- 1Research Department, Sidra Medicine, Doha, Qatar
- 2Reproductive Medicine Unit, Sidra Medicine, Doha, Qatar
Female infertility is a major public health concern and a global challenge. It is a disorder of the reproductive system, defined as the inability to achieve a clinical pregnancy. Nutrition and other environmental factors are found to impact reproductive health in women as well as the outcome of assisted reproductive technologies (ART). Dietary factors, such as polyunsaturated fatty acids (PUFA), fiber as well as the intake of Mediterranean diet appear to exert beneficial effects on female reproductive outcomes. The exact mechanisms associating diet to female fertility are yet to be identified, although genomic, epigenomic, and microbial pathways may be implicated. This review aims to summarize the current knowledge on the impact of dietary components on female reproduction and ART outcomes, and to discuss the relevant interplay of diet with genome, epigenome and microbial composition.
Introduction
According to the WHO, infertility is a global health issue affecting around 48 million couples around the world (1). Infertility is a disorder of the reproductive system defined as the inability to develop clinical pregnancy after 1 year of unprotected sexual intercourse (1). It is estimated that up to 15% of reproductive-aged couples worldwide are affected (1). Despite recent scientific advances and increased access and use of Assisted Reproductive Technologies (ART) globally, the overall burden of infertility has not shown any decline over the last two decades. Indeed, although in vitro fertilization (IVF) has revolutionized the landscape of infertility treatment, it remains far from being a panacea and success rates over the recent years have plateaued (2). The increasing prevalence of female impaired fecundity along with the high financial costs of fertility treatments and their relatively low success rates, make the identification of potentially modifiable factors and non-pharmacological treatments that can positively influence fertility-related outcomes, a pressing need. As different nutritional factors are found to affect female reproductive health and the potential success of ART, great interest is growing in understanding the relationship between them and the relevant research (3–7). The study of human fertility and fecundity is nonetheless very challenging. Therefore, most researchers focus on surrogate measures, such as time to intended pregnancy, pregnancy loss, or reproductive outcomes in women undergoing ART. Other commonly used fecundity parameters include reproductive hormonal profiles and markers of ovarian reserve such as antral follicle count and anti-Müllerian hormone (AMH). Admittedly, there is an inherent difficulty in studying the potential effect of diet and nutrition in infertility, which is anyway unique to any other pathology or condition as it is affected by the status of two rather than one individual- due to the multifactorial and extremely complex nature of reproduction (8). To complicate matters further, there is significant evidence that environmental factors may lead to direct toxicity, hormonal disruption and reactive oxygen species (ROS) production, demonstrating a causal association with female reproductive disorders (9). In today's world, food is not only a means to obtain nutrients, but invariably contains non-nutritive chemical substances which may occur either as environmental contaminants or are synthetic byproducts of food processing and packaging (7). Taking into consideration all the above constraints, we aim to present and appraise the available literature as a narrative review on the effects of diet on female reproduction. In addition, we will review the current knowledge on the impact of nutritional factors on the genome, epigenome and microbial composition, and explore associations with female reproductive health. The topic of diet and male infertility will not be addressed in this review, although the influence of nutrition and environmental factors on male fertility appears to be equally significant. We quote separately the available evidence and studies focusing either generically on women's reproductive health and various reproductive markers or on those women undergoing treatment with ART where the outcomes are more reproducible as we believe that these two categories have distinct characteristics that can draw interesting conclusions and comparisons. Finally, we assembled 3 tables summarizing the available evidence (Tables 1–3). Due to the variability of studies and outcomes and the small number of interventional studies, providing specific recommendations is particularly challenging. However, dietary patterns that improve reproductive outcomes do emerge and the interplay with the gene function could offer a valuable and more precise guide to women and health professionals alike.
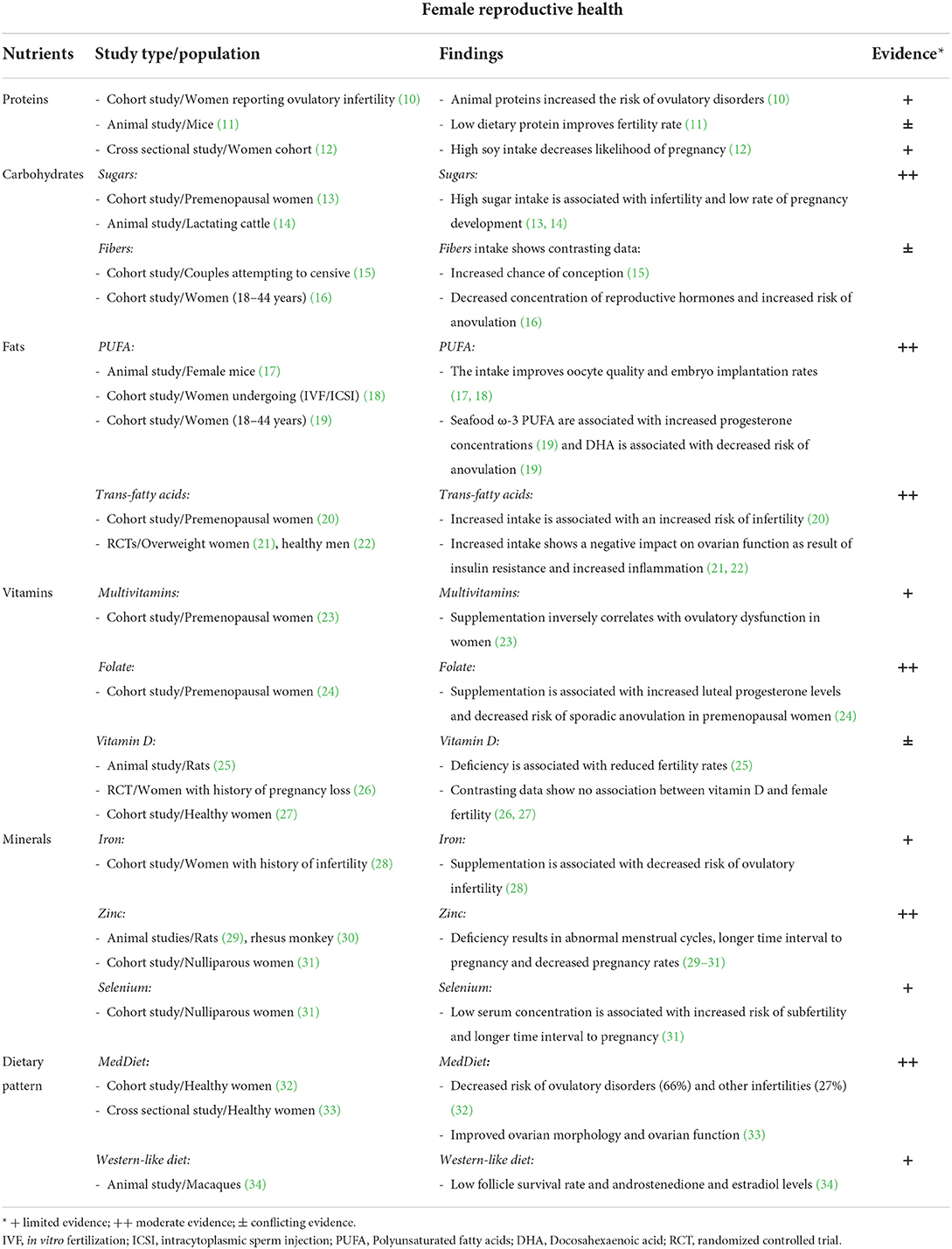
Table 1. The effect of several nutritional factors and dietary patterns on female reproductive health.
Influence of diet on reproductive health in women
Proteins
The Nurses' Health Study II (NHS II) suggested that healthy women who consumed animal-based proteins were at increased risk of ovulatory disorders compared to those who received plant-based proteins (10), which indicates a putative effect of the source of dietary protein on female reproductive health. Diets enriched with proteins derived from plant sources could influence favorably insulin sensitivity and Insulin-like Growth factor (IGF-I) levels as observed in other conditions (88–90). Moreover, studies conducted on ruminants showed that a high intake of protein affects ovarian activity through non-Luteinizing hormone (LH) mediated pathways (91), including the ovarian IGF system, which enhances intrafollicular IGF leading to increased follicle-stimulating hormone (FSH) sensitivity (92). Low dietary protein intake in mice resulted in improved fertility rates and controlled the ovarian primordial follicle reserve via Fibroblast Growth Factor 21 (FGF21) pathway (11).
Recently, protein intake and its effect on female reproductive health have received great attention due to its possible association with increased levels of environmental contaminants. Red meat and seafood, which are considered important protein sources, may have elevated levels of different contaminants, such as antibiotics, dioxins, mercury, and organochlorines (4, 93, 94).
Soy is another source of protein which has been under scrutiny due to its potential interference with the mechanisms of reproduction. It is enriched with phytoestrogens, which are potential reproductive disruptors as previously described in animal models (95, 96). There are few human studies evaluating the effects of soy on female reproductive health. However, a cross-sectional study found that women with a high intake of soy isoflavones were 13% less likely to achieve a pregnancy compared to women with a standard intake (12).
Conversely, the reduced intake of animal proteins, such as in the vegan diet, can cause the deficiency of important micronutrients, such as vitamin B12, zinc, calcium, and selenium (97). Particularly, the deficiency in vitamin B12 was observed in a cross-sectional study on Australian women in child-bearing age (18–44 years) that can negatively affect women reproductive health (98).
Overall, the exact influence of protein intake on female reproductive health is yet to be determined due to inherent difficulties in defining the impact on ovulatory function and fertility-related outcomes as well as the great variability of different protein sources (Table 1).
Carbohydrates
There are limited studies evaluating the effect of carbohydrate intake on ovulatory function and fertility rate in healthy women. Although a diet low in carbohydrates has been shown to be associated with low insulin levels and improved pregnancy outcomes in women with polycystic ovarian syndrome (PCOS), this relationship has not been fully explored in healthy women (99).
The glycemic index and load are important indicators of the effect of a carbohydrate diet on blood glucose levels (100). Chavarro et al. found that higher dietary carbohydrates and glycemic load were associated with an increased incidence of infertility in healthy women (13). One may postulate that increased insulin levels resulting in elevated IGF-I and androgen levels (101) could create endocrine conditions similar to those associated with PCOS (102). Alternatively, high carbohydrate intake may occur at the expense of natural fats, which are prerequisite for the maintenance of ovulatory function (13, 103).
Simple sugars have also been associated with adverse effects on the reproductive performance of women, leading to a lower fertility potential. A diet rich in sugars was also associated with a lower likelihood of pregnancy in dairy cows (14). The biological mechanisms underlying these observed associations are yet to be identified. When female rats were fed a diet rich in sugar (sucrose 16%) followed by 1-day starvation, Sadowska et al. observed an increased glutathione peroxidase activity and malondialdehyde concentration and decreased activity of catalase in the uterus as well as decreased glutathione peroxidase and catalase activity and malondialdehyde concentration in the ovaries. The authors suggested changes in the oxidative balance as a plausible cause for fertility problems (104).
On another note, increased intake of non-digested carbohydrates, such as soluble fibers, was positively associated with the likelihood of conception. Unlike simple carbohydrates, the beneficial influence of a high fiber diet on female reproduction could be mediated by its effect on lowering blood glucose and thus resulting in a low dietary glycemic load and index (15). However, Gaskins et al. found that a high dietary fiber intake was associated with a reduction in FSH, LH, progesterone and estradiol levels, in addition to an increase in anovulatory cycles (16). A lower fermentation rate of fibers is known to promote gut microbial growth with health benefits for the host (105) and it can exert benefic effects on female reproductive health and ART outcomes, as discussed below. A summary of the evidence about carbohydrates intake is provided in Table 1.
Fats
Different studies showed that fatty acids are needed in early reproductive events, such as oocyte maturation and embryo implantation (106, 107). It has been reported that diets rich in fatty acids may affect fertility and pregnancy rates positively by enhancing the production of steroids and prostaglandins (108). Polyunsaturated fatty acids (PUFA) intake appears to have beneficial effects on female reproduction, namely on oocyte quality and embryo implantation (17, 18). The type and amount of PUFA may have direct effects on ovarian steroid synthesis, oocyte maturation and pregnancy outcome (109–112). Marine ω-3 fatty acids for instance were associated with increased progesterone concentrations, while docosapentaenoic acid (DPA) was correlated with decreased anovulation (19). The underlying biological mechanisms could be explained by an effect on steroidogenesis and prostaglandin synthesis (113).
In contrast, trans-fatty acids were found to negatively impact ovulatory function in women by promoting insulin resistance (21, 114). A negative association with fertility was also supported by the NHS-II study (20). Possible mechanisms may involve binding to peroxisome proliferator-activated receptor (PPAR) and thus modulating its expression (115). PPAR is known to be implicated in different ovarian functions such as follicular rapture, corpus luteum formation and steroid hormones metabolism (116–118). Increased intake of trans fatty acids also leads to insulin resistance and an increase in inflammatory markers (21, 22).
