- 1Department of Life Sciences, Presidency University, Kolkata, India
- 2Department of Botany, Dr. Kanailal Bhattacharyya College, Howrah, India
- 3Department of Chemistry, Faculty of Science, University of Hradec Kralove, Hradec Kralove, Czechia
- 4Department of Biotechnology, School of Engineering and Technology, Sharda University, Greater Noida, Uttar Pradesh, India
- 5Department of Biotechnology, School of Applied and Life Sciences, Uttaranchal University, Dehradun, India
- 6Department of Biotechnology Engineering and Food Technology, Chandigarh University, Mohali, India
- 7Chemical and Biochemical Processing Division, ICAR-Central Institute for Research on Cotton Technology, Mumbai, India
- 8School of Biological and Environmental Sciences, Shoolini University of Biotechnology and Management Sciences, Solan, India
- 9Department of Plant Biology, Institute of Environmental Biology, Wrocław University of Environmental and Life Sciences, Kożuchowska, Poland
- 10Biotechnology of Macromolecules, Instituto de Productos Naturales y Agrobiología, IPNA (CSIC). Avda, Astrofísico Francisco Sánchez, San Cristóbal de la Laguna, Spain
Allium sativum L. (Garlic) is a fragrant herb and tuber-derived spice that is one of the most sought-after botanicals, used as a culinary and ethnomedicine for a variety of diseases around the world. An array of pharmacological attributes such as antioxidant, hypoglycemic, anti-inflammatory, antihyperlipidemic, anticancer, antimicrobial, and hepatoprotective activities of this species have been established by previous studies. A. sativum houses many sulfur-containing phytochemical compounds such as allicin, diallyl disulfide (DADS), vinyldithiins, ajoenes (E-ajoene, Z-ajoene), diallyl trisulfide (DATS), micronutrient selenium (Se) etc. Organosulfur compounds are correlated with modulations in its antioxidant properties. The garlic compounds have also been recorded as promising immune-boosters or act as potent immunostimulants. A. sativum helps to treat cardiovascular ailments, neoplastic growth, rheumatism, diabetes, intestinal worms, flatulence, colic, dysentery, liver diseases, facial paralysis, tuberculosis, bronchitis, high blood pressure, and several other diseases. The present review aims to comprehensively enumerate the ethnobotanical and pharmacological aspects of A. sativum with notes on its phytochemistry, ethnopharmacology, toxicological aspects, and clinical studies from the retrieved literature from the last decade with notes on recent breakthroughs and bottlenecks. Future directions related to garlic research is also discussed.
Highlights
- Due to the numerous health benefits of Allium sativum, there is a growing interest in its use in various industries.
- Phytochemistry, ethnobotanical, and various pharmacological activities of A. sativum are extensively reviewed.
- Allium sativum contains various phytochemical compounds such as allicin, E-ajoene, and Z-ajoene that are of various therapeutic importance.
- Some of the sulfur-containing compounds extracted from A. sativum are reviewed along with their structures.
- Toxicological and clinical studies of A. sativum are summarized.
Introduction
Allium sativum L. (Garlic) belongs to the family Amaryllidaceae, has been originated in Asia, and is also widely cultivated in Egypt, Mexico, China, and Europe (1). This plant is highly consumed in Iran, where its foliage, flowers, and cloves are employed in local medicine (1). All parts of A. sativum, bulbs, leaves, cloves, and flowers are utilized to prepare mixtures and decoctions to deal with various ailments. It is also a common spice and food additive. Studies on its phytochemistry indicate that sulfur-containing compounds, such as allicin, are the essential components. Allicin (diallyl-dithiosulfinate) is the most important alkaloid responsible for its beneficial effects. Other sulfur-containing phytochemicals found in A. sativum include diallyl disulphide (DDS), diallyl trisulfide (DTS), and S-allyl cysteine (SAC), which have a variety of pharmacological properties (1). In India, A. sativum is used to treat fever, coughs and is administered topically against scabies, graying of hair, and eczema, as well as against inflammation of the tetanus and lungs (2). In Pakistan, the plant extract is consumed orally against stomach ailments, respiratory problems, and fever. In Nepal, the Middle East and East Asia, the plant is applied against fevers, rheumatism, liver disorders, diabetes, colic, intestinal worms, dysentery, flatulence, tuberculosis, high blood pressure, facial paralysis, and bronchitis. In Africa, the plant has been reported to be an antibiotic, antiviral, hypolipidemic, hypoglycemic, and antithrombotic (2–4). In this review, the phytotherapeutic properties of garlic have been comprehensively investigated to provide an updated overall view of one of the most used (and best-selling) medicinal and food plants in the world with notes on ethnobotanical information validated by preclinical bioactivities (i.e., in vitro and in vivo), emphasizing the mechanisms and signaling pathways involved. We have also discussed the recent breakthroughs and bottlenecks of relevant research on garlic with notes on future perspectives on garlic research.
Botany and geographical distribution of Allium sativum
A. sativum L. (Figure 1), commonly known as garlic, belongs to the family Amaryllidaceae (5). The bulb is mostly used to treat ailments and the perennial herbaceous plant is large, with upright flowering stems that extend up to 1 m (6). The leaf blades are linear, flattened, robust, and approximately 0.5--1.0 inch (1.25--2.5 cm) long, with a pointed apex and violet to fuchsia flowers that bloom in the Northern Hemisphere during monsoons. Slender leaves on the exterior of the odoriferous bulb surround an internal sheath containing the cloves, and each bulb contains 10--20 cloves.1 Its medical benefits have been documented in Sanskrit texts dating back about 5,000 years and it first appeared in traditional Chinese medicine (TCM) at least 3,000 years ago (5). Today, garlic is grown almost everywhere and is known to have more than 300 varieties (7). At present, A. sativum is cultivated around the world. It was first discovered in Central Asia, then spread throughout China, the Near East, and the Mediterranean before making its way to the southern and middle parts of Europe, Mexico, and northern Africa, especially Egypt (5). Garlic is a perennial herb that thrives in mild regions and can be grown all year. Sowing each clove in the ground is a method to propagate the plant in cultivation asexually. Cloves are usually sown 6 weeks before the land freezes in the cold season. The bulbs only produce roots and have no stems above the surface.
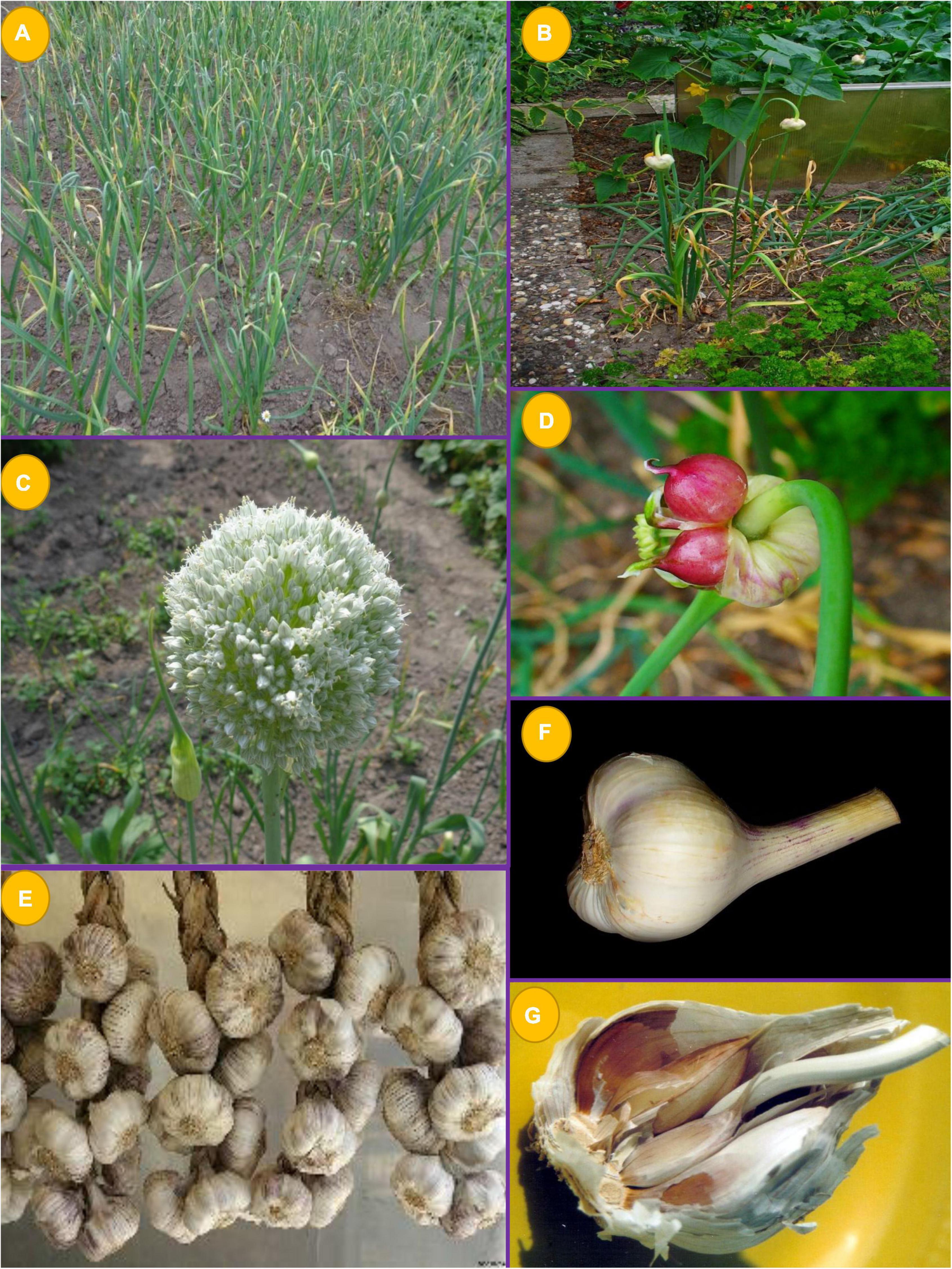
Figure 1. Different parts of garlic (A,B) habit; (C,D) flowers; (E–G) bulb (obtained from Wikimedia commons).
Phytochemistry of Allium sativum
A. sativum bulbs are reported to have many bioactive compounds, many of which are sulfur-containing, viz. thiosulfinates (allicin), sulfides [diallyl disulfide (DADS)], vinyldithiins (2-vinyl-(4H)-1,3-dithiin, 3-vinyl-(4H)-1,2-dithiin), ajoenes (E-ajoene and Z-ajoene) diallyl trisulfide (DATS), and so on constituting up to 82% of the total sulfur content in garlic. Allicin, S-methyl cysteine sulfoxide (MCSO), and S-propylcysteine sulfoxide (PCSO) are the main noxious compounds, with allicin being the predominant cysteine sulfoxide. Allicin, MCSO and PCSO, upon acted on by various enzymes, further produce molecules that include allyl methane thiosulfinates, methyl methanethiosulfonate and other thiosulfinates (8–10). Table 1 depicts a list of phytochemicals present in A. sativum with their molecular formula and IUPAC names. Figure 2 represents the chemical structures of phytochemicals (obtained from ChemSpider and PubChem).
