- 1Department of Clinical Pharmacy and Therapeutic, Applied Science Private University, Amman, Jordan
- 2Department of Pharmacy, Princess Sarvath Community College, Amman, Jordan
Colorectal cancer (CRC) is the third most common cancer and the second most deadly cancer worldwide. Nevertheless, more than 70% of CRC cases are resulted from sporadic tumorigenesis and are not inherited. Since adenoma-carcinoma development is a slow process and may take up to 20 years, diet-based chemoprevention could be an effective approach in sporadic CRC. The Mediterranean diet is an example of a healthy diet pattern that consists of a combination of nutraceuticals that prevent several chronic diseases and cancer. Many epidemiological studies have shown the correlation between adherence to the Mediterranean diet and low incidence of CRC. The goal of this review is to shed the light on the anti-inflammatory and anti-colorectal cancer potentials of the natural bioactive compounds derived from the main foods in the Mediterranean diet.
Introduction
The Mediterranean diet (MD) are one of the many studied and well-known dietary pattern worldwide, and it has been associated with a broad range of benefits for health as well. Besides, the MD appears as the best diet pattern to reflect many characteristics of an ideal healthy diet. The roots of the traditional MD pattern are seen in civilizations encircling the Mediterranean Sea; historically, some of the 22 countries bordering the Mediterranean Sea. So that this pattern has been closely associated with the social behaviors and lifestyles of that region (1). The traditional MD is arranged from a high intake of plant foods (fruits, vegetables, pieces of bread and other cereals, potatoes, beans, nuts, and seeds); or fresh fruits as a typical dessert, and olive oil as the vital source of fat, reaching to a low intake of foods like red meat and sweets containing sugars or honey. The health benefits of individual foods and components of the MD (e.g., extra-virgin olive oil and nuts) have been well-documented (Figure 1) (2, 3). The benefits of the MD are not due to exclusively one component, but it is the whole food pattern as well as the wide range of traditional cuisine and lifestyle (4). Many factors are associated with the positive outcomes of the MD, such as traditional cooking methods, fasting practices, unique recipes, and using home garden vegetables (5). As well, the MD has been subject to many changes that shaped the current form today, those changes including culture, religion, agriculture production, climatic conditions, poverty, and economy (5, 6). According to the United Nations Educational, Scientific and Cultural Organization (UNESCO), the MD is recognized as “a set of traditional practices, knowledge, and skills passed on from generation to generation and providing a sense of belonging and continuity to the concerned communities” (7). The most consistent and robust evidence for the health benefits of MD has been observed in cardiovascular conditions, type 2 diabetes, metabolic syndrome, obesity, cancer, cognitive decline, and many others (8, 9). In observational studies, higher adherence to the MD was inversely associated with different types of cancer, including breast, colorectal, head, neck, respiratory tract, bladder, and liver (10).
Colorectal cancer (CRC) is the third most familiar malignancy and the second most deadly cancer. It has been reported about 1.9 million incidence cases and 0.9 million deaths worldwide in the year 2020 (11). There is evidence of higher CRC risk in Westernized society whose behaviors are characterized by a more elevated consumption of red and processed meat than in people living along the Mediterranean coast, who have a decreased overall cancer mortality correlated to their eating habits such as MD (12). Chronic intestinal inflammation, such as Crohn’s disease and ulcerative colitis, is predisposed to CRC (13). In addition, the upregulation of proinflammatory factors such as cyclooxygenase-2 is observed in inflammatory bowel disease-related CRC. The capacity for the MD to prevent CRC is likely due in part to the total anti-inflammatory effects exerted by the diverse food components that contribute to this dietary pattern, specifically those foods and beverages contributing a significant load of phenolic compounds (i.e., olive and fish oil, and plant-based foods) (14).
This review aims to summarize the most recent clinical and preclinical studies of the main micronutrients included in the components of the MD and to verify the correlation between their anti-inflammatory, gut microbiome modulation, and chemopreventive effects in CRC.
Factors associated with the development of colorectal cancer
Impact of genetic abnormalities on colorectal cancer initiation and progression
Colorectal cancer can be promoted when intestinal epithelial cells are exposed to different genetic and epigenetic modifications that make them hyperproliferative (15). There are several distinct molecular pathways that modulate the progression of adenoma-carcinoma sequences, including chromosomal instability, microsatellite instability, and CpG island methylation (15–18). Hence, chromosomal abnormalities may associate with mutations that occur in particular oncogenes or tumor suppressor genes like APC, KRAS, PIK3CA, BRAF, SMAD4, or TP53 (19). Besides, when mutations happened in DNA mismatch repair genes this is known as microsatellite instability which is found in 10–15% of sporadic CRCs (20). Moreover, DNA CpG methylation is involved in the early stage of CRC development (21) and associated with BRAF and KRAS mutations as well as MLH1 methylation (22). CRC development begins from normal cells changed to hyperplastic polyp and then converted to sessile serrated adenomas ending up with cancer (Figure 2) (23). As a result, CRC is classified into five stages: stage 0 (benign polyp), stage I (tumor invades the muscularis propria), stage II (tumor invades tissue in the serosa), stage III (involved of visceral peritoneum), and stage IV (metastasis) (Figure 2) (24).
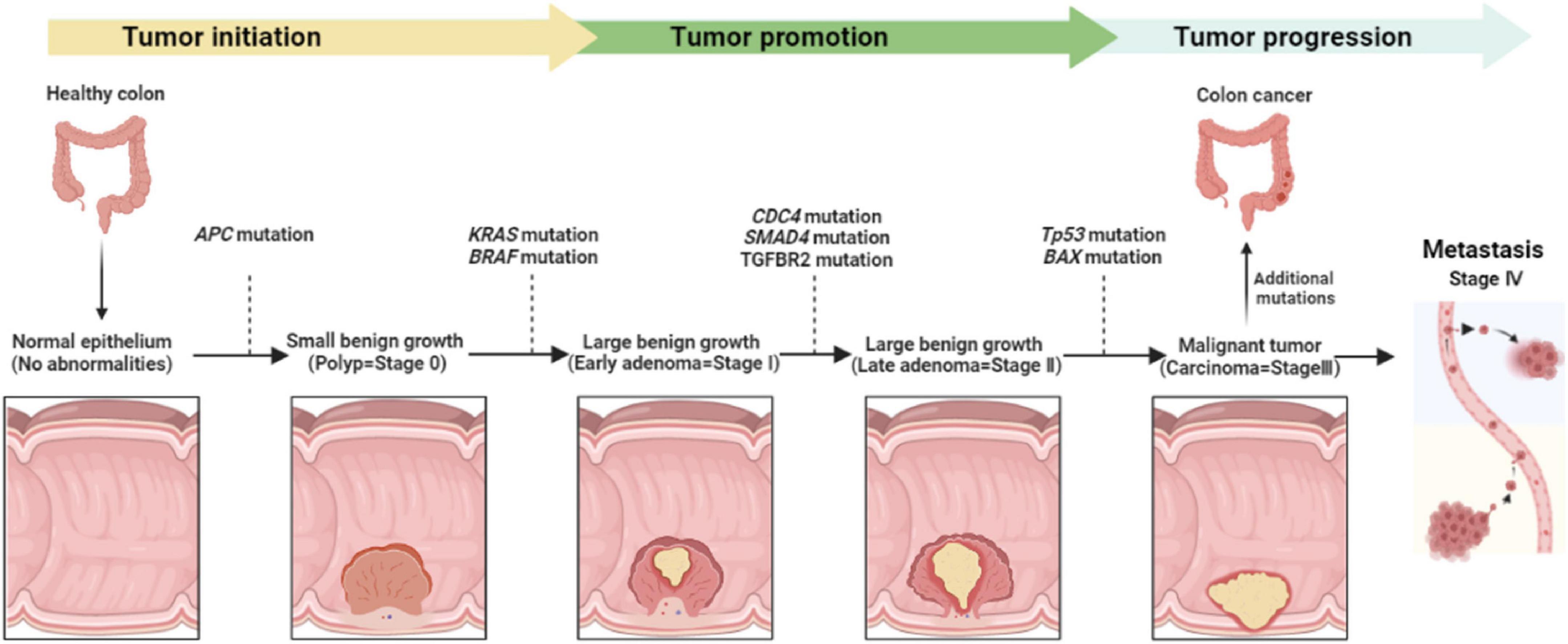
Figure 2. Colorectal cancer development, stages, and the main genetic modifications all along tumor progression.
Colorectal cancer and inflammation
In humans, up to 20% of all cancers result from chronic inflammation and persistent infections (25). CRC can be categorized as either sporadic, with inflammation following cancer onset, or colitis-associated CRCs induced by chronic inflammation. Both inflammatory bowel diseases, ulcerative colitis, and Crohn’s disease have a clear correlation with a significantly increased CRC risk, indicating chronic inflammation’s role in carcinogenesis (26). CRC that comes from inflammatory bowel disease (IBD) is responsible for about 2% of CRC mortality yearly. Despite optimal medical treatment, the chronic inflammatory condition associated with IBD raises the chance for high-grade dysplasia and CRC, along with the effect of the genetic and environmental risk factors and the microbiome (27).
Several studies have demonstrated the strong correlation between chronic inflammation and tumorigenesis. Chronic inflammation could be prompted by: infections (viruses and bacteria), environmental factors (smoking and pollution), dietary factors, stress, and obesity (28). Besides, inflammation plays a role in tumor development, which mediates epigenetic alteration and modulation of oncogenes expression, DNA damage induced by oxidative stress and mutagens, as well as unrestricted tissue regeneration and proliferation (16, 29). In particular, chronic intestinal inflammation triggered different signaling pathways that augment tumor initiation and progression in CRC (Figure 3) (16). On the other hand, significant data have been associated between unbalanced gut microbiota and gastrointestinal tumorigenesis (30). The function of the intestinal epithelial barrier is affected by both chronic inflammation and microbial pathogens (31). They expanded gut permeability leading to ease in the translocation of microbial substances and stimulating an immune response (30, 31).
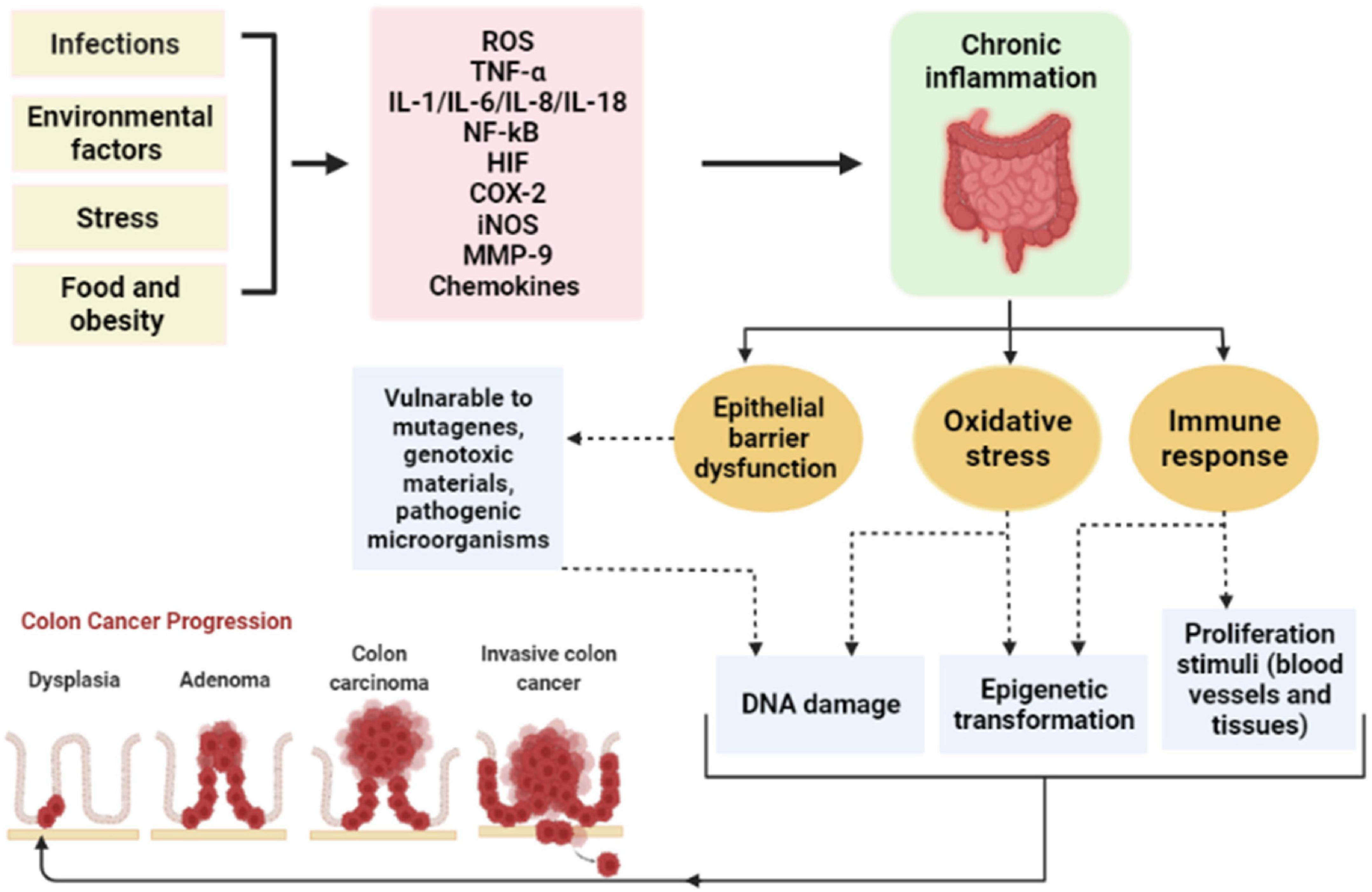
Figure 3. The correlation between chronic inflammation and the development of CRC. ROS, reactive oxygen species; TNF-α, tumor necrosis factor-alpha; IL, interleukin; NF-kB, nuclear factor kappa B; HIF, hypoxia-inducible factor; COX-2, cyclo-oxygenase-2; iNOS, inducible nitric oxide synthase; MMP-9, matrix metalloproteinases-9.
Colorectal cancer and microbiome
The gut microbiota is categorized into commensal and pathogenic bacteria. There are four distinct groups of bacteria found in the gut microbiota, including Firmicutes, Bacteroidetes, Actinobacteria, and Proteobacteria (32, 33). Healthy gut microbiota plays an essential role in keeping the immune system active and able to attack opportunistic bacteria through particular receptors (e.g., Toll-like receptors) or gut microbiota’s metabolites (e.g., short-chain fatty acid) (34). Dysbiosis is the state of an imbalanced microbiome which can lead to a wide range of digestive problems such as irritable bowel syndrome (IBD) and CRC. An unhealthy gut microbiome can initiate inflammation and modify several signaling pathways resulting in the carcinogenesis of CRC (35). However, there are specific bacterial strains associated with the development of CRC, including Fusobacterium nucleatum, Escherichia coli, and Bacteroides fragilis (32). Tumorigenesis effects of dysbiosis can be summarized as the following: the genotoxicity (DNA damaging and mutations) effect of some bacteria and their metabolites, disrupting the gut surface permeability, which may promote inflammation, and modulation of the immune response (16, 32). Several studies have shown that modulation of the gut microbiome can improve the prognosis, treatment, and prevention of CRC (32).
Selected components of the Mediterranean diet and their effect on reducing colorectal cancer risk
Extra virgin olive oil: Phenolic compounds
Olive oil (OO) is an essential component of the MD with high nutritional values due to the presence of various bioactive compounds (36). Simple phenols, fatty acids, flavonoids, lignans, hydrocarbons, triterpenes, and phytosterols are the main chemical compositions of olive oil (37). Moreover, extraction methods play a critical role in determining the natural nutrients in (OO). Thus, applying cold extraction methods will produce extra virgin olive oil (EVOO), which is recognized with high phenolic content and low free fatty acids (38, 39). Extra virgin olive oil is known for its protective effect on CRC, conquers intestinal inflammation, and improves gut microbiota (40–42).
Hydroxytyrosol (3,4-DHPEA) and its secoiridoid derivatives are the main polyphenolic compounds in EVOO, and it originated from the hydrolysis of oleuropein during the ripening of olives (43). Hydroxytyrosol (HT) is known for its diverse pharmacological effects, including antitumor, anti-inflammatory, immunomodulatory, antimicrobial, and neuroprotective potential (44–48). Recently, Hydroxytyrosol has been tested in mice subjected to dextran sulfate sodium (DSS)-induced colitis (49). The study revealed a high potency of (HT) in suppressing inflammation and alleviating colitis symptoms via downregulation of IL-6, IL-1β, TNF-α, and myeloperoxidase enzyme. Moreover, hydroxytyrosol was able to reduce NLRP3 inflammasome expression, and thus suppressed the expression of IL-18, IL-1β, and caspase-1 in DSS-induced ulcerative colitis (50). It also improved oxidative biomarkers and downregulated colon malondialdehyde, myeloperoxidase, and NO levels along with a significant reduction in mortality rate and disease activity index of albino rats with induced ulcerative colitis (51).
Besides the anti-inflammatory effects of (HT), it is also known to have anticancer properties and is involved in cancer hallmarks modification and tumor regression effect (52, 53). Hormozi et al. reported that (HT) induced apoptosis via upregulation of the caspase-3 gene and increased BAX/Bcl2 ratio in a human CRC cell line (LS180 cells) (54). It enhanced the expression of the antioxidant enzymes, which emphasized the antiproliferation effect (54, 55). As well, another anticancer mechanism of hydroxytyrosol was the inhibition of thioredoxin reductase 1(TrxR1) enzyme and promoted G1/S cell cycle arrest (48). It is noteworthy that a high level of (TrxR1) enzyme has been detected in CRC cells, which correlated with poor prognosis and chemotherapy response (56, 57). Moreover, hydroxytyrosol repressed the growth of human colorectal adenocarcinoma cells (HT-29) in both models in vitro and in vivo through downregulation of epidermal growth factor receptors (58). As well, it increased the expression of the CRC -associated-1 gene (COLACA1) in the same cell line leading to reduce tumorigenesis and an upraised survival rate (59).
Oleuropein is the ester form of hydroxytyrosol with β-glucosylated elenolic acid. It is found in olive leaves and EVOO with different content, and it gives olives a bitter and pungent taste (39, 60). Oleuropein has been involved in many pharmacological applications due to its properties, such as antioxidant, anti-inflammatory, and antineoplastic properties (61, 62). Oleuropein exhibited an anti-inflammatory effect via the suppression of inflammatory mediators, including NF-Kβ, COX-2, caspase-3, TNF-α, and Inos (61, 63, 64). Besides, it conquers inflammation by inhibiting of MAPK/NF-κB signaling pathway (65) and reduces the expression of IL-6, TNFR60, TNFR80, and ICAM-1 (66). In a recent study, Motawea et al. suggested that oleuropein was effective in reducing the following pro-inflammatory cytokines: IL-1β, TNF-α, IL-10, COX-2, iNOS, TGF-β1, MCP-1, and NF-κB in an induced colitis rat model (67). Besides, nanostructured lipid carrier-oleuropein was tested in the DSS-induced colitis experimental model and it exhibited a modulation of the inflammatory biomarkers via decreasing the level of TNF-α, IL-6, and hindering neutrophil infiltration (68). As well, oral intake of a diet supplemented with olive cream and probiotics revealed a synergistic anti-inflammatory effect in DSS-induced chronic colitis (69).
Previous studies also demonstrated the anticancer effect of oleuropein, including cell proliferation and migration inhibition, apoptosis induction, and growth signals modulation (39, 62, 70). Cárdeno et al. suggested that oleuropein significantly improved apoptotic mediators and decreased HIF-α expression in human CRC (HT-29 cells) (71). Besides, oleuropein repressed the activity of the main transcription proteins, including NF-κB, STAT3, PI3K/Akt, and β-catenin in AOM/DSS-induced CRC mice (72).
Tomato: Lycopene
Vegetables are consumed in the MD abundantly, and tomatoes are one of the universal MD components in the countries of the Mediterranean basin (73). Tomatoes are the edible fruits of the tomato plant (Solanum lycopersicum) that belongs to the Solanaceae family (74). The consumption of tomatoes has been related to a low incidence of chronic degenerative diseases, and various types of cancer (74, 75). These health benefits are expected to be associated with the presence of a wide range of phytochemicals, including carotenoids (lycopene and β-carotene), and polyphenolic compounds (flavonoids, flavanones, and flavones). Besides, high concentrations of other nutrients such as vitamin A, ascorbic acid, potassium, and folate have been reported in the chemical composition of tomatoes (73, 76). According to the chemical structure of the carotenoids, they are classified into carotenes (purely hydrocarbons such as lycopene and β-carotene) and xanthophylls (having oxygen in their structure such as lutein, zeaxanthin, and β-cryptoxanthin) (77, 78). Lycopene (LC) is a lipid-soluble pigment of natural carotenoids, which could be found in fresh tomatoes and processed tomato products (79, 80). Moreover, it is responsible for the red color of many fruits and vegetables like tomatoes, pink grapefruit, red guava, and watermelons (77). Many epidemiological studies have shown the biological activities of lycopene, including antioxidant, anti-inflammatory, cardioprotective, and anticancer effects (73, 81, 82).
From an anti-inflammatory perspective, lycopene was able to reduce the following inflammatory biomarkers: TNF-α, IL-1β, and IL-6 in the acetic acid-induced ulcerative colitis rat model (83). As well, it has significantly suppressed the level of NF-kB, TGF-β1, and caspase-3 along with upregulation of GSH expression and catalase activity in the same experimental model (84). A recent study has shown the effect of lycopene on colitis progression by lowering the disease activity index score, improving the colon length, and rising the expression of catalase, GSH-Px, and SOD (85).
On the other hand, several studies have described the antiproliferation activity of lycopene in CRC cells. Lin et al. have shown the chemo-preventive effect of lycopene in human colon cancer cells (HT-29). It downregulated the MMP-7 expression and hindered tumor development and tumor cell invasion (86). In a mouse xenograft model, LC decreased the expression of PCNA and β-catenin proteins, which are associated with tumor growth and progression. As well, lycopene attenuated the level of MMP-9 in tumor-bearing mice (87). MMPs are known to enhance the tumor microenvironment and promote cell invasion resulting in poor prognosis and low survival rate in CRC patients (88).
Herbs and spices
Medicinal plants have remained the primary source of medications; many of the pharmaceuticals that are now accessible were derived from them, either directly or indirectly. Many plants have been shown to have vital roles in the treatment and prevention of various illnesses in various regions of the globe (89, 90). The bioactive phytochemical elements of many plants have traditionally been employed in Asian medicine (91, 92). Health care services are provided by the traditional healer plants, which are founded on religious and cultural backgrounds, knowledge, attitudes, and beliefs (90, 91, 93). Recent years have seen a surge in interest in assessing plant foods and discovering phytochemicals with the potential to inhibit carcinogenesis.
Onion: Quercetin
Onions (Allium cepa) are members of the Liliaceae (94). The Liliaceae family has around 250 genera and 3700 species (95–97). Allium cepa is one of the world’s oldest and most frequently grown vegetables, growing in practically every climate zone, from tropical to cold temperate (98). Although A. cepa is referred to as the “Queen of the Kitchen,” it is distinguishable by the color of its outer scales (yellow, red, or white), its taste (sweet or bitter), and whether it is consumed fresh or powdered (99, 100).
Onion is roughly 90% water, with a significant concentration of nutritional fiber and carbohydrates. In terms of vitamins and minerals, onion has a low salt level while being rich in vitamin B6, vitamin C, folic acid, and minerals (Ca, Fe, S, Se, Mg, Ph, and k) (101–103). On the other hand, has a low lipid content and a pool of free amino acids (104, 105). Onions are high in a range of phytochemicals with beneficial properties, including organosulfur compounds, phenolic compounds, polysaccharides, and saponins (106–113). Sulfur compounds such as DATS, diallyl disulfide (DADS), ajoene, and sallylmercaptocysteine (SAMC), onionin A (114). Two flavonoid subgroups are abundant in onion: anthocyanins, which give certain kinds of their Reddish-purple color, and the primary pigments are flavonols, which include quercetin, which is found conjugated as quercetin 4′–O-glycopyranoside, quercetin 3,4′–O-diglycopyranoside, and quercetin 3,7,4′–O-triglycopyranoside (115–117). Along with quercetin, additional flavonols found in onions include kaempferol, luteolin, and isorhamnetin (118, 119).
