- 1Department of Animal Nutrition and Feed Science, College of Animal Science and Technology, Huazhong Agricultural University, Wuhan, China
- 2Faculty of Veterinary and Animal Sciences, Muhammad Nawaz Shareef University of Agriculture Multan, Multan, Pakistan
Epidemiological studies have shown that excessive intake of fructose is largely responsible for the increasing incidence of non-alcoholic fatty liver, obesity, and diabetes. However, depending on the amount of fructose consumption from diet, the metabolic role of fructose is controversial. Recently, there have been increasing studies reporting that diets low in fructose expand the surface area of the gut and increase nutrient absorption in mouse model, which is widely used in fructose-related studies. However, excessive fructose consumption spills over from the small intestine into the liver for steatosis and increases the risk of colon cancer. Therefore, suitable animal models may be needed to study fructose-induced metabolic changes. Along with its use in global meat production, pig is well-known as a biomedical model with an advantage over murine and other animal models as it has similar nutrition and metabolism to human in anatomical and physiological aspects. Here, we review the characteristics and metabolism of fructose and summarize observations of fructose in pig reproduction, growth, and development as well as acting as a human biomedical model. This review highlights fructose metabolism from the intestine to the blood cycle and presents the critical role of fructose in pig, which could provide new strategies for curbing human metabolic diseases and promoting pig production.
Introduction
Overwhelming evidence has established a strong causal link between excessive fructose intake and metabolic diseases (1). For instance, non-alcoholic-fatty liver disease (NAFLD) is currently the most prevalent liver disease worldwide (2), and excessive consumption of fructose intake is considered to boost diseases (3–5). However, the role of fructose is multifactorial. Diets low in fructose increase the length of the intestines and the height of intestinal villi (6), contributing to weight gain and promoting growth, which suggests the potential and beneficial role of fructose with appropriate concentration. But excessive fructose consumption spills over from the small intestine into the liver, causing steatosis and increasing the risk of colon cancer (7–12). Therefore, it is necessary to clarify the critical role of fructose metabolism and its relationship with metabolic diseases.
Animal models are widely employed for studying fructose-induced metabolic changes. Mice fed a high fructose diet (HFD), such as high fructose corn syrup (HFCS), and conditional genetic mouse models help to address fructose-induced metabolic disorders (13, 14). Notably, pig is well-known as a biomedical model because pig is more similar to human. As omnivores, humans and pigs have a large number of similarities in anatomy, physiology, metabolism, and pathology, e.g., they have similar gastrointestinal anatomy and function, pancreas morphology, and metabolic regulation (15). In addition, pork accounts for more than one-third of meat produced worldwide (16), but there are still some problems such as low growth efficiency in the pig industry.
Currently, some important reviews have summarized the progress in the research on fructose metabolism (11, 17–24). However, fructose consumption and its metabolic role in pig are still poorly understood. Hence, this review will focus on the characteristics and metabolism of fructose and its applications in pig reproduction, growth, and development. The review will also highlight the pig biomedical models in fructose metabolism.
Characteristics and beneficial function of fructose in physiology
Characteristics of fructose: A low glycemic sugar
Fructose has been traditionally viewed as a simple 6-carbon monosaccharide found widely in fruit, honey, and some vegetables (17). Over the past few decades, the consumption of soft drinks, especially carbonated drinks, has increased significantly, which are sweetened with sweeteners containing a high percentage of fructose (25, 26).
Fructose is added to beverages and foods in the form of sucrose (50% fructose) or the industrial product high fructose corn syrup (usually 55% fructose) (17). Both fructose and glucose are well absorbed from the intestine into the blood cycle. Admittedly, glucose, not fructose, is the predominant circulating sugar in animals (17), which reflects the innate differences in mammalian glucose and fructose metabolism in circulating blood levels. The normal fasting blood glucose concentration in peripheral blood is 5 mM, while under fasting conditions, the circulating concentration of fructose is about 0.02 mM (27, 28). Early studies reported the average plasma fructose concentration of fructose-fed mice was 1.5 mM, while that of starch-fed mice was 0.23 mM (29). Furthermore, glucose is the main fuel for most tissues and cell types. In contrast, the fructose ingested from sucrose is quickly removed by intestines and liver, converted into glucose and its polymer storage form, glycogen, or fatty acids, and stored in the form of triglycerides in the liver (17). It is of note that fructose has a low glycemic index. Several pieces of evidence have established that the hyperglycemic effect of fructