- 1Laboratory of Aquatic Nutrition and Feed, College of Fisheries, Guangdong Ocean University, Zhanjiang, China
- 2Yichang Huatai Biological Technology Co., Ltd., Yichang, China
- 3Aquatic Animals Precision Nutrition and High Efficiency Feed Engineering Research Center of Guangdong Province, Zhanjiang, China
- 4Southern Marine Science and Engineering Guangdong Laboratory, Zhanjiang, China
Background: At present, fish meal (FM) resources are in short supply, and competition for food between humans and animals is becoming increasingly critical. Finding non-grain protein sources that can replace FM is the key to solving the rapid development of aquaculture.
Methods: Seven trial diets were prepared with 0 g/kg (EP0), 30 g/kg (EP3), 60 g/kg (EP6), 90 g/kg (EP9), 120 g/kg (EP12), 150 g/kg (EP15), and 180 g/kg (EP18) of enzyme-digested poultry by-product meal (EPBM) by replacing of FM. A total of 630 hybrid groupers (Epinephelus fuscoguttatus ♀ × E. lanceolatus ♂) were equally portioned into 21 tanks. At 8:00 and 16:00 each day, groupers were fed until they were full for a cumulative period of 8 weeks.
Results: The results showed that 30 g/kg of EPBM significantly increased the rates of weight gain and special growth (P < 0.05). Significantly higher activities of serum glutamic pyruvic transaminase, glutamic oxaloacetic transaminase, catalase, and superoxide dismutase were observed in the EP3 group (P < 0.05). The categories and numbers of the top 10 dominant bacteria in the phylum and genus levels were not significantly influenced by feed (P > 0.05). In the proximal intestine and distal intestine, there were significantly higher expressions of SNAT3, LAAT1, CAT2, and CAT1 in the EP3 group compared with the EP0 group (P < 0.05). In the EP3 group, the expressions of PepT1, LAAT1, B0, +AT, and CAT2 were significantly increased in MI than those in all other groups (except the EP0 group, P < 0.05).
Conclusion: When FM was replaced by 30 g/kg of EPBM, growth performance, antioxidant capacity, and the ability to transport amino acids and peptides of hybrid grouper were significantly improved.
Introduction
Fish meal (FM) is considered a high-quality feed material for aquatic animals due to its balanced amino acid composition, high protein content, rich in unsaturated fatty acids, and absence of anti-nutritional factors (1). The rapid expansion of the global aquaculture industry and the long-term transitional fishing of fisheries have led to a decrease in FM production year by year (2). The price of FM is rising, which severely restricted sustainable aquaculture development (3). In the past 10 years, replacing FM with alternative protein ingredients in aqua-feed has been recognized as a way (4) for the successful reduction in FM content in feed by using less expensive vegetable protein (5, 6). However, the absence of some essential amino acids (7), the high level of anti-nutritional factors (8), and the low digestibility (9) of plant protein resources have prevented their extensive use in feeds (10–12). Therefore, finding a cost-effective, safe, and healthy alternative source of protein has become one of the effective ways to solve the FM shortage problem.
Poultry by-product meal (PBM) is an important source of animal protein produced by the by-products produced (muscles of the tibia) during poultry processing through cooking, pressing, drying, and pulverizing. With the exception of methionine and lysine, PBM has a similar composition of amino acids to FM, has a high protein content, and is a valuable feed ingredient (13). Studies have reported that the use of moderate amounts of PBM replacement in aquafeeds achieved positive results on gilthead seabream (Sparus aurata) (14), striped bass (Morone chrysops × M. saxatilis) (15), cuneate drum (Nibea miichthioides) (16), cobia (Rachycentron canadum) (17), and turbot (Scophthalmus maeoticus) (18). However, a higher proportion of PBM replacing FM could reduce the growth performance (19, 20). The main problem with PBM replacing FM is the lack of restrictive amino acids and poor palatability (21). Compared with FM, PBM, lacking lysine and methionine, can affect its amino acid utilization and then inhibit fish growth (13). The replacement of FM with PBM can reduce the palatability of the feed, and it is the main reason for the significant reduction in the growth of fish (22, 23).
Further refinement of the PBM (such as enzyme digestion) can enhance nutrient availability and optimize the nutritional structure (24). The enzyme-digested poultry by-product meal (EPBM) was enriched with various peptides and free amino acids (25). The absorption of small peptides has the characteristics of fast transfer speed, low energy consumption, and difficulty to saturate the carrier (26). In addition, small peptides can be directly absorbed to avoid competition with amino acids, promote protein synthesis (27), accelerate the absorption of mineral elements (28), and improve the palatability of feeds (29), which can greatly improve economic benefits.
Grouper production, ranking third in marine cultured fish, reached 159,579 tons in 2021 (30). In China, the grouper (Epinephelus fuscoguttatus ♀ × E. lanceolatus ♂) is a marine fish of economic importance (31). The research into hybrid grouper has received a great deal of attention due to its advantages of growth performance, survival rate, and delicious taste (32). Therefore, this experiment investigated the effects of EPBM on growth, intestinal microbiota and morphology, non-specific immunity, and expression of amino acid and peptide transporters in hybrid grouper.
Materials and methods
Experimental diets
Seven trial diets (Table 1) were prepared with 0 g/kg (EP0), 30 g/kg (EP3), 60 g/kg (EP6), 90 g/kg (EP9), 120 g/kg (EP12), 150 g/kg (EP15), and 180 g/kg (EP18) of EPBM. The ingredients were crushed, weighed, and mixed thoroughly. The 3-mm diameter pellets were made using a twin-screw extruder (MY56 × 2A). The pellets were dehydrated at 25°C for approximately 36 h, at which point the moisture contents of the pellets were around 100 g/kg. The feed pellets were stored at −20°C.
Feeding trial
Hybrid groupers were purchased from the Zhanjiang grouper fry farm of Guangdong province, China. The groupers were domesticated for a fortnight to adapt to the experimental environment. During this period, industrial feeds with 500 g/kg crude protein were used to feed groupers. A total of 630 hybrid groupers (7.50 ± 0.02 g) were randomly divided into 7 groups, with three tanks (300 L) per group and 30 groupers in each tank. At 8:00 and 16:00 each day, groupers were fed until they were full for a cumulative period of 8 weeks. To ensure a consistent environment, each tank was replaced with approximately 210 L of water per day. During the experimental period, pH was 6.8–7.3, salinity was 28–31 g/L, the temperature range was 29.0–31.0°C, dissolved oxygen was not less than 5.0 mg/L, and ammonia nitrogen was not above 0.06 mg/L. All groupers and caretaking procedures were approved by the Institutional Animal Care and Use Committee of Guangdong Ocean University (Zhanjiang, China), in accordance with NIH guidelines (NIH Pub. No. 85 to 23, revised 1996).
Sample collection and analysis
To obtain basal body metabolism, a 24-h fast was imposed on all groupers at the end of the 8-week culture experiment. MS-222 (1:10,000) was used to anesthetize groupers prior to sample collection. Groupers were weighed and counted to calculate survival rate (SR), feed coefficient rate (FCR), intaking feed rate (IFR), special growth rate (SGR), protein efficiency rate (PER), and weight gain rate (WGR). Three groupers were randomly selected, weighed, and measured their length to determine condition factor (CF), hepatopancreas and viscera were taken and weighed to determine the hepatopancreas somatic indices (HSI), and viscera somatic index (VSI). Three fish from each tank were used to determine the nutrient composition and to calculate the deposition rate of lipid and protein (LDR and PDR).
The AOAC (33) was used for analyzing whole-body and feed nutrient composition. The moisture content was determined by weight to a constant weight. Determination of ether extract (EE) by constant weight was performed after ether extraction. The Kjeldahl method was used in the analysis of crude protein (CP). The distribution of peptide molecular weight of EPBM (Figure 1) was analyzed using the national standard method (GB/T 22729-2008). During the feeding trial, after the groupers had been feeding for 4 h, feces were collected and used to calculate apparent digestibility coefficients for phosphorus (ADC P), calcium (ADC Ca), crude protein (ADC CP), and dry matter (ADC DM).
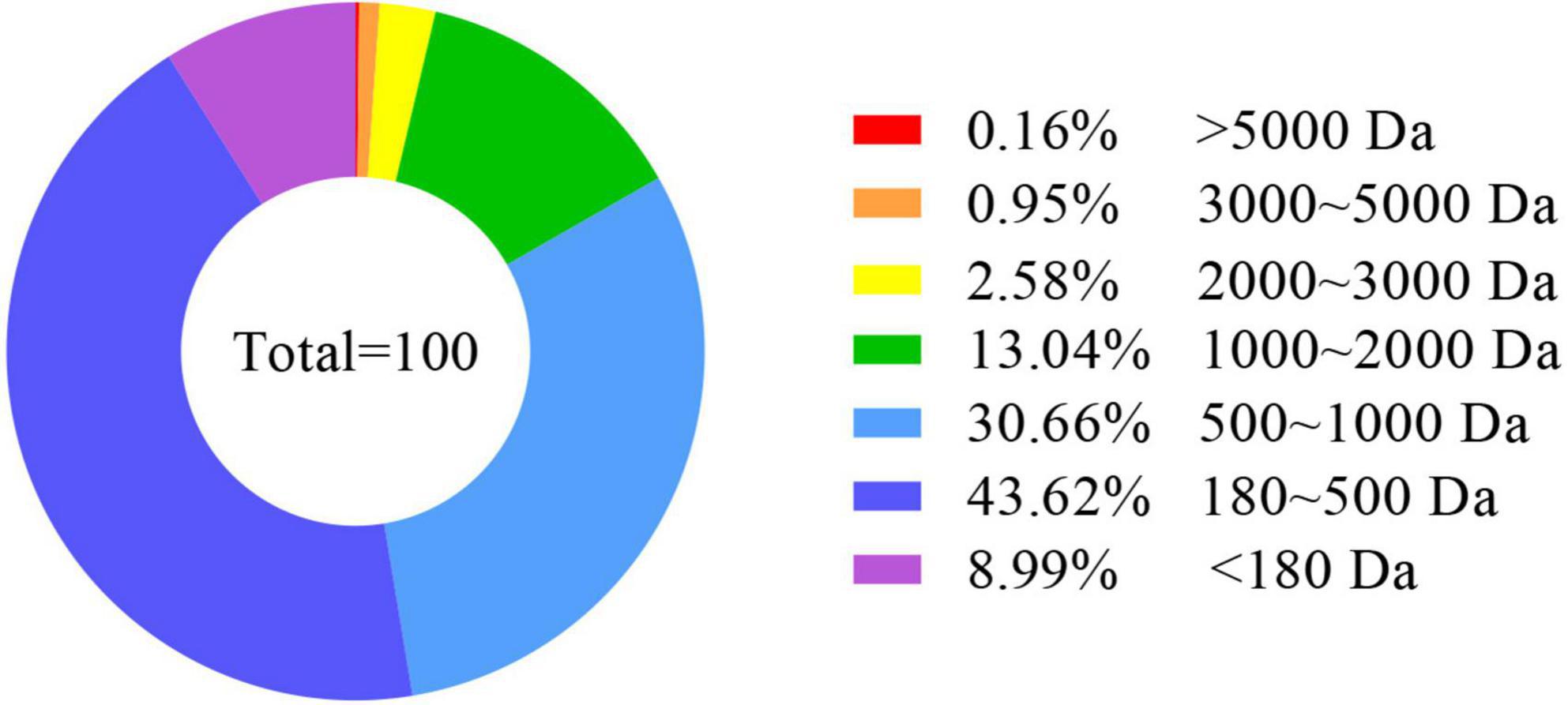
Figure 1. Peptide molecular weight distribution of enzyme-digested poultry by-product meal. Da, Dalton.
A 1-ml sterile syringe was used to collect blood samples from the spine of three groupers in each tank. After storage at 4°C for 12 h, the serum was collected by centrifugation (4,000 g) at 4°C for 15 min. The kit developed by Nanjing Jiancheng Bioengineering Institute (Nanjing, China) was used to measure total cholesterol (CHOL), total triglyceride (TG), glucose (GLU), albumin (Alb), total protein (TP), acid phosphatase (ACP), alkaline phosphatase (AKP), glutamic pyruvic transaminase (GPT), catalase (CAT), glutamic oxaloacetic transaminase (GOT), and superoxide dismutase (SOD) in serum.
Three fish from each tank were used to collect the distal intestine (DI), mid-intestine (MI), and proximal intestine (PI), and they were preserved in paraformaldehyde solution. The samples were dyed with hematoxylin and eosin, sectioned, and measured for muscle thickness (MT), villus width (VW), villus length (VL), crypt depth (CD), and the value of VL/CD.
The livers and intestines of three fish in each tank were collected. They were kept at −20°C for testing the enzyme activities. After wiping the outer skin of the intestines of three groupers in each tank with 75% alcohol, the intestines were placed at −80°C for the detection of microbial community. The PI, MI, and DI of three fish in each tank were stocked in RNA-later (Ambion, United States), and posteriorly placed at −80°C to analyze the expression of mRNA.
Intestinal microbiota
The NucleoSpin® Soil kits (Macherey-Nagel, Germany) were used to extract the bacterial DNA. The V3 + V4 of bacterial 16S rDNA genes were amplified by using universal primers. Amplification reactions were performed in a system containing 5 μL of KOD FX Neo Buffer, 2 μL of dNTP, 0.2 μL of KOD FX Neo, 0.3 μL of each primer, 50 ng bacterial DNA, and sterilized double-distilled water. The target region PCR was carried out at 95°C for 5 min; then 25 cycles of 30 s at 95°C, 30 s at 50°C, and 40 s at 72°C; and 72°C for 7 min. The Solexa PCR was performed in a system containing 10 μL of 2 × Q5 HF MM, 5 μL of target region PCR, and 2.5 μL of MPPI-a and MPPI-b, separately. The Solexa PCR was performed for 30 s at 98°C; then 10 cycles at 98°C for 10 s, 65°C for 30 s, and 72°C for 30 s; and lastly 5 min at 72°C. Following purification of the amplification products, sequencing and construction were performed on the sequencing platform. Sequencing results were classified into operational taxonomic units (OTUs). Based on relative abundance per OTU and the total number of OTUs, the alpha diversity index (such as abundance-based coverage estimator, ACE; Chao1; Shannon index; Simpson index; and Good’s coverage) and beta diversity index (such as analysis of similarities, ANOSIM; and principal coordinates analysis, PCoA) were calculated using Mothur (version 1.3.0) and QIIME (v. 1.7.0), respectively. Detections of differentially marked species were analyzed using line discriminant analysis effect size (LEfSe).
Relative mRNA expression
The mRNA expression of oligopeptide transporter (PepT1), amino acid transporter of B0,+-type (B0, +AT, SLC6A19), sodium-coupled neutral amino acid transporter 3 (SNAT3, SLC38A3), excitatory amino acid transporter 1 (EAAT1, SLC1A3), sodium-dependent neutral amino acid transporter B0AT2-like (B0AT2, SLC6A15), cationic amino acid transporter 2 (CAT2, SLC7A2), proton-coupled amino acid transporter 1 (PAT1, SLC36A1), lysosomal amino acid transporter 1 (LAAT1, SLC38A9), and cationic amino acid transporter 1 (CAT1, SLC7A1) in the intestines were tested. These are shown in Table 2, which lists the sequences of exon boundary-spanning primers for the genes. The β-actin gene was used as a housekeeping gene, and the control group was used as the reference group. TRIzol reagent (Beijing Trans Gen Biotech Co., Ltd., China) was designed to produce total RNA from PI, MI, and DI. The DNA pollution in the RNA extract was removed by Recombinant DNase I (Takara, Japan). High purity of the RNA extract was indicated when the absorbance ratio at 260/280 nm on the spectrophotometer was 1.86 to 2.00. The Script™ reagent Kit (Takara, Japan) was used to reversely transcribed cDNA. The Premix Taq™ II Kit (Takara, Japan) was utilized for quantitative real-time PCR (qRT-PCR). The qRT-PCR was performed in a 10-μL volume with 3.2 μL of sterilized double-distilled water, 0.4 μL of each primer, 1 μL of cDNA, and 5 μL of Master Mix (Bio-Rad Labs). The qRT-PCR was performed under the procedure of Yang et al. (34). According to the method of Mu et al. (35) for 2–Δ Δ CT, expressions of genes were analyzed.
Statistics and calculations
The following formula was used to calculate growth performance:
Intaking feed rate (IFR,%/d) = Wd/(t × (Wf + Wi)/2) × 100
Protein efficiency rate (PER) = (Wf - Wi)/Wf × CPd
Special growth rate (SGR,%/d) = [ln(Wf) -ln(Wi)]/t × 100
Weight gain rate (WGR,%) = (Wf - Wi)/Wi × 100
Feed coefficient rate (FCR) = Wd/(Wf - Wi)
Survival rate (SR,%) = Nf/Ni × 100
Condition factor (CF,%) = Wb/(Lb)3 × 100
Hepatopancreas somatic indices (HSI,%) = 100 × Wh/Wb
Viscera somatic index (VSI,%) = 100 × Wv/Wb
Protein deposition rate (PDR,%) = (Wf × CPf – Wi × CPi)/(Wd × CPd) × 100
Lipid deposition rate (LDR,%) = (Wf × EEf – Wi × EEi)/Wd × EEd × 100
Apparent digestibility coefficient of dry matter (ADCDM,%) = (1 – (Yd/Yf)) × 100
Apparent digestibility coefficient of nutrients (ADCN,%) = (1 – (Nff/Nd) × (Yd/Yf)) × 100
where t is the days of the feeding experiment, Wi (g) is the mean initial body weight, Wf (g) is the mean final body weight, Wd (g) is the diet weight of each groups fish intaking (dry weight), CPd (%) is the percentage of crude protein of diet, Ni is the fish amount of initial test, Nf is the fish amount of final test, Lb (cm) is the body length of the single fish, Wb (g) is the body weight of the single fish, Wv (g) is the viscera weight of the single fish, Wh (g) is the hepatopancreas weight of the single fish, CPf (%) is the percentage of crude protein of fish body of final test, CPi (%) is the percentage of crude protein of fish body of initial test, EEf (%) is the percentage of ether extract of fish body of final test, EEi (%) is the percentage of ether extract of fish body of initial test, EEd (%) is the percentage of ether extract of diet, Yd (%) is the percentage of Y2O3 of diet, Yf (%) is the percentage of Y2O3 of feces, Nff (%) is the percentage of nutrients of feces, and Nd (%) is the percentage of nutrients of diet.
An ANOVA (one-way analysis of variance) was used for the statistical analysis of all original data. Comparison of means among treatments using Tukey’s test. SPSS 22.0 was used to conduct all statistical analyses. The expression of all data as means ± SEM. When P-values were <0.05, the difference was considered to be significant. Different letters indicate significant differences among groups (a > b > c > d).
Results
Growth performance
The FBW, WGR, SGR, and HSI in the EP3 group were significantly better than those in the EP0, EP12, EP15, and EP18 groups (P < 0.05). With the increase in EPBM content, PER was gradually decreased and was the lowest in the EP18 group (P < 0.05). The trend of FCR and IFR was opposite to that of PER. The FCR and IFR were significantly higher in the EP18 group than that in the EP0, EP3, and EP6 groups (P < 0.05). The LDR showed a maximum value when the feed was supplemented with EPBM at a level of 60 g/kg, which was significantly higher than the group with EPBM additions of 90–180 g/kg (P < 0.05). The SR, CF, VSI, and PDR were not significantly influenced by diet (P > 0.05; Table 3).
Apparent digestibility coefficient
The ADC DM in the EP6 group, the ADC CP in the EP0 group, and the ADC Ca in the EP3 group were considerably larger than those in the EP9, EP15, and EP18 groups (P < 0.05; Table 3). Compared with the EP0 group, the ADC P was substantially higher in the EP12 group (P < 0.05).
Whole-body composition
In the EP12 group, CP content was significantly best, except in the EP18 group (P < 0.05). The EE content in the EP6 group was considerably higher than that in the EP9 and EP18 groups (P < 0.05). Lower ash content in the group uses EPBM instead of FM compared to the EP0 group (P < 0.05). The moisture was not significantly impacted by the experimental diet (P > 0.05; Table 4).
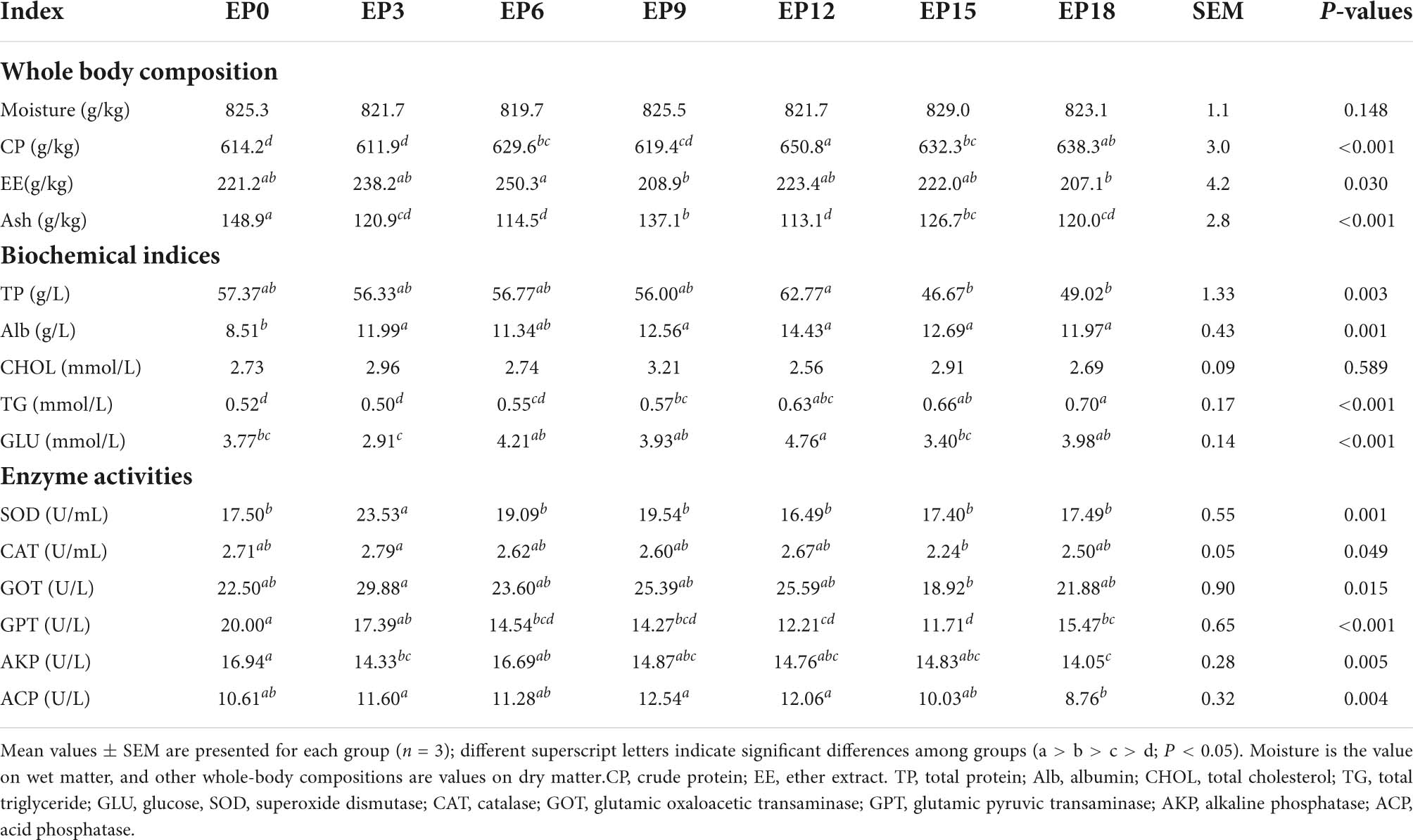
Table 4. Whole-body composition, serum biochemical indices, and enzyme activities of hybrid groupers fed with diets.
