- 1School of Agriculture and Biology, Shanghai Jiao Tong University, Shanghai, China
- 2Shanghai Key Laboratory for Veterinary and Biotechnology, Shanghai, China
- 3Shanghai Center of Agri-Products Quality and Safety, Shanghai, China
To investigate the effect of Flammulina velutipes polysaccharides (FVPs) on mice intestinal inflammation, FVPs were extracted from Flammulina velutipes (FV) using a solid anaerobic fermentation technique. The antioxidant and anti-inflammatory capacities of FVP and fermented FVP (FFVP) induced by lipopolysaccharide (LPS) were investigated in vitro and in vivo. The results showed that the yield of FFVP (9.44%) was higher than that of FVP (8.65%), but the molecular weight (MW) of FFVP (15,702 Da) was lower than that of FVP (15,961 Da). The antioxidant and anti-inflammatory capacities of FFVP were higher than that of FVP in preventing mice diarrhea, enhancing antioxidant capacities, and reducing the secretion and mRNA expression of interleukin-1β (IL-1β), IL-6, IL-18, and tumor necrosis factor-α (TNF-α). The anti-inflammatory mechanisms of FVP and FFVP were analyzed by inhibiting the activation of the NLRP3 signaling pathway using an LPS-induced mice model. This study indicated that FFVP could be used as a functional antioxidant, indicating a potential application in functional food and health products.
Highlights
- First report the extraction of fermented Flammulina velutipes polysaccharides (FFVP).
- FFVP with low molecular weight has higher anti-inflammatory capacities than FVP.
- FFVP regulates inflammatory response by inhibiting NLRP3 signal pathway activation.
Introduction
Flammulina velutipes (FV), as one of the four major cultivated edible fungi, is widely artificially cultivated in the world (1). It is well-known that FV mainly contains abundant polysaccharides and proteins, and among them, FV polysaccharides (FVP) have shown many excellent biological activities, including antioxidant, antimicrobial, anti-aging, anti-tumor, and immunomodulatory properties (2, 3). For instance, previous studies have reported that FVP could improve antioxidant capacities and anti-inflammatory properties by increasing the immunoglobulin and immune factors in mice serum (4, 5), and play an immunomodulatory role in regulating the intestinal environment and improving the balance of flora (6).
It is well-known that the preparation and purification of polysaccharides are critical to investigating their functional capacities. The traditional extraction methods of polysaccharides include hot water extraction (HWE), enzyme extraction (EE), chemistry assisted extraction (CAE), and pressurized liquid extraction (PLE) (7). However, the low extraction efficacy, high cost, and environmental pollution are the pitfalls of these methods. Recently, microbial fermentation has been widely employed to extract bioactive compounds and is applied in food, agriculture, environment, and medicine fields (8). Moreover, the solid-state fermentation, one of the most common forms of microbial fermentation, is an alternative method to produce large amounts of fungal polysaccharides, which has the advantages of a shorter production cycle, less environmental pollution, higher yield, and better product quality (9, 10). For instance, polysaccharides were extracted from Monascus purpureus using microbial fermentation, which enhanced immunomodulatory capacities by significantly improving pinocytic and phagocytic capacities (11). Moreover, polysaccharides were extracted from Inonotus hispidus using solid-state fermentation, showing that they had high yields and antioxidant capacities and could reduce H2O2-induced oxidative damage to cells in vitro (12).
NLRP3 (Nucleotide- binding oligomerization domain, leucine- rich repeat and pyrin domain- containing 3) inflammasome is a multiprotein complex located in the cytoplasm and has been proven to be closely related to the pathological process of intestinal inflammation (13). Cui et al. (14) reported that colitis could be ameliorated by polysaccharides from Scutellaria baicalensis Georgi via suppressing NLRP3 inflammasome activation. Thus, FVP has excellent antioxidant and anti-inflammatory capacities. There has few research on the extraction of fermented FVPs and their antioxidant and anti-inflammatory capacities. Therefore, we hypothesized that FVP extracted using microbial fermentation has higher extraction efficacy and biological functions than unfermented ones in vitro and in vivo. In this work, the yields and molecular weight (MW) of FFVP and unfermented FVP were compared, and anti-inflammatory capacities were studied using lipopolysaccharide (LPS)-induced mice model. This study could provide a new idea for developing a functional additive to alleviate intestinal inflammation.
Materials and Methods
Materials and Reagents
Flammulina velutipes was obtained by Shanghai Guangming Senyuan Biotechnology Co., Ltd., China. The microbial culture starter, including Bacillus subtilis CMCCB 63501, Bifidobacterium longum ATCC 15707, and Saccharomyces cerevisiae ATCC 9763, was a kind gift from Shanghai Chuangbo Ecological Engineering Co., Ltd., China (production batch No. CB08190529). LPS was obtained from Sigma–Aldrich (St. Louis, MO, United States). Other chemicals and reagents were of analytical grade and were purchased from Sigma–Aldrich (St. Louis, MO, United States).
Extraction of FFVP
Flammulina velutipes was pulverized using Waring blender (Shanghai Shibang Machinery Co., Ltd., China) and passed through a 0.75 mm sieve. Microbial culture starter (0.10%, v/w) was then added to the smashed FV and stirred (200 rpm) in a fermentation tank (Shanghai xiaohan industrial development co., Ltd., Shanghai, China) at the ambient room temperature. The optimal fermentation conditions, including the amounts of molasses contents (3%), temperature (28°C), moisture contents (40%), and culture time (10 days), were previously reported for the production of FFVP (15).
Ultrahigh pressure (HPP600/5L, Suzhou, China)–ultrasonic extraction method (HA131–50–01, Nantong supercritical extraction Co., Jiangsu, China) was used to extract the FVP and FFVP from FV and FFV, respectively. The optimized extraction conditions, including pH = 5, ultrahigh pressure of 360 MPa, ultrahigh pressure time of 5 min, ultrasonic power of 180 W, extraction temperature of 80°C, and extraction time of 40 min, have been reported based on a previous study (15). During the FVP extraction, lipids, pigments, and small molecular compounds were all washed out from FV first. The residues were then extracted with distilled water, and the supernatant was filtered, combined, and concentrated. The crude polysaccharides were then precipitated by adding ethanol and washed successively with anhydrous ethanol, acetone, and petroleum ether (16). The extracted crude FVP was then harvested after deproteinization with Sevag solution (chloroform: butylalcohol = 5:1) and fractionated through diethylaminoethyl (DEAE)–Sepharose fast flow column (elution conditions: distilled water and 0.05, 0.1, 0.2, 0.3, and 0.5 mol/L NaCl gradient solutions) (1).
Molecular Weight Determination
The MW of FVP and FFVP was determined using HPLC (Waters Arc HPLC, Shanghai, China) equipped with a diode array and differential detector. Analyses were performed on a charged surface hybrid (CSH) C18 column (5 μm, 4.6 × 150 mm). The treatment conditions consisted of a mobile phase: 0.1% formic acid aqueous solution (A) and methanol (B), a flow rate of 0.6 ml/min, and an injection volume of 20 μl, respectively. A standard curve with the elution volume plotted against the logarithm of MW was constructed using the Dextran T standards and glucose according to Tang's method (17), and the MW of FVP/FFVP was calculated by the calibration curve equation based on their elution volume (18).
Animals and Experimental Design
Four-week-old male BALB/c mice obtained from Shanghai JieSiJie Laboratory Animals Co., Ltd. (Shanghai, China) were put in polypropylene cages. All mice were raised under standard conditions of 12 h light/dark cycle at 25 ± 2°C, with a relative humidity of 55 ± 5%. The mice had free access to a basal diet (Jiangsu Xietong Inc., Nanjing, China) and water. After one week adaption, 60 mice were randomly divided into 6 groups with 10 mice in each group and filled the stomachs with 2 mL sample everyday as follows: CON group (normal saline), LPS group (normal saline), LFVP group (50 mg/kg FVP), HFVP group (100 mg/kg FVP), LFFVP group (50 mg/kg FFVP) and HFFVP group (100 mg/kg FFVP). The experiment lasted for 60 days. On the last day, the CON group was injected intraperitoneally with normal saline (2 ml), while the LPS group, LFVP group, HFVP group, LFFVP group, and HFFVP group were injected intraperitoneally with LPS (2 ml, 3 mg/kg) to induce intestinal inflammation in mice (19). All experimental procedures were approved by the Institutional Animal Ethics Committee of Shanghai Jiao Tong University under animal protocol number 202101207.
Behavior and Appearance Observation of Mice
A total of three mice were randomly selected from each group and placed in a box after intraperitoneal injection. The behavior and appearance, including diarrhea occurrence and mice hair, were observed after 4 h (20).
Serum Oxidative Stress and Inflammatory Factor Analysis
Blood was collected from the eyeball of mice under anesthesia, and then the serum was obtained after centrifugation (5420, Eppendorf, Hamburg, Germany; 4,000 rpm, 10 min). The H2O2 and malondialdehyde (MDA) levels, catalase (CAT), glutathione peroxidase (GSH-Px), superoxide dismutase (SOD) capacities, and total antioxidant capacities (T-AOC) in serum were detected using an assay kit (Beyotime Biotechnology, Shanghai, China). Besides, the levels of interleukin-1β (IL-1β), IL-6, IL-18, and tumor necrosis factor-α (TNF-α) in serum were detected by enzyme linked immunosorbent assay method (ELISA) using ELISA Kit (Nanjing Jiancheng Bioengineering Institute, Nanjing, China).
Histological Analysis of Mice Intestine
The jejunum, ileum, and colon tissue sections were fixed in 10% formalin and embedded in paraffin to analyze the pathological changes. Sections (5 μm thick) were stained with a hematoxylin and eosin (H&E) stain kit (D006-1-1, Nanjing Jiancheng Bioengineering Institute, Nanjing, China) and observed under a light microscope (Eclipse Ci-L, Nikon, Tokyo, Japan). Image-Pro Plus 6.0 (Media Cybernetics, Rockville, MD, United States) was used for analysis. The height of intact villi and the depth of crypts in each section were measured, respectively, and the ratio of villi height to crypt depth (V/C value) was calculated (n = 5).
Quantitative Real-Time Polymerase Chain Reaction Analysis
Total RNA of intestinal samples was isolated with Trizol Reagent (Takara, Kyoto, Japan) after incubation for 24 h (21). RNA amount and quality were determined using the spectrophotometer (GeneQuant 1300 GE, Austin, TX, United States). The absorbance of samples between 1.8 and 2.0 at 260-280 nm was subjected to acceptable quality and integrity. The cDNA was synthesized with TransScript First-Strand complementary DNA synthesis SuperMix (AT341-01, Roche, Basel, Switzerland). The primer sequences are shown in Supplementary Table 1. Polymerase chain reaction (PCR) was performed in StepOnePlus Real-time PCR system (ABI, Carlsbad, CA, United States) using SYBR Green PCR Core Reagents (Roche, Basel, Switzerland). The housekeeping gene, β-actin, was used as a reference gene for normalization. Data were analyzed according to the 2−ΔΔCt method. Results were expressed as relative mRNA levels.
Western Blot Analysis
The intestinal samples were lysed in radio immunoprecipitation assay (RIPA) buffer (Beyotime, Shanghai, China) with protease and phosphatase inhibitor cocktails (Sigma Co., St. Louis, MO, United States) for 10 min on a rocker at 4°C. Proteins of samples were analyzed using sodium dodecyl sulfate polyacrylamide gel electrophoresis (SDS-PAGE) and then transferred onto polyvinylidene fluoride (PVDF) membranes (0.45 μm, Millipore, Billerica, MA, United States). Each PVDF membrane was blocked with tris buffered saline tween (TBST; 100 mM Tris-HCl,150 mM NaCl, 0.05% Tween 20, pH 7.5) with 5% non-fat dried milk for 2 h, and then incubated with the following primary antibodies: NLRP3 (1:1,500), ASC (1:100), Caspase-1 (1:800), IL-1β (1:400), and β-actin (1: 5,000, Sungene biotech, China) overnight at 4°C on a shaker (SC390C, Shanghai Brave Construction Development co., Ltd., Shanghai, China). The goat anti-rabbit of 1:3000 (ZSGE-BIO, China) horse radish peroxidase (HRP) conjugate secondary antibody was then added and the WB results was observed through electrochemical luminescence (ECL) imaging system after adding the enhanced ECL reagent (Beyotime, Shanghai, China). The β-actin was used as a protein loading control. Quantitative analysis was carried out using Amersham Imager 600 (Cytiva, Montana, United States).
Statistical Analysis
All analysis was performed with the SPSS 22.0 software (SPSS Incorporated, Armonk, NY, United States), and statistical significance was analyzed using one-way ANOVA followed by an LSD test as a post-test. Data were expressed as mean ± standard error, and all measurements were conducted in triplicate. The values of P < 0.05 and P < 0.01 were considered significant and markedly significant, respectively, in different treatment groups.
Results and Discussions
Physicochemical Analysis of FVP and FFVP
The yields of FVP and FFVP were 8.65 and 9.44%, respectively, and MW of 15,961 and 15,702 Da (Figure 1), respectively. Besides, the MW/MN (Weight-average Molecular Weight/Number-average Molecular Weight) of FVP and FFVP were 1.20 and 1.09 (Supplementary Figure 2), respectively, which was similar to a previous report (1.042) (16). A previous study has also reported that the contents and MW of tea polysaccharides, compared with the unfermented group, were, respectively, increased and decreased after fermentation treatment, and the biological capacities of fermented fractions were also better than unfermented ones (22). For instance, the MW of Nostoc commune Vauch polysaccharides (NCVP) was higher than that of fermented ones, while the antioxidant capacities were significantly improved during in vitro digestion (23). Thus, microbial fermentation treatment could increase extraction yield and decrease MW of FVP, and the reason could be that one or more glycosidic bonds of FVP may be broken during the fermentation (24, 25).