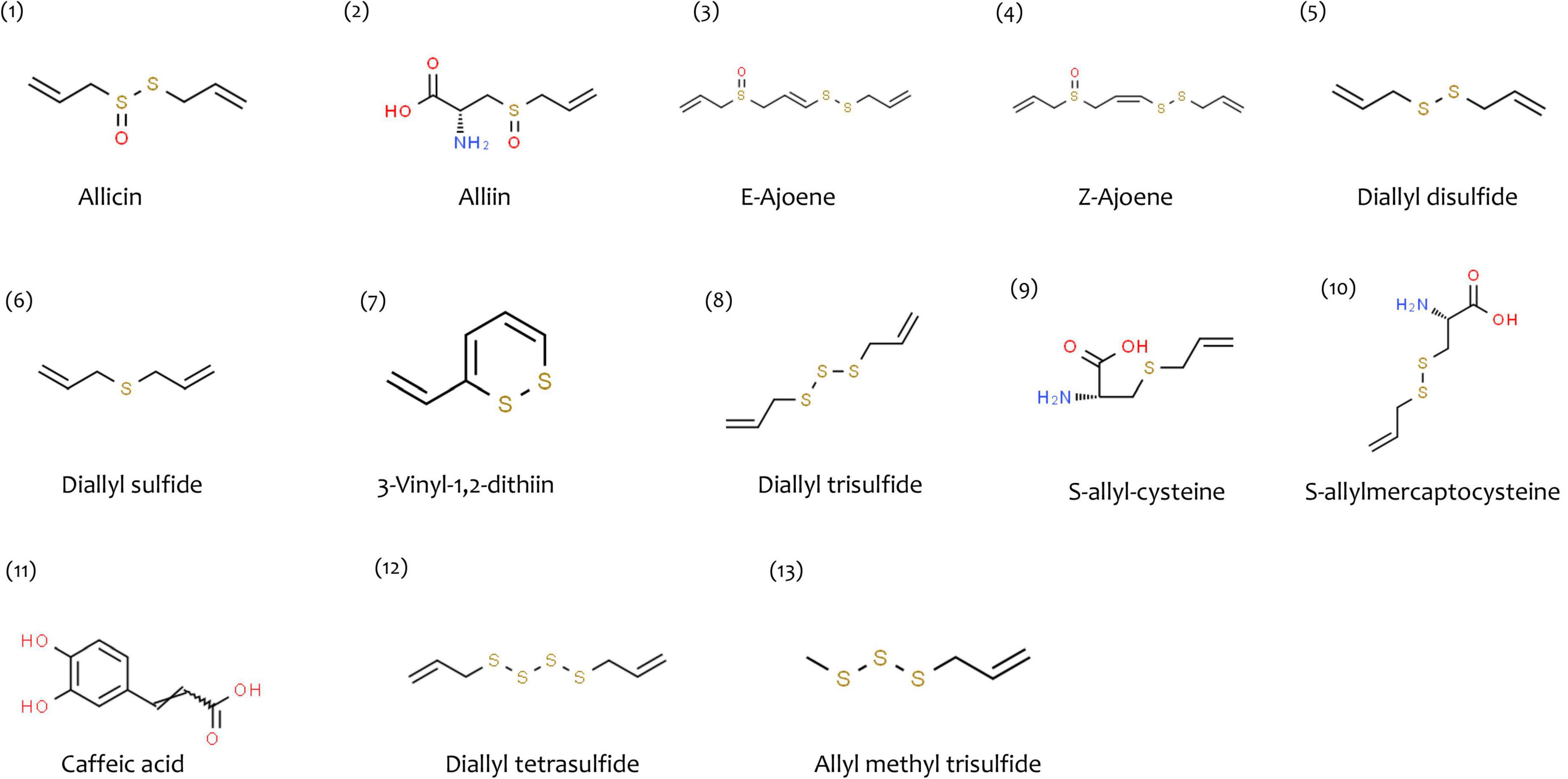
Figure 2. Structures of some of the phytochemicals reported from A. sativum (obtained from ChemSpider and PubChem).
Ethnobotanical uses of Allium sativum
Garlic is a well-known culinary ingredient and seasoning due to its strong aroma, which is attributed to organosulfur compounds such as allicin and DADS. Garlic’s promising therapeutic advantages in ethnomedicine include its application against hypertension, pneumonia, hair loss, snakebite, diabetes, wounds, cough, paralysis, scabies, malaria, hemorrhoids, carbuncles, heart diseases, asthma, pain, respiratory disorders, influenza, female infertility, etc., which are mainly attributed to its antidiabetic, anti-atherosclerotic, antimicrobial, antihypertensive, anticancer, cardioprotective, diuretic, aphrodisiac, sedative, carminative, and antipyretic properties evidenced by various studies. Supplementary Table 1 shows the ethnobotanical and ethnotherapeutic uses of A. sativum with information on the place of the report, local names, using ethnic groups, modes of administration, preparatory techniques and applied dosages.
Pharmacology of Allium sativum
The ethno-medicinal applications of A. sativum have been studied in a number of pharmaceutical studies and clinical investigations. The next section will gather the results of in vitro, in vivo, and ex vivo therapeutic studies using the plant to investigate pharmacological functions. The pharmacokinetic characteristics of A. sativum are shown in Table 2.
Biological activity of plant extracts
Antioxidant activity
According to research, A. sativum possesses high antioxidant properties. A study compared the antioxidant qualities of raw and cooked cloves, finding that uncooked garlic has an intense antioxidant potential, while prepared ones have extensive antioxidant effects due to -carotene decolorization (11). Oxygen radical absorption capacity (ORAC) and DPPH experiments with HT22 rodent hippocampus cell culture revealed that the ethanolic infusion of garlic seedlings had antioxidant properties by DPPH scavenging activity and suppression of ROS (12). AG or aged garlic, as well as NG or non-aged garlic by-products that were extracted with distilled water, ethyl alcohol, or dichloromethane, showed distinct antioxidant effects as measured by radical scavenging tests ABTS, DPHH and H2O2, total Fe3+ reducing antioxidant power (FRAP) assay and Fe2+ chelating experiment (13). Unlike MCG or multi clove garlic isolates, SCG or single clove garlic ones exhibited a substantial increase in scavenging activity in the DPPH test and superoxide radicals. SCG is more resistant to CCl4-induced hepatotoxicity than MCG, and it may be an effective substitute drug for severe oxidative hepatotoxicity (14).
Hepatoprotective activity
The liver-protecting potential of fermented garlic extracts produced by lactic acid bacteria toward acetaminophen (AAP) resulted in acute liver damage in rodents was discovered in a study. Bacteria suppress AAP-induced cell death in hepatocytes by inhibiting the MAPK phosphorylation route and down-regulating p53, which is involved in liver autophagy, and by cytoplasmic redox command, as evidenced by reduction of oxidative stress, glutathione and ATP exhaustion, and antioxidant enzyme actions (15). Further analysis revealed that alcohol administration increased ROS/RNS generation in various animals. It depleted liver antioxidant status, decreased liver glutathione (GSH) concentrations, and decreased superoxide dismutase activity (SOD). Only garlic compounds can shield the liver from ethanol-induced peroxidation, as evidenced by a decrease in the marker of oxidative damage (malondialdehyde, MDA) and recovery of hepatoprotective action (16). The consequences of aqueous Garlic bulb isolate on alloxan-induced plasma advancements in liver enzymes and urinary metabolic indicators in Wistar rats were examined. The liver enzymes alanine transaminase, aspartate transaminase, and alkaline phosphatase have been associated with the leakage of cytoplasm from hepatocytes into the circulation as a condition of hepatotoxicity. The result showed that when compared to control, the plasma levels of all the parameters evaluated in the rats given garlic [urea, creatinine, albumin, aspartate transaminase (AST), alanine transaminase (ALT), and alkaline phosphate (ALP)] were unaltered (17). In the livers of albino rodents, Kaur and Sharma (18) found that ethanol extracts of garlic and ascorbic acid mixtures have hepatoprotective action toward Cd damage. Cadmium administered mice had a substantial increase in the amount of malignant growth, such as multinucleated nuclei and gigantic cells, which was reversed by administering ascorbic acid and ethanolic extract, which provided protection against the toxicity. Garlic also show hepatoprotective properties due to its organosulfur compounds like diallyldisulfide (DDS) and diallyltrisulfides (DTS), which have antioxidant and detoxifying properties. These compounds can reduce Cd-induced oxidative stress below the threshold level, produce preconditioning effects by activating survival signals, and activate DNA repair systems by reducing the binding of Cd with DNA (18).
Anti-inflammatory activity
In lipopolysaccharide treated J774A.1 macrophages (19) probed the anti-inflammatory attributes of Allium 14-kDa protein, something that impeded inflammatory agents such as nitric oxide (NO), prostaglandin E (PGE), TNF-, and interleukin (IL)-1 by impairing the nuclear factor-kappa B (NF-kB) signaling route (19). Morihara et al. (20) discovered that AG extract has a significant anti-inflammatory action and can assist to inhibit inflammatory responses in mouse models with apolipoprotein E knockout (ApoE-KO). In hepatocytes, AG treatment decreased TNF constructions, a prominent stimulus resulting in CRP synthesis, by 35%, downregulated interleukin-1 receptor-associated kinase 4 (IRAK4) by 60%, and increased adenosine monophosphate-activated protein kinase (AMPK) production (20). Ethyl linoleate (ELA), an essential fatty acid separated from garlic cloves, was demonstrated to suppress inducible nitric oxide synthase (iNOS) formed as a result of LPS treatment, transcription of cyclooxygenase-2 (COX-2) and the generation of pro-inflammatory cytokines. The inactivation of NF-B as well as the MAPKs and phosphorylation of the Akt circuits were responsible for this action. In RAW 264.7 cells, ELA-triggered heme oxygenase-1 (HO-1) regulates the reduction of LPS-induced NO and pro-inflammatory cytokine synthesis. In this study, the anti-inflammatory effects of ELA were established and ELA could be employed as a medical therapy to treat inflammation-related disorders (21). In BALB/c mice experiencing schistosomiasis, Metwally et al. (22) evaluated the possible anti-inflammatory effect of garlic extract and allicin against liver inflammatory indicators (S. mansoni infection). Both preventive and therapeutic injection of garlic extract or allicin into affected mice showed considerable immunomodulatory and anti-inflammatory benefits in this investigation. The immunohistochemical production of fibronectin and -SMA, as well as the transcription of inflammatory cytokines mRNA as indicators of liver fibrosis, indicate these consequences. Garlic significantly inhibited inflammatory cytokine expression, suggesting that the altered Th1/Th2 cytokine balance was the cause of the serum concentrations of liver fibrosis markers and proinflammatory cytokines. These markers were maintained by decreasing serum ALT and AST levels, granuloma size as well as the number of inflammatory cells, collagen fibers, and eggs in the granuloma (22).
Cardioprotective activity
Takashima et al. (23) found that the vasorelaxant impact of AG on the rat aorta had a substantial cardioprotective action in lowering arterial pressure. Endothelium-dependent vasorelaxation of the aorta is caused by AGE, which promotes vasodilation by increasing the synthesis of NO controlled by eNOS. L-arginine, a NOS precursor, was found to be one of the key elements of the AGE’s vasorelaxant action. Additionally, the source of AGE’s action to reduce arterial pressure in drug testing and in vivo testing remains unknown (23). On 22 total cholesterol tests (TC), 17 LDL cholesterol experiments (LDL-C), 18 HDL cholesterol tests (HDL-C), four fasting blood glucose tests (FBG), nine tribulations of systolic blood pressure (SBP) and 10 tests of diastolic blood pressure (DBP), A. sativum particle isolate was found to have cardioprotective activity. Garlic flour formulations were found to be a universal cardiac and circulatory tonic, lowering blood TC, LDL-C, HDL-C, FBG, SBP, and DBP (24). Another study looked at the effects of fermented garlic extract (FGE) on pulmonary arterial hypertension in rats given monocrotaline (MCT). In the right ventricular, MCT treatment increased weight, arterial stiffness, and atrial natriuretic peptide levels, although not in the left ventricle. FGE decreased these impacts, as well as pulmonary arteriole endothelial dysfunction and medial hypertrophy, pulmonary fibrosis produced by translations of PKG, MCT, and eNOS proteins in the lung, and increased translations of the VCAM-1 and MMP-9 proteins in the lung. FGE also inhibited an available guanylyl cyclase (sGC) inhibitor. The impact of FGE on MCT-induced heart attack in rats has been found to have cardioprotective effects, according to this research (25).
Anticancer activity
In rodents with 1,2-dimethylhydrazine (DMH)-induced tumorigenesis and multiplication of human colon tumor cells, Jikihara et al. (26) studied the anticancer effects of alcohol extracts of A. sativum. Pathological study shows that A. sativum extract can decrease adenocarcinoma and adenoma. Jikihara et al. (26) investigated the cytotoxic activity of A. sativum aqueous preparations in rodents with 1,2-dimethylhydrazine (DMH)-induced carcinogenesis and human colorectal tumor cell proliferation. Adenocarcinoma and adenoma can be reduced by isolate of A. sativum, according to histopathological research. Apoptosis was not induced by AGE, but it did slow down cell cycle progression by decreasing the expression of cyclin B1 and cdk1 in human colorectal cancer cells (26). The impacts of garlic extract on the growth of human breast cancer cell lines (MCF-7), prostate (PC-3), liver (Hep-G2), and colon (Caco-2), as well as mice macrophage cells, were investigated in a study (TIB-71). Exposure of Hep-G2, MCF-7, TIB-71, and PC-3 cells with crude garlic excerpt decreased cell proliferation by 80–90%, but only 40–55% in Caco-2 cells. It also caused growth arrest and a four-fold increase in caspase activation (apoptosis) in PC-3 cells. According to this research, crude garlic powder contains reactive fatty components and could be employed as a chemoprevention (27). A study examined the antitumor properties of diallyl trisulfide (DATS). In vitro and in vivo treatment of patient gastric cancer cell SGC-7901 using a flavonoid molecule produced from A. sativum isolate. Treatment with DATS hindered SGC-7901 cell growth by inducing apoptosis and amplifying the MAPK route by phosphorylating JNK, ERK, and p38. It also inhibited invasiveness by attenuating the utterances of the MMP9 and E cadherin proteins, induce cell death and amplifies the MAPK route by initiating JNK, ERK, and p38. Furthermore, DATS therapy increased cytokine release, such as TNF- and IFN-, which promoted the inflammatory system of the host in cancer care (28).