Overall, despite numerous study limitations, the bulk of the evidence favors a negative effect of a diet that is rich in trans fatty acid and low in PUFA on the reproductive outcomes in healthy women (6) (Table 1).
Micronutrients
Vitamins and minerals are essential dietary components that are implicated in different catabolic and anabolic processes associated with female reproduction (119). According to the NHS-II cohort study, multivitamin supplementation is inversely related to anovulatory dysfunction in women (23). Folate plays an essential role in human reproduction by affecting the synthesis of DNA, amino acids and methionine (120). The BioCycle study showed that intake of synthetic folate was associated with increased luteal progesterone levels and decreased risk of sporadic anovulatory cycles in premenopausal women (24). Vitamin D is another micronutrient implicated in the physiology of female reproduction, with receptors expressed in the ovaries, endometrium and placenta (121–123). A vitamin D deficient diet was associated with reduced fertility rates in female rodents (25). The same study showed restored reproductive capacity following vitamin D supplementation (25). It should be noted however, that these findings were not supported by other studies (26, 27).
Studies evaluating the effects of minerals on ovulatory infertility reported favorable outcomes following iron supplementation (28). Zinc deficiency was associated with abnormal menstruation and decreased pregnancy rates (29, 30). Women with low serum concentrations of zinc and selenium were more likely to experience longer time intervals to pregnancy (31). Deficiency of animal source nutrients, impacting levels of minerals such zinc, calcium and selenium, has been observed in women following vegan diet that could pose a risk for reproductive health (98).
Additional research is needed to further understand the role of different micronutrients on female reproductive health and thus shed light on the use of specific targeted nutrition as a therapeutic tool (Table 1).
Dietary patterns
Dietary patterns are important practical nutritional tools that provide insight into the individual's dietary habits. Dietary patterns are implicated in multiple complex nutritional interactions that affect general health and wellbeing (124). Mediterranean dietary patterns and Western-style diets are found to have a positive and a negative effect on female reproductive health outcomes, respectively (32–34).
Chavarro et al. evaluated the influence of dietary patterns on female fertility. They described the optimal “fertility diet” to resemble very closely the Mediterranean dietary pattern. Such a diet, characterized by a high vegetarian to animal protein ratio, full-fat dairy products, decreased glycemic load, vitamins, and increased monounsaturated fatty acids (MUFA) to trans saturated fats ratio, was associated with a 66% reduction in ovulatory disorders and a 27% decrease in infertility risks (32). The same group of authors also showed that consumption of fruits and vegetables with high pesticide residues was associated with low rates of clinical pregnancy and live births after ART treatment (125). The pathway analysis showed that high pesticide exposure was associated with metabolic pathways involving vitamins, energy and enzyme metabolism (126). Kazemi et al. furthermore showed that the Mediterranean diet may improve ovarian morphology and ovarian function in women of reproductive age (33). The authors suggested an association between diet-related improvements in ovarian morphology and a decrease in insulin resistance, weight and hyperandrogenism (33).
In contrast, the Western dietary pattern prevalent in developed countries is characterized by a high intake of red meat, fat, sugar drinks and refined grains (127). Although linked to poor reproductive outcomes, the underlying mechanisms remain unclear. When female macaques were fed a Western-like diet, they expressed lower follicle survival rates and lower androstenedione and estradiol serum levels compared with controls (34). Further research is needed to elucidate potential molecular and cellular mechanisms associating dietary patterns to female reproduction (Table 1).
The effect of diet on the outcomes of assisted reproductive technologies
Proteins and ART
Similar to the effect of protein intake on general female fertility, various protein sources have been associated with different effects on ART success (35, 36). A cohort study showed that the intake of animal-sourced proteins (red meat) correlated negatively with embryo development and with the likelihood of clinical pregnancy after intracytoplasmic sperm injection (ICSI). The same study also showed that a higher intake of dietary elements enriched in ω-3 PUFA and other protein sources (fish and white meat) correlated positively with the likelihood of blastocyst formation (35). Women who consumed fish as a primary source of proteins had higher probability of live births after ART compared with controls (36). In addition, Braga et al. found that the consumption of animal-based proteins (red meat with 26–27% protein content) before IVF treatment was negatively associated with embryo development and pregnancy rates (35). This negative association could be explained by the increased absorption of advanced glycation end products (AGE), which are prone to be developed during the cooking process in animal-derived food rich in protein (128). It is suggested that AGE accumulation is associated with poor follicular and embryonic development as well as with a low likelihood of pregnancy due to intracellular damage by cross-linking, molecular trapping or binding to specific cell surface receptors (129, 130).
Interestingly, studies investigating the effect of the soy intake showed contrasting findings (12, 37, 40). The intake of soy isoflavones was found to be associated with a 13% higher live birth rate (age adjusted) in the EARTH study (37). Similarly, a positive association was established between the isoflavone supplementation and progesterone concentration, endometrial thickness and pregnancy rates in women undergoing infertility treatment (38). The same findings were observed following intrauterine insemination (IUI) and IVF treatments, in which increased endometrial thickness as well as pregnancy rates were also observed (39, 42). On the contrary, a study conducted by Jacobsen et al. found a negative association between isoflavone intake and the likelihood of pregnancy (12). Similarly, a study conducted by Chavarro et al. found that urinary bisphenol A (BPA) concentration was correlated with lower rate of implantation, pregnancy and birth rates in women not consuming soy (40). However, no relation was observed between these outcomes and BPA levels in women consuming soy (40). Finally, no associations were observed between phytoestrogens intake and female fecundability (41).
Carbohydrates and ART
It is well-known that glucose homeostasis and insulin sensitivity are both affected by the type and amount of dietary carbohydrates, which further influence ovarian androgen production and function (15, 101). The intake of a hypocaloric diet (low glycemic index and glycemic load) was found to be positively associated with reproductive outcomes, such as the number of oocytes retrieved and live births in obese infertile women undergoing IVF and increased spontaneous pregnancy (43). This observed beneficial effect could be mediated through a reduction in body mass index (BMI), leptin concentration and body fat percentage (43). In another study, a high carbohydrate as well as fiber intake and a high dietary glycemic load were associated with poor ovarian response (44). However, an Italian cohort study found no significant associations between carbohydrate intake, dietary glycemic load/index and dietary fiber with IVF outcomes (45). Instead, complex carbohydrate intake, such as whole grain, was positively associated with the birth rate in women undergoing IVF, which could possibly be mediated through its effect on enhancing the endometrial thickness and endometrial receptivity (131).
Fats and ART
Fatty acids, mainly PUFA, play an important role in the development and maturation at different reproductive stages, thus influencing fertility and its related outcomes in women (106, 107). During oocyte maturation and early embryo development, they act as energy substrates as well as precursors for prostaglandins and steroid hormones synthesis, which impact pregnancy maintenance (106, 107). The beneficial effects of dietary PUFA intake on female reproductive endpoints were also reported in ART settings. Moran et al. found that the intake of PUFA (ω-6 PUFAs and ω-3 PUFA) was associated with improved pregnancy outcomes in obese and overweight women undergoing IVF treatment (46). Moreover, a significant association was observed between the intake of ω-3 PUFA, estradiol (E2) levels, embryo morphology, and the number of follicles in these women (18). The EARTH cohort showed similar findings, where the intake of long-chain ω-3 PUFA was positively associated with the likelihood of birth rates (47). A positive association was also observed in women undergoing ICSI between follicular fluid and serum levels of specific PUFA (linolenic and linoleic acids) which cannot be synthesized by the body (132). A 6-weeks intervention trial using daily supplement with ω-3 PUFA and vitamin D in 56 couples undergoing IVF or ICSI showed no difference in the second cell cycle (CC2) but faster fourth cell cycle (CC4) and a significantly shorted third cell cycle (S3) improving embryo quality (48). It should also be noted that several other studies showed conflicting results with a negative association between elevated level of different PUFA and ART outcomes (49–51). These discrepancies can be attributed to the genetic variation among different ethnic backgrounds in fatty acid metabolism plus the complexity of PUFA metabolism (47, 133). In addition, inconsistencies in the type of PUFA investigated in different research studies could explain this discrepancy, since ovarian follicles might metabolize specific fatty acids differently (50). This was suggested by Jungheim et al., who described a weak association between fatty acid concentrations in follicular fluid and serum, as well as differences in the levels of fatty acid types in the follicular fluid [linoleic (25%), oleic (31%), palmitic (27%) and stearic acids (12%)] (50). In contrast to the general beneficial effect of PUFA on ART outcomes, the intake of dietary trans fatty acid has been associated with a negative influence on reproductive health in women undergoing ART (51). A study conducted by Eskew et al. showed a negative association between the trans fatty acid index and IVF-related outcomes, such are fertilization and blastulation rate, but no association with the intake of ω-3 PUFA (51). The authors of this study suggest that the potential mechanism behind this observed effect may be multifactorial and could involve modulation of PPAR expression and glucose homeostasis (51, 115).
Micronutrients and ART
In the same way that the intake of different micronutrients is found to positively impact female reproductive health, this association is also observed with ART outcomes (5, 52, 53). It has been reported that women adhering to a “pro-fertility” diet, which is characterized by the intake of vitamin D, B12, folic acid whole grains, soy foods, dairy, fruits and vegetables, have increased likelihood of live birth after ART (52). In addition, in a cohort of women eligible for IVF treatment, women who consumed folic acid have decreased concentration of homocysteine in follicular fluid and serum, and showed improved quality and increased maturity of oocytes (53). High folate intake in women undergoing IVF/ICSI was also associated with increased rate of implantation, clinical pregnancy and live birth rates (5).
Vitamin D is an important micronutrient that has been linked with female reproductive health (25) and ART outcomes (54) although the existing evidence is controversial. In one study, women who were undergoing IVF and had higher levels of vitamin D (in both follicular fluid and serum) were found to have an impressive 4-folds higher pregnancy rate compared with vitamin D deficient women, thereby, suggesting a strong beneficial effect of vitamin D supplementation on ART outcomes (54). A systematic review also showed a positive relationship between vitamin D and rate of live births in women undergoing ART, suggesting that vitamin D supplementation could be a possible therapeutic tool (134). In addition, higher rates of clinical pregnancy per IVF cycle are observed in women with sufficient vitamin D level (54.9%) compared to those with vitamin D insufficiency (34.7%) (55). Similarly, a study conducted by Rudick et al. found that women undergoing IVF treatment and vitamin D deficient have a lower rate of clinical pregnancy development and lower birth rates (56). The authors of this study suggested that the impact of vitamin D on IVF could be mediated through a direct effect on the endometrium (56). In another group of women undergoing IVF and elective single embryo transfer (ESET), vitamin D deficiency was also found to be negatively correlated with clinical pregnancy rates (57). However, a number of studies have reported no association between vitamin D and fertility-related outcomes in women undergoing ART (58–60). The exact cause-effect relationship between vitamin D and ART outcomes is yet to be identified due to the high heterogeneity observed among the different observational and interventional studies (135).
Last but not least, various studies have shown that women undergoing IVF have reduced levels of several trace elements, mainly selenium, copper and zinc, in the follicular fluid, which impact the quality of the microfollicular environment (61–63). However, the level of these trace elements is restored after dietary supplementation and therefore indicates its possible role in improving fertility rates and ART outcomes (61–63). Albeit another study conducted by Ng et al. didn't find any association between the concentration level of zinc, calcium and copper in the follicular fluid with follicle volume, oocyte fertilization rate and a number of oocytes in the follicle in women undergoing IVF. These mixed results likely stem from the inherent difficulties of assessing reproductive outcomes in the infertile population (64).
Dietary pattern and ART
Several studies have shown the potential positive impact of adherence to a Mediterranean diet in women undergoing IVF (65). Vujkovic et al. showed that adherence to the “Mediterranean” dietary pattern led to a 40% increased probability of achieving pregnancy after IVF/ICSI treatment (65). The adherence to this diet was reflected by relatively high concentrations of folate and vitamin B6 both in blood and in follicular fluid (65). Another study by Karayiannis et al. showed that adherence to the Mediterranean diet as assessed by using the validated MedDiet Score was associated with a 2.7-folds increase in clinical pregnancy and live birth rate in women aged <35. Interestingly the same effect was not evident in older women in the study (66). In a more recent investigation in women undergoing IVF, a positive association was found between adherence to the Mediterranean diet, fertilization rates and embryo yield (67). However, in this study Mediterranean diet was not correlated with clinical pregnancy and implantation rates (67). It is suggested that the Mediterranean dietary pattern is associated with improved ART outcomes as this pattern is rich in antioxidants, which are known to alleviate the oxidative damage on female reproductive health, as well as, being associated with improved fertility rates (67, 68). Another study conducted by Kermack et al. aimed to investigate the influence of pre-conceptional Mediterranean dietary intervention in women before IVF or ICSI treatment on key morpho-kinetic markers of early embryo development (48). The authors of this study observed that embryos derived from women consuming the “Mediterranean diet” (ω-3 PUFA, vitamin D and olive oil) have shortened the fourth cell cycle (CC4) and synchrony of the third cell cycle (S3) (48). Although several studies showed that the intake of the Mediterranean diet is associated with beneficial effects on female reproductive health and successful ART outcome, in an Italian cohort study of 474 women, adherence to the Mediterranean diet was not associated with favorable IVF outcomes (69). Another study showed that women undergoing IVF who adhere to the Dutch dietary recommendations (whole wheat bread, monounsaturated or polyunsaturated oils, vegetables, fruit and fish) have an 65% increased chance of ongoing pregnancy (136). On the other hand, the short-term intake of a Western-like diet was found to promote a proinflammatory intrafollicular microenvironment, which is associated with impaired preimplantation development in the female rhesus macaques model (70).