Serum biochemical indices
There was no considerable variation in CHOL (P > 0.05; Table 4). The EPBM substitution of FM resulted in significantly higher serum Alb levels in grouper (P < 0.05, except in the EP6 group). As the proportion of FM was replaced by EPBM increased, the TG content gradually increased, with a maximum value in the EP18 group (P < 0.05). The TP and GLU content in the EP12 group was substantially greater than that in the EP15 group (P < 0.05).
Enzyme activity index in serum
The CAT, GOT, and SOD activities in the EP3 group were prominently greater than in the EP15 group (P < 0.05; Table 4). As the proportion of FM replaced by EPBM was increased, GPT and AKP activity was significantly decreased (P < 0.05). ACP activities were markedly greater in the EP3, EP9, and EP12 groups than those in the EP18 group (P < 0.05).
Digestive enzyme activities
In the intestine, the chymotrypsin, lipase, and trypsin activities were prominently increased in the EP12 and EP15 groups compared to the EP0 group (P < 0.05). Amylase activities in the EP12 and EP18 groups were prominently increased than those in the EP6 group (P < 0.05). In the liver, compared with the EP0 group, the chymotrypsin and amylase activities were substantially increased by adding 120 g/kg of EPBM (P < 0.05), with significantly greater activity of trypsin in the EP3 group than that in the EP0 group (P < 0.05). The lipase was not significantly different (P > 0.05; Table 5).
Intestinal morphology
There was no obvious difference in the MT of PI, VW, MT, and VL/CD value of MI, as well as VL and VW of DI (P > 0.05; Figure 2 and Table 6). The MT and CD of DI, as well as VW and VL of PI in the EP15 group, were substantially greater than that in the EP0 and EP3 groups (P < 0.05). The VL and CD of MI were significantly higher in the EP18 group (P < 0.05). The CD of DI in the EP18 group was significantly higher than that in all other groups, except for the EP15 group (P < 0.05). The VL/CD values of PI and DI in the EP3 group were significantly greater than those in the EP15 group (P < 0.05).
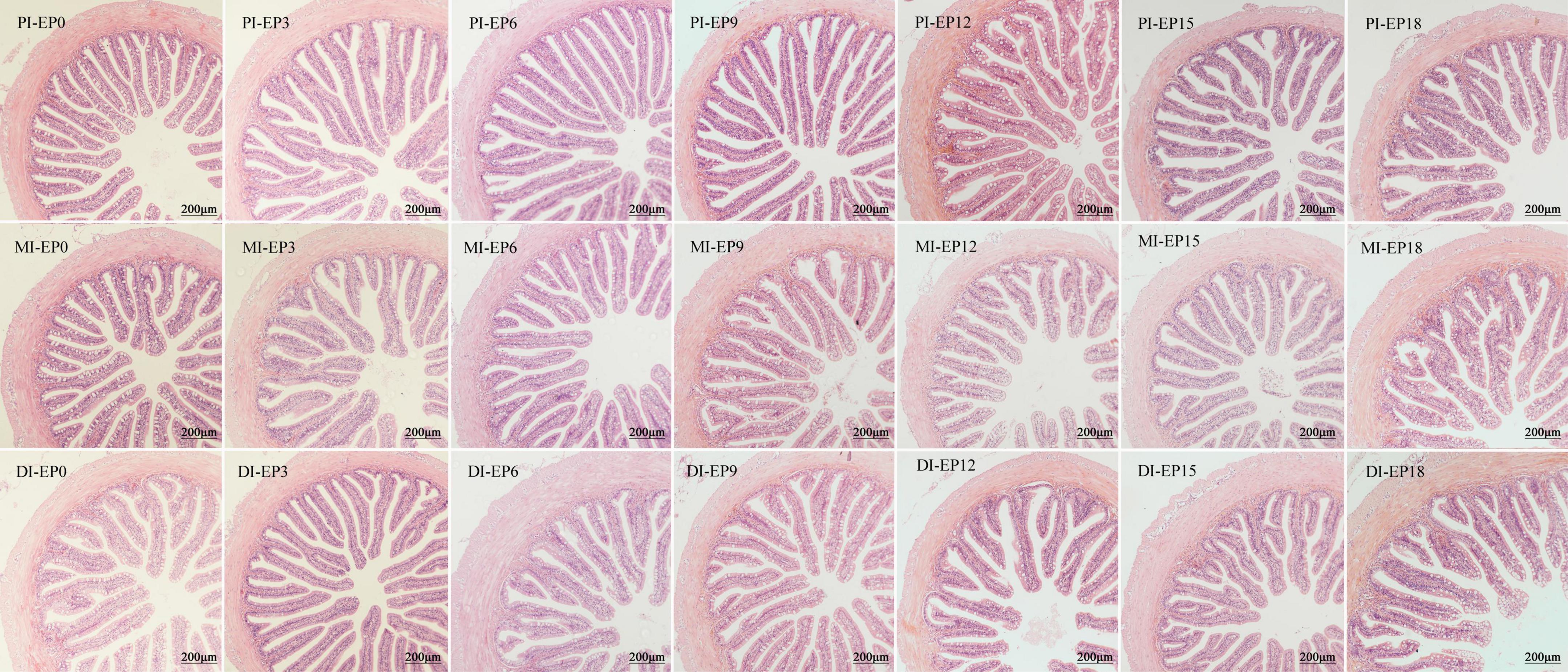
Figure 2. Light microscopy (20×) of the proximal intestine (PI), mid-intestine (MI), and distal intestine (DI) morphology of hybrid groupers fed with diets. Scale bar: 200 μm.
Intestinal microbiota
The samples were sequenced, and 1,680,219 pairs of reads were acquired. Following filtration and splicing of double-end reads, 1,593,640 clean tags were produced. At least 74,041 clean tags were produced per sample, with an average of 75,888 clean tags. In all samples, the rarefaction curves of the OTU numbers were observed to have the same trend and gradually converged to a saturation plateau (Figure 3), indicating that the sequencing has been accomplished.
The coverage was not less than 0.9950, indicating that the sequencing depth has met the experimental requirements (Table 7). The Chao 1, ACE, Simpson index, Shannon index, Good’s coverage, and OTUs were not significantly impacted (P > 0.05; Table 7). The scattered sample of the seven experimental groups was basically concentrated on the right side of the coordinate axis (Figure 4A). ANOSIM showed that the R-value was 0.178, indicating that there was no major change among or within the groups. The P-value was 0.047, which was less than 0.05, indicating that the test result was highly reliable (Figure 4B).
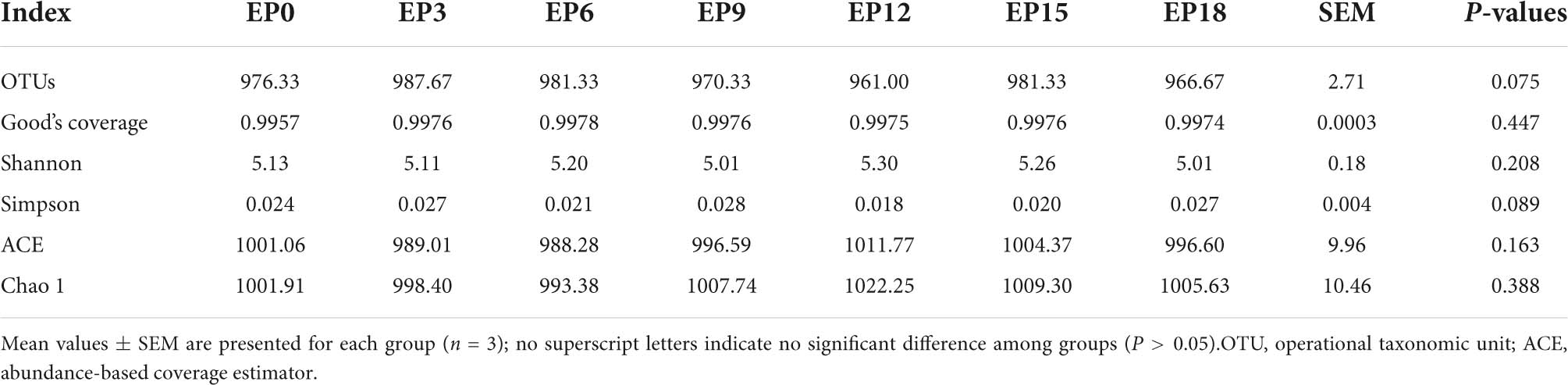
Table 7. Numbers of OTUs and alpha diversity index of the intestinal microbiota in hybrid groupers fed with diets.
