- 1Clinical Nutrition and Metabolism, Department of Public Health and Caring Sciences, Faculty of Medicine, Uppsala University, Uppsala, Sweden
- 2Steno Diabetes Center Aarhus, Aarhus University Hospital, Aarhus, Denmark
- 3Department of Clinical Medicine, Aarhus University, Aarhus, Denmark
- 4The George Institute for Global Health, Faculty of Medicine, UNSW Sydney, Sydney, NSW, Australia
- 5Department of Epidemiology, Johns Hopkins Bloomberg School of Public Health, Johns Hopkins University, Baltimore, MD, United States
Background: High carbohydrate, i.e., sugars, intake potentially drives the liver into a lipogenic state leading to elevated plasma fatty acids. Excessive intake of saturated fat and sugar-sweetened soda induces liver fat accumulation, but studying the effect of high intake from sugar-sweetened soda on the de novo lipogenesis (DNL) fatty acids in long-term randomized trials is lacking.
Objective: To study the effect of consuming 1 L/day of sugar-sweetened soda, semi-skimmed milk (milk), aspartame-sweetened soda or water over 24 weeks on DNL-derived fatty acids (i.e., palmitate (primary outcome) and other saturated and monounsaturated fatty acids), and markers of stearoyl-CoA desaturase activity (SCD1) in plasma phospholipids (PL), cholesteryl esters (CE), and triglycerides (TG).
Design and methods: A randomized parallel study was conducted simultaneously at Aarhus University Hospital and Copenhagen University, Denmark, including (n = 41) individuals aged 20–50 years, with BMI of 26–40 kg/m2, and without diabetes. The groups consisted of 9 individuals in the sugar-sweetened soda, 10 in the milk, 11 in the aspartame-sweetened soda, and 11 in the water. The change at 24 weeks was assessed and compared across the groups using ANCOVA and mixed-effects models. Correlations of fatty acid changes with liver fat accumulation (magnetic resonance imaging) were explored.
Results: After 24 weeks, the groups differed in palmitate proportions in PL, oleate in CE and PL, and palmitoleate and SCD1 in all fractions (p < 0.05). Compared with water, the relative proportion of palmitate in PL increased by approximately 1% during both sugar-sweetened soda (p = 0.011) and milk (p = 0.006), whereas oleate and palmitoleate increased only during sugar-sweetened soda (CE 2.77%, p < 0.001; PL 1.51%, p = 0.002 and CE 1.46%, PL 0.24%, TG 1.31%, all p < 0.001, respectively). Liver fat accumulation correlated consistently with changes in palmitoleate, whereas correlations with palmitate and oleate were inconsistent across lipid fractions.
Conclusions: Although both sugar-sweetened soda and milk increased palmitate in PL, only excess intake of sugar-sweetened soda increased palmitoleate in all lipid fractions and correlated with liver fat. In contrast, isocaloric milk intake did not increase plasma monounsaturated fatty acids.
Clinical trial registration: [https://clinicaltrials.gov/ct2/show/NCT00777647], identifier [NCT00777647].
Introduction
De novo lipogenesis (DNL) is the metabolic pathway by which fatty acids are endogenously synthesized, mainly from excess intake of carbohydrates. DNL is increased in nonalcoholic fatty liver disease (NAFLD) (1, 2), whereas in healthy subjects, DNL may be upregulated due to excessive energy intake composed mainly of high carbohydrate (i.e., sugars) and low-fat diets (3). When glycogen depots in the liver and muscles are saturated, glycogenesis is inhibited, whereas DNL is stimulated in the presence of insulin to utilize plasma glucose to synthesize palmitate (16:0), the primary DNL product (4). Synthesized 16:0 can further be elongated into other saturated fatty acids (SFA), e.g., stearate (18:0), and/or desaturated by stearoyl-CoA desaturase (SCD1) to monounsaturated fatty acids (MUFA), e.g., palmitoleate (16:1n7) (5). This notion could partly explain the weak association between circulating even-chain SFA and dietary SFA, as carbohydrates might at very high intakes induce de novo synthesis of even-chain SFA and potentially increase these SFA in circulating lipids (6). Therefore, plasma 16:0 and some MUFA might reflect carbohydrate intake in certain circumstances, especially in the cases of excessive intake of sugars, as they are more lipogenic than complex carbohydrates (7). While higher circulating 16:0 and SCD1-derived MUFA levels may be potential biomarkers of excessive sugar intake (8), in addition to reflecting increased saturated fat intake (9), more studies are needed to assess the generalizability to different dietary conditions and in various populations.
Since increased 16:0 in plasma is consistently linked to an increased risk of cardiometabolic diseases (10–12), it is important to understand different dietary contributors to plasma 16:0. Previous interventional studies have had short duration (only days or a few weeks) and with different doses and types of carbohydrates and fat have yielded inconsistent results (13–16). In addition, the reported data on the effect of intake of sugar-sweetened beverages on the DNL have commonly done so in populations with a dietary pattern that is characterized by excess intake of added sugars, especially fructose (17–20). Another distinct feature of the aforementioned populations is the commercially available sugar-sweetened beverages that are typically sweetened with high-fructose corn syrup, while, on the contrary, such beverages are sweetened with sucrose, for instance, in Europe (21). Thus, whereas fructose intake has been of great interest, sugar intake per se is also of importance. In several, but not all (22), shorter-term studies, diets rich in sugar and/or sugar-sweetened beverages containing fructose were found to induce DNL in most (17, 19, 23–26), and the potential links to liver fat accumulation are unclear. Therefore, it is of interest to investigate excessive sugar intake over an extended period to understand the effects on plasma SFA and MUFA and to examine the possible link to liver fat accumulation. RCTs with long duration using sugar-sweetened soda (SS), in addition to previous short-term studies, are needed to understand responses in plasma 16:0 after excessive sugar intake from commonly consumed beverages. Such data could also be useful in interpreting fatty acid data in epidemiological and interventional studies. The aim of this secondary analysis study was to investigate the effect of high intake of SS on plasma SFA and MUFA in different lipid fractions, as compared to daily consumption of aspartame-sweetened soda (AS), semi-skimmed milk, and water over 24 weeks. We also investigated the correlation between changes in plasma fatty acids and changes in liver fat content.
Materials and methods
Study design and intervention
The study population and protocol have been described in detail previously (27). In brief, the study was undertaken simultaneously at Aarhus University Hospital and the Department of Nutrition, Exercise, and Sports, Copenhagen University, Denmark. Participants without diabetes (n = 47) with a BMI range of 26–40 kg/m2 and age between 20 and 50 years were included in the study (Table 1). Exclusion criteria included diagnosis with diabetes, hypertension, pregnancy, lactose intolerance, or phenylketonuria. Subjects were also excluded if they were smoking, breastfeeding, exercising more than 10 h/week, or using medications that affect body weight, blood glucose, or lipids. Body implants, i.e., made of metal, were an additional cause for exclusion due to the conflict with magnetic resonance imaging (MRI) scans. All participants provided their written informed consent. The Ethical Committee of Middle Jutland, Denmark, approved the study protocol, and the study was conducted according to the Declaration of Helsinki.
The study design consisted of four parallel treatment groups (Figure 1). In total, 60 subjects were randomized to the test beverages, 13 declined to participate after the allocations, leaving 47 subjects for fatty acid analyses (Figure 1). The participants were randomly assigned to consume one of the four test beverages on a daily basis for 24 weeks. The test beverages included (1 L/day) SS, semi-skimmed milk, AS, or water (Table 2). All the test beverages were provided free of charge two or three times per month by the research centers. The subjects were asked to return the empty containers to the research center every 3–4 weeks to monitor and motivate compliance. Furthermore, compliance checks were performed every 6 weeks. Subjects who were randomly assigned to consume soft drinks were provided with toothpaste, instructed about dental hygiene, and examined by a dentist at baseline and every 1.5 months throughout the intervention to check for caries or acid erosion of the enamel. None of the subjects developed these problems.
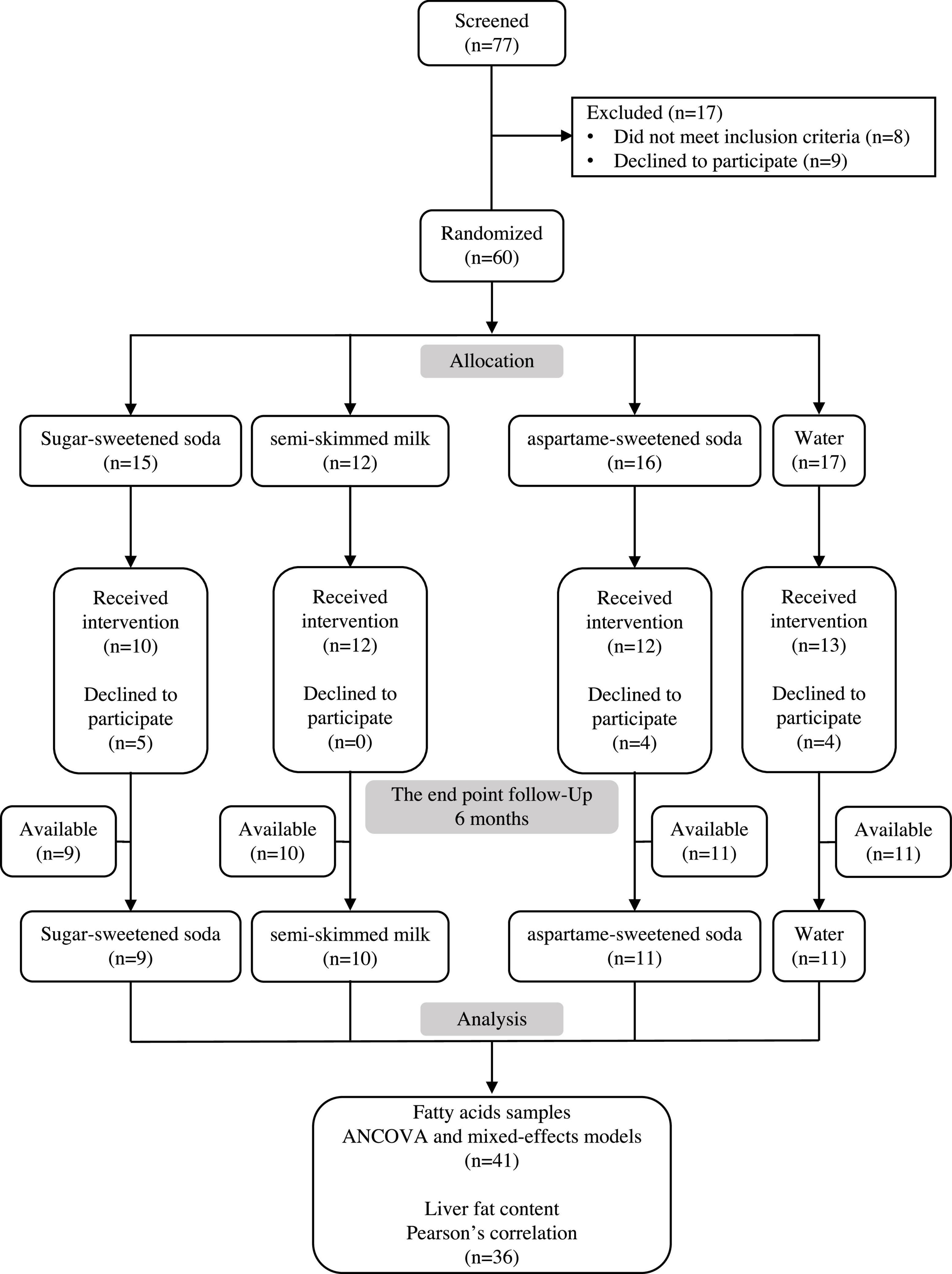
Figure 1. The consort flow diagram. Seventy-seven subjects were screened for eligibility to enroll in the study and 60 subjects were randomized.
Measurements
The composition of plasma fatty acid
Blood samples were collected in the morning after an overnight fasting. Subjects were asked to refrain from any occasional medicine, alcohol, or vigorous exercise for 24 h before the visit. Samples of blood plasma were aliquoted into several tubes and stored at –80°C. Due to the prior use of the samples in previous analyses, only 41 blood samples were available at both the baseline and end-point (24 weeks) (Figure 1). The samples were transported to the Unit of Clinical Nutrition and Metabolism, the Department of Public Health and Caring Sciences, Uppsala University, Sweden to analyze the composition of fatty acids in blood plasma. Plasma lipids were extracted using Folch’s extraction method (chloroform/methanol) (28). Thereafter, lipid fractions were separated using thin-layer chromatography (TLC) to obtain cholesteryl ester (CE), phospholipid (PL), and triglyceride (TG) fractions from the plasma. The lipid fractions were then individually extracted from the TLC plates and prepared further to assess the composition of fatty acid using the gas–liquid chromatography technique as previously described (29). Fatty acids were expressed accordingly as percentages of total fatty acids in the investigated plasma lipids. The activity of the SCD1 enzyme was estimated using a 16:1n7 to 16:0 ratio. The primary outcome was 16:0, and the secondary outcomes included myristate (14:0), the SCD1 products (16:1n7) and oleate (18:1n9), and its latter precursor (18:0).
Assessment of liver fat content
Intrahepatic fat content was assessed with MRI techniques using a Signa Excite 1.5 Tesla Twin-Speed scanner (GE Medical Systems, Milwaukee, WI, United States), as previously described in detail (27). Due to some difficulties during procedures of the MRI scan, as reported during the primary investigations, MRI data on liver fat was only available in 36 subjects.
Statistical analysis
Fatty acids distributions were evaluated, and 18:0 values were log-transformed to approximate normality before statistical analyses due to a skewed distribution. Fatty acids at the end of the intervention (i.e., after 24 weeks) were utilized to examine the difference between the groups. A completer (n = 41) analysis of covariance (ANCOVA) was computed, including group, baseline values of fatty acids, sex, body weight at the end of the study, and age as covariates. Baseline and endpoint fatty acids data were utilized in mixed-effects models with water as the reference treatment, to assess differences in changes between the reference and each of the alternative treatments. The mixed effects model included the fatty acid of interest as the dependent variable (outcome); individual as a random intercept; group, time, body weight, sex, and age as fixed effects, and an interaction term of group and time. Exploratory correlations between the change in log-transformed plasma fatty acid levels and the change in liver fat content during 24 weeks of the intervention were assessed using Pearson’s correlation coefficients (for the whole population, n = 36). All analyses were performed using Stata version 15.1 (StataCorp LCC); two-sided p-values < 0.05 were considered statistically significant.
Results
Participant characteristics
The baseline characteristics of the participants are shown in Table 1. The groups were matched for age and BMI, but there was an unequal distribution of sex between the groups. A total of 13 women dropped out after randomization; of which 4 were assigned to water, 5 to SS, and 4 to AS. A total of 47 subjects (30 women and 17 men) completed the study. Due to the prior use of the samples for analyses in previous publications, some blood samples [1 SS, 2 milk, 1 AS, and 2 water (Figure 1)] were insufficient at both the baseline and after 24 weeks, leaving 41 blood samples that have been used in the analysis of this study. At baseline, SS intake was not different between the 4 groups, and the mean intake in these subjects was 184 ml/day.
Treatment effects on fatty acids in the de novo lipogenesis pathway
After adjustments for baseline levels of the investigated fatty acids, sex, age, and body weight, the proportions of 16:0 in PL, but not in CE or TG, differed between groups (ANCOVA p = 0.026) at the end of the treatment (Table 3 and Supplementary Table 1). Compared with water, 16:0 increased in PL, but not in CE or TG, during both SS (mean difference:1.0%; 95% CI:0.2,1.8; p = 0.011) and milk (1.1%; 0.3,1.8; p = 0.006) (Figure 2 and Supplementary Table 2).
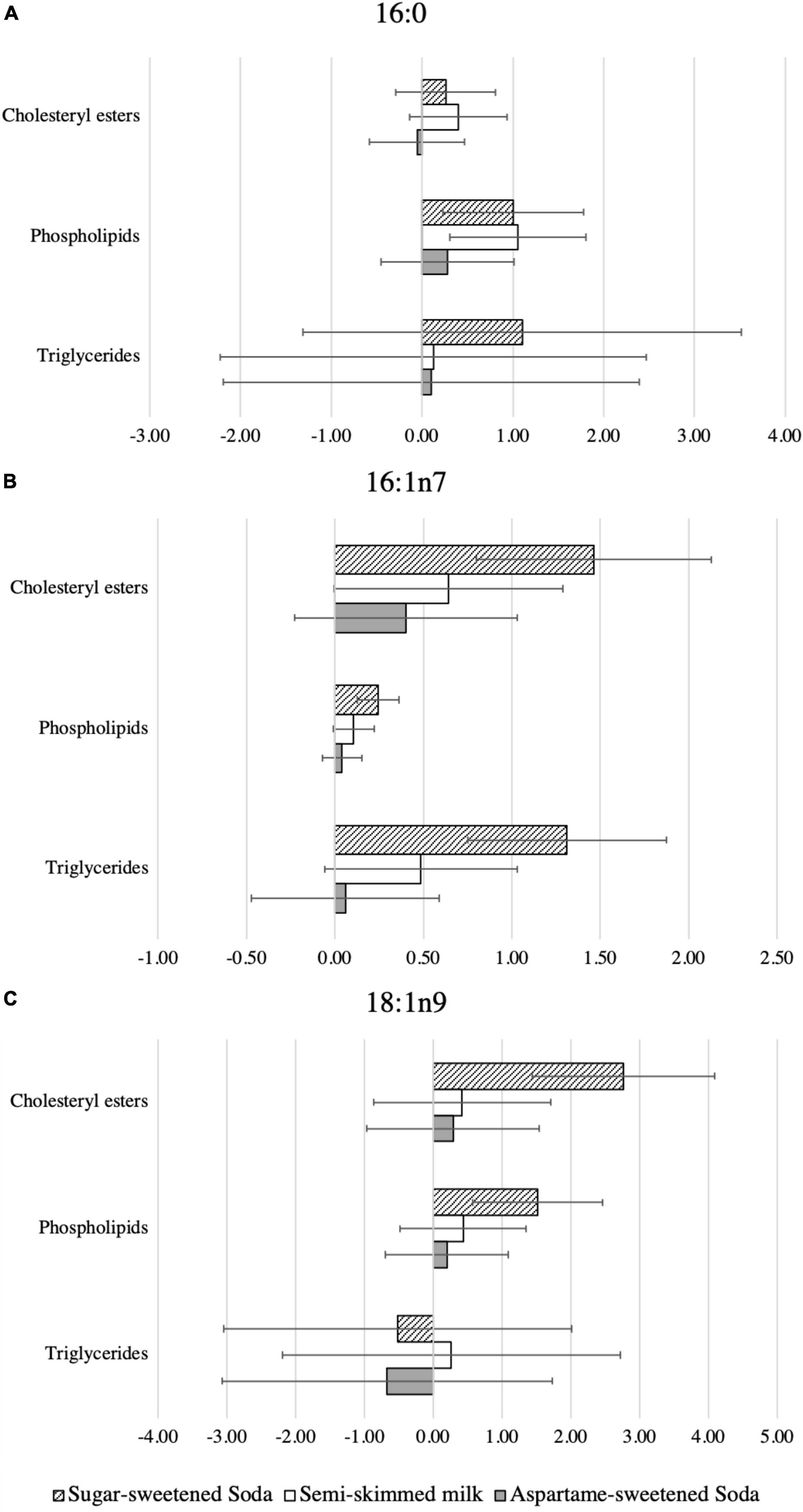
Figure 2. Data are differences in the relative changes of plasma fatty acids comparing water with each of the test beverages. Percentages of the relative changes as indicated by the mixed effects model, with each individual fatty acid as the dependent variable; individual as a random intercept; group, time, weight, sex, and age as fixed effects; and an interaction term of group and time. (A) Palmitate (16:0), (B) palmitoleate (16:1n7), and (C) oleate (18:1n9). The columns in the charts exhibit the mean values of relative change (the baseline values were subtracted from the 24 weeks values) in the levels of each one of the included fatty acids in each plasma lipid fraction for the included test beverage, in comparison to water. The distinct columns exhibit three test beverages; the diagonal pattern columns represent sugar-sweetened soda, the white columns represent semi-skimmed milk, and the gray columns represent aspartame-sweetened soda.
The 16:1n7 and SCD1 activity index differed between groups at the end of the treatment in all plasma lipid fractions (ANCOVA p ≤ 0.023) (Table 3). Compared with water, SS intake, but not milk or AS intake, increased 16:1n7 consistently across lipid fractions (Supplementary Table 2). Similarly, compared with water, SS intake increased SCD1 activity across lipid fractions, milk increased SCD1 in TG only (0.02%; 0.00, 0.03; p = 0.049), and no clear effect on SCD1 activity index in CE, PL, or TG lipid fractions was caused by AS intake (Supplementary Table 2).
Levels of 14:0 differed between groups in CE (ANCOVA p = 0.03) and compared to water, the 14:0 levels increased during both SS (0.26%; 0.12, 0.39; p < 0.001) and milk (0.22%; 0.09, 0.36; p = 0.001) (Table 3 and Supplementary Table 2). Levels of 14:0 did not differ overall greatly between the groups in the PL and TG (ANCOVA p = 0.443, and p = 0.629, respectively) (Table 3), although when compared to water, modestly higher 14:0 levels in CE were observed for the SS group (0.26%; 0.12, 0.39; p < 0.001) and the milk group (0.22%; 0.09, 0.36; p = 0.001) (Supplementary Table 2).
The increase in 18:1n9 levels in PL and CE was similar to those of 16:1n7 and SCD1 activity, although no clear treatment effect was observed in TG (Figure 2 and Supplementary Table 2).
No consistent treatment effects were observed for 18:0, although PL 18:0 decreased (–0.05%, –0.09, –0.02; p = 0.005) after milk (compared to water). Differences between groups and estimated treatment effects on other measured fatty acids are available in the online Supplementary material (Supplementary Tables 1, 2).
Exploratory correlations between fatty acids and liver fat content
Change in liver fat content was correlated with change in 16:0 in TG (r = 0.50, p = 0.002), but not in other fractions (r ≤ 0.16, p ≥ 0.34) (Figure 3 and Table 4). The change in 14:0 correlated with liver fat accumulation in CE only (Table 4), while there was no clear correlation between changes in liver fat and the change of 18:0 in any fraction. Liver fat accumulation was correlated with changes in 16:1n7 in all fractions (r ≥ 0.39, p ≤ 0.02) (Figure 3 and Table 4), whereas changes in SCD1 were correlated in CE and PL (r ≥ 0.47, p ≤ 0.004) but not in TG (r = 0.14, p = 0.43). Liver fat accumulation was correlated directly with increases in 18:1n9 in CE and PL (r ≥ 0.34, p ≤ 0.04) but surprisingly correlated inversely with the same fatty acid measured in TG (r = –0.38, p = 0.02). Correlations between changes in liver fat and changes in other measured fatty acids are presented in the online Supplementary material (Supplementary Table 3).
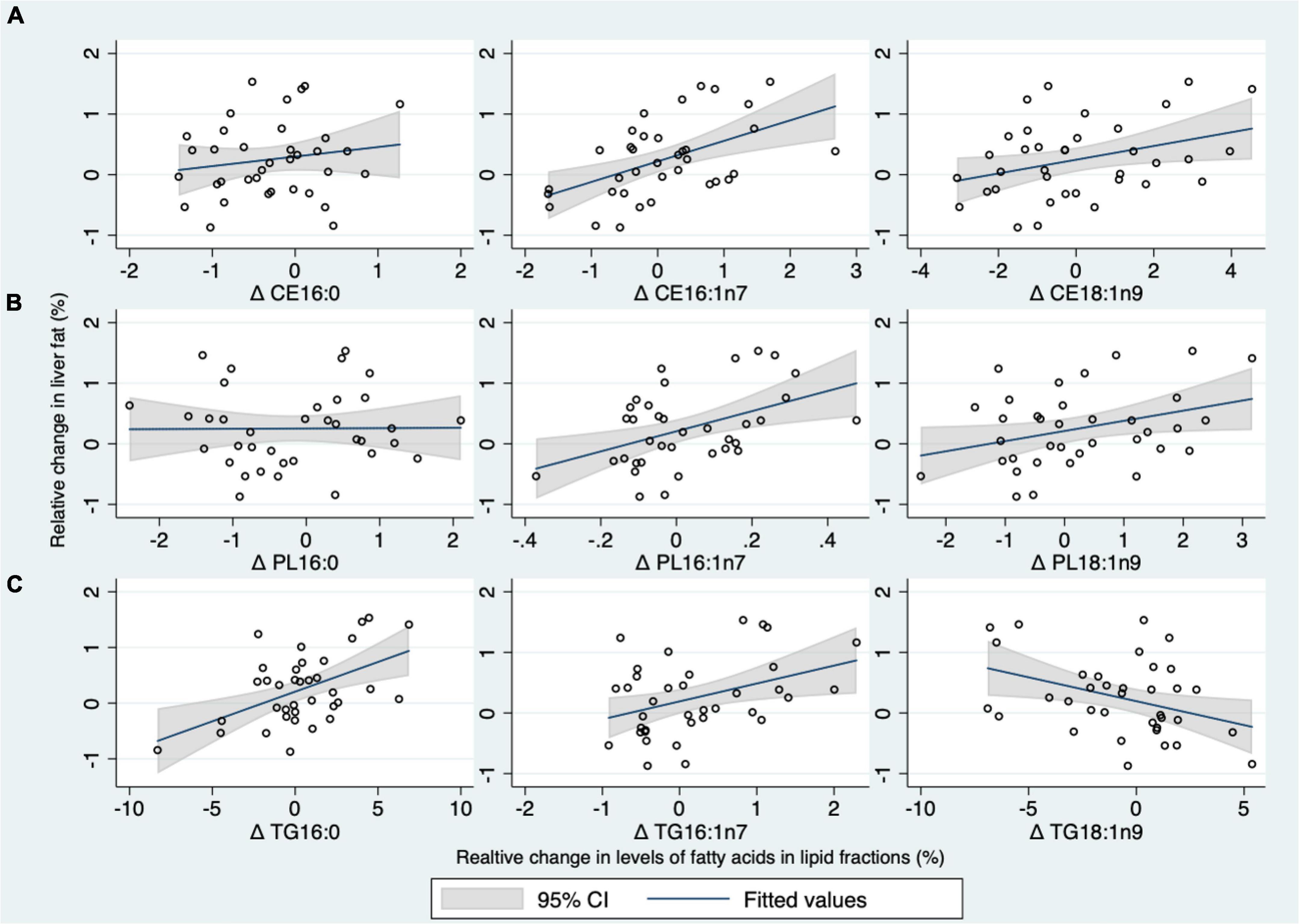
Figure 3. (A–C) Scatterplots of correlation of the relative changes (i.e., percentage points) in plasma palmitate (16:0), palmitoleate (16:1n7), oleate (18:1n9) in phospholipids (PL), cholesteryl esters (CE), and triglycerides (TG) lipid fractions with the relative changes in liver fat content after 24 weeks intervention in the whole population. The correlation of relative changes in plasma (16:0, 16:1n7, and 18:1n9) in the CE fraction with relative changes in liver fat content (A), The correlation of relative changes in plasma (16:0, 16:1n7, and 18:1n9) in the PL fraction with relative changes in liver fat content (B), and the correlation of relative changes in plasma (16:0, 16:1n7, and 18:1n9) in the TG fraction with relative changes in liver fat content (C).