Onion bulbs are used not only for their taste and aroma but also for the nutritional value they provide to the human diet. Several studies have demonstrated that onion and its bioactive components have a variety of pharmacological effects (120), including anti-inflammatory (121), anti-obesity (121), anti-spasmodic agent (100, 122), anticancer (123–125), and wound healer (126). Additionally, it has long been recognized as a helpful therapy for a variety of medical conditions including diabetes (95, 127), cardiovascular disease (128), hypertension (106), anxiety (129), and asthma (130). Furthermore, A. cepa demonstrated that it suppressed gram-positive bacteria more efficiently than gram-negative bacteria (131) and that it reduces DNA damage and breaking owing to the presence of quercetin, a potent antioxidant (109, 132).
The onion bulb extract has been shown to both prevent and reverse colitis by modulating several pro-inflammatory signaling pathways, including the mechanistic target of rapamycin (mTOR), the mitogen-activated protein kinase family (MAPK), cyclooxygenase-2 (COX-2), and tissue inhibitors of metalloproteinases (TIMP), as well as several molecules involved in the apoptotic pathway (121, 133, 134). They also found several phytochemicals such as saponins, tannins, and anthocyanin can help fight inflammation (104, 105). In the MC3T3-E1 preosteoblastic cell line, quercetin in a dose-dependent manner strongly inhibited the nuclear factor kappa-light-chain-enhancer of activated B cells (NF-jB) (135). Furthermore, in animal and human investigations, quercetin lowered TNFa/IL-10 and IL-8/IL-10 ratios (136–138). Umoh et al. showed that red onion may reduce inflammation by inhibiting NF-jB, MARK, and STAT-1, perhaps via the action of its active component quercetin (139). Besides, quercetin was able to reduce inflammation in DDS-induced colitis mice via upregulation of GSH levels (140).
In recent years, scientists have concentrated their efforts in vitro, in vivo, and human investigations on the prevention of cancer through diets with a high percentage of onion. Inhibiting cell cycle, triggering apoptosis, inhibiting tyrosine kinase, regulating p53 protein, and inhibiting antioxidant activity that interferes with several phases of cancer cell creation, development, differentiation, and metastasis (117, 139, 141–145). Onion extracts or their key bioactive constituents have shown strong anticancer activity against prostate, stomach, breast, lung, colorectal, laryngeal, and esophageal cancers, pancreatic, adenocarcinoma, and glioblastoma (146, 147). Quercetin, a novel onion component, might cause G (2) phase arrest, reduce colon cancer cell growth, and trigger autophagic cell death (148). In particular, it has been detected the endocannabinoid receptor (CB1-R) expression, PI3K/Akt/mTOR pathways, and the pro-apoptotic JNK/JUN pathways in Caco2 and DLD-1 cells. It was found a considerable increase in the expression of the endocannabinoid receptor (CB1-R), as well as suppression of important survival signals including PI3K/Akt/mTOR (145). The administration of quercetin was obsessively monitored for 48 h. In both the Colo-320 and Colo-741 cell lines, there was an increase in BAX immunoreactivity after quercetin treatment, but only in the Colo-320 main cell line was there existing a substantial reduction in Bcl-2 immunoreactivity (149). By a remarkable mechanism, quercetin (5 M) was able to significantly reduce the migratory and invasive potential of Caco-2 cells, resulting in decreased MMP-2 and MMP-9 expressions, whereas E-cadherin was downregulated. furthermore, quercetin has been shown to inhibit the production of inflammatory mediators such as TNF-α, COX-2, and IL-6 (150). Male Wistar rats were given (200 mg/kg, 28 days) or (0.5 g, 27 weeks) of onion-rich of quercetin had a big impact on ACF formation, mucin depletion, mitosis, and increasing the apoptosis percent in the treatment group. Although significant influence on cell proliferation and the expressions of p53 and BAX (151). (152) demonstrated the quercetin-loaded MPEG–PCL nanomicelles (Qu-M) dispersed entirely in water and released quercetin for a long time in vitro and in vivo. In vitro, Qu-M enhanced apoptosis induction and inhibited cell proliferation in CT26 cells. Furthermore, the mice (BALB/c) subcutaneous CT26 colon cancer model was constructed to assess the therapeutic effectiveness of Qu-M in greater detail. Qu-M investigates a high impact on cell death, preventing tumor angiogenesis, and limiting cell proliferation (152). Supplying quercetin (30 mg/kg, 4 weeks) to AOM/DSS-induced colon cancer mice (Wild-type C57BL/6J mice) decreased the number and size of tumors by a significant margin including, reduce the inflammation produced by AOM/DSS, recovered leukocyte numbers, also reduces oxidative stress indicators such as lipid peroxide (LPO), nitric oxide (NO), superoxide dismutase (SOD), glucose-6-phosphate (G6PD), and glutathione (GSH) (153).
Garlic: Allicin
Garlic or Allium sativum L. is a bulbous plant of the Alliaceae family that grows in the Mediterranean region (154, 155). In addition to its medicinal properties, garlic is also widely used as a food and spice (156, 157). Garlic is distinguishable from other members of the allium family by its clove-shaped bulbs and flat leaves (158, 159). Garlic includes at least 33 sulfur compounds, various enzymes such as peroxidase, allinase, and myrosinase, 17 amino acids, and minerals such as selenium, calcium, copper, iron, potassium, magnesium, and zinc, as well as vitamins A, B1, and C, fiber, and water (156, 160–163). It has the highest concentration of sulfur compounds of all Allium species (158, 163). Sulfur compounds are responsible for garlic’s strong odor as well as many of its therapeutic properties (163). Saponins (proto-eruboside B, eruboside B, sativoside), lectins, and flavonoids are some of the other elements found in this plant (159, 160). A compound called allicin is one of the most important biologically active compounds. Garlic does not have allicin until it is crushed or cut. The enzyme allinase, which breaks down alliin into allicin, is activated when the garlic bulb is damaged. Once it’s made, it quickly breaks down, but the speed of this reaction changes depending on the temperature. Allicin can still be found in garlic that has been refrigerated for a few days. At room temperature, it breaks down into smelly sulfur compounds like diallyl di- and tri-sulfides, ajoene, and vinyldithiins in just a few hours (164–168).
Garlic was highly prized in ancient Egyptian, Greek, and Chinese cultures as a food and medicinal (169, 170). It has been studied clinically for a variety of illnesses, including hypertension, hypercholesterolemia, diabetes, common cold, and cancer prevention (171–178). This plant has antibacterial, antifungal, antioxidant, immune system stimulant, and anti-parasitic properties (159, 162, 176). Garlic’s medicinal potential has also been investigated in a variety of inflammatory illnesses, including allergic rhinitis, allergic asthma, IBD, rheumatoid arthritis, and atherosclerosis (158, 177). Breath odor is a common adverse effect of using garlic, both orally and intravenously (162, 179).
A person’s risk of developing malignant tumors may be increased or decreased depending on the amount of some foods consumed or the number of others omitted from their diet. On this premise, dietary treatments are regarded to have the capacity to prevent or modify malignancies. One of these natural compounds, garlic (Allium sativum), has been studied for medicinal purposes. Allicin, a compound found in garlic, has been shown to inhibit CRC metastasis by strengthening the immune system and limiting the growth of tumor arteries (180).
Using allicin, an active ingredient derived from the popular seasoning agent or condiment Allium sativum L., was able to reduce the secretion of pro-inflammatory factors such as interleukin-6 (IL-6), prostaglandins (PG), nitric oxide (NO), interleukin-1 (IL-1), interleukin-6 (IL-6), and TNF-a, while simultaneously increasing anti-inflammatory cytokines such as IL-10 (181–184). Rats with Acrylamide (AA)-induced intestinal damage were used to examine the possible therapeutic benefits of allicin food supplementation. Allicin significantly reduced the expression of Toll-like receptor 4 (TLR4), NF-kB signaling pathway proteins, and proinflammatory cytokines in AA-treated rats by boosting the synthesis of SCFAs (185). In Caco-2 cells, allicin (25 mg/ml) can significantly inhibit p-38 and the JNK pathway activation. Allicin also suppressed the production of TNF-α and IL-6 generated by IL-1 at the mRNA and protein level in a dosage-dependent manner (186). According to Li et al. (186), an oral dose of allicin enhanced the colonic histopathology score and investigated the synergistic effects of allicin (30 mg/kg)-mesalazine (30 mg/kg) and allicin (30 mg/kg)-sulfasalazine (100 mg/kg) on TNBS (50 mg/kg) induced Wistar rats. Therapy with allicin-mesalazine decreased the colonic histopathology score from 5.83 to 2.10, whereas treatment with allicin sulfasalazine decreased it to 3.38. TNF-a levels were lowered by allicin-mesalazine therapy to 2.65 (pg/ml) from 6 (pg/ml), while allicin or mesalazine treatment alone reduced them to roughly 3.8 (pg/ml). TNBS therapy decreased IL-4 concentration to less than 4 (pg/ml), while mesalazine-allicin treatment increased their concentration to 5.76 (pg/ml), but neither allicin nor mesalazine alone could boost their expression to synergistic levels.
It was investigated the impact of allicin on cell proliferation in colon cancer cell lines HCT-116, LS174T, HT-29, and Caco-2 in vitro, as well as the underlying processes. Allicin has been demonstrated to have chemo-preventive effects on critical cellular processes such as mitochondrial membrane potential maintenance, intracellular redox control, and cell division. Allicin triggers G2/M arrest alters intracellular glutathione (GSH) levels (187) and causes a transitory decrease in intracellular GSH levels (187). Allicin treatment caused apoptotic cell death in HCT-116, as shown by increased hypodiploid DNA content, reduced levels of B-cell non-Hodgkin lymphoma-2 (Bcl-2), increased levels of BAX, and increased capacity to release cytochrome c from mitochondria to the cytosol. Allicin also caused NF-E2-related factor-2 (Nrf2) to be translocated to the nucleus of HCT-116 cells. Although Allicin’s cytotoxic effects were considerable when evaluated in four distinct human colon cancer cell lines (188). Experiments on animal models of carcinogenesis showed that components of garlic (e.g., allyl sulfides) suppress both the start and promotion phases of tumorigenesis in a wide range of malignancies including colorectal, lung, and skin (189). Perez-Ortiz et al. (190) Test the efficacy of a thiosulfinate-enriched garlic extract in combination with 5-fluorouracil (5-FU) or oxaliplatin chemotherapy in colon cancer cells as a new chemotherapy regimen that may also lower the cost of clinical treatment. The cytotoxic effects of an Allium sativum extract enriched in thiosulfate were investigated in two distinct human colon cancer cell lines, Caco-2 and HT-29, respectively. The doses of allicin (43–60 g/mL) were discovered to substantially decrease colon cancer cell growth and induce apoptosis. The impact of Allicin on the azoxymethane/dextran sodium sulfate (AOM/DSS) CRC mice model on STAT3 is being studied. Through various ligand-mediated phosphorylation, STAT3 plays key roles in cytokine signaling pathways, as well as cell proliferation and death. STAT3 activation causes the transcription of target genes such as Bcl-2, Bcl-xL, Mcl-1, and p21, all of which are important in cell survival and proliferation (180, 191). STAT3 activation may increase cell proliferation, angiogenesis, and inhibit apoptosis in human cancer cells. According to the western blot results, Allicin reduced the levels of phosphorylated STAT3. Allicin also inhibited the expression of Mcl-1, Bcl-2, and Bcl-xL. Therefore, allicin may be able to prevent colonic carcinogenesis in AOM/DSS mice in vivo (180). As an example, Diallyl trisulfide (DATS), an organosulfur compound isolated from garlic, has shown anticancer activity both in vitro and in vivo by reducing tumor mitosis and enhancing histone acetylation of H3 and H4 in both tumors and healthy cells (168).
Oregano: Carvacrol
Oregano is one of the most highly prized spices in the world, both commercially and culinary (192). oregano is formed from the terms “Oros” and “Ganos,” which both refer to the beauty of the mountains in ancient Greek (193). Oregano is the common name for at least 61 different species spread over 17 different genera and six different families (194). The family Lamiaceae contains the genus Origanum, which is the primary source of well-known oregano spice (194). All of the other plant families (Rubiaceae, Scrophulariaceae, Apiaceae, and Asteraceae) play a minor role (194–196). Monoterpenes and sesquiterpenes make up the majority of the essential oils in the Lamiaceae family. Their action is linked to the presence of carvacrol and thymol, which are combined with the primary elements of oregano, p-cymene, and terpinenes (196–198). Also, Oregano has a high nutritional value since it includes considerable quantities of vitamins and minerals while having a low salt level (195, 199, 200). Numerous studies have demonstrated the beneficial effects of oregano on human health, including its use in the treatment of a wide range of ailments, including respiratory tract disorders, gastrointestinal disorders (anti-stomachic and tonic agent), as an oral antiseptic, analgesic, urinary tract disorders (as a diuretic and antiseptic agent), anti-inflammatory, and even anticarcinogenic properties (201–205).
Oregano plant species have been extensively utilized in traditional medicine to treat inflammation-related disorders via a variety of mechanisms, including Reduced synthesis of proinflammatory cytokines such as TNF-α, IL-1, and IL-6, and increased production of anti-inflammatory cytokines such as IL-10 (206), as well as inhibition of aldose reductase and lipoxygenase (206, 207). Oregano, which is high in essential oils, has been shown to inhibit the COX-2 enzyme, which is linked to tissue inflammation (208). Carvacrol may also play a role in reducing the side effects of chemotherapy, in addition to its anticancer properties. The anticancer medication irinotecan hydrochloride causes a condition in which inflammation and cell damage are triggered by the transient receptor potential cation channel subfamily A, member 1 receptor. Carvacrol is an agonist of this receptor leading to an effective reduction of inflammation biomarkers, such as nuclear factor b and cyclooxygenase 2, as well as oxidative stress, as measured by glutathione, malondialdehyde, and NOx levels in a mouse model of inflammatory arthritis (209).
There are a lot of different Flavonoids and phenolic compounds in species of oregano, and some of them have been shown to fight colon cancer (210). As a result, research has looked into whether oregano flavonoids and phenolic compounds could be used as anti-colon cancer.
The “shutting down” of many cancer survival pathways, including the ERK/MAPK and PI3K/Akt pathways, may be responsible for the overall suppression of colon cancer cell growth following treatment with Origanum syriacum ethanol extract (211). The whole extract of Origanum vulgare is responsible for the apoptosis-inducing action (212). Carvacrol significantly slowed the growth, migration, and invasion of colon cancer cells by stopping cells at the G2/M phase and causing them to die, reduced Bcl-2 expression, phosphorylated extracellular regulated protein kinase (p-ERK) and p-Akt, and increased BAX and c-Jun N-terminal kinase (p-JNK) expression (LoVo and SW620 metastatic cells line) (213). Showcase of Mexican oregano in colon cancer cells, there was an increase in the expression of BAX (apoptotic protein) and a decrease in the expression of Bcl-2, PARP, and Survivin (anti-apoptotic proteins), as well as an increase in the expression of caspase-3 in various oregano cultured plants (wild type, in vitro and ex vitro plant tissue culture) (202). Thymol, another phenolic component typically found in O. syriacum, was discovered to suppress the growth of bladder and colon cancer cells (HCT116, LoVo, and Caco2 cells line) in vitro, which was promising (214, 215). The key mechanistic activity of thymol’s action was identified to be the inhibition of JNK and p38 as the main mediators (214, 215). In another investigation, oregano aqueous infusion had the strongest radical scavenging efficacy in HT29 cells. Oregano’s strong antioxidant activity has been linked to a variety of substances including carvacrol and other phenolic compounds (202).
Saffron: Crocin
Saffron, the world’s most expensive botanical spice, commonly known as “red gold,” is made from the dried stigmas of Crocus sativus L., a member of the broad Iridaceae family (216, 217). It is an autumn-flowering, high-value, low-volume spice crop plant that originated in the Middle East and is now farmed in China, India, Iran, Azerbaijan, Turkey, Egypt, Morocco, Greece, Spain, Italy, France, and Mexico (218–221). In addition to the elements that already contained in saffron, such as protein, fiber, lipids, vital minerals, and vitamins B1 and B2 (222–224). There are various key metabolite components, including carotenoids (crocetin, crocin, zeaxanthin), monoterpene aldehydes (picrocrocin and safranal), monoterpenoids, and phenolic compounds (anthocyanins and flavonoids) that contribute to the diverse pharmacological effects of this substance (216, 225–227). Three major bioactive chemicals (crocin, picrocrocin, and safranal) are found in significant amounts among these metabolites, and they are responsible for the Safran’s distinctive red color (crocin), bitter taste (picrocrocin), odor, and aroma (safranal), and other characteristics (146, 228, 229).
Saffron consumption correlates with a lower risk of many types of cancer (227, 230–232), improvement of depression and memory loss (226, 227), regulation of menstruation (233), accelerated wound healing in burn injuries, and relief of cough and asthmatic breathing (229, 230). It has also been shown to be an antihypertensive (225, 234), antianxiety (233), insulin resistance lowering agent (233, 235), cardioprotective (236), and gastroprotective properties (233).
Various research has shown that the anti-inflammatory and antioxidant effects of saffron constituents are due to their significant inhibitory effects against cyclooxygenase 1 and 2 enzymes and prostaglandin E2 production (237), attenuating endoplasmic reticulum stress signaling, blocking pro-inflammatory cytokine production such as TNF-α, inhibiting transcription factors such as nuclear factor kappa B (NF-kB), which exacerbates chronic inflammation, and suppressing inflammatory gene expression (223, 238). Additionally, the anti-inflammatory properties of saffron derivatives affect neuroinflammation (237). Following the study (239), the researcher concluded that crocin protects rat gastric mucosa ethanol-induced injury through the expression of anti-inflammatory, antioxidant, antiapoptotic, and mucin secretagogue mechanisms, which are most likely mediated through increased PGE2 release. After only 4 weeks, Kawabata et al. found that crocin feeding could prevent Dextran Sulfate Sodium (DSS)-induced colitis and decrease TNF-α expression, IL-1β, IL-6, IFN-γ, NF-kB, COX-2, and iNOS in the colorectal mucosa and increased nuclear factor (erythroid-derived 2)-like 2 (Nrf2) expression (240). Concerning the effectiveness of saffron in the treatment of ulcerative colitis (US), studies have shown that oral administration of crocetin to mice (25–100 mg/kg per day) for 8 days significantly ameliorated TNBS-induced UC, as evidenced by a reduction in NO, neutrophil infiltration, and lipid peroxidation in the inflamed colon, favorable expression of TH1 and TH2 cytokines, and down-regulation of the NF-kB (241).
According to the literature, saffron and its components have chemopreventive activity via the inhibitory effect of saffron on cellular DNA and RNA synthesis, modulation of lipid peroxidation, antioxidants, immune modulation, enhancement of cell differentiation, inhibition of cell proliferation, modulation of carcinogen metabolism, cell cycle arrest through p53 dependent and independent mechanisms causing apoptosis, the interaction of carotenoids with topoisomerase II (227, 242–247). While the majority of in vivo and in vitro studies focused on isolated bioactive compounds from saffron, Aung et al. (248) revealed that C. sativus and its primary ingredient, crocin, effectively reduced the proliferation of CRC cell lines (HCT-116, HT-29, SW-480) and non-small cell lung cancer cell lines (NSCLC) by MTS test while not affect normal cells. Aung et al. (248) demonstrated that saffron crudes and its main compound crocin can be used to supplement current CRC treatments by limiting cancer cell proliferation and motility progression by targeting the Metastasis-Associated in Colon Cancer 1 (MACC1) as a major causal metastasis-inducing gene. Crocetin (0.8 mmol/L) significantly triggered cell cycle arrest and P21 induction and caused cytotoxicity in SW480 cells by increasing apoptosis and lowering DNA repair capability in a time-dependent manner (249). Another research revealed that long-term intraperitoneal injection of crocin (400 mg/kg body weight) improves survival and inhibits tumor development in female rats with colon cancer generated by subcutaneous injection of rat adenocarcinoma DHD/K12 PROb cells (227). Crocin exhibited antiproliferation activity against the HCT116 cell line via induction of apoptosis and attenuation of the ratio of p-STAT3/STAT3 (250). As well, it suppressed tumor growth in colitis-associated CRC mouse model via modulation of the Wnt/PI3K pathway. Crocin was also able to lower disease-activity index and mucosal ulcer inflammation by regulating antioxidant markers, including catalase (CAT) activity and malondialdehyde (MDA) (251).
Rosemary: Rosmarinic acid
Rosmarinus officinalis (Rosemary) is a typical houseplant growing around the globe that belongs to the Lamiaceae family (252, 253). The chemical composition of rosemary extract was examined to determine its active principles, which indicated the existence of many compounds, including rosmarinic acid (RA), caffeic acid (CA), chlorogenic acid, carnosic acid, rosmanol, and carnosol (252, 254–256). Therefore, three types of chemicals have been linked to the biological activity of R. officinalis L.: a volatile fraction, phenolic compounds, and di and triterpenes (254, 255).
Rosemary has a long history of usage in food to change and improve tastes. On the other hand, rosemary extracts have anti-inflammatory, antioxidant, antimicrobial, antitumor, antispasmodic, and anti-diabetic bioactivities. The low toxicity and strong cardioprotective, hepatoprotective, neuroprotective, diuretic effect, estrogenic effect, as well as memory enhancement and pain relief have been investigated in the reviewed literature (253, 257–262). On the other hand, rosmarinic acid (RA) was able to reduce inflammation in AOM/DSS-induced colon cancer mouse model by inhibiting NF-kB and STAT3 pathways (263). 264 suggested that RA alone or in combination with black rice extract can suppress colitis disease in DSS-induced colitis mice. The results of the study have shown a reduction in the inflammatory mediators expression, including IL-6, IL-1β, TNF-α, iNOS, and COX-2 (264).
Rosemary has been shown anticancer properties in both models: in vitro and in vivo. Several of these properties have been ascribed to its principal constituents, including carnosic acid, carnosol, ursolic acid, and rosmarinic acid (252, 265). Rosemary has been shown to have significant antiproliferative activity against several human cancer cell lines (266–269), induce apoptosis via nitric oxide production (270, 271), antioxidant activity (265, 266, 272), decreased TNF-α, IL-6, and COX2 levels (273, 274), suppress lipid peroxidation (261, 275, 276), prevent carcinogen-DNA formation (265), stimulation of p53 and BAX (277), reduction of Bcl-2, Mdm2, and Bcl-xL expression, and stimulation of caspase-3 and -9 expression (272, 274, 277, 278). Another probable method is via inhibiting Akt phosphorylation, which is required for cancer cell proliferation, growth, and survival (279). A study has tested the antiproliferation activity of rosemary extract on HT-29 and SW480 cells. Rosemary extract suppressed tumor cell growth via increasing intracellular ROS, reducing in G0/G1 phase, and improving cells accumulation in the G2/M phase (280). Moreover, Valdés et al. reported that carnosol-enriched extract showed significant inhibition of cell growth by inducing G2/M cell cycle arrest in colon cancer cells (SW480 and HT-29 cell lines) (281). A recent study suggested that rosmarinic acid (RA) can reduce the rate of adenocarcinoma formation in azoxymethane-induced CRC in rats. It upregulated the total antioxidant status (TAS) and decreased the expression of IL-6 and MCP-1 (282). Using male Wistar rats with DMH-induced colon cancer, RA exhibited a chemoprotective effect via suppressing tumor formation and decreasing lipid peroxidation (276). Figure 4 summarized the anticancer effect of herbs and spices in CRC.