Overall despite the difficulties in evaluating dietary patterns and controlling the variable confounding factors it seems that nutritional modifications in subfertile women may positively affect fertility rates after ART and female reproductive health as a whole (137). A summary of the current evidence is presented in Table 2.
Equally physical activity and energy expenditure have an important role in fertility however the evidence is still contrasting on the efficacy of the physical activity and the difference according to the type of patients: increasing energy expenditure will benefit the normal population (138), where excessive exercise can be detrimental in female athletes (139). As many reviews are available on the topic and the argument (138–141), we do not discuss it further in this review.
Nutrigenetics and nutrigenomics
Nutrigenomics is the science investigating the influence of nutritional factors on gene expression, epigenetics, protein expression, metabolome, and microbiome, while nutrigenetics studies the influence of genetic variations on nutrients metabolism (142, 143). The development of these sciences helped to shed light on the potential interplay between genes function and nutritional intake which can contribute to the establishment of more precise nutritional assessment tools (144). A better understanding of these interconnections may aid in the development of personalized nutritional recommendations for the purpose of optimizing fertility outcomes (Table 3).
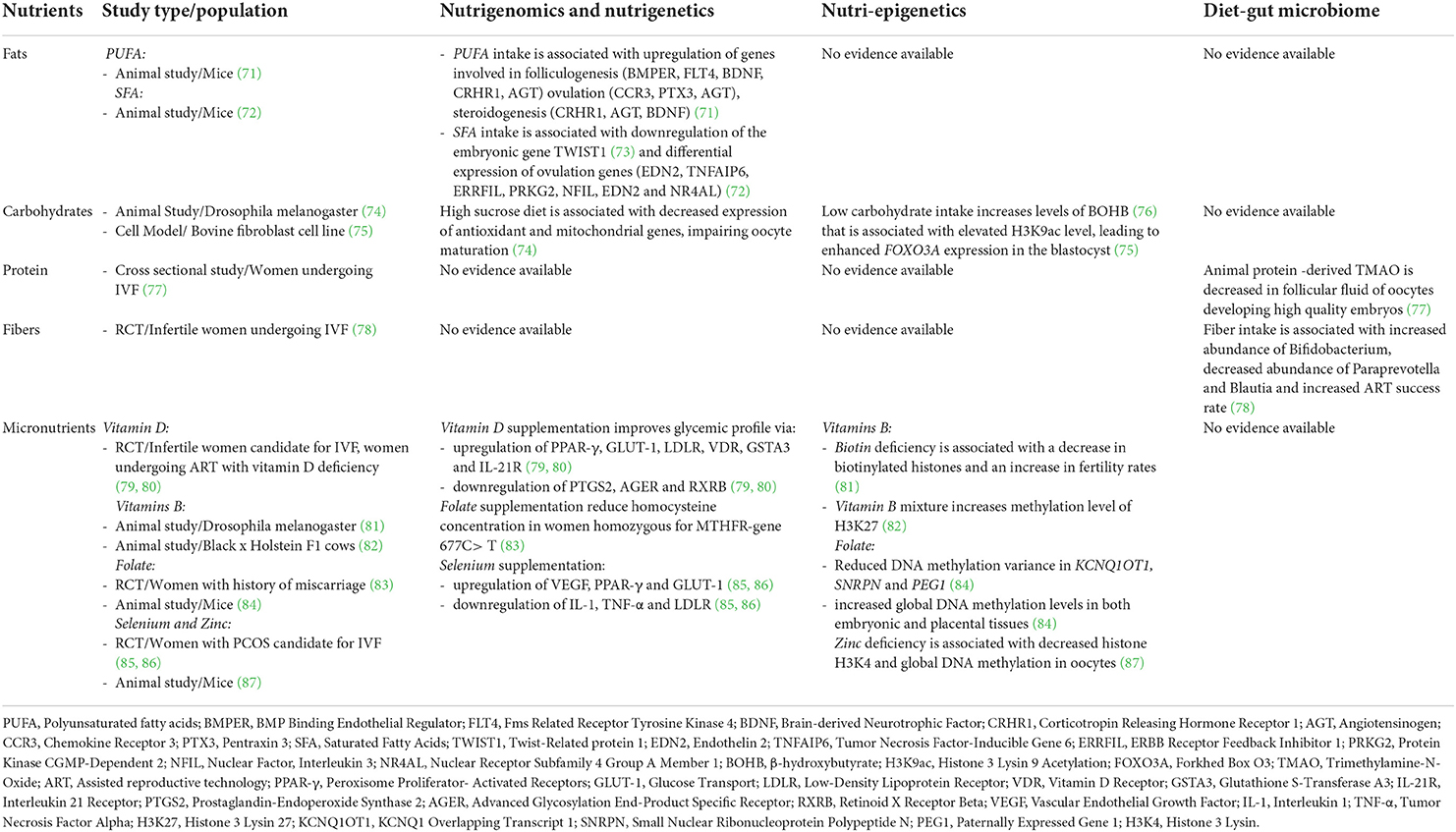
Table 3. The role of nutrigenomics, nutri-epigenetics and diet - gut microbiome axis in influencing ART outcomes.
Nutrigenetics and nutrigenomics in female reproduction and ART
Although female infertility is strongly affected by environmental factors, genetics play an essential part in its pathogenesis, in what appears to be a polygenic inheritance pattern (145). Both ovulatory dysfunction and endometriosis are recognized causes of female infertility and have been known to have familial predisposition indicating genetic involvement (146). In addition, different genetic abnormalities have been reported to cause female infertility, such as chromosomal alterations and single gene mutations [Anosmin 1 (ANOS1), Gonadotropin Releasing Hormone (GnRH) and Homoebox (HOX) genes]. These genetic risk factors lead to the development of endometriosis, hypogonadism and ovarian dysfunction, which ultimately lead to infertility (146).
Genetic variations are known to impact also on nutritients metabolism and dietary requirements (147) and certain genetic variants can affect ART outcome. Two variants of the methylenetetrahydrofolate reductase (MTHFR) gene (c.677C > T and c.1298A > C) are well-known to reduce its enzymatic activity and increase homocysteine levels, which alter folate metabolism (148). A study conducted by Nelen et al. showed that daily supplementation with folic acid reduced homocysteine concentration in women homozygous for MTHFR-gene 677C > T mutation with a history of recurrent miscarriages (83). It appears that folic acid supplementation has a beneficial role in female patients with MTHFR polymorphisms. In their study, Laanpere et al. showed that folate-metabolizing gene polymorphisms may affect IVF outcomes, suggesting that heterozygotes rather than wild type homozygotes have overall more favorable ART outcomes including embryo quality and clinical pregnancy rates (149). Another study in women undergoing ICSI for male factor infertility showed that in women with the TT genotype for MTHFR (c.677C > T) serum AMH levels were increased however there was a non-significant trend toward lower clinical pregnancy rates compared to the ones with the CT genotype (150). Finally, a case-series report showed that couples carrying MTHFR variants (C677T or A1298C) suffer from fertility problems or failed ART, and they can benefit from 5-methyltetrahydrofolate (5-MTHFF) supplementation vs. folic acid (151). The study showed that high intake of folic acid in these patients resulted in un-metabolized folic acid syndrome. Moreover, the treatment with 5-MTHFF increased the chances of pregnancy development.
Nutrients have been known for a long time to act as dietary signals which affect gene expression, protein production and hormonal function (142). Macro- and micro-nutrients are able to control the transcription process. Flavonoids for instance facilitate transcriptional activation by binding to nuclear receptors leading to co-repressor dissociation and co-activator recruitment (142, 152). Irani et al. found that vitamin D supplementation has a beneficial effect on folliculogenesis in women with PCOS, by attenuating AGE-mediated inflammation (153).
Chronic consumption of high-fat diet (exceeding the 35% calories recommendation) (154), has been associated with metabolic and physiological disturbances involving the reproductive system. Ruebel et al. aimed to compare oocyte gene expression in overweight/obese women undergoing fertility treatment on the basis of their dietary intake of fats and carbohydrates compared to normal weight controls (73). They found a positive association between saturated fatty acid (SFA) intake and downregulation of the oocyte Twist Family BHLH Transcription Factor 1 (TWIST1) gene expression in overweight/obese women. No significant associations were found with BMI or adiposity, suggesting that maternal fat intake plays an important role in modulating the inflammatory response of the oocyte (73). TWIST1 is a transcription factor involved in embryonic development and influences lipid metabolism and inflammation (155, 156). Increased fat intake, regardless of obesity status, was also found to be associated with menstrual irregularity and ovarian reserve depletion. The fat diet also altered the expression of genes controlling ovulation, luteinization and luteolysis (72). Another study investigated the effects of an acute dietary intake of docosahexaenoic acid (DHA), an ω-3 PUFA, on restoring ovarian gene expression following chronic high fat diet consumption in female mice (71). The investigator reported a beneficial outcome of DHA supplementation on the upregulation of ovarian genes involved in folliculogenesis, ovulation and steroidogenesis. The expression of the Gm42508 genetic marker was nonetheless increased after high-fat feeding and was unaffected by DHA, which suggests a potential role in high fat diet-induced ovarian dysfunction (71). Oseikria et al. investigated the effects of DHA supplementation on oocyte maturation and embryo development in cattle undergoing in vitro maturation (IVM). Low dose DHA supplementation during IVM was associated with improved oocyte development without affecting lipid metabolism gene expression (157). Furthermore, in female rhesus macaques, the intake of Western-like diet was found to be associated with dysregulation of genes involved in mitochondrial function, protein channel activity, RNA binding and cell differentiation (70).
Increased intake of dietary sugars was also observed to negatively impact female reproductive efficiency and the expression of essential genes associated with fertility. A study conducted using Drosophila melanogaster as animal model found that female adults fed with a high sucrose diet developed insulin resistance, accumulated lipids in the ovaries and had impaired oocyte maturation. Decreased expression of antioxidant and key mitochondrial genes were also detected (74). This study also showed that a high sucrose diet-induced maternal obesity (158), and impacted negatively fertility, without nonetheless lending support for a direct effect of high sucrose intake (74).
Micronutrients (vitamins and minerals) are found to impact female reproductive health and play essential roles in different stages of conception (119). In a randomized controlled trial (RCT), Dastornia et al. investigated the effects of vitamin D supplementation on gene expression in PCOS patients who were candidates for IVF (79). They found an upregulation of PPAR-γ, Glucose transport (GLUT-1) and low-density lipoprotein receptor (LDLR) genes after vitamin D supplementation when compared to the controls (79). Another randomized controlled trial observed the upregulation of vitamin D receptor (VDR), glutathione S-transferase A3 (GSTA3) and interleukin 21 receptor (IL-21R), as well as the downregulation of prostaglandin-endoperoxide synthase 2 (PTGS2), advanced glycosylation end-product specific receptor (AGER) and retinoid X receptor beta (RXRB) in women receiving vitamin D supplementation compared to placebo (80). The supplementation improved the glycemic profile affecting the glycemic and lipid metabolism (79) and activating the antioxidant pathways (80). While selenium was also implicated in improving reproductive health, the exact mechanism is not yet identified (159). In a recent study conducted by Heidar et al. the investigators found the expression of interleukin-1 (IL-1) and tumor necrosis factor alpha (TNF-α) to be downregulated following selenium supplementation in PCOS women undergoing IVF. Conversely, vascular endothelial growth factor (VEGF) was upregulated in the same study population (85). The same investigators also found that selenium supplementation resulted in the upregulation of PPAR-γ and GLUT-1, along with the downregulation of LDLR (86).
Nutri-epigenetics in female reproduction and ART
Nutrition has a strong impact on the epigenome during early embryo development. It also affects oocyte maturation and oocyte stores of mitochondria and metabolites (160), thus a better understanding of the interplay between diet and epigenetic modifications and its impact on female reproductive health is needed.