Gastric cancer chemoprevention by allicin
Allicin, a component of A. sativum, was found to be effective against stomach cancer, the fifth most common cancer in the world. For a long time, the mode of action was unknown. Allicin functions by halting the cell cycle during the G2/M phase and apoptosis in gastric tumor cells, while normal stomach cells remain unaffected, according to numerous investigations. Furthermore, even in the scientific results, allicin-mediated inhibition of stomach tumorigenesis can be seen. TGF-alpha (TGF-) and its receptor epidermal growth factor receptor (EGFR) are both inhibited by allicin (EGFR). This causes cyclin E and cyclin D1 to be down-regulated, which can cause cells in the G2 phase to enter the M phase. Allicin can cause DNA damage by causing reactive oxygen species (ROS), resulting in phosphorylated P53 and P21 proteins. P21 then inhibits the cyclin-dependent kinase 4/6 (CDK4/6)-cyclin D complex, causing the cell to enter G2/M arrest.
Furthermore, the P21 polypeptide can block the P21-CDK2 and P21-proliferating cell nuclear antigen (PCNA) assemblages, causing the CDK1-cyclin B1 complex to diminish and the G2/M cell stage to be arrested. The particle’s lipid-soluble nature enables it to simply transfer across cellular barriers. Allicin significantly lowers the potential of the exterior mitochondrial membrane. Elevation in the proportion of pro-apoptotic polypeptide associated with BCL2 (BAX) to anti-apoptotic protein of B cell lymphoma 2 (BCL2) caused by allicin may promote cell death via the mitochondrial route. A greater BAX/BCL2 ratio causes cytochrome c to be released into the cytoplasm, accompanied by caspase-9 involvement. First, caspase-3 is triggered which results in poly ADP-ribose polymerase (PARP) and apoptosis-inducing polymerase (AIP) activation. Intrinsic stimulation of p38 mitogen-activated kinase or p38 MAPK activity was greater in allicin-induced gastric tumor cells. The synthesis of the Fas membrane-spanning peptide and P53 may be increased if p38 MAPK is increased. The Fas protein is part of the tumor necrosis factor or TNF receptor superfamily and contains 3 domains (extracellular, cytoplasmic, and transmembrane). The association of Fas’ cysteine-rich signal peptide with the Fas ligand (FasL) is a critical regulator of tumor growth (29). Caspase-8 is activated as a result of the interaction and can stimulate two separate apoptosis mechanisms. Caspases-8 trigger caspase-3 and caspase-7 in the mitochondrial independent route to trigger apoptosis. Caspase-8 is responsible for the liberation of Cyt c from mitochondria in the mitochondrial-dependent route (30). Allicin can also result in cell death through a process devoid of caspases. In this route, mitochondria generate apoptosis effectors such as apoptosis-inducing factor (AIF) and endonuclease G. (EndoG) which is the coactivator of AIF. EndoG and AIF are then teleported to the nuclei, where they lead to cell death through breaks in the nuclear material strands. Furthermore, allicin can cause apoptotic cell death by boosting unbound Ca2+ cation concentrations and inducing endoplasmic reticulum (ER) strain (Figure 3) (29).
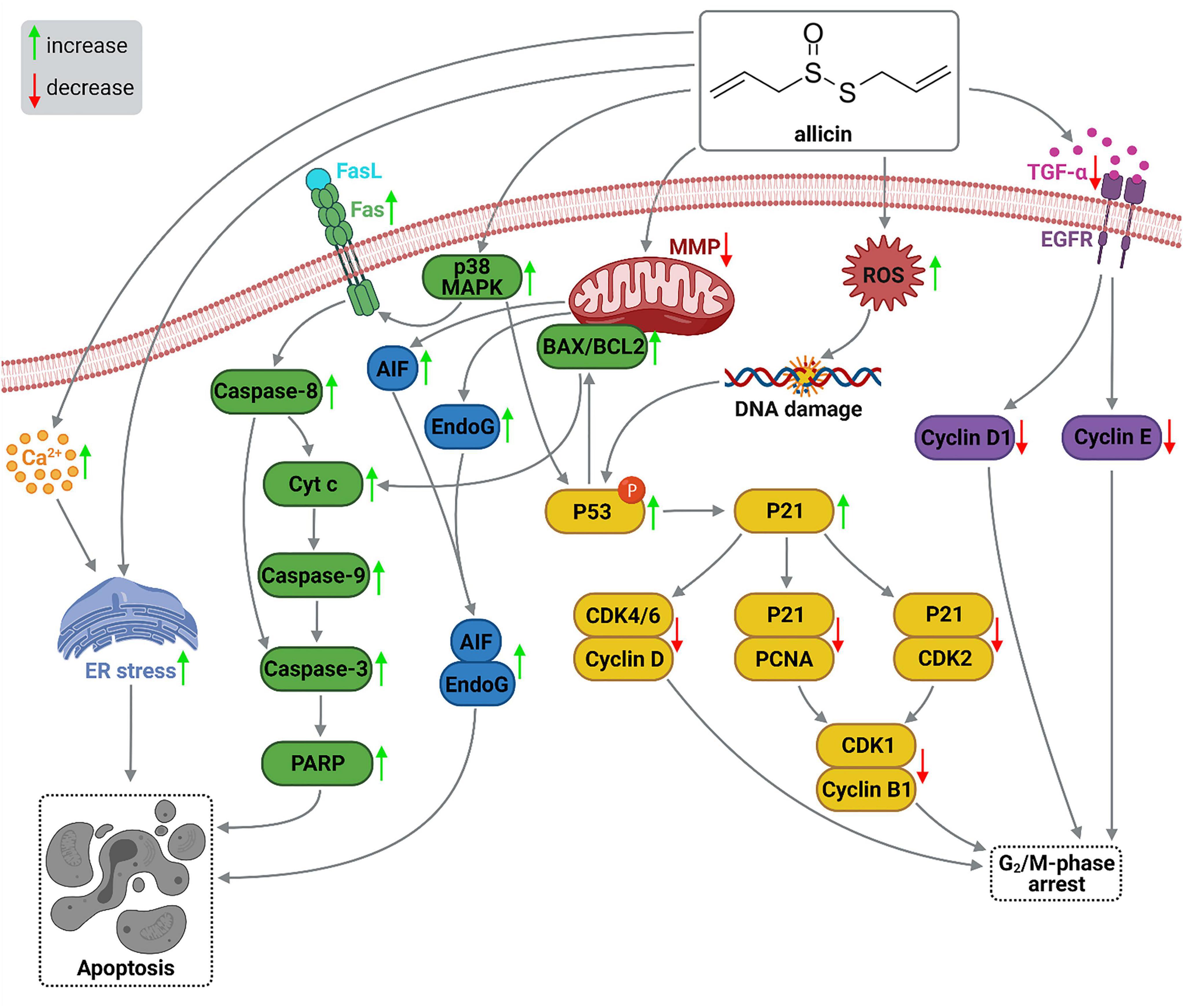
Figure 3. Allicin-mediated apoptosis signaling pathways in gastric cancer cells. AIF, apoptosis induce factor; BAX, BCL2-associated X protein; BCL2, B-cell lymphoma 2 protein; CDK, cyclin-dependent kinase; Cyt c, cytochrome c; EGFR, epidermal growth factor receptor; EndoG, endonuclease G; ER, endoplasmic reticulum; Fas, apoptosis antigen 1 protein; FasL, Fas receptor ligand; MAPK, mitogen-activated protein kinase; MMP, mitochondrial membrane potential; PARP, poly ADP-ribose polymerase; PCNA, proliferating cell nuclear antigen; ROS, reactive oxygen species; TGF-α, transforming growth factor alpha (29). This figure was prepared with BioRender.com.
Antimicrobial activity
The antibacterial activities of Allium sativum are extensive. Garlic essential oil [rich in diallyl monosulfide, diallyl disulfide (DADS), diallyl trisulfide, and diallyl tetrasulfide] extracted from raw bulbs had a good antibacterial action against Pseudomonas aeruginosa, Staphylococcus aureus, and Escherichia coli. The results suggest that the presence of the allyl group is essential for the antimicrobial property of such sulfide derivatives found in Allium (31). Additional studies have demonstrated that watery bulb infusion has high antibacterial efficacy against Bacillus subtilis, Staphylococcus aureus, Klebsiella pneumoniae, Escherichia coli, and Candida albicans due to disfunction in phospholipid bilayer synthesis by the activity of allicin (6). Garlic essential oil suppresses the fungal pathogen Penicillium funiculosum, presumably by infiltrating membranes and compartments, disrupting the cytoskeleton, and causing cytoplasmic and biomolecules leaks. In addition, proteomic analysis revealed the ability of garlic oil to increase or decrease the expression of few major proteins in relation to physiological metabolism (32). The antimicrobial effect of Australian garlic methanol and aqueous clove formulations was also described on Candida albicans, Bacillus cereus, Escherichia coli, Staphylococcus aureus, Listeria monocytogenes, Pseudomonas aeruginosa, and Rhodotorula mucilaginosa. The identification and quantification of pharmacologically potent compounds was done using ultra-high performance liquid chromatography with mass spectrometry and photodiode array detection (UHPLC-PDA-MS) and a correlation assessment was performed between the compounds and antioxidant and antimicrobial properties (33).
Antidiabetic activity
According to a research article, due to enhanced insulin-like efficacy, the antidiabetic behavior of A. sativum ethyl ether extract (at 0.0025 g/kg) was investigated in alloxan-induced diabetic rodents. The parietal cells of the pancreas are stimulated by ingestion of ethanol excerpt, pulp, and oil. Allicin boosted liver metabolism and pancreatic β cell insulin response (34). Another investigation found that 3 progressive doses of aged AE extracts have hypoglycemic activities in rats treated with streptozotocin. Diabetic rats were divided into two groups: control diabetics (CD), which served as negative control, and AGE-treated diabetics (AGE-D). AGE-Ds were classified into 3 groups and given AGE at 600, 300, and 100 mg/kg every day for a period of 2 months, as well as a normal control group (CN) which was the positive control for comparison. CD rodents had higher sugar levels (almost four times), twice the amount of serum cholesterol, triglycerides, and erythrocyte glycosylated hemoglobin, and thrice the amount of kidney and liver fatty acid oxidation, compared to the CN cohort. In the streptozotocin-treated diabetic group, the findings revealed that AGE had a dosage-dependent mitigative effect on hyperglycemia markers (35). In prediabetic expectant mothers, Faroughi et al. (36) evaluated the effects of the A. sativum tablet on the primary outcomes of fasting blood sugar or FBS and the recurrence of signs of prediabetes, as well as secondary outcomes such as hypertension, infant anthropometric parameters, and delivery method. Throughout 2015–2016, 49 mothers with prediabetes at 24–28 weeks of gestation were included in the triple-blind, randomly selected controlled study in Tabriz, Iran. The average concentration of FBS in the garlic-treated cohort reduced from 106.6 (11.1) mg/dL before therapy to 83.6 (6.3) mg/dL after 4 weeks and 79.4 (6.1) mg/dL 8 weeks later, according to the findings. The garlic drug also resulted in a substantial reduction in pre-diabetic signs at 4 weeks after therapy and diastolic arterial tension at 4 and 8 weeks. There was no substantial difference in systolic pressure between the cohorts at 4 and 8 weeks after treatment. As a result, the A. sativum tablet reduced FBS levels, prediabetes indicators, and diastolic BP in this study (36).
Antiviral activity
A. sativum has long been traditionally used to cure a variety of viral illnesses. Garlic isolates or compounds have been shown to have antiviral action in several investigations (Figure 4). Extracts or isolates include chemical constituents that can attack different stages of the viral life cycle. Prevention of viral infection can be as simple as inhibiting the virus’s admission stage. Both encapsulated and non-enveloped pathogens are destroyed by this technique. A. sativum and its potent organosulfur components have been shown to promote antiviral activity by interacting with components on the exterior of the virion. This results in partial inhibition or complete blocking of the virus entry step (3). The aqueous extract of A. sativum expressed an effective inhibition of viral infection of influenza A subtype H1N1 with EC50 = 0.01 mg/ml in the Madin-Darby canine kidney (MDCK) cell line (37). The exact value of EC50 (0.01 mg/ml) was observed against Measles morbillivirus in Vero cells treated with gold nanoparticles containing aqueous extracts of A. sativum because it causes an obstruction of the viral receptors, stopping cell adsorption and the start of viral infection in the host cell (38). Furthermore, garlic extracts inhibited the binding of Newcastle disease virus (NDV) to chick embryo cell receptors (39). Quercetin, a flavonoid commonly present in fruits and vegetables, including A. sativum, possesses antiviral properties against influenza virus and enterovirus by affecting viral attachment to the surface of the host cell. Furthermore, organosulfur compounds in garlic such as allicin, ajoene, and diallyl trisulfide play an important role in the antiviral properties of garlic. These substances can prevent a virus from attaching to a host cell, change how the viral genome is translated in the host cell, and affect viral RNA polymerase, which is required for viral replication. They can also prevent the viral process that changes the host cell’s signaling pathway, and prevent viral multiplication (40). Even if the virus enters the host cell, there are still various steps suitable for its inhibition. One of these represents inhibition in the viral replication step. Replication may occur in the cytoplasm or in the nucleus of the host cell. In the current history, numerous investigations have proven the ability of A. sativum to prevent viral proliferation. The methanolic and ethanolic garlic extracts revealed inhibition of viral RNA polymerase and nucleoprotein synthesis activity against the influenza A (H1N1) pdm09 virus. This property may be attributed to their ability to block viral attachment and to suppress viral hemagglutinin (HA) (41). Furthermore, the study evaluating the influence of aqueous garlic extracts on avian infectious bronchitis virus (IBV), a coronavirus that infects birds of the Coronaviridae family, suggested the potential of A. sativum to inhibit the viral replication step (42). The members of the retrovirus family utilize reverse transcriptase (RT) to convert viral RNA into DNA. As a result, RT suppression is a major clinical strategy in the treatment of retrovirus transmission (3). The hexane extracts of A. sativum expressed a powerful activity against the RT of human immunodeficiency virus 1 (HIV-1) with an IC50 of approximately 64.08 ± 1.09 μg/mL (43). Furthermore, allicin was evaluated in the study to relieve immune dysfunction caused by the reticuloendotheliosis virus (REV). The study suggests that allicin downregulated the ERK/MAPK signaling pathway resulting in inactivation of REV replication (3, 44).
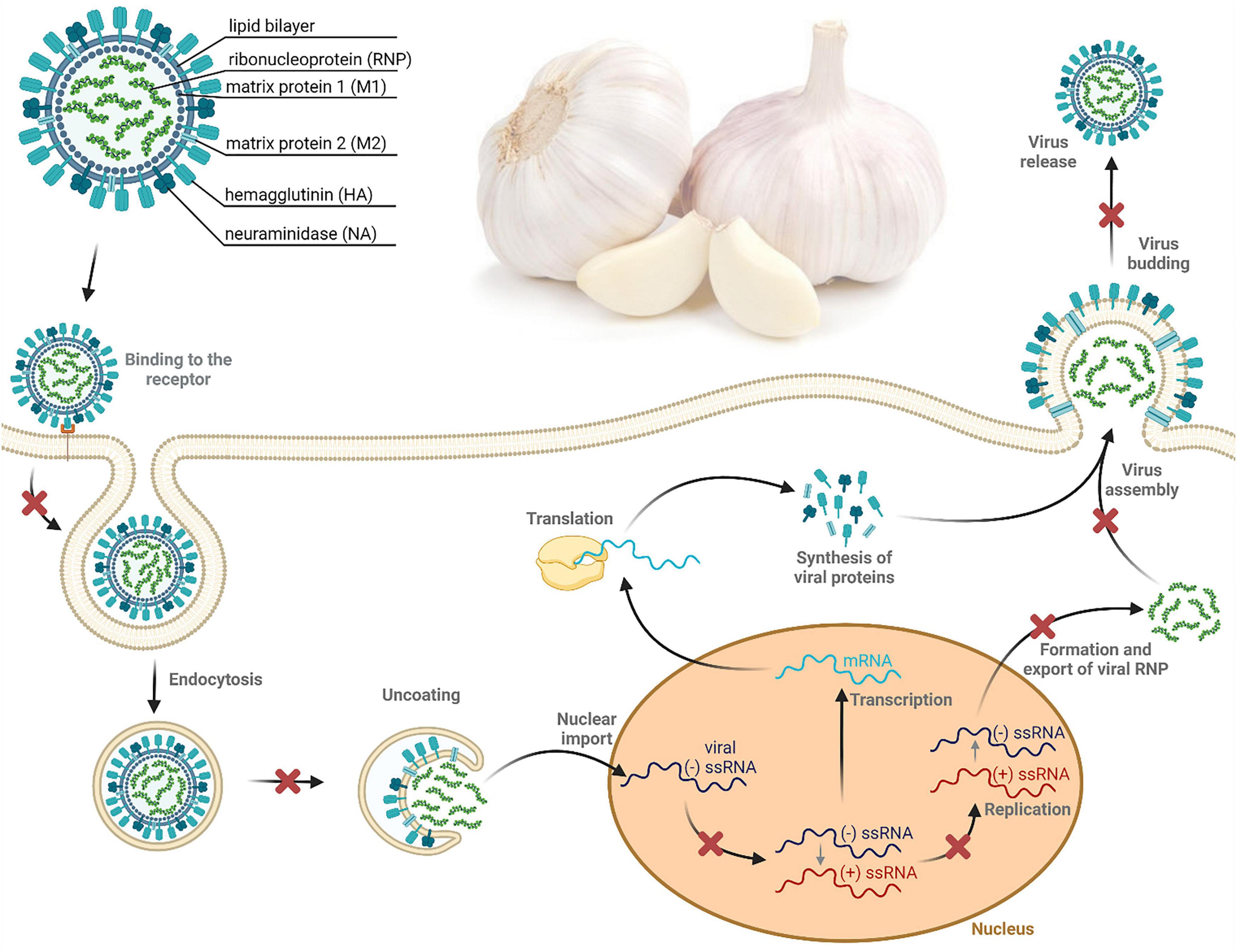
Figure 4. Proposed antiviral effects of A. sativum against (–) ssRNA viruses. This figure depicts the life cycle steps of influenza A with possible inhibition (shown as a red X-shaped symbol) mediated by garlic extracts or its organosulfur constituents. As shown in the figure, A. sativum bears potential for the prevention of viral penetration into the host membrane, virus uncoating associated with the release of viral genetic material into the cytoplasm, conversion of viral (–) ssRNA to viral (+) ssRNA, replication of the viral RNA, virus assembly and release (3). Figure made using BioRender.com.
The World Health Organization (WHO) proclaimed a worldwide pandemic of COVID-19 on March 11th, 2020, triggered by SARS-CoV-2. Although various studies have been reported for the treatment of COVID-19, an effective remedy is still missing. However, several herbal or pharmaceutical drugs have been reported with possible beneficial effects on COVID-19. One of them is A. sativum. As mentioned above, aqueous garlic extracts possess an inhibitory effect against IBV of the Coronaviridae family. Therefore, it is assumed that it may also be beneficial against other members of the virus family, including SARS-CoV-2. Furthermore, some constituents of A. sativum seem to be helpful in reducing viral replication. Quercetin, a flavonoid present in garlic, bears the potential to inhibit the main protease of SARS-CoV-2 (Mpro), a crucial antiviral target (45). As reported before, quercetin-3-β-galactoside was a suppressor of SARS-CoV-1 Mpro with IC50 = 42.79 ± 4.97 μM (46). Furthermore, there is a high structural similarity of Mpro between SARS-CoV-1 and 2 (∼96%). In silico molecular docking of organosulfur compounds in A. sativum revealed an inhibitory potential of alliin against Mpro SARS-CoV-2. The results suggested that alliin has potential antiviral activity against COVID-19 by binding to Mpro of SARS-CoV-2 (47). On the other hand, after the coronavirus outbreak, various hoaxes have been posted on the Internet about preventing COVID-19. Some of them argued that: “eating garlic prevents from COVID-19” (48). However, the WHO subsequently declared that there is no indication that garlic consumption has safeguarded patients from the novel coronavirus in the recent outbreak. Indeed, to date, no studies have been reported to confirm the prevention of A. sativum against COVID-19. However, there are no doubts about the beneficial effects of garlic on human health. In addition, studies recently reported confirming that despite garlic’s inability to prevent COVID-19, its antiviral activity can play a crucial role in alleviating patients suffering from COVID-19.
Toxicological studies
Many investigations have been conducted on the toxicity assessment of A. sativum in laboratory animals. According to OECD standards 423, research explained the acute toxicity of A. sativum ethyl acetate concentrates and then determined the LD50 to demonstrate the acceptability of the extract in rodents (49). To test cytotoxic activity, all 3 animals in each set received ethanolic extract of A. sativum in a solitary administration of 3, 20, and 50 g/kg of lean mass. For each treatment, effects on body mass, skin tone, salivation, cornea, mucous system, drowsiness, tremor, unconsciousness, spasm, malaise, diarrhea, and death were observed after 30 min, 4 h, 24 h, 48 h, 1 week, and 2 weeks (49). Sections of the lungs, spleen, heart, kidneys, liver, and intestines were viewed during gross postmortem and microscopy using standard forensic methods. The rodents did not die as a consequence of the dose increase and there was no significant change in any of the criteria used for general post mortem and histopathological alteration. As a result, the extract is fine for humans to consume (49). Another investigation looked at whether A. sativum preparations had a suppressive effect on lead nitrate poisoning. Their potential to eliminate free radicals and avoid GSH deficiency could describe the function of garlic organosulfur compounds in reducing Pb(NO3)2 poisoning (50). Total erythrocyte count, hemoglobin concentration, total leukocyte count, lymphocyte and monocyte percentage all decreased significantly after chronic consumption of lead nitrate. In the renal and central nervous system, the intake of lead nitrate resulted in an increase in the levels of reactive components of thiobarbituric acid, as well as a reduction in glutathione peroxidase levels and antioxidant molecules such as superoxide dismutase and catalase. Nitrate aids lower peptide levels, whereas cholesterol and lead burden increase exponentially in lead-exposed mice, resulting in decreased plaque count, immunoglobulin level, phagocytic index, and macrophage viability (50). Orally ingested extracts of A. sativum by exposed groups to Pb(NO3)2, on the other hand, may be a preventive strategy against heavy metal toxicity (50). In cultivated Vero cells, Abid-Essefi et al. (51) explored the role of an aqueous extract of A. sativum (AEA) vs. zearalenone (ZEN) mediated lethality, reactive oxygen species (ROS), and genomic instability. The findings revealed that ZEN caused several harmful consequences and major changes in the body, which were regulated through the oxidative stress system. Administration with the smallest amount of AEA (250 g/ml) in combination with ZEN resulted in a considerable decrease in ZEN-induced impairments for each marker evaluated, as well as a notable fall in DNA disintegration. As a result, AEA can assist guards against ZEN risks. The great potential of AEA to compensate for the inflammatory process generated by ZEN is probably responsible for its preventive action (51). Many research has suggested that garlic could be used as a food additive for people who are not protected from ecological toxins. Irkin et al. (52) studied the effects of dietary garlic granules on young European sea bass (Dicentrarchus labrax). For 2 months, the fish received enhanced meals with A. sativum powder at concentrations of 0 (control), 2 percent, 4 percent, or 6 percent. The RBC count, hemoglobin level, amount of hemoglobin and mean corpuscular hemoglobin in treated fish at dietary incorporation stages of 4 and 6% were considerably less than baseline data after this study. In contrast to the reference cohort, serum sugar levels in fish supplemented with garlic powder were considerably lower, and cholesterol values in the treatment groups of 6 and 2% were smaller than those of the control group. As a consequence, it is recommended that garlic powder fortification in the diets of juvenile fish not surpass 2%. As a result, this experiment concluded that nutritional garlic powder is critical to improving the physiological and hematological state of immune improvements in young sea bass (52).
Clinical studies
A. sativum preparations are used in drug testing to alleviate a variety of human disorders. The bulbs have been used successfully as nutrition and treatment in human societies since antiquity. The possible hypoglycemic benefits of A. sativum in patients with type 2 diabetes were examined by Ashraf et al. (53). The purpose of this study was to compare the impact of garlic pills exposure to routine anti-diabetic analyzes on glucose levels in 60 confirmed type 2 diabetic patients with fasting blood glucose levels above 126 mg/dl. Participants were placed into two categories. Serum triglycerides and fasting blood glucose were measured at weeks 0, 12, and 24. Compared to group 2, group 1 reported a substantial reduction in fasting blood glucose at week 24. A mixture of A. sativum with traditional anti-diabetic medication improved glucose control and hypocholesterolemic action, according to this research. Garlic’s antilipidemic effects are thought to be caused by allicin’s ability to inhibit hydroxymethylglutaryl-CoA reductase, which lowers LDL-C levels as well as total cholesterol (53). Sukandar et al. (54) investigated the efficacy and tolerability of combining curcumin and A. sativum powder as an antidiabetic and hypocholesterolemic treatment for type 2 diabetes-dyslipidemia. 3 replicates were calculated which were 1.2, 1.6, and 2.4 g, respectively. The garlic-curcuma mixture was observed to lower glucose concentration and HbA1C while also improving dyslipidemia. The 2.4 g dose was found to reduce fasting glucose, glucose levels two hours after lunch, lipid profile, HbA1C, LDLs, cholesterol, and body mass index and increased HDLs greater than the other two prescriptions (54). The benefits of A. sativum supplementation on serum concentrations of certain markers of inflammation, somatic manifestations, and exhaustion in females with chronic rheumatoid arthritis or RA were studied by Moosavian et al. (55). In this investigation, a double-blind, placebo-controlled, randomized experiment was used. A group of 70 women with RA were randomly classified into two parts for 8 weeks, where the treatment group received 1,000 mg of A. sativum and the control team received a placebo (55). After therapy, the bioavailability of the C-reactive peptide (CRP) and TNF (TNF-a) was found to be considerably lower in the garlic cohort compared to the placebo category. Additionally, relative to the placebo group, pain severity, sensitive joint tally, disease activity score, and exhaustion were considerably lower, and the number of enlarged joints was markedly smaller in the garlic unit, but nothing of this type was demonstrated in the placebo. The result showed that garlic improved the inflammatory mediators like serum level of CRP and TNF-a, though there was no change in erythrocyte sedimentation rate (ESR) (55).
