- 1Department of Orthopedic Surgery, Fujian Medical University Union Hospital, Fuzhou, China
- 2Department of Ophthalmology, Fujian Provincial Hospital, Fuzhou, China
- 3Department of Emergency, Zhaotong Traditional Chinese Medicine Hospital, Zhaotong, China
Background: Iron deficiency or overload may contribute to complications associated with diseases, but the link between iron status and skeletal muscle disorder is poorly understood. This study aimed to investigate the relationship between serum iron status, reflected by serum ferritin concentration, and muscle mass in U.S. adults.
Methods: We utilized data from National Health and Nutrition Examination Survey (NHANES) 2015-2018 for analysis. Data on serum ferritin, appendicular skeletal muscle mass (ASM), body mass index (BMI) and confounding factors were extracted and analyzed. Multivariate linear regression analyses and smooth curve fittings were employed to investigate the association between serum ferritin and muscle mass. Subgroup analysis based on iron status, age, gender and race were performed.
Results: A total of 2,078 participants were included, and divided into iron deficiency (n = 225), normal iron status (n = 1,366), and iron overload (n = 487) groups. Participants with iron overload had significantly lower ASM and appendicular skeletal muscle index (ASMI) (ASM: 19.329 ± 4.879, ASMI: 0.709 ± 0.138) compared to those with iron deficiency (ASM: 22.660 ± 6.789, ASMI: 0.803 ± 0.206) and normal iron status (ASM: 22.235 ± 6.167, ASMI: 0.807 ± 0.201). The serum ferritin was negatively linked with muscle mass after adjusting for potential confounders (β = −0.0001, 95% CI: −0.0001, −0.0000). When stratified by iron status, the trend test between them remained significant (P for trend: 0.008). Furthermore, subgroup analysis identified a stronger association in men (β = −0.0001, 95% CI: −0.0002, −0.0001), age ≥ 40 years (β = −0.0001, 95% CI: −0.0002, −0.0000), non-Hispanic black (β = −0.0002, 95% CI: −0.0003, −0.0001) and other races (β = −0.0002, 95% CI: −0.0003, −0.0000).
Conclusions: Our study revealed an inverse relationship between serum iron status and muscle mass in adults. This finding improves our understanding of the impact of serum iron status on muscle mass, and sheds new light on the prevention and treatment of muscle loss.
Introduction
Aging is a progressive, deleterious process that adversely affects the function of multiple organ systems (1). The effects of aging in skeletal muscle have been characterized by decline of muscle mass, strength and function, which is commonly referred to as sarcopenia (2). It is well accepted that sarcopenia is closely related with adverse outcomes, such as falls, fractures, disability, frailty, and mortality (2, 3).
Although the epidemiology and clinical manifestations of sarcopenia are much better understood, its pathogenesis has not been fully elucidated (4). Recently, the role of minerals in muscle metabolism has generated much attention. A number of studies have confirmed the relationships between magnesium, selenium, calcium and skeletal muscle disorder (5–7). Iron is an essential trace element, which plays a pivotal role in cellular metabolism, survival and proliferation (8). Several studies reported that iron deficient caused anemia, neurocognitive dysfunction, and impaired functional capacity (9, 10), whereas iron overload resulted in osteoporosis, neurodegeneration, and cardiovascular diseases (11–13). In the field of skeletal muscle research, recent studies also demonstrated an association between iron status and muscle disorders in experimental animals (14, 15), but the link between serum iron status and muscle mass in human is poorly understood. Therefore, we performed this study to investigate the relationship between serum iron status, reflected by serum ferritin concentration, and muscle mass in U.S. adults.
Materials and Methods
Data Source
The National Health and Nutrition Examination Survey (NHANES) is a cross-sectional survey, which provides a large amount of information on the nutrition and health of the non-institutionalized U.S. population. The NHANES protocols are approved by the Ethic Review Board of the National Center for Health Statistics, and all participants have consented to the use of their information for research (16).
Study Population
For this study, we utilized publicly available data from 2 two-year cycles (2015–2016 and 2017–2018) of NHANES for analysis. The inclusion criteria were as follows: participants aged ≥20 years old, with complete data of ferritin, ASM and BMI, without factors (inflammation, infection, malignancy, etc.) that might influence serum iron status. We excluded participants who received anemia treatment (n = 619), with a liver condition (n = 264), a malignancy (n = 1,007), infection (white blood cell count >10 × 109/L; n = 1,513), inflammation (CRP > 5 mg/L; n = 1,715), pregnant (n = 51), age <20 years old (n = 6,947), with missing data of ferritin (n = 3,291), ASM (n = 1,734) and BMI (n = 6). (The participant selection flow-chart is demonstrated in Figure 1). For each included participants, data on ferritin, ASM, BMI and confounding factors were extracted and aggregated.
Study Variables
Muscle Mass
The lean soft tissue measurement was conducted using whole-body dual-energy x-ray absorptiometry (DXA) scans (Hologic, Inc., Bedford, Massachusetts). ASM (g) was calculated as the sum of lean soft tissue mass of both arms and legs. BMI (kg/m2) was defined as weight divided by height2. We further quantified muscle mass using ASMI, calculated as the ASM divided by BMI (17).
Serum Ferritin
The serum ferritin (ug/L) was measured using electrochemiluminescence immunoassay method. Serum iron status was classified according to WHO guideline: males and females with serum ferritin <15 ug/L were defined as iron deficiency; females with serum ferritin >150 ug/L and males with serum ferritin >200 ug/L were defined as iron overload; other serum ferritin levels were considered as normal iron status (18).
Other Covariates
According to literatures, the following variables were regarded as covariates. Demographic variables including age, sex (Male, Female), race (Hispanic, Non-Hispanic White, Non-Hispanic Black, and Other Races), education (< High school, High school, >High school) were collected from demographic data. The medical conditions including hypertension and diabetes were defined by the participants' self-reported physician's diagnosis. The serum albumin (g/dL), globulin (g/dL), AST (IU/L), total protein (g/dL), uric acid (mg/dL) were measured using Beckman UniCel® DxC 800 Synchron & Beckman UniCel® DxC 660i Synchron Access Clinical Systems (Identical Method). White blood cell counts (1,000 cells/uL) and hemoglobin (g/dL) measurement were performed using the Coulter® DxH 800 analyzer. High sensitivity C-Reactive Protein (HS-CRP) was measured using Beckman UniCel® DxC 600 Synchron & Beckman UniCel® DxC 660i Synchron Access Clinical Systems (Identical Method). Details of the specimen collection, processing, quality assurance and monitoring are available at NHANES website.
Statistical Analysis
All analyses were performed using R 3.4.3 (https://www.r-project.org/) and EmpowerStats software (http://www.empowerstats.com), with P < 0.05 considered to be statistically significant. Sample weights were taken into account for calculating all estimates. Weighted linear regression models (for continuous variables) and weighted chi-square tests (for categorical variables) were performed to compare the baseline characteristics. Weighted multivariable linear regression analysis was performed to estimate the relationship between serum ferritin and ASMI. Subgroup analysis stratified by iron status, age, gender, and race were also performed. Furthermore, smooth curve fitting was applied to examine linear or non-linear relationship between them. If a non-linear relationship was identified, threshold effect analysis was conducted using two-piecewise linear regression model.
Results
Based on the inclusion and exclusion criteria, a total of 2,078 participants were included for final analysis. As shown in Table 1, participants were categorized according to their iron status. There were 225 participants with iron deficiency, 1,366 participants with normal iron status, 487 participants with iron overload. Participants with iron overload had significantly lower serum uric acid, hemoglobin, HS-CRP, ASM, ASMI, and higher white blood cell count and ferritin when compared to the other groups.
Association Between Serum Ferritin and Muscle Mass
Table 2 shows the results of the multivariate linear regression analysis. The serum ferritin was negatively linked with muscle mass in the unadjusted model (model 1: β = −0.0002, 95%CI: −0.0003, −0.0001). After adjusting for covariates, this significant association was remained apparent in the fully adjusted model (model 3: β = −0.0001, 95%CI: −0.0001, −0.0000), but not in partially adjusted model (model 2: β = −0.0000, 95%CI: −0.0000, 0.0000). Similarly, when stratified by iron status, the trend test between them remained significant in unadjusted model and fully adjusted model (P for trend <0.05).
In subgroup analysis stratified by age, this negative association was maintained in participants ≥40 years old (model 1: β = −0.0002, 95%CI: −0.0003, −0.0002 and model 3: β = −0.0001, 95%CI: −0.0002, −0.0000), but not in participants <40 years old (model 2: β = 0.0000, 95%CI: −0.0000, 0.0001 and model 3: β = −0.0000, 95%CI: −0.0001, 0.0000). When stratified by gender, the significant association was maintained in men (all three models), but not in women (all three models). When stratified by race, significantly negative associations were observed only in Non-Hispanic Black and Other Races (all three models) (Table 3).
Threshold Effect Analysis
Generalized additive models and smooth curve fittings used to detect the non-linear relationship between serum ferritin and muscle mass are shown in Figures 2, 3. The results demonstrated linear relationships between ferritin and muscle mass in participants ≥40 years old (Figure 3A) and men (Figure 3B), and non-linear relationships in participants <40 years old (Figure 3A), Hispanic and Non-Hispanic Black (Figure 3C). Among participants <40 years old and Hispanics, the relationship between ferritin and muscle mass was a U-shape curve, with the infection point identified at 231 ug/L in participants <40 years old and 223 ug/L in Hispanics (Table 4).
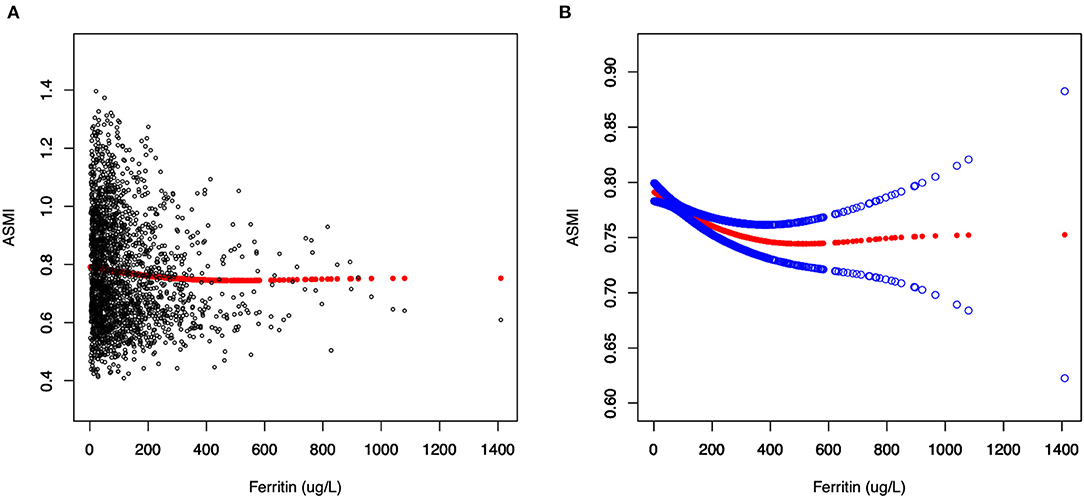
Figure 2. The relationship between serum ferritin and muscle mass. (A) Each black point represents a sample. (B) Solid red line represents the smooth curve fit between variables. Blue bands represent the 95% of confidence interval from the fit.
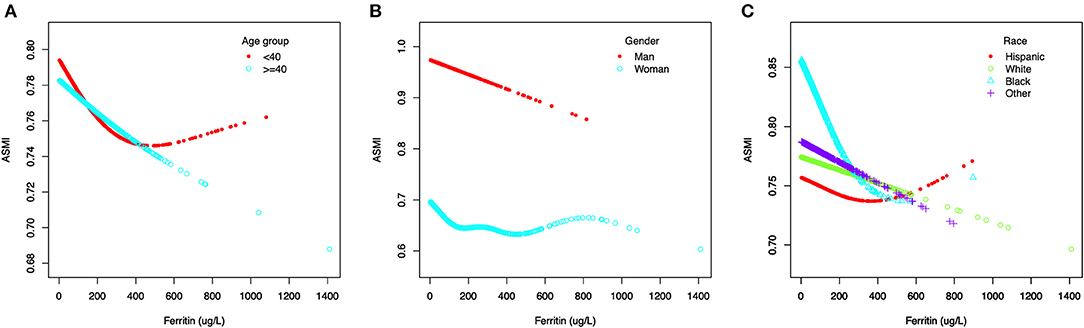
Figure 3. The association between serum ferritin and muscle mass, stratified by age (A), stratified by gender (B), stratified by race (C).
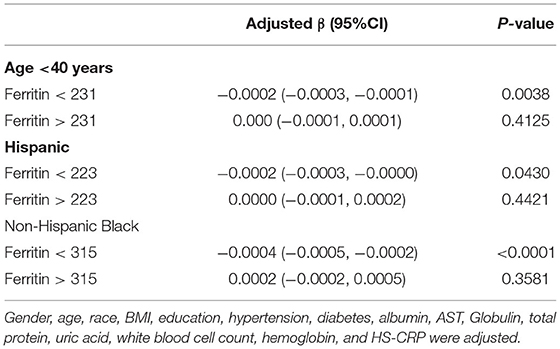
Table 4. Threshold effect analysis of ferritin on ASMI in age <40 years, Hispanic and Non-Hispanic Black using the two-piecewise linear regression model.
Discussion
Muscle loss is the main feature of sarcopenia, which contributes to increased risk of fractures, impaired physical function, and reduced quality of life (19). Although remarkable strides have been made in understanding its etiology and pathogenesis, little is known about the relationship between serum iron status and muscle mass. The findings of our study demonstrated a significantly negative association between serum ferritin and muscle mass, even after adjusting for potential confounders. The association was stronger in men, age ≥40 years, non-Hispanic black and other races.
As an essential micronutrient, iron plays crucial roles in various biological processes, including enzymatic activities, mitochondrial function, energy metabolism, RNA and DNA synthesis (20, 21). However, due to a lack of effective excretory route, iron accumulation occurs easily during aging process (22). There is growing evidence that excess iron is significantly correlated with multiple diseases, including liver fibrosis, heart attack, neurodegeneration, and cancer (11, 23). In regarding to musculoskeletal system, several experimental studies also detected a link between iron accumulation and muscle disorders. Altun et al. (24) and Jung et al. (25) found a significantly elevated non-heme iron level in the skeletal muscle of aged rats. Xu et al. (26) indicated the iron accumulation might be considered as a hallmark of aged skeletal muscle. In another study, Reardon et al. demonstrated that intraperitoneal administration of iron to mice caused not only an increased oxidative stress within muscle tissue, but also a significantly decreased muscle force (15). Furthermore, Huang et al. (14) revealed that ferroptosis, an iron-dependent form of programmed cell death, triggered by iron accumulation might be a primary cause of muscle loss. Taken together, these experimental results strongly suggested that iron accumulation might be a potential contributor to the decline of muscle mass and function.
In contrast to experimental studies, the results of clinical studies remain controversial. While Bartali et al. (27) did not find a significant association between serum iron level and physical function in a longitudinal study involving 698 participants. Waters et al. (28) indicated a significant association between iron intake and gait speed in 315 older adults. Nakagawa et al. (29) reported that higher serum ferritin, even mildly, was significantly correlated in an inverse manner with lower muscle strength in 300 hemodialysis patients. In another cohort study involving 639 hospitalized elderly, Perna et al. (30) revealed a significantly higher serum ferritin level in sarcopenia group compared with the health controls. Furthermore, the serum ferritin levels exceeded the normal range (>145 ug/dl) in patients with sarcopenia (30). Our study enrolled a larger community-dwelling healthy participants, the result showed a significantly negative association between serum ferritin and muscle mass. Moreover, such association was influenced by gender, age, and ethnicity, with stronger associations among men, age ≥40 years, non-Hispanic black and other races.
Although the above experimental and clinical findings demonstrated a negative relationship between iron status and muscle mass, the underlying mechanisms remain to be fully elucidated. There is growing evidence that elevated reactive oxygen species (ROS) and increased local oxidative stress caused by excess iron may play an important role. In one hand, ROS can cause lipids, proteins, mitochondria, and nucleic acids damage, finally resulting in cellular dysfunction and ferroptosis (14, 24, 31). On the other hand, oxidative stress may lead to muscle tissue damage by increasing expression of inflammatory cytokines, such as interleukin-1 (IL-1), tumor necrosis factor-α (TNF-α), and IL-6 (32). Furthermore, several studies indicated the iron accumulation and insulin resistance were interconnected. It has been established that insulin resistance is closely linked to muscle loss (23, 33).
Limitation
There were some limitations in our study. Firstly, due to the cross-sectional nature of this study, no causal relationship between serum ferritin and muscle loss could be concluded. Secondly, data on muscle strength and function were not available in this study, so the relationships between these variables and ferritin could not be investigated. Finally, whole body DXA scans were only performed to participants aged 8–59 years old in NHANES, there was a lack of research on elderly participants who were more likely to suffer from muscle loss. Therefore, more research and exploration in this field are still needed.
Conclusion
The present study revealed an inverse relationship between serum iron status and muscle mass in community-dwelling adults, especially in men, age ≥40 years, non-Hispanic black and other races. This finding improves our understanding of the impact of serum iron status on muscle mass, and sheds new light on the prevention and treatment of muscle loss.
Data Availability Statement
The original contributions presented in the study are included in the article/supplementary material, further inquiries can be directed to the corresponding author.
Ethics Statement
The studies involving human participants were reviewed and approved by the National Center for Health Statistics Ethics Review Board. The patients/participants provided their written informed consent to participate in this study.
Author Contributions
ZC wrote the manuscript. ZC, JC, CS, and JS collected and analyzed the data. WL designed this study and revised the manuscript. All authors read and approved the final manuscript.
Conflict of Interest
The authors declare that the research was conducted in the absence of any commercial or financial relationships that could be construed as a potential conflict of interest.
Publisher's Note
All claims expressed in this article are solely those of the authors and do not necessarily represent those of their affiliated organizations, or those of the publisher, the editors and the reviewers. Any product that may be evaluated in this article, or claim that may be made by its manufacturer, is not guaranteed or endorsed by the publisher.
References
1. Pokkunuri I, Ali Q, Asghar M. Grape powder improves age-related decline in mitochondrial and kidney functions in fischer 344 rats. Oxid Med Cell Longev. (2016) 2016:6135319. doi: 10.1155/2016/6135319
2. Cruz-Jentoft AJ, Sayer AA. Sarcopenia. Lancet. (2019) 393:2636–46. doi: 10.1016/S0140-6736(19)31138-9
3. Bloom I, Shand C, Cooper C, Robinson S, Baird J. Diet quality and sarcopenia in older adults: a systematic review. Nutrients. (2018) 10:308. doi: 10.3390/nu10030308
4. Cruz-Jentoft AJ, Bahat G, Bauer J, Boirie Y, Bruyère O, Cederholm T, et al. Sarcopenia: revised European consensus on definition and diagnosis. Age Ageing. (2019) 48:601. doi: 10.1093/ageing/afz046
5. van Dronkelaar C, van Velzen A, Abdelrazek M, van der Steen A, Weijs PJM, Tieland M. Minerals and sarcopenia; the role of calcium, iron, magnesium, phosphorus, potassium, selenium, sodium, and zinc on muscle mass, muscle strength, and physical performance in older adults: a systematic review. J Am Med Dir Assoc. (2018) 19:6–11. doi: 10.1016/j.jamda.2017.05.026
6. Chen YL, Yang KC, Chang HH, Lee LT, Lu CW, Huang KC. Low serum selenium level is associated with low muscle mass in the community-dwelling elderly. J Am Med Dir Assoc. (2014) 15:807–11. doi: 10.1016/j.jamda.2014.06.014
7. Hayhoe RPG, Lentjes MAH, Mulligan AA, Luben RN, Khaw KT, Welch AA. Cross-sectional associations of dietary and circulating magnesium with skeletal muscle mass in the EPIC-Norfolk cohort. Clin Nutr. (2019) 38:317–23. doi: 10.1016/j.clnu.2018.01.014
8. Zheng Q, Guan Y, Xia L, Wang Z, Jiang Y, Zhang X, et al. Effect of yi gong san decoction on iron homeostasis in a mouse model of acute inflammation. Evid Based Complement Alternat Med. (2016) 2016:2696480. doi: 10.1155/2016/2696480
9. Gattermann N, Muckenthaler MU, Kulozik AE, Metzgeroth G, Hastka J. The evaluation of iron deficiency and iron overload. Dtsch Arztebl Int. (2021) 118:847–56. doi: 10.3238/arztebl.m2021.0290
10. Doehner W, Scherbakov N, Schellenberg T, Jankowska EA, Scheitz JF, von Haehling S, et al. Iron deficiency is related to low functional outcome in patients at early rehabilitation after acute stroke. J Cachexia Sarcopenia Muscle. (2022) 13:1036–44. doi: 10.1002/jcsm.12927
11. Kadoglou NPE, Biddulph JP, Rafnsson SB, Trivella M, Nihoyannopoulos P, Demakakos P. The association of ferritin with cardiovascular and all-cause mortality in community-dwellers: the English longitudinal study of ageing. PLoS ONE. (2017) 12:e0178994. doi: 10.1371/journal.pone.0178994
12. Mena NP, Urrutia PJ, Lourido F, Carrasco CM, Núñez MT. Mitochondrial iron homeostasis and its dysfunctions in neurodegenerative disorders. Mitochondrion. (2015) 21:92–105. doi: 10.1016/j.mito.2015.02.001
13. Li GF, Pan YZ, Sirois P, Li K, Xu YJ. Iron homeostasis in osteoporosis and its clinical implications. Osteoporos Int. (2012) 23:2403–8. doi: 10.1007/s00198-012-1982-1
14. Huang Y, Wu B, Shen D, Chen J, Yu Z, Chen C. Ferroptosis in a sarcopenia model of senescence accelerated mouse prone 8 (SAMP8). Int J Biol Sci. (2021) 17:151–62. doi: 10.7150/ijbs.53126
15. Reardon TF, Allen DG. Iron injections in mice increase skeletal muscle iron content, induce oxidative stress and reduce exercise performance. Exp Physiol. (2009) 94:720–30. doi: 10.1113/expphysiol.2008.046045
16. Wolffenbuttel BHR, Heiner-Fokkema MR, Green R, Gans ROB. Relationship between serum B12 concentrations and mortality: experience in NHANES. BMC Med. (2020) 18:307. doi: 10.1186/s12916-020-01771-y
17. Studenski SA, Peters KW, Alley DE, Cawthon PM, McLean RR, Harris TB, et al. The FNIH sarcopenia project: rationale, study description, conference recommendations, and final estimates. J Gerontol A Biol Sci Med Sci. (2014) 69:547–58. doi: 10.1093/gerona/glu010
18. WHO Guidelines Approved by the Guidelines Review Committee. WHO Guideline on Use of Ferritin Concentrations to Assess Iron Status in Individuals and Populations. Geneva: World Health Organization (2020).