The relationship between nutritional factors, histone modification and fertility is not clearly understood. However, in a study by Sangalli et al there was a potential relationship between intake of β-hydroxybutyrate (BOHB), histone acetylases (H3K9ac) and embryonic development. The authors also found that BOHB has an epigenetic effect (enhancing the level of H3K9ac) in the early zygote stage that was maintained until the blastocyst stage and resulted in enhanced Forkhed Box O3 (FOXO3A) expression and blastocyst production (75). Increased BOHB level is associated with fasting and lower carbohydrate intake (76), thereby, this study shed the light on the potential interplay between maternal nutrition, histone modification and reproduction. Another study conducted on Drosophila melanogaster showed that flies maintained on a biotin-deficient medium are associated with a decrease in biotinylated histones and an increase in fertility rates (81). In addition, vitamin mixtures (vitamin B and vitamin B-like substances) are found to decrease the mRNA expression level of thioredoxin-interacting protein (TXNIP) and increase level of H3K27 trimethylation after IVF treatment of bovine embryos (82). A study conducted by Tian et al. observed a decrease in histone H3K4 methylation level and global DNA methylation in oocytes treated with a zinc deficient diet. The authors of this study also found that supplementing zinc deficient animals with methyl donors during IVM restored histone H3K4 and increased IVF success rates (87).
Regarding DNA methylation, there are limited data about the possible interplay between diet and female reproductive health. In general, nutrients modulate DNA methylation by acting as methyl donors (methionine, choline, and folate), providing co-factors essential for methyltransferases activity (vitamins B2, B6, and B12) and modulating the activity of enzymes in the methylation process (polyphenol) (161). Folic acid supplementation is recommended during pregnancy due to its prophylactic role, but its influence on women undergoing ART is not as widely recognized. One study showed that a moderate intake of folic acid is positively associated with a decreased proportion of developmentally delayed embryos in women undergoing ART (84). The authors of this study also observed that a moderate intake of folic acid in the ART population is associated with reduced DNA methylation variance in imprinting control regions [KCNQ1 overlapping transcript 1 (Kcnq1ot1), small nuclear ribonucleoprotein polypeptide N (SNRPN) and paternally expressed gene 1 (PEG1)] and increased global DNA methylation levels in both embryonic and placental tissues (84).
It has been shown that several dietary components influence the epigenome and have beneficial health outcomes. Therefore, the development of an “epigenetic diet” used to neutralize epigenomic aberrations might pave the way toward an innovative approach to improve fertility rates and female reproductive health (162).
Microbiota in female reproduction and ART
It is well-known that dietary components influence the composition of the microbiome, however, whether this effect has an impact on female reproductive health and fertility rates is not clearly understood. Nutritional factors shape the microbiome either directly by enhancing/inhibiting microbial growth or indirectly by influencing the immune system and metabolism (163). The relationship between diet and gut microbiome and its influence on female reproductive health and ART outcomes is not clearly understood. A study conducted by Nagy et al. found that elevated levels of trimethylamine-N-oxide (TMAO) are negative predictors of oocyte fertilization and embryo quality in women undergoing IVF (77). TMAO is a diet-induced microbial metabolite that is produced from microbial utilization of dietary choline (present in fish and dairy products) and L-carnitine (present in red meat) (164). The authors reported decreased levels of TMAO and its intermediate gamma-butyrobetaine in follicular fluid of oocytes developing into top-quality embryos compared to oocytes developing into low-quality embryos. However, a non-significant association was found between levels of TMAO precursors (choline and L-carnitine) and oocyte and embryo quality (77). Another study showed the positive impact of dietary fiber intake on female infertile patients treated with ART (78). The intake of partially hydrolyzed guar gum (PHGG), a prebiotic water-soluble fiber known to modulate the gut microbiome, improved gut dysbiosis and the success rate of ART in these women. Enhanced Bifidobacterium abundance and reduced Paraprevotella and Blautia abundance were identified as potential predictive microbial markers of successful pregnancy (78).
Vaginal microbial composition is also an important indicator of female reproductive health and potential ART success. A recent prospective exploratory study suggested that identifying vaginal microbial profiles before IVF-ICSI could help to predict pregnancy outcomes and improve success rates (165). The authors showed that female patients with a non-favorable vaginal microbial profile (relative Lactobacillus load <20%, Proteobacteria >28% and presence of G. vaginalis) had seven times lower chance of pregnancy than those with a more favorable profile (high abundance of Lactobacillus crispatus and Lactobacillus iners) (165). These findings indicate a critical role for the vaginal microbiome in the female reproductive health and ART outcome. Undoubtedly the study of associations between diet, vaginal microbiome and fertility is an area of significant interest where additional research is required.
Future perspective of personalized nutrition in ART
The use of nutraceuticals has been suggested as a potential therapeutic tool to treat women with reproductive disorders. Nutraceuticals are defined as food products that can be administered as dietary supplements with physiological benefits beyond basic nutritional needs (e.g., vitamins, minerals, herbs, fatty acids, etc.) (166). They are considered relatively safe and inexpensive compared to other chemical agents and are even thought to be effective in modifying the course of some disorders like breast cancer, cervical cancer, and ovarian cancer (167). Encouraging data support the use of nutraceuticals for female reproductive disorders. In women undergoing IVF, the intake of DHA and alpha-linoleic acid (ALA) has been positively associated with an increased number of follicles, E2 serum levels and favorable embryo quality (18). Other studies have suggested that regular intake of multivitamins and iron reduces the risk of ovulatory dysfunction (23, 28). The therapeutic effectiveness and safety of nutraceuticals depend mainly on their formulations (food extracts, isolated active constituents, etc.); thereby, quality control programs are critical to monitor their production. One main limitation identified is the absence of governmental agencies to regulate manufacturing practices and quality standards. As a result, many products invade the market with no scientific evaluation and validation. National and international policies are therefore urgently needed to standardize and validate production, and also to regulate research and development (168).
Recently, the use of probiotics in clinics has gained popularity amongst patients and general consumers due to the perception that they may modulate the microbial composition of the human body and affect disease processes (169). Gut and vaginal microbial composition are known to play a significant role in the wellbeing of the female reproductive system, which explains the growing interests in the field of human reproduction and ART (77, 78). According to the International Scientific Association of Probiotics and Prebiotics (ISAPP), a probiotic is defined as the adequate administration of living microorganisms that confer a benefit on the host (170). Probiotics have been therefore suggested as potential therapeutic tools for the healing of fertility-related disorders and enhancers of female reproductive health in general.
The oral administration of probiotics (Lactobacillus rhamnosus GR-1 and Lactobacillus fermentum RC-14) has been shown to maintain and restore normal vaginal microbial composition in patients previously diagnosed with vaginal dysbiosis (171). In addition, low colonization of Lactobacillus species was found to be associated with poor reproductive outcomes, namely implantation failure, pregnancy loss and adverse pregnancy outcomes (172–174). Additional research is needed to investigate the potential benefits of probiotics on female reproduction and to characterize strain-specific biological effects (169, 175). The pattern of probiotic administration and the identification of the target population should be taken into account when considering this therapeutic approach (176). As probiotics may affect pathophysiologic disease pathways and modulate their risk factors, the proper selection of the study population should take into account basic physiology. In addition, the use of both epidemiological background data and intervention methods may help in identifying the optimal probiotic to be used for the specific population based on their genetics, microbial profile and other clinical parameters (176).
The use of prebiotics has also been suggested as a potential therapeutic tool, as they are non-digestible carbohydrates that help probiotic microorganisms to grow and achieve their potential beneficial effect on human health. In addition, prebiotics, such as inulin and fructo-oligosaccharides, are believed to have a synergistic impact on health when combined with probiotics (177). A study by Cho et al. concluded that the intake of prebiotics (oligo-fructose) improves the pregnancy index in obese rat (178). Another study however observed no abnormalities or changes in the fertility rate and other reproductive function parameters in rats treated with prebiotics (galacto-oligosaccharides) (179). Kyno et al. found that IVF patients presenting with non-Lactobacillus-dominated microbiota (NLDM) in their endometrium had increased pregnancy rates following the restoration of their vaginal microbial flora to Lactobacillus-dominated microbiota (LDM) with the use of antibiotics and prebiotic/probiotic supplements (180). Needless to say, additional research is required to identify the true impact of prebiotics/probiotics on female reproductive health and shed light on its potential use as a therapeutic intervention.
The introduction of nutritional therapy opened the door for a new therapeutic intervention for disorders of the female reproductive system. However, there are several challenges to this end. There are no official or identified nutritional guidelines established for female fertility and related outcomes. This is likely a result of the inherent complexity in investigating the potential interplay between nutritional factors, microbial composition, genome and epigenome and its impact on female reproduction (77).
Conclusion
The “Food as Medicine” movement has been gaining popularity for several years. More and more people worldwide are becoming consciously aware of their nutritional habits and engage in efforts to modify their diets to reap various health benefits. In parallel, health professionals are increasingly open to the practice of “prescribing” nutritional changes or launching specialized diet programs aiming to prevent, limit or even reverse disease by changing what patients eat. In particular, couples who struggle with fertility issues and more so those who embark on ART treatments, are often highly motivated and willing to follow specialist advice especially when it comes to lifestyle changes, diet or intake of supplements, as the possibility that they will be able to overcome infertility by natural methods without the use of invasive treatments or drugs is usually very appealing to them. It does appear that nutrition plays an essential role in female reproductive health and outcomes by modulating the genome, epigenome and microbiome composition and in response to the individual genetic background. Several dietary factors, such as PUFA, folate, fiber, starch as well as the Mediterranean diet, appear to exert beneficial effects on female fertility and the success of ART (Figure 1). In contrast, other dietary factors (e.g., trans saturated fatty acids) may negatively impact female reproductive health, contributing to ovulatory disorders, reduced fecundability and endometriosis (6, 20). Understanding the direct link between dietary intake and female fertility is crucial as diet is directly implicated in the development of other chronic metabolic conditions, such as obesity, that have an impact on reproductive health. Despite the growing research interest and available evidence as discussed in our review, significant work remains to be done. A large number of relevant studies rely on relatively subjective tools and there is always the element of bias, more so in emotionally burdened infertile couples who complete specific interviews or nutritional questionnaires Fortunately, novel advances in genomic sciences are emerging which are expected to enhance our understanding of the nature and determinants of reproductive functions and the interplay with nutrition. The development of Nutrigenomics & Nutri(epi)genetics has the potential to pave the way for the adoption of precision nutrition in infertility management. Specific patients may have diverse dietary needs and tailored nutritional changes may activate relevant molecular pathways to meet the final goals of treatment Therefore, in the not-so-distant future, personalized nutritional advice and prescription of nutraceuticals for women who desire conception may become the new normal bringing a paradigm shift in the management of reproductive system disorders.
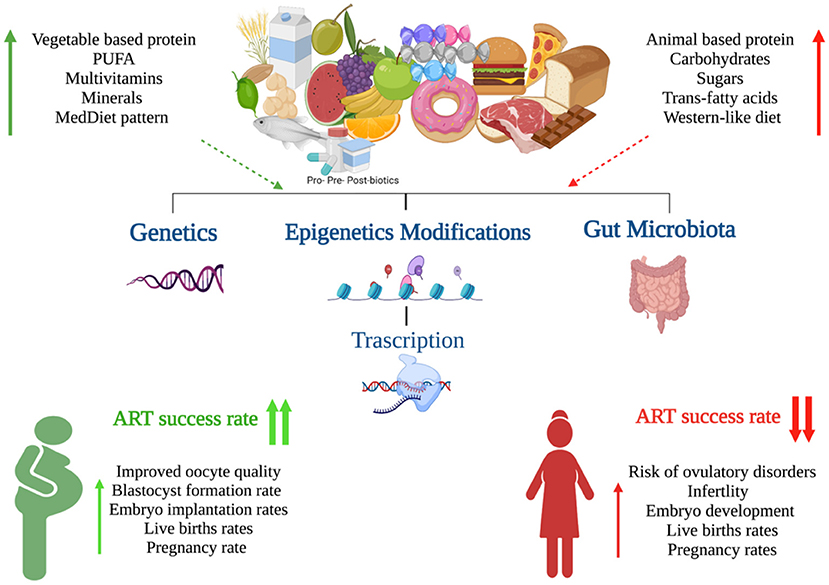
Figure 1. Graphical summary of the impact of nutrition on female fertility (Created with BioRender.com).
Author contributions
AT and SC designed the content. AK and SC draft the manuscript. AT, SA, AL, SA, and JA reviewed the draft. All authors contributed to the article and approved the submitted version.
Funding
This work was supported by Sidra Medicine funds (SDR400174).
Conflicts of interest
The authors declare that the research was conducted in the absence of any commercial or financial relationships that could be construed as a potential conflict of interest.
Publisher's note
All claims expressed in this article are solely those of the authors and do not necessarily represent those of their affiliated organizations, or those of the publisher, the editors and the reviewers. Any product that may be evaluated in this article, or claim that may be made by its manufacturer, is not guaranteed or endorsed by the publisher.
References
2. Kushnir VA, Barad DH, Albertini DF, Darmon SK, Gleicher N. Systematic review of worldwide trends in assisted reproductive technology 2004–2013. Reprod Biol Endocrinol. (2017) 15:6. doi: 10.1186/s12958-016-0225-2
3. Panth N, Gavarkovs A, Tamez M, Mattei J. The influence of diet on fertility and the implications for public health nutrition in the United States. Front Public Health. (2018) 6:211. doi: 10.3389/fpubh.2018.00211
4. Gaskins AJ, Chavarro JE. Diet and fertility: a review. Am J Obstet Gynecol. (2018) 218:379–89. doi: 10.1016/j.ajog.2017.08.010
5. Gaskins AJ, Afeiche MC, Wright DL, Toth TL, Williams PL, Gillman MW, et al. Dietary folate and reproductive success among women undergoing assisted reproduction. Obstet Gynecol. (2014) 124:801–9. doi: 10.1097/AOG.0000000000000477
6. Wise LA, Wesselink AK, Tucker KL, Saklani S, Mikkelsen EM, Cueto H, et al. Dietary fat intake and fecundability in 2 preconception cohort studies. Am J Epidemiol. (2018) 187:60–74. doi: 10.1093/aje/kwx204
7. Chiu YH, Chavarro JE, Souter I. Diet and female fertility: doctor, what should I eat? Fertil Steril. (2018) 110:560–9. doi: 10.1016/j.fertnstert.2018.05.027
8. Sanderman EA, Willis SK, Wise LA. Female dietary patterns and outcomes of in vitro fertilization (IVF): a systematic literature review. Nutr J. (2022) 21:5. doi: 10.1186/s12937-021-00757-7
9. Wang L, Tang J, Wang L, Tan F, Song H, Zhou J, et al. Oxidative stress in oocyte aging and female reproduction. J Cell Physiol. (2021) 236:7966–83. doi: 10.1002/jcp.30468
10. Chavarro JE, Rich-Edwards JW, Rosner BA, Willett WC. Protein intake and ovulatory infertility. Am J Obstet Gynecol. (2008) 198:210.e1–7. doi: 10.1016/j.ajog.2007.06.057
11. Zhuo Y, Hua L, Feng B, Jiang X, Li J, Jiang D, et al. Fibroblast growth factor 21 coordinates adiponectin to mediate the beneficial effects of low-protein diet on primordial follicle reserve. EBioMedicine. (2019) 41:623–35. doi: 10.1016/j.ebiom.2019.02.020
12. Jacobsen BK, Jaceldo-Siegl K, Knutsen SF, Fan J, Oda K, Fraser GE. Soy isoflavone intake and the likelihood of ever becoming a mother: the Adventist Health Study-2. Int J Womens Health. (2014) 6:377–84. doi: 10.2147/IJWH.S57137
13. Chavarro JE, Rich-Edwards JW, Rosner BA, Willett WC. A prospective study of dietary carbohydrate quantity and quality in relation to risk of ovulatory infertility. Eur J Clin Nutr. (2009) 63:78–86. doi: 10.1038/sj.ejcn.1602904
14. Rodney R, Celi P, Scott W, Breinhild K, Santos J, Lean I. Effects of nutrition on the fertility of lactating dairy cattle. J Dairy Sci. (2018) 101:5115–33. doi: 10.3168/jds.2017-14064
15. Willis SK, Wise LA, Wesselink AK, Rothman KJ, Mikkelsen EM, Tucker KL, et al. Glycemic load, dietary fiber, and added sugar and fecundability in 2 preconception cohorts. Am J Clin Nutr. (2020) 112:27–38. doi: 10.1093/ajcn/nqz312
16. Gaskins AJ, Mumford SL, Zhang C, Wactawski-Wende J, Hovey KM, Whitcomb BW, et al. Effect of daily fiber intake on reproductive function: the BioCycle Study. Am J Clin Nutr. (2009) 90:1061–9. doi: 10.3945/ajcn.2009.27990
17. Nehra D, Le HD, Fallon EM, Carlson SJ, Woods D, White YA, et al. Prolonging the female reproductive lifespan and improving egg quality with dietary omega-3 fatty acids. Aging Cell. (2012) 11:1046–54. doi: 10.1111/acel.12006
18. Hammiche F, Vujkovic M, Wijburg W, de Vries JH, Macklon NS, Laven JS, et al. Increased preconception omega-3 polyunsaturated fatty acid intake improves embryo morphology. Fertil Steril. (2011) 95:1820–3. doi: 10.1016/j.fertnstert.2010.11.021
19. Mumford SL, Chavarro JE, Zhang C, Perkins NJ, Sjaarda LA, Pollack AZ, et al. Dietary fat intake and reproductive hormone concentrations and ovulation in regularly menstruating women. Am J Clin Nutr. (2016) 103:868–77. doi: 10.3945/ajcn.115.119321
20. Chavarro JE, Rich-Edwards JW, Rosner BA, Willett WC. Dietary fatty acid intakes and the risk of ovulatory infertility. Am J Clin Nutr. (2007) 85:231–7. doi: 10.1093/ajcn/85.1.231
21. Lefevre M, Lovejoy JC, Smith SR, Delany JP, Champagne C, Most MM, et al. Comparison of the acute response to meals enriched with cis- or trans-fatty acids on glucose and lipids in overweight individuals with differing FABP2 genotypes. Metabolism. (2005) 54:1652–8. doi: 10.1016/j.metabol.2005.06.015
22. Baer DJ, Judd JT, Clevidence BA, Tracy RP. Dietary fatty acids affect plasma markers of inflammation in healthy men fed controlled diets: a randomized crossover study. Am J Clin Nutr. (2004) 79:969–73. doi: 10.1093/ajcn/79.6.969
23. Chavarro JE, Rich-Edwards JW, Rosner BA, Willett WC. Use of multivitamins, intake of B vitamins, and risk of ovulatory infertility. Fertil Steril. (2008) 89:668–76. doi: 10.1016/j.fertnstert.2007.03.089
24. Gaskins AJ, Mumford SL, Chavarro JE, Zhang C, Pollack AZ, Wactawski-Wende J, et al. The impact of dietary folate intake on reproductive function in premenopausal women: a prospective cohort study. PLoS One. (2012) 7:e46276. doi: 10.1371/journal.pone.0046276
25. Kwiecinksi GG, Petrie GI, DeLuca HF. 1,25-Dihydroxyvitamin D3 restores fertility of vitamin D-deficient female rats. Am J Physiol. (1989) 256:E483–7. doi: 10.1152/ajpendo.1989.256.4.E483
26. Mumford SL, Silver R, Sjaarda LA, Galai N, Stanford J, Lynch A, et al. Vitamin D and Ovarian Reserve and Fecundability among Women with Proven Fecundity. FASEB J. (2016) 30:290.6.
27. Møller UK, Streym S, Heickendorff L, Mosekilde L, Rejnmark L. Effects of 25OHD concentrations on chances of pregnancy and pregnancy outcomes: a cohort study in healthy Danish women. Eur J Clin Nutr. (2012) 66:862–8. doi: 10.1038/ejcn.2012.18
28. Chavarro JE, Rich-Edwards JW, Rosner BA, Willett WC. Iron intake and risk of ovulatory infertility. Obstet Gynecol. (2006) 108:1145–52. doi: 10.1097/01.AOG.0000238333.37423.ab
29. Swenerton H, Hurley LS. Severe zinc deficiency in male and female rats. J Nutr. (1968) 95:8–18. doi: 10.1093/jn/95.1.8
30. Swenerton H, Hurley LS. Zinc deficiency in rhesus and bonnet monkeys, including effects on reproduction. J Nutr. (1980) 110:575–83. doi: 10.1093/jn/110.3.575
31. Grieger JA, Grzeskowiak LE, Wilson RL, Bianco-Miotto T, Leemaqz SY, Jankovic-Karasoulos T, et al. Maternal selenium, copper and zinc concentrations in early pregnancy, and the association with fertility. Nutrients. (2019) 11:1609. doi: 10.3390/nu11071609
32. Chavarro JE, Rich-Edwards JW, Rosner BA, Willett WC. Diet and lifestyle in the prevention of ovulatory disorder infertility. Obstet Gynecol. (2007) 110:1050–8. doi: 10.1097/01.AOG.0000287293.25465.e1
33. Kazemi M, Jarrett BY, Vanden Brink H, Lin AW, Hoeger KM, Spandorfer SD, et al. Obesity, insulin resistance, and hyperandrogenism mediate the link between poor diet quality and ovarian dysmorphology in reproductive-aged women. Nutrients. (2020) 12:1953. doi: 10.3390/nu12071953
34. Xu J, McGee WK, Bishop CV, Park BS, Cameron JL, Zelinski MB, et al. Exposure of female macaques to Western-style diet with or without chronic T in vivo alters secondary follicle function during encapsulated 3-dimensional culture. Endocrinology. (2015) 156:1133–42. doi: 10.1210/en.2014-1711
35. Braga DP, Halpern G, Setti AS, Figueira RC, Iaconelli A Jr, Borges E Jr. The impact of food intake and social habits on embryo quality and the likelihood of blastocyst formation. Reprod Biomed Online. (2015) 31:30–8. doi: 10.1016/j.rbmo.2015.03.007
36. Nassan FL, Chiu Y-H, Vanegas JC, Gaskins AJ, Williams PL, Ford JB, et al. Intake of protein-rich foods in relation to outcomes of infertility treatment with assisted reproductive technologies. Am J Clin Nutr. (2018) 108:1104–12. doi: 10.1093/ajcn/nqy185
37. Vanegas JC, Afeiche MC, Gaskins AJ, Mínguez-Alarcón L, Williams PL, Wright DL, et al. Soy food intake and treatment outcomes of women undergoing assisted reproductive technology. Fertil Steril. (2015) 103:749–55.e2. doi: 10.1016/j.fertnstert.2014.12.104
38. Shahin AY, Ismail AM, Zahran KM, Makhlouf AM. Adding phytoestrogens to clomiphene induction in unexplained infertility patients–a randomized trial. Reprod Biomed Online. (2008) 16:580–8. doi: 10.1016/S1472-6483(10)60465-8
39. Unfer V, Casini ML, Costabile L, Mignosa M, Gerli S, Di Renzo GC. High dose of phytoestrogens can reverse the antiestrogenic effects of clomiphene citrate on the endometrium in patients undergoing intrauterine insemination: a randomized trial. J Soc Gynecol Investig. (2004) 11:323–8. doi: 10.1016/j.jsgi.2003.12.007
40. Chavarro JE, Mínguez-Alarcón L, Chiu Y-H, Gaskins AJ, Souter I, Williams PL, et al. Soy intake modifies the relation between Urinary Bisphenol A concentrations and pregnancy outcomes among women undergoing assisted reproduction. J Clin Endocrinol Metab. (2016) 101:1082–90. doi: 10.1210/jc.2015-3473
41. Wesselink AK, Hatch EE, Mikkelsen EM, Trolle E, Willis SK, McCann SE, et al. Dietary phytoestrogen intakes of adult women are not strongly related to fecundability in 2 preconception cohort studies. J Nutr. (2020) 150:1240–51. doi: 10.1093/jn/nxz335
42. Unfer V, Casini ML, Gerli S, Costabile L, Mignosa M, Di Renzo GC. Phytoestrogens may improve the pregnancy rate in in vitro fertilization-embryo transfer cycles: a prospective, controlled, randomized trial. Fertil Steril. (2004) 82:1509–13. doi: 10.1016/j.fertnstert.2004.07.934
43. Becker GF, Passos EP, Moulin CC. Short-term effects of a hypocaloric diet with low glycemic index and low glycemic load on body adiposity, metabolic variables, ghrelin, leptin, and pregnancy rate in overweight and obese infertile women: a randomized controlled trial. Am J Clin Nutr. (2015) 102:1365–72. doi: 10.3945/ajcn.115.117200
44. Noli SA, Ferrari S, Ricci E, Reschini M, Cipriani S, Dallagiovanna C, et al. The role of diet in unexpected poor response to ovarian stimulation: a cross-sectional study. Reprod Biomed Online. (2020) 41:874–83. doi: 10.1016/j.rbmo.2020.07.011
45. Noli SA, Ricci E, Cipriani S, Ferrari S, Castiglioni M, La Vecchia I, et al. Dietary carbohydrate intake, dietary glycemic load and outcomes of in vitro fertilization: findings from an observational Italian cohort study. Nutrients. (2020) 12:1568. doi: 10.3390/nu12061568
46. Moran LJ, Tsagareli V, Noakes M, Norman R. Altered preconception fatty acid intake is associated with improved pregnancy rates in overweight and obese women undertaking in vitro fertilisation. Nutrients. (2016) 8:10. doi: 10.3390/nu8010010
47. Chiu YH, Karmon AE, Gaskins AJ, Arvizu M, Williams PL, Souter I, et al. Serum omega-3 fatty acids and treatment outcomes among women undergoing assisted reproduction. Hum Reprod. (2018) 33:156–65. doi: 10.1093/humrep/dex335
48. Kermack AJ, Lowen P, Wellstead SJ, Fisk HL, Montag M, Cheong Y, et al. Effect of a 6-week “Mediterranean” dietary intervention on in vitro human embryo development: the Preconception Dietary Supplements in Assisted Reproduction double-blinded randomized controlled trial. Fertil Steril. (2020) 113:260–9. doi: 10.1016/j.fertnstert.2019.09.041
49. Jungheim ES, Macones GA, Odem RR, Patterson BW, Moley KH. Elevated serum α-linolenic acid levels are associated with decreased chance of pregnancy after in vitro fertilization. Fertil Steril. (2011) 96:880–3. doi: 10.1016/j.fertnstert.2011.07.1115
50. Jungheim ES, Macones GA, Odem RR, Patterson BW, Lanzendorf SE, Ratts VS, et al. Associations between free fatty acids, cumulus oocyte complex morphology and ovarian function during in vitro fertilization. Fertil Steril. (2011) 95:1970–4. doi: 10.1016/j.fertnstert.2011.01.154
51. Eskew AM, Wormer KC, Matthews ML, Norton HJ, Papadakis MA, Hurst BS. The association between fatty acid index and in vitro fertilization outcomes. J Assist Reprod Genet. (2017) 34:1627–32. doi: 10.1007/s10815-017-1032-1
52. Gaskins AJ, Nassan FL, Chiu Y-H, Arvizu M, Williams PL, Keller MG, et al. Dietary patterns and outcomes of assisted reproduction. Am J Obstet Gynecol. (2019) 220:567.e1–.e18. doi: 10.1016/j.ajog.2019.02.004
53. Szymański W, Kazdepka-Ziemińska A. [Effect of homocysteine concentration in follicular fluid on a degree of oocyte maturity]. Ginekol Pol. (2003) 74:1392–6.
54. Ozkan S, Jindal S, Greenseid K, Shu J, Zeitlian G, Hickmon C, et al. Replete vitamin D stores predict reproductive success following in vitro fertilization. Fertil Steril. (2010) 94:1314–9. doi: 10.1016/j.fertnstert.2009.05.019
55. Garbedian K, Boggild M, Moody J, Liu KE. Effect of vitamin D status on clinical pregnancy rates following in vitro fertilization. CMAJ Open. (2013) 1:E77–82. doi: 10.9778/cmajo.20120032
56. Rudick BJ, Ingles SA, Chung K, Stanczyk FZ, Paulson RJ, Bendikson KA. Influence of vitamin D levels on in vitro fertilization outcomes in donor-recipient cycles. Fertil Steril. (2014) 101:447–52. doi: 10.1016/j.fertnstert.2013.10.008
57. Polyzos NP, Anckaert E, Guzman L, Schiettecatte J, Van Landuyt L, Camus M, et al. Vitamin D deficiency and pregnancy rates in women undergoing single embryo, blastocyst stage, transfer (SET) for IVF/ICSI. Hum Reprod. (2014) 29:2032–40. doi: 10.1093/humrep/deu156
58. Aflatoonian A, Arabjahvani F, Eftekhar M, Sayadi M. Effect of vitamin D insufficiency treatment on fertility outcomes in frozen-thawed embryo transfer cycles: a randomized clinical trial. Iran J Reprod Med. (2014) 12:595–600. doi: 10.18502/ijrm.v12i9.582
59. Aleyasin A, Hosseini MA, Mahdavi A, Safdarian L, Fallahi P, Mohajeri MR, et al. Predictive value of the level of vitamin D in follicular fluid on the outcome of assisted reproductive technology. Eur J Obstet Gynecol Reprod Biol. (2011) 159:132–7. doi: 10.1016/j.ejogrb.2011.07.006
60. Neville G, Martyn F, Kilbane M, O'Riordan M, Wingfield M, McKenna M, et al. Vitamin D status and fertility outcomes during winter among couples undergoing in vitro fertilization/intracytoplasmic sperm injection. Int J Gynaecol Obstet. (2016) 135:172–6. doi: 10.1016/j.ijgo.2016.04.018
61. Sun N, Xu C, Zhang Q, Lu X, Li W. Impact of multivitamin supplementation on trace element levels in serum and follicular fluid of women undergoing in vitro fertilisation. J Develop Med. (2013) 1:74–7.
62. Ingle ME, Bloom MS, Parsons PJ, Steuerwald AJ, Kruger P, Fujimoto VY. Associations between IVF outcomes and essential trace elements measured in follicular fluid and urine: a pilot study. J Assist Reprod Genet. (2017) 34:253–61. doi: 10.1007/s10815-016-0853-7
63. Özkaya MO, Naziroglu M, Barak C, Berkkanoglu M. Effects of multivitamin/mineral supplementation on trace element levels in serum and follicular fluid of women undergoing in vitro fertilization (IVF). Biol Trace Elem Res. (2011) 139:1–9. doi: 10.1007/s12011-010-8637-x
64. Ng SC, Karunanithy R, Edirisinghe WR, Roy AC, Wong PC, Ratnam SS. Human follicular fluid levels of calcium, copper and zinc. Gynecol Obstet Invest. (1987) 23:129–32. doi: 10.1159/000298848
65. Vujkovic M, de Vries JH, Lindemans J, Macklon NS, van der Spek PJ, Steegers EA, et al. The preconception Mediterranean dietary pattern in couples undergoing in vitro fertilization/intracytoplasmic sperm injection treatment increases the chance of pregnancy. Fertil Steril. (2010) 94:2096–101. doi: 10.1016/j.fertnstert.2009.12.079
66. Karayiannis D, Kontogianni MD, Mendorou C, Mastrominas M, Yiannakouris N. Adherence to the Mediterranean diet and IVF success rate among non-obese women attempting fertility. Hum Reprod. (2018) 33:494–502. doi: 10.1093/humrep/dey003
67. Sun H, Lin Y, Lin D, Zou C, Zou X, Fu L, et al. Mediterranean diet improves embryo yield in IVF: a prospective cohort study. Rep Biol Endocrinol. (2019) 17:73. doi: 10.1186/s12958-019-0520-9
68. Ruder EH, Hartman TJ, Reindollar RH, Goldman MB. Female dietary antioxidant intake and time to pregnancy among couples treated for unexplained infertility. Fertil Steril. (2014) 101:759–66. doi: 10.1016/j.fertnstert.2013.11.008
69. Ricci E, Bravi F, Noli S, Somigliana E, Cipriani S, Castiglioni M, et al. Mediterranean diet and outcomes of assisted reproduction: an Italian cohort study. Am J Obstet Gynecol. (2019) 221:627.e1–14. doi: 10.1016/j.ajog.2019.07.011
70. Ravisankar S, Ting AY, Murphy MJ, Redmayne N, Wang D, McArthur CA, et al. Short-term Western-style diet negatively impacts reproductive outcomes in primates. JCI Insight. (2021) 6:e138312. doi: 10.1172/jci.insight.138312
71. Hohos NM, Elliott EM, Cho KJ, Lin IS, Rudolph MC, Skaznik-Wikiel ME. High-fat diet-induced dysregulation of ovarian gene expression is restored with chronic omega-3 fatty acid supplementation. Mol Cell Endocrinol. (2020) 499:110615. doi: 10.1016/j.mce.2019.110615
72. Hohos NM, Cho KJ, Swindle DC, Skaznik-Wikiel ME. High-fat diet exposure, regardless of induction of obesity, is associated with altered expression of genes critical to normal ovulatory function. Mol Cell Endocrinol. (2018) 470:199–207. doi: 10.1016/j.mce.2017.10.016
73. Ruebel ML, Cotter M, Sims CR, Moutos DM, Badger TM, Cleves MA, et al. Obesity modulates inflammation and lipid metabolism oocyte gene expression: a single-cell transcriptome perspective. J Clin Endocrinol Metab. (2017) 102:2029–38. doi: 10.1210/jc.2016-3524
74. Brookheart RT, Swearingen AR, Collins CA, Cline LM, Duncan JG. High-sucrose-induced maternal obesity disrupts ovarian function and decreases fertility in Drosophila melanogaster. Biochim Biophysica Acta Mol Basis Dis. (2017) 1863:1255–63. doi: 10.1016/j.bbadis.2017.03.014
75. Sangalli JR, Sampaio RV, Del Collado M, da Silveira JC, De Bem THC, Perecin F, et al. Metabolic gene expression and epigenetic effects of the ketone body β-hydroxybutyrate on H3K9ac in bovine cells, oocytes and embryos. Sci Rep. (2018) 8:17219. doi: 10.1038/s41598-018-35527-9
76. Harreiter J, Simmons D, Desoye G, Corcoy R, Adelantado JM, Devlieger R, et al. Nutritional lifestyle intervention in obese pregnant women, including lower carbohydrate intake, is associated with increased maternal free fatty acids, 3-β-Hydroxybutyrate, and fasting glucose concentrations: a secondary factorial analysis of the European multicenter, randomized controlled DALI lifestyle intervention trial. Diabetes Care. (2019) 42:1380–9. doi: 10.2337/dc19-0418
77. Nagy RA, Homminga I, Jia C, Liu F, Anderson JLC, Hoek A, et al. Trimethylamine-N-oxide is present in human follicular fluid and is a negative predictor of embryo quality. Hum Reprod. (2020) 35:81–8. doi: 10.1093/humrep/dez224
78. Komiya S, Naito Y, Okada H, Matsuo Y, Hirota K, Takagi T, et al. Characterizing the gut microbiota in females with infertility and preliminary results of a water-soluble dietary fiber intervention study. J Clin Biochem Nutr. (2020) 67:105–11. doi: 10.3164/jcbn.20-53
79. Dastorani M, Aghadavod E, Mirhosseini N, Foroozanfard F, Zadeh Modarres S, Amiri Siavashani M, et al. The effects of vitamin D supplementation on metabolic profiles and gene expression of insulin and lipid metabolism in infertile polycystic ovary syndrome candidates for in vitro fertilization. Reprod Biol Endocrinol. (2018) 16:94. doi: 10.1186/s12958-018-0413-3
80. Makieva S, Reschini M, Ferrari S, Bonesi F, Polledri E, Fustinoni S, et al. Oral Vitamin D supplementation impacts gene expression in granulosa cells in women undergoing IVF. Hum Reprod. (2021) 36:130–44. doi: 10.1093/humrep/deaa262
81. Smith EM, Hoi JT, Eissenberg JC, Shoemaker JD, Neckameyer WS, Ilvarsonn AM, et al. Feeding Drosophila a biotin-deficient diet for multiple generations increases stress resistance and lifespan and alters gene expression and histone biotinylation patterns. J Nutr. (2007) 137:2006–12. doi: 10.1093/jn/137.9.2006
82. Ikeda S, Sugimoto M, Kume S. The RPMI-1640 vitamin mixture promotes bovine blastocyst development in vitro and downregulates gene expression of TXNIP with epigenetic modification of associated histones. J Dev Orig Health Dis. (2018) 9:87–94. doi: 10.1017/S2040174417000563
83. Nelen WLDM, Blom HJ, Thomas CMG, Steegers EAP, Boers GHJ, Eskes TKAB. Methylenetetrahydrofolate reductase polymorphism affects the change in homocysteine and folate concentrations resulting from low dose folic acid supplementation in women with unexplained recurrent miscarriages. J Nutr. (1998) 128:1336–41. doi: 10.1093/jn/128.8.1336
84. Rahimi S, Martel J, Karahan G, Angle C, Behan NA, Chan D, et al. Moderate maternal folic acid supplementation ameliorates adverse embryonic and epigenetic outcomes associated with assisted reproduction in a mouse model. Hum Reprod. (2019) 34:851–62. doi: 10.1093/humrep/dez036
85. Heidar Z, Hamzepour N, Zadeh Modarres S, Mirzamoradi M, Aghadavod E, Pourhanifeh MH, et al. The effects of selenium supplementation on clinical symptoms and gene expression related to inflammation and vascular endothelial growth factor in infertile women candidate for in vitro fertilization. Biol Trace Elem Res. (2020) 193:319–25. doi: 10.1007/s12011-019-01715-5
86. Zadeh Modarres S, Heidar Z, Foroozanfard F, Rahmati Z, Aghadavod E, Asemi Z. The effects of selenium supplementation on gene expression related to insulin and lipid in infertile polycystic ovary syndrome women candidate for in vitro fertilization: a randomized, double-blind, placebo-controlled trial. Biol Trace Elem Res. (2018) 183:218–25. doi: 10.1007/s12011-017-1148-2
87. Tian X, Diaz FJ. Acute dietary zinc deficiency before conception compromises oocyte epigenetic programming and disrupts embryonic development. Dev Biol. (2013) 376:51–61. doi: 10.1016/j.ydbio.2013.01.015
88. Gannon MC, Nuttall FQ, Neil BJ, Westphal SA. The insulin and glucose responses to meals of glucose plus various proteins in type II diabetic subjects. Metabolism. (1988) 37:1081–8. doi: 10.1016/0026-0495(88)90072-8
89. Hubbard R, Kosch CL, Sanchez A, Sabate J, Berk L, Shavlik G. Effect of dietary protein on serum insulin and glucagon levels in hyper- and normocholesterolemic men. Atherosclerosis. (1989) 76:55–61. doi: 10.1016/0021-9150(89)90193-7
90. Holmes MD, Pollak MN, Willett WC, Hankinson SE. Dietary correlates of plasma insulin-like growth factor I and insulin-like growth factor binding protein 3 concentrations. Cancer Epidemiol Biomarkers Prev. (2002) 11:852–61.
91. Meza-Herrera CA, Hallford DM, Ortiz JA, Cuevas RA, Sanchez JM, Salinas H, et al. Body condition and protein supplementation positively affect periovulatory ovarian activity by non LH-mediated pathways in goats. Anim Reprod Sci. (2008) 106:412–20. doi: 10.1016/j.anireprosci.2007.06.004
92. Armstrong DG, McEvoy TG, Baxter G, Robinson JJ, Hogg CO, Woad KJ, et al. Effect of dietary energy and protein on bovine follicular dynamics and embryo production in vitro: associations with the ovarian insulin-like growth factor system. Biol Reprod. (2001) 64:1624–32. doi: 10.1095/biolreprod64.6.1624
93. Jeong SH, Kang D, Lim MW, Kang CS, Sung HJ. Risk assessment of growth hormones and antimicrobial residues in meat. Toxicol Res. (2010) 26:301–13. doi: 10.5487/TR.2010.26.4.301
94. Vandermeersch G, Lourenço HM, Alvarez-Muñoz D, Cunha S, Diogène J, Cano-Sancho G, et al. Environmental contaminants of emerging concern in seafood–European database on contaminant levels. Environ Res. (2015) 143:29–45. doi: 10.1016/j.envres.2015.06.011
95. Seppen J. A diet containing the soy phytoestrogen genistein causes infertility in female rats partially deficient in UDP glucuronyltransferase. Toxicol Appl Pharmacol. (2012) 264:335–42. doi: 10.1016/j.taap.2012.09.013
96. Setchell KD, Gosselin SJ, Welsh MB, Johnston JO, Balistreri WF, Kramer LW, et al. Dietary estrogens–a probable cause of infertility and liver disease in captive cheetahs. Gastroenterology. (1987) 93:225–33. doi: 10.1016/0016-5085(87)91006-7
97. Bakaloudi DR, Halloran A, Rippin HL, Oikonomidou AC, Dardavesis TI, Williams J, et al. Intake and adequacy of the vegan diet. A systematic review of the evidence. Clin Nutr. (2021) 40:3503–21. doi: 10.1016/j.clnu.2020.11.035
98. Benham AJ, Gallegos D, Hanna KL, Hannan-Jones MT. Intake of vitamin B12 and other characteristics of women of reproductive age on a vegan diet in Australia. Public Health Nutr. (2021) 24:4397–407. doi: 10.1017/S1368980021001695
99. McGrice M, Porter J. The effect of low carbohydrate diets on fertility hormones and outcomes in overweight and obese women: a systematic review. Nutrients. (2017) 9:204. doi: 10.3390/nu9030204
100. Augustin LS, Kendall CW, Jenkins DJ, Willett WC, Astrup A, Barclay AW, et al. Glycemic index, glycemic load and glycemic response: an International Scientific Consensus Summit from the International Carbohydrate Quality Consortium (ICQC). Nutr Metab Cardiovasc Dis. (2015) 25:795–815. doi: 10.1016/j.numecd.2015.05.005
101. Kaaks R, Lukanova A. Energy balance and cancer: the role of insulin and insulin-like growth factor-I. Proc Nutr Soc. (2001) 60:91–106. doi: 10.1079/PNS200070
102. Dunaif A. Insulin resistance and the polycystic ovary syndrome: mechanism and implications for pathogenesis. Endocr Rev. (1997) 18:774–800. doi: 10.1210/er.18.6.774
103. Chavarro JE, Rich-Edwards JW, Rosner B, Willett WC. A prospective study of dairy foods intake and anovulatory infertility. Hum Reprod. (2007) 22:1340–7. doi: 10.1093/humrep/dem019
104. Sadowska J, Dudzińska W, Skotnicka E, Sielatycka K, Daniel I. The impact of a diet containing sucrose and systematically repeated starvation on the oxidative status of the uterus and ovary of rats. Nutrients. (2019) 11:1544. doi: 10.3390/nu11071544
105. Makki K, Deehan EC, Walter J, Bäckhed F. The impact of dietary fiber on gut microbiota in host health and disease. Cell Host Microbe. (2018) 23:705–15. doi: 10.1016/j.chom.2018.05.012
106. Sturmey RG, Reis A, Leese HJ, McEvoy TG. Role of fatty acids in energy provision during oocyte maturation and early embryo development. Reprod Domest Anim. (2009) 44:50–8. doi: 10.1111/j.1439-0531.2009.01402.x
107. Norwitz ER, Schust DJ, Fisher SJ. Implantation and the survival of early pregnancy. N Engl J Med. (2001) 345:1400–8. doi: 10.1056/NEJMra000763
108. Silvestris E, Lovero D, Palmirotta R. Nutrition and female fertility: an interdependent correlation. Front Endocrinol. (2019) 10:346. doi: 10.3389/fendo.2019.00346
109. Hughes J, Kwong WY, Li D, Salter AM, Lea RG, Sinclair KD. Effects of omega-3 and−6 polyunsaturated fatty acids on ovine follicular cell steroidogenesis, embryo development and molecular markers of fatty acid metabolism. Reproduction. (2011) 141:105–18. doi: 10.1530/REP-10-0337
110. Kim PY, Zhong M, Kim YS, Sanborn BM, Allen KG. Long chain polyunsaturated fatty acids alter oxytocin signaling and receptor density in cultured pregnant human myometrial smooth muscle cells. PLoS ONE. (2012) 7:e41708. doi: 10.1371/journal.pone.0041708
111. Wonnacott KE, Kwong WY, Hughes J, Salter AM, Lea RG, Garnsworthy PC, et al. Dietary omega-3 and−6 polyunsaturated fatty acids affect the composition and development of sheep granulosa cells, oocytes and embryos. Reproduction. (2010) 139:57–69. doi: 10.1530/REP-09-0219
112. Wathes DC, Abayasekara DR, Aitken RJ. Polyunsaturated fatty acids in male and female reproduction. Biol Reprod. (2007) 77:190–201. doi: 10.1095/biolreprod.107.060558
113. Abayasekara DR, Wathes DC. Effects of altering dietary fatty acid composition on prostaglandin synthesis and fertility. Prostaglandins Leukot Essent Fatty Acids. (1999) 61:275–87. doi: 10.1054/plef.1999.0101
114. Belani M, Purohit N, Pillai P, Gupta S, Gupta S. Modulation of steroidogenic pathway in rat granulosa cells with subclinical Cd exposure and insulin resistance: an impact on female fertility. Biomed Res Int. (2014) 2014:460251. doi: 10.1155/2014/460251
115. Saravanan N, Haseeb A, Ehtesham NZ, Ghafoorunissa. Differential effects of dietary saturated and trans-fatty acids on expression of genes associated with insulin sensitivity in rat adipose tissue. Eur J Endocrinol. (2005) 153:159–65. doi: 10.1530/eje.1.01946
116. Komar CM, Braissant O, Wahli W, Curry TE Jr. Expression and localization of PPARs in the rat ovary during follicular development and the periovulatory period. Endocrinology. (2001) 142:4831–8. doi: 10.1210/endo.142.11.8429
117. Kurzynska A, Bogacki M, Chojnowska K, Bogacka I. Peroxisome proliferator activated receptor ligands affect progesterone and 17β-estradiol secretion by porcine corpus luteum during early pregnancy. J Physiol Pharmacol. (2014) 65:709–17.
118. Banerjee J, Komar CM. Effects of luteinizing hormone on peroxisome proliferator-activated receptor gamma in the rat ovary before and after the gonadotropin surge. Reproduction. (2006) 131:93–101. doi: 10.1530/rep.1.00730
119. Schaefer E, Nock D. The impact of preconceptional multiple-micronutrient supplementation on female fertility. Clin Med Insights Womens Health. (2019) 12:1179562X19843868. doi: 10.1177/1179562X19843868
120. Ebisch IM, Thomas CM, Peters WH, Braat DD, Steegers-Theunissen RP. The importance of folate, zinc and antioxidants in the pathogenesis and prevention of subfertility. Hum Reprod Update. (2007) 13:163–74. doi: 10.1093/humupd/dml054
121. Parikh G, Varadinova M, Suwandhi P, Araki T, Rosenwaks Z, Poretsky L, et al. Vitamin D regulates steroidogenesis and insulin-like growth factor binding protein-1 (IGFBP-1) production in human ovarian cells. Horm Metab Res. (2010) 42:754–7. doi: 10.1055/s-0030-1262837
122. Agic A, Xu H, Altgassen C, Noack F, Wolfler MM, Diedrich K, et al. Relative expression of 1,25-dihydroxyvitamin D3 receptor, vitamin D 1 alpha-hydroxylase, vitamin D 24-hydroxylase, and vitamin D 25-hydroxylase in endometriosis and gynecologic cancers. Reprod Sci. (2007) 14:486–97. doi: 10.1177/1933719107304565
123. Tanamura A, Nomura S, Kurauchi O, Furui T, Mizutani S, Tomoda Y. Purification and characterization of 1,25(OH)2D3 receptor from human placenta. J Obstet Gynaecol. (1995) 21:631–9. doi: 10.1111/j.1447-0756.1995.tb00923.x
124. Hu FB. Dietary pattern analysis: a new direction in nutritional epidemiology. Curr Opin Lipidol. (2002) 13:3–9. doi: 10.1097/00041433-200202000-00002
125. Chiu Y-H, Williams PL, Gillman MW, Gaskins AJ, Mínguez-Alarcón L, Souter I, et al. Association between pesticide residue intake from consumption of fruits and vegetables and pregnancy outcomes among women undergoing infertility treatment with assisted reproductive technology. JAMA Intern Med. (2018) 178:17–26. doi: 10.1001/jamainternmed.2017.5038
126. Hood RB, Liang D, Chiu Y-H, Sandoval-Insausti H, Chavarro JE, Jones D, et al. Pesticide residue intake from fruits and vegetables and alterations in the serum metabolome of women undergoing infertility treatment. Environ Int. (2022) 160:107061. doi: 10.1016/j.envint.2021.107061
127. Nazni P. Association of western diet & lifestyle with decreased fertility. Indian J Med Res. (2014) 140:S78–81.
128. Uribarri J, Woodruff S, Goodman S, Cai W, Chen X, Pyzik R, et al. Advanced glycation end products in foods and a practical guide to their reduction in the diet. J Am Diet Assoc. (2010) 110:911–16.e12. doi: 10.1016/j.jada.2010.03.018
129. Jinno M, Takeuchi M, Watanabe A, Teruya K, Hirohama J, Eguchi N, et al. Advanced glycation end-products accumulation compromises embryonic development and achievement of pregnancy by assisted reproductive technology. Hum Reprod. (2011) 26:604–10. doi: 10.1093/humrep/deq388
130. Thomas M, Baynes J, Thorpe S, Cooper M. The role of AGEs and AGE inhibitors in diabetic cardiovascular disease. Curr Drug Targets. (2005) 6:453–74. doi: 10.2174/1389450054021873
131. Gaskins AJ, Chiu YH, Williams PL, Keller MG, Toth TL, Hauser R, et al. Maternal whole grain intake and outcomes of in vitro fertilization. Fertil Steril. (2016) 105:1503–10.e4. doi: 10.1016/j.fertnstert.2016.02.015
132. Mirabi P, Chaichi MJ, Esmaeilzadeh S, Ali Jorsaraei SG, Bijani A, Ehsani M, et al. The role of fatty acids on ICSI outcomes: a prospective cohort study. Lipids Health Dis. (2017) 16:18. doi: 10.1186/s12944-016-0396-z
133. Simopoulos AP. Genetic variants in the metabolism of omega-6 and omega-3 fatty acids: their role in the determination of nutritional requirements and chronic disease risk. Exp Biol Med. (2010) 235:785–95. doi: 10.1258/ebm.2010.009298
134. Chu J, Gallos I, Tobias A, Tan B, Eapen A, Coomarasamy A. Vitamin D and assisted reproductive treatment outcome: a systematic review and meta-analysis. Hum Reprod. (2018) 33:65–80. doi: 10.1093/humrep/dex326
135. Voulgaris N, Papanastasiou L, Piaditis G, Angelousi A, Kaltsas G, Mastorakos G, et al. Vitamin D and aspects of female fertility. Hormones. (2017) 16:5–21. doi: 10.14310/horm.2002.1715
136. Twigt JM, Bolhuis MEC, Steegers EAP, Hammiche F, van Inzen WG, Laven JSE, et al. The preconception diet is associated with the chance of ongoing pregnancy in women undergoing IVF/ICSI treatment. Hum Reprod. (2012) 27:2526–31. doi: 10.1093/humrep/des157
137. Hammiche F, Laven JS, van Mil N, de Cock M, de Vries JH, Lindemans J, et al. Tailored preconceptional dietary and lifestyle counselling in a tertiary outpatient clinic in The Netherlands. Hum Reprod. (2011) 26:2432–41. doi: 10.1093/humrep/der225
138. Mena GP, Mielke GI, Brown WJ. The effect of physical activity on reproductive health outcomes in young women: a systematic review and meta-analysis. Hum Reprod Update. (2019) 25:542–64. doi: 10.1093/humupd/dmz013
139. Hakimi O, Cameron LC. Effect of exercise on ovulation: a systematic review. Sports Med. (2017) 47:1555–67. doi: 10.1007/s40279-016-0669-8
140. Garruti G, Depalo R, De Angelis M. Weighing the impact of diet and lifestyle on female reproductive function. Curr Med Chem. (2019) 26:3584–92. doi: 10.2174/0929867324666170518101008
141. Orio F, Muscogiuri G, Ascione A, Marciano F, Volpe A, La Sala G, et al. Effects of physical exercise on the female reproductive system. Minerva Endocrinol. (2013) 38:305–19.