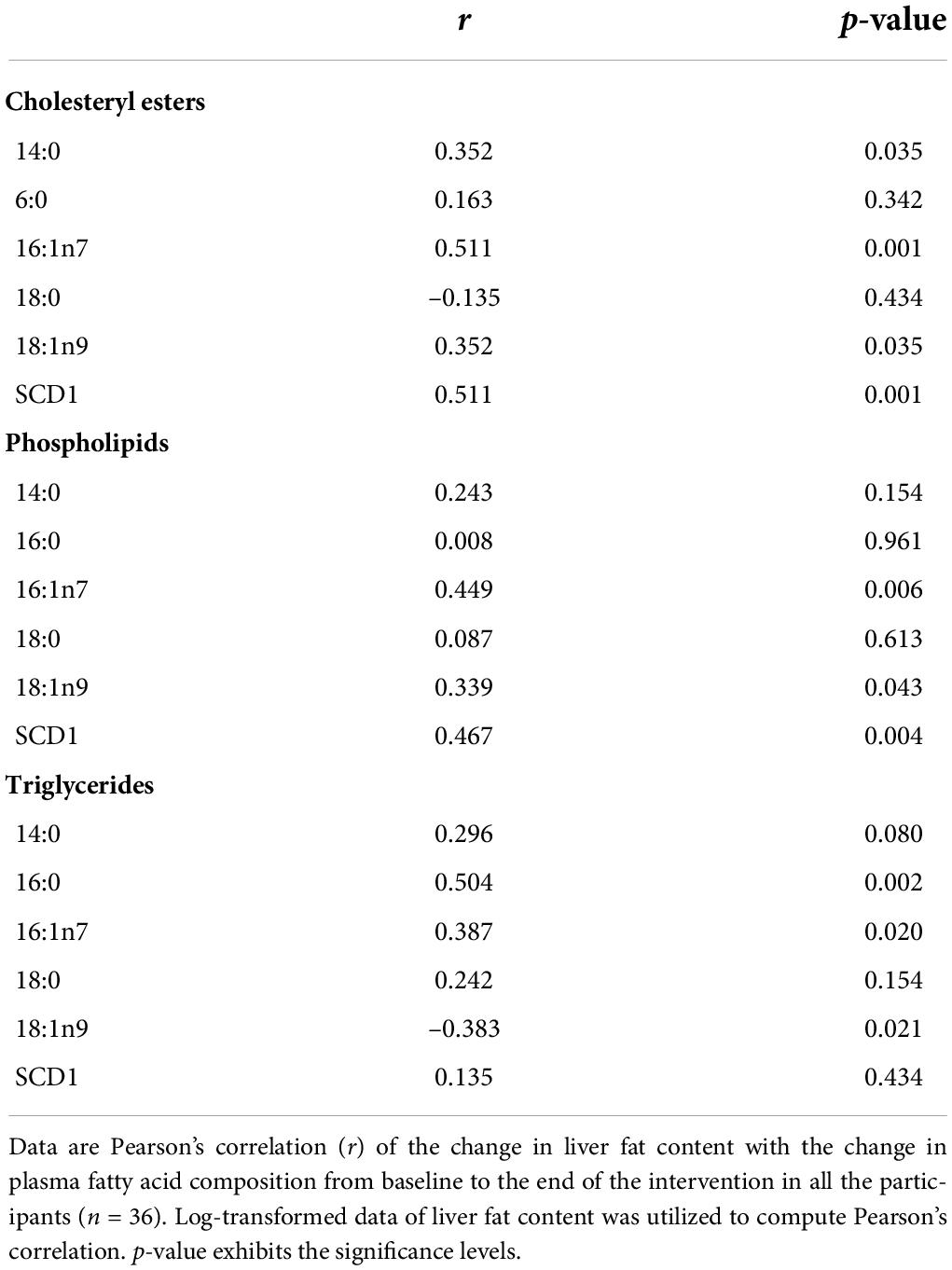
Table 4. Correlations between the change of liver fat content and the change of plasma fatty acid composition in all participants.
Discussion
In this study, intake of SS over 24 weeks increased plasma 16:0 in PL but not in CE or TG fractions. A similar increase was also evident during the intake of milk in PL compared with water. Levels of 14:0 increased in a comparable manner during intake of SS and milk in CE. Levels of 16:1n7 and SCD1 increased consistently during intake of SS in the three fractions, and 18:1n9 increased in a comparable manner in CE and PL. Thus, 16:1n7 seemed to be the most responsive fatty acid with regard to excessive intake of SS, and possibly a better marker than the direct DNL product 16:0. In addition, we demonstrate direct associations between the changes in 16:0 and 16:1n7 and liver fat accumulation, with consistent associations especially observed for 16:1n7 in all lipid fractions.
We have previously shown from this study that the relative change in liver fat was markedly higher after the daily intake of 1 L of SS for 24 weeks than after the daily intake of 1 L of isocaloric milk, noncaloric AS, or water (27). The change in 16:0 in the TG fraction was positively correlated with the change in liver fat. Levels of 16:1n7, and to some extent also 18:1n9, were, however, consistently positively correlated with liver fat accumulation in all lipid fractions, most evidently in CE.
It is noteworthy that isocaloric intake of milk did not induce any changes in 16:1n7 or 18:1n9, and only in 16:0 in the PL fraction. Plasma 16:0 PL was also reported to change in studies investigating distinct carbohydrate diets (controlled feeding) that showed a PL-specific change by Volk et al. but not in CE and TG fractions (14), but the change of 16:0 was shown in both PL and TG by Hyde et al. (30), and in both PL and CE by Raatz et al. (31) and King et al. (32), after higher intakes of carbohydrates, respectively. Therefore, the relevance of a PL-specific effect is unclear and not consistent among studies. However, it is possible that the higher relative abundance of 16:0 in the PL fraction, as compared with the other fractions and in CE in particular, might partly explain the current significant increase of 16:0 in PL vs. the other lipid fractions. Although SS has more than twice the carbohydrate content of milk, the total increase in carbohydrates from lactose (47 g, 188 kcal) from 1 L of milk intake per day must be regarded as a significant dietary contribution. Thus, high consumption of milk for 24 weeks does not appear to have any major effects on the fatty acid composition of the proposed lipogenic markers, except in PL 16:0. Whether this lack of effect may reflect, or possibly even explain, the lack of liver fat increase after milk intake is unclear, but an interesting possibility that requires further investigation.
We are not aware of any studies that have investigated the effect of sugar-sweetened cola or other SS on plasma fatty acid composition in different lipid fractions. In this study, we focused on the proposed lipogenic fatty acid markers that are directly (16:0) or indirectly (16:1n7, 18:1n9) involved in the lipogenic pathway. Some studies have investigated the DNL effects of increased intake of carbohydrates, either in place of fat, or as an addition to the habitual diet (17, 19, 23–26), but no studies have examined the effects of SS per se on longitudinal lipogenic fatty acid markers, i.e., in CE and PL. However, Luukkonen et al. recently showed that overfeeding (excess 1,000 kcal/day) for 3 weeks with a high-carbohydrate diet consisting of 100% simple sugars, including ∼4 dl of sugar-sweetened beverage and ∼3 dl daily of orange juice, significantly increased 16:0, 16:1n7 and 14:0 in plasma VLDL-TG, without change in 18:0 and 18:1n9 (23). Thus, although the shorter time period, and pronounced overfeeding conditions, those results are partly in line with the current findings. Of clear interest from the Luukkonen et al.’s study is the finding that isocaloric overfeeding with saturated fat (including 16:0) appeared to cause a higher increase of 16:0 in plasma VLDL-TG and a more robust increase in other SFA, including 14:0 and 18:0, compared to overfeeding with simple sugars, despite hepatic DNL being upregulated during overfeeding with sugars (23). However, this may not be surprising considering that 16:0 levels in the diet were increased, 16:0 appears to be tightly regulated and does not always increase in a pronounced fashion despite marked changes in dietary intake (33). However, other studies reporting effects of sugar-rich diets and/or sugar-sweetened beverages on lipogenic fatty acid markers have done so in VLDL-TG only, which may not be informative for interpreting results from large cohorts typically assessing fatty acids in PL and CE. Our results, reporting effects on PL and CE as well as total plasma TG, may be more informative for interpreting results from observational studies, but caution is advised when comparing with studies reporting only on VLDL-TG.
Our finding that a change in 16:1n7 in all lipid fractions, potentially reflecting increased SCD1 activity, was consistently associated with the change in liver fat content is in line with previous reports from both isocaloric and hyper-caloric trials studies in individuals fed with high amounts of saturated fat (34–36).
The randomized controlled trial design over a relatively long period of time is the main strength of this study. The equal distribution of the metabolic baseline characteristics, utilizing objective measurement to assess the composition of fatty acids, and the regularly checked compliance were other strengths of this study. Furthermore, examining the investigated change in the composition of fatty acid in the lipid fractions with the change in liver fat was an additional strength of this study. One limitation of this study was the relatively small sample size that limited the statistical power of the analyses. Although the study groups were randomized, the unknown variance in physical activity might not have been evenly distributed between the groups due to their small size. Furthermore, it should be considered that conducting multiple statistical tests without correction increases the risks of chance findings. Another possible limitation was measuring SCD1 activity indirectly using fatty acid ratios (16:1n7/16:0) in the lipid fractions. Further, due to the nature of the study, it could not be blinded that might have influenced the subjects into behavior that undermined the effects of the intervention, especially in the energy-containing test beverages.
Conclusion
This 24-week randomized study showed that excessive intake, well above the average intake in this Scandinavian population, from both of the energy-containing beverages (SS and milk), significantly increased 16:0 in plasma PL but not in CE and TG fractions. Notably, 16:1n7 increased with greater magnitude during SS in all lipid fractions and was consistently associated with liver fat accumulation, possibly reflecting upregulated DNL and SCD1 activity in this overweight population. Thus, PL might be the most sensitive lipid fraction to capture changes in 16:0, whereas changes in 16:1n7 in response to high SS were reflected also in other lipid fractions. The isocaloric intake of milk showed a similar effect on 16:0 as SS, although milk did not induce such consistent changes in monounsaturated fatty acids, findings that are in line with the neutral effect of milk on liver fat content (27).
Data availability statement
The original contributions presented in this study are included in the article/Supplementary material, further inquiries can be directed to the corresponding author.
Ethics statement
The studies involving human participants were reviewed and approved by the Ethics Committee of Middle Jutland, Denmark. The patients/participants provided their written informed consent to participate in this study.
Author contributions
UR and BR designed the research. MB, JB, FR, and MM conducted the research. MB and MM analyzed the data. MB wrote the first draft. UR had primary responsibility of the final content and conceived the study concept. All authors reviewed, provided intellectual input, revised, and approved the final manuscript.
Funding
This study was supported by the Danish Council for Strategic Research, Swedish Research Council for Sustainable Development (FORMAS Grant No. 2016-01639), Excellence in Diabetes Research in Sweden (EXODIAB), the Swedish Diabetes Foundation, the Swedish Heart and Lung Foundation, and Erik, Karin och Gösta Selander Foundation.