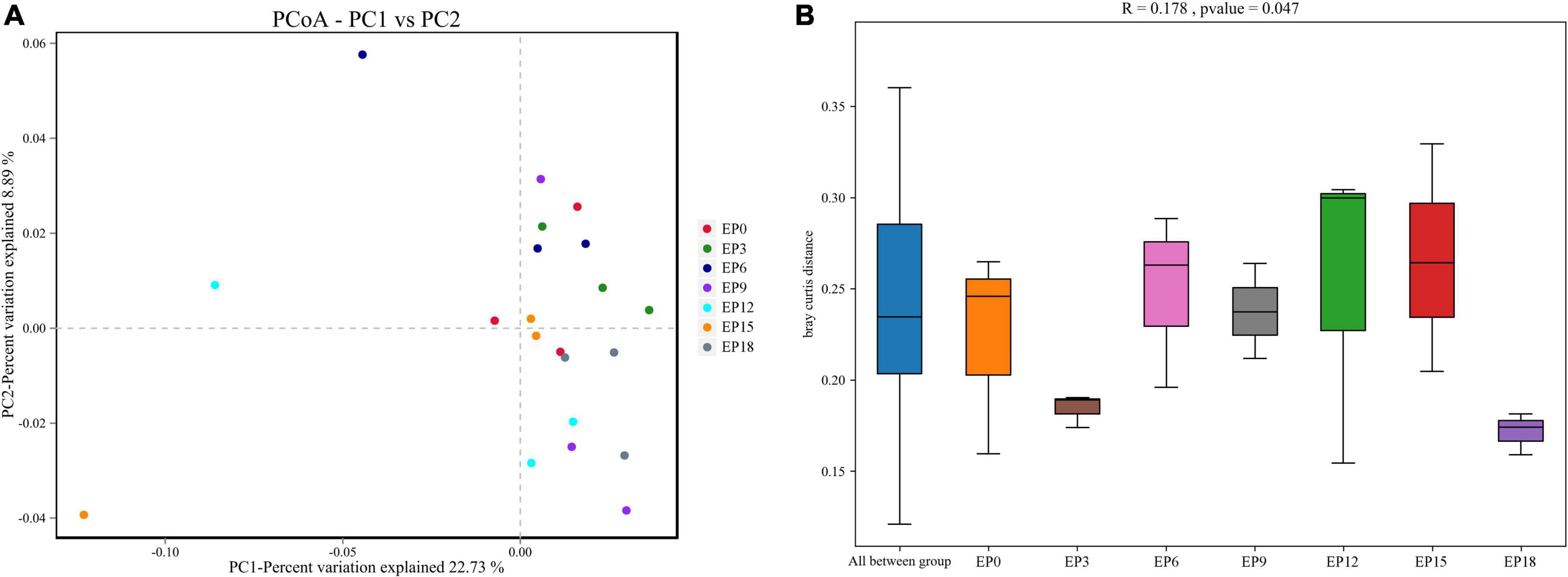
Figure 4. Principal coordinates analysis, PCoA (A); and analysis of similarities, ANOSIM (B) of the intestinal microbiota in hybrid groupers fed with diets.
The Venn diagram shows the unique and shared OTUs (Figure 5). The number of the shared OTUs in the EP3, EP6, EP9, EP12, EP15, and EP18 groups was 1,032, 1,038, 1,037, 1,035, 1,034, and 1,031, and the number of unique OTUs was 9, 9, 9, 10, 10, and 7, respectively, compared to the EP0 group. Interestingly, in the experimental samples of seven groups, the shared OTU was 1,014, and there was no unique OTU.
The top 10 dominant bacteria in the intestine of hybrid grouper, at the phylum level, were Patescibacteria, Verrucomicrobia, Chloroflexi, Cyanobacteria, Actinobacteria, Spirochetes, Fusobacteria, Proteobacteria, Bacteroidetes, and Firmicutes, respectively (Figure 6A). The top 10 dominant bacteria at the genus level were Lachnospiraceae_NK4A136_group, Blautia, Plesiomonas, Terrisporobacter, Ruminococcaceae_UCG-005, Clostridium Clostridium_sensu_stricto_1, Lactobacillus, Streptococcus, and uncultured_bacterium_f_Muribaculaceae, respectively (Figure 6B).
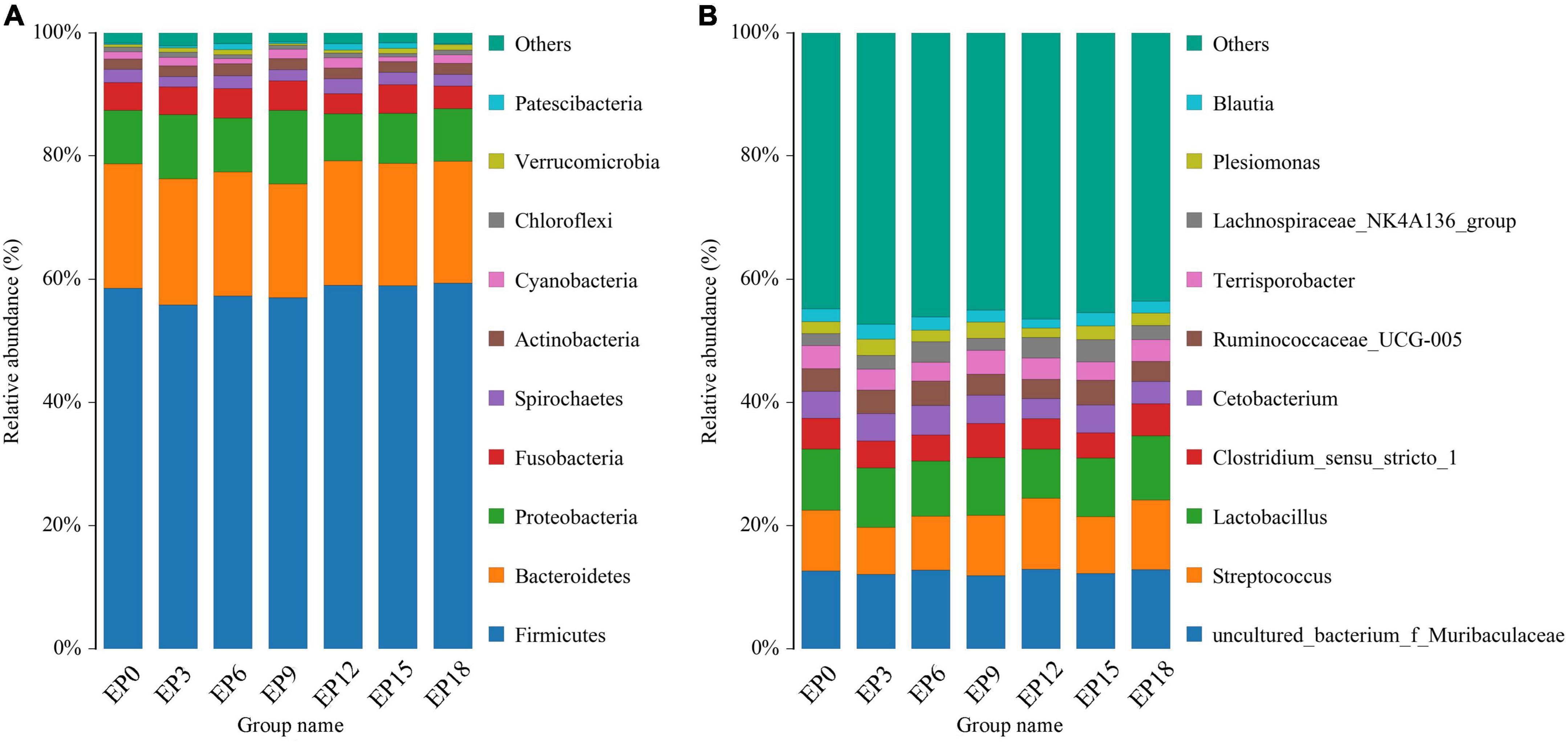
Figure 6. Taxonomy classification of reads at the phylum (A) and genus (B) taxonomic levels of the intestinal microbiota in hybrid groupers fed with diets.
The biomarkers with statistical differences in the EP0 group were Helicobacter, followed by Acidovorax, uncultured_bacterium_o_Rhodospirillales, Aequorivita, Thiobacillus, and Ottowia, according to LEfSe analysis (Figure 7). The biomarkers with statistical differences in the EP3 group were Prevotellaceae_Ga6A1_group, Parasutterella, Helicobacter, Holdemanella, Lachnospiraceae_UCG_008, Chroococcidiopsis_SAG_2023, Ruminococcus, and Fournierella. The biomarkers with statistical differences in the EP6 group were Ruminococcaceae_NK4A214_group, Muribaculum, and Cloacibacterium. The biomarker with statistical differences in the EP9 group was Flavobacteriaceae. The biomarkers with statistical differences in the EP12 group were Streptococcus, Xanthomonas, and Enterococcus. The biomarkers with statistical differences in the EP15 group were Erysipelatoclostridium and Eubacterium__coprostanoligenes_group. The biomarker with statistical differences in the EP18 group was Coprococcus_2.
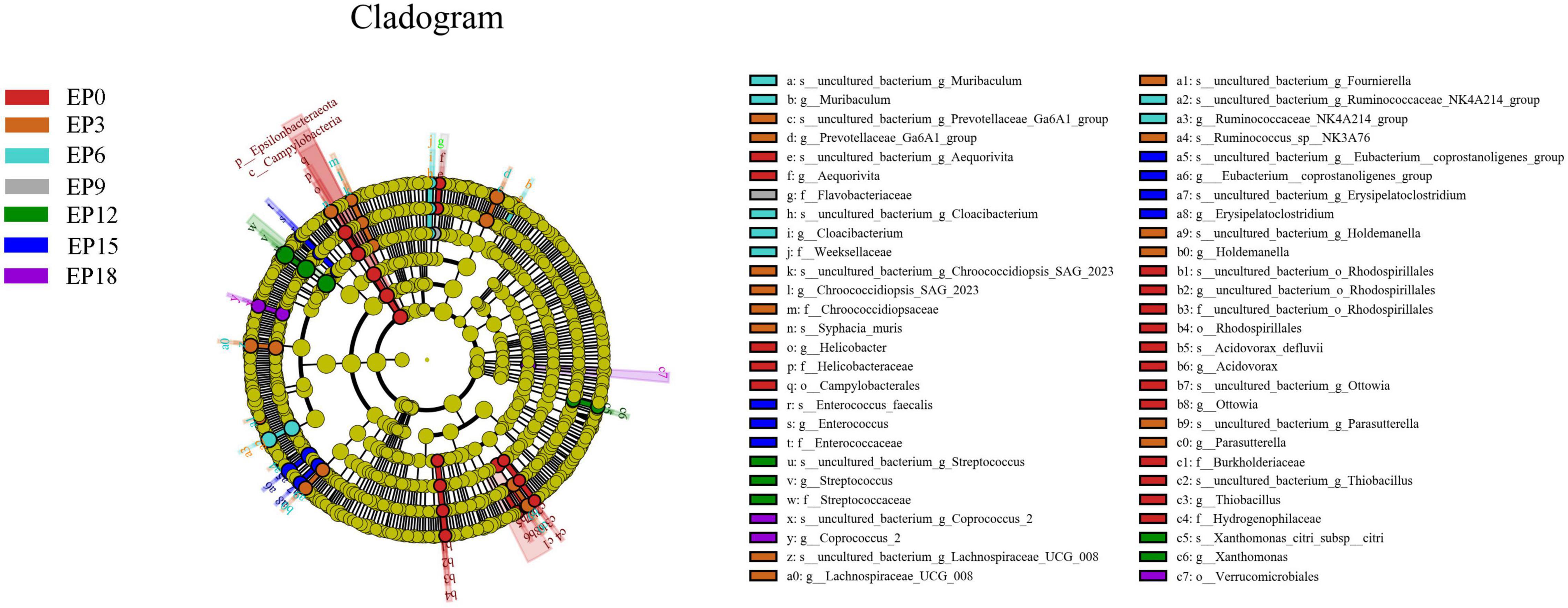
Figure 7. LEfSe analysis of the intestinal bacteria microbiota in hybrid groupers fed with diets. The circles radiating from the inside to the outside of the evolutionary branch diagram represent the classification levels of phylum (p), class (c), order (o), family (f), genus (g), and species (s).
Relative mRNA expression
In the PI, as the EPBM amount increased, the expressions of SNAT3, B0AT2, LAAT1, CAT1, and CAT2 showed an increase followed by a decrease, and the expressions of the above five genes were significantly higher when the EPBM amount was 30 g/kg (P < 0.05). The EAAT1 expression was prominently greater in the EP0 group, except for the EP6 group (P < 0.05). The experimental diet had no significant effect on the expressions of PAT1, B0, +AT, and PepT1 (P > 0.05; Figure 8A).