142. Müller M, Kersten S. Nutrigenomics: goals and strategies. Nat Rev Genet. (2003) 4:315–22. doi: 10.1038/nrg1047
143. Bouchard C, Ordovas JM. Fundamentals of nutrigenetics and nutrigenomics. Prog Mol Biol Transl Sci. (2012) 108:1–15. doi: 10.1016/B978-0-12-398397-8.00001-0
144. Dawson KA. Nutrigenomics: Feeding the genes for improved fertility. Anim Reprod Sci. (2006) 96:312–22. doi: 10.1016/j.anireprosci.2006.08.009
145. Patel B, Parets S, Akana M, Kellogg G, Jansen M, Chang C, et al. Comprehensive genetic testing for female and male infertility using next-generation sequencing. J Assist Reprod Genet. (2018) 35:1489–96. doi: 10.1007/s10815-018-1204-7
146. Mallepaly R, Butler PR, Herati AS, Lamb DJ. Genetic basis of male and female infertility. Genet Hum Infertil. (2017) 21:1–16. doi: 10.1159/000477275
147. Stover PJ. Influence of human genetic variation on nutritional requirements. Am J Clin Nutr. (2006) 83:436S−42S. doi: 10.1093/ajcn/83.2.436S
148. Reyes-Engel A, Muñoz E, Gaitan MJ, Fabre E, Gallo M, Dieguez JL, et al. Implications on human fertility of the 677C–>T and 1298A–>C polymorphisms of the MTHFR gene: consequences of a possible genetic selection. Mol Hum Reprod. (2002) 8:952–7. doi: 10.1093/molehr/8.10.952
149. Laanpere M, Altmäe S, Kaart T, Stavreus-Evers A, Nilsson TK, Salumets A. Folate-metabolizing gene variants and pregnancy outcome of IVF. Reprod Biomed Online. (2011) 22:603–14. doi: 10.1016/j.rbmo.2011.03.002
150. Shahrokhi SZ, Kazerouni F, Ghaffari F, Rahimipour A, Omrani MD, Arabipoor A, et al. The relationship between the MTHFR C677T genotypes to serum anti-müllerian hormone concentrations and in vitro fertilization/intracytoplasmic sperm injection outcome. Clin Lab. (2017) 63:927–34. doi: 10.7754/Clin.Lab.2016.161104
151. Servy EJ, Jacquesson-Fournols L, Cohen M, Menezo YJR. MTHFR isoform carriers. 5-MTHF (5-methyl tetrahydrofolate) vs folic acid: a key to pregnancy outcome: a case series. J Assist Reprod Genet. (2018) 35:1431–5. doi: 10.1007/s10815-018-1225-2
152. Beerda B, Wyszynska-Koko J, Te Pas MF, de Wit AA, Veerkamp RF. Expression profiles of genes regulating dairy cow fertility: recent findings, ongoing activities and future possibilities. Animal. (2008) 2:1158–67. doi: 10.1017/S1751731108002371
153. Irani M, Minkoff H, Seifer DB, Merhi Z. Vitamin D increases serum levels of the soluble receptor for advanced glycation end products in women with PCOS. J Clin Endocrinol Metab. (2014) 99:E886–90. doi: 10.1210/jc.2013-4374
154. Vannice G, Rasmussen H. Position of the academy of nutrition and dietetics: dietary fatty acids for healthy adults. J Acad Nutr Diet. (2014) 114:136–53. doi: 10.1016/j.jand.2013.11.001
155. Lu X, He Y, Zhu C, Wang H, Chen S, Lin HY. Twist1 is involved in trophoblast syncytialization by regulating GCM1. Placenta. (2016) 39:45–54. doi: 10.1016/j.placenta.2016.01.008
156. Dobrian AD. A tale with a Twist: a developmental gene with potential relevance for metabolic dysfunction and inflammation in adipose tissue. Front Endocrinol. (2012) 3:108–. doi: 10.3389/fendo.2012.00108
157. Oseikria M, Elis S, Maillard V, Corbin E, Uzbekova S. N-3 polyunsaturated fatty acid DHA during IVM affected oocyte developmental competence in cattle. Theriogenology. (2016) 85:1625–34.e2. doi: 10.1016/j.theriogenology.2016.01.019
158. Poston L, Caleyachetty R, Cnattingius S, Corvalán C, Uauy R, Herring S, et al. Preconceptional and maternal obesity: epidemiology and health consequences. Lancet Diabetes Endocrinol. (2016) 4:1025–36. doi: 10.1016/S2213-8587(16)30217-0
159. Yang H, Qazi IH, Pan B, Angel C, Guo S, Yang J, et al. Dietary selenium supplementation ameliorates female reproductive efficiency in aging mice. Antioxidants. (2019) 8:634. doi: 10.3390/antiox8120634
160. Lane M, Robker RL, Robertson SA. Parenting from before conception. Science. (2014) 345:756–60. doi: 10.1126/science.1254400
161. Jiménez-Chillarón JC, Díaz R, Martínez D, Pentinat T, Ramón-Krauel M, Ribó S, et al. The role of nutrition on epigenetic modifications and their implications on health. Biochimie. (2012) 94:2242–63. doi: 10.1016/j.biochi.2012.06.012
162. Franzago M, Santurbano D, Vitacolonna E, Stuppia L. Genes and diet in the prevention of chronic diseases in future generations. Int J Mol Sci. (2020) 21:2633. doi: 10.3390/ijms21072633
163. Zmora N, Suez J, Elinav E. You are what you eat: diet, health and the gut microbiota. Nat Rev Gastroenterol Hepatol. (2019) 16:35–56. doi: 10.1038/s41575-018-0061-2
164. Koeth RA, Levison BS, Culley MK, Buffa JA, Wang Z, Gregory JC, et al. γ-Butyrobetaine is a proatherogenic intermediate in gut microbial metabolism of L-carnitine to TMAO. Cell Metab. (2014) 20:799–812. doi: 10.1016/j.cmet.2014.10.006
165. Koedooder R, Singer M, Schoenmakers S, Savelkoul PHM, Morré SA, de Jonge JD, et al. The vaginal microbiome as a predictor for outcome of in vitro fertilization with or without intracytoplasmic sperm injection: a prospective study. Hum Reprod. (2019) 34:1042–54. doi: 10.1093/humrep/dez065
166. Sharma M, Dwivedi P, Singh Rawat A, Dwivedi AK. Nutrition nutraceuticals: a proactive approach for healthcare. Nutraceuticals. (2016) 4:79–116. doi: 10.1016/B978-0-12-804305-9.00003-8
167. Maiuolo J, Gliozzi M, Carresi C, Musolino V, Oppedisano F, Scarano F, et al. Nutraceuticals and cancer: potential for natural polyphenols. Nutrients. (2021) 13:3834. doi: 10.3390/nu13113834
168. Singh RK. “Chapter 10-nutraceuticals in reproductive and developmental disorders,” in Nutraceuticals, ed R. C. Gupta (Boston: Academic Press (2016). p. 123–34. doi: 10.1016/B978-0-12-802147-7.00010-3
169. López-Moreno A, Aguilera M. Probiotics dietary supplementation for modulating endocrine and fertility microbiota dysbiosis. Nutrients. (2020) 12:757. doi: 10.3390/nu12030757
170. Hill C, Guarner F, Reid G, Gibson GR, Merenstein DJ, Pot B, et al. Expert consensus document. The International Scientific Association for Probiotics and Prebiotics consensus statement on the scope and appropriate use of the term probiotic. Nat Rev Gastroenterol Hepatol. (2014) 11:506–14. doi: 10.1038/nrgastro.2014.66
171. Reid G, Beuerman D, Heinemann C, Bruce AW. Probiotic Lactobacillus dose required to restore and maintain a normal vaginal flora. FEMS Immunol Med Microbiol. (2001) 32:37–41. doi: 10.1111/j.1574-695X.2001.tb00531.x
172. Romero R, Hassan SS, Gajer P, Tarca AL, Fadrosh DW, Bieda J, et al. The vaginal microbiota of pregnant women who subsequently have spontaneous preterm labor and delivery and those with a normal delivery at term. Microbiome. (2014) 2:18. doi: 10.1186/2049-2618-2-18
173. Ravel J, Gajer P, Abdo Z, Schneider GM, Koenig SS, McCulle SL, et al. Vaginal microbiome of reproductive-age women. Proc Natl Acad Sci USA. (2011) 108:4680–7. doi: 10.1073/pnas.1002611107
174. Moreno I, Codoñer FM, Vilella F, Valbuena D, Martinez-Blanch JF, Jimenez-Almazán J, et al. Evidence that the endometrial microbiota has an effect on implantation success or failure. Am J Obstet Gynecol. (2016) 215:684–703. doi: 10.1016/j.ajog.2016.09.075
175. Azaïs-Braesco V, Bresson JL, Guarner F, Corthier G. Not all lactic acid bacteria are probiotics,.but some are. Br J Nutr. (2010) 103:1079–81. doi: 10.1017/S0007114510000723
176. Rijkers GT, Bengmark S, Enck P, Haller D, Herz U, Kalliomaki M, et al. Guidance for substantiating the evidence for beneficial effects of probiotics: current status and recommendations for future research. J Nutr. (2010) 140:671s−6s. doi: 10.3945/jn.109.113779
177. Slavin J. Fiber and prebiotics: mechanisms and health benefits. Nutrients. (2013) 5:1417–35. doi: 10.3390/nu5041417
178. Cho NA, Klancic T, Nettleton JE, Paul HA, Reimer RA. Impact of food ingredients (Aspartame, Stevia, Prebiotic Oligofructose) on fertility and reproductive outcomes in obese rats. Obesity. (2018) 26:1692–5. doi: 10.1002/oby.22325
179. Kobayashi T, Takano M, Kaneko K, Onoue M. A one-generation reproduction toxicity study in rats treated orally with a novel galacto-oligosaccharide. Hum Exp Toxicol. (2014) 33:814–21. doi: 10.1177/0960327113510328
180. Kyono K, Hashimoto T, Kikuchi S, Nagai Y, Sakuraba Y. A pilot study and case reports on endometrial microbiota and pregnancy outcome: an analysis using 16S rRNA gene sequencing among IVF patients, and trial therapeutic intervention for dysbiotic endometrium. Reprod Med Biol. (2018) 18:72–82. doi: 10.1002/rmb2.12105
Keywords: diet, fertility, female reproductive health, art, nutrigenetics, nutrigenomics, nutriepigenome, microbiome
Citation: Kohil A, Chouliaras S, Alabduljabbar S, Lakshmanan AP, Ahmed SH, Awwad J and Terranegra A (2022) Female infertility and diet, is there a role for a personalized nutritional approach in assisted reproductive technologies? A Narrative Review. Front. Nutr. 9:927972. doi: 10.3389/fnut.2022.927972
Received: 25 April 2022; Accepted: 27 June 2022;
Published: 22 July 2022.
Edited by:
Ahmed El-Sohemy, University of Toronto, CanadaReviewed by:
Zhengrong Zhou, Jiangsu University, ChinaBibiana Garcia-Bailo, University of Toronto, Canada
Copyright © 2022 Kohil, Chouliaras, Alabduljabbar, Lakshmanan, Ahmed, Awwad and Terranegra. This is an open-access article distributed under the terms of the Creative Commons Attribution License (CC BY). The use, distribution or reproduction in other forums is permitted, provided the original author(s) and the copyright owner(s) are credited and that the original publication in this journal is cited, in accordance with accepted academic practice. No use, distribution or reproduction is permitted which does not comply with these terms.
*Correspondence: Annalisa Terranegra, aterranegra@sidra.org
†These authors have contributed equally to this work and share first authorship