Acknowledgments
We thank Maria Maersk for excellent work with this study and data collection. Jazan University, Saudi Arabia, for the financial support and the scholarship of MB as a doctoral student.
Conflict of interest
The authors declare that the research was conducted in the absence of any commercial or financial relationships that could be construed as a potential conflict of interest.
Publisher’s note
All claims expressed in this article are solely those of the authors and do not necessarily represent those of their affiliated organizations, or those of the publisher, the editors and the reviewers. Any product that may be evaluated in this article, or claim that may be made by its manufacturer, is not guaranteed or endorsed by the publisher.
Supplementary material
The Supplementary Material for this article can be found online at: https://www.frontiersin.org/articles/10.3389/fnut.2022.936828/full#supplementary-material
Abbreviations
DNL, De novo lipogenesis; NAFLD, non-alcoholic fatty liver disease; SCD1, stearoyl-CoA desaturase; SFA, saturated fatty acids; MUFA, monounsaturated fatty acids; SS, Sugar-sweetened soda; AS, aspartame-sweetened soda; MRI, magnetic resonance imaging; TLC, thin-layer chromatography; CE, cholesteryl esters; PL, phospholipids; TG, triglycerides; 14:0, myristate; 16:0, palmitate; 16:1n7, palmitoleate; 18:0, stearate; 18:1n9, oleate.
References
1. Paglialunga S, Dehn CA. Clinical assessment of hepatic de novo lipogenesis in non-alcoholic fatty liver disease. Lipids Health Dis. (2016) 15:159. doi: 10.1186/s12944-016-0321-5
2. Ameer F, Scandiuzzi L, Hasnain S, Kalbacher H, Zaidi N. De novo lipogenesis in health and disease. Metabolism. (2014) 63:895–902. doi: 10.1016/j.metabol.2014.04.003
3. Parks EJ. Dietary carbohydrate’s effects on lipogenesis and the relationship of lipogenesis to blood insulin and glucose concentrations. Br J Nutr. (2002) 87(Suppl. 2):S247–53. doi: 10.1079/BJN/2002544
4. Samuel VT, Shulman GI. The pathogenesis of insulin resistance: integrating signaling pathways and substrate flux. J Clin Invest. (2016) 126:12–22. doi: 10.1172/JCI77812
5. Collins JM, Neville MJ, Pinnick KE, Hodson L, Ruyter B, van Dijk TH, et al. De novo lipogenesis in the differentiating human adipocyte can provide all fatty acids necessary for maturation. J Lipid Res. (2011) 52:1683–92. doi: 10.1194/jlr.M012195
6. Hodson L, Skeaff CM, Fielding BA. Fatty acid composition of adipose tissue and blood in humans and its use as a biomarker of dietary intake. Prog Lipid Res. (2008) 47:348–80. doi: 10.1016/j.plipres.2008.03.003
7. Hudgins LC, Seidman CE, Diakun J, Hirsch J. Human fatty acid synthesis is reduced after the substitution of dietary starch for sugar. Am J Clin Nutr. (1998) 67:631–9. doi: 10.1093/ajcn/67.4.631
8. Siri-Tarino PW, Chiu S, Bergeron N, Krauss RM. Saturated fats versus polyunsaturated fats versus carbohydrates for cardiovascular disease prevention and treatment. Annu Rev Nutr. (2015) 35:517–43. doi: 10.1146/annurev-nutr-071714-034449
9. Warensjö E, Risérus U, Gustafsson IB, Mohsen R, Cederholm T, Vessby B. Effects of saturated and unsaturated fatty acids on estimated desaturase activities during a controlled dietary intervention. Nutr Metab Cardiovasc Dis. (2008) 18:683–90. doi: 10.1016/j.numecd.2007.11.002
10. Forouhi NG, Koulman A, Sharp SJ, Imamura F, Kröger J, Schulze MB, et al. Differences in the prospective association between individual plasma phospholipid saturated fatty acids and incident type 2 diabetes: the EPIC-interact case-cohort study. Lancet Diabetes Endocrinol. (2014) 2:810–8. doi: 10.1016/S2213-8587(14)70146-9
11. Warensjö E, Sundström J, Vessby B, Cederholm T, Risérus U. Markers of dietary fat quality and fatty acid desaturation as predictors of total and cardiovascular mortality- a population-based prospective study. Am J Clin Nutr. (2008) 88:203–9. doi: 10.1093/ajcn/88.1.203
12. World Health Organization. World Health Statistics 2018: Monitoring Health for the SDGs, Sustainable Development Goals. Geneva:World Health Organization (2018).
13. Alsharari ZD, Leander K, Sjogren P, Carlsson A, Cederholm T, de Faire U, et al. Association between carbohydrate intake and fatty acids in the de novo lipogenic pathway in serum phospholipids and adipose tissue in a population of Swedish men. Eur J Nutr. (2020) 59:2089–97.