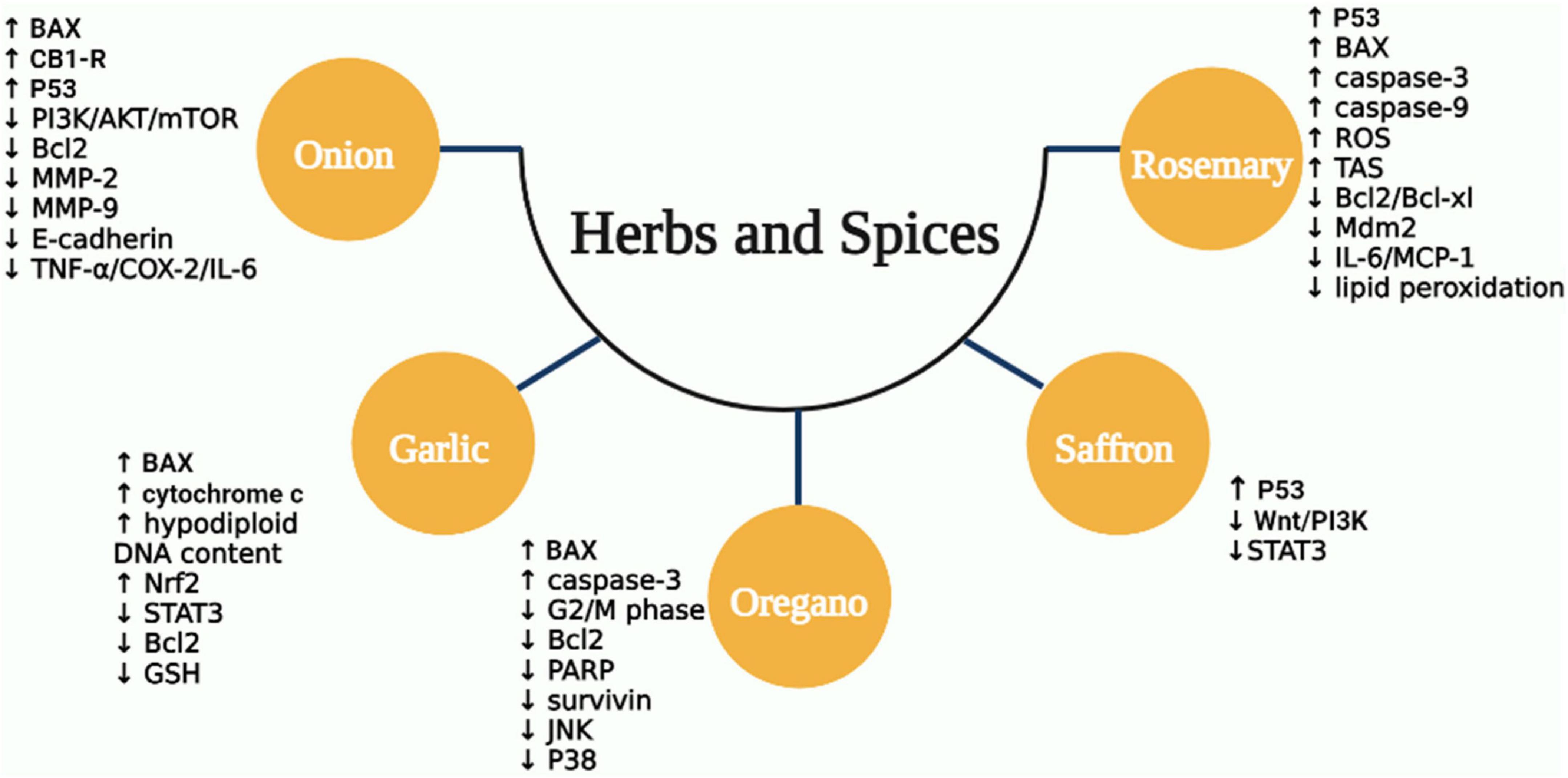
Figure 4. The mechanisms of anticancer activity for some types of MD herbs and spices. ROS, reactive oxygen species; TNF-α, tumor necrosis factor-alpha; IL, interleukin; COX-2, cyclo-oxygenase-2; MMP-9, matrix metalloproteinases-9; JNK, Jun N-terminal kinase; BAX, Bcl-2-associated X protein; PARP, poly adenosine diphosphate ribose polymerase; Nrf2, nuclear factor-erythroid factor 2; STAT3, signal transducer and activator of transcription 3; GSH, glutathione; CB1-R, cannabinoid receptor type 1; Mdm2, mouse double minute 2 homolog; MCP-1, monocyte chemoattractant protein-1; Wnt, wingless-related integration; PI3K, phosphoinositide 3-kinase.
Whole grains and cereals: β-D-glucan
Whole grains are cereals that have the complete grain kernel (bran, germ, and endosperm) in contrast with refined grains that contain the endosperm only. There is considerable evidence that chronic diseases could be avoided by the consumption of whole-grain cereal products, which can reduce the risk of obesity, metabolic syndrome, type 2 diabetes, cardiovascular disease, cancer, and mortality from these chronic diseases (283, 284). Whole-grain cereals are an abundant source of fiber and bioactive compounds. For example, whole-grain wheat consists of 13% dietary fiber and at least 2% bioactive compounds excluding fiber. In the bran and germ fractions, still greater proportions are attained: about 45 and 18% of dietary fiber, and about 7% and at least 6% of bioactive compounds, respectively (285). The total dietary fiber of wheat ranges from 9 –to 20% and it consists of both soluble and insoluble portions. The two major components of dietary fiber in wheat are arabinoxylan (AX) and β-D-glucan (283). While barley and oats contain β-glucan as the primary fiber in the whole kernel, AX is present in much less content (286). As well, cellulose and hemicellulose are the major fiber components of corn bran and brown rice (287).
Dietary fibers provide numerous benefits, including a lower risk of cancer and enhanced colon health, Where low dietary fiber consumption has been linked to both local and systemic chronic inflammation (288). Recently, a study using a synbiotic composed of arabinoxylan (AX) and Lactobacillus fermentum HFY06 was tested to determine its ability to relieve DSS-induced colitis. AX and L. fermentum HFY06 inhibited the activation of the NF-κB signaling pathway, downregulated the mRNA expression levels of NF-kBp65 and inhibited the TNF-α, and exerted anti-colitis effects (289). As well, the short-chain fatty acids (SCFA), particularly butyrate, are byproducts of dietary fiber fermentation by certain microorganisms in the intestinal colon, and they exhibited anti-inflammatory actions on both gut epithelial and immune cells. Hence, inflammation signaling pathways involving nuclear factor kappa-B (NF-kB) and deacetylase are inhibited by SCFA (288, 290). Several studies have been established to investigate the anti-inflammatory effect of the major types of dietary fibers. Such as the beneficial effect of oat β-D-glucan has been tested on Sprague–Dawley rats with TNBS-induced colitis. The results showed a significant reduction in IL-6, IL-10, C reactive protein (CRP), and IL-12. As well, β-D-glucan reduces some selected inflammatory markers, including COX, PGE2, and thromboxane A2 (TXA2). The results indicate the therapeutic effect of dietary oat beta-glucan supplementation in colitis (291). In another study, oat beta-glucan has been tested also on male Sprague Dawley rats with TNBS-induced colitis. The results proved the in-direct antioxidant effect of beta-glucans by agonistic binding of immune cells to membrane receptors, which results in increased antioxidant response and removing systemic effects of colon inflammation (292).
Another well-known function of dietary fiber is to lower the CRC incidence, as it reduces the concentrations of carcinogens and procarcinogens in the feces. Furthermore, it shortens the residence time of carcinogens in the lower gastrointestinal tract, reducing their absorbance and contact time with colon epithelium cells (293). As previously stated, dietary fibers digested by intestinal bacteria produce SCFA which has a protective effect against the growth of cancer cells (294, 295). 296 examined the role of dietary fiber in polyposis by using TS4Cre × cAPCl°x 468 mice. The results showed that a high fiber diet significantly increased SCFA-producing bacterium as well as SCFA levels. This was associated with an increase in SCFA butyrate receptor and a significant decrease in polyposis. The prebiotic activity of fiber could be the key mechanism for the protective effects of fiber on colon carcinogenesis. Overall, the findings revealed that insoluble fermentable fiber may protect against CRC (296). As well, a dietary fiber (β-glucan) with quercetin anti-colonic cancer effect has been tested. The findings demonstrated that alternating β-glucan and quercetin consumption alleviated colon damage and reduced mortality in CRC mice, with a 12.5% reduction in mortality. Consumption of β-glucan and quercetin alternated dramatically reduced TNF-α, increased the relative frequency of Parabacteroides, and downregulated three genes linked to inflammation and cancer (Hmgcs2, Fabp2, and Gpt) (297). Moreover, β-glucan was tested in human colon cancer cells (SNU-C4) and it exhibited antiproliferation activity by reducing Bcl2 expression and upregulation of BAX and caspase-3 levels (298).
Fish: PUFA/n-3 fats
Fish is another important component of the traditional MD diet. In recent years, there has been a lot of emphasis on the positive effects of fish eating, which has been reinforced by the idea that the ocean is a fantastic source of new compounds. Fish meat is abundant in anti-inflammatory n-3 polyunsaturated fatty acids (PUFA), such as eicosapentaenoic acid (EPA) and docosahexaenoic acid (DHA), which have been proven to reduce the risk of cardiac mortality by 30–45%, and lessen ischemic stroke incidence (299, 300). As well, n-3 PUFA appears to be protective against dementia and Alzheimer’s in the elderly (301). It has been proven to affect various types of cancer, including breast, ovarian, prostate, lung, skin, colon, colorectal, pancreatic, and stomach cancers. These benefits of n-3 PUFA are a result of their antioxidant, anti-inflammatory, anti-apoptotic, and neurotrophic properties (302). As recently reviewed, n-3 PUFAs have anti-inflammatory effects against inflammatory diseases, including IBD, psoriasis, and rheumatoid arthritis by lowering arachidonic acid (AA) proinflammatory activities, increasing the production of endocannabinoids with EPA or DHA in their structure and thus anti-inflammatory properties, lowering the production of inflammatory cytokines like IL-1, IL-6, and TNF-α, lessening in T-cell proliferation and the formation of IL-2 (303). Moreover, they increase the production of anti-inflammatory markers; e.g., soluble IL-6r, IL-10, transforming growth factor-β (TGF-β) and lowering the expression of adhesion molecules on immune cells and endothelium. As well, the homeostasis of tissues has been restored after inflammation as a result of n-3 PUFA metabolites production such as resolvins, protectins, and maresins, which act as specialized pro-resolving mediators. These pro-resolving bioactive lipids act as “stop-signaling” of the inflammatory response and have important anti-inflammatory and anti-carcinogenic properties, by increasing macrophage phagocytosis, efferocytosis, and leukocytes egress (304, 305).
Recently, the impact of n-3 PUFA and probiotics have been investigated in BALB/c mice subjected to 2,4-Dinitrobenzenesulfonic acid (DNBS) induced-chronic colitis. Administration of combination reduced the concentrations of inflammatory mediators such as IFN-γ, TNF-α, and IL-17A. The findings showed that the combined effect of probiotics and n-3 PUFA might have a protective effect against colon injury and inflammation by creating synergistic effects (306). Moreover, the anti-inflammatory effect of n-3 PUFA was investigated in a rat model of acetic acid-induced UC. The results showed that after administration of n-3PUFAs, the expression levels of IL-1 and Caspase-3 were downregulated, whereas Bcl-2 was upregulated. These findings imply that n-3 PUFAs protect the colonic mucosa of rats against acetic acid-induced UC, and may aid in the repair of UC via anti-inflammatory and anti-apoptotic properties, as well as a regenerative endogenous antioxidant mechanism (307).
Interestingly, n-3 PUFA is also known to have anticancer potentials and is associated with the alteration of cancer hallmarks and tumor regression activity. It can modulate cyclooxygenase (COX) metabolism and reduce the production of several prostanoids including prostaglandin (PG) E2 in tumors whilst possibly increasing the production of lipid mediators involved in the resolution of inflammation such as lipoxins and resolvins, which may have anti-cancer properties (308). As well, n-3 PUFA has been found to bind to the plasma membrane of cancer cells, changing the content and fluidity of the lipid membranes. This can cause signal transduction to be inhibited, reducing cancer cell viability and encouraging apoptosis (309). Other CRC-promoting signaling pathways, including the Wnt/ß-catenin pathway, the MAPK/ERK pathway, and the PI3K-PTEN system have been reported to be downregulated by n-3 PUFA (310). In a recent study on CRC cells, SW620 and HCT-116 parental and HCT-116 mutant cells isogenic for constitutively active PI3K were treated with free or ethyl esterified n-3 PUFA. The results showed the ability of n-3 PUFA ethyl esters to inhibit PI3K activity confers their potency to reduce CRC cell invasion, but not proliferation. (311) Furthermore, n-3 PUFAs can activate pro-apoptotic signaling by interacting with G protein-coupled receptors (GPCRs), resulting in an anti-CRC activity. Non-epithelial cells including adipocytes and macrophages have been found to express these GPCRs. Activation has the potential to change macrophage polarization and reduce inflammation, both of which are critical for n-3 PUFA anti-cancer action (312). Additionally, in the LS174T human CRC cell line, the effect of EPA derived from n-3 PUFAs on cell number, cell proliferation rate, and caspase-3 enzyme activity was studied. When EPA concentrations were increased, caspase-3 activity rose by 3.4 times relative to untreated control cells at 200 mol EPA and reduces the number of CRC cells and their growth rate (313). A further study examined the effect of DHA on migration in CRC cell lines and found that 100 mM DHA inhibited Granzyme B expression in three human CRC cell lines (HCT116, CSC4, and HT-8), limiting their capacity to undergo epithelial-mesenchymal transition (EMT) (314).
Grapes: Resveratrol
Grapes have been associated with health benefits for many years, despite a lack of scientific evidence, and have been closely linked with diet since ancient times, particularly in Mediterranean countries. Several studies conducted around the world over the last two decades have shown that consuming grapes have beneficial impacts on antioxidant capacity, lipid profile, and the coagulation system (315).
Grape juice is made mostly from European (Vitis vinifera) and American grape species (Vitis labrusca). Both species have high levels of polyphenolic compounds, such as caffeic acid, gallic acid, p-coumaric acid, and stilbenes (trans-resveratrol). Resveratrol (RV) is one of the most important polyphenols found in grapes. As well as, Flavonoids such as quercetin, rutin, myricetin, catechin, and epicatechin (315, 316). The amount of RV in grapes varies by grape genotype, cultivar, growing season, and climatic factors. It can be found in the leaf, skin, bud, stem, seed, bud, and root. Regardless, the majority of it is found in the grape skin, with much less in the juice and wine. Grape skin and juice contain more glycosylated RV than free RV (317). The benefits of RV are numerous. The most well-known benefits include anti-inflammatory, anti-proliferative, and chemopreventive. Multiple lines of evidence from in vivo and in vitro laboratory research suggest that the anti-inflammatory properties of RV can be explained by preventing the synthesis of anti-inflammatory factors (318). Chronic inflammation is one of the main mechanisms involved in colon cancer. Therefore, the anti-inflammatory effect may be beneficial in the treatment of CRC.
Resveratrol has been shown in vitro to reduce the production of pro-inflammatory mediators such as IL-1 and IL-6, as well as to down-regulate both mRNA expression and protein secretion of IL-17 in a dose-dependent manner. Resveratrol is also associated with the inhibition of 5-lipoxygenase, cyclo-oxygenase-2 (COX-2), and Nuclear Factor-kB (NF-kB). As well, suppressing the expression of tumor necrosis factor α (TNF-α), interleukin 8 (IL-8), and interferon-γ (IFN-γ) will lead to a decrease in the ulcerative colitis process (319–321). Ren et al. investigated the anti-inflammatory effect of RV on HEK-293T cells and HeLa cells the results have shown that RV suppressed endogenous NF-kB activity and TNF-α induced NF-kB activation (322). Another study has demonstrated the anti-inflammatory activity of RV in the DSS-induced colitis mouse model. The results exhibited a reduction in IL-2, IFN-γ, GM-CSF, IL-1β, IL-6, KC/GRO, and TNF-α, along with a significant effect on gut microbiota by increasing the abundance of Bifidobacterium (323).
Besides the anti-inflammatory effect of resveratrol in grapes, it also has a high potential for inhibiting tumor initiation, development, and promotion. The majority of research on grapes’ cancer-preventive properties focuses on resveratrol (324). The anticancer activity of resveratrol is mediated by a variety of molecular mechanisms and signaling pathways. Such as activation of the mitochondrial and caspase cascade enzymatic systems, as well as death-induced cytokines and their receptors are upregulated, as are cyclin-dependent kinase inhibitors, and tumor suppressor genes. Additionally, resveratrol will lead to downregulation of survivin, cFLIP, cIAPs, and antiapoptotic proteins (Bcl-2 and Bcl-xL), all of which are linked to the development of chemoresistance. Although resveratrol suppresses tumor cell proliferation by activation of proapoptotic proteins (P21 and P53) and suppression of hippo–YAP, inhibition of MAPK, phosphoinositide 3-kinase (PI3K)/Akt, nuclear factor β (NF-β), activating protein-1 (AP-1) HIF-1α and signal transducer and activator of transcription 3 (STAT3) (325–327).
Several studies were conducted to investigate the effect of resveratrol on different cancer cell lines. Such as in vitro study on human CRC cells HCT116 and SW620, the results indicated that RV dose-dependently upregulated the expression of several proapoptotic proteins such as BAX, cytochrome c, cleaved caspase-9, and caspase-3, while anti-apoptotic protein Bcl-2 expression levels was reduced in RSV-treated CRC cells. Overall, resulted in the suppression of CRC cell viability, increased cell apoptosis, and ROS levels compared with the control group, as well as activated the mitochondrial apoptotic pathway (328). In another in vitro study of resveratrol on HCT116, a human CRC cell line, the treated cells showed cell growth inhibition and apoptosis induction, as well as downregulation of intracellular AKT1 and IL-6 expression (329). Moreover, an in vitro cellular model of aggressive and resistant colon cancer enriched in CSCs was chosen as doxorubicin-resistant LoVo/Dx cells (a subline of the LoVo cell line), which were treated with RV and celastrol. The results have shown that celastrol and resveratrol produce an antitumor activity against metastatic LoVo cells and cancer stem-like by inducing apoptosis and cell cycle arrest, by increasing SIRT1 gene expression, resulting in overcoming apoptosis resistance in LoVo colon cancer cells (330). Furthermore, a study using CRC-derived cell lines, LoVo and HCT116, found that resveratrol inhibited CRC invasion and metastasis by suppressing Wnt/-catenin signaling and, as a result, the expression of its target genes such as c-Myc, MMP-7, and MALAT1, which leads to the inhibition of CRC invasion and metastasis (331).
Nuts: Hazelnuts/β-sitosterol
Hazelnuts (HN) produced by Corylus avellana L., a member of the genus Corylus of the Betulaceae family, are widely consumed around the world, and the common hazel is widely dispersed along the southern European coast and the Black Sea region (332). HN has several health benefits. Many studies showed the effect of HN on the reduction of LDL-C levels, and a tendency to lower total cholesterol accumulation. Besides, it reduced the incidence of certain chronic diseases such as cancers (333). The main chemical compositions of HN are simple phenols, lipids, and monounsaturated fatty acids, as well as, a source of minerals, tocopherols, tocotrienols, squalene, triterpenes, and phytosterols. The total phytosterol content of HN varies between 133.8 mg/100 g and 263 mg/100 g of oil. The most common is β-sitosterol (BS), which accounts for 83.6% of the total (334, 335).
β-sitosterol has immunomodulatory and anti-inflammatory activity, several studies suggested that BS can suppress inflammation through the NF-κB pathway (336, 337). Furthermore, BS stimulates the activity of T helper (Th) cells, as well as T cells and natural killer cells (338). A study investigated the effects of both stigmasterol and BS on DSS-induced colitis in C57BL/6J male mice. The results showed that both BS and stigmasterol significantly inhibited colon shortening, minimized fecal hemoglobin contents, and lowered the severity of colitis in the middle and distal colon. As well, they significantly suppressed the activation of the inflammatory master regulator NF-kB (339). Moreover, the effect of BS on 2,4,6-trinitrobenzene sulfonic acid (TNBS)-induced colitis in mice was also examined to see if it also exhibits anti-colitis properties. The study showed that BS inhibited colon shortening and resulted in lower macroscopic scores and myeloperoxidase activity. In the colons of TNBS-induced colitis mice, BS reduced the expression of proinflammatory cytokines TNF-α, IL-1, and IL-6, as well as COX-2 and activation of NF-kB. These data suggest that BS may help with colitis by suppressing the NF-kB pathway (340). Furthermore, in DDS-induced colitis in male C57BL/6 mice, BS was able to reduce the levels of TNF, IL-6, and IL-1 in intestinal tissue, indicating that β-sitosterol administration significantly reduced inflammatory damage to colonic tissues, including colon edema, crypt distortion, goblet cell loss, and mononuclear cell infiltration. These findings suggest that it could be useful in treating chronic colitis (341).
Besides the anti-inflammatory effects of BS found in hazelnuts, it has also been shown to protect against cancers such as breast, colon, colorectal, and prostate cancer. BS can halt tumor development and promote programmed cell death in cancer cells (342). A recent study tested the anticancer effect of BS-mediated silver nanoparticles (AgNP) on human colon cancer (HT-29) cells. The results suggested that BS improved apoptosis via inducing p53 expression in HT-29 cells (343). Furthermore, it inhibited the expression of breast cancer resistance protein (BCRP) and restored oxaliplatin (OXA) sensitivity in drug-resistant CRC cells. The study also found that BS could activate p53 by disrupting the p53–MDM2 interaction, resulting in increased p53 translocation to the nucleus and silencing the NF-κB pathway, which is required for BCRP expression. These findings showed that β-sitosterol can regulate CRC response to chemotherapy by mediating the p53/NF-B/BCRP signaling axis (344). Shen et al. (345) have reported that liposomal β-sitosterol can prevent tumor migration of colon carcinoma via downregulation of MMP-9 expression and modulation of Th1 immune markers. Additionally, BS, campesterol, and stigmasterol have been tested on colon cancer cells (Caco-2). The results revealed BS and other polyphenols induced reversible arrest in phase G0/G1 of the cell cycle (346). In HCT116 cells, BS induced apoptosis was accompanied by a decrease in anti-apoptotic Bcl-2 protein and mRNA and a concurrent rise in proapoptotic BAX protein and mRNA, as well as cytochrome c release from the mitochondria into the cytoplasm. The expression of cellular inhibitor of apoptosis protein-1 (cIAP1) was also suppressed by BS treatment (347). As well, the anti-CRC effects of BS were investigated in BALB/c nude mice. The study has proved that the treatment of mice with β-sitosterol decreased tumor growth by lowering PI3K/Akt expression, promoting Bad activation, decreasing Bcl-xl, and increasing cytochrome-c release, resulting in caspase-9 and caspase-3 activation, PARP cleavage, and apoptosis.
Hot pepper: Capsaicin
Hot pepper (Capsicum annuum L.), usually called chili, is a diploid, facultative, self-pollinating crop that belongs to the Solanaceae family (closely related to the potato, tomato, eggplant, tobacco, and petunia). Hot pepper contains many essential vitamins, minerals, and nutrients that have a significant role in human health (348–351). Peppers are a rich origin of both vitamins C and E (352, 353). The major components of most capsicum species are capsaicin (69%), dihydrocapsaicin (22%), nordihydrocapsaicin (7%), homocapsaicin (1%), and homodihydrocapsaicin (1%), respectively (354). Capsaicin (8-methyl-N-vanillyl-trans-6-non-enamide) is a naturally occurring alkaloid derived from chilies. It is responsible for its hot pungent taste, characterized by its odorless fat-soluble compound that is rapidly absorbed via the skin.
Lycium barbarum polysaccharides and capsaicin have a protective effect in rats with dextran sulfate sodium-induced ulcerative colitis through anti-inflammation and antioxidation actions (355). Oral capsaicin has downregulated IL-6 and protein expression of TRPV1 and TRPA1 as well as increased SOD level and catalase activities (356). Besides, dietary capsaicin exhibited an anti-colitis effect in DSS-induced colitis wide-type (WT) mice by improving Na+ absorption, and reducing Cl– level. It ameliorated intestinal inflammation by suppressing the hyperactivity of TRPV4 channels (357).
Capsaicin has an anticancer impact on HCT116 and LoVo cells (human colon cancer cells) through influencing cell cycle G0/G1 phase arrest and apoptosis, which was associated with an elevate of p21, BAX, and cleaved PARP. The capsaicin anticancer mechanism was attributed to the stabilization and activation of p53. It lengthened the half-life and boosted the transcriptional activity of p53 (358). Synergistic induction of both apoptosis and inhibition of cell proliferation was shown in HCT116, SW480, LoVo, Caco-2, and HT-29 (human CRC cells) that were treated with the combination of capsaicin and 3,3’-diindolylmethane. Also, these two compounds activated the transcriptional activity of NF-kB and p53 synergistically. The combination treatment stabilized nuclear p53 and up-or downregulated expression of several target genes that are downstream of NF-κB and p53 (359).