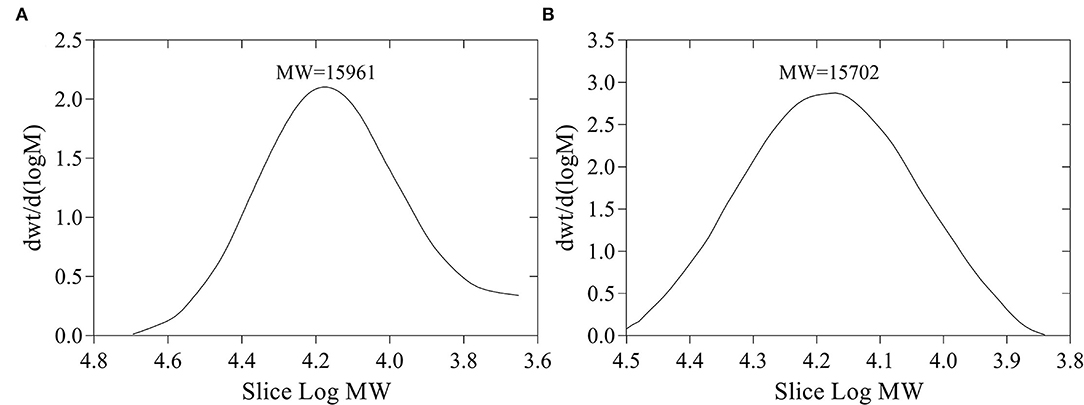
Figure 1. Molecular weight (MW) of FVP (A) and FFVP (B). FVP and FFVP are Flammulina velutipes polysaccharides and fermented Flammulina velutipes polysaccharides.
Behavior and Appearance Observation of Mice
Diarrhea is a life-threatening condition and could cause extreme loss of fluid and salts from the animal body (26). Compared with the CON group, the mice of the LPS group had diarrhea with shaggy hair (Supplementary Figure 1). All of the LFVP group, HFVP group, LFFVP group, and HFFVP group did not have diarrhea, and only the LFVP group was shaggy. Besides, the mice of the CON group and HFFVP group were vigorous with shiny hair, while others gathered in the corner. A previous study has also reported that diarrhea could be prevented using plant polysaccharides including banana, guar, and soya polysaccharides (27). For instance, polysaccharides from S. chinensis could prevent diarrhea in rats by improving the ultrastructure of the gut (28). Besides, the polysaccharides from Alpiniae oxyphyllae and Pogostemon cablin also decreased diarrhea by inhibiting porcine epidemic diarrhea virus (PEDV) reproduction (29, 30).
Serum Antioxidant Capacities and Inflammatory Factor Contents of FVP and FFVP
Serum antioxidant capacities of FVP and FFVP are shown in Supplementary Table 2. Compared with the CON group, H2O2 (3.66 nmol/ml) and MDA (7.64 nmol/ml) contents of LPS group's serum was increased significantly (P < 0.05), while the CAT (0.49 U/ml), GSH-Px (1,319.61 U/ml), SOD (226.87 U/ml), and T-AOC (1.23 U/ml) were decreased significantly (P < 0.05). The antioxidant capacities gradually increased after FVP and FFVP gavage. Serum inflammatory factor contents of FVP and FFVP are shown in Figure 2. Compared with the CON group, the contents of IL-1β, IL-6, IL-18, and TNF-α in the LPS group's serum were increased significantly (P < 0.05). For instance, the IL-1β contents of the LPS group (38.17 pg/ml) were 1.45 times more than the CON group (26.34 pg/ml). Serum inflammatory factor content of the LFVP group, HFVP group, LFFVP group, and HFFVP group decreased significantly (P < 0.05) compared with the LPS group, whose IL-1β contents were 33.05, 31.97, 31.83, and 27.65 pg/ml, respectively. Besides, IL-1β, IL-6, and IL-18 contents of the HFFVP group were lower than that of the HFVP group.
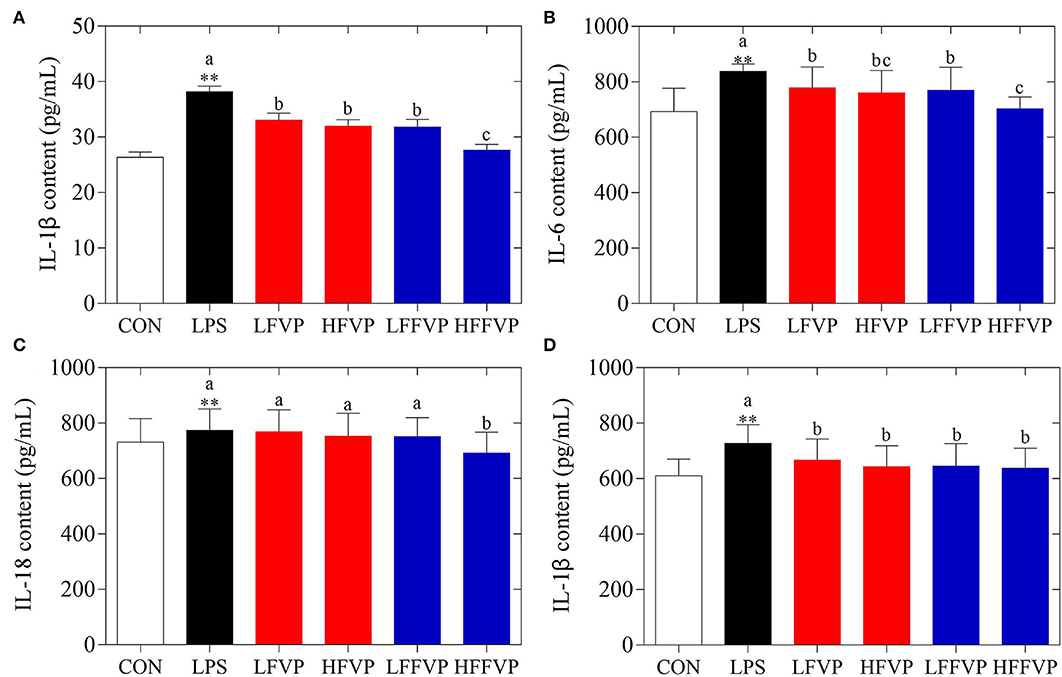
Figure 2. Serum inflammatory factor contents, including IL-1β (A), IL-6 (B), IL-18 (C), and TNF-α (D). Mice were stimulated with 3 mg/kg LPS and/or 50 and 100 mg/kg FVP and FFVP. CON group, LPS group (3 mg/kg LPS), FVP and FFVP groups (50/100 mg/kg FVP and FFVP plus 3 mg/kg LPS). FV, Flammulina velutipes; FVP, Flammulina velutipes polysaccharides; CON, control group; LPS, lipopolysaccharide; LFVP, low dose FVP group; HFVP, high dose FVP group; LFFVP, low dose FFVP group; HFFVP, high dose FFVP group. **P < 0.01 represents LPS vs. CON group; a, b and c (P < 0.05) represent FVP and FFVP groups vs. LPS treated mice.
Wang et al. (31) reported the antioxidant and anti-inflammatory effects of Gynostemma pentaphyllum herb polysaccharides (GPP) in diabetic mice, and the results indicated that GPP could enhance SOD, CAT and GSH-Px capacities, as well as decreasing MDA and IL-6 contents, which was consistent to the present study. Moreover, the antioxidant and anti-inflammatory activities of FFVP were also better than that of FVP. Jiang et al. (32) also reported that low MW Enteromorpha prolifera polysaccharides could enhance anti-inflammatory capacities and decrease inflammatory response through multiple signaling pathways, including TLR2/NF-κB, PKC/ERK/MAPK, and PI3K/Akt pathways (33, 34). Besides, low MW polysaccharides had higher antioxidant capacities than high MW ones (35).
Histological Analysis of Mice Intestine
The jejunum, ileum, and colon intestinal morphology are shown in Figure 3 and the ratio of villus height to crypt depth (V/C value) is show in Table 1. For the LPS group, intestinal epithelial tissue structure was dissolved, most of the inflammatory cells were infiltrated, and mucosa was severely damaged with a large range of ulcers. Compared with the LPS group, intestinal epithelial tissue and intestinal villi were improved in the LFVP group, HFVP group, LFFVP group, and HFFVP group to varying degrees. Meanwhile, intestinal inflammatory damage in the LFVP and HFVP groups were more serious than that in the LFFVP and HFFVP groups, respectively. A previous study showed that FVP could ameliorate bowel inflammation and modulate the gut microbiota on dextran sulfate sodium (DSS)-induced inflammatory bowel disease (36). Besides, FVP could also improve the clinical symptoms and attenuate the mRNA and protein expressions of inflammatory cytokines and oxidative markers in colon tissue.
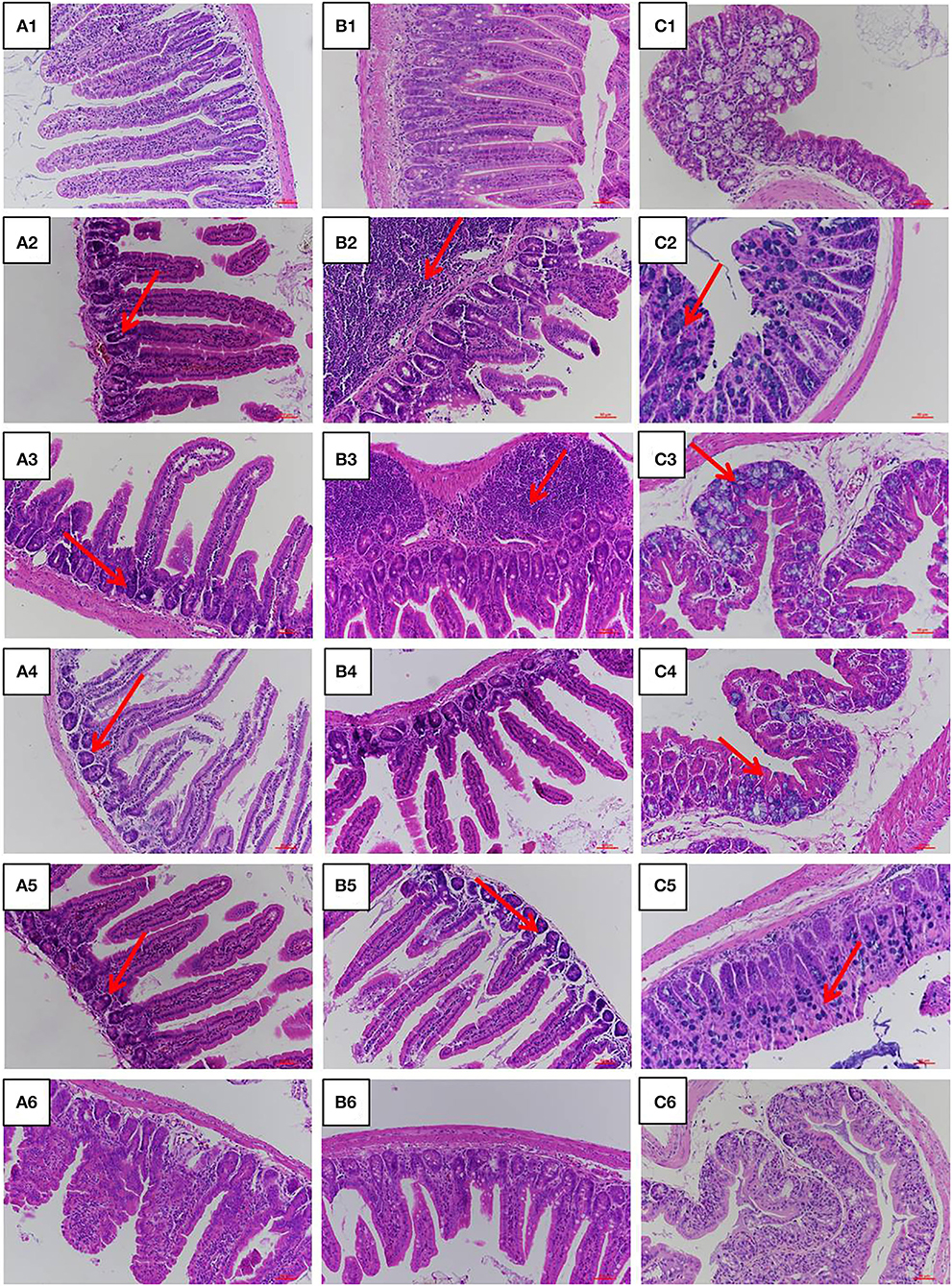
Figure 3. Jejunum (A), ileum (B), and colon (C) intestinal morphology of CON group (1), LPS group (2), LFVP group (3), HFVP group (4), LFFVP group (5), HFFVP group (6). The magnification is 20 ×. FV, Flammulina velutipes; FVP, Flammulina velutipes polysaccharides; CON, control group; LPS, lipopolysaccharide; LFVP, low dose FVP group; HFVP, high dose FVP group; LFFVP, low dose FFVP group; HFFVP, high dose FFVP group.
mRNA Expression of Inflammatory Factors
Lipopolysaccharide-induced IL-1β, IL-6, IL-18, and TNF-α mRNA expression treated by FVP and FFVP in jejunum, ileum, and colon are shown in Figure 4. The mRNA expression of inflammatory factors in the LPS group was significantly increased compared with the CON group (P < 0.05). The LFVP group, HFVP group, LFFVP group, and HFFVP group's mRNA expression of inflammatory factors were lower than that of the LPS group. For instance, IL-1β mRNA expression of the LPS group in the colon was 1.94, while in the LFVP group, HFVP group, LFFVP group, and HFFVP group were 1.87, 1.69, 1.67, and 1.49, respectively. Besides, HFVP's mRNA expressions of all four inflammatory factors were significantly higher than that of HFFVP's (P < 0.05). Han et al. (37) also reported that Gracilaria Lemaneiformis polysaccharides could prevent colitis in Balb/c mice and inhibited pro-inflammatory cytokines expression to attenuate acute inflammation, which was consistent with this study. Therefore, FVP and FFVP play an anti-inflammatory effect by inhibiting pro-inflammatory cytokines mRNA expression.