14. Volk BM, Kunces LJ, Freidenreich DJ, Kupchak BR, Saenz C, Artistizabal JC, et al. Effects of step-wise increases in dietary carbohydrate on circulating saturated Fatty acids and palmitoleic acid in adults with metabolic syndrome. PLoS One. (2014) 9:e113605. doi: 10.1371/journal.pone.0113605
15. Chong MF, Hodson L, Bickerton AS, Roberts R, Neville M, Karpe F, et al. Parallel activation of de novo lipogenesis and stearoyl-CoA desaturase activity after 3 d of high-carbohydrate feeding. Am J Clin Nutr. (2008) 87:817–23. doi: 10.1093/ajcn/87.4.817
16. Hellerstein MK. De novo lipogenesis in humans: metabolic and regulatory aspects. Eur J Clin Nutr. (1999) 53(Suppl. 1):S53–65. doi: 10.1038/sj.ejcn.1600744
17. Schwarz JM, Noworolski SM, Wen MJ, Dyachenko A, Prior JL, Weinberg ME, et al. Effect of a high-fructose weight-maintaining diet on lipogenesis and liver fat. J Clin Endocrinol Metab. (2015) 100:2434–42. doi: 10.1210/jc.2014-3678
18. Stanhope KL, Bremer AA, Medici V, Nakajima K, Ito Y, Nakano T, et al. Consumption of fructose and high fructose corn syrup increase postprandial triglycerides, LDL-cholesterol, and apolipoprotein-B in young men and women. J Clin Endocrinol Metab. (2011) 96:E1596–605. doi: 10.1210/jc.2011-1251
19. Stanhope KL, Schwarz JM, Keim NL, Griffen SC, Bremer AA, Graham JL, et al. Consuming fructose-sweetened, not glucose-sweetened, beverages increases visceral adiposity and lipids and decreases insulin sensitivity in overweight/obese humans. J Clin Invest. (2009) 119:1322–34. doi: 10.1172/JCI37385
20. Swarbrick MM, Stanhope KL, Elliott SS, Graham JL, Krauss RM, Christiansen MP, et al. Consumption of fructose-sweetened beverages for 10 weeks increases postprandial triacylglycerol and apolipoprotein-B concentrations in overweight and obese women. Br J Nutr. (2008) 100:947–52. doi: 10.1017/S0007114508968252
21. Varsamis P, Larsen RN, Dunstan DW, Jennings GL, Owen N, Kingwell BA. The sugar content of soft drinks in Australia, Europe and the United States. Med J Aust. (2017) 206:454–5. doi: 10.5694/mja16.01316
22. Parry SA, Rosqvist F, Mozes FE, Cornfield T, Hutchinson M, Piche ME, et al. Intrahepatic fat and postprandial glycemia increase after consumption of a diet enriched in saturated fat compared with free sugars. Diabetes Care. (2020) 43:1134–41. doi: 10.2337/dc19-2331
23. Luukkonen PK, Sadevirta S, Zhou Y, Kayser B, Ali A, Ahonen L, et al. Saturated fat is more metabolically harmful for the human liver than unsaturated fat or simple sugars. Diabetes Care. (2018) 41:1732–9. doi: 10.2337/dc18-0071
24. McDevitt RM, Bott SJ, Harding M, Coward WA, Bluck LJ, Prentice AM. De novo lipogenesis during controlled overfeeding with sucrose or glucose in lean and obese women. Am J Clin Nutr. (2001) 74:737–46. doi: 10.1093/ajcn/74.6.737
25. Geidl-Flueck B, Hochuli M, Németh Á, Eberl A, Derron N, Köfeler HC, et al. Fructose- and sucrose- but not glucose-sweetened beverages promote hepatic de novo lipogenesis: a randomized controlled trial. J Hepatol. (2021) 75:46–54. doi: 10.1016/j.jhep.2021.02.027
26. Umpleby AM, Shojaee-Moradie F, Fielding B, Li X, Marino A, Alsini N, et al. Impact of liver fat on the differential partitioning of hepatic triacylglycerol into VLDL subclasses on high and low sugar diets. Clin Sci (Lond). (2017) 131:2561–73. doi: 10.1042/CS20171208
27. Maersk M, Belza A, Stodkilde-Jorgensen H, Ringgaard S, Chabanova E, Thomsen H, et al. Sucrose-sweetened beverages increase fat storage in the liver, muscle, and visceral fat depot: a 6-mo randomized intervention study. Am J Clin Nutr. (2012) 95:283–9. doi: 10.3945/ajcn.111.022533
28. Folch J, Lees M, Sloane Stanley GH. A simple method for the isolation and purification of total lipides from animal tissues. J Biol Chem. (1957) 226:497–509. doi: 10.1016/S0021-9258(18)64849-5
29. Rosqvist F, Bjermo H, Kullberg J, Johansson L, Michaelsson K, Ahlstrom H, et al. Fatty acid composition in serum cholesterol esters and phospholipids is linked to visceral and subcutaneous adipose tissue content in elderly individuals: a cross-sectional study. Lipids Health Dis. (2017) 16:68. doi: 10.1186/s12944-017-0445-2
30. Hyde PN, Sapper TN, Crabtree CD, LaFountain RA, Bowling ML, Buga A, et al. Dietary carbohydrate restriction improves metabolic syndrome independent of weight loss. JCI Insight. (2019) 4:e128308. doi: 10.1172/jci.insight.128308
31. Raatz SK, Bibus D, Thomas W, Kris-Etherton P. Total fat intake modifies plasma fatty acid composition in humans. J Nutr. (2001) 131:231–4. doi: 10.1093/jn/131.2.231
32. King IB, Lemaitre RN, Kestin M. Effect of a low-fat diet on fatty acid composition in red cells, plasma phospholipids, and cholesterol esters: investigation of a biomarker of total fat intake. Am J Clin Nutr. (2006) 83:227–36. doi: 10.1093/ajcn/83.2.227
33. Vessby B, Gustafsson IB, Tengblad S, Boberg M, Andersson A. Desaturation and elongation of fatty acids and insulin action. Ann N Y Acad Sci. (2002) 967:183–95. doi: 10.1111/j.1749-6632.2002.tb04275.x
34. Bjermo H, Iggman D, Kullberg J, Dahlman I, Johansson L, Persson L, et al. Effects of n-6 PUFAs compared with SFAs on liver fat, lipoproteins, and inflammation in abdominal obesity: a randomized controlled trial. Am J Clin Nutr. (2012) 95:1003–12. doi: 10.3945/ajcn.111.030114
35. Rosqvist F, Iggman D, Kullberg J, Cedernaes J, Johansson HE, Larsson A, et al. Overfeeding polyunsaturated and saturated fat causes distinct effects on liver and visceral fat accumulation in humans. Diabetes. (2014) 63:2356–68. doi: 10.2337/db13-1622
Keywords: sugar-sweetened beverages, carbohydrate intake, de novo lipogenesis, plasma fatty acid composition, saturated, monounsaturated, liver fat, biomarkers
Citation: Bajahzer MF, Bruun JM, Rosqvist F, Marklund M, Richelsen B and Risérus U (2022) Effects of sugar-sweetened soda on plasma saturated and monounsaturated fatty acids in individuals with obesity: A randomized study. Front. Nutr. 9:936828. doi: 10.3389/fnut.2022.936828
Received: 05 May 2022; Accepted: 03 August 2022;
Published: 31 August 2022.
Edited by:
Anne Marie Minihane, University of East Anglia, United KingdomReviewed by:
Rosalind Fallaize, University of Hertfordshire, United KingdomChristopher Peter Corpe, King’s College London, United Kingdom
Copyright © 2022 Bajahzer, Bruun, Rosqvist, Marklund, Richelsen and Risérus. This is an open-access article distributed under the terms of the Creative Commons Attribution License (CC BY). The use, distribution or reproduction in other forums is permitted, provided the original author(s) and the copyright owner(s) are credited and that the original publication in this journal is cited, in accordance with accepted academic practice. No use, distribution or reproduction is permitted which does not comply with these terms.
*Correspondence: Ulf Risérus, dWxmLnJpc2VydXNAcHViY2FyZS51dS5zZQ==
†These authors have contributed equally to this work