductive capacity of Caenorhabditis elegans supplied. (B) Age-dependent motor activity of C. elegans. (C) Pharyngeal pumping rates of C. elegans. (D) Growth rate of Escherichia coli OP50 under 50 μg/mL BA treatment. (E–H) Lipofuscin induced auto-fluorescence under 550/605 nm (Ex/Em) and 355/445 nm (Ex/Em) detection. Data were displayed as mean ± SEM. For panel (C), comparisons represent the One-way ANOVA followed by post-hoc Bonferroni’s test. For panels (A,F,H), comparisons represent two-tailed unpaired Student’s t-test. *P < 0.05; **P < 0.01; ns = nonsignificant.
The lifespan assay requires feeding C. elegans with E. coli OP50, a living organism that may lead to unwanted metabolism of BA and non-specific phenotypes. To eliminate this possibility, the lifespan assay was carried out using the inactivated E. coli OP50, and the lifespan extension phenotype (Figure 1C) could be reproduced. Together, these data demonstrated that BA exhibits the ability to extend lifespan in C. elegans.
Summary of mean lifespan and statistical analysis for Figure 1B. Mean lifespan values were calculated by a log-rank test. P-values were calculated for individual experiments and each consisting of control and treatment groups.
Betulinic acid improves healthspan in Caenorhabditis elegans
In general, aging affects both lifespan and healthspan, which can be measured by the functional decline in performance tests (28). The behavioral and functional phenotypes closely associated with aging were surveyed. The first was reproductive capacity, which has been considered inseparable from the lifespan (29). For anti-aging research, it is important to evaluate the brood size of C. elegans to determine whether BA damage the reproductive ability. As shown in Figure 2A, BA prolonged longevity without sacrificing reproductive capacity. The second phenotype, mobility, reflects the motor ability of animals and declines in the aging process. C. elegans motility was scored on the 4th, 8th, and 12th days according to the three-grade score: A, the animals moved spontaneously and smoothly; B, the animals did not move unless stimulated; C, the animals only wiggled their nose or tail in response to touch. Clearly, C. elegans motility decreased over time while BA application ameliorated this phenotype (Figure 2B).
Some diets and drugs may affect the feeding efficiency of C. elegans, which will lead to a dietary restriction (DR) effect that prolongs their lifespan (30). Therefore, the pharyngeal pumping rates were examined to determine the food intake behavior of C. elegans. Intriguingly, BA application enhanced the pharyngeal pumping rates on the 4th and 8th day. However, there was no significant difference on day 12 (Figure 2C). In light of the potential bacteriostasis of BA, which may cause non-specific dietary restriction, the growth of E. coli OP50 was evaluated, and this scenario was also ruled out (Figure 2D). This data indicated that dietary restriction was not a primary factor in BA anti-aging effect.
Lipofuscin is an auto-fluorescent substance that accumulates during the aging process due to the elevated levels of oxidized proteins in lysosomes (31). Lipofuscin produces red fluorescence that gradually accumulates as C. elegans grows, whereas blue fluorescence occurs before animals die. BA application decreased the intensity of both red and blue fluorescence (Figures 2E–H), consisting of its antioxidant activity from in vitro studies. Together, these results demonstrated that BA promotes healthspan in C. elegans.
Betulinic acid enhances heat stress resistance in Caenorhabditis elegans
Caenorhabditis elegans is extremely sensitive to changes in temperature. It has been proved that higher temperature results in faster senescence. For example, C. elegans life cycle grown at 25°C is 2.1 times shorter than those at 16°C (26). On the other hand, the improvement in C. elegans ability to resist heat stress usually corresponds to an extension of their lifespan. Wild-type animals were subjected to heat stress at 35, 38, and 40°C, and their viability was scored. BA application dramatically enhanced the heat stress resistance in all three conditions and was much more pronounced at high temperatures (Figure 3 and Table 3).
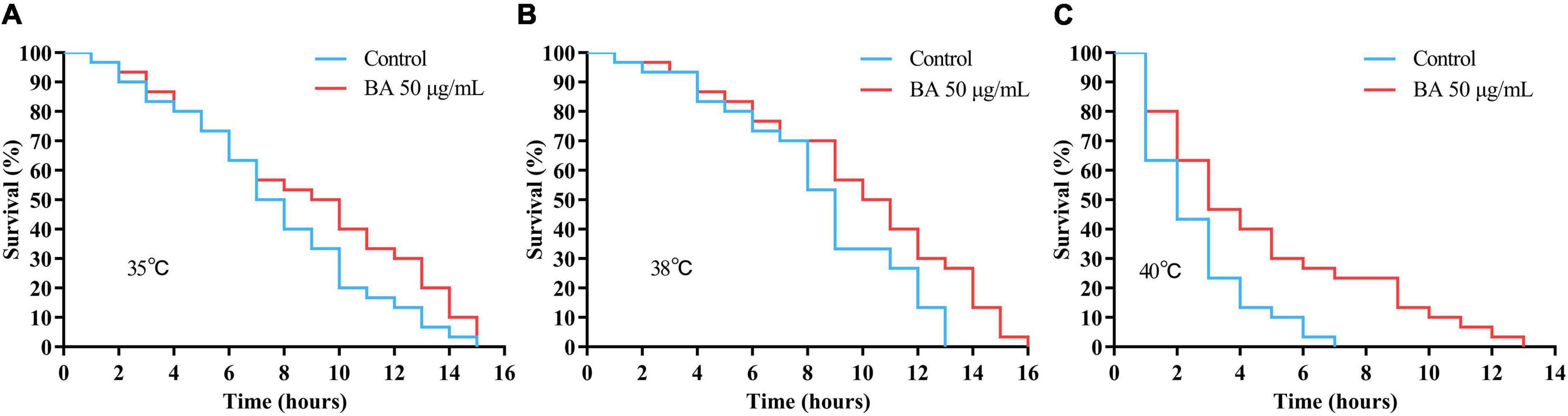
Figure 3. Effects of betulinic acid (BA) on heat stress resistance in Caenorhabditis elegans. Wild-type C. elegans were exposed to heat shock stress as indicated: (A) 35°C; (B) 38°C; (C) 40°C. The protective effects of BA were analyzed by Kaplan-Meier survival curves.
Summary of mean lifespan and statistical analysis for Figure 3. Mean lifespan values were calculated by a log-rank test. P-values were calculated for individual experiments and each consisting of control and treatment groups.
Betulinic acid enhances oxidative stress resistance in Caenorhabditis elegans
The free radical theory of aging is the most consistent across experimental data and model systems. C. elegans advocates that cell and tissue injury by oxidative stress contributes to the aging process. As shown in Figure 4A, juglone, a commonly used oxidative stress inducer (32), led to a quick death within 6 h in C. elegans. However, BA application extended their survival time to 12 h (Table 4), confirming that BA has robust antioxidant activity. Moreover, this was also visualized by detecting the reactive oxygen species (ROS) in the C. elegans body. DCFA-DA staining showed that ROS accumulated rapidly over time, while BA exhibited a consistent inhibitory effect (Figures 4B,C).
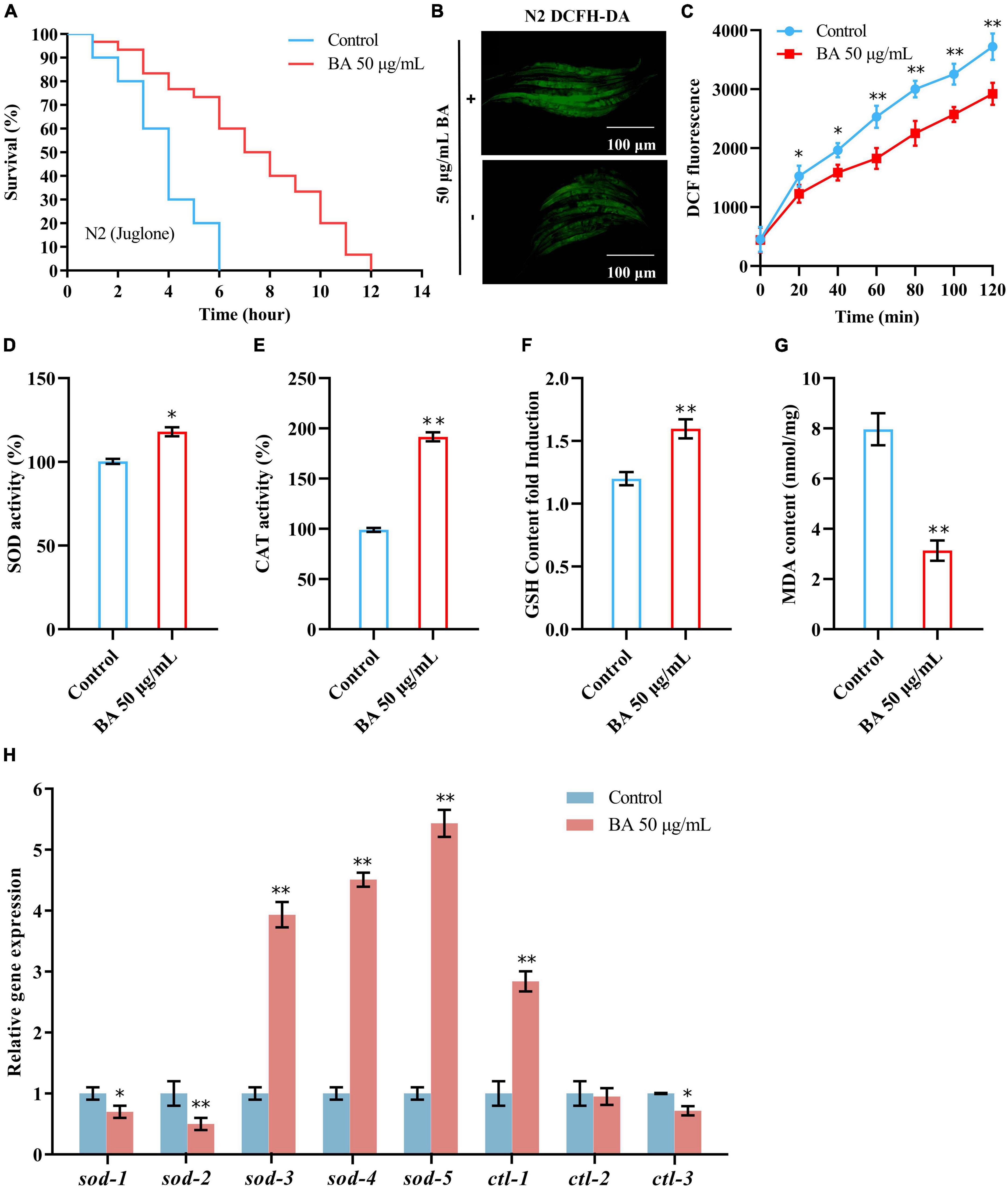
Figure 4. Effects of betulinic acid (BA) on oxidative stress in Caenorhabditis elegans. (A) Effects of BA on juglone-induced oxidative stress. (B,C) BA decreased ROS levels in C. elegans. Representative images of DCFH-DA staining (B) and quantification of fluorescence intensity (C) were presented. (D–G) Levels of lipid oxidation: SOD activity (D), CAT activity (E), MDA content (F), and GSH-Px content (G). (H) RT-qPCR detection of the expression of antioxidant genes. Data were displayed as the mean ± SEM. For panels (C,H), comparisons represent the One-way ANOVA followed by post-hoc Bonferroni’s test. For panels (D–G), comparisons represent two-tailed unpaired Student’s t-test. *P < 0.05; **P < 0.01.
Then, the impacts of BA on antioxidant enzymes were investigated. The activities of SOD, CAT, and GSH-Px were elevated, while the MDA content, a parameter reflecting the lipid oxidation level, decreased (Figures 4D–G). At the mRNA level, a generally similar tendency was observed. 5 SOD genes and three CAT genes were detected, and the expression levels of sod-3, sod-4, sod-5, and ctl-1 were increased dramatically (Figure 4H).
The rest were mildly decreased (sod-1, sod-2, and ctl-3) or unchanged (ctl-2). Together, these data confirmed the robust effect of BA in enhancing oxidative stress resistance.
Summary of mean lifespan and statistical analysis for Figure 4A. Mean lifespan values were calculated by a log-rank test. P-value was calculated for control and treatment group.
Anti-oxidant activity of betulinic acid is dependent on DAF-16/FOXO
To dissect the underlying mechanism of BA action, the classical longevity mutants were utilized for genetic analysis: clk-1, the human coenzyme Q7 homolog and corresponds to the mitochondrial signaling; glp-1, the human notch receptor homolog and corresponds to the reproductive restriction signaling; eat-2, the human α7 nicotinic receptor subunit homolog and corresponds to the dietary restriction signaling; daf-16, the human FOXO homolog and corresponds to the insulin signaling pathways. Juglone-induced oxidative stress experiments were performed, and BA applications were added on top of the above mutants. Interestingly, BA enhanced oxidative stress resistance in the conditions of clk-1, glp-1, and eat-2 (Figures 5A–C). However, this effect was absent when DAF-16 was loss-of-function (Figure 5D). Thus, BA depends on the insulin signaling pathway to mediate its effects against oxidative stress in C. elegans (Table 5)
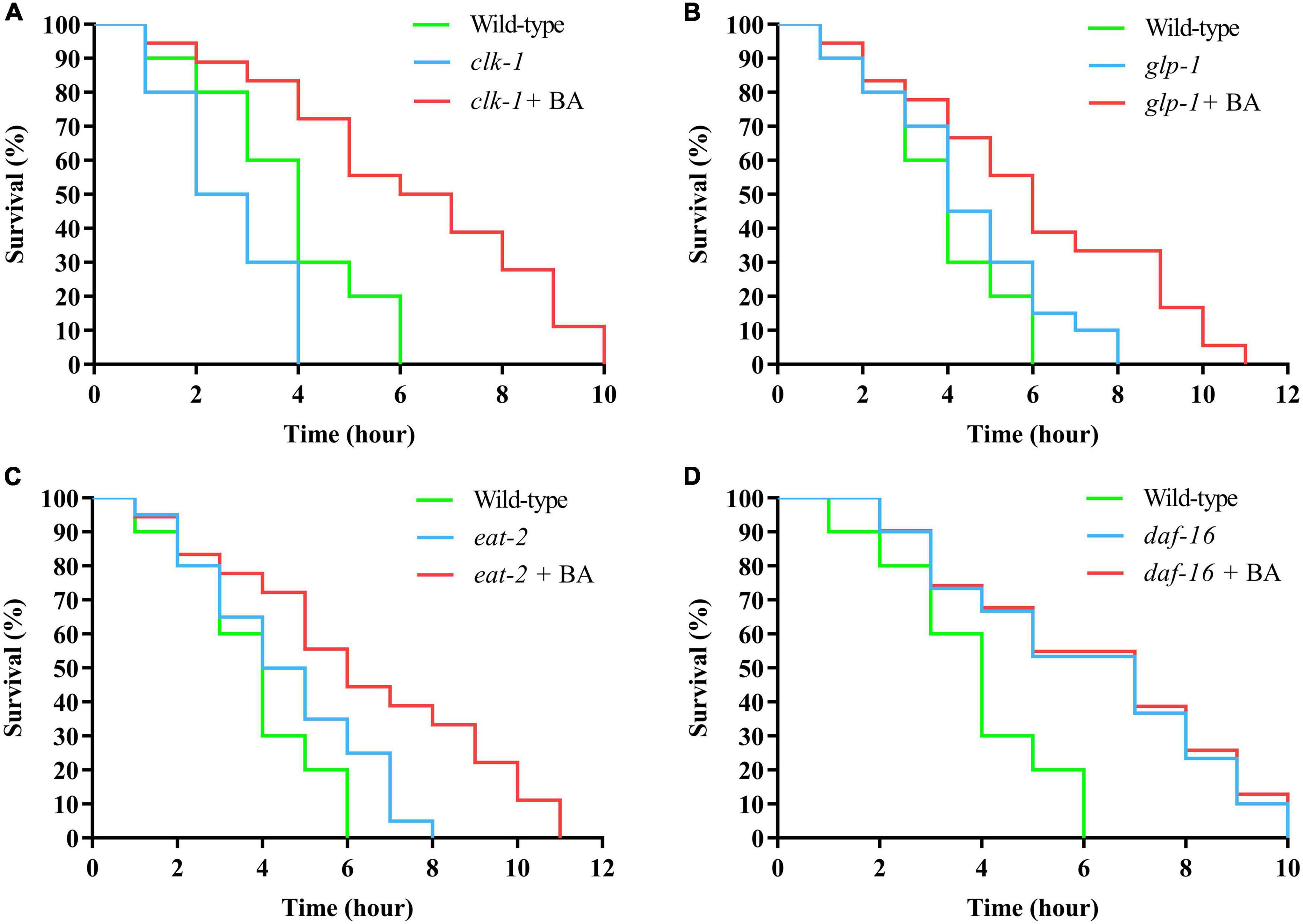
Figure 5. DAF-16 is required for betulinic acid (BA)-induced oxidative stress resistance. The classical longevity mutants, including clk-1 (A), glp-1 (B), eat-2 (C), and daf-16 (D), were tested for juglone-induced oxidative stress. The Kaplan-Meier survival curves were generated to analyze the effects of BA.
Summary of mean lifespan and statistical analysis for Figure 5. Mean lifespan values were calculated by a log-rank test. P-values were calculated for individual experiments and each consisting of wild-type, mutant, and treatment groups.
Insulin/IGF-1 receptor signaling mediates betulinic acid-induced longevity and stress resistance
The DAF-16/FOXO transcription factor is the major downstream output of the insulin/IGF-1 receptor signaling pathway. The RNAi strategy was deployed to evaluate the pivotal role of DAF-16/FOXO in BA-induced longevity and stress resistance. The knock-down efficiency was measured by quantifying the fluorescence intensity of DAF-16:GFP (Figures 6A,B). Consistent with the previous result (Figure 5D), daf-16 RNAi eliminated the prolonged lifespan induced by BA (Figures 6C–F). The target genes of DAF-16, sod-3, ctl-2, and dod-17 and their altered expression induced by BA application were abolished (Figure 6G).
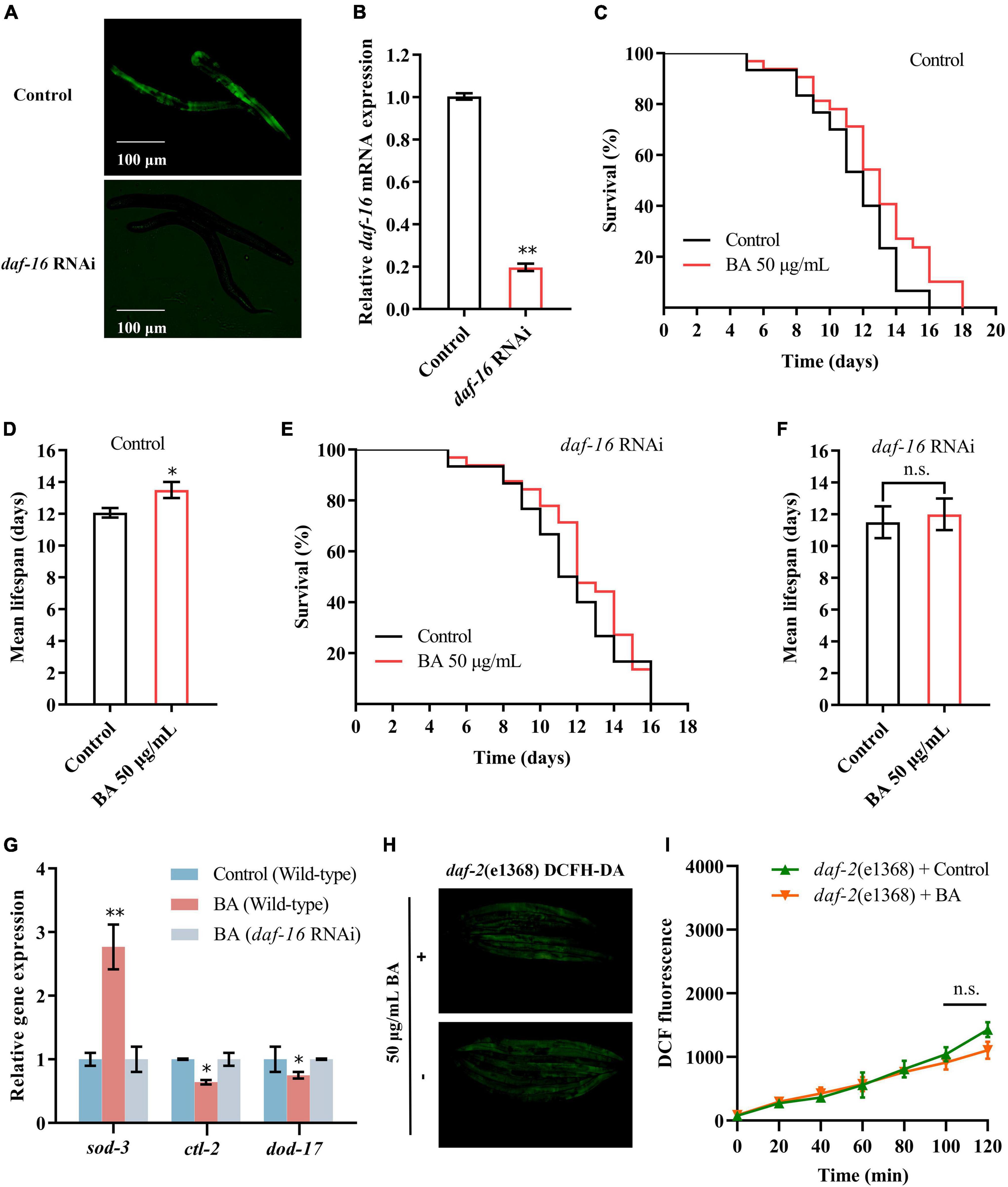
Figure 6. Insulin/IGF-1 receptor signaling mediates betulinic acid (BA)-induced longevity and stress resistance. (A) Evaluation of daf-16 RNAi efficiency using the transgenic Caenorhabditis elegans expressing DAF-16:GFP. (B) RT-qPCR quantification of daf-16 mRNA levels after RNAi. (C,D) Kaplan-Meier survival curve and mean lifespan of BA-induced longevity in wild-type animals. (E,F) Kaplan-Meier survival curve and mean lifespan of BA-induced longevity in daf-16 RNAi animals. (G) Effects of BA on the expression of daf-16 downstream genes (sod-3, ctl-2, and dod-17). (H,I) Effects of BA on ROS level in wild-type animals and daf-2 mutants. Representative images of DCFH-DA staining (H) and quantification of fluorescence intensity (I) were presented. Data were displayed as the mean ± SEM. For panels (G,I), comparisons represent the One-way ANOVA followed by post-hoc Bonferroni’s test. For panels (B,D,F), comparisons represent two-tailed unpaired Student’s t-test. *P < 0.05; **P < 0.01; ns = nonsignificant.
To further validate the involvement of the insulin/IGF-1 receptor signaling, DAF-2, the human IGF-1 receptor homolog in C. elegans, was examined. As shown in Figures 6H,I, there was no difference between the control and BA application in DCFA-DA staining detected ROS levels. Together, insulin/IGF-1 receptor signaling is required for BA-induced longevity and stress resistance.
Discussion
Betulinic acid has a wide range of physiological activities, and numerous efforts have been made to pursue its applications in food and pharmaceutical industries. First of all, the isolation and synthesis of BA have progressed significantly. For example, the current researchers extracted BA from birch bark and achieved a purity of 97.6% (33). As a lupin-type pentacyclic triterpenoid, BA is easily soluble in solvents such as DMSO, pyridine, and tetrahydrofuran but is insoluble in water. It has been known that DMSO is slightly toxic when serving as a vehicle for treating C. elegans (34). Therefore, the dose usage of BA dissolved in DMSO was carefully checked. It was determined that DMSO below 0.3% did not affect the health and growth of C. elegans; furthermore, the optimal dose of BA exhibited the maximal effect in lifespan extension.
The advantages of C. elegans model in aging research have been well-documented (35). As an intact animal, there are multi-dimensional aging-related readouts not only reflecting lifespan but also healthspan. The behaviors, including reproductivity, motility, pharyngeal pumping, and stress resistance, are declined over age. Hence, C. elegans offers a great chance to look for biological and pharmacological interventions in anti-aging. A large set of new regulators and natural compounds were initially found to prevent healthy aging in C. elegans and then further verified in higher models (36–39). Based on C. elegans model, a systemic evaluation was performed to investigate BA effects on healthspan. BA did not affect the reproductive ability or pharyngeal pumping rate; instead, it enhanced motor ability and heat stress resistance remarkably. These are vital signs to indicate BA has the potential to improve healthspan. This assumption was further validated by the in-depth analysis of oxidative stress and ROS.
Reactive oxygen species are highly reactive oxygen molecules that induce genotoxicity and physiological damages that destroy the innate stress resistance mechanisms. It leads to DNA damage, alters gene expression, perturbs cellular signaling, and ultimately results in cell senescence and death (40). The level of ROS in cells is a balance between ROS production by stressors and ROS by antioxidants. Therefore, reduction in ROS levels is inseparable from the regulation of antioxidant enzyme inhibitors such as T-SOD, MDA, CAT, and GSH-Px (41). Notably, BA application evoked the high contents and activities of these enzymes and then suppressed ROS accumulation in various scenarios. These results are consistent with the previous in vivo and in vitro data (42–45). In conclusion, BA exhibited a robust antioxidative effect.
So far, multiple signaling pathways are known to regulate lifespan extension (46). Therefore, the pathway responsible for aging and anti-aging can be screened in C. elegans efficiently (47). The insulin/IGF-1 receptor signaling pathway is a hub that integrates many upstream and downstream factors (48). In search of the molecular pathway mediating BA action, DAF-16/FOXO, the primary output of insulin/IGF-1 receptor signaling in C. elegans, determines BA-induced longevity and stress resistance. The pathway was further validated by the genetic analysis of upstream receptor DAF-2 and downstream targets sod-3, ctl-2, and dod-17. DAF-2, DAF-16, and the insulin/IGF-1 receptor signaling pathway are highly conserved across species. Therefore, it is reasonable to speculate that BA may have similar functions in higher organisms. In fact, BA has been implicated in several insulin/IGF-1 receptor signaling-related phenotypes. For example, BA has a potent insulin secretagogue effect in pancreatic islets (49) and exhibits the anti-diabetic effect on mouse model (50). BA improves insulin sensitivity in metabolic syndrome rats (51), but inhibits insulin/IGF-1 receptor signaling to suppress de novo lipogenesis in HepG2 cells (52). Although the molecular mechanisms remain to be elucidated, the current studies have provided appreciable evidence showing that insulin/IGF-1 receptor signaling plays a pivotal role in BA’s pharmacological activities.
Strengths and limitations
The present study deployed C. elegans model and revealed the anti-aging effect of BA for the first time. The finding that insulin/IGF-1 receptor signaling mediates BA function will reinforce further development of BA in food and pharmaceutical applications.
Two significant limits need to be solved in the future:
1. It is unclear whether BA works through insulin/IGF-1 receptor signaling directly or indirectly.
2. Additional studies in invertebrate and mammalian model organisms are necessary to validate and expand the anti-aging effect of BA.
Conclusion
1. Betulinic acid prolongs the lifespan and improves the healthspan in C. elegans.
2. Betulinic acid has robust antioxidant activity in vivo.
3. The insulin/IGF-1 receptor signaling pathway mediates BA-induced longevity and oxidative stress resistance.
Data availability statement
The original contributions presented in this study are included in the article/supplementary files, further inquiries can be directed to the corresponding author.
Author contributions
RL: conceptualization, methodology, writing—review and editing, and supervision. FZ: data curation and formal analysis. LL: validation and project administration. TH: software and supervision. ZL: writing—review and editing. HC: supervision, project administration, funding acquisition, and writing— review and editing. All authors have read and agreed to the published version of the manuscript.
Funding
This research was funded by the Youth initiative fund project of Changchun Sci-Tech University (grant number: 20220101).
Acknowledgments
We acknowledge the support of Youth initiative fund project of Changchun Sci-Tech University (grant number: 20220101).
Conflict of interest
The authors declare that the research was conducted in the absence of any commercial or financial relationships that could be construed as a potential conflict of interest.
Publisher’s note
All claims expressed in this article are solely those of the authors and do not necessarily represent those of their affiliated organizations, or those of the publisher, the editors and the reviewers. Any product that may be evaluated in this article, or claim that may be made by its manufacturer, is not guaranteed or endorsed by the publisher.
References
1. Li H, Jia J, Yang Z. Mini-mental state examination in elderly Chinese: a population-based normative study. J Alzheimers Dis. (2016) 53:487–96. doi: 10.3233/JAD-160119
2. Birch J, Gil J. Senescence and the SASP: many therapeutic avenues. Genes Dev. (2020) 34:1565–76. doi: 10.1101/gad.343129.120
3. Kapahi P, Chen D, Rogers AN, Katewa SD, Li PW, Thomas EL, et al. With TOR, less is more: a key role for the conserved nutrient-sensing TOR pathway in aging. Cell Metab. (2010) 11:453–65. doi: 10.1016/j.cmet.2010.05.001
4. Imai S, Armstrong CM, Kaeberlein M, Guarente L. Transcriptional silencing and longevity protein Sir2 is an NAD-dependent histone deacetylase. Nature. (2000) 403:795–800. doi: 10.1038/35001622
5. Kenyon C, Chang J, Gensch E, Rudner A, Tabtiang R. A C. elegans mutant that lives twice as long as wild type. Nature. (1993) 366:461–4. doi: 10.1038/366461a0
6. Franceschi C, Campisi J. Chronic infammation (infammaging) and its potential contribution to age-associated diseases. J Gerontol A. (2014) 69:S4–9. doi: 10.1093/gerona/glu057
7. Houtkooper RH, Mouchiroud L, Ryu D, Moullan N, Katsyuba E, Knott G, et al. Mitonuclear protein imbalance as a conserved longevity mechanism. Nature. (2013) 497:451–7. doi: 10.1038/nature12188
8. Campisi J, Kapahi P, Lithgow GJ, Melov S, Newman JC, Verdin E. From discoveries in ageing research to therapeutics for healthy ageing. Nature. (2019) 571:183–92. doi: 10.1038/s41586-019-1365-2
9. Qi Z, Ji H, Le M, Li H, Wieland A, Bauer S, et al. Sulforaphane promotes C. elegans longevity and healthspan via DAF-16/DAF-2 insulin/IGF-1 signaling. Aging. (2021) 13:1649–70. doi: 10.18632/aging.202512
10. Park SJ, Ahmad F, Philp A, Baar K, Williams T, Luo H, et al. Resveratrol ameliorates aging-related metabolic phenotypes by inhibiting cAMP phosphodiesterases. Cell. (2012) 148:421–33. doi: 10.1016/j.cell.2012.01.017
11. Yogeeswari P, Sriram D. Betulinic acid and its derivatives: a review on their biological properties. Curr Med Chem. (2005) 12:657–66. doi: 10.2174/0929867053202214
12. Chen H, Xiao H, Pang J. Parameter optimization and potential bioactivity evaluation of a betulin extract from white birch bark. Plants (Basel). (2020) 9:392. doi: 10.3390/plants9030392
13. Saneja A, Arora D, Kumar R, Dubey RD, Panda AK, Gupta PN. Therapeutic applications of betulinic acid nanoformulations. Ann N Y Acad Sci. (2018) 1421:5–18. doi: 10.1111/nyas.13570
14. Kirstein-Miles J, Morimoto RI. Caenorhabditis elegans as a model system to study intercompartmental proteostasis: interrelation of mitochondrial function, longevity, and neurodegenerative diseases. Dev Dyn. (2010) 239:1529–38. doi: 10.1002/dvdy.22292
15. Hsin H, Kenyon C. Signals from the reproductive system regulate the lifespan of C. elegans. Nature. (1999) 399:362–6. doi: 10.1038/20694
16. An JH, Blackwell TK. SKN-1 links C. elegans mesendodermal specification to a conserved oxidative stress response. Genes Dev. (2003) 17:1882–93. doi: 10.1101/gad.1107803
17. Walker G, Houthoofd K, Vanfleteren JR, Gems D. Dietary restriction in C. elegans: from rate-of-living effects to nutrient sensing pathways. Mech Ageing Dev. (2005) 126:929–37. doi: 10.1016/j.mad.2005.03.014
18. Wang Y. Research progress on extraction, synthesis and pharmacological effects of betulinic acid. Biol Bull. (2020) 55:1–5. doi: 10.1016/j.bmc.2008.09.053
19. Li R, Tao M, Xu T, Huang Y, Zogona D, Pan S, et al. Artemisia selengensis Turcz. leaf extract promotes longevity and stress resistance in Caenorhabditis elegans. J Sci Food Agric. (2022) 102:4532–41. doi: 10.1002/jsfa.11808
20. Pandey S, Phulara SC, Mishra SK, Bajpai R, Kumar A, Niranjan A, et al. Betula utilis extract prolongs life expectancy, protects against amyloid-β toxicity and reduces alpha synuclien in Caenorhabditis elegans via DAF-16 and SKN-1. Comp Biochem Physiol C Toxicol Pharmacol. (2020) 228:108647. doi: 10.1016/j.cbpc.2019.108647
21. Wu D, Cai W, Zhang X, Lan J, Zou L, Chen SJ, et al. Inhibition of PAR-1 delays aging via activating AMPK in C. elegans. Aging (Albany NY). (2020) 12:25700–17. doi: 10.18632/aging.104180
22. Hou L, Wang D, Chen D, Liu Y, Zhang Y, Cheng H, et al. A systems approach to reverse engineer lifespan extension by dietary restriction. Cell Metab. (2016) 23:529–40. doi: 10.1016/j.cmet.2016.02.002
23. Deng Y, Liu H, Huang Q, Tu L, Hu L, Zheng B, et al. Mechanism of longevity extension of Caenorhabditis elegans induced by schizophyllum commune fermented supernatant with added radix puerariae. Front Nutr. (2022) 9:847064. doi: 10.3389/fnut.2022.847064
24. Liu M, Li N, Lu X, Shan S, Gao X, Cao Y, et al. Sweet tea (Rubus Suavissmus S. Lee) polysaccharides promote the longevity of Caenorhabditis elegans through autophagy-dependent insulin and mitochondrial pathways. Int J Biol Macromol. (2022) 207:883–92. doi: 10.1016/j.ijbiomac.2022.03.138
25. Hu R, Zhang Y, Qian W, Leng Y, Long Y, Liu X, et al. Pediococcus acidilactici promotes the longevity of C. elegans by regulating the insulin/IGF-1 and JNK/MAPK signaling, fat accumulation and chloride ion. Front Nutr. (2022) 9:821685. doi: 10.3389/fnut.2022.821685
26. Wang X, Li H, Liu Y, Wu H, Wang H, Jin S, et al. Velvet antler methanol extracts (MEs) protects against oxidative stress in Caenorhabditis elegans by SKN-1. Biomed Pharmacother. (2020) 121:109668. doi: 10.1016/j.biopha.2019.109668
27. Li YF, Li HY, Zhao LH, Wang XH. Effect of anti-oxidant property of short branched-chain fatty acids in Caenorhabditis elegans. Chin J Biochem Mol Biol. (2019) 35:404–12. doi: 10.13865/j.cnki.cjbmb.2019.04.09
28. Guarente L. Aging research-where do we stand and where are we going? Cell. (2014) 159:15–9. doi: 10.1016/j.cell.2014.08.041
29. Ghazi A. Transcriptional networks that mediate signals from reproductive tissues to influence lifespan. Genesis. (2013) 51:1–15. doi: 10.1002/dvg.22345
30. Weir HJ, Yao P, Huynh FK, Escoubas CC, Goncalves RL, Burkewitz K, et al. Dietary restriction and AMPK increase lifespan via mitochondrial network and peroxisome remodeling. Cell Metab. (2017) 26:884–96.e5. doi: 10.1016/j.cmet.2017.09.024
31. Takeishi A, Takagaki N, Kuhara A. Temperature signaling underlying thermotaxis and cold tolerance in Caenorhabditis elegans. J Neurogenet. (2020) 34:351–62. doi: 10.1080/01677063.2020.1734001
32. Kim B, Lee J, Kim Y, Lee SV. Regulatory systems that mediate the effects of temperature on the lifespan of Caenorhabditis elegans. J Neurogenet. (2020) 34:518–26. doi: 10.1080/01677063.2020.1781849
33. Cui PC. Optimization of Betulin Alcohol Extraction Process and its Intervention on Lipid Metabolism in Mice With High-Fat Diet. Changchun: Jilin Agricultural University (2020).
34. Wang X, Wang X, Li L, Wang D. Lifespan extension in Caenorhabditis elegans by DMSO is dependent on sir-2.1 and daf-16. Biochem Biophys Res Commun. (2010) 400:613–8. doi: 10.1016/j.bbrc.2010.08.113
35. Uno M, Nishida E. Lifespan-regulating genes in C. elegans. NPJ Aging Mech Dis. (2016) 2:16010. doi: 10.1038/npjamd.2016.10
36. Yuan J, Chang SY, Yin SG, Liu ZY, Cheng X, Liu XJ, et al. Two conserved epigenetic regulators prevent healthy ageing. Nature. (2020) 579:118–22. doi: 10.1038/s41586-020-2037-y
37. Yin JA, Gao G, Liu XJ, Hao ZQ, Li K, Kang XL, et al. Genetic variation in glia-neuron signalling modulates aging rate. Nature. (2017) 551:198–203. doi: 10.1038/nature24463
38. Zhou JB, Zheng YL, Zeng YX, Wang JW, Pei Z, Pang JY. Marine derived xyloketal derivatives exhibit anti-stress and anti-ageing effects through HSF pathway in Caenorhabditis elegans. Eur J Med Chem. (2018) 148:63–72. doi: 10.1016/j.ejmech.2018.02.028
39. Jattujan P, Chalorak P, Siangcham T, Sangpairoj K, Nobsathian S, Poomtong T, et al. Holothuria scabra extracts possess anti-oxidant activity and promote stress resistance and lifespan extension in Caenorhabditis elegans. Exp Gerontol. (2018) 110:158–71. doi: 10.1016/j.exger.2018.06.006
40. Bhattacharjee S. Reactive Oxygen Species in Plant Biology. New Delhi: Springer (2019). p. 107–25. doi: 10.1007/978-81-322-3941-3
41. Ghezzi P, Mooradian AD. Demystifying oxidative stress. Handb Exp Pharmacol. (2021) 264:3–26. doi: 10.1007/164_2020_379
42. Yi J, Xia W, Wu J, Yuan L, Wu J, Tu D, et al. Betulinic acid prevents alcohol-induced liver damage by improving the antioxidant system in mice. J Vet Sci. (2014) 15:141–8. doi: 10.4142/jvs.2014.15.1.141
43. Yi J, Zhu R, Wu J, Wu J, Xia W, Zhu L, et al. In vivo protective effect of betulinic acid on dexamethasone-induced thymocyte apoptosis by reducing oxidative stress. Pharmacol Rep. (2016) 68:95–100. doi: 10.1016/j.pharep.2015.07.003
44. Deng G, Ma C, Zhao H, Zhang S, Liu J, Liu F, et al. Anti-edema and antioxidant combination therapy for ischemic stroke via glyburide-loaded betulinic acid nanoparticles. Theranostics. (2019) 9:6991–7002. doi: 10.7150/thno.35791
45. Ríos JL, Máñez S. New pharmacological opportunities for betulinic acid. Planta Med. (2018) 84:8–19. doi: 10.1055/s-0043-123472
46. Wong SQ, Kumar AV, Mills J, Lapierre LR. Autophagy in aging and longevity. Hum Genet. (2020) 139:277–90. doi: 10.1007/s00439-019-02031-7
47. Taormina G, Ferrante F, Vieni S, Grassi N, Russo A, Mirisola MG. Longevity: lesson from model organisms. Genes (Basel). (2019) 10:518. doi: 10.3390/genes10070518
48. Balaji V, Pokrzywa W, Hoppe T. Ubiquitylation pathways in insulin signaling and organismal homeostasis. Bioessays. (2018) 40:e1700223. doi: 10.1002/bies.201700223
49. Sacks D, Baxter B, Campbell C, Carpenter J, Cognard C, Dippel D, et al. Multisociety consensus quality improvement revised consensus statement for endovascular therapy of acute ischemic stroke. Int J Stroke. (2018) 13:612–32. doi: 10.1177/1747493018778713
50. Ahangarpour A, Oroojan AA, Khorsandi L, Shabani R, Mojaddami S. Preventive effects of betulinic acid on streptozotocinnicotinamide induced diabetic nephropathy in male mouse. J Nephropathol. (2016) 5:128–33. doi: 10.15171/jnp.2016.24
51. Dasgupta Y, Golovine K, Nieborowska-Skorska M, Luo L, Matlawska-Wasowska K, Mullighan CG, et al. Drugging DNA repair to target T-ALL cells. Leuk Lymphoma. (2018) 59:1746–9. doi: 10.1080/10428194.2017.1397662
Keywords: betulinic acid, C. elegans, lifespan, stress resistance, DAF-16
Citation: Chen H, Li R, Zhao F, Luan L, Han T and Li Z (2022) Betulinic acid increases lifespan and stress resistance via insulin/IGF-1 signaling pathway in Caenorhabditis elegans. Front. Nutr. 9:960239. doi: 10.3389/fnut.2022.960239
Received: 02 June 2022; Accepted: 13 July 2022;
Published: 29 July 2022.
Edited by:
A. M. Abd El-Aty, Cairo University, EgyptCopyright © 2022 Chen, Li, Zhao, Luan, Han and Li. This is an open-access article distributed under the terms of the Creative Commons Attribution License (CC BY). The use, distribution or reproduction in other forums is permitted, provided the original author(s) and the copyright owner(s) are credited and that the original publication in this journal is cited, in accordance with accepted academic practice. No use, distribution or reproduction is permitted which does not comply with these terms.
*Correspondence: Haiyan Chen, MTAwMzYxQGNzdHUuZWR1LmNu
†These authors have contributed equally to this work