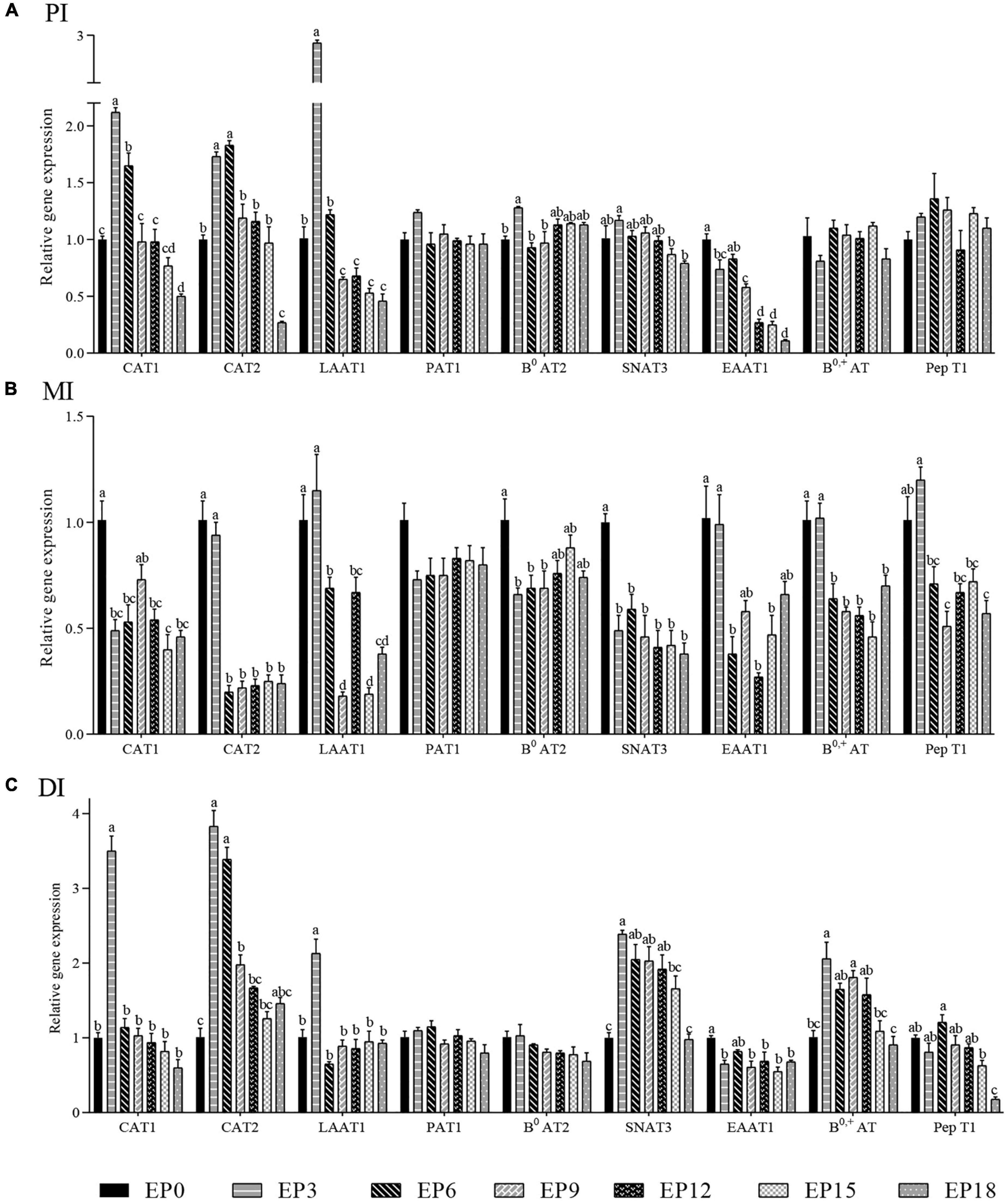
Figure 8. Relative mRNA expression of amino acid and small peptide-related transport in the (A) proximal intestine (PI), (B) mid-intestine (MI), and (C) distal intestine (DI) of hybrid groupers fed with diets, respectively. The β-actin gene was used as a housekeeping gene, and the control group was used as the reference group. Columns represented the mean values ± S. E. M. of each group. Different letters in each figure indicate significant differences among groups (a > b > c > d; P < 0.05).
In the MI, the expressions of CAT2, LAAT1, EAAT1, B0, +AT, and PepT1 were not significantly dissimilar in the EP3 group from that in the EP0 group (P > 0.05). EPBM substitution of FM led to a significant reduction in the expression of CAT1, B0AT2, and SNAT3 (P < 0.05). The effect of EPBM on the expression of PAT1 was not significant (P > 0.05; Figure 8B).
In the DI, the expression of B0, +AT, SNAT3, LAAT1, CAT2, and CAT1 gradually increased with increasing EPBM levels, showing a maximum in the EP3 group, followed by a gradual decrease (P < 0.05). The expression of PepT1 in the EP6 group was prominently increased than that in the EP15 and EP18 groups (P < 0.05). There were no major changes in the expressions of PAT1 and B0AT2 (P > 0.05; Figure 8C).
Discussion
In this research, the theoretical basis for the application of EPBM instead of FM in grouper feed was provided. The results showed that as the proportion of EPBM replacing FM increased, the LDR, HSI, SGR, and WGR of hybrid grouper tended to increase and then decrease, PER showed a downward trend, and IFR showed an upward trend. FM (from 360 to 210 g/kg) could be potentially replaced by 150 g/kg of EPBM in equal amounts without reducing growth performance. Studies indicated that PBM was replaced by more FM in the feed, the growth performance of giant croaker (Nibea japonica) (FM (from 400 to 240 g/kg) was replaced by 139 g/kg PBM) (35), hybrid striped bass (FM (from 250 to 162 g/kg) was replaced by 172 g/kg PBM) (19), and turbot (FM (from 773 to 380 g/kg) was replaced by 432 g/kg PBM) (36), which were significantly reduced. This may be caused by less FM content resulting in a weakening of the promotion effects of the attractants and growth-promoting factors (37).
In this experiment, replacing FM (from 360 to 330 g/kg) with an equivalent amount of 30 g/kg of EPBM significantly improved the growth performance, while the FM content was reduced to 210 g/kg, and growth performance was not reduced. This may be because 832.7 g/kg of the small peptides in the EPBM has a peptide molecular weight of less than 1,000 Da. In animals, small peptide proteins (dipeptide and tripeptide) can be directly absorbed (38). The uptake of peptides takes place through a special transport system, which has a fast absorption speed and low energy consumption, the carriers of peptide transport are not easy to saturate, and peptide transports are not competitive and inhibitory (39). This increases the rate of protein absorption, reduces the energy consumption of the animal to break down proteins, and saves energy for growth (40). Small peptides in feed have a significant role in promoting the growth of aquatic animals (41, 42) as well as improving the PDR and LDR of the hybrid grouper.
Amino acid balance is an important factor for growth (43). The supplementation of essential amino acids in this test was also one of the reasons why growth (the FM content was reduced to 210 g/kg) was not inhibited. The amino acid composition of EPBM was similar to FM. The supplementation of exogenous amino acids optimizes the amino acid structure and satisfies the nutritional requirements of the grouper. When FM was replaced by 180 g/kg of EPBM, WGR and SGR were reduced. It is possible that the excessive level of free amino acid is the cause. Excess amino acid produced by low molecular weight peptides in EPBM can cause competition of the transport mechanism and saturation of the transport system (44), restrict amino acid absorption, which is the main reason for the reduced growth, PER and ADCCP, and increase FCR in hybrid grouper (45). This suggests that more enzymatic protein contents in the feed may be detrimental to growth. Similar results were found in carp (46) and gilthead seabream (47).
Enzyme-digested poultry by-product meal is rich in flavor and taste-stimulating peptides (48). This may account for the gradual increase in IFR with the increase in EPBM content. With the increase in the proportion of FM replaced by EPBM, the HSI increased first and then decreased and showed a maximum value in the EP3 group. This may be because the WGR of the EP3 group was higher, and more protein and lipid were deposited in the liver so that the weight of the liver was greatly increased and finally leads to an increase in HSI.
The apparent digestibility of growing pigs was positively correlated with growth (49). In this experiment, the change of ADCCP and ADCDM was similar to the growth performance, and a significant decrease was found when FM was replaced by 90 g/kg of EPBM. ADCCP of feed was between 70 and 89%, while FM was replaced by PBM in fish feed (50–52). The ADCCP of the feed in this experiment was between 90.43 and 93.10%. It is closely related to the small peptides in EPBM which are easily digested and absorbed. Plus, the ADC CP in the EP3 group was not substantially diverse from that in the EP0 group, which was also a factor for the better growth performance of the EP3 and EP6 groups.
Many studies have reported that the replacement of FM by PBM has no significant effect on the whole-body composition of yellow catfish (Pelteobagrus fulvidraco) (20), turbot (21), spotted rose snapper (Lutjanus guttatus) (37), Atlantic salmon (Salmo salar) (50), and crayfish (Pacifastacus leniusculus Dana, Astacidae) (53). However, replacing FM with a protein source containing small peptides has a significant effect on the whole-body composition of hybrid grouper (hydrolyzed krill meal) (54), turbot (peptides hydrolyzed from poultry by-products) (55), and yellow catfish (fish protein hydrolyzate) (56). This may be caused by the rich small peptides in the feed that change the absorption of protein and lipid. In this study, the moisture content of the whole body was not positively influenced. As the EPBM levels increase, CP and EE contents tended to increase first and then decrease. It was consistent with the trend of PDR and LDR. When FM was replaced by 60 g/kg of EPBM, LDR of hybrid grouper was the highest, and more lipid was deposited. This also resulted in higher EE contents in whole body. With the further increase in EPBM content in feed, the EE content was decreased, and the serum TG content was increased, which may be caused by peptides that promoted the metabolism of lipids (57).
With the increase in the small peptide contents, the activities of digestive enzymes in the liver and intestine are mostly increasing and then decreasing. It was consistent with the results obtained from sea bass (Dicentrarchus labrax) (41), turbot (55), and pacific white shrimp (58). The changing trend of the intestinal digestive enzyme activity was consistent with the VW, VL, and VL/CD value of PI, as well as MT and VL/CD value of DI. The small peptides can accelerate villus growth, promote intestinal digestive function, and induce and stimulate the increase in enzyme activity (59). When FM was replaced by 150 g/kg of EPBM, improvements in digestive enzyme activities and the amelioration of intestinal morphology were important factors in the absence of negative effects on the growth of hybrid grouper.
Active peptides can provide the nitrogen skeleton for digestive enzyme synthesis, promote the secretion of enzymes, and stimulate hormone receptors in the intestine (60). Higher intestinal chymotrypsin and trypsin activities in the EP12 and EP15 groups indicate that increased EPBM required more proteases for adequate protein absorption. This also resulted in higher levels of Alb and TP in serum and CP in the whole body. However, there was no significant action on the composition of large yellow croaker (Larimichthys crocea) (42) and turbot (61). The different sources and differences in nutritional ingredients of small peptides are also important reasons for whole-body composition (62).
Antioxidant capacity is commonly referred to as an indicator of aquatic animals’ health (63). Antioxidant enzymes protect against free radical damage, and CAT and SOD can reduce oxidative damage and keep the balance of free radicals (64). A low concentration of reactive oxygen species is one of the necessary conditions for cells to perform normal physiological functions (65). Animals can protect tissues and cells from oxidative stress by regulating CAT and SOD activity (66). Small peptides in feed can improve the antioxidant capacity of hybrid grouper (54), turbot (55), carp (Carassius auratus gibelio) (67), and Japanese flounder (Paralichthys olivaceus) (68). In this experiment, the changes in CAT and SOD activities were similar to the growth performance, reaching the maximum when FM was replaced with 30 g/kg of EPBM. This suggested that substituting FM with EPBM could improve the antioxidant capacity of the hybrid grouper. The increasement in EPBM treatments for antioxidative ability could be due to the high proportion (472.3 g/kg) of peptides with molecular weight from 500 to 5,000 Da in EPBM. Since previous reports showed that medium-sized bioactive peptides (molecular weight from 300 to 5,000 Da) can have antioxidative and immunity stimulating properties (69). This is also the reason for the increase in ACP activity. The GPT and GOT are important amino acid metabolizing enzymes (70). Under normal circumstances, the aminotransferase activity in serum is low. When animals suffer from various malnutrition, especially when the liver is damaged, serum GOT and GPT activities could increase (71). EPBM replacement of FM reduced the GPT activity in serum, which indicated a protective effect of EPBM on the liver. Similar situations were found on Jian carp (72) and largemouth bass (Micropterus salmoides) (73).
The structure and diversity of the intestinal bacterial community directly affect the digestion and absorption, growth performance, and immunity of fish (74). Many factors affect the dynamic balance of the intestinal bacterial community, such as the breeding environment, external stress, and feed composition (75–77). In this study, replacing FM with different levels of EPBM had no significant effect on Chao1 and ACE, indicating no significant effect on species abundance. The Shannon and Simpson indices were not significantly affected by diets, indicating no significant effect on species diversity. There was no variation in the numbers of OTUs, and the number of unique OTUs in each group was 0. The top 10 dominant phylum and dominant genus have no significant difference in category and abundance. These data suggest that the replacement of FM with EPBM may have little effect on the intestinal microbiota of the hybrid grouper. Research has shown that the dominant phylum of vertebrates contains Proteobacteria, Firmicutes, and Bacteroidetes (78). Similar results were obtained in our study, and the dominant phylum of hybrid grouper contained Firmicutes, Bacteroidetes, and Proteobacteria, accounting for 87.07% of the relative abundance. The dominant genus included uncultured_bacterium_f_Muribaculaceae, Streptococcus, and Lactobacillus, accounting for 31.66% of the relative abundance. These genera of absolute advantage of the intestine in hybrid grouper may be associated with the intestinal microbes that have been set at the seedling stage. Pond et al. believe that after the hatched juvenile fish were exposed to the culture environment, a variety of bacteria will enter the intestinal tract and then set its value in the intestinal epithelium (79). In the future process of growth, the dominant bacterial community was not significantly influenced by the breeding environment and feed.
Amino acid transporter is a functional protein commonly found in the biofilm system (80). It is an important nutrient-sensing molecule and plays a key role in protein synthesis (81). Proteins are absorbed by the body in the form of peptides and amino acids, and the latter is the better absorption form (81), which transport through the peptide transporters (82). The interesting things were that the proper amount of EPBM instead of FM can increase the expression of LAAT1, CAT2, and CAT1 in the PI and DI, SNAT3 and B0AT2 in the PI, and B0, +AT in the DI. The relative expression of these genes gradually decreased when the EPBM content of the feed was further increased. This may be attributed to the fact that EPBM is rich in free amino acids. Excess-free amino acids in the feed can lead to saturation of the intestinal amino acid transport system and transport competition, resulting in reduced expression of amino acid transport-related genes, which consequently makes amino acid transport impaired. The proper amount of EPBM instead of FM can increase the expression of PepT1 in the DI and MI. This might be because EPBM is rich in tripeptides and dipeptides and increased the expression of PepT1 (83), which allows more small peptide proteins to be absorbed by hybrid grouper. Similar results were obtained on yellow perch (Perca flavescens) (84) and rainbow trout (Oncorhynchus mykiss) (84). More amino acids were transported from extracellular to intracellular with the increase in amino acid transport expressions (85). The transport of small peptides and amino acids can facilitate each other (86, 87). When hybrid groupers were fed a diet containing 150 g/kg of EPBM, the improved expression of peptide and amino acid transporter was one of the important factors for uninhibited growth performance. In this test, the expression of PAT1 was not significantly influenced by diet. It may be because PAT1 is mainly expressed in muscle, and the expression in the intestine is low (88), and the relative change is not obvious.
Conclusion
This study showed that replacing FM with 30 g/kg of EPBM can significantly improve growth performance, antioxidant capacity, and the ability to transport amino acids and peptides in hybrid grouper.
Data availability statement
The original contributions presented in this study are included in the article/supplementary material, further inquiries can be directed to the corresponding authors.
Ethics statement
The animal study was reviewed and approved by the Institutional Animal Care and Use Committee of Guangdong Ocean University. Written informed consent was obtained from the owners for the participation of their animals in this study.
Author contributions
XY: conceptualization, investigation, formal analysis, and writing – original draft. XZ, GW, XD, QY, HL, and SZ: methodology and resources. BT: methodology, resources, and funding acquisition. SC: conceptualization, writing – reviewing and editing, supervision, project administration, and funding acquisition. All authors have read and approved the final manuscript.
Funding
This study was supported by the Fund of the National Key R&D Program of China (2019YFD0900200); the Southern Marine Science and Engineering Guangdong Laboratory (Zhanjiang) (No. ZJW-2019-06); and the China Agriculture Research System (CARS-47).
Conflict of interest
XZ and GW were employed by Yichang Huatai Biological Technology Co., Ltd., Yichang, China.
The remaining authors declare that the research was conducted in the absence of any commercial or financial relationships that could be construed as a potential conflict of interest.
Publisher’s note
All claims expressed in this article are solely those of the authors and do not necessarily represent those of their affiliated organizations, or those of the publisher, the editors and the reviewers. Any product that may be evaluated in this article, or claim that may be made by its manufacturer, is not guaranteed or endorsed by the publisher.
References
1. Olsen RL, Hasan MR. A limited supply of fishmeal: impact on future increases in global aquaculture production. Trends Food Sci Tech. (2012) 27:120–8. doi: 10.1016/j.tifs.2012.06.003
2. Wu YB, Ren X, Chai XJ, Li P, Wang Y. Replacing fish meal with a blend of poultry by-product meal and feather meal in diets for giant croaker (Nibea japonica). Aquacult Nutr. (2018) 24:1085–91. doi: 10.1111/anu.12647
3. Rhodes MA, Zhou Y, Salze GP, Hanson TR, Davis DA. Development of plant-based diets and the evaluation of dietary attractants for juvenile Florida pompano, Trachinotus carolinus L. Aquacult Nutr. (2017) 23:1065–75. doi: 10.1111/anu.12474
4. Yuan XY, Liu WB, Liang C, Sun CX, Xue YF, Wan ZD, et al. Effects of partial replacement of fish meal by yeast hydrolysate on complement system and stress resistance in juvenile Jian carp (Cyprinus carpio var. Jian). Fish Shellfish Immunol. (2017) 67:312–21. doi: 10.1016/j.fsi.2017.06.028
5. Fournier V, Huelvan C, Desbruyeres E. Incorporation of a mixture of plant feedstuffs as substitute for fish meal in diets of juvenile turbot (Psetta maxima). Aquaculture. (2004) 236:451–65. doi: 10.1016/j.aquaculture.2004.01.035
6. Bonvini E, Parma L, Badiani A, Fontanillas R, Gatta PP, Sirri F, et al. Integrated study on production performance and quality traits of European sea bass (Dicentrarchus labrax) fed high plant protein diets. Aquaculture. (2018) 484:126–32. doi: 10.1016/j.aquaculture.2017.10.041
7. Gomez-Requeni P, Mingarro M, Calduch-Giner JA, Medale F, Martin SAM, Houlihan DF, et al. Protein growth performance, amino acid utilisation and somatotropic axis responsiveness to fish meal replacement by plant protein sources in gilthead sea bream (Sparus aurata). Aquaculture. (2004) 232:493–510. doi: 10.1016/S0044-8486(03)00532-5
8. Gatlin DM, Barrows FT, Brown P, Dabrowski K, Gaylord TG, Hardy RW, et al. Expanding the utilization of sustainable plant products in aquafeeds: a review. Aquac Res. (2007) 38:551–79. doi: 10.1111/j.1365-2109.2007.01704.x
9. Santigosa E, Sanchez J, Medale F, Kaushik S, Perez-Sanchez J, Gallardo MA. Modifications of digestive enzymes in trout (Oncorhynchus mykiss) and sea bream (Sparus aurata) in response to dietary fish meal replacement by plant protein sources. Aquaculture. (2008) 282:68–74. doi: 10.1016/j.aquaculture.2008.06.007
10. Li W, Li L, Liu H, Tan B, Dong X, Yang Q, et al. Effects of Clostridium butyricum on growth, antioxidant capacity and non-specific immunology of Litopenaeus vannamei fed with concentrated cottonseed protein replacement of fishmeal. J Ocean U China. (2022) 42:29–37. doi: 10.3969/j.issn.1673-9159.2022.02.004
11. Gui L, Mai H, Chi S, Zhou W, Li Y, Tan B, et al. Effects of yeast culture on growth performance, hematological parameters, immunity and disease resistance of Litopenaeus vannamei. J Ocean U China. (2019) 39:30–7. doi: 10.3969/j.issn.1673-9159.2019.03.005
12. Liu H, Li L-X, Ayiku S, Tang Z, Fang W, Tan B, et al. Effects of dietary yeast culture supplementation on growth, intestinal morphology, immunity, and disease resistance in Epinephelus fuscoguttatus ♀× Epinephelus lanceolatu ♂. J Ocean U China. (2021) 41:1–11. doi: 10.3969/j.issn.1673-9159.2021.03.001
13. Gonzalez-Rodriguez A, Celada JD, Carral JM, Saez-Royuela M, Garcia V, Fuertes JB. Evaluation of poultry by-product meal as partial replacement of fish meal in practical diets for juvenile tench (Tinca tinca L.). Aquac Res. (2016) 47:1612–21. doi: 10.1111/are.12622
14. Karapanagiotidis IT, Psofakis P, Mente E, Malandrakis E, Golomazou E. Effect of fishmeal replacement by poultry by-product meal on growth performance, proximate composition, digestive enzyme activity, haematological parameters and gene expression of gilthead seabream (Sparus aurata). Aquacult Nutr. (2019) 25:3–14. doi: 10.1111/anu.12824
15. Webster CD, Thompson KR, Morgan AM, Grisby EJ, Gannam AL. Use of hempseed meal, poultry by-product meal, and canola meal in practical diets without fish meal for sunshine bass (Morone chrysops × M. saxatilis). Aquaculture. (2000) 188:299–309. doi: 10.1016/S0044-8486(00)00338-0
16. Wang Y, Guo JL, Bureau DP, Cui ZH. Replacement of fish meal by rendered animal protein ingredients in feeds for cuneate drum (Nibea miichthioides). Aquaculture. (2006) 252:476–83. doi: 10.1016/j.aquaculture.2005.07.018
17. Watson AM, Buentello A, Place AR. Partial replacement of fishmeal, poultry by-product meal and soy protein concentrate with two non-genetically modified soybean cultivars in diets for juvenile cobia, Rachycentron canadum. Aquaculture. (2014) 434:129–36. doi: 10.1016/j.aquaculture.2014.08.003
18. Turker A, Yigit M, Ergun S, Karaali B, Erteken A. Potential of poultry by-product meal as a substitute for fishmeal in diets for Black Sea turbot Scophthalmus maeoticus: growth and nutrient utilization in winter. Isr J Aquacult Bamid. (2005) 57:49–61. doi: 10.46989/001c.20392
19. Rawles SD, Riche M, Gaylord TG, Webb J, Freeman DW, Davis M. Evaluation of poultry by-product meal in commercial diets for hybrid striped bass (Morone chrysops ♀ x M. saxatilis ♂) in recirculated tank production. Aquaculture. (2006) 259:377–89. doi: 10.1016/j.aquaculture.2006.05.053
20. Luo JX, Huang WW, Yuan Y, Chen LC, Ting ZT, Cun ZQ. Effects of fish meal replacement with poultry by-product meal on growth performance, feed utilization, digestive enzyme activities and antioxidant capacity of juvenile yellow catfish (Pelteobagrus fulvidraco). Chin J Anim Nutrit. (2017) 29:3970–9. doi: 10.3969/j.issn.1006-267x.2017.11.017
21. Li ZS, Wu LX, Li SM, Peng BW, Zhao BW. Effects of replacing fish meal with poultry by-product meal on growth performance and body composition of juvenile turbot Scophthalmus maximus. Fisher Sci. (2016) 35:486–91. doi: 10.16378/j.cnki.1003-1111.2016.05.005
22. Amirkolaie AK, Shahsavari M, Hedayatyfard M. Full replacement of fishmeal by poultry by-product meal in rainbow trout, Oncorhynchus mykiss (Walbaum, 1972) diet. Iran J Fish Sci. (2014) 13:1069–81.
23. Wang Y, Ma XZ, Wang F, Wu YB, Qin JG, Li P. Supplementations of poultry by-product meal and selenium yeast increase fish meal replacement by soybean meal in golden pompano (Trachinotus ovatus) diet. Aquac Res. (2017) 48:1904–14. doi: 10.1111/are.13028
24. Barbour GW, Nemasetoni R, Lilburn MS, Werling M, Yersin AG. The effect of enzyme predigestion on the quality of poultry by-product meal from whole turkey mortality. Poult Sci. (1995) 74:1180–90. doi: 10.3382/ps.0741180
25. Cordova-Murueta JH, Garcia-Carreno FL. Nutritive value of squid and hydrolyzed protein supplement in shrimp feed. Aquaculture. (2002) 210:371–84. doi: 10.1016/S0044-8486(02)00011-X
26. Webb KE Jr. Intestinal absorption of protein hydrolysis products: a review. J Anim Sci. (1990) 68:3011–22. doi: 10.2527/1990.6893011x
27. Li MJ, Li CB, Song SX, Zhao F, Xu XL, Zhou GH. Meat proteins had different effects on oligopeptide transporter PEPT1 in the small intestine of young rats. Int J Food Sci Nutr. (2016) 67:995–1004. doi: 10.1080/09637486.2016.1210574
28. Martinez-Alvarez O, Chamorro S, Brenes A. Protein hydrolysates from animal processing by-products as a source of bioactive molecules with interest in animal feeding: a review. Food Res Int. (2015) 73:204–12. doi: 10.1016/j.foodres.2015.04.005
29. Refstie S, Olli JJ, Standal H. Feed intake, growth, and protein utilisation by post-smolt Atlantic salmon (Salmo salar) in response to graded levels of fish protein hydrolysate in the diet. Aquaculture. (2004) 239:331–49. doi: 10.1016/j.aquaculture.2004.06.015
30. Yang XY, Zhi XY, Song ZL, Wang GH, Zhao XM, Chi SY, et al. Flesh quality of hybrid grouper (Epinephelus fuscoguttatus × E. lanceolatus ♂) fed with hydrolyzed porcine mucosa-supplemented low fishmeal diet. Anim Nutr. (2022) 8:114–24. doi: 10.1016/j.aninu.2021.05.011
31. Lim LS, Mukai Y. Morphogenesis of sense organs and behavioural changes in larvae of the brown-marbled grouper Epinephelus fuscoguttatus (Forsskal). Mar Freshw Behav Phy. (2014) 47:313–27. doi: 10.1080/10236244.2014.940689
32. Harikrishnan R, Balasundaram C, Heo MS. Molecular studies, disease status and prophylactic measures in grouper aquaculture: economic importance, diseases and immunology. Aquaculture. (2010) 309:1–14. doi: 10.1016/j.aquaculture.2010.09.011
33. Horwitz W. Agricultural chemicals, contaminants, drugs. In: Horwitz W editor. Official Methods of Analysis of AOAC International. (Vol. I), Gaithersburg, ML: AOAC International (2010).
34. Yang XY, Wang GH, Zhao XM, Dong XH, Chi SY, Tan BP. Addition of hydrolysed porcine mucosa to low-fishmeal feed improves intestinal morphology and the expressions of intestinal amino acids and small peptide transporters in hybrid groupers (Epinephelus fuscoguttatus ♀× E. lanceolatus ♂). Aquaculture. (2021) 535:736389. doi: 10.1016/j.aquaculture.2021.736389
35. Mu H, Shen HH, Liu JH, Xie FL, Zhang WB, Mai KS. High level of dietary soybean oil depresses the growth and anti-oxidative capacity and induces inflammatory response in large yellow croaker Larimichthys crocea. Fish Shellfish Immunol. (2018) 77:465–73. doi: 10.1016/j.fsi.2018.04.017
36. Yigit M, Erdem M, Koshio S, Ergun S, Turker A, Karaali B. Substituting fish meal with poultry by-product meal in diets for black Sea turbot Psetta maeotica. Aquacult Nutr. (2006) 12:340–7. doi: 10.1111/j.1365-2095.2006.00409.x
37. Hernandez C, Sanchez-Gutierrez Y, Hardy RW, Benitez-Hernandez A, Dominguez-Jimenez P, Gonzalez-Rodriguez B, et al. The potential of pet-grade poultry by-product meal to replace fish meal in the diet of the juvenile spotted rose snapper Lutjanus guttatus (Steindachner, 1869). Aquacult Nutr. (2014) 20:623–31. doi: 10.1111/anu.12122
38. Ki MR, Lee JH, Yun SK, Choi KM, Hwang SY. Roles of the peptide transport systems and aminopeptidase PepA in peptide assimilation by Helicobacter pylori. J Microbiol Biotechnol. (2015) 25:1629–33. doi: 10.4014/jmb.1505.05099
39. Stelzl T, Baranov T, Geillinger KE, Kottra G, Daniel H. Effect of N-glycosylation on the transport activity of the peptide transporter PEPT1. Am J Physiol Gastr L. (2016) 310:G128–41. doi: 10.1152/ajpgi.00350.2015
40. Jiang LS, Chen W, Li BS, Sun YZ, Wang JY, Huang BS, et al. Effects of replacing fishmeal by protein hydrolysates on the growth performance and physiological metabolism of Scophthalmus maximus. Mar Fisher. (2019) 41:596–605. doi: 10.13233/j.cnki.mar.fish.2019.05.007
41. Zambonino Infante JL, Cahu CL, Peres A. Partial substitution of di-and tripeptides for native proteins in sea bass diet improves Dicentrarchus labrax larval development. J Nutr. (1997) 127:608–14. doi: 10.1093/jn/127.4.608
42. Cai ZN, Li WJ, Mai KS, Xu W, Zhang YJ, Ai QH. Effects of dietary size-fractionated fish hydrolysates on growth, activities of digestive enzymes and aminotransferases and expression of some protein metabolism related genes in large yellow croaker (Larimichthys crocea) larvae. Aquaculture. (2015) 440:40–7. doi: 10.1016/j.aquaculture.2015.01.026
43. Lin JW, Zhang CX, Sun YZ, Zhai SW, Song K, Ye JD. Complete replacement of fish meal by poultry by-product meal in practical diets supplemented with coated amino acids for pacific white shrimp Litopenaeus vannamei. Acta Hydrobiol Sin. (2016) 40:700–11. doi: 10.7541/2016.93
44. Plakas SM, Katayama T. Apparent digestibilities of amino acids from three regions of the gastrointestinal tract of carp (Cyprinus carpio) after ingestion of a protein and a corresponding free amino acid diet. Aquacult Int. (1981) 24:309–14. doi: 10.1016/0044-8486(81)90065-X
45. Aragao C, Conceicao LEC, Martins D, Ronnestad I, Gomes E, Dinis MT. A balanced dietary amino acid profile improves amino acid retention in post-larval Senegalese sole (Solea senegalensis). Aquaculture. (2004) 233:293–304. doi: 10.1016/j.aquaculture.2003.08.007
46. Carvalho AP, Sa R, Oliva-Teles A, Bergot P. Solubility and peptide profile affect the utilization of dietary protein by common carp (Cyprinus carpio) during early larval stages. Aquaculture. (2004) 234:319–33. doi: 10.1016/j.aquaculture.2004.01.007
47. Kolkovski S, Tandler A. The use of squid protein hydrolysate as a protein source in microdiets for gilthead seabream Sparus aurata larvae. Aquacult Nutr. (2000) 6:11–5. doi: 10.1046/j.1365-2095.2000.00125.x
48. Gallego M, Mora L, Escudero E, Toldra F. Bioactive peptides and free amino acids profiles in different types of European dry-fermented sausages. Int J Food Microbiol. (2018) 276:71–8. doi: 10.1016/j.ijfoodmicro.2018.04.009
49. Conejos JRV, Acda SP, Capitan SS, Agbisit EM, Merca FE. Mannan oligosaccharides from yeast (Saccharomyces cerevisiae) cell wall improves nutrient digestibility and intestinal morphology of growing pigs [Sus domesticus (Erxleben)]. Philipp Agric Sci. (2012) 95:305–11. doi: 10.3098/ah.2012.86.4.235
50. Hatlen B, Jakobsen JV, Crampton V, Alm M, Langmyhr E, Espe M, et al. Growth, feed utilization and endocrine responses in Atlantic salmon (Salmo salar) fed diets added poultry by-product meal and blood meal in combination with poultry oil. Aquacult Nutr. (2015) 21:714–25. doi: 10.1111/anu.12194
51. Cheng ZJJ, Hardy RW. Apparent digestibility coefficients of nutrients and nutritional value of poultry by-product meals for rainbow trout Oncorhynchus mykiss measured in vivo using settlement. J World Aquacult Soc. (2002) 33:458–65. doi: 10.1111/j.1749-7345.2002.tb00025.x
52. Gaylord TG, Barrows FT, Rawles SD. Apparent digestibility of gross nutrients from feedstuffs in extruded feeds for rainbow trout, Oncorhynchus mykiss. J World Aquacult Soc. (2008) 39:827–34. doi: 10.1111/j.1749-7345.2008.00220.x
53. Fuertes JB, Celada JD, Carral JM, Saez-Royuela M, Gonzalez-Rodriguez A. Replacement of fish meal with poultry by-product meal in practical diets for juvenile crayfish (Pacifastacus leniusculus Dana, Astacidae) from the onset of exogenous feeding. Aquaculture. (2013) 404:22–7. doi: 10.1016/j.aquaculture.2013.04.019
54. Wei JL, Wang JY, Song ZD, Huang Y, Sun YZ, Zhang LM. Effects of the partial substitute for fish meal by hydrolyzed krill meal on growth performance, the body composition and serum biochemical parameters of juvenile pearl gentian grouper. Prog Fish Sci. (2016) 37:100–10. doi: 10.11758/yykxjz.20141211002
55. Jia GW, Guo R, Zhang Y, Li XH, Xia H, Gong CG, et al. Effects of partial replacement of fish meal by peptides hydrolyzed from poultry by-products on growth performance, digestive indices and specific immune indices of turbot. Chin J Anim Nutrit. (2019) 31:2201–11. doi: 10.3969/j.issn.1006-267x.2019.05.027
56. Zhou LY, Wu DW, Gao MM, He J, Sun F, Yu N, et al. The effects of fish meal replacement on growth performance of yellow catfish (Pelteobagrus fulvidraco). Acta Hydrobiol Sin. (2019) 43:504–16. doi: 10.7541/2019.062
57. Drevon CA. Fatty acids and expression of adipokines. BBA Mol Basis Dis. (2005) 1740:287–92. doi: 10.1016/j.bbadis.2004.11.019
58. Xu PY, Zhou HQ. Effects of small peptides on the protease and amylase activities of Litopenaeus vannamei. J Shanghai Fisher University. (2005) 14:133–7. doi: 10.1360/biodiv.050121
59. Wang XX, Wei YL, Liang MQ. Fish protein hydrolysates: properties and applications. Chin J Anim Nutrit. (2012) 24:1636–42. doi: 10.3969/j.issn.1006-267x.2012.09.004
60. Chen L, Zhang RJ. Bioactive peptide or oligopeptide feed additive research and applications. Acta Zoonutrimenta Sin. (2004) 16:12–4. doi: 10.3969/j.issn.1006-267X.2004.02.003
61. Oliva-Teles A, Cerqueira AL, Gonçalves P. The utilization of diets containing high levels of fish protein hydrolysate by turbot (Scophthalmus maximus) juveniles. Aquaculture. (1999) 179:195–201. doi: 10.1016/S0044-8486(99)00162-3
62. Chang YM, Yan H, Su BF, Sun B, Zhang LM, Liang LQ. Analysis of muscular nutritional composition in farmed Leuciscus spp. and their hybrids in a low saline-alkaline pond. J Fishery Sci China. (2017) 24:332–40. doi: 10.3724/SP.J.1118.2017.16188
63. Yu LL, Yu HH, Liang XF, Li N, Wang X, Li FH, et al. Dietary butylated hydroxytoluene improves lipid metabolism, antioxidant and anti-apoptotic response of largemouth bass (Micropterus salmoides). Fish Shellfish Immunol. (2018) 72:220–9. doi: 10.1016/j.fsi.2017.10.054
64. Gong YL, Yang F, Hu JP, Liu C, Liu HK, Han D, et al. Effects of dietary yeast hydrolysate on the growth, antioxidant response, immune response and disease resistance of largemouth bass (Micropterus salmoides). Fish Shellfish Immunol. (2019) 94:548–57. doi: 10.1016/j.fsi.2019.09.044
65. Mates JM. Effects of antioxidant enzymes in the molecular control of reactive oxygen species toxicology. Toxicology. (2000) 153:83–104. doi: 10.1016/s0300-483x(00)00306-1
66. Reda RM, Selim KM, Mahmoud R, El-Araby IE. Effect of dietary yeast nucleotide on antioxidant activity, non-specific immunity, intestinal cytokines, and disease resistance in Nile Tilapia. Fish Shellfish Immunol. (2018) 80:281–90. doi: 10.1016/j.fsi.2018.06.016
67. Liu WB, Zhan YC, Wang T. Nutrition value of plant protein hydrolysates for alloggnogeuetic crucian carp. J Chin Cereals Oils Assoc. (2007) 22:108–12. doi: 10.1360/yc-007-1071
68. Xu TH, Gao XP, Liang MQ, Wang XX, Zheng KK, Chang Q, et al. Effects of small size-fractionated fish protein hydrolysate substitution of fish meal in high plant protein diets on the growth performance and non-specific immunity of Japanese flounder, Paralichthys olivaceus. Prog Fishery Sci. (2012) 33:60–9. doi: 10.3969/j.issn.1000-7075.2012.03.009
69. Wu DW, Zhou LY, Gao MM, Wang MY, Wang B, He J, et al. Effects of stickwater hydrolysates on growth performance for yellow catfish (Pelteobagrus fulvidraco). Aquaculture. (2018) 488:161–73. doi: 10.1016/j.aquaculture.2018.01.031
70. Bibiano Melo JF, Lundstedt LM, Meton I, Baanante IV, Moraes G. Effects of dietary levels of protein on nitrogenous metabolism of Rhamdia quelen (Teleostei: Pimelodidae). Comp Biochem Phys A. (2006) 145:181–7. doi: 10.1016/j.cbpa.2006.06.007
71. Nyblom H, Berggren U, Balldin J, Olsson R. High AST/ALT ratio may indicate advanced alcoholic liver disease rather than heavy drinking. Alcohol Alcoholism. (2004) 39:336–9. doi: 10.1093/alcalc/agh074
72. Xia W, Liu WB, Qiao QS, Li GF, Zhang YJ. Effects of cottonseed meal hydrolysate on growth performance and biochemical indices of Cyprinus carpio var. Jian. Freshwater Fisher. (2012) 42:46–51. doi: 10.3969/j.issn.1000-6907.2012.01.009
73. Zhang GG, Li X, Cai XB, Zhang SX, Hua XM, Huang ZY, et al. Effects of enzymatic hydrolyzed soybean meal on growth performance, liver function and metabolism of largemouth bass (Micropterus salmoides). Acta Hydrobiol Sin. (2019) 43:1001–12. doi: 10.7541/2019.119
74. Xun PW, Lin HZ, Wang RX, Huang Z, Zhou CP, Yu W, et al. Effects of dietary vitamin B1 on growth performance, intestinal digestion and absorption, intestinal microflora and immune response of juvenile golden pompano (Trachinotus ovatus). Aquaculture. (2019) 506:75–83. doi: 10.1016/j.aquaculture.2019.03.017
75. Cardona E, Gueguen Y, Magre K, Lorgeoux B, Piquemal D, Pierrat F, et al. Bacterial community characterization of water and intestine of the shrimp Litopenaeus stylirostris in a biofloc system. BMC Microbiol. (2016) 16:157. doi: 10.1186/s12866-016-0770-z
76. Sullam KE, Essinger SD, Lozupone CA, O’Connor MP, Rosen GL, Knight R, et al. Environmental and ecological factors that shape the gut bacterial communities of fish: a meta-analysis. Mol Ecol. (2012) 21:3363–78. doi: 10.1111/j.1365-294X.2012.05552.x
77. Merrifield DL, Burnard D, Bradley G, Davies SJ, Baker RTM. Microbial community diversity associated with the intestinal mucosa of farmed rainbow trout (Oncoryhnchus mykiss Walbaum). Aquac Res. (2009) 40:1064–72. doi: 10.1111/j.1365-2109.2009.02200.x
78. Qin JJ, Li RQ, Raes J, Arumugam M, Burgdorf KS, Manichanh C, et al. A human gut microbial gene catalogue established by metagenomic sequencing. Nature. (2010) 464:59–U70. doi: 10.1038/nature08821
79. Pond MJ, Stone DM, Alderman DJ. Comparison of conventional and molecular techniques to investigate the intestinal microflora of rainbow trout (Oncorhynchus mykiss). Aquaculture. (2006) 261:194–203. doi: 10.1016/j.aquaculture.2006.06.037
80. Duan YH, Li FN, Liu HN, Li YH, Liu YY, Kong XF, et al. Nutritional and regulatory roles of leucine in muscle growth and fat reduction. Front Biosci Landmark. (2015) 20:796–813. doi: 10.2741/4338
81. Jewell JL, Guan KL. Nutrient signaling to mTOR and cell growth. Trends Biochem Sci. (2013) 38:233–42. doi: 10.1016/j.tibs.2013.01.004
82. Liu ZX, Zhang JD, Liu XY, Li HH, Wang KZ, Chen L, et al. Molecular characterization and expression research of oligopeptide transporter PepT1 in Siniperca chuatsi. Acta Hydrobiol Sin. (2014) 38:556–62. doi: 10.7541/2014.78
83. Xu XR, Liu CQ, Feng BS, Liu ZJ. Dysregulation of mucosal immune response in pathogenesis of inflammatory bowel disease. World J Gastroentero. (2014) 20:3255–64. doi: 10.3748/wjg.v20.i12.3255
84. Kwasek K, Terova G, Wojno M, Dabrowski K, Wick M. The effect of dietary dipeptide lysine-glycine on growth, muscle proteins, and intestine PepT1 gene expression in juvenile yellow perch. Rev Fish Biol Fisher. (2012) 22:797–812. doi: 10.1007/s11160-012-9266-6
85. Zhou P, Luo YQ, Zhang L, Li JL, Zhang BL, Xing S, et al. Effects of cysteamine supplementation on the intestinal expression of amino acid and peptide transporters and intestinal health in finishing pigs. Anim Sci J. (2017) 88:314–21. doi: 10.1111/asj.12626
86. Wenzel U, Meissner B, Doring F, Daniel H. PEPT1-mediated uptake of dipeptides enhances the intestinal absorption of amino acids via transport system b(0,+). J Cell Physiol. (2001) 186:251–9. doi: 10.1002/1097-4652(200102)186:23.0.CO;2-F
87. Gilbert ER, Wong EA, Webb KE. Board-invited review: peptide absorption and utilization: implications for animal nutrition and health. J Anim Sci. (2008) 86:2135–55. doi: 10.2527/jas.2007-0826
Keywords: growth performance, amino acid and peptide transporters, enzyme-digested poultry by-product meal, intestinal microbiota, non-specific immunity, hybrid grouper
Citation: Yang X, Zhao X, Wang G, Dong X, Yang Q, Liu H, Zhang S, Tan B and Chi S (2022) Improvement of hybrid grouper (Epinephelus fuscoguttatus ♀ × E. lanceolatus ♂) by enzyme-digested poultry by-product: Growth performance, amino acid and peptide transport capacity, and intestinal morphology. Front. Nutr. 9:955734. doi: 10.3389/fnut.2022.955734
Received: 29 May 2022; Accepted: 27 June 2022;
Published: 19 July 2022.
Edited by:
Abraham Wall-Medrano, Universidad Autónoma de Ciudad Juárez, MexicoReviewed by:
Victor Ortiz, Instituto Nacional de Ciencias Médicas y Nutrición Salvador Zubirán (INCMNSZ), MexicoGabriela Alemán, Instituto Nacional de Ciencias Médicas y Nutrición Salvador Zubirán (INCMNSZ), Mexico
Copyright © 2022 Yang, Zhao, Wang, Dong, Yang, Liu, Zhang, Tan and Chi. This is an open-access article distributed under the terms of the Creative Commons Attribution License (CC BY). The use, distribution or reproduction in other forums is permitted, provided the original author(s) and the copyright owner(s) are credited and that the original publication in this journal is cited, in accordance with accepted academic practice. No use, distribution or reproduction is permitted which does not comply with these terms.
*Correspondence: Beiping Tan, YnB0YW5AMTI2LmNvbQ==; Shuyan Chi, Y2hpc2h1eWFuNzdAMTYzLmNvbQ==