Discussion
A. sativum was found to have a spectrum of therapeutic benefits in this investigation, including antibacterial, cardioprotective, anti-inflammatory, antitumor, and antispasmodic activities, among others. The most essential components of A. sativum are organosulfur molecules, which are responsible for most of their medicinal uses. allyl methyl sulfide, Allicin, ajoene, and DTS are the primary physiologically active chemicals responsible for the antibacterial, antifungal, antiviral, and antiprotozoal effects of A. sativum, respectively (1). The main ingredient of garlic, allicin, can cause stomach disturbances, particularly when taken in large doses. Most cardiac investigations have focused on triglycerides, LDL, cholesterol level, HDL, and arteriosclerosis (56). According to suggestions, A. sativum lowers LDL and triglyceride concentrations in people with dyslipidemia (56). Consumption of A. sativum reduces triglyceride levels, which may help slow the progression of hypertension. In addition to considerable studies, its antidiabetic, antibacterial, anti-inflammatory, and antioxidant effects have been linked in various models. Several investigations have looked into the antitumor and anticarcinogenic properties of A. sativum and its components in vitro and in animal experiments. According to the findings, A. sativum encompasses a set of crucially significant compounds with anticancer and chemopreventive activities. Garlic includes various physiologically and pharmacologically important components, according to clinical and scientific research. For cardiac, hyperglycemia, rheumatism, gastrointestinal disorders, colitis, liver disorders, bloating, diarrhea, bronchitis, sensory loss, hypertension, asthma, and other ailments, these are vital for health. Several investigations are underway throughout the world to make efficient and unscented garlic formulations and identify bioactive constituents that may have medicinal value (57–59). However, the extraction, production, molecular, physicochemical and structural properties and structure–activity relationships of garlic compounds (60–64) are beyond the scope of the present review. The metabolic processes of ingested garlic constituents (as food or supplements), their mechanisms of action in healthy humans have not been detailed well. Besides structure-bioactivity relationships remain unclear in many pharmacological attributes.
Conclusion
For centuries, A. sativum has been widely consumed as a culinary and therapeutic herb as protective and curative agents. It contains essential minerals, vitamins, and protein and is widely used as a spice or condiment in continental cooking. In addition, this plant has various potential pharmacological activities against various diseases and disorders owing to its potent antioxidative, anti-inflammatory and immunomodulatory properties. Based on preclinical studies, A. sativum compounds especially the sulfur-containing compounds, some flavonoids and polyphenols may help treat certain human conditions, particularly those related to cancer and cardiovascular disease. The roles of A. sativum and the preparations of A. sativum in human health will help to benefit from additional well-designed human studies and various human diseases that carefully characterize the garlic interferences used and examine possible differential effects in several human populations. This research also looked at the key ingredients of the plant, such as sulfur-containing chemicals. Many significant advances have been found in the phytochemistry and related medicinal properties of A. sativum; data regarding clinical aspects and impact on human health-related properties are acceptable. Therefore, it is inferred that A. sativum is a fantastic seasoning that needs to be handled with prudence to fully benefit from its immense medicinal benefits, taking into account that misapplication may result in unintended consequences.
Author contributions
CT, TD, MG, PB, DS, PO, NJ, MK, Radha, JP, JMP, and AD contributed to the concept, literature mining, writing, and methodology of the review, provided critical feedback, and critically revised the manuscript. CT came up with the study idea, planned and designed the review structure, wrote the first draft of the manuscript, prepared the table and figures, and had the right to list his name first in his CV, any other scientific documents, and scientific profile. TD, MG, PB, and DS contributed to writing review and editing, arranged references, and revised the table and figures. PO contributed to study idea, writing-review and editing, prepared the table and figures, arranged the references, and acquired funding. NJ, MK, and Radha participated in review structure, writing-review and editing, completed the critical revision of the entire manuscript, response, data validation, and suggestions. JP completed the critical revision of the entire manuscript, formal interpretation, and supervised the drafting process of the review, response, final draft, and resources. JMP contributed to manuscript revision, formal interpretation, supervision, response, resources, project administration, and funding acquisition. AD came up with the study idea, revised the review structure, suggestions, completed the critical revision of the entire manuscript, formal interpretation, and supervised the drafting process of the review, resources, and final draft. All authors have read and approved the final version of the manuscript for submission to this journal and contributed to the writing or revision of the final manuscript.
Funding
This work was supported by the UHK (Project No. VT2019-2021), APOGEO (Cooperation Program INTERREG-MAC 2014–2020), with European Funds for Regional Development-FEDER, the “Agencia Canaria de Investigación, Innovación y Sociedad de la Información (ACIISI) Gobierno de Canarias” (Project No. ProID2020010134), and Caja Canarias (Project No. 2019SP43).
Acknowledgments
We are grateful to our respective departments/institutes for providing the space and other necessary facilities that helped draft this manuscript.
Conflict of interest
The authors declare that the research was conducted in the absence of any commercial or financial relationships that could be construed as a potential conflict of interest.
Publisher’s note
All claims expressed in this article are solely those of the authors and do not necessarily represent those of their affiliated organizations, or those of the publisher, the editors and the reviewers. Any product that may be evaluated in this article, or claim that may be made by its manufacturer, is not guaranteed or endorsed by the publisher.
Supplementary material
The Supplementary Material for this article can be found online at: https://www.frontiersin.org/articles/10.3389/fnut.2022.929554/full#supplementary-material
Footnotes
References
1. Subroto E, Cahyana Y, Tensiska M, Lembong F, Filianty E, Kurniati E, et al. Bioactive compounds in garlic (Allium sativum L.) as a source of antioxidants and its potential to improve the immune system: a review. Food Res. (2021) 5:1. doi: 10.26656/fr.2017.5(6).042
2. Qiu Z, Qiao Y, Zhang B, Sun-Waterhouse D, Zheng Z. Bioactive polysaccharides and oligosaccharides from garlic (Allium sativum L.): production, physicochemical and biological properties, and structure–function relationships. Comprehens Rev Food Sci Food Saf. (2022) 21:3033–95. doi: 10.1111/1541-4337.12972
3. Rouf R, Uddin SJ, Sarker DK, Islam MT, Ali ES, Shilpi JA, et al. Antiviral potential of garlic (Allium sativum) and its organosulfur compounds: a systematic update of pre-clinical and clinical data. Trends Food Sci Technol. (2020) 104:219–34. doi: 10.1016/j.tifs.2020.08.006
4. Bhatwalkar SB, Mondal R, Krishna SB, Adam JK, Govender P, Anupam R. Antibacterial properties of organosulfur compounds of garlic (Allium sativum). Front Microbiol. (2021) 12:613077. doi: 10.3389/fmicb.2021.613077
5. Londhe VP. Role of garlic (Allium sativum) in various diseases: an overview. Angiogenesis. (2011) 12:13.
6. Meriga B, Mopuri R, MuraliKrishna T. Insecticidal, antimicrobial and antioxidant activities of bulb extracts of Allium sativum. Asian Pacific J Trop Med. (2012) 5:391–5. doi: 10.1016/S1995-7645(12)60065-0
7. Majewski M. Allium sativum: facts and myths regarding human health. Roczniki Państwowego Zakładu Higieny. (2014) 65:1–8.
8. Al-Snafi A. Pharmacological effects of Allium species grown in Iraq. An overview. Int J Pharmaceutical Healthcare Res. (2013) 1:132–47.
9. Zeng Y, Li Y, Yang J, Pu X, Du J, Yang X, et al. Therapeutic role of functional components in alliums for preventive chronic disease in human being. Evid Based Complement Alternat Med. (2017) 2017:9402849. doi: 10.1155/2017/9402849
10. El-Saber Batiha G, Magdy Beshbishy A, Wasef L, Elewa YH, Al-Sagan A, El-Hack A, et al. Chemical constituents and pharmacological activities of garlic (Allium sativum L.): a review. Nutrients. (2020) 12:872. doi: 10.3390/nu12030872
11. Locatelli DA, Nazareno MA, Fusari CM, Camargo AB. Cooked garlic and antioxidant activity: correlation with organosulfur compound composition. Food Chem. (2017) 220:219–24. doi: 10.1016/j.foodchem.2016.10.001
12. Zakarova A, Seo JY, Kim HY, Kim JH, Shin JH, Cho KM, et al. Garlic sprouting is associated with increased antioxidant activity and concomitant changes in the metabolite profile. J Agric Food Chem. (2014) 62:1875–80. doi: 10.1021/jf500603v
13. Jang HJ, Lee HJ, Yoon DK, Ji DS, Kim JH, Lee CH. Antioxidant and antimicrobial activities of fresh garlic and aged garlic by-products extracted with different solvents. Food Sci Biotechnol. (2018) 27:219–25. doi: 10.1007/s10068-017-0246-4
14. Naji KM, Al-Shaibani ES, Alhadi FA, D’souza MR. Hepatoprotective and antioxidant effects of single clove garlic against CCl 4-induced hepatic damage in rabbits. BMC Complement Alternat Med. (2017) 17:411. doi: 10.1186/s12906-017-1916-8
15. Lee HS, Lim WC, Lee SJ, Lee SH, Yu HJ, Lee JH, et al. Hepatoprotective effects of lactic acid-fermented garlic extract against acetaminophen-induced acute liver injury in rats. Food Sci Biotechnol. (2016) 25:867–73. doi: 10.1007/s10068-016-0143-2
16. Guan MJ, Zhao N, Xie KQ, Zeng T. Hepatoprotective effects of garlic against ethanol-induced liver injury: a mini-review. Food Chem Toxicol. (2018) 111:467–73. doi: 10.1016/j.fct.2017.11.059
17. Aprioku JS, Amah-Tariah FS. Garlic (Allium sativum L.) protects hepatic and renal toxicity of alloxan in rats. J Pharmaceutical Res Int. (2017) 17:1–7. doi: 10.9734/JPRI/2017/34909
18. Kaur S, Sharma S. A histometric study to assess preventive action of ascorbic acid and garlic on cadmium induced hepatotoxicity in albino mice. Int J Pharmaceutical Phytopharmacol Res. (2015) 5:8–14.
19. Rabe SZT, Ghazanfari T, Siadat Z, Rastin M, Zamani Taghizadeh Rabe S, Mahmoudi M. Anti-inflammatory effect of garlic 14-kDa protein on LPS-stimulated-J774A. 1 macrophages. Immunopharmacol Immunotoxicol. (2015) 37:158–64. doi: 10.3109/08923973.2015.1005229
20. Morihara N, Hino A, Miki S, Takashima M, Suzuki JI. Aged garlic extract suppresses inflammation in apolipoprotein E-knockout mice. Mol Nutr Food Res. (2017) 61:1700308. doi: 10.1002/mnfr.201700308
21. Park SY, Seetharaman R, Ko MJ, Kim TH, Yoon MK, Kwak JH, et al. Ethyl linoleate from garlic attenuates lipopolysaccharide-induced pro-inflammatory cytokine production by inducing heme oxygenase-1 in RAW264. 7 cells. Int Immunopharmacol. (2014) 19:253–61. doi: 10.1016/j.intimp.2014.01.017
22. Metwally DM, Al-Olayan EM, Alanazi M, Alzahrany SB, Semlali A. Antischistosomal and anti-inflammatory activity of garlic and allicin compared with that of praziquantel in vivo. BMC Complement Alternat Med. (2018) 18:135. doi: 10.1186/s12906-018-2191-z
23. Takashima M, Kanamori Y, Kodera Y, Morihara N, Tamura K. Aged garlic extract exerts endothelium-dependent vasorelaxant effect on rat aorta by increasing nitric oxide production. Phytomedicine. (2017) 24:56–61. doi: 10.1016/j.phymed.2016.11.016
24. Kwak JS, Kim JY, Paek JE, Lee YJ, Kim HR, Park DS, et al. Garlic powder intake and cardiovascular risk factors: a meta-analysis of randomized controlled clinical trials. Nutr Res Pract. (2014) 8:644–54. doi: 10.4162/nrp.2014.8.6.644
25. Park BM, Chun H, Chae SW, Kim SH. Fermented garlic extract ameliorates monocrotaline-induced pulmonary hypertension in rats. J Funct Foods. (2017) 30:247–53. doi: 10.1016/j.jff.2017.01.024
26. Jikihara H, Qi G, Nozoe K, Hirokawa M, Sato H, Sugihara Y, et al. Aged garlic extract inhibits 1, 2-dimethylhydrazine-induced colon tumor development by suppressing cell proliferation. Oncol Rep. (2015) 33:1131–40. doi: 10.3892/or.2014.3705
27. Bagul M, Kakumanu S, Wilson TA. Crude garlic extract inhibits cell proliferation and induces cell cycle arrest and apoptosis of cancer cells in vitro. J Med Food. (2015) 18:731–7. doi: 10.1089/jmf.2014.0064
28. Jiang X, Zhu X, Huang W, Xu H, Zhao Z, Li S, et al. Garlic-derived organosulfur compound exerts antitumor efficacy via activation of MAPK pathway and modulation of cytokines in SGC-7901 tumor-bearing mice. Int Immunopharmacol. (2017) 48:135–45. doi: 10.1016/j.intimp.2017.05.004
29. Luo R, Fang D, Hang H, Tang Z. The mechanism in gastric cancer chemoprevention by allicin. Anti Cancer Agents Med Chem. (2016) 16:802–9. doi: 10.2174/1871520616666151111115443
30. Burz C, Berindan-Neagoe I, Balacescu O, Irimie A. Apoptosis in cancer: key molecular signaling pathways and therapy targets. Acta Oncol. (2009) 48:811–21. doi: 10.1080/02841860902974175
31. Casella S, Leonardi M, Melai B, Fratini F, Pistelli L. The role of diallyl sulfides and dipropyl sulfides in the in vitro antimicrobial activity of the essential oil of garlic, Allium sativum L., and leek, Allium porrum L. Phytother Res. (2013) 27:380–3. doi: 10.1002/ptr.4725
32. Li WR, Shi QS, Liang Q, Huang XM, Chen YB. Antifungal effect and mechanism of garlic oil on Penicillium funiculosum. Appl Microbiol Biotechnol. (2014) 98:8337–46. doi: 10.1007/s00253-014-5919-9
33. Phan ADT, Netzel G, Chhim P, Netzel ME, Sultanbawa Y. Phytochemical characteristics and antimicrobial activity of australian grown garlic (Allium sativum L.) cultivars. Foods. (2019) 8:358. doi: 10.3390/foods8090358
34. Patel DK, Prasad SK, Kumar R, Hemalatha S. An overview on antidiabetic medicinal plants having insulin mimetic property. Asian Pacific J Trop Biomed. (2012) 2:320–30. doi: 10.1016/S2221-1691(12)60032-X
35. Thomson M, Al-Qattan KK, Divya JS, Ali M. Anti-diabetic and anti-oxidant potential of aged garlic extract (AGE) in streptozotocin-induced diabetic rats. BMC Complement Alternat Med. (2015) 16:17. doi: 10.1186/s12906-016-0992-5
36. Faroughi F, Charandabi SMA, Javadzadeh Y, Mirghafourvand M. Effects of garlic pill on blood glucose level in borderline gestational diabetes mellitus: a triple blind, randomized clinical trial. Iran Red Crescent Med J. (2018) 20. doi: 10.5812/ircmj.60675
37. Mehrbod P, Amini E, Tavassoti-Kheiri M. Antiviral activity of garlic extract on influenza virus. Iran J Virol. (2009) 3:19–23. doi: 10.21859/isv.3.1.19
38. Meléndez-Villanueva MA, Morán-Santibañez K, Martínez-Sanmiguel JJ, Rangel-López R, Garza-Navarro MA, Rodríguez-Padilla C, et al. Virucidal activity of gold nanoparticles synthesized by green chemistry using garlic extract. Viruses. (2019) 11:1111. doi: 10.3390/v11121111
39. Harazem R, Rahman S, Kenawy A. Evaluation of antiviral activity of Allium cepa and Allium sativum extracts against newcastle disease virus. AJVS. (2019) 61:108. doi: 10.5455/ajvs.29663
40. Sharma N. Efficacy of garlic and onion against viru. Int J Res Pharmaceutical Sci. (2019) 10:3578–86. doi: 10.26452/ijrps.v10i4.1738
41. Chavan RD, Shinde P, Girkar K, Madage R, Chowdhary A. Assessment of Anti-Influenza activity and hemagglutination inhibition of Plumbago indica and Allium sativum extracts. Pharmacogn Res. (2016) 8:105. doi: 10.4103/0974-8490.172562
42. Mohajer Shojai T, Ghalyanchi Langeroudi A, Karimi V, Barin A, Sadri N. The effect of Allium sativum (Garlic) extract on infectious bronchitis virus in specific pathogen free embryonic egg. Avicenna J Phytomed. (2016) 6:458–267.
43. Silprasit K, Seetaha S, Pongsanarakul P, Hannongbua S, Choowongkomon K. Anti-HIV-1 reverse transcriptase activities of hexane extracts from some Asian medicinal plants. JMPR (2011) 5:4899–906.
44. Wang L, Jiao H, Zhao J, Wang X, Sun S, Lin H. Allicin alleviates reticuloendotheliosis virus-induced immunosuppression via ERK/Mitogen-activated protein kinase pathway in specific pathogen-free chickens. Front Immunol. (2017) 8:1856. doi: 10.3389/fimmu.2017.01856
45. Khubber S, Hashemifesharaki R, Mohammadi M, Gharibzahedi SMT. Garlic (Allium sativum L.): a potential unique therapeutic food rich in organosulfur and flavonoid compounds to fight with COVID-19. Nutr J. (2020) 19:124. doi: 10.1186/s12937-020-00643-8
46. Chen L, Li J, Luo C, Liu H, Xu W, Chen G, et al. Binding interaction of quercetin-3-β-galactoside and its synthetic derivatives with SARS-CoV 3CLpro: structure–activity relationship studies reveal salient pharmacophore features. Bioorg Med Chem. (2006) 14:8295–306. doi: 10.1016/j.bmc.2006.09.014
47. Pandey P, Khan F, Kumar A, Srivastava A, Jha NK. Screening of potent inhibitors against 2019 novel coronavirus (Covid-19) from Allium sativum and Allium cepa: an in silico approach. Biointerface Res Appl Chem. (2021) 11:7981–93. doi: 10.33263/BRIAC111.79817993
48. Hernández-García I, Giménez-Júlvez T. Characteristics of YouTube videos in spanish on how to prevent COVID-19. Int J Environ Res Public Health. (2020) 17:4671. doi: 10.3390/ijerph17134671
49. Njue LG, Ombui JN, Kanja LW, Gathumbi JK, Nduhiu JG. Evaluation of oral toxicity level of ethyl acetate extract, from garlic (Allium sativum) in onorrh dawleys rats as per oecd guidelines 423. J Food Sci Technol. (2015) 2:56–64.
50. Sharma V, Sharma A, Kansal L. The effect of oral administration of Allium sativum extracts on lead nitrate induced toxicity in male mice. Food Chem Toxicol. (2010) 48:928–36. doi: 10.1016/j.fct.2010.01.002
51. Abid-Essefi S, Zaied C, Bouaziz C, Salem IB, Kaderi R, Bacha H. Protective effect of aqueous extract of Allium sativum against zearalenone toxicity mediated by oxidative stress. Exp Toxicol Pathol. (2012) 64:689–95. doi: 10.1016/j.etp.2010.12.012
52. Irkin LC, Yigit M, Yilmaz S, Maita M. Toxicological evaluation of dietary garlic (Allium sativum) powder in european sea bass Dicentrarchuslabrax juveniles. Food Nutr Sci. (2014) 5:1–8.
53. Ashraf R, Khan RA, Ashraf I. Garlic (Allium sativum) supplementation with standard antidiabetic agent provides better diabetic control in type 2 diabetes patients. Pakistan J Pharmaceutical Sci. (2011) 24:565–70.
54. Sukandar EY, Permana H, Adnyana IK, Sigit JI, Ilyas RA, Hasimun P, et al. Clinical study of turmeric (Curcuma longa L.) and garlic (Allium sativum L.) extracts as antihyperglycemic and antihyperlipidemic agent in type-2 diabetes-dyslipidemia patients. Int J Pharmacol. (2010) 6:456–63. doi: 10.3923/ijp.2010.456.463
55. Moosavian SP, Paknahad Z, Habibagahi Z, Maracy M. The effects of garlic (Allium sativum) supplementation on inflammatory biomarkers, fatigue, and clinical symptoms in patients with active rheumatoid arthritis: a randomized, double-blind, placebo-controlled trial. Phytother Res. (2020) 34:2953–62. doi: 10.1002/ptr.6723
56. Bhandari PR. Garlic (Allium sativum L.): a review of potential therapeutic applications. Int J Green Pharm. (2012) 6:118. doi: 10.4103/0973-8258.102826
57. Drugs.com. Garlic. (2018). Available online at: https://www.drugs.com/npp/garlic.html (accessed October 31, 2018).
58. Bakht J, Muhammad T, Ali H, Islam A, Shafi M. Effect of different solvent extracted sample of Allium sativum (Linn) on bacteria and fungi. Afr J Biotechnol. (2011) 10:5910–5. doi: 10.5897/AJB11.232
59. Charron CS, Milner JA, Novotny JA. Garlic. (2016). p. 184–90. doi: 10.1016/B978-0-12-384947-2.00346-9
60. Goldy R. October is Garlic Planting Time, Michigan State University Extension, 2011. East Lansin, MI: Michigan State University (2019).
61. Hosseini A, Hosseinzadeh H. A review on the effects of Allium sativum (Garlic) in metabolic syndrome. J Endocrinol Investigat. (2015) 38:1147–57. doi: 10.1007/s40618-015-0313-8
62. Karuppiah P, Rajaram S. Antibacterial effect of Allium sativum cloves and Zingiber officinale rhizomes against multiple-drug resistant clinical pathogens. Asian Pacific J Trop Biomed. (2012) 2:597–601. doi: 10.1016/S2221-1691(12)60104-X
63. Rahman AHMM, Kabir EZMF, Sima SN, Sultana RS, Nasiruddin M, Naderuzzaman ATM. Study of an ethnobotany at the village Dohanagar, Naogaon. J Appl Sci Res. (2010) 6:1466–73.
64. Rahman MM, Fazlic V, Saad NW. Antioxidant properties of raw garlic (Allium sativum) extract. Int Food Res J. (2012) 19:589–91.
65. Martins N, Petropoulos S, Ferreira IC. Chemical composition and bioactive compounds of garlic (Allium sativum L.) as affected by pre-and post-harvest conditions: a review. Food Chem. (2016) 211:41–50. doi: 10.1016/j.foodchem.2016.05.029
66. Kallel F, Driss D, Chaari F, Belghith L, Bouaziz F, Ghorbel R, et al. Garlic (Allium sativum L.) husk waste as a potential source of phenolic compounds: influence of extracting solvents on its antimicrobial and antioxidant properties. Industrial Crops Prod. (2014) 62:34–41. doi: 10.1016/j.indcrop.2014.07.047
67. Suleria HAR, Butt MS, Khalid N, Sultan S, Raza A, Aleem M, et al. Garlic (Allium sativum): diet based therapy of 21st century–a review. Asian Pacific J Trop Dis. (2015) 5:271–8. doi: 10.1016/S2222-1808(14)60782-9
68. Chekki RZ, Snoussi A, Hamrouni I, Bouzouita N. Chemical composition, antibacterial and antioxidant activities of Tunisian garlic (Allium sativum) essential oil and ethanol extract. Mediterranean J Chem. (2014) 3:947–56. doi: 10.13171/mjc.3.4.2014.09.07.11
69. Capasso A. Antioxidant action and therapeutic efficacy of Allium sativum L. Molecules. (2013) 18:690–700. doi: 10.3390/molecules18010690
70. Zhao NN, Zhang H, Zhang XC, Luan XB, Zhou C, Liu QZ, et al. Evaluation of acute toxicity of essential oil of garlic (Allium sativum) and its selected major constituent compounds against overwintering Cacopsylla chinensis (Hemiptera: psyllidae). J Econ Entomol. (2013) 106:1349–54. doi: 10.1603/EC12191
71. Dziri S, Casabianca H, Hanchi B, Hosni K. Composition of garlic essential oil (Allium sativum L.) as influenced by drying method. J Essential Oil Res. (2014) 26:91–6. doi: 10.1080/10412905.2013.868329
72. Mikaili P, Maadirad S, Moloudizargari M, Aghajanshakeri S, Sarahroodi S. Therapeutic uses and pharmacological properties of garlic, shallot, and their biologically active compounds. Iran J Basic Med Sci. (2013) 16:1031.