Figure 5 demonstrated the seven MD components and their effects on cancer biomarkers and CRC development. Figure 6 displays the molecular mechanism of the MD in CRC.
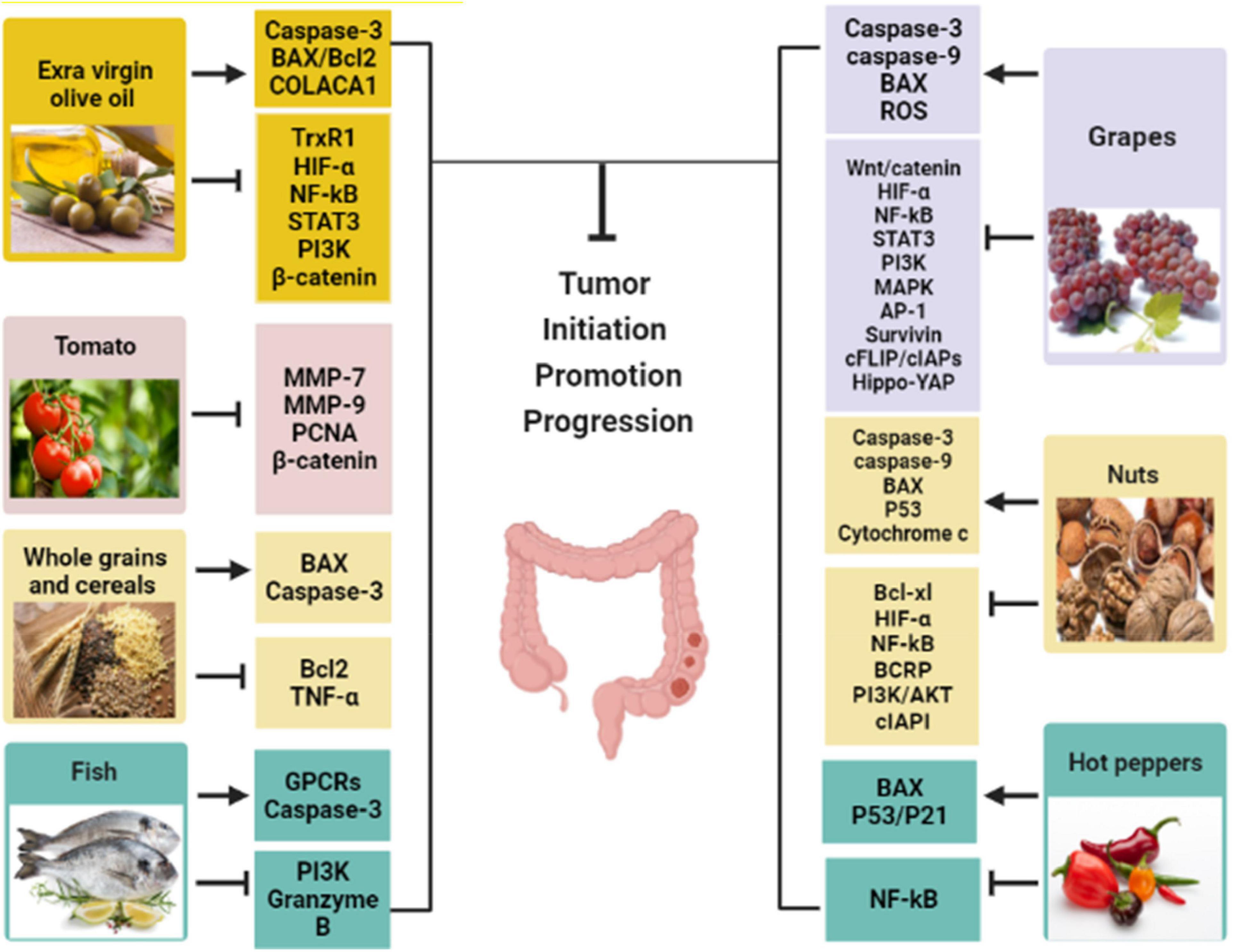
Figure 5. The Mediterranean diet components with their effects on cancer biomarkers. (→, activation; ⊤, inhibition; ROS, reactive oxygen species; TNF-α, tumor necrosis factor-alpha; IL, interleukin; MMP-9, matrix metalloproteinases-9; BAX, Bcl-2-associated X protein; Wnt, wingless-related integration; PI3K, phosphoinositide 3-kinase; PCNA, proliferating-cell nuclear antigen; TrxR1, thioredoxin reductase 1; COLACA1, colorectal cancer associated-1 gene; Bcl2, B-cell lymphoma 2; GPCRs, G protein-coupled receptors; MAPK, mitogen-activated protein kinase; AP-1, activator protein-1; c FLIP, cellular (FAAD-like IL-1β-converting enzyme)-inhibitory protein; cIAPs, cellular inhibitory of apoptosis proteins; BCRP, breast cancer resistance protein.
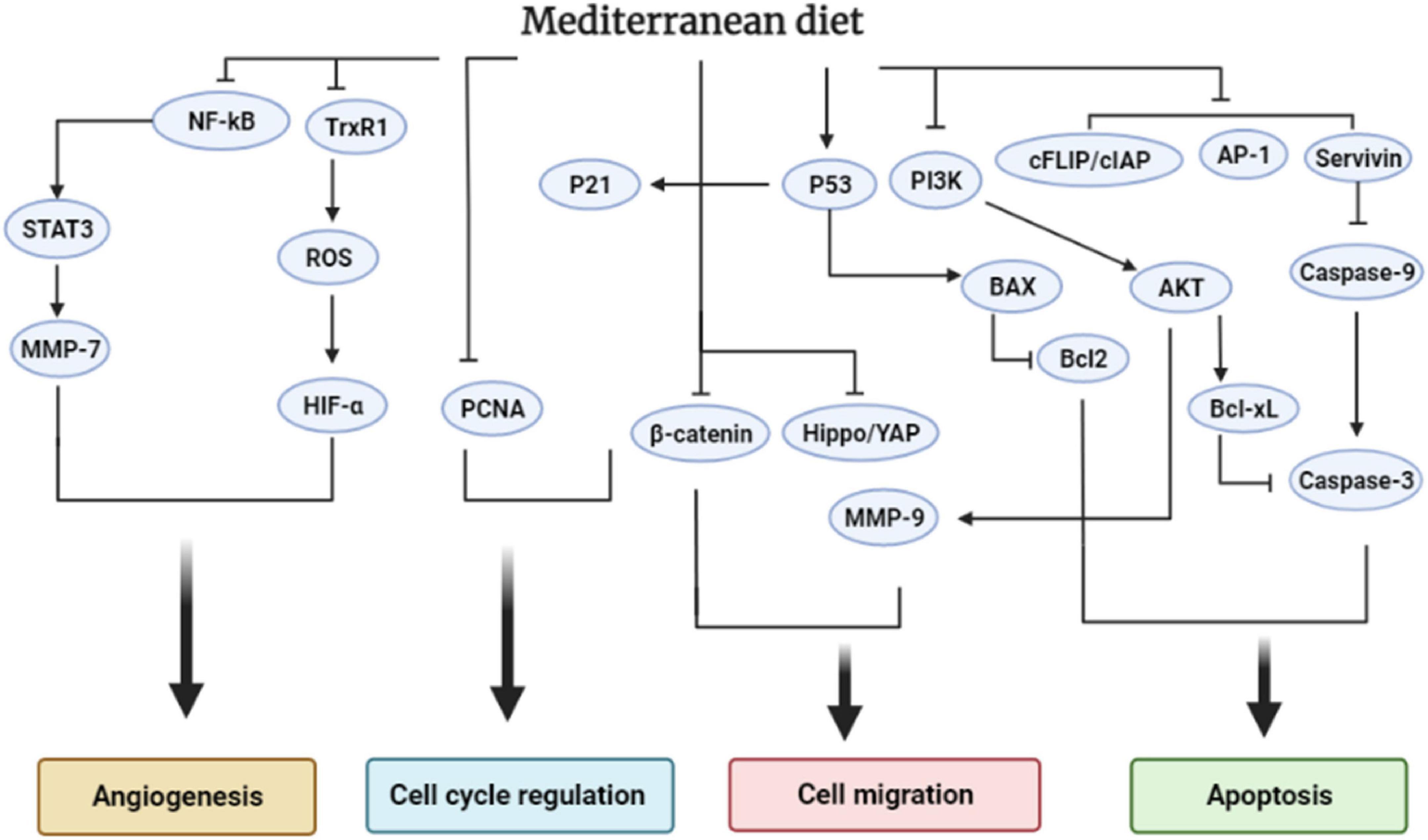
Figure 6. The effect of the Mediterranean diet on the cellular signaling pathways that regulate colorectal cancer progression.
Table 1 summarized the anti-inflammatory effects of all the discussed MD components. Table 2 summarized the anticancer effects of the MD components.
Role of the Mediterranean diet and its components in modulation of the gut microbiome
Several studies have shed the light on the role of the diet in the modulation and improvement of the gut microbiome (363). In particular, the MD which is recognized for its high plant-based food has shown a remarkable impact on gut microbiota profile (364, 365). It was found that adherence to MD has increased the presence of SCFA and fiber-degrading bacteria as well as reduced the presence of E. coli bacteria (365, 366). Besides, the positive impact of the MD on the gut microbiota content has been recognized by Garcia-Mantrana et al. (367), it was observed an improvement in Bifidobacteria abundance and higher production of SCFAs. Concurrent literature has described the beneficial modulation of the gut microbiome by the different components of the MD. Interestingly, the intervention treatment of hydroxytyrosol, a main phytochemical in EVOO, has modulated the gut microbiota leading to a lower density of inflammation-related microbes and enhancing the presence of probiotics (49, 50). Recently, Rocchetti et al. reported that oleuropein derived from olive leaf extract and EVOO has improved gut microbiota and potentiated the growth of bacteria associated with healthy metabolic markers (368). (360) reported the anti-inflammatory effect of lycopene in DSS-induced colitis mice. The study suggested that lycopene reduced the expression of TNF-α, IL-1β, TLR-4, and iNOS as well as modulated the gut microbiome by decreasing the density of proteobacteria and improving the presence of Bifidobacterium and Lactobacillus (360). Furthermore, allicin altered the structure of the gut microbiota and raised the number of beneficial bacteria. Koch and Lawson found that allicin suppresses the development of Escherichia coli and Staphylococcus aureus (369). Allicin (100 mg/kg/d) dramatically increased the relative abundance of Ruminococcaceae, Clostridiales, Bacteroidales, and Facklamiaets while decreasing the relative abundance of Firmicutes, Corynebacteriales, and Lactobacillales (370). Dietary supplementation with 100 mg/kg of carvacrol–thymol (CV–TH) (1:1) blend as animal feed for 14 days reduced weaning-induced intestinal oxidative stress and inflammation in piglets by decreasing tumor necrosis factor mRNA levels. It is worth noting that the CV–TH blend increased the population of Lactobacillus species and decreased the populations of Enterococcus and E. coli (361). Moreover, dietary fiber consumption enhances the creation and maintenance of a healthy, viable, and diversified colonic microbiota, acting as prebiotics. Prebiotics are ingredients that are resistant to gastric acidity and hydrolysis by enzymes (295). On the other hand, β-sitosterol maintained gut microbiota compositions leading to the production of beneficial metabolites including SCFAs that promote tumor apoptosis (362). As well, pungent food, particularly Capsaicin, has a positive action on gut flora, by decreasing the disease-causing enteric pathogens and encouraging the growth of useful bacteria (371).
Chemopreventive effect of the Mediterranean diet and its components: Clinical studies
Despite the effectiveness of colonoscopy screening and recent improvements in cancer therapy, CRC remains one of the most prevalent and deadly types of cancer (372). Many studies have shown that a diet rich in fruits, vegetables, and tea is associated with lower rates of cancer, particularly colon cancer (373–375). In this context, a double-blind randomized clinical trial, case-control study, and meta-analysis revealed a substantial impact on colon disease and cancer patients. Fruit and deep-yellow vegetables, dark-green vegetables, onions, and garlic are moderately associated with a lower risk of colorectal adenoma, a precursor to CRC (376–378). Case-control studies generally revealed a lower risk of CRC with onion eating. In Argentina, the effect was more pronounced for consumption of a combination of garlic, onions, and pepper (379). In other case-control studies, the data points to a positive outcome. A food frequency questionnaire was used to analyze the intake of onions and garlic in a network of Italian and Swiss research that comprised 1037 cases and 2020 controls (380). The researchers discovered that onions and garlic were both protective against large bowel cancer. All levels of onion consumption were related to a lower risk of CRC. Also, Garlic usage at intermediate and high levels was linked to a lower risk of CRC. Several studies have indicated that flavonols, such as quercetin, can reduce the incidence of colon cancer with or without additional supplement therapy such as aspirin or NSAIDs (381–383). A dose-response meta-analysis revealed that an increase in dietary flavonols (such as quercetin) intake of 10 mg per day was significantly related to a reduced risk of CRC (383). Furthermore, taking 3.65 kg of garlic supplements per year for 2 years was connected with a lower incidence of colorectal adenoma, a precursor of CRC (375, 376). Epidemiological investigations of randomized controlled trials revealed that treatment of aged garlic extract reduced colon adenomas and CRC in patients with CRC via increasing NK cell activity (384). In a Japanese study, patients with both colorectal aberrant crypt foci (ACF) and colorectal polyps who were planning polypectomy had a double-blind, randomized controlled experiment to investigate the effectiveness of omega-3 FAs in humans. After a month of supplementation, EPA (2.7 g per day) was found to be more effective at inhibiting colorectal aberrant crypt foci than the placebo control group (385). Another clinical trial looked at how co-supplementing with vitamin D and omega-3 fatty acids affected inflammatory markers and the tumor marker CEA in chemotherapy-treated CRC patients. Eighty-one patients with stage I or stage II CRC were given two omega-3 fatty acid capsules and a 50,000 IU vitamin D soft gel once a week for 8 weeks. The findings demonstrated that, when compared to baseline, omega-3, vitamin D, and co-supplementation significantly reduced serum levels of TNF-, IL-1, IL-6, IL-8, and tumor marker CEA. In comparison to baseline, NF-kB activity was significantly reduced in the vitamin D and co-supplementation groups (386). Numerous clinical pilot investigations have demonstrated that resveratrol in high doses is generally safe. Twenty CRC patients received resveratrol before surgery, at doses of 0.5 g or 1.0 g taken orally for 8 days. According to the findings, resveratrol was well tolerated. In CRC resection tissue, resveratrol and its metabolites were discovered. Resveratrol (0.5 or 1.0 g) was sufficient to provide anticarcinogenic effects in colon cancers by reducing tumor cell proliferation by 5% (P < 0.005) (387) Furthermore, in nine patients with colon cancer and liver metastases, a daily injection of 5 g micronized resveratrol resulted in a 39% rise in cleaved caspase-3, a marker of apoptosis (388). In a double-blind, randomized, placebo-controlled study, tomato lycopene extract exhibited a chemopreventive effect in colon cancer patients (n = 56) via downregulation of insulin-like growth factor-1 levels (389). As well, an Italian case-control study confirmed that high adherence to MD can reduce CRC risk (390). However, more research involving human clinical studies is needed to prove the therapeutic effects of these phytochemical substances in the treatment of CRC.
Future prospects
The future of the MD is rather unclear, and the MD’s health-protective qualities might be lost even before we completely realize the activity of the chemicals and the processes by which health results are attained. To maximize the potential health benefits, it is also essential to pay closer attention to the preservation of traditional foods and a faithful reflection on cultural traditions and the MD diet. Many studies have shown that high adherence to the Mediterranean pattern could significantly reduce the incidence of CRC. Hence, the recommendation of these diet patterns is usually as chemopreventive and in particular, cases can be applied as a complementary treatment to reduce tumor recurrence or protect from second tumors in recovered patients. However, more clinical research is required to determine the suitable and effective food patterns that can be administrated in CRC cases either for prevention or as a therapy. Besides, focusing on investigating the molecular mechanisms of MD components and their phytochemicals will be essential to upgrading the complementary therapies to the rank of established anticancer agents.
Conclusion
The MD components are rich in phytochemicals with spectacular medicinal properties. It is believed that these components exert a nutritional synergy when consumed in combination. Many preclinical and clinical studies have demonstrated the cancer-preventive effects of the natural compounds involved in the MD patterns. Based on the collected facts in this review, these nutraceuticals could prevent CRC by either reducing inflammation or preserving a healthy microbiota in the intestine.
Author contributions
All authors listed have made a substantial, direct, and intellectual contribution to the work, and approved it for publication.
Conflict of interest
The authors declare that the research was conducted in the absence of any commercial or financial relationships that could be construed as a potential conflict of interest.
Publisher’s note
All claims expressed in this article are solely those of the authors and do not necessarily represent those of their affiliated organizations, or those of the publisher, the editors and the reviewers. Any product that may be evaluated in this article, or claim that may be made by its manufacturer, is not guaranteed or endorsed by the publisher.
References
1. Willett WC, Sacks F, Trichopoulou A, Drescher G, Ferro-Luzzi A, Helsing E, et al. Mediterranean diet pyramid: A cultural model for healthy eating. Am J Clin Nutr. (1995) 61:1402S–6S. doi: 10.1093/ajcn/61.6.1402S
2. Keys A. From Naples to seven countries–a sentimental journey. Prog Biochem Pharmacol. (1983) 19:1–30.
3. Keys A, Fidanza F, Scardi V, Bergami G, Keys MH, Di Lorenzo F. Studies on serum cholesterol and other characteristics of clinically healthy men in Naples. AMA Arch Intern Med. (1954) 93:328–36. doi: 10.1001/archinte.1954.00240270014002
4. Widmer RJ, Flammer AJ, Lerman LO, Lerman A. The Mediterranean diet, its components, and cardiovascular disease. Am J Med. (2015) 128:229–38. doi: 10.1016/j.amjmed.2014.10.014
5. Radd-Vagenas S, Kouris-Blazos A, Singh MF, Flood VM. Evolution of Mediterranean diets and cuisine: Concepts and definitions. Asia Pac J Clin Nutr. (2017) 26:749–63.
6. Vasilopoulou E, Georga K, Joergensen MB, Naska A, Trichopoulou A. The antioxidant properties of Greek foods and the flavonoid content of the Mediterranean menu. Curr Med Chem Immunol Endocr Metab Agents. (2005) 5:33–45. doi: 10.2174/1568013053005508
7. Fournier L-S. Intangible cultural heritage in France: From state culture to local development. Heritage Regimes State. (2013) 6:327. doi: 10.4000/books.gup.399
8. Dinu M, Pagliai G, Casini A, Sofi F. Mediterranean diet and multiple health outcomes: An umbrella review of meta-analyses of observational studies and randomised trials. Eur J Clin Nutr. (2018) 72:30–43. doi: 10.1038/ejcn.2017.58
9. Becerra-Tomás N, Blanco Mejía S, Viguiliouk E, Khan T, Kendall CW, Kahleova H, et al. Mediterranean diet, cardiovascular disease and mortality in diabetes: A systematic review and meta-analysis of prospective cohort studies and randomized clinical trials. Crit Rev Food Sci Nutr. (2020) 60:1207–27. doi: 10.1080/10408398.2019.1565281
10. Morze J, Danielewicz A, Przybyłowicz K, Zeng H, Hoffmann G, Schwingshackl L. An updated systematic review and meta-analysis on adherence to Mediterranean diet and risk of cancer. Eur J Nutr. (2021) 60:1561–86. doi: 10.1007/s00394-020-02346-6
11. Xi Y, Xu P. Global colorectal cancer burden in 2020 and projections to 2040. Transl Oncol. (2021) 14:101174. doi: 10.1016/j.tranon.2021.101174
12. Thomson CA, McCullough ML, Wertheim BC, Chlebowski RT, Martinez ME, Stefanick ML, et al. Nutrition and physical activity cancer prevention guidelines, cancer risk, and mortality in the women’s health initiative. Cancer Prev Res. (2014) 7:42–53. doi: 10.1158/1940-6207.CAPR-13-0258
13. Terzic J, Grivennikov S, Karin E, Karin M. Inflammation and colon cancer. Gastroenterology. (2010) 138:2101–14. doi: 10.1053/j.gastro.2010.01.058
14. Donovan MG, Selmin OI, Doetschman TC, Romagnolo DF. Mediterranean diet: Prevention of colorectal cancer. Front Nutr. (2017) 4:59. doi: 10.3389/fnut.2017.00059
15. Testa U, Pelosi E, Castelli G. Colorectal cancer: Genetic abnormalities, tumor progression, tumor heterogeneity, clonal evolution and tumor-initiating cells. Med Sci. (2018) 6:31. doi: 10.3390/medsci6020031
16. Schmitt M, Greten FR. The inflammatory pathogenesis of colorectal cancer. Nat Rev Immunol. (2021) 21:653–67. doi: 10.1038/s41577-021-00534-x
17. Malki A, ElRuz RA, Gupta I, Allouch A, Vranic S, Al Moustafa A-E. Molecular mechanisms of colon cancer progression and metastasis: Recent insights and advancements. Int J Mol Sci. (2020) 22:130. doi: 10.3390/ijms22010130
18. Nguyen HT, Duong HQ. The molecular characteristics of colorectal cancer: Implications for diagnosis and therapy. Oncol Lett. (2018) 16:9–18. doi: 10.3892/ol.2018.8679
19. Fearon ER. Molecular genetics of colorectal cancer. Annu Rev Pathol. (2011) 6:479–507. doi: 10.1146/annurev-pathol-011110-130235
20. Kang S, Na Y, Joung SY, Lee SI, Oh SC, Min BW. The significance of microsatellite instability in colorectal cancer after controlling for clinicopathological factors. Medicine. (2018) 97:e0019. doi: 10.1097/MD.0000000000010019
21. Raut JR, Guan Z, Schrotz-King P, Brenner H. Fecal DNA methylation markers for detecting stages of colorectal cancer and its precursors: A systematic review. Clin Epigenetics. (2020) 12:122. doi: 10.1186/s13148-020-00904-7
22. Shen L, Toyota M, Kondo Y, Lin E, Zhang L, Guo Y, et al. Integrated genetic and epigenetic analysis identifies three different subclasses of colon cancer. Proc Natl Acad Sci USA. (2007) 104:18654–9. doi: 10.1073/pnas.0704652104
23. Keum N, Giovannucci E. Global burden of colorectal cancer: Emerging trends, risk factors and prevention strategies. Nat Rev Gastroenterol Hepatol. (2019) 16:713–32. doi: 10.1038/s41575-019-0189-8
24. Hossain MS, Karuniawati H, Jairoun AA, Urbi Z, Ooi DJ, John A, et al. Colorectal cancer: A review of carcinogenesis, global epidemiology, current challenges, risk factors, preventive and treatment strategies. Cancers. (2022) 14:1732. doi: 10.3390/cancers14071732
25. Wang K, Karin M. Tumor-elicited inflammation and colorectal cancer. Adv Cancer Res. (2015) 128:173–96. doi: 10.1016/bs.acr.2015.04.014
26. Formica V, Cereda V, Nardecchia A, Tesauro M, Roselli M. Immune reaction and colorectal cancer: Friends or foes? World J Gastroenterol. (2014) 20:12407. doi: 10.3748/wjg.v20.i35.12407
27. Keller D, Windsor A, Cohen R, Chand M. Colorectal cancer in inflammatory bowel disease: Review of the evidence. Tech Coloproctol. (2019) 23:3–13. doi: 10.1007/s10151-019-1926-2
28. Schäfer M, Werner S. Cancer as an overhealing wound: An old hypothesis revisited. Nat Rev Mol Cell Biol. (2008) 9:628–38. doi: 10.1038/nrm2455
29. Meira LB, Bugni JM, Green SL, Lee C-W, Pang B, Borenshtein D, et al. DNA damage induced by chronic inflammation contributes to colon carcinogenesis in mice. J Clin Invest. (2008) 118:2516–25. doi: 10.1172/JCI35073
30. Brennan CA, Garrett WS. Gut microbiota, inflammation, and colorectal cancer. Annu Rev Microbiol. (2016) 70:395–411. doi: 10.1146/annurev-micro-102215-095513
31. Schwabe RF, Jobin C. The microbiome and cancer. Nat Rev Cancer. (2013) 13:800–12. doi: 10.1038/nrc3610
32. Wong SH, Yu J. Gut microbiota in colorectal cancer: Mechanisms of action and clinical applications. Nat Rev Gastroenterol Hepatol. (2019) 16:690–704. doi: 10.1038/s41575-019-0209-8
33. Grenham S, Clarke G, Cryan JF, Dinan TG. Brain–gut–microbe communication in health and disease. Front Physiol. (2011) 2:94. doi: 10.3389/fphys.2011.00094
34. Gopalakrishnan V, Helmink BA, Spencer CN, Reuben A, Wargo JA. The influence of the gut microbiome on cancer, immunity, and cancer immunotherapy. Cancer Cell. (2018) 33:570–80. doi: 10.1016/j.ccell.2018.03.015
35. Rebersek M. Gut microbiome and its role in colorectal cancer. BMC Cancer. (2021) 21:1325. doi: 10.1186/s12885-021-09054-2
36. Mazzocchi A, Leone L, Agostoni C, Pali-Schöll I. The secrets of the Mediterranean diet. Does [only] olive oil matter? Nutrients. (2019) 11:2941. doi: 10.3390/nu11122941
37. Gorzynik-Debicka M, Przychodzen P, Cappello F, Kuban-Jankowska A, Marino Gammazza A, Knap N, et al. Potential health benefits of olive oil and plant polyphenols. Int J Mol Sci. (2018) 19:686. doi: 10.3390/ijms19030686
38. Fernández AG, Adams MR, Fernandez-Diez MJ. Table olives: Production and processing. Berlin: Springer Science & Business Media (1997).