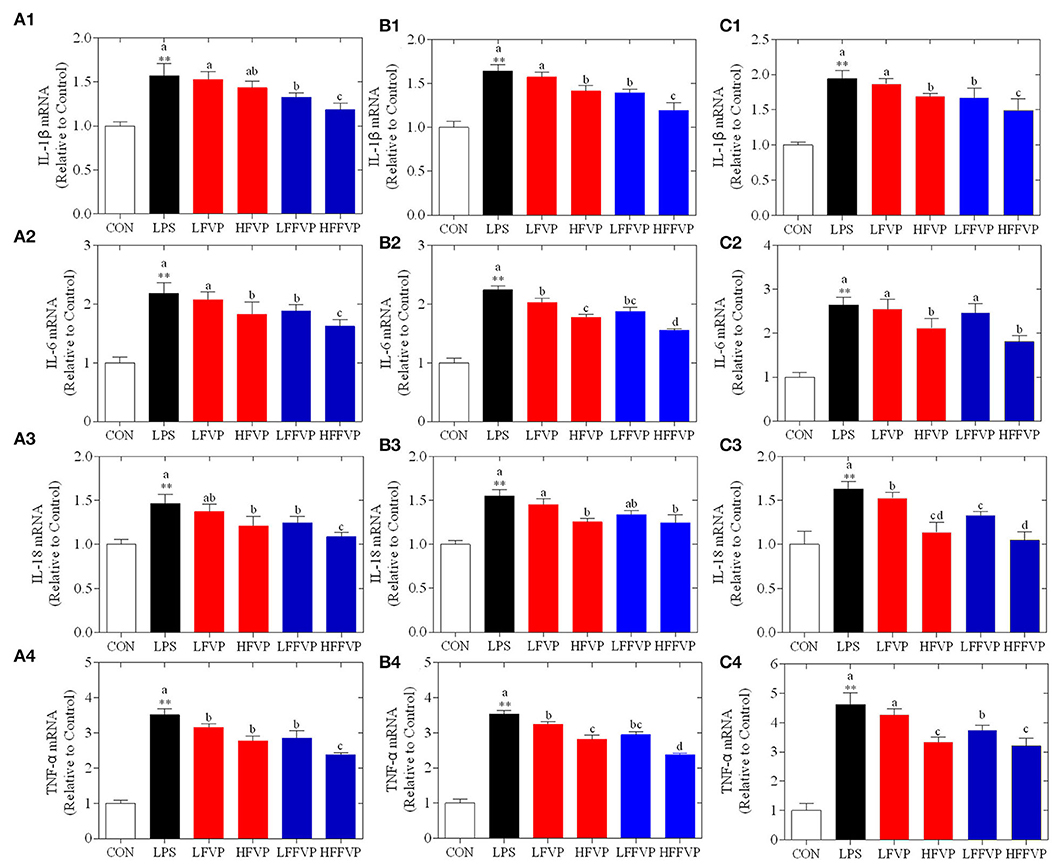
Figure 4. LPS-induced IL-1β (1), IL-6 (2), IL-18 (3) and TNF-α (4) mRNA expression treated by FVP and FFVP in jejunum (A), ileum (B), and colon (C). Mice were stimulated with 3 mg/kg LPS and/or 50 and 100 mg/kg FVP and FFVP. CON group, LPS group (3 mg/kg LPS), FVP and FFVP groups (50/100 mg/kg FVP and FFVP plus 3 mg/kg LPS). FV, Flammulina velutipes; FVP, Flammulina velutipes polysaccharides; CON, control group; LPS, lipopolysaccharide; LFVP, low dose FVP group; HFVP, high dose FVP group; LFFVP, low dose FFVP group; HFFVP, high dose FFVP group. **P < 0.01 represents LPS vs. CON group; a, b and c (P < 0.05) represent FVP and FFVP groups vs. LPS treated mice.
Protein Expression of NLRP3 Signaling Pathway
The NLRP3 signaling pathway was one of the most important anti-inflammatory signaling pathways (38). It could be activated by LPS and mediated by immune response of host to microbial infection and cell injury via agglomeration of NLRP3 inflammasome, resulting in producing activated Caspase-1 and mature IL-1β and IL-18 (39). As the colon was sensitive to LPS-induced inflammatory response, the colon was selected for the subsequent Western Blot test. Compared with the CON group, protein expression of NLRP3, ASC, Caspase-1, and IL-1β were significantly increased in the LPS group. Protein expressions of LFVP, HFVP, LFFVP, and HFFVP groups were lower than that of the LPS group. At the same dose, protein expression of FFVP group was significantly lower than that of FVP group (Figure 5).