73. Quintero-Fabián S, Ortuño-Sahagún D, Vázquez-Carrera M, López-Roa RI. Alliin, a garlic (Allium sativum) compound, prevents LPS-induced inflammation in 3T3-L1 adipocytes. Mediators Inflamm. (2013) 2013:1–11. doi: 10.1155/2013/381815
74. Devi A, Rakshit K, Sarania B. Ethnobotanical notes on Allium species of Arunachal Pradesh, India. Ind J Trad Knowledge. (2014) 13: 606–12.
75. Jariæ S, Maèukanoviæ-Jociæ M, Djurdjeviæ L, Mitroviæ M, Kostiæ O, Karadžiæ B, et al. An ethnobotanical survey of traditionally used plants on Suva planina mountain (south-eastern Serbia). J. Ethnopharmacol. (2015) 175:93–108. doi: 10.1016/j.jep.2015.09.002
76. Gbolade A. Ethnobotanical study of plants used in treating hypertension in Edo State of Nigeria. J Ethnopharmacol. (2012) 144:1–10. doi: 10.1016/j.jep.2012.07.018
77. Ugulu I. Traditional ethnobotanical knowledge about medicinal plants used for external therapies in Alasehir, Turkey. Int J Med Aromatic Plants. (2011) 1:101–6.
78. Soladoye MO, Adetayo MO, Chukwuma EC, Adetunji AN. Ethnobotanical survey of plants used in the treatment of haemorrhoids in South-Western Nigeria. Ann Biol Res. (2010) 1:1–15.
79. Ogbole OO, Gbolade AA, Ajaiyeoba EO. Ethnobotanical survey of plants used in treatment of inflammatory diseases in Ogun State of Nigeria. Eur J Sci Res. (2010) 43:183–91.
80. Soladoye MO, Amusa NA, Raji-Esan SO, Chukwuma EC, Taiwo AA. Ethnobotanical survey of anti-cancer plants in Ogun State, Nigeria. Ann Biol Res. (2010) 1:261–73.
81. Keter LK, Mutiso PC. Ethnobotanical studies of medicinal plants used by Traditional Health Practitioners in the management of diabetes in Lower Eastern Province, Kenya. J Ethnopharmacol. (2012) 139:74–80. doi: 10.1016/j.jep.2011.10.014
82. Barkaoui M, Katiri A, Boubaker H, Msanda F. Ethnobotanical survey of medicinal plants used in the traditional treatment of diabetes in Chtouka Ait Baha and Tiznit (Western Anti-Atlas), Morocco. J Ethnopharmacol. (2017) 198:338–50. doi: 10.1016/j.jep.2017.01.023
83. Phondani PC, Maikhuri RK, Rawat LS, Farooquee NA, Kala CP, Vishvakarma SR, et al. Ethnobotanical uses of plants among the Bhotiya tribal communities of Niti Valley in Central Himalaya, India. Ethnobot Res Appl. (2010) 8:233–44. doi: 10.17348/era.8.0.233-244
84. Teklay A, Abera B, Giday M. An ethnobotanical study of medicinal plants used in Kilte Awulaelo District, Tigray Region of Ethiopia. J Ethnobiol Ethnomed. (2013) 9:65. doi: 10.1186/1746-4269-9-65
85. Lagnika L, Adjileye RAA, Yedomonhan H, Amadou BSK, Sanni A. Ethnobotanical survey on antihypertensive medicinal plants in municipality of Ouémé, Southern Benin. Adv Herb Med. (2016) 2:20–32.
86. Karou SD, Tchacondo T, Djikpo Tchibozo MA, Abdoul-Rahaman S, Anani K, Koudouvo K, et al. Ethnobotanical study of medicinal plants used in the management of diabetes mellitus and hypertension in the Central Region of Togo. Pharmaceutical Biol. (2011) 49:1286–97. doi: 10.3109/13880209.2011.621959
87. Sher Z, Khan Z, Hussain F. Ethnobotanical studies of some plants of Chagharzai valley, district Buner, Pakistan. Pakistan J Bot. (2011) 43:1445–52.
88. Shende JJ, Rajurkar BM, Mhaiskar MN, Dalal LP. Ethnobotanical studies of Samudrapur Tahsil of Wardha district. Int Organ Sci Res. (2014) 9:16–23.
89. Getaneh S, Girma Z. An ethnobotanical study of medicinal plants in Debre Libanos Wereda, Central Ethiopia. Afr J Plant Sci. (2014) 8:366–79. doi: 10.5897/AJPS2013.1041
90. Kayani S, Ahmad M, Zafar M, Sultana S, Khan MPZ, Ashraf MA, et al. Ethnobotanical uses of medicinal plants for respiratory disorders among the inhabitants of Gallies–Abbottabad, Northern Pakistan. J Ethnopharmacol. (2014) 156:47–60. doi: 10.1016/j.jep.2014.08.005
91. Calvo MI, Akerreta S, Cavero RY. Pharmaceutical ethnobotany in the riverside of Navarra (Iberian Peninsula). J Ethnopharmacol. (2011) 135:22–33. doi: 10.1016/j.jep.2011.02.016
92. Mesfin F, Seta T, Assefa A. An ethnobotanical study of medicinal plants in Amaro Woreda, Ethiopia. Ethnobotany Res Appl. (2014) 12:341–54. doi: 10.17348/era.12.0.341-354
93. Soladoye MO, Chukwuma EC, Sulaiman OM, Feyisola RT. Ethnobotanical survey of plants used in the traditional treatment of female infertility in Southwestern Nigeria. Ethnobotany Res Appl. (2014) 12:81–90.
94. Bekele G, Reddy PR. Ethnobotanical study of medicinal plants used to treat human ailments by Guji Oromo tribes in Abaya District, Borana, Oromia, Ethiopia. Univ J Plant Sci. (2015) 3:4. doi: 10.13189/ujps.2015.030101
95. Sen S, Chakraborty R, De B, Devanna N. An ethnobotanical survey of medicinal plants used by ethnic people in West and South district of Tripura, India. J Forestry Res. (2011) 22:417. doi: 10.1007/s11676-011-0184-6
96. Birhanu T, Abera D, Ejeta E, Nekemte E. Ethnobotanical study of medicinal plants in selected Horro Gudurru Woredas, Western Ethiopia. J Biol Agric Healthcare. (2015) 5:83–93.
97. Šavikin K, Zduniæ G, Menkoviæ N, Živkoviæ J, Æujiæ N, Terešèenko M, et al. Ethnobotanical study on traditional use of medicinal plants in South-Western Serbia, Zlatibor district. J Ethnopharmacol. (2013) 146:803–10. doi: 10.1016/j.jep.2013.02.006
98. Thapa S. Medico-ethnobotany of Magar community in Salija VDC of Parbat district, central Nepal. Our Nat. (2012) 10:176–90. doi: 10.3126/on.v10i1.7780
99. Šariæ-Kundaliæ B, Dobeš C, Klatte-Asselmeyer V, Saukel J. Ethnobotanical study on medicinal use of wild and cultivated plants in middle, south and west Bosnia and Herzegovina. J Ethnopharmacol. (2010) 131:33–55. doi: 10.1016/j.jep.2010.05.061
100. Megersa M, Asfaw Z, Kelbessa E, Beyene A, Woldeab B. An ethnobotanical study of medicinal plants in Wayu Tuka district, east Welega zone of oromia regional state, West Ethiopia. J Ethnobiol Ethnomed. (2013) 9:68. doi: 10.1186/1746-4269-9-68
101. Olanipekun MK, Arowosegbe S, Kayode JO, Oluwole TR. Ethnobotanical survey of medicinal plants used in the treatment of women related diseases in Akoko Region of Ondo-State, Nigeria. J Med Plants Res. (2016) 10:270–7.
102. González JA, García-Barriuso M, Amich F. Ethnobotanical study of medicinal plants traditionally used in the Arribes del Duero, western Spain. J Ethnopharmacol. (2010) 131:343–55. doi: 10.1016/j.jep.2010.07.022
103. Asase A, Yohonu DT. Ethnobotanical study of herbal medicines for management of diabetes mellitus in Dangme West District of southern Ghana. J Herb Med. (2016) 6:204–9. doi: 10.1016/j.hermed.2016.07.002
104. Polat R, Satıl F. An ethnobotanical survey of medicinal plants in Edremit Gulf (Balıkesir–Turkey). J Ethnopharmacol. (2012) 139:626–41. doi: 10.1016/j.jep.2011.12.004
105. Mustafa B, Hajdari A, Pajazita Q, Syla B, Quave CL, Pieroni A. An ethnobotanical survey of the Gollak region, Kosovo. Genet Resour Crop Evol. (2012) 59:739–54. doi: 10.1007/s10722-011-9715-4
106. Regassa R, Bekele T, Megersa M. Ethnobotanical study of traditional medicinal plants used to treat human ailments by Halaba people, southern Ethiopia. J Med Plants Stud. (2017) 5:36–47.
107. Fred-Jaiyesimi A, Ajibesin KK, Tolulope O, Gbemisola O. Ethnobotanical studies of folklore phytocosmetics of South West Nigeria. Pharmaceutical Biol. (2015) 53:313–8. doi: 10.3109/13880209.2014.918155
108. Agize M, Demissew S, Asfaw Z. Ethnobotany of medicinal plants in Loma and Gena bosa districts (woredas) of dawro zone, southern Ethiopia. Topclass J Herb Med. (2013) 2:194–212.
109. Mustafa B, Hajdari A, Krasniqi F, Hoxha E, Ademi H, Quave CL, et al. Medical ethnobotany of the Albanian Alps in Kosovo. J Ethnobiol Ethnomed. (2012) 8:6. doi: 10.1186/1746-4269-8-6
110. Karunamoorthi K, Hailu T. Insect repellent plants traditional usage practices in the Ethiopian malaria epidemic-prone setting: an ethnobotanical survey. J Ethnobiol Ethnomed. (2014) 10:22. doi: 10.1186/1746-4269-10-22
111. Alemayehu G, Asfaw Z, Kelbessa E. Ethnobotanical study of medicinal plants used by local communities of Minjar-Shenkora District, North Shewa Zone of Amhara Region, Ethiopia. J Med Plants Stud. (2015) 3:1–11.
112. Olorunnisola OS, Adetutu A, Balogun EA, Afolayan AJ. Ethnobotanical survey of medicinal plants used in the treatment of malarial in Ogbomoso, Southwest Nigeria. J Ethnopharmacol. (2013) 150:71–8. doi: 10.1016/j.jep.2013.07.038
113. Sarkhel S. Ethnobotanical survey of folklore plants used in treatment of snakebite in Paschim Medinipur district, West Bengal. Asian Pacific J Trop Biomed. (2014) 4:416–20. doi: 10.12980/APJTB.4.2014C1120
114. Cavero RY, Akerreta S, Calvo MI. Pharmaceutical ethnobotany in the Middle Navarra (Iberian Peninsula). J Ethnopharmacol. (2011) 137:844–55. doi: 10.1016/j.jep.2011.07.001
115. Abouri M, El Mousadik A, Msanda F, Boubaker H, Saadi B, Cherifi K. An ethnobotanical survey of medicinal plants used in the Tata Province, Morocco. Int J Med Plants Res. (2012) 1:99–123.
116. Kichu M, Malewska T, Akter K, Imchen I, Harrington D, Kohen J, et al. An ethnobotanical study of medicinal plants of Chungtia village, Nagaland, India. J Ethnopharmacol. (2015) 166:5–17. doi: 10.1016/j.jep.2015.02.053
117. Pieroni A, Giusti ME, Quave CL. Cross-cultural ethnobiology in the Western Balkans: medical ethnobotany and ethnozoology among Albanians and Serbs in the Pešter Plateau, Sandžak, South-Western Serbia. Hum Ecol. (2011) 39:333. doi: 10.1007/s10745-011-9401-3
118. Rigat M, Vallès J, Gras A, Iglésias J, Garnatje T. Plants with topical uses in the Ripollès district (Pyrenees, Catalonia, Iberian Peninsula): ethnobotanical survey and pharmacological validation in the literature. J Ethnopharmacol. (2015) 164:162–79. doi: 10.1016/j.jep.2015.01.055
119. Ohemu TL, Agunu A, Olotu PN, Ajima U, Dafam DG, Azila JJ. Ethnobotanical survey of medical plants used in the traditional treatment of viral infections in Jos, plateau state, Nigeria. Int J Med Aromatic Plants. (2014) 4:74–81.