19. Chen LK, Liu LK, Woo J, Assantachai P, Auyeung TW, Bahyah KS, et al. Sarcopenia in Asia: consensus report of the Asian working group for sarcopenia. J Am Med Dir Assoc. (2014) 15:95–101. doi: 10.1016/j.jamda.2013.11.025
20. Jing X, Du T, Li T, Yang X, Wang G, Liu X, et al. The detrimental effect of iron on OA chondrocytes: Importance of pro-inflammatory cytokines induced iron influx and oxidative stress. J Cell Mol Med. (2021) 25:5671–80. doi: 10.1111/jcmm.16581
21. Katsura Y, Ohara T, Noma K, Ninomiya T, Kashima H, Kato T, et al. A novel combination cancer therapy with iron chelator targeting cancer stem cells via suppressing stemness. Cancers. (2019) 11:177. doi: 10.3390/cancers11020177
22. Zhao G. Is iron accumulation a possible risk factor for sarcopenia? Biol Trace Elem Res. (2018) 186:379–83. doi: 10.1007/s12011-018-1332-z
23. Halon-Golabek M, Borkowska A, Herman-Antosiewicz A, Antosiewicz J. Iron metabolism of the skeletal muscle and neurodegeneration. Front Neurosci. (2019) 13:165. doi: 10.3389/fnins.2019.00165
24. Altun M, Edström E, Spooner E, Flores-Moralez A, Bergman E, Tollet-Egnell P, et al. Iron load and redox stress in skeletal muscle of aged rats. Muscle Nerve. (2007) 36:223–33. doi: 10.1002/mus.20808
25. Jung SH, DeRuisseau LR, Kavazis AN, DeRuisseau KC. Plantaris muscle of aged rats demonstrates iron accumulation and altered expression of iron regulation proteins. Exp Physiol. (2008) 93:407–14. doi: 10.1113/expphysiol.2007.039453
26. Xu J, Knutson MD, Carter CS, Leeuwenburgh C. Iron accumulation with age, oxidative stress and functional decline. PLoS ONE. (2008) 3:e2865. doi: 10.1371/journal.pone.0002865
27. Bartali B, Frongillo EA, Guralnik JM, Stipanuk MH, Allore HG, Cherubini A, et al. Serum micronutrient concentrations and decline in physical function among older persons. JAMA. (2008) 299:308–15. doi: 10.1001/jama.299.3.308
28. Waters DL, Wayne SJ, Andrieu S, Cesari M, Villareal DT, Garry P, et al. Sexually dimorphic patterns of nutritional intake and eating behaviors in community-dwelling older adults with normal and slow gait speed. J Nutr Health Aging. (2014) 18:228–33. doi: 10.1007/s12603-014-0004-8
29. Nakagawa C, Inaba M, Ishimura E, Yamakawa T, Shoji S, Okuno S. Association of increased serum ferritin with impaired muscle strength/quality in hemodialysis patients. J Renal Nutr. (2016) 26:253–7. doi: 10.1053/j.jrn.2016.01.011
30. Perna S, Peroni G, Faliva MA, Bartolo A, Naso M, Miccono A, et al. Sarcopenia and sarcopenic obesity in comparison: prevalence, metabolic profile, and key differences. a cross-sectional study in Italian hospitalized elderly. Aging Clin Exp Res. (2017) 29:1249–58. doi: 10.1007/s40520-016-0701-8
31. Rondanelli M, Faliva M, Monteferrario F, Peroni G, Repaci E, Allieri F, et al. Novel insights on nutrient management of sarcopenia in elderly. Biomed Res Int. (2015) 2015:524948. doi: 10.1155/2015/524948
32. Schaap LA, Pluijm SM, Deeg DJ, Visser M. Inflammatory markers and loss of muscle mass (sarcopenia) and strength. Am J Med. (2006) 119:526.e529–17. doi: 10.1016/j.amjmed.2005.10.049
Keywords: ferritin, iron, skeletal muscle, NHANES, sarcopenia
Citation: Chen Z, Chen J, Song C, Sun J and Liu W (2022) Association Between Serum Iron Status and Muscle Mass in Adults: Results From NHANES 2015–2018. Front. Nutr. 9:941093. doi: 10.3389/fnut.2022.941093
Received: 11 May 2022; Accepted: 22 June 2022;
Published: 11 July 2022.
Edited by:
Juan Mielgo-Ayuso, University of Burgos, SpainReviewed by:
Jeanette Mary Andrade, University of Florida, United StatesJasenka Gajdoš Kljusurić, University of Zagreb, Croatia
Copyright © 2022 Chen, Chen, Song, Sun and Liu. This is an open-access article distributed under the terms of the Creative Commons Attribution License (CC BY). The use, distribution or reproduction in other forums is permitted, provided the original author(s) and the copyright owner(s) are credited and that the original publication in this journal is cited, in accordance with accepted academic practice. No use, distribution or reproduction is permitted which does not comply with these terms.
*Correspondence: Wenge Liu, bHdnc3BpbmVAMTI2LmNvbQ==
†These authors have contributed equally to this work