39. Borzì AM, Biondi A, Basile F, Luca S, Vicari ESD, Vacante M. Olive oil effects on colorectal cancer. Nutrients. (2019) 11:32. doi: 10.3390/nu11010032
40. Sain A, Sahu S, Naskar D. Potential of olive oil and its phenolic compounds as therapeutic intervention against colorectal cancer: a comprehensive review. Br J Nutr. (2021) 2:1–17. doi: 10.1017/S0007114521002919
41. Rodríguez-García C, Sánchez-Quesada C, Algarra I, Gaforio JJ. The high-fat diet based on extra-virgin olive oil causes dysbiosis linked to colorectal cancer prevention. Nutrients. (2020) 12:1705. doi: 10.3390/nu12061705
42. Millman JF, Okamoto S, Teruya T, Uema T, Ikematsu S, Shimabukuro M, et al. Extra-virgin olive oil and the gut-brain axis: Influence on gut microbiota, mucosal immunity, and cardiometabolic and cognitive health. Nutr Rev. (2021) 79:1362–74. doi: 10.1093/nutrit/nuaa148
43. Bonoli M, Montanucci M, Toschi TG, Lercker G. Fast separation and determination of tyrosol, hydroxytyrosol and other phenolic compounds in extra-virgin olive oil by capillary zone electrophoresis with ultraviolet-diode array detection. J Chromatogr A. (2003) 1011:163–72. doi: 10.1016/S0021-9673(03)01100-2
44. Medina E, De Castro A, Romero C, Brenes M. Comparison of the concentrations of phenolic compounds in olive oils and other plant oils: Correlation with antimicrobial activity. J Agric Food Chem. (2006) 54:4954–61. doi: 10.1021/jf0602267
45. Ghalandari M, Naghmachi M, Oliverio M, Nardi M, Shirazi HRG, Eilami O. Antimicrobial effect of hydroxytyrosol, hydroxytyrosol acetate and hydroxytyrosol oleate on staphylococcus aureus and staphylococcus epidermidis. Electron J Gen Med. (2018) 15:1–7. doi: 10.29333/ejgm/85686
46. Shan C, Miao F. Immunomodulatory and antioxidant effects of hydroxytyrosol in cyclophosphamide-induced immunosuppressed broilers. Poult Sci. (2022) 101:101516. doi: 10.1016/j.psj.2021.101516
47. Chen C, Wei Y-H. Potential role of hydroxytyrosol in neuroprotection. J Funct Foods. (2021) 82:104506. doi: 10.1016/j.jff.2021.104506
48. Zhang S-P, Zhou J, Fan Q-Z, Lv X-M, Wang T, Wang F, et al. Discovery of hydroxytyrosol as thioredoxin reductase 1 inhibitor to induce apoptosis and G 1/S cell cycle arrest in human colorectal cancer cells via ROS generation. Exp Ther Med. (2021) 22:1–13. doi: 10.3892/etm.2021.10261
49. Wang Q, Wang C, Abdullah, Tian W, Qiu Z, Song M, et al. Hydroxytyrosol alleviates dextran sulfate sodium-induced colitis by modulating inflammatory responses, intestinal barrier, and microbiome. J Agric Food Chem. (2022) 70:2241–52. doi: 10.1021/acs.jafc.1c07568
50. Miao F. Hydroxytyrosol alleviates dextran sodium sulfate–induced colitis by inhibiting NLRP3 inflammasome activation and modulating gut microbiota in vivo. Nutrition. (2022) 97:111579. doi: 10.1016/j.nut.2021.111579
51. Elmaksoud HAA, Motawea MH, Desoky AA, Elharrif MG, Ibrahimi A. Hydroxytyrosol alleviate intestinal inflammation, oxidative stress and apoptosis resulted in ulcerative colitis. Biomed Pharmacother. (2021) 142:112073. doi: 10.1016/j.biopha.2021.112073
52. Serreli G, Deiana M. Biological relevance of extra virgin olive oil polyphenols metabolites. Antioxidants. (2018) 7:170. doi: 10.3390/antiox7120170
53. Bertelli M, Kiani AK, Paolacci S, Manara E, Kurti D, Dhuli K, et al. Hydroxytyrosol: A natural compound with promising pharmacological activities. J Biotechnol. (2020) 309:29–33. doi: 10.1016/j.jbiotec.2019.12.016
54. Hormozi M, Marzijerani AS, Baharvand P. Effects of hydroxytyrosol on expression of apoptotic genes and activity of antioxidant enzymes in LS180 cells. Cancer Manag Res. (2020) 12:7913. doi: 10.2147/CMAR.S253591
55. Sun L, Luo C, Liu J. Hydroxytyrosol induces apoptosis in human colon cancer cells through ROS generation. Food Funct. (2014) 5:1909–14. doi: 10.1039/C4FO00187G
56. Peng W, Zhou Z, Zhong Y, Sun Y, Wang Y, Zhu Z, et al. Plasma activity of thioredoxin reductase as a novel biomarker in gastric cancer. Sci Rep. (2019) 9:1–11. doi: 10.1038/s41598-019-55641-6
57. Xu L, Zhao Y, Pan F, Zhu M, Yao L, Liu Y, et al. Inhibition of the Nrf2-TrxR axis sensitizes the drug-resistant chronic myelogenous leukemia cell line K562/G01 to imatinib treatments. Biomed Res Int. (2019) 2019:1–2. doi: 10.1155/2019/6502793
58. Terzuoli E, Giachetti A, Ziche M, Donnini S. Hydroxytyrosol, a product from olive oil, reduces colon cancer growth by enhancing epidermal growth factor receptor degradation. Mol Nutr Food Res. (2016) 60:519–29. doi: 10.1002/mnfr.201500498
59. Heidari M, Hosseinpourfeizi M, Sani NS, Dastmalchi N, Tehrani MSM, Soleyman-Nejad M, et al. Evaluation of the effect of PLGA-PAA nano-encapsulated hydroxytyrosol on inhibiting the colorectal cancer cell line HT-29 and underlying mechanism of action. J Basic Res Med Sci. (2021) 8: 41–8.
60. Amiot MJ, Fleuriet A, Macheix JJ. Importance and evolution of phenolic compounds in olive during growth and maturation. J Agric Food Chem. (1986) 34:823–6. doi: 10.1021/jf00071a014
61. Hassen I, Casabianca H, Hosni K. Biological activities of the natural antioxidant oleuropein: Exceeding the expectation – A mini-review. J Funct Foods. (2015) 18:926–40. doi: 10.1016/j.jff.2014.09.001
62. Zheng Y, Liu Z, Yang X, Liu L, Ahn KS. An updated review on the potential antineoplastic actions of oleuropein. Phytother Res. (2022) 36:365–79. doi: 10.1002/ptr.7325
63. Domitrović R, Jakovac H, Marchesi VV, Šain I, Romić Ž, Rahelić D. Preventive and therapeutic effects of oleuropein against carbon tetrachloride-induced liver damage in mice. Pharmacol Res. (2012) 65:451–64. doi: 10.1016/j.phrs.2011.12.005
64. Qabaha K, Al-Rimawi F, Qasem A, Naser SA. Oleuropein is responsible for the major anti-inflammatory effects of olive leaf extract. J Med Food. (2018) 21:302–5. doi: 10.1089/jmf.2017.0070
65. Hsu M-L, Huang W-C, Zhou Y-R, Hu S, Huang CH, Wu S-J. Oleuropein protects human retinal pigment epithelium cells from IL-1β–induced inflammation by blocking MAPK/NF-κB signaling pathways. Inflammation. (2022) 45:297–307. doi: 10.1007/s10753-021-01546-4
66. Urpi-Sarda M, Casas R, Chiva-Blanch G, Romero-Mamani ES, Valderas-Martínez P, Arranz S, et al. Virgin olive oil and nuts as key foods of the Mediterranean diet effects on inflammatory biomarkers related to atherosclerosis. Pharmacol Res. (2012) 65:577–83. doi: 10.1016/j.phrs.2012.03.006
67. Motawea MH, Abd Elmaksoud HA, Elharrif MG, Desoky AAE, Ibrahimi A. Evaluation of anti-inflammatory and antioxidant profile of oleuropein in experimentally induced ulcerative colitis. Int J Mol Cell Med. (2020) 9:224.
68. Huguet-Casquero A, Xu Y, Gainza E, Pedraz JL, Beloqui A. Oral delivery of oleuropein-loaded lipid nanocarriers alleviates inflammation and oxidative stress in acute colitis. Int J Pharm. (2020) 586:119515. doi: 10.1016/j.ijpharm.2020.119515
69. Vetuschi A, Battista N, Pompili S, Cappariello A, Prete R, Taticchi A, et al. The antiinflammatory and antifibrotic effect of olive phenols and Lactiplantibacillus plantarum IMC513 in dextran sodium sulfate–induced chronic colitis. Nutrition. (2022) 94:111511. doi: 10.1016/j.nut.2021.111511
70. Antoniou C, Hull J. The anti-cancer effect of Olea europaea L. products: A review. Curr Nutr Rep. (2021) 10:99–124. doi: 10.1007/s13668-021-00350-8
71. Cárdeno A, Sánchez-Hidalgo M, Rosillo MA, de la Lastra CA. Oleuropein, a secoiridoid derived from olive tree, inhibits the proliferation of human colorectal cancer cell through downregulation of HIF-1α. Nutr Cancer. (2013) 65:147–56. doi: 10.1080/01635581.2013.741758
72. Giner E, Recio MC, Ríos JL, Cerdá-Nicolás JM, Giner RM. Chemopreventive effect of oleuropein in colitis-associated colorectal cancer in c57bl/6 mice. Mol Nutr Food Res. (2016) 60:242–55. doi: 10.1002/mnfr.201500605
73. Gómez-Romero M, Arráez-Román D, Segura-Carretero A, Fernández-Gutiérrez A. Analytical determination of antioxidants in tomato: typical components of the Mediterranean diet. J Sep Sci. (2007) 30:452–61. doi: 10.1002/jssc.200600400
74. Bhowmik D, Kumar KPS, Paswan S, Srivastava S. Tomato-a natural medicine and its health benefits. J Pharmacogn Phytochem. (2012) 1:33–43.
75. de Alvarenga JFR, Lozano-Castellón J, Martínez-Huélamo M, Vallverdú-Queralt A, Lamuela-Raventós RM. Cooking practice and the matrix effect on the health properties of mediterranean diet: A study in tomato sauce. Advances in plant phenolics: From chemistry to human health. Washington, DC: ACS Publications (2018). p. 305–14. doi: 10.1021/bk-2018-1286.ch016
76. Chaudhary P, Sharma A, Singh B, Nagpal AK. Bioactivities of phytochemicals present in tomato. J Food Sci Technol. (2018) 55:2833–49. doi: 10.1007/s13197-018-3221-z
77. Arballo J, Amengual J, Erdman JW. Lycopene: A critical review of digestion, absorption, metabolism, and excretion. Antioxidants. (2021) 10:342. doi: 10.3390/antiox10030342
78. Meléndez-Martínez AJ, Stinco CM, Mapelli-Brahm P. Skin carotenoids in public health and nutricosmetics: The emerging roles and applications of the UV radiation-absorbing colourless carotenoids phytoene and phytofluene. Nutrients. (2019) 11:1093. doi: 10.3390/nu11051093
79. Kaliora AC, Dedoussis GVZ, Schmidt H. Dietary antioxidants in preventing atherogenesis. Atherosclerosis. (2006) 187:1–17. doi: 10.1016/j.atherosclerosis.2005.11.001
80. Reboul E. Absorption of vitamin A and carotenoids by the enterocyte: Focus on transport proteins. Nutrients. (2013) 5:3563–81. doi: 10.3390/nu5093563
81. Puah B-P, Jalil J, Attiq A, Kamisah Y. New insights into molecular mechanism behind anti-cancer activities of lycopene. Molecules. (2021) 26:3888. doi: 10.3390/molecules26133888
82. Costa-Rodrigues J, Pinho O, Monteiro PRR. Can lycopene be considered an effective protection against cardiovascular disease? Food Chem. (2018) 245:1148–53. doi: 10.1016/j.foodchem.2017.11.055
83. Tekeli İO, Ateşşahin A, Sakin F, Aslan A, Çeribaşı S, Yipel M. Protective effects of conventional and colon-targeted lycopene and linalool on ulcerative colitis induced by acetic acid in rats. Inflammopharmacology. (2019) 27:313–22. doi: 10.1007/s10787-018-0485-x
84. Hashem H, Hussein SA. Lycopene mitigates experimental colitis in rats by inhibiting oxidative stress-mediated inflammation and apoptosis. Benha Veterinary Med J. (2020) 39:16–21. doi: 10.21608/bvmj.2020.37614.1236
85. Li Y, Pan X, Yin M, Li C, Han L. Preventive effect of lycopene in dextran sulfate sodium-induced ulcerative colitis mice through the regulation of TLR4/TRIF/NF-κB signaling pathway and tight junctions. J Agric Food Chem. (2021) 69:13500–9. doi: 10.1021/acs.jafc.1c05128
86. Lin M-C, Wang F-Y, Kuo Y-H, Tang F-Y. Cancer chemopreventive effects of lycopene: Suppression of MMP-7 expression and cell invasion in human colon cancer cells. J Agric Food Chem. (2011) 59:11304–18. doi: 10.1021/jf202433f
87. Tang F-Y, Pai M-H, Wang X-D. Consumption of lycopene inhibits the growth and progression of colon cancer in a mouse xenograft model. J Agric Food Chem. (2011) 59:9011–21. doi: 10.1021/jf2017644
88. Zuzga DS, Gibbons AV, Li P, Lubbe WJ, Chervoneva I, Pitari GM. Overexpression of matrix metalloproteinase 9 in tumor epithelial cells correlates with colorectal cancer metastasis. Clin Transl Sci. (2008) 1:136–41. doi: 10.1111/j.1752-8062.2008.00037.x
89. Soleymani A, Shahrajabian MH. Changes in germination and seedling growth of different cultivars of cumin to drought stress. Cercetari Agronomice in Moldova. (2018) 1:91–100. doi: 10.2478/cerce-2018-0008
90. Shahrajabian MH, Sun W, Cheng Q. Clinical aspects and health benefits of ginger (Zingiber officinale) in both traditional Chinese medicine and modern industry. Acta Agric Scand. (2019) 69:546–56. doi: 10.1080/09064710.2019.1606930
91. Shahrajabian MH, Sun W, Cheng Q. A review of ginseng species in different regions as a multipurpose herb in traditional Chinese medicine, modern herbology and pharmacological science. J Med Plants Res. (2019) 13:213–26.
92. Shahrajabian MH, Sun W, Cheng Q. DNA methylation as the most important content of epigenetics in traditional Chinese herbal medicine. J Med Plants Res. (2019) 13:357–69. doi: 10.5897/JMPR2019.6803
93. Xu Y, Xu Z, Liu X, Cai L, Zheng H, Huang Y, et al. Clinical findings in critically ill patients infected with SARS-CoV-2 in Guangdong Province, China: A multi-center, retrospective, observational study. MedRxiv [Preprint] (2020) doi: 10.1101/2020.03.03.20030668
94. Corzo-Martínez M, Corzo N, Villamiel M. Biological properties of onions and garlic. Trends Food Sci Technol. (2007) 18:609–25. doi: 10.1016/j.tifs.2007.07.011
95. Nasri S, Anoush M, Khatami N. Evaluation of analgesic and anti-inflammatory effects of fresh onion juice in experimental animals. Afr J Pharmacy Pharmacol. (2012) 6:1679–84. doi: 10.5897/AJPP12.179
96. Akash MSH, Rehman K, Chen S. Spice plant Allium cepa: Dietary supplement for treatment of type 2 diabetes mellitus. Nutrition. (2014) 30:1128–37. doi: 10.1016/j.nut.2014.02.011
97. Bisen PS, Emerald M. Nutritional and therapeutic potential of garlic and onion (Allium sp.). Curr Nutr Food Sci. (2016) 12:190–9. doi: 10.2174/1573401312666160608121954
98. ThupstanTsewang VV, Acharya S, Kapila S. Onion-herbal medication and its applications. J Pharmacogn Phytochem. (2021) 10:1131–5.
100. Albishi T, John JA, Al-Khalifa AS, Shahidi F. Antioxidant, anti-inflammatory and DNA scission inhibitory activities of phenolic compounds in selected onion and potato varieties. J Funct Foods. (2013) 5:930–9. doi: 10.1016/j.jff.2013.02.005
101. Mlcek J, Valsikova M, Druzbikova H, Ryant P, Jurikova T, Sochor J, et al. The antioxidant capacity and macroelement content of several onion cultivars. Turk J Agric For. (2015) 39:999–1004. doi: 10.3906/tar-1501-71
102. Sami R, Alshehry G, Elgarni E, Helal M. Saudi community care awareness food facts, nutrients, immune system and COVID-19 prevention in taif city among different age categories. African J Food Agric Nutr Dev. (2021) 21:17213–33. doi: 10.18697/ajfand.96.20440
103. Sharma K, Mahato N, Nile SH, Lee ET, Lee YR. Economical and environmentally-friendly approaches for usage of onion (Allium cepa L.) waste. Food Funct. (2016) 7:3354–69. doi: 10.1039/C6FO00251J
104. Marefati N, Ghorani V, Shakeri F, Boskabady M, Kianian F, Rezaee R, et al. A review of anti-inflammatory, antioxidant, and immunomodulatory effects of Allium cepa and its main constituents. Pharm Biol. (2021) 59:287–302. doi: 10.1080/13880209.2021.1874028
105. Suleria HAR, Butt MS, Anjum FM, Saeed F, Khalid N. Onion: Nature protection against physiological threats. Crit Rev Food Sci Nutr. (2015) 55:50–66. doi: 10.1080/10408398.2011.646364
106. Shahrajabian MH, Sun W, Cheng Q. Chinese onion (Allium chinense), an evergreen vegetable: A brief review. J Agron. (2020) 42:40–5.
107. Dahlawi SM, Nazir W, Iqbal R, Asghar W, Khalid N. Formulation and characterization of oil-in-water nanoemulsions stabilized by crude saponins isolated from onion skin waste. RSC Adv. (2020) 10:39700–7. doi: 10.1039/D0RA07756A
108. Lanzotti V, Romano A, Lanzuise S, Bonanomi G, Scala F. Antifungal saponins from bulbs of white onion, Allium cepa L. Phytochemistry. (2012) 74:133–9. doi: 10.1016/j.phytochem.2011.11.008
109. Ma Y-L, Zhu D-Y, Thakur K, Wang C-H, Wang H, Ren Y-F, et al. Antioxidant and antibacterial evaluation of polysaccharides sequentially extracted from onion (Allium cepa L.). Int J Biol Macromol. (2018) 111:92–101. doi: 10.1016/j.ijbiomac.2017.12.154
110. Viera VB, Piovesan N, Rodrigues JB, de O Mello R, Prestes RC, Dos Santos RCV, et al. Extraction of phenolic compounds and evaluation of the antioxidant and antimicrobial capacity of red onion skin (Allium cepa L.). Int Food Res J. (2017) 24:990–9.