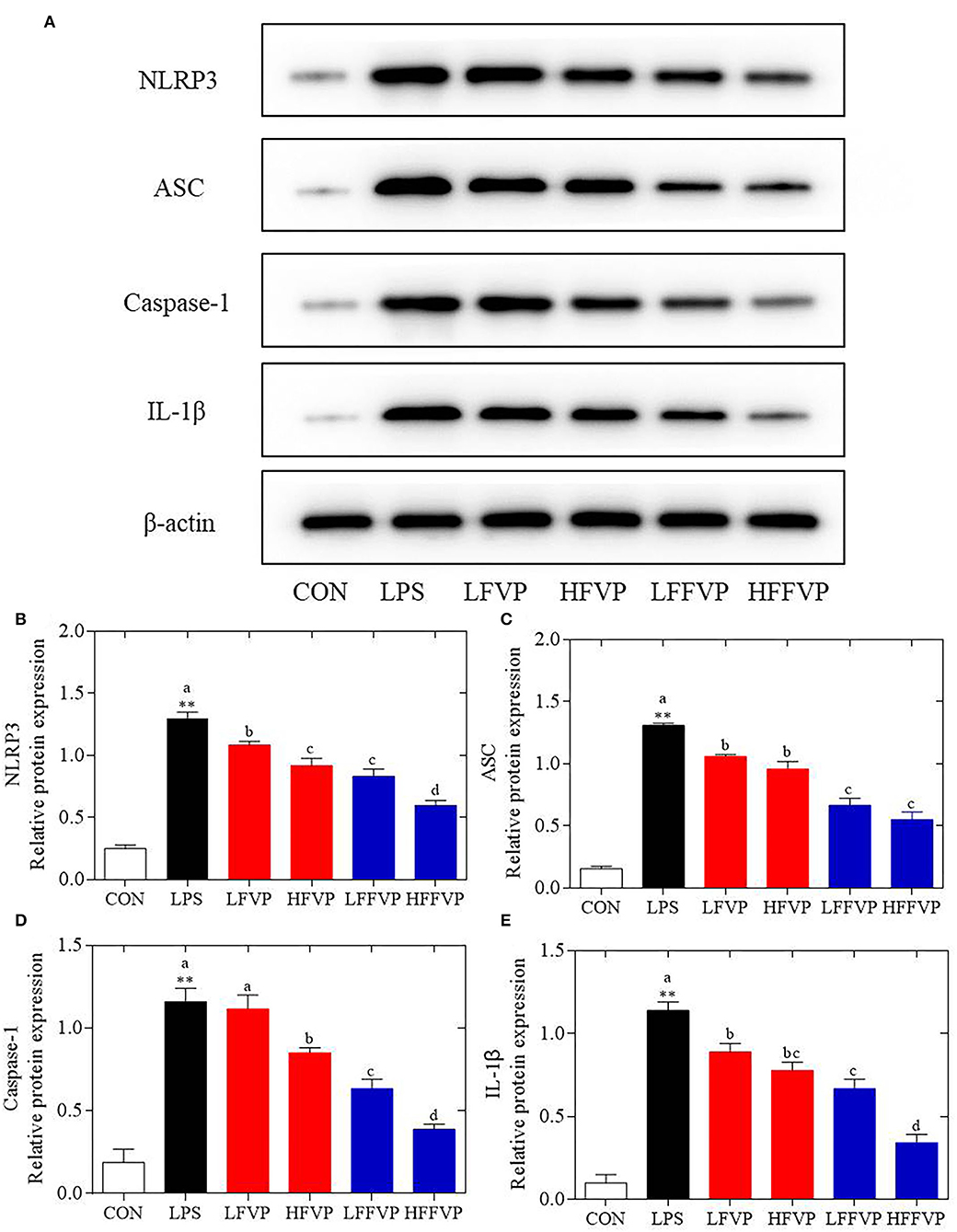
Figure 5. LPS-induced NLRP3/β-actin (A,B), ASC/β-actin (A,C), Caspase-1/β-actin (A,D) and IL-1β/β-actin (A,E) protein expression treated by FVP and FFVP. FV, Flammulina velutipes; FVP, Flammulina velutipes polysaccharides; CON, control group; LPS, lipopolysaccharide; LFVP, low dose FVP group; HFVP, high dose FVP group; LFFVP, low dose FFVP group; HFFVP, high dose FFVP group. **P < 0.01 represents LPS vs. CON group; a, b and c (P < 0.05) represent FVP and FFVP groups vs. LPS treated mice.
Pan et al. (40) reported that polysaccharides from Smilax china L. could ameliorate ulcerative colitis by inhibiting the galectin-3/NLRP3 inflammasome pathway. Besides, Chayote polysaccharides could also reduce active Caspase-1 protein levels and downregulate NLRP3 and IL-1β gene expression (41), suggesting that the NLRP3 inflammasome activation was dependent on the processing of inactive pro-Caspase-1 to active Caspase-1, which enhances IL-1β release, reactive oxygen species (ROS) production, and pyroptosis, an inflammatory form of programmed cell death (42). In this work, protein expression of NLRP3 ASC, Caspase-1 and IL-1β were significantly increased in the LPS group compared with CON group. Besides, these protein expression decreased after treated by FVP/FFVP compared with LPS group. Thus, the anti-inflammatory capacities of FVP and FFVP were also inhibited via activation of an NLRP3 signaling pathway.
Conclusion
The FFVP has higher antioxidant and anti-inflammatory capacities than FVP in preventing diarrhea, enhancing antioxidant capacities, and reducing the secretion and mRNA expression of IL-1β, IL-6, IL-18, and TNF-α. Besides, the anti-inflammatory capacities of FVP and FFVP are to inhibit the activation of an NLRP3 signaling pathway. This study indicated that FFVP could be used as functional additives and confered to its beneficial functions such as antioxidant and anti- inflammation.
Data Availability Statement
The original contributions presented in the study are included in the article/Supplementary Material, further inquiries can be directed to the corresponding author/s.
Ethics Statement
The animal study was reviewed and approved by Institutional Animal Ethics Committee of Shanghai Jiao Tong University.
Author Contributions
SM, WX, JZ, WZ, and JX: design and conceptualization. SM, HZ, and JX: manuscript writing and proofreading. SM, TL, WX, JZ, HZ, WZ, and JX: experimental work, data analysis, and interpretation. JX: funding acquisition. All authors have read and agreed to the published version of the manuscript.
Funding
This research was funded by Shanghai Agriculture Applied Technology Development Program, China (Grant No. X2019-02-08-00-08-F01155) and National Natural Science Foundation of China (Grant No. 31872367).
Conflict of Interest
The authors declare that the research was conducted in the absence of any commercial or financial relationships that could be construed as a potential conflict of interest.
Publisher's Note
All claims expressed in this article are solely those of the authors and do not necessarily represent those of their affiliated organizations, or those of the publisher, the editors and the reviewers. Any product that may be evaluated in this article, or claim that may be made by its manufacturer, is not guaranteed or endorsed by the publisher.
Supplementary Material
The Supplementary Material for this article can be found online at: https://www.frontiersin.org/articles/10.3389/fnut.2022.934073/full#supplementary-material
Abbreviations
FV, Flammulina velutipes; FVP, Flammulina velutipes polysaccharides; FFVP, fermented Flammulina velutipes polysaccharides; LFVP, low dose FVP group; HFVP, high dose FVP group; LFFVP, low dose FFVP group; HFFVP, high dose FFVP group; LPS, lipopolysaccharide; SDS-PAGE, sodium dodecyl sulfate polyacrylamide gel electrophoresis; PVDF, polyvinylidene fluoride; TBST, Tris buffered saline tween; RIPA, radio immunoprecipitation assay; ECL, electrochemical luminescence; HWE, hot water extraction; EE, enzyme extraction; CAE, chemistry assisted extraction; PLE, pressurized liquid extraction; MW, molecular weight; MDA, Malondialdehyde; CAT, catalase; GSH-Px, Glutathioneperoxidase; SOD, Super oxide dismutase; T-AOC, total antioxidant capacity; H&E, hematoxylin and eosin; V/C, villi height to crypt depth; HRP, horse radish peroxidase; NCVP, Nostoc commune Vauch polysaccharides; PEDV, porcine epidemic diarrhea virus; GPP, Gynostemma pentaphyllum herb polysaccharides.
References
1. Hao Y, Liao X, Wang X, Lao S, Liao W. The biological regulatory activities of Flammulina velutipes polysaccharide in mice intestinal microbiota, immune repertoire and heart transcriptome. Int J Biol Macromol. (2021) 185:582–91. doi: 10.1016/j.ijbiomac.2021.06.175
2. Chen F, Zhang Q, Wu P, Zhao Y, Chen Y. Green fabrication of seedbed-like Flammulina velutipes polysaccharides–derived scaffolds accelerating full-thickness skin wound healing accompanied by hair follicle regeneration. Int J Biol Macromol. (2021) 167:117–29. doi: 10.1016/j.ijbiomac.2020.11.154
3. Zhang T, Ye J, Xue C. Structural characteristics and bioactive properties of a novel polysaccharide from Flammulina velutipes. Carbohydr Polym. (2018) 197:147–56. doi: 10.1016/j.carbpol.2018.05.069
4. Wu M, Luo X, Xu XY, Wei W, Yu MY, Jiang N, et al. Antioxidant and immunomodulatory activities of a polysaccharide from Flammulina velutipes. J Tradit Chin Med. (2014) 34:733–40. doi: 10.1016/S0254-6272(15)30089-3
5. Zhao RQ, Hu QH, Ma GX, Su AX, Xie MH, Li XF, et al. Effects of Flammulina velutipes polysaccharide on immune response and intestinal microbiota in mice. J Funct Foods. (2019) 56:255–64. doi: 10.1016/j.jff.2019.03.031
6. Liang QX, Zhao QC, Hao XT, Wang JM, Ma CY, Xi XF, et al. The effect of Flammulina velutipes polysaccharide on immunization analyzed by intestinal flora and proteomics. Front Nutr. (2022) 9:1–17. doi: 10.3389/fnut.2022.841230
7. Hao W, Wang SF, Zhao J, Li SP. Effects of extraction methods on immunology activity and chemical profiles of Lycium barbarum polysaccharides. J Pharmaceut Biomed. (2020) 185:113219. doi: 10.1016/j.jpba.2020.113219
8. Jiang Z, Mao J, Huang J, Wu Y, Cai C, Fan Y. Changes in antioxidant activity of blueberry-ferment during natural fermentation process, Sci Technol Food Ind. (2013) 2:194–201. doi: 10.13386/j.issn1002-0306.2013.02.059.
9. Meshram V, Saxena S, Paul K, Gupta M, Kapoor N. Production, purification and characterization of a potential fibrinolytic protease from endophytic Xylaria curta by solid substrate fermentation. Appl Biochem Biotechnol. (2016) 181:1–17. doi: 10.1007/s12010-016-2298-y
10. Singhania RR, Patel AK, Soccol CR, Pandey A. Recent advances in solid-state fermentation. Biochem Eng J. (2009) 44:13–8. doi: 10.1016/j.bej.2008.10.019
11. Xie LM, Huang ZB, Meng H, Shi XY, Xie JH. Immunomodulation effect of polysaccharides from liquid fermentation of Monascus purpureus 40269 via membrane TLR-4 to activate the MAPK and NF-κB signaling pathways. Int J Biol Macromol. (2022) 201:480–91. doi: 10.1016/j.ijbiomac.2022.01.045
12. Liu X, Hou RL, Xu KQ, Chen L, Wu XP, Lin WX, et al. Extraction, characterization and antioxidant activity analysis of the polysaccharide from the solid-state fermentation substrate of Inonotus hispidus. Int J Biol Macromol. (2019) 123:468–76. doi: 10.1016/j.ijbiomac.2018.11.069
13. Bauer C, Duewell P, Mayer C, Lehr HA, Fitzgerald KA, Dauer M, et al. Colitis induced in mice with dextran sulfate sodium (DSS) is mediated by the NLRP3 inflammasome. Gut. (2010) 59:1192–9. doi: 10.1136/gut.2009.197822
14. Cui L, Wang W, Luo Y, Ning Q, Xia Z, Chen J, et al. Polysaccharide from Scutellaria baicalensis Georgi ameliorates colitis via suppressing NF-κB signaling and NLRP3 inflammasome activation. Int J Biol Macromol. (2019) 132:393–405. doi: 10.1016/j.ijbiomac.2019.03.230
15. Ma S, Shen C, Xu JX. Extraction, structure and antioxidant activity of polysaccharide from fermented Flammulina velutipes root. Food Sci Technol. (2021) 46:147–54. doi: 10.13684/j.cnki.spkj.2021.03.026
16. Liang ZH, Zheng KW, Zhao QC, Shao WJ, Li CQ, Wang JM, et al. Structural identification and coagulation effect of Flammulina velutipes polysaccharides. Appl Sci. (2021) 11:1736. doi: 10.3390/app11041736
17. Tang W, Lin LH, Xie JH, Wang ZJ, Wang H, Dong YJ, et al. Effect of ultrasonic treatment on the physicochemical properties and antioxidant activities of polysaccharide from Cyclocarya paliurus. Carbohydr Polym. (2016) 151:305–12. doi: 10.1016/j.carbpol.2016.05.078
18. Xie JH, Liu X, Shen MY, Nie SP, Zhang H, Li C, et al. Purification, physicochemical characterization and anticancer activity of a polysaccharide from Cyclocarya paliurus leaves. Food Chem. (2013) 136 1453–60. doi: 10.1016/j.foodchem.2012.09.078
19. Liu R, Xiao N, Tian K. The role of PMN apoptosis delay in acute lung injury induced by administrating LPS. Zhonghua Yi Xue Za Zhi. (2001) 81:617–21. (in Chinese).
20. Lainiol M, Linden AM, Aitta-aho T. Behavioral responses of mGluR3-KO mice to the lipopolysaccharide-induced innate inflammatory reaction. Pharmacol Biochem Behav. (2020) 190:172852. doi: 10.1016/j.pbb.2020.172852
21. Wei MJ, Wang ZN, Yang Y, Zhang SJ, Bi CL. Selenium attenuates S. aureus-induced inflammation by regulation TLR2 signaling pathway and NLRP3 inflammasome in RAW 264.7 macrophages. Biol Trace Elem Res. (2021) 2021:1–7. doi: 10.1007/s12011-021-02676-4
22. Qin H, Huang L, Teng J, Wei B, Xia N, Ye Y. Purification, characterization, and bioactivity of Liupao tea polysaccharides before and after fermentation. Food Chem. (2021) 353:129419. doi: 10.1016/j.foodchem.2021.129419
23. Li HL, Liu S, Liu Y, Li WN, Niu AJ, Ren P, et al. Effects of in vitro digestion and fermentation of Nostoc commune Vauch. polysaccharides on properties and gut microbiota. Carbohyd Polym. (2022) 281:119055. doi: 10.1016/j.carbpol.2021.119055
24. Wu D, Yuan Q, Guo H, Fu Y, Li F, Wang S, et al. Dynamic changes of structural characteristics of snow chrysanthemum polysaccharides during in vitro digestion and fecal fermentation and related impacts on gut microbiota. Food Res Int. (2021) 141:109888. doi: 10.1016/j.foodres.2020.109888
25. Luo Z, Wang L, Zhou P, Feng R, Li X. Effect of in vitro simulated gastrointestinal digestion on structural characteristics and anti-proliferative activities of the polysaccharides from the shells of Juglans regia L. Food Chem Toxicol. (2021) 150:112100. doi: 10.1016/j.fct.2021.112100
26. Qi X, Tester RF. Starch containing formulations for diarrhea therapy. Clin Nutr ESPEN. (2018) 122:572–7. doi: 10.1016/j.clnesp.2018.08.003
27. Qi X, Tester RF. Utilization of dietary fiber (non-starch polysaccharide and resistant starch) molecules for diarrhea therapy: a mini-review. Int J Biol Macromol. (2019) 122:572–7. doi: 10.1016/j.ijbiomac.2018.10.195
28. Qi YL, Chen LX, Gao K, Shao ZJ, Huo XH. Effects of Schisandra chinensis polysaccharides on rats with antibiotic-associated diarrhea. Int J Biol Macromol. (2019) 124:627–34. doi: 10.1016/j.ijbiomac.2018.11.250
29. Chen Y, Zhang Y, Luo QY, Zhu YJ, Du HJ, Liao SY, et al. Inhibition of porcine epidemic diarrhea virus by Alpiniae oxyphyllae fructus polysaccharide 3. Res Vet Sci. (2021) 141:146–55. doi: 10.1016/j.rvsc.2021.10.026
30. Chen Y, Luo QY, Li SM, Li CH, Liao SY, Yang X, et al. Antiviral activity against porcine epidemic diarrhea virus of Pogostemon cablin polysaccharide. J Ethnopharmacol. (2020) 259:113009. doi: 10.1016/j.jep.2020.113009
31. Wang Z, Wang Z, Huang W, Suo J, Zhang H. Antioxidant and anti-inflammatory activities of an anti-diabetic polysaccharide extracted from Gynostemma pentaphyllum herb. Int J Biol Macromol. (2020) 145:484–91. doi: 10.1016/j.ijbiomac.2019.12.213
32. Jiang F, Ding YY, Tian Y, Yang RX, Quan ML, Tong ZY, et al. Hydrolyzed low-molecular-weight polysaccharide from Enteromorpha prolifera exhibits high anti-inflammatory activity and promotes wound healing. Mater Sci Eng C Mater Biol Appl. (2021) 112637. doi: 10.1016/j.msec.2021.112637
33. Huang L, Shen M, Morris GA, Xie J. Sulfated polysaccharides: immunomodulation and signaling mechanisms. Trends Food Sci Technol. (2019) 92:1–11. doi: 10.1016/j.tifs.2019.08.008
34. Hou C, Chen L, Yang L, Ji X. An insight into anti-inflammatory effects of natural polysaccharides. Int J Biol Macromol. (2020) 153:248–55. doi: 10.1016/j.ijbiomac.2020.02.315
35. Zhang L, Ma L, Pan Y, Zheng X, Qiao H. Effect of molecular weight on the antibacterial activity of polysaccharides produced by Chaetomium globosum CGMCC 6882 Int J Biol Macromol. (2021) 188:863–9. doi: 10.1016/j.ijbiomac.2021.08.059
36. Tsopmejio ISN, Ding M, Wei JL, Zhao C, Jiang Y, Li YT, et al. Auricularia polytricha and Flammulina velutipes ameliorate inflammation and modulate the gut microbiota via regulation of NF-κB and Keap1/Nrf2 signaling pathways on DSS-induced inflammatory bowel disease. Food Biosci. (2022) 47:101426. doi: 10.1016/j.fbio.2021.101426
37. Han R, Wang L, Zhao ZG, You LJ, Pedisićd S, Kulikouskaya V, et al. Polysaccharide from Gracilaria Lemaneiformis prevents colitis in Balb/c mice via enhancing intestinal barrier function and attenuating intestinal inflammation. Food Hydrocoll. (2020) 109:106048. doi: 10.1016/j.foodhyd.2020.106048
38. He Y, Hara H, Núez G. Mechanism and regulation of NLRP3 inflammasome activation. Trends Biochem Sci. (2016) 41:1012–21. doi: 10.1016/j.tibs.2016.09.002
39. Hou B, Zhang Y, Liang P, He Y, He X. Inhibition of the NLRP3-inflammasome prevents cognitive deficits in experimental autoimmune encephalomyelitis mice via the alteration of astrocyte phenotype. Cell Death Dis. (2020) 11:377. doi: 10.1038/s41419-020-2565-2
40. Pan XL, Wang HY, Zheng ZM, Huang X, Yang LL, Liu JX, et al. Pectic polysaccharide from Smilax China L. ameliorated ulcerative colitis by inhibiting the galectin-3/NLRP3 inflammasome pathway Carbohyd Polym. (2022) 277:118864. doi: 10.1016/j.carbpol.2021.118864
41. Castro-Alves VC, Shiga TM, Nascimento JRO. Polysaccharides from chayote enhance lipid efflux and regulate NLRP3 inflammasome priming in macrophage-like THP-1 cells exposed to cholesterol crystals. Int J Biol Macromol. (2019) 127:502–10. doi: 10.1016/j.ijbiomac.2019.01.048
Keywords: Flammulina velutipes polysaccharides, solid anaerobic fermentation, anti-inflammatory capacities, NLRP3 signaling pathway, antioxidant capacities
Citation: Ma S, Xu J, Lai T, Xu W, Zhang J, Zhang H and Zhang W (2022) Inhibitory Effect of Fermented Flammulina velutipes Polysaccharides on Mice Intestinal Inflammation. Front. Nutr. 9:934073. doi: 10.3389/fnut.2022.934073
Received: 02 May 2022; Accepted: 16 May 2022;
Published: 21 June 2022.
Edited by:
Bin Du, Hebei Normal University of Science and Technology, ChinaCopyright © 2022 Ma, Xu, Lai, Xu, Zhang, Zhang and Zhang. This is an open-access article distributed under the terms of the Creative Commons Attribution License (CC BY). The use, distribution or reproduction in other forums is permitted, provided the original author(s) and the copyright owner(s) are credited and that the original publication in this journal is cited, in accordance with accepted academic practice. No use, distribution or reproduction is permitted which does not comply with these terms.
*Correspondence: Hongcai Zhang, hczhang@sjtu.edu.cn; Jianxiong Xu, jxxu1962@sjtu.edu.cn; Weiyi Zhang, zhangharewei@163.com