120. Maryo M, Nemomissa S, Bekele T. An ethnobotanical study of medicinal plants of the Kembatta ethnic group in Enset-based agricultural landscape of Kembatta Tembaro (KT) Zone, Southern Ethiopia. Asian J Plant Sci Res. (2015) 5:42–61.
121. Purbashree S, Rajamohan MR, Kumar PA. Ethnobotany of Chothe tribe of Bishnupur district (Manipur). Ind J Nat Prod Resour. (2012) 3:414–25.
122. Abbasi AM, Khan MA, Ahmed M, Zafar M. Herbal medicines used to cure various ailments by the inhabitants of Abbottabad district, North West Frontier Province, Pakistan. Ind J Trad Knowledge. (2010) 9:175–83.
123. Nath M, Choudhury MD. Ethno-medico-botanical aspects of Hmar tribe of Cachar district, Assam (Part I). Ind J Trad Knowledge. (2010) 9:760–4.
124. Singh VN, Chanu LI, Baruah MK. An ethnobotanical study of Chirus-A less known tribe of Assam. Ind J Trad Knowledge. (2011) 10:572–4.
125. Gaur RD, Sharma J, Painuli RM. Plants used in traditional healthcare of livestock by Gujjar community of Sub-Himalayan tracts, Uttarakhand, India. Ind J Nat Prod Resour. (2010) 1:243–8.
126. Saha MR, Sarker DD, Sen A. Ethnoveterinary practices among the tribal community of Malda district of West Bengal, India. Ind J Trad Knowledge. (2014) 13:359–67.
127. Shaheen H, Nazir J, Firdous SS, Khalid AUR. Cosmetic ethnobotany practiced by tribal women of Kashmir Himalayas. Avicenna J Phytomed. (2014) 4:239.
128. Murthy SM, Vidyasagar GM. Traditional knowledge on medicinal plants used in the treatment of respiratory disorders in Bellary district, Karnataka, India. Ind J Natural Prod Resour. (2013) 4:189–93. doi: 10.5958/j.0975-4261.4.4.029
129. Namsa ND, Mandal M, Tangjang S, Mandal SC. Ethnobotany of the Monpa ethnic group at Arunachal Pradesh, India. J Ethnobiol Ethnomed. (2011) 7:31. doi: 10.1186/1746-4269-7-31
130. Thirumalai T, Beverly CD, Sathiyaraj K, Senthilkumar B, David E. Ethnobotanical Study of Anti-diabetic medicinal plants used by the local people in Javadhu hills Tamilnadu, India. Asian Pacific J Trop Biomed. (2012) 2:910–3. doi: 10.1016/S2221-1691(12)60335-9
131. Sargın SA, Akçicek E, Selvi S. An ethnobotanical study of medicinal plants used by the local people of Alaşehir (Manisa) in Turkey. J Ethnopharmacol. (2013) 150:860–74. doi: 10.1016/j.jep.2013.09.040
132. Abe R, Ohtani K. An ethnobotanical study of medicinal plants and traditional therapies on Batan Island, the Philippines. J Ethnopharmacol. (2013) 145:554–65. doi: 10.1016/j.jep.2012.11.029
133. Sonibare NA, Abegunde RB. Ethnobotanical study of medicinal plants used by the Laniba village people in South Western Nigeria. Afr J Pharm Pharmacol. (2012) 6:1726–32. doi: 10.5897/AJPP11.680
134. Gupta R, Vairale MG, Deshmukh RR, Chaudhary PR, Wate SR. Ethnomedicinal uses of some plants used by Gond tribe of Bhandara district, Maharashtra. Ind J Trad Knowledge. (2010) 9:713–7.
135. Jain SP, Srivastava S, Singh J, Singh SC. Traditional phytotherapy of Balaghat district, Madhya Pradesh, India. Ind J Trad Knowledge. (2011) 10:334–8.
136. Baharvand-Ahmadi B, Asadi-Samani M. A mini-review on the most important effective medicinal plants to treat hypertension in ethnobotanical evidence of Iran. J Nephropharmacol. (2017) 6:3. doi: 10.15171/jnp.2017.20
137. Kefalew A, Asfaw Z, Kelbessa E. Ethnobotany of medicinal plants in Ada’a District, East Shewa Zone of Oromia regional state, Ethiopia. J Ethnobiol Ethnomed. (2015) 11:25. doi: 10.1186/s13002-015-0014-6
138. Otang WM, Grierson DS, Ndip RN. Ethnobotanical survey of medicinal plants used in the management of opportunistic fungal infections in HIV/AIDS patients in the Amathole District of the Eastern Cape Province, South Africa. J Med Plants Res. (2012) 6:2071–80. doi: 10.5897/JMPR11.069
139. Zenebe G, Zerihun M, Solomon Z. An ethnobotanical study of medicinal plants in Asgede Tsimbila district, Northwestern Tigray, northern Ethiopia. Ethnobot Res Appl. (2012) 10:305–20. doi: 10.17348/era.10.0.305-320
140. Mekonnen N, Abebe E. Ethnobotanical knowledge and practices of traditional healers in Harar, Haramaya, Bati and Garamuleta, Eastern Ethiopia. Ethiopian Veter J. (2017) 21:40–61. doi: 10.4314/evj.v21i2.4
141. Nigam G, Sharma NK. Ethnoveterinary plants of Jhansi district, Uttar Pradesh. Ind J Trad Knowledge. (2010) 9:664–7.
142. Akgül G, Yılmaz N, Celep A, Celep F, Çakılcıoğlu U. Ethnobotanical purposes of plants sold by herbalists and folk bazaars in the center of Cappadocica (Nevşehir, Turkey). Ind J Trad Knowledge. (2016) 15:103–8.
143. Liu J, Guo W, Yang M, Liu L, Huang S, Tao L, et al. Investigation of the dynamic changes in the chemical constituents of Chinese “Laba” garlic during traditional processing. RSC Adv. (2018) 8:41872–83. doi: 10.1039/C8RA09657K
144. Kang JS, Kim SO, Kim GY, Hwang HJ, Kim BW, Chang YC, et al. An exploration of the antioxidant effects of garlic saponins in mouse-derived C2C12 myoblasts. Int J Mol Med. (2016) 37:149–56. doi: 10.3892/ijmm.2015.2398
145. Choi JH, Kim MS, Yu HJ, Kim KH, Lee HS, Cho HY, et al. Hepatoprotective effects of lactic acid-fermented garlic extracts on fatty liver-induced mouse by alcohol. J Korean Soc Food Sci Nutr. (2014) 43:1642–7. doi: 10.3746/jkfn.2014.43.11.1642
146. Park BM, Cha SA, Kim HY, Kang DK, Yuan K, Chun H, et al. Fermented garlic extract decreases blood pressure through nitrite and sGC-cGMP-PKG pathway in spontaneously hypertensive rats. J Funct Foods. (2016) 22:156–65. doi: 10.1016/j.jff.2016.01.034
147. Ha AW, Ying T, Kim WK. The effects of black garlic (Allium satvium) extracts on lipid metabolism in rats fed a high fat diet. Nutr Res Pract. (2015) 9:30–6. doi: 10.4162/nrp.2015.9.1.30
148. Khatua TN, Borkar RM, Mohammed SA, Dinda AK, Srinivas R, Banerjee SK. Novel sulfur metabolites of garlic attenuate cardiac hypertrophy and remodeling through induction of Na+/K+-ATPase expression. Front Pharmacol. (2017) 8:18. doi: 10.3389/fphar.2017.00018
149. Sultana MR, Bagul PK, Katare PB, Mohammed SA, Padiya R, Banerjee SK. Garlic activates SIRT-3 to prevent cardiac oxidative stress and mitochondrial dysfunction in diabetes. Life Sci. (2016) 164:42–51. doi: 10.1016/j.lfs.2016.08.030
150. Supakul L, Pintana H, Apaijai N, Chattipakorn S, Shinlapawittayatorn K, Chattipakorn N. Protective effects of garlic extract on cardiac function, heart rate variability, and cardiac mitochondria in obese insulin-resistant rats. Eur J Nutr. (2014) 53:919–28. doi: 10.1007/s00394-013-0595-6
151. Gomaa AM, Abdelhafez AT, Aamer HA. Garlic (Allium sativum) exhibits a cardioprotective effect in experimental chronic renal failure rat model by reducing oxidative stress and controlling cardiac Na+/K+-ATPase activity and Ca 2+ levels. Cell Stress Chaperones. (2018) 23:913–20. doi: 10.1007/s12192-018-0898-x
152. Kaschula CH, Hunter R, Cotton J, Tuveri R, Ngarande E, Dzobo K, et al. The garlic compound ajoene targets protein folding in the endoplasmic reticulum of cancer cells. Mol Carcinogenesis. (2016) 55:1213–28. doi: 10.1002/mc.22364
153. Zhang Y, Li HY, Zhang ZH, Bian HL, Lin G. Garlic-derived compound S-allylmercaptocysteine inhibits cell growth and induces apoptosis via the JNK and p38 pathways in human colorectal carcinoma cells. Oncol Lett. (2014) 8:2591–6. doi: 10.3892/ol.2014.2579
154. Mansingh DP, Dalpati N, Sali VK, Vasanthi AHR. Alliin the precursor of allicin in garlic extract mitigates proliferation of gastric adenocarcinoma cells by modulating apoptosis. Pharmacogn Magazine. (2018) 14:84. doi: 10.4103/pm.pm_342_17
155. Xiao J, Xing F, Liu Y, Lv Y, Wang X, Ling MT, et al. Garlic-derived compound S-allylmercaptocysteine inhibits hepatocarcinogenesis through targeting LRP6/Wnt pathway. Acta Pharmaceutica Sin B. (2018) 8:575–86. doi: 10.1016/j.apsb.2017.10.003
156. Talib WH. Consumption of garlic and lemon aqueous extracts combination reduces tumor burden by angiogenesis inhibition, apoptosis induction, and immune system modulation. Nutrition. (2017) 43:89–97. doi: 10.1016/j.nut.2017.06.015
157. Fratianni F, Riccardi R, Spigno P, Ombra MN, Cozzolino A, Tremonte P, et al. Biochemical characterization and antimicrobial and antifungal activity of two endemic varieties of garlic (Allium sativum L.) of the campania region, southern Italy. J Med Food. (2016) 19:686–91. doi: 10.1089/jmf.2016.0027
158. Wallock-Richards D, Doherty CJ, Doherty L, Clarke DJ, Place M, Govan JR, et al. Garlic revisited: antimicrobial activity of allicin-containing garlic extracts against Burkholderia cepacia complex. PLoS One. (2014) 9:e112726. doi: 10.1371/journal.pone.0112726
159. Pundir RK, Jain P, Sharma C. Antimicrobial activity of ethanolic extracts of Syzygium aromaticum and Allium sativum against food associated bacteria and fungi. Ethnobotanical Leaflets. (2010) 2010:11.
Keywords: allium sativum, traditional uses, ethnobotany, phytochemistry, pharmacology, toxicology
Citation: Tudu CK, Dutta T, Ghorai M, Biswas P, Samanta D, Oleksak P, Jha NK, Kumar M, Radha, Proćków J, Pérez de la Lastra JM and Dey A (2022) Traditional uses, phytochemistry, pharmacology and toxicology of garlic (Allium sativum), a storehouse of diverse phytochemicals: A review of research from the last decade focusing on health and nutritional implications. Front. Nutr. 9:949554. doi: 10.3389/fnut.2022.929554
Received: 27 April 2022; Accepted: 05 September 2022;
Published: 28 October 2022.
Edited by:
Gengjun Chen, Kansas State University, United StatesReviewed by:
Martin C. H. Gruhlke, RWTH Aachen University, GermanyChunpeng (Craig) Wan, Jiangxi Agricultural University, China
Copyright © 2022 Tudu, Dutta, Ghorai, Biswas, Samanta, Oleksak, Jha, Kumar, Radha, Proćków, Pérez de la Lastra and Dey. This is an open-access article distributed under the terms of the Creative Commons Attribution License (CC BY). The use, distribution or reproduction in other forums is permitted, provided the original author(s) and the copyright owner(s) are credited and that the original publication in this journal is cited, in accordance with accepted academic practice. No use, distribution or reproduction is permitted which does not comply with these terms.
*Correspondence: Jarosław Proćków, jaroslaw.prockow@upwr.edu.pl; José M. Pérez de la Lastra, jm.perezdelalastra@csic.es; Abhijit Dey, abhijit.dbs@presiuniv.ac.in
†Present Address: Tusheema Dutta, School of Biology, Indian Institute of Science Education and Research, Thiruvananthapuram, India