111. Ren F, Reilly K, Kerry JP, Gaffney M, Hossain M, Rai DK. Higher antioxidant activity, total flavonols, and specific quercetin glucosides in two different onion (Allium cepa L.) varieties grown under organic production: Results from a 6-year field study. J Agric Food Chem. (2017) 65:5122–32. doi: 10.1021/acs.jafc.7b01352
112. Newman R, Waterland N, Moon Y, Tou JC. Selenium biofortification of agricultural crops and effects on plant nutrients and bioactive compounds important for human health and disease prevention–a review. Plant Foods Hum Nutr. (2019) 74:449–60. doi: 10.1007/s11130-019-00769-z
113. Lee SG, Parks JS, Kang HW. Quercetin, a functional compound of onion peel, remodels white adipocytes to brown-like adipocytes. J Nutr Biochem. (2017) 42:62–71. doi: 10.1016/j.jnutbio.2016.12.018
114. Upadhyay RK. Nutritional and therapeutic potential of Allium vegetables. J Nutr Ther. (2017) 6:18–37. doi: 10.6000/1929-5634.2017.06.01.3
115. Sami R, Elhakem A, Alharbi M, Almatrafi M, Benajiba N, Ahmed Mohamed T, et al. In-vitro evaluation of the antioxidant and anti-inflammatory activity of volatile compounds and minerals in five different onion varieties. Separations. (2021) 8:57. doi: 10.3390/separations8050057
116. Naczk M, Shahidi F. Phenolics in cereals, fruits and vegetables: Occurrence, extraction and analysis. J Pharm Biomed Anal. (2006) 41:1523–42. doi: 10.1016/j.jpba.2006.04.002
117. Pareek S, Sagar NA, Sharma S, Kumar V. Onion (Allium cepa L.). Fruit and vegetable phytochemicals: Chemistry and human health. 2nd ed. Hoboken, NJ: John Wiley and Sons Ltd (2017). p. 1145–62. doi: 10.1002/9781119158042.ch58
118. De Greef D, Barton EM, Sandberg EN, Croley CR, Pumarol J, Wong TL, et al. Anticancer potential of garlic and its bioactive constituents: A systematic and comprehensive review. Semin Cancer Biol. (2021) 73:219–64. doi: 10.1016/j.semcancer.2020.11.020
119. Ghoran SH, Rahimi H, Kazemi A, Scognamiglio M, Naderian M, Iraji A, et al. Allium hooshidaryae (Alliaceae); chemical compositions, biological and ethnomedicine uses. J Ethnopharmacol. (2021) 274:113918. doi: 10.1016/j.jep.2021.113918
120. Zhao X-X, Lin F-J, Li H, Li H-B, Wu D-T, Geng F, et al. Recent advances in bioactive compounds, health functions, and safety concerns of onion (Allium cepa L.). Front Nutr. (2021) 8:669805. doi: 10.3389/fnut.2021.669805
121. Jakaria M, Azam S, Cho D-Y, Haque M, Kim I-S, Choi D-K. The methanol extract of Allium cepa L. protects inflammatory markers in LPS-induced BV-2 microglial cells and upregulates the antiapoptotic gene and antioxidant enzymes in N27-A cells. Antioxidants. (2019) 8:348. doi: 10.3390/antiox8090348
122. Benmalek Y, Yahia OA, Belkebir A, Fardeau M-L. Anti-microbial and anti-oxidant activities of Illicium verum, Crataegus oxyacantha ssp monogyna and Allium cepa red and white varieties. Bioengineered. (2013) 4:244–8. doi: 10.4161/bioe.24435
123. Ren K-W, Li Y-H, Wu G, Ren J-Z, Lu H-B, Li Z-M, et al. Quercetin nanoparticles display antitumor activity via proliferation inhibition and apoptosis induction in liver cancer cells. Int J Oncol. (2017) 50:1299–311. doi: 10.3892/ijo.2017.3886
124. Wang R, Yang L, Li S, Ye D, Yang L, Liu Q, et al. Quercetin inhibits breast cancer stem cells via downregulation of aldehyde dehydrogenase 1A1 (ALDH1A1), chemokine receptor type 4 (CXCR4), mucin 1 (MUC1), and epithelial cell adhesion molecule (EpCAM). Med Sci Monit. (2018) 24:412. doi: 10.12659/MSM.908022
125. Tsuboki J, Fujiwara Y, Horlad H, Shiraishi D, Nohara T, Tayama S, et al. Onionin A inhibits ovarian cancer progression by suppressing cancer cell proliferation and the protumour function of macrophages. Sci Rep. (2016) 6:1–11. doi: 10.1038/srep29588
126. Draelos ZD. The ability of onion extract gel to improve the cosmetic appearance of postsurgical scars. J Cosmet Dermatol. (2008) 7:101–4. doi: 10.1111/j.1473-2165.2008.00371.x
127. El-Aasr M, Fujiwara Y, Takeya M, Ikeda T, Tsukamoto S, Ono M, et al. Onionin A from Allium cepa inhibits macrophage activation. J Nat Prod. (2010) 73:1306–8. doi: 10.1021/np100105u
128. Colina-Coca C, González-Peña D, de Ancos B, Sánchez-Moreno C. Dietary onion ameliorates antioxidant defence, inflammatory response, and cardiovascular risk biomarkers in hypercholesterolemic Wistar rats. J Funct Foods. (2017) 36:300–9. doi: 10.1016/j.jff.2017.07.014
129. Samad N, Saleem A. Administration of Allium cepa L. bulb attenuates stress-produced anxiety and depression and improves memory in male mice. Metab Brain Dis. (2018) 33:271–81. doi: 10.1007/s11011-017-0159-1
130. Oliveira TT, Campos KM, Cerqueira-Lima AT, Cana Brasil Carneiro T, da Silva Velozo E, Ribeiro Melo ICA, et al. Potential therapeutic effect of Allium cepa L. and quercetin in a murine model of Blomia tropicalis induced asthma. DARU J Pharm Sci. (2015) 23:1–12. doi: 10.1186/s40199-015-0098-5
131. Loredana L, Giuseppina A, Filomena N, Florinda F, Marisa DM, Donatella A. Biochemical, antioxidant properties and antimicrobial activity of different onion varieties in the Mediterranean area. J Food Meas Charact. (2019) 13:1232–41. doi: 10.1007/s11694-019-00038-2
132. Lee B, Jung J-H, Kim H-S. Assessment of red onion on antioxidant activity in rat. Food Chem Toxicol. (2012) 50:3912–9. doi: 10.1016/j.fct.2012.08.004
133. Khajah MA, Orabi KY, Hawai S, Sary HG, El-Hashim AZ. Onion bulb extract reduces colitis severity in mice via modulation of colonic inflammatory pathways and the apoptotic machinery. J Ethnopharmacol. (2019) 241:112008. doi: 10.1016/j.jep.2019.112008
134. Lawrence DW, Shornick LP, Kornbluth J. Mice deficient in NKLAM have attenuated inflammatory cytokine production in a Sendai virus pneumonia model. PLoS One. (2019) 14:e0222802. doi: 10.1371/journal.pone.0222802
135. Yamaguchi M, Weitzmann MN. Quercetin, a potent suppressor of NF-κB and Smad activation in osteoblasts. Int J Mol Med. (2011) 28:521–5. doi: 10.3892/ijmm.2011.749
136. Boots AW, Drent M, de Boer VCJ, Bast A, Haenen GRMM. Quercetin reduces markers of oxidative stress and inflammation in sarcoidosis. Clin Nutr. (2011) 30:506–12. doi: 10.1016/j.clnu.2011.01.010
137. Rivera L, Morón R, Sánchez M, Zarzuelo A, Galisteo M. Quercetin ameliorates metabolic syndrome and improves the inflammatory status in obese zucker rats. Obesity. (2008) 16:2081–7. doi: 10.1038/oby.2008.315
138. Lanzotti V. The analysis of onion and garlic. J Chromatogr A. (2006) 1112:3–22. doi: 10.1016/j.chroma.2005.12.016
139. Umoh U, Umoh R, Enema O, Ekpo E, Ajibesin KK, Eseyin OA. Anti-inflammatory constituents of plants: A review. J Chem Pharm Res. (2019) 11:74–85.
140. Dong Y, Hou Q, Lei J, Wolf PG, Ayansola H, Zhang B. Quercetin alleviates intestinal oxidative damage induced by H2O2 via modulation of GSH: In vitro screening and in vivo evaluation in a colitis model of mice. ACS Omega. (2020) 5:8334–46. doi: 10.1021/acsomega.0c00804
141. Sak K. Site-specific anticancer effects of dietary flavonoid quercetin. Nutr Cancer. (2014) 66:177–93. doi: 10.1080/01635581.2014.864418
142. Maurya AK, Vinayak M. Quercetin regresses Dalton’s lymphoma growth via suppression of PI3K/AKT signaling leading to upregulation of p53 and decrease in energy metabolism. Nutr Cancer. (2015) 67:354–63. doi: 10.1080/01635581.2015.990574
143. Srivastava S, Somasagara RR, Hegde M, Nishana M, Tadi SK, Srivastava M, et al. Quercetin, a natural flavonoid interacts with DNA, arrests cell cycle and causes tumor regression by activating mitochondrial pathway of apoptosis. Sci Rep. (2016) 6:1–13. doi: 10.1038/srep24049
144. Brisdelli F, Coccia C, Cinque B, Cifone MG, Bozzi A. Induction of apoptosis by quercetin: Different response of human chronic myeloid (K562) and acute lymphoblastic (HSB-2) leukemia cells. Mol Cell Biochem. (2007) 296:137–49. doi: 10.1007/s11010-006-9307-3
145. Refolo MG, D’Alessandro R, Malerba N, Laezza C, Bifulco M, Messa C, et al. Anti proliferative and pro apoptotic effects of flavonoid quercetin are mediated by CB1 receptor in human colon cancer cell lines. J Cell Physiol. (2015) 230:2973–80. doi: 10.1002/jcp.25026
146. Kumar S, Pandey AK. Chemistry and biological activities of flavonoids: An overview. ScientificWorldJournal. (2013) 2013:162750. doi: 10.1155/2013/162750
147. Kim S-H, Choi K-C. Anti-cancer effect and underlying mechanism(s) of kaempferol, a phytoestrogen, on the regulation of apoptosis in diverse cancer cell models. Toxicol Res. (2013) 29:229–34. doi: 10.5487/TR.2013.29.4.229
148. Zhao Y, Fan D, Zheng Z-P, Li ETS, Chen F, Cheng K-W, et al. 8-C-(E-phenylethenyl)quercetin from onion/beef soup induces autophagic cell death in colon cancer cells through ERK activation. Mol Nutr Food Res. (2017) 61:1600437. doi: 10.1002/mnfr.201600437
149. Özsoy S, Becer E, Kabadayı H, Vatansever HS, Yücecan S. Quercetin-mediated apoptosis and cellular senescence in human colon cancer. Anticancer Agents Med Chem. (2020) 20:1387–96. doi: 10.2174/1871520620666200408082026
150. Han M, Song Y, Zhang X. Quercetin suppresses the migration and invasion in human colon cancer Caco-2 cells through regulating toll-like receptor 4/nuclear factor-kappa B pathway. Pharmacogn Mag. (2016) 12:S237. doi: 10.4103/0973-1296.182154
151. Roy S, Das R, Ghosh B, Chakraborty T. Deciphering the biochemical and molecular mechanism underlying the in vitro and in vivo chemotherapeutic efficacy of ruthenium quercetin complex in colon cancer. Mol Carcinog. (2018) 57:700–21. doi: 10.1002/mc.22792
152. Xu G, Shi H, Ren L, Gou H, Gong D, Gao X, et al. Enhancing the anti-colon cancer activity of quercetin by self-assembled micelles. Int J Nanomed. (2015) 10:2051. doi: 10.2147/IJN.S75550
153. Lin R, Piao M, Song Y, Liu C. Quercetin suppresses AOM/DSS-induced colon carcinogenesis through its anti-inflammation effects in mice. J Immunol Res. (2020) 2020:9242601. doi: 10.1155/2020/9242601
154. Aviello G, Abenavoli L, Borrelli F, Capasso R, Izzo AA, Lembo F, et al. Garlic: Empiricism or science? Nat Prod Commun. (2009) 4:1785–96. doi: 10.1177/1934578X0900401231
155. Ernst E. The efficacy of herbal medicine–an overview. Fundam Clin Pharmacol. (2005) 19:405–9. doi: 10.1111/j.1472-8206.2005.00335.x
156. Nouroz F, Mehboob M, Noreen S, Zaidi F, Mobin T. A review on anticancer activities of garlic (Allium sativum L.). Middle East J Sci Res. (2015) 23:1145–51.
157. Satyal P, Craft JD, Dosoky NS, Setzer WN. The chemical compositions of the volatile oils of garlic (Allium sativum) and wild garlic (Allium vineale). Foods. (2017) 6:63. doi: 10.3390/foods6080063
158. Singh R, Singh K. Garlic: A spice with wide medicinal actions. J Pharmacogn Phytochem. (2019) 8:1349–55.
159. Saif S, Hanif MA, Rehman R, Riaz M. Garlic. Medicinal plants of South Asia. Amsterdam: Elsevier (2020). p. 301–15. doi: 10.1016/B978-0-08-102659-5.00023-9
160. Borrelli F, Capasso R, Izzo AA. Garlic (Allium sativum L.): Adverse effects and drug interactions in humans. Mol Nutr Food Res. (2007) 51:1386–97. doi: 10.1002/mnfr.200700072
161. Khubber S, Hashemifesharaki R, Mohammadi M, Gharibzahedi SMT. Garlic (Allium sativum L.): A potential unique therapeutic food rich in organosulfur and flavonoid compounds to fight with COVID-19. Nutr J. (2020) 19:1–3. doi: 10.1186/s12937-020-00643-8
162. Gebreselema G, Mebrahtu G. Medicinal values of garlic: A review. Int J Med Med Sci. (2013) 5:401–8.
164. Mandal SK, Das A, Dey S, Sahoo U, Bose S, Bose A, et al. Bioactivities of allicin and related organosulfur compounds from garlic: Overview of the literature since 2010. Egypt J Chem. (2019) 62:1–11. doi: 10.21608/ejchem.2019.15787.1954
165. Lawson LD, Hunsaker SM. Allicin bioavailability and bioequivalence from garlic supplements and garlic foods. Nutrients. (2018) 10:812. doi: 10.3390/nu10070812
166. Sharifi-Rad J, Cristina Cirone Silva N, Jantwal A, Bhatt ID, Sharopov F, Cho WC, et al. Therapeutic potential of allicin-rich garlic preparations: Emphasis on clinical evidence toward upcoming drugs formulation. Appl Sci. (2019) 9:5555. doi: 10.3390/app9245555
167. Ishaque S, Asrhad A, Haider MA, Fatima F. Biosafety and biosecurity of lab and hospital acquired infections. Biol Clin Sci Res J. (2021) 2021:e008. doi: 10.54112/bcsrj.v2021i1.55
168. Bayan L, Koulivand PH, Gorji A. Garlic: A review of potential therapeutic effects. Avicenna J Phytomed. (2014) 4:1.
169. Nair KP. Garlic. Minor spices and condiments. New York, NY: Springer (2021). p. 23–37. doi: 10.1007/978-3-030-82246-0_3
170. Londhe VP, Gavasane AT, Nipate SS, Bandawane DD, Chaudhari PD. Role of garlic (Allium sativum) in various diseases: An overview. Angiogenesis. (2011) 12:13.
171. Chan W-JJ, McLachlan AJ, Luca EJ, Harnett JE. Garlic (Allium sativum L.) in the management of hypertension and dyslipidemia–A systematic review. J Herb Med. (2020) 19:100292. doi: 10.1016/j.hermed.2019.100292
172. Shinjyo N, Waddell G, Green J. A tale of two cinnamons: A comparative review of the clinical evidence of Cinnamomum verum and C. cassia as diabetes interventions. J Herb Med. (2020) 21:100342. doi: 10.1016/j.hermed.2020.100342
173. Zhu Y, Anand R, Geng X, Ding Y. A mini review: Garlic extract and vascular diseases. Neurol Res. (2018) 40:421–5. doi: 10.1080/01616412.2018.1451269
174. Matsutomo T. Potential benefits of garlic and other dietary supplements for the management of hypertension. Exp Ther Med. (2020) 19:1479–84. doi: 10.3892/etm.2019.8375
175. Chidinma O, Timothy OC, Samuel S, Isaac E, Hauwa S. Therapeutic effects of garlic: A review. Sci J Biol Life Sci. (2019) 1:2019. doi: 10.33552/SJBLS.2019.01.000502
177. Ansary J, Forbes-Hernández TY, Gil E, Cianciosi D, Zhang J, Elexpuru-Zabaleta M, et al. Potential health benefit of garlic based on human intervention studies: A brief overview. Antioxidants. (2020) 9:619. doi: 10.3390/antiox9070619
178. Ribeiro M, Alvarenga L, Cardozo LFMF, Chermut TR, Sequeira J, Moreira LdSG, et al. From the distinctive smell to therapeutic effects: Garlic for cardiovascular, hepatic, gut, diabetes and chronic kidney disease. Clin Nutr. (2021) 40:4807–19. doi: 10.1016/j.clnu.2021.03.005
179. Papu S, Jaivir S, Sweta S, Singh BR. Medicinal values of garlic (Allium sativum L.) in human life: An overview. Greener J Agric Sci. (2014) 4:265–80. doi: 10.15580/GJAS.2014.6.031914151
180. Sarvizadeh M, Hasanpour O, Ghale-Noie ZN, Mollazadeh S, Rezaei M, Pourghadamyari H, et al. Allicin and digestive system cancers: From chemical structure to its therapeutic opportunities. Front Oncol. (2021) 11:650256. doi: 10.3389/fonc.2021.650256
181. Schafer G, Kaschula CH. The immunomodulation and anti-inflammatory effects of garlic organosulfur compounds in cancer chemoprevention. Anticancer Agents Med Chem. (2014) 14:233–40. doi: 10.2174/18715206113136660370
182. Azab A, Nassar A, Azab AN. Anti-inflammatory activity of natural products. Molecules. (2016) 21:1321. doi: 10.3390/molecules21101321
183. Wang L, Hu Y, Song B, Xiong Y, Wang J, Chen D. Targeting JAK/STAT signaling pathways in treatment of inflammatory bowel disease. Inflamm Res. (2021) 70:753–64. doi: 10.1007/s00011-021-01482-x
184. Lee DY, Li H, Lim HJ, Lee HJ, Jeon R, Ryu J-H. Anti-inflammatory activity of sulfur-containing compounds from garlic. J Med Food. (2012) 15:992–9. doi: 10.1089/jmf.2012.2275
185. Yuan Y, Lu L, Bo N, Chaoyue Y, Haiyang Y. Allicin ameliorates intestinal barrier damage via microbiota-regulated short-chain fatty acids-TLR4/MyD88/NF-κB cascade response in acrylamide-induced rats. J Agric Food Chem. (2021) 69:12837–52. doi: 10.1021/acs.jafc.1c05014
186. Li C, Lun W, Zhao X, Lei S, Guo Y, Ma J, et al. Allicin alleviates inflammation of trinitrobenzenesulfonic acid-induced rats and suppresses P38 and JNK pathways in Caco-2 cells. Mediators Inflamm. (2015) 2015:434692. doi: 10.1155/2015/434692
187. Jakubikova J, Sedlak J. Garlic-derived organosulfides induce cytotoxicity, apoptosis, cell cycle arrest and oxidative stress in human colon carcinoma cell lines. Neoplasma. (2006) 53:191–9.
188. Bat-Chen W, Golan T, Peri I, Ludmer Z, Schwartz B. Allicin purified from fresh garlic cloves induces apoptosis in colon cancer cells via Nrf2. Nutr Cancer. (2010) 62:947–57. doi: 10.1080/01635581.2010.509837
189. Miroddi M, Calapai F, Calapai G. Potential beneficial effects of garlic in oncohematology. Mini Rev Med Chem. (2011) 11:461–72. doi: 10.2174/138955711795843293
190. Perez-Ortiz JM, Galan-Moya EM, de la Cruz-Morcillo MA, Rodriguez JF, Gracia I, Garcia MT, et al. Cost effective use of a thiosulfinate-enriched Allium sativum extract in combination with chemotherapy in colon cancer. Int J Mol Sci. (2020) 21:2766. doi: 10.3390/ijms21082766
191. Li X, Ni J, Tang Y, Wang X, Tang H, Li H, et al. Allicin inhibits mouse colorectal tumorigenesis through suppressing the activation of STAT3 signaling pathway. Nat Prod Res. (2019) 33:2722–5. doi: 10.1080/14786419.2018.1465425
192. Alagawany M, Farag MR, Salah AS, Mahmoud MA. The role of oregano herb and its derivatives as immunomodulators in fish. Rev Aquac. (2020) 12:2481–92. doi: 10.1111/raq.12453
193. Kintzios SE. Oregano. 2nd ed. In: KV Peter editor. Handbook of herbs and spices. (Cambridge: Wood head Publishing) (2012). p. 417–36. doi: 10.1533/9780857095688.417
195. Holland B, McCance RA, Widdowson EM, Unwin ID, Buss DH. Vegetables, herbs and spices: Fifth supplement to mccance and widdowson’s the composition of foods. London: Royal Society of Chemistry (1991).
196. Gutiérrez-Grijalva EP, Picos-Salas MA, Leyva-López N, Criollo-Mendoza MS, Vazquez-Olivo G, Heredia JB. Flavonoids and phenolic acids from oregano: Occurrence, biological activity and health benefits. Plants. (2017) 7:2. doi: 10.3390/plants7010002
197. El Gendy AN, Leonardi M, Mugnaini L, Bertelloni F, Ebani VV, Nardoni S, et al. Chemical composition and antimicrobial activity of essential oil of wild and cultivated Origanum syriacum plants grown in Sinai, Egypt. Ind Crops Prod. (2015) 67:201–7. doi: 10.1016/j.indcrop.2015.01.038
198. Cui S, Zhang S, Ge S, Xiong L, Sun Q. Green preparation and characterization of size-controlled nanocrystalline cellulose via ultrasonic-assisted enzymatic hydrolysis. Ind Crops Prod. (2016) 83:346–52. doi: 10.1016/j.indcrop.2016.01.019
199. Lagouri V, Boskou D. Nutrient antioxidants in oregano. Int J Food Sci Nutr. (1996) 47:493–7. doi: 10.3109/09637489609031878
200. Zaguła G, Fabisiak A, Bajcar M, Czernicka M, Saletnik B, Puchalski C. Mineral components analysis of selected dried herbs. Econtechmod. (2016) 5:127–32.
201. Gonçalves S, Moreira E, Grosso C, Andrade PB, Valentão P, Romano A. Phenolic profile, antioxidant activity and enzyme inhibitory activities of extracts from aromatic plants used in Mediterranean diet. J Food Sci Technol. (2017) 54:219–27. doi: 10.1007/s13197-016-2453-z
202. García-Pérez E, Noratto GD, García-Lara S, Gutiérrez-Uribe JA, Mertens-Talcott SU. Micropropagation Effect on the anti-carcinogenic activitiy of polyphenolics from mexican oregano (Poliomintha glabrescens Gray) in human colon cancer cells HT-29. Plant Foods Hum Nutr. (2013) 68:155–62. doi: 10.1007/s11130-013-0344-2
203. Chishti S, Kaloo ZA, Sultan P. Medicinal importance of genus Origanum: A review. J Pharmacogn Phytotherapy. (2013) 5:170–7.
204. Alekseeva M, Zagorcheva T, Atanassov I, Rusanov K. Origanum vulgare L.—a review on genetic diversity, cultivation, biological activities, and perspectives for molecular breeding. Bulg J Agric Sci. (2020) 26:1183–97.
205. Steinfeld B, Scott J, Vilander G, Marx L, Quirk M, Lindberg J, et al. The role of lean process improvement in implementation of evidence-based practices in behavioral health care. J Behav Health Serv Res. (2015) 42:504–18. doi: 10.1007/s11414-013-9386-3
206. Ocana-Fuentes A, Arranz-Gutierrez E, Senorans FJ, Reglero G. Supercritical fluid extraction of oregano (Origanum vulgare) essentials oils: Anti-inflammatory properties based on cytokine response on THP-1 macrophages. Food Chem Toxicol. (2010) 48:1568–75. doi: 10.1016/j.fct.2010.03.026
207. Koukoulitsa C, Zika C, Hadjipavlou–Litina D, Demopoulos VJ, Skaltsa H. Inhibitory effect of polar oregano extracts on aldose reductase and soybean lipoxygenase in vitro. Phytother Res. (2006) 20:605–6. doi: 10.1002/ptr.1901
208. Begum N, Keshetti S, Vattikuti UMR. Evaluation of in vitro anti-inflammatory and COX-2 inhibitory activity of leaves of Origanum vulgare. Pharma Innov. (2016) 5:18.
209. Alvarenga EM, Souza LKM, Araújo TSL, Nogueira KM, Sousa FBM, Araújo AR, et al. Carvacrol reduces irinotecan-induced intestinal mucositis through inhibition of inflammation and oxidative damage via TRPA1 receptor activation. Chem Biol Interact. (2016) 260:129–40. doi: 10.1016/j.cbi.2016.11.009
210. Lin L-Z, Mukhopadhyay S, Robbins RJ, Harnly JM. Identification and quantification of flavonoids of Mexican oregano (Lippia graveolens) by LC-DAD-ESI/MS analysis. J Food Compos Anal. (2007) 20:361–9. doi: 10.1016/j.jfca.2006.09.005
211. Aldisi SS, Jaganjac M, Eid AH, Goktepe I. Evaluation of apoptotic, antiproliferative, and antimigratory activity of Origanum syriacum against metastatic colon cancer cells. J Herbs Spices Med Plants. (2019) 25:202–17. doi: 10.1080/10496475.2019.1587674
212. Savini I, Arnone R, Catani MV, Avigliano L. Origanum vulgare induces apoptosis in human colon cancer Caco2 cells. Nutr Cancer. (2009) 61:381–9. doi: 10.1080/01635580802582769
213. Fan K, Li X, Cao Y, Qi H, Li L, Zhang Q, et al. Carvacrol inhibits proliferation and induces apoptosis in human colon cancer cells. AntiCancer Drugs. (2015) 26:813–23. doi: 10.1097/CAD.0000000000000263
214. Chauhan AK, Bahuguna A, Paul S, Kang SC. Thymol elicits HCT-116 colorectal carcinoma cell death through induction of oxidative stress. Anticancer Agents Med Chem. (2017) 17:1942–50. doi: 10.2174/1871520617666170327121228
215. Li G, He Y, Yao J, Huang C, Song X, Deng Y, et al. Angelicin inhibits human lung carcinoma A549 cell growth and migration through regulating JNK and ERK pathways. Oncol Rep. (2016) 36:3504–12. doi: 10.3892/or.2016.5166
216. Shahi T, Assadpour E, Jafari SM. Main chemical compounds and pharmacological activities of stigmas and tepals of ‘red gold’; saffron. Trends Food Sci Technol. (2016) 58:69–78. doi: 10.1016/j.tifs.2016.10.010
217. Charles DJ. Cinnamon. In: DJ Charles editor. Antioxidant properties of spices, herbs and other sources. (New York, NY: Springer New York) (2013). p. 231–43. doi: 10.1007/978-1-4614-4310-0_19
218. Ghorbani R, Koocheki A. Sustainable cultivation of Saffron in Iran. In: E Lichtfouse editor. Sustainable agriculture reviews. (Cham: Springer International Publishing) (2017). p. 169–203. doi: 10.1007/978-3-319-58679-3_6
219. Negbi M. Saffron cultivation : Past, present and future prospects. In: M Negbi editor. Saffron Crocus sativus L. (Amsterdam: Harwood Academic Publishers) (1999). p. 1–19. doi: 10.1201/9780203303665
220. Melnyk JP, Wang S, Marcone MF. Chemical and biological properties of the world’s most expensive spice: Saffron. Food Res Int. (2010) 43:1981–9. doi: 10.1016/j.foodres.2010.07.033
221. Dessein J. Territorialisation in practice. The case of saffron cultivation in Morocco. In: L Horlings, E Battaglini, J Dessein editors. Cultural sustainability and regional development, theories and practices of territorialsation. (London: Routledge) (2015). p. 108–24. doi: 10.4324/9781315737430
222. Mollazadeh H, Emami SA, Hosseinzadeh H. Razi’s Al-Hawi and saffron (Crocus sativus): A review. Iran J Basic Med Sci. (2015) 18:1153–66.
223. Hadizadeh F, Mohajeri SA, Seifi M. Extraction and purification of crocin from saffron stigmas employing a simple and efficient crystallization method. Pak J Biol Sci. (2010) 13:691–8. doi: 10.3923/pjbs.2010.691.698
224. Zheng C-J, Li L, Ma W-H, Han T, Qin L-P. Chemical constituents and bioactivities of the liposoluble fraction from different medicinal parts of Crocus sativus. Pharm Biol. (2011) 49:756–63. doi: 10.3109/13880209.2010.547206
225. Rameshrad M, Razavi BM, Hosseinzadeh H. Saffron and its derivatives, crocin, crocetin and safranal: A patent review. Expert Opin Ther Pat. (2018) 28:147–65. doi: 10.1080/13543776.2017.1355909
226. Srivastava R, Ahmed H, Dixit R. Dharamveer, Saraf S. Crocus sativus L.: A comprehensive review. Pharmacogn Rev. (2010) 4:200. doi: 10.4103/0973-7847.70919
227. Samarghandian S, Borji A. Anticarcinogenic effect of saffron (Crocus sativus L.) and its ingredients. Pharmacognosy Res. (2014) 6:99–107. doi: 10.4103/0974-8490.128963
228. Gregory MJ, Menary RC, Davies NW. Effect of drying temperature and air flow on the production and retention of secondary metabolites in saffron. J Agric Food Chem. (2005) 53:5969–75. doi: 10.1021/jf047989j
229. Lage M, Cantrell CL. Quantification of saffron (Crocus sativus L.) metabolites crocins, picrocrocin and safranal for quality determination of the spice grown under different environmental Moroccan conditions. Sci Hortic. (2009) 121:366–73. doi: 10.1016/j.scienta.2009.02.017
230. Rastgoo M, Hosseinzadeh H, Alavizadeh H, Abbasi A, Ayati Z, Jaafari MR. Antitumor activity of PEGylated nanoliposomes containing crocin in mice bearing C26 colon carcinoma. Planta Med. (2013) 79:447–51. doi: 10.1055/s-0032-1328363
231. Amin B, Malekzadeh M, Heidari MR, Hosseinzadeh H. Effect of Crocus sativus extracts and its active constituent safranal on the harmaline-induced tremor in mice. Iran J Basic Med Sci. (2015) 18:449–58.
232. Chermahini SH, Majid FAA, Sarmidi MR, Taghizadeh E, Salehnezhad S. Impact of saffron as an anti-cancer and anti-tumor herb. Afr J Pharmacy Pharmacol. (2010) 4:834–40.
233. Xing B, Li S, Yang J, Lin D, Feng Y, Lu J, et al. Phytochemistry, pharmacology, and potential clinical applications of saffron: A review. J Ethnopharmacol. (2021) 281:114555. doi: 10.1016/j.jep.2021.114555
234. Imenshahidi M, Razavi BM, Faal A, Gholampoor A, Mousavi SM, Hosseinzadeh H. The effect of chronic administration of saffron (Crocus sativus) stigma aqueous extract on systolic blood pressure in rats. Jundishapur J Nat Pharm Prod. (2013) 8:175–9. doi: 10.17795/jjnpp-12475
235. Giannoulaki P, Kotzakioulafi E, Chourdakis M, Hatzitolios A, Didangelos T. Impact of Crocus sativus L. on metabolic profile in patients with diabetes mellitus or metabolic syndrome: A systematic review. Nutrients. (2020) 12:1424. doi: 10.3390/nu12051424
236. Mehdizadeh R, Parizadeh M-R, Khooei A-R, Mehri S, Hosseinzadeh H. Cardioprotective effect of saffron extract and safranal in isoproterenol-induced myocardial infarction in wistar rats. Iran J Basic Med Sci. (2013) 16:56–63.
237. Boskabady M-H, Gholamnezhad Z, Khazdair M-R, Tavakol-Afshari J. Chapter 25 – Antiinflammatory and immunomodulatory effects of saffron and its derivatives. In: A Koocheki, M Khajeh-Hosseini editors. Saffron. (Sawston: Woodhead Publishing) (2020). p. 405–21. doi: 10.1016/B978-0-12-818638-1.00026-5
238. Ghaffari S, Roshanravan N. Saffron; an updated review on biological properties with special focus on cardiovascular effects. Biomed Pharmacother. (2019) 109:21–7. doi: 10.1016/j.biopha.2018.10.031
239. El-Maraghy SA, Rizk SM, Shahin NN. Gastroprotective effect of crocin in ethanol-induced gastric injury in rats. Chem Biol Interact. (2015) 229:26–35. doi: 10.1016/j.cbi.2015.01.015
240. Kawabata K, Tung NH, Shoyama Y, Sugie S, Mori T, Tanaka T. Dietary crocin inhibits colitis and colitis-associated colorectal carcinogenesis in male ICR mice. Evid Based Complement Altern Med. (2012) 2012:820415. doi: 10.1155/2012/820415
241. Kazi HA, Qian Z. Crocetin reduces TNBS-induced experimental colitis in mice by downregulation of NFkB. Saudi J Gastroenterol. (2009) 15:181. doi: 10.4103/1319-3767.54750
242. Nair SC, Kurumboor SK, Hasegawa JH. Saffron chemoprevention in biology and medicine: A review. Cancer Biotherapy. (1995) 10:257–64. doi: 10.1089/cbr.1995.10.257
243. Nair SC, Pannikar B, Panikkar KR. Antitumour activity of saffron (Crocus sativus). Cancer Lett. (1991) 57:109–14. doi: 10.1016/0304-3835(91)90203-T
244. Smith TA. Carotenoids and cancer : Prevention and potential therapy. Br J Biomed Sci. (1998) 55:268–75.
245. Abdullaev FI, Frenkel GD. Effect of saffron on cell colony formation and cellular nucleic acid and protein synthesis. Biofactors. (1992) 3:201–4.
246. Zhang Z, Wang C-Z, Wen X-D, Shoyama Y, Yuan C-S. Role of saffron and its constituents on cancer chemoprevention. Pharm Biol. (2013) 51:920–4. doi: 10.3109/13880209.2013.771190
247. Patel S, Sarwat M, Khan TH. Mechanism behind the anti-tumour potential of saffron (Crocus sativus L.): The molecular perspective. Crit Rev Oncol Hematol. (2017) 115:27–35. doi: 10.1016/j.critrevonc.2017.04.010
248. Aung HH, Wang CZ, Ni M, Fishbein A, Mehendale SR, Xie JT, et al. Crocin from Crocus sativus possesses significant anti-proliferation effects on human colorectal cancer cells. Exp Oncol. (2007) 29:175–80.
249. Li C-Y, Huang W-F, Wang Q-L, Wang F, Cai E, Hu B, et al. Crocetin induces cytotoxicity in colon cancer cells via p53-independent mechanisms. Asian Pac J Cancer Prev. (2012) 13:3757–61. doi: 10.7314/APJCP.2012.13.8.3757
250. Wang J, Ke Y, Shu T. Crocin has pharmacological effects against the pathological behavior of colon cancer cells by interacting with the STAT3 signaling pathway. Exp Ther Med. (2020) 19:1297–303. doi: 10.3892/etm.2019.8329
251. Amerizadeh F, Rezaei N, Rahmani F, Hassanian SM, Moradi-Marjaneh R, Fiuji H, et al. Crocin synergistically enhances the antiproliferative activity of 5-flurouracil through Wnt/PI3K pathway in a mouse model of colitis-associated colorectal cancer. J Cell Biochem. (2018) 119:10250–61. doi: 10.1002/jcb.27367
252. Andrade JM, Faustino C, Garcia C, Ladeiras D, Reis CP, Rijo P. Rosmarinus officinalis L.: An update review of its phytochemistry and biological activity. Future Sci OA. (2018) 4:FSO283. doi: 10.4155/fsoa-2017-0124
253. Ribeiro-Santos R, Carvalho-Costa D, Cavaleiro C, Costa HS, Albuquerque TG, Castilho MC, et al. A novel insight on an ancient aromatic plant: The rosemary (Rosmarinus officinalis L.). Trends Food Sci Technol. (2015) 45:355–68. doi: 10.1016/j.tifs.2015.07.015
254. Borges RS, Ortiz BLS, Pereira ACM, Keita H, Carvalho JCT. Rosmarinus officinalis essential oil: A review of its phytochemistry, anti-inflammatory activity, and mechanisms of action involved. J Ethnopharmacol. (2019) 229:29–45. doi: 10.1016/j.jep.2018.09.038
255. Moore J, Yousef M, Tsiani E. Anticancer effects of rosemary (Rosmarinus officinalis L.) extract and rosemary extract polyphenols. Nutrients. (2016) 8:731. doi: 10.3390/nu8110731
256. Mena P, Cirlini M, Tassotti M, Herrlinger KA, Dall’Asta C, Del Rio D. Phytochemical profiling of flavonoids, phenolic acids, terpenoids, and volatile fraction of a rosemary (Rosmarinus officinalis L.) extract. Molecules. (2016) 21:1576. doi: 10.3390/molecules21111576
257. Zagorchev P, Apostolova E, Kokova V, Peychev L. Activation of KCNQ channels located on the skeletal muscle membrane by retigabine and its influence on the maximal muscle force in rat muscle strips. Naunyn Schmiedebergs Arch Pharmacol. (2016) 389:439–46. doi: 10.1007/s00210-016-1211-0
258. González-Minero FJ, Bravo-Díaz L, Ayala-Gómez A. Rosmarinus officinalis L. (Rosemary): An ancient plant with uses in personal healthcare and cosmetics. Cosmetics. (2020) 7:77. doi: 10.3390/cosmetics7040077
259. Sharifi-Rad J, Ezzat SM, El Bishbishy MH, Mnayer D, Sharopov F, Kılıç CS, et al. Rosmarinus plants: Key farm concepts towards food applications. Phytother Res. (2020) 34:1474–518. doi: 10.1002/ptr.6622
260. Neves JA, Neves JA, Oliveira RdCM. Pharmacological and biotechnological advances with Rosmarinus officinalis L. Expert Opin Ther Pat. (2018) 28:399–413. doi: 10.1080/13543776.2018.1459570
261. de Oliveira JR, Camargo SEA, de Oliveira LD. Rosmarinus officinalis L. (rosemary) as therapeutic and prophylactic agent. J Biomed Sci. (2019) 26:5. doi: 10.1186/s12929-019-0499-8
262. Ghasemzadeh Rahbardar M, Hosseinzadeh H. Therapeutic effects of rosemary (Rosmarinus officinalis L.) and its active constituents on nervous system disorders. Iran J Basic Med Sci. (2020) 23:1100–12.
263. Jin B-R, Chung K-S, Hwang S, Hwang SN, Rhee K-J, Lee M, et al. Rosmarinic acid represses colitis-associated colon cancer: A pivotal involvement of the TLR4-mediated NF-κB-STAT3 axis. Neoplasia. (2021) 23:561–73. doi: 10.1016/j.neo.2021.05.002
264. Zhao L, Zhang Y, Liu G, Hao S, Wang C, Wang Y. Black rice anthocyanin-rich extract and rosmarinic acid, alone and in combination, protect against DSS-induced colitis in mice. Food Funct. (2018) 9:2796–808. doi: 10.1039/C7FO01490B
265. González-Vallinas M, Reglero G, Ramírez de Molina A. Rosemary (Rosmarinus officinalis L.) extract as a potential complementary agent in anticancer therapy. Nutr Cancer. (2015) 67:1223–31. doi: 10.1080/01635581.2015.1082110
266. Karthikkumar V, Sivagami G, Vinothkumar R, Rajkumar D, Nalini N. Modulatory efficacy of rosmarinic acid on premalignant lesions and antioxidant status in 1, 2-dimethylhydrazine induced rat colon carcinogenesis. Environ Toxicol Pharmacol. (2012) 34:949–58. doi: 10.1016/j.etap.2012.07.014
267. Kontogianni VG, Tomic G, Nikolic I, Nerantzaki AA, Sayyad N, Stosic-Grujicic S, et al. Phytochemical profile of Rosmarinus officinalis and Salvia officinalis extracts and correlation to their antioxidant and anti-proliferative activity. Food Chem. (2013) 136:120–9. doi: 10.1016/j.foodchem.2012.07.091
268. Benincá JP, Dalmarco JB, Pizzolatti MG, Fröde TS. Analysis of the anti-inflammatory properties of Rosmarinus officinalis L. in mice. Food Chem. (2011) 124:468–75. doi: 10.1016/j.foodchem.2010.06.056
269. Silva AM, Machado ID, Santin JR, de Melo IL, Pedrosa GV, Genovese MI, et al. Aqueous extract of Rosmarinus officinalis L. inhibits neutrophil influx and cytokine secretion. Phytother Res. (2015) 29:125–33. doi: 10.1002/ptr.5238
270. Gonzalez-Vallinas M, Molina S, Vicente G, Zarza V, Martin-Hernandez R, Garcia-Risco MR, et al. Expression of microRNA-15b and the glycosyltransferase GCNT3 correlates with antitumor efficacy of Rosemary diterpenes in colon and pancreatic cancer. PLoS One. (2014) 9:e98556. doi: 10.1371/journal.pone.0098556
271. Ibáñez C, Simó C, García-Cañas V, Gómez-Martínez Á, Ferragut JA, Cifuentes A. CE/LC-MS multiplatform for broad metabolomic analysis of dietary polyphenols effect on colon cancer cells proliferation. Electrophoresis. (2012) 33:2328–36. doi: 10.1002/elps.201200143
272. Allegra A, Tonacci A, Pioggia G, Musolino C, Gangemi S. Anticancer activity of Rosmarinus officinalis L.: Mechanisms of action and therapeutic potentials. Nutrients. (2020) 12:1739. doi: 10.3390/nu12061739
273. Jung K-J, Min K-J, Bae JH, Kwon TK. Carnosic acid sensitized TRAIL-mediated apoptosis through down-regulation of c-FLIP and Bcl-2 expression at the post translational levels and CHOP-dependent up-regulation of DR5, Bim, and PUMA expression in human carcinoma caki cells. Oncotarget. (2015) 6:1556. doi: 10.18632/oncotarget.2727
274. Manimaran A, Manoharan S, Karthikeyan S, Nirmal MR. Anti-cell proliferative, anti-inflammatory and anti-angiogenic potential of lupeol in 7, 12-dimethylbenz (a) anthracene induced hamster buccal pouch carcinogenesis. Br J Med Med Res. (2015) 6:587. doi: 10.9734/BJMMR/2015/14951
275. Manosroi J, Boonpisuttinant K, Manosroi W, Manosroi A. Anti-proliferative activities on HeLa cancer cell line of Thai medicinal plant recipes selected from MANOSROI II database. J Ethnopharmacol. (2012) 142:422–31. doi: 10.1016/j.jep.2012.05.012
276. Venkatachalam K, Gunasekaran S, Jesudoss VAS, Namasivayam N. The effect of rosmarinic acid on 1, 2-dimethylhydrazine induced colon carcinogenesis. Exp Toxicol Pathol. (2013) 65:409–18. doi: 10.1016/j.etp.2011.12.005
277. Jang Y-G, Hwang K-A, Choi K-C. Rosmarinic acid, a component of rosemary tea, induced the cell cycle arrest and apoptosis through modulation of HDAC2 expression in prostate cancer cell lines. Nutrients. (2018) 10:1784. doi: 10.3390/nu10111784
278. Shrestha S, Song YW, Kim H, Lee DS, Cho SK. Sageone, a diterpene from Rosmarinus officinalis, synergizes with cisplatin cytotoxicity in SNU-1 human gastric cancer cells. Phytomedicine. (2016) 23:1671–9. doi: 10.1016/j.phymed.2016.09.008
279. del Pilar Sánchez-Camargo A, Herrero M. Rosemary (Rosmarinus officinalis) as a functional ingredient: Recent scientific evidence. Curr Opin Food Sci. (2017) 14:13–9. doi: 10.1016/j.cofs.2016.12.003
280. Pérez-Sánchez A, Barrajón-Catalán E, Ruiz-Torres V, Agulló-Chazarra L, Herranz-López M, Valdés A, et al. Rosemary (Rosmarinus officinalis) extract causes ROS-induced necrotic cell death and inhibits tumor growth in vivo. Sci Rep. (2019) 9:1–11. doi: 10.1038/s41598-018-37173-7
281. Valdés A, García-Cañas V, Rocamora-Reverte L, Gómez-Martínez Á, Ferragut JA, Cifuentes A. Effect of rosemary polyphenols on human colon cancer cells: Transcriptomic profiling and functional enrichment analysis. Genes Nutr. (2013) 8:43–60. doi: 10.1007/s12263-012-0311-9
282. Ilhan N, Bektas I, Susam S, Ozercan IH. Protective effects of rosmarinic acid against azoxymethane-induced colorectal cancer in rats. J Biochem Mol Toxicol. (2022) 36:e22961. doi: 10.1002/jbt.22961
283. Joye IJ. Dietary fibre from whole grains and their benefits on metabolic health. Nutrients. (2020) 12:3045. doi: 10.3390/nu12103045
284. Barrett EM, Batterham MJ, Ray S, Beck EJ. Whole grain, bran and cereal fibre consumption and CVD: A systematic review. Br J Nutr. (2019) 121:914–37. doi: 10.1017/S000711451900031X
285. Fardet A. New hypotheses for the health-protective mechanisms of whole-grain cereals: What is beyond fibre? Nutr Res Rev. (2010) 23:65–134. doi: 10.1017/S0954422410000041
286. Johnson J, Wallace TC. Whole grains and their bioactives: Composition and health. Hoboken, NJ: John Wiley & Sons (2019). doi: 10.1002/9781119129486
287. Arendt EK, Zannini E. Cereal grains for the food and beverage industries. Amsterdam: Elsevier (2013). doi: 10.1533/9780857098924
288. Bach Knudsen KE, Lćrke HN, Hedemann MS, Nielsen TS, Ingerslev AK, Gundelund Nielsen DS, et al. Impact of diet-modulated butyrate production on intestinal barrier function and inflammation. Nutrients. (2018) 10:1499. doi: 10.3390/nu10101499
289. Li F, Huang H, Zhu F, Zhou X, Yang Z, Zhao X. A Mixture of Lactobacillus fermentum HFY06 and arabinoxylan ameliorates dextran sulfate sodium-induced acute ulcerative colitis in mice. J Inflamm Res. (2021) 14:6575. doi: 10.2147/JIR.S344695
290. McNabney S, Henagan T. Short chain fatty acids in the colon and peripheral tissues: A focus on butyrate, colon cancer. Obes Insulin Resist Nutr. (2017) 9:1348. doi: 10.3390/nu9121348
291. Żyła E, Dziendzikowska K, Gajewska M, Wilczak J, Harasym J, Gromadzka-Ostrowska J. Beneficial effects of oat beta-glucan dietary supplementation in colitis depend on its molecular weight. Molecules. (2019) 24:3591. doi: 10.3390/molecules24193591
292. Kopiasz Ł, Dziendzikowska K, Gajewska M, Wilczak J, Harasym J, Żyła E, et al. Time-dependent indirect antioxidative effects of oat beta-glucans on peripheral blood parameters in the animal model of colon inflammation. Antioxidants. (2020) 9:375. doi: 10.3390/antiox9050375
293. Oh H, Kim H, Lee DH, Lee A, Giovannucci EL, Kang S-S, et al. Different dietary fibre sources and risks of colorectal cancer and adenoma: A dose–response meta-analysis of prospective studies. Br J Nutr. (2019) 122:605–15. doi: 10.1017/S0007114519001454
294. Prasad KN, Bondy SC. Dietary fibers and their fermented short-chain fatty acids in prevention of human diseases. Bioact Carbohydr Diet Fibre. (2019) 17:100170. doi: 10.1016/j.bcdf.2018.09.001
295. Davani-Davari D, Negahdaripour M, Karimzadeh I, Seifan M, Mohkam M, Masoumi SJ, et al. Prebiotics: Definition, types, sources, mechanisms, and clinical applications. Foods. (2019) 8:92. doi: 10.3390/foods8030092
296. Bishehsari F, Engen PA, Preite NZ, Tuncil YE, Naqib A, Shaikh M, et al. Dietary fiber treatment corrects the composition of gut microbiota, promotes SCFA production, and suppresses colon carcinogenesis. Genes. (2018) 9:102. doi: 10.3390/genes9020102
297. Qi J, Yu J, Li Y, Luo J, Zhang C, Ou S, et al. Alternating consumption of β−glucan and quercetin reduces mortality in mice with colorectal cancer. Food Sci Nutr. (2019) 7:3273–85. doi: 10.1002/fsn3.1187
298. Kim M-J, Hong S-Y, Kim S-K, Cheong C, Park H-J, Chun H-K, et al. β-Glucan enhanced apoptosis in human colon cancer cells SNU-C4. Nutr Res Pract. (2009) 3:180–4. doi: 10.4162/nrp.2009.3.3.180
299. Elagizi A, Lavie CJ, O’keefe E, Marshall K, O’keefe JH, Milani RV. An update on omega-3 polyunsaturated fatty acids and cardiovascular health. Nutrients. (2021) 13:204. doi: 10.3390/nu13010204
300. Shibabaw T. Omega-3 polyunsaturated fatty acids: Anti-inflammatory and anti-hypertriglyceridemia mechanisms in cardiovascular disease. Mol Cell Biochem. (2021) 476:993–1003. doi: 10.1007/s11010-020-03965-7
301. Thomas A, Baillet M, Proust-Lima C, Féart C, Foubert-Samier A, Helmer C, et al. Blood polyunsaturated omega-3 fatty acids, brain atrophy, cognitive decline, and dementia risk. Alzheimers Dement. (2021) 17:407–16. doi: 10.1002/alz.12195
302. Shahidi F, Ambigaipalan P. Omega-3 polyunsaturated fatty acids and their health benefits. Annu Rev Food Sci Technol. (2018) 9:345–81. doi: 10.1146/annurev-food-111317-095850
303. Ishihara T, Yoshida M, Arita M. Omega-3 fatty acid-derived mediators that control inflammation and tissue homeostasis. Int Immunol. (2019) 31:559–67. doi: 10.1093/intimm/dxz001
304. Souza PR, Marques RM, Gomez EA, Colas RA, De Matteis R, Zak A, et al. Enriched marine oil supplements increase peripheral blood specialized pro-resolving mediators concentrations and reprogram host immune responses: A randomized double-blind placebo-controlled study. Circ Res. (2020) 126:75–90. doi: 10.1161/CIRCRESAHA.119.315506
305. Norris PC, Skulas-Ray AC, Riley I, Richter CK, Kris-Etherton PM, Jensen GL, et al. Identification of specialized pro-resolving mediator clusters from healthy adults after intravenous low-dose endotoxin and omega-3 supplementation: A methodological validation. Sci Rep. (2018) 8:1–13. doi: 10.1038/s41598-018-36679-4
306. Ilktac HY, Kiziltan G, Lanpir AD, Ozansoy M, Gunal MY, Togay SO, et al. Combined therapy with probiotic VSL# 3 and Omega-3 fatty acids attenuates colonic injury and inflammation in chronic DNBS-induced colitis in mice. Folia Biologica (Kraków). (2021) 69:135–46. doi: 10.3409/fb_70-1.05
307. Hussein S, Abd Elazem MB, Mostafa Ali HA. The potential anti-inflammatory effect of Omega-3 polyunsaturated fatty acids on experimentally induced ulcerative colitis in rats. Benha Veterinary Med J. (2019) 37:237–44. doi: 10.21608/bvmj.2019.13806.1022
308. Sulciner ML, Serhan CN, Gilligan MM, Mudge DK, Chang J, Gartung A, et al. Resolvins suppress tumor growth and enhance cancer therapy. J Exp Med. (2018) 215:115–40. doi: 10.1084/jem.20170681
309. Mukerjee S, Saeedan AS, Ansari M, Singh M. Polyunsaturated fatty acids mediated regulation of membrane biochemistry and tumor cell membrane integrity. Membranes. (2021) 11:479. doi: 10.3390/membranes11070479
310. Hawcroft G, Volpato M, Marston G, Ingram N, Perry S, Cockbain A, et al. The omega-3 polyunsaturated fatty acid eicosapentaenoic acid inhibits mouse MC-26 colorectal cancer cell liver metastasis via inhibition of PGE2-dependent cell motility. Br J Pharmacol. (2012) 166:1724–37. doi: 10.1111/j.1476-5381.2012.01882.x
311. Pfister E, Smith R, Lane MA. N-3 Polyunsaturated fatty acid ethyl esters decrease the invasion, but not the proliferation, of human colorectal cancer cells via a PI3K-dependent mechanism in vitro. Prostaglandins Leukot Essent Fatty Acids. (2021) 167:102273. doi: 10.1016/j.plefa.2021.102273
312. Zhang K, Hu Z, Qi H, Shi Z, Chang Y, Yao Q, et al. G-protein-coupled receptors mediate ω-3 PUFAs-inhibited colorectal cancer by activating the Hippo pathway. Oncotarget. (2016) 7:58315. doi: 10.18632/oncotarget.11089
313. Kalbkhani F, Sam MR. The effect of fish-oil derived eicosapentaenoic acid on cell proliferation and caspase-3 activity in human colorectal cancer cell line. Tehran Univ Med J. (2018) 76:374–9.
314. D’Eliseo D, Di Rocco G, Loria R, Soddu S, Santoni A, Velotti F. Epitelial-to-mesenchimal transition and invasion are upmodulated by tumor-expressed granzyme B and inhibited by docosahexaenoic acid in human colorectal cancer cells. J Exp Clin Cancer Res. (2016) 35:1–11. doi: 10.1186/s13046-016-0302-6
315. Hussain SZ, Naseer B, Qadri T, Fatima T, Bhat TA. Grapes (Vitis vinifera)—morphology, taxonomy, composition and health benefits. Fruits grown in highland regions of the himalayas. New York, NY: Springer (2021). p. 103–15. doi: 10.1007/978-3-030-75502-7_8
316. Tian B, Liu J. Resveratrol: A review of plant sources, synthesis, stability, modification and food application. J Sci Food Agric. (2020) 100:1392–404. doi: 10.1002/jsfa.10152
317. Ramalingam A, Santhanathas T, Shaukat Ali S, Zainalabidin S. Resveratrol supplementation protects against nicotine-induced kidney injury. Int J Environ Res Public Health. (2019) 16:4445. doi: 10.3390/ijerph16224445
318. Kaur A, Tiwari R, Tiwari G, Ramachandran V. Resveratrol: A vital therapeutic agent with multiple health benefits. Drug Res. (2022) 72:5–17. doi: 10.1055/a-1555-2919
319. Fuggetta MP, Bordignon V, Cottarelli A, Macchi B, Frezza C, Cordiali-Fei P, et al. Downregulation of proinflammatory cytokines in HTLV-1-infected T cells by resveratrol. J Exp Clin Cancer Res. (2016) 35:1–9. doi: 10.1186/s13046-016-0398-8
320. Meng T, Xiao D, Muhammed A, Deng J, Chen L, He J. Anti-inflammatory action and mechanisms of resveratrol. Molecules. (2021) 26:229. doi: 10.3390/molecules26010229
321. Shi Y, Zhou J, Jiang B, Miao M. Resveratrol and inflammatory bowel disease. Ann N Y Acad Sci. (2017) 1403:38–47. doi: 10.1111/nyas.13426
322. Ren Z, Wang L, Cui J, Huoc Z, Xue J, Cui H, et al. Resveratrol inhibits NF-κB signaling through suppression of p65 and IB kinase activities. Die Pharmazie Int J Pharmaceutical Sci. (2013) 68:689–94.
323. Li F, Han Y, Cai X, Gu M, Sun J, Qi C, et al. Dietary resveratrol attenuated colitis and modulated gut microbiota in dextran sulfate sodium-treated mice. Food Funct. (2020) 11:1063–73. doi: 10.1039/C9FO01519A
324. Banc R, Socaciu C, Miere D, Filip L, Cozma A, Stanciu O, et al. Benefits of wine polyphenols on human health: A review. Bull Uasvm Food Sci Technol. (2014) 71:79–87. doi: 10.15835/buasvmcn-fst:10860
325. Farrand L, Byun S, Kim JY, Im-Aram A, Lee J, Lim S, et al. Piceatannol enhances cisplatin sensitivity in ovarian cancer via modulation of p53, X-linked inhibitor of apoptosis protein (XIAP), and mitochondrial fission. J Biol Chem. (2013) 288:23740–50. doi: 10.1074/jbc.M113.487686
326. Baek SH, Ko J-H, Lee H, Jung J, Kong M, Lee J-w, et al. Resveratrol inhibits STAT3 signaling pathway through the induction of SOCS-1: Role in apoptosis induction and radiosensitization in head and neck tumor cells. Phytomedicine. (2016) 23:566–77. doi: 10.1016/j.phymed.2016.02.011
327. Rauf A, Imran M, Butt MS, Nadeem M, Peters DG, Mubarak MS. Resveratrol as an anti-cancer agent: A review. Crit Rev Food Sci Nutr. (2018) 58:1428–47. doi: 10.1080/10408398.2016.1263597
328. Fu Y, Ye Y, Zhu G, Xu Y, Sun J, Wu H, et al. Resveratrol induces human colorectal cancer cell apoptosis by activating the mitochondrial pathway via increasing reactive oxygen species. Mol Med Rep. (2021) 23:170. doi: 10.3892/mmr.2020.11809
329. Li R, Ma X, Song Y, Zhang Y, Xiong W, Li L, et al. Anti-colorectal cancer targets of resveratrol and biological molecular mechanism: Analyses of network pharmacology, human and experimental data. J Cell Biochem. (2019) 120:11265–73. doi: 10.1002/jcb.28404
330. Moreira H, Szyjka A, Grzesik J, Pelc K, Żuk M, Kulma A, et al. Celastrol and resveratrol modulate SIRT genes expression and exert anticancer activity in colon cancer cells and cancer stem-like cells. Cancers. (2022) 14:1372. doi: 10.3390/cancers14061372
331. Ji Q, Liu X, Fu X, Zhang L, Sui H, Zhou L, et al. Resveratrol inhibits invasion and metastasis of colorectal cancer cells via MALAT1 mediated Wnt/β-catenin signal pathway. PLoS One. (2013) 8:e78700. doi: 10.1371/journal.pone.0078700
332. Bottone A, Cerulli A, D’Urso G, Masullo M, Montoro P, Napolitano A, et al. Plant specialized metabolites in hazelnut (Corylus avellana) kernel and byproducts: An update on chemistry, biological activity, and analytical aspects. Planta Med. (2019) 85:840–55. doi: 10.1055/a-0947-5725
334. Silvestri C, Bacchetta L, Bellincontro A, Cristofori V. Advances in cultivar choice, hazelnut orchard management, and nut storage to enhance product quality and safety: An overview. J Sci Food Agric. (2021) 101:27–43. doi: 10.1002/jsfa.10557
335. Antunes F, Erustes AG, Costa AJ, Nascimento AC, Bincoletto C, Ureshino RP, et al. Autophagy and intermittent fasting: The connection for cancer therapy? Clinics. (2018) 73:e814s. doi: 10.6061/clinics/2018/e814s
336. Yin Y, Liu X, Liu J, Cai E, Zhu H, Li H, et al. Beta-sitosterol and its derivatives repress lipopolysaccharide/d-galactosamine-induced acute hepatic injury by inhibiting the oxidation and inflammation in mice. Bioorg Med Chem Lett. (2018) 28:1525–33. doi: 10.1016/j.bmcl.2018.03.073
337. Bin Sayeed MS, Karim SMR, Sharmin T, Morshed MM. Critical analysis on characterization, systemic effect, and therapeutic potential of beta-sitosterol: A plant-derived orphan phytosterol. Medicines. (2016) 3:29. doi: 10.3390/medicines3040029
338. Khan SL, Siddiqui FA. Beta-Sitosterol: As immunostimulant, antioxidant and inhibitor of SARS-CoV-2 spike glycoprotein. Arch Pharmacol Thera. (2020) 2:1–5. doi: 10.33696/Pharmacol.2.014
339. Feng S, Dai Z, Liu A, Wang H, Chen J, Luo Z, et al. β-Sitosterol and stigmasterol ameliorate dextran sulfate sodium-induced colitis in mice fed a high fat Western-style diet. Food Funct. (2017) 8:4179–86. doi: 10.1039/C7FO00375G
340. Lee I-A, Kim E-J, Kim D-H. Inhibitory effect of β-sitosterol on TNBS-induced colitis in mice. Planta Med. (2012) 78:896–8. doi: 10.1055/s-0031-1298486
341. Ding K, Tan YY, Ding Y, Fang Y, Yang X, Fang J, et al. β−Sitosterol improves experimental colitis in mice with a target against pathogenic bacteria. J Cell Biochem. (2019) 120:5687–94. doi: 10.1002/jcb.27853
342. Alvarez-Sala A, Attanzio A, Tesoriere L, Garcia-Llatas G, Barberá R, Cilla A. Apoptotic effect of a phytosterol-ingredient and its main phytosterol (β-sitosterol) in human cancer cell lines. Int J Food Sci Nutr. (2019) 70:323–34. doi: 10.1080/09637486.2018.1511689
343. Shathviha PC, Ezhilarasan D, Rajeshkumar S, Selvaraj J. β-sitosterol mediated silver nanoparticles induce cytotoxicity in human colon cancer HT-29 cells. Avicenna J Med Biotechnol. (2021) 13:42.
344. Wang Z, Zhan Y, Xu J, Wang Y, Sun M, Chen J, et al. β-sitosterol reverses multidrug resistance via BCRP suppression by inhibiting the p53–MDM2 interaction in colorectal cancer. J Agric Food Chem. (2020) 68:3850–8. doi: 10.1021/acs.jafc.0c00107
345. Shen C-Y, Lee C-F, Chou W-T, Hwang J-J, Tyan Y-S, Chuang H-Y. Liposomal &beta-sitosterol suppresses metastasis of CT26/luc colon carcinoma via inhibition of MMP-9 and evoke of immune system. Pharmaceutics. (2022) 14:1214. doi: 10.3390/pharmaceutics14061214
346. de la Cruz-López KG, Castro-Muñoz LJ, Reyes-Hernández DO, García-Carrancá A, Manzo-Merino J. Lactate in the regulation of tumor microenvironment and therapeutic approaches. Front Oncol. (2019) 9:1143. doi: 10.3389/fonc.2019.01143
347. Bin Sayeed MS, Ameen SS. Beta-sitosterol: A promising but orphan nutraceutical to fight against cancer. Nutr Cancer. (2015) 67:1216–22. doi: 10.1080/01635581.2015.1087042
348. Marín A, Ferreres F, Tomás-Barberán FA, Gil MI. Characterization and quantitation of antioxidant constituents of sweet pepper (Capsicum annuum L.). J Agric Food Chem. (2004) 52:3861–9. doi: 10.1021/jf0497915
349. Matsufuji H, Ishikawa K, Nunomura O, Chino M, Takeda M. Anti-oxidant content of different coloured sweet peppers, white, green, yellow, orange and red (Capsicum annuum L.). Int J Food Sci Technol. (2007) 42:1482–8. doi: 10.1111/j.1365-2621.2006.01368.x
350. Matus Z, Deli J, Szabolcs J. Carotenoid composition of yellow pepper during ripening: Isolation of. Beta.-cryptoxanthin 5, 6-epoxide. J Agric Food Chem. (1991) 39:1907–14. doi: 10.1021/jf00011a003
351. Mejia L, Hudson E, de Mejia EG, Vazquez F. Carotenoid content and vitamin A activity of some common cultivars of Mexican peppers (Caps&m annuum) as determined by HPLC. J Food Sci. (1988) 53:1440–3. doi: 10.1111/j.1365-2621.1988.tb09295.x
352. Palevitch D, Craker L. Nutritional and medical importance of red pepper (Capsicum spp.). J Herbs Spices Med Plants. (1996) 3:55–83. doi: 10.1300/J044v03n02_08
353. Daood HG, Vinkler M, Markus F, Hebshi E, Biacs P. Antioxidant vitamin content of spice red pepper (paprika) as affected by technological and varietal factors. Food Chem. (1996) 55:365–72. doi: 10.1016/0308-8146(95)00136-0
354. Perry L, Dickau R, Zarrillo S, Holst I, Pearsall DM, Piperno DR, et al. Starch fossils and the domestication and dispersal of chili peppers (Capsicum spp. L.) in the Americas. Science. (2007) 315:986–8. doi: 10.1126/science.1136914
355. Chen Y-S, Lian YZ, Chao J. Protective effect of Lycium barbarum polysaccharides and capsaicin in rats with dextran sulfate sodium-induced ulcerative colitis via anti-inflammation and antioxidation. Curr Dev Nutr. (2021) 5:306. doi: 10.1093/cdn/nzab037_016
356. Chen Y-S, Lian YZ, Chen W-C, Chang C-C, Tinkov AA, Skalny AV, et al. Lycium barbarum polysaccharides and capsaicin inhibit oxidative stress, inflammatory responses, and pain signaling in rats with dextran sulfate sodium-induced colitis. Int J Mol Sci. (2022) 23:2423. doi: 10.3390/ijms23052423
357. Wan H, Chen XY, Zhang F, Chen J, Chu F, Sellers ZM, et al. Capsaicin inhibits intestinal Cl-secretion and promotes Na+ absorption by blocking TRPV4 channels in healthy and colitic mice. J Biol Chem. (2022) 298:101847. doi: 10.1016/j.jbc.2022.101847
358. Jin J, Lin G, Huang H, Xu D, Yu H, Ma X, et al. Capsaicin mediates cell cycle arrest and apoptosis in human colon cancer cells via stabilizing and activating p53. Int J Biol Sci. (2014) 10:285. doi: 10.7150/ijbs.7730
359. Clark R, Lee J, Lee S-H. Synergistic anticancer activity of capsaicin and 3, 3’-diindolylmethane in human colorectal cancer. J Agric Food Chem. (2015) 63:4297–304. doi: 10.1021/jf506098s
360. Zhao B, Wu J, Li J, Bai Y, Luo Y, Ji B, et al. Lycopene alleviates DSS-induced colitis and behavioral disorders via mediating microbes-gut–brain axis balance. J Agric Food Chem. (2020) 68:3963–75. doi: 10.1021/acs.jafc.0c00196
361. Wei HK, Xue HX, Zhou ZX, Peng J. A carvacrol–thymol blend decreased intestinal oxidative stress and influenced selected microbes without changing the messenger RNA levels of tight junction proteins in jejunal mucosa of weaning piglets. Animal. (2017) 11:193–201. doi: 10.1017/S1751731116001397
362. Ma H, Yu Y, Wang M, Li Z, Xu H, Tian C, et al. Correlation between microbes and colorectal cancer: Tumor apoptosis is induced by sitosterols through promoting gut microbiota to produce short-chain fatty acids. Apoptosis. (2019) 24:168–83. doi: 10.1007/s10495-018-1500-9
363. Wu GD, Compher C, Chen EZ, Smith SA, Shah RD, Bittinger K, et al. Comparative metabolomics in vegans and omnivores reveal constraints on diet-dependent gut microbiota metabolite production. Gut. (2016) 65:63–72. doi: 10.1136/gutjnl-2014-308209
364. Merra G, Noce A, Marrone G, Cintoni M, Tarsitano MG, Capacci A, et al. Influence of mediterranean diet on human gut microbiota. Nutrients. (2020) 13:7. doi: 10.3390/nu13010007
365. De Filippis F, Pellegrini N, Vannini L, Jeffery IB, La Storia A, Laghi L, et al. High-level adherence to a Mediterranean diet beneficially impacts the gut microbiota and associated metabolome. Gut. (2016) 65:1812–21. doi: 10.1136/gutjnl-2015-309957
366. Mitsou EK, Kakali A, Antonopoulou S, Mountzouris KC, Yannakoulia M, Panagiotakos DB, et al. Adherence to the Mediterranean diet is associated with the gut microbiota pattern and gastrointestinal characteristics in an adult population. Br J Nutr. (2017) 117:1645–55. doi: 10.1017/S0007114517001593
367. Garcia-Mantrana I, Selma-Royo M, Alcantara C, Collado MC. Shifts on gut microbiota associated to mediterranean diet adherence and specific dietary intakes on general adult population. Front Microbiol. (2018) 9:890. doi: 10.3389/fmicb.2018.00890
368. Rocchetti G, Luisa Callegari M, Senizza A, Giuberti G, Ruzzolini J, Romani A, et al. Oleuropein from olive leaf extracts and extra-virgin olive oil provides distinctive phenolic profiles and modulation of microbiota in the large intestine. Food Chem. (2022) 380:132187. doi: 10.1016/j.foodchem.2022.132187
369. Borlinghaus J, Albrecht F, Gruhlke MCH, Nwachukwu ID, Slusarenko AJ. Allicin: Chemistry and biological properties. Molecules. (2014) 19:12591–618. doi: 10.3390/molecules190812591
370. Shi X, Zhou X, Chu X, Wang J, Xie B, Ge J, et al. Allicin improves metabolism in high-fat diet-induced obese mice by modulating the gut microbiota. Nutrients. (2019) 11:2909. doi: 10.3390/nu11122909
371. Wang F, Huang X, Chen Y, Zhang D, Chen D, Chen L, et al. Study on the effect of capsaicin on the intestinal flora through high-throughput sequencing. ACS Omega. (2020) 5:1246–53. doi: 10.1021/acsomega.9b03798
372. Hughes LAE, Simons CCJM, van den Brandt PA, van Engeland M, Weijenberg MP. Lifestyle, diet, and colorectal cancer risk according to (epi) genetic instability: Current evidence and future directions of molecular pathological epidemiology. Curr Colorectal Cancer Rep. (2017) 13:455–69. doi: 10.1007/s11888-017-0395-0
373. Gu M-J, Huang Q-C, Bao C-Z, Li Y-J, Li X-Q, Ye D, et al. Attributable causes of colorectal cancer in China. BMC Cancer. (2018) 18:38. doi: 10.1186/s12885-017-3968-z
374. Feng Y-L, Shu L, Zheng P-F, Zhang X-Y, Si C-J, Yu X-L, et al. Dietary patterns and colorectal cancer risk: A meta-analysis. Eur J Cancer Prev. (2017) 26:201–11. doi: 10.1097/CEJ.0000000000000245
375. Cirmi S, Navarra M, Woodside JV, Cantwell MM. Citrus fruits intake and oral cancer risk: A systematic review and meta-analysis. Pharmacol Res. (2018) 133:187–94. doi: 10.1016/j.phrs.2018.05.008
376. Dreher ML. Dietary patterns and whole plant foods in aging and disease. New York, NY: Springer (2018). doi: 10.1007/978-3-319-59180-3
377. Wu X, Shi J, Fang WX, Guo XY, Zhang LY, Liu YP, et al. Allium vegetables are associated with reduced risk of colorectal cancer: A hospital-based matched case-control study in China. Asia Pac J Clin Oncol. (2019) 15:e132–41. doi: 10.1111/ajco.13133
378. Ngo SNT, Williams DB, Cobiac L, Head RJ. Does garlic reduce risk of colorectal cancer? A systematic review. J Nutr. (2007) 137:2264–9. doi: 10.1093/jn/137.10.2264
379. Iscovich JM, L’Abbe KA, Castelleto R, Calzona A, Bernedo A, Chopita NA, et al. Colon cancer in Argentina. I: Risk from intake of dietary items. Int J Cancer. (1992) 51:851–7. doi: 10.1002/ijc.2910510603
380. Simons CCJM, Hughes LAE, Arts ICW, Goldbohm RA, van den Brandt PA, Weijenberg MP. Dietary flavonol, flavone and catechin intake and risk of colorectal cancer in the Netherlands Cohort Study. Int J Cancer. (2009) 125:2945–52. doi: 10.1002/ijc.24645
381. Kyle JAM, Sharp L, Little J, Duthie GG, McNeill G. Dietary flavonoid intake and colorectal cancer: A case–control study. Br J Nutr. (2010) 103:429–36. doi: 10.1017/S0007114509991784
382. Rossi M, Negri E, Talamini R, Bosetti C, Parpinel M, Gnagnarella P, et al. Flavonoids and colorectal cancer in Italy. Cancer Epidemiol Biomark Prev. (2006) 15:1555–8. doi: 10.1158/1055-9965.EPI-06-0017
383. Chang H, Lei L, Zhou Y, Ye F, Zhao G. Dietary flavonoids and the risk of colorectal cancer: An updated meta-analysis of epidemiological studies. Nutrients. (2018) 10:950. doi: 10.3390/nu10070950
384. Ishikawa H, Saeki T, Otani T, Suzuki T, Shimozuma K, Nishino H, et al. Aged garlic extract prevents a decline of NK cell number and activity in patients with advanced cancer. J Nutr. (2006) 136:816S–20S. doi: 10.1093/jn/136.3.816S
385. Higurashi T, Hosono K, Endo H, Takahashi H, Iida H, Uchiyama T, et al. Eicosapentaenoic acid (EPA) efficacy for colorectal aberrant crypt foci (ACF): A double-blind randomized controlled trial. BMC Cancer. (2012) 12:413. doi: 10.1186/1471-2407-12-413
386. Haidari F, Abiri B, Iravani M, Ahmadi-Angali K, Vafa M. Effects of vitamin D and omega-3 fatty acids Co-supplementation on inflammatory factors and tumor marker CEA in colorectal cancer patients undergoing chemotherapy: A randomized, double-blind, placebo-controlled clinical trial. Nutr Cancer. (2020) 72:948–58. doi: 10.1080/01635581.2019.1659380
387. Patel KR, Brown VA, Jones DJ, Britton RG, Hemingway D, Miller AS, et al. Clinical pharmacology of resveratrol and its metabolites in colorectal cancer patientsresveratrol in colorectal cancer patients. Cancer Res. (2010) 70:7392–9. doi: 10.1158/0008-5472.CAN-10-2027
388. Howells LM, Berry DP, Elliott PJ, Jacobson EW, Hoffmann E, Hegarty B, et al. Phase I randomized, double-blind pilot study of micronized resveratrol (SRT501) in patients with hepatic metastases—safety, pharmacokinetics, and pharmacodynamics. Cancer Prev Res. (2011) 4:1419–25. doi: 10.1158/1940-6207.CAPR-11-0148
389. Walfisch S, Walfisch Y, Kirilov E, Linde N, Mnitentag H, Agbaria R, et al. Tomato lycopene extract supplementation decreases insulin-like growth factor-I levels in colon cancer patients. Eur J Cancer Prev. (2007) 16:298–303. doi: 10.1097/01.cej.0000236251.09232.7b
Keywords: Mediterranean diet, inflammation, colorectal cancer, chemoprevention, natural compounds
Citation: Mahmod AI, Haif SK, Kamal A, Al-ataby IA and Talib WH (2022) Chemoprevention effect of the Mediterranean diet on colorectal cancer: Current studies and future prospects. Front. Nutr. 9:924192. doi: 10.3389/fnut.2022.924192
Received: 20 April 2022; Accepted: 18 July 2022;
Published: 04 August 2022.
Edited by:
Alessandra Durazzo, Council for Agricultural Research and Economics, ItalyReviewed by:
Aamir Ahmad, University of Alabama at Birmingham, United StatesManu Prasad, Memorial Sloan Kettering Cancer Center, United States
Copyright © 2022 Mahmod, Haif, Kamal, Al-ataby and Talib. This is an open-access article distributed under the terms of the Creative Commons Attribution License (CC BY). The use, distribution or reproduction in other forums is permitted, provided the original author(s) and the copyright owner(s) are credited and that the original publication in this journal is cited, in accordance with accepted academic practice. No use, distribution or reproduction is permitted which does not comply with these terms.
*Correspondence: Wamidh H. Talib, d190YWxpYkBhc3UuZWR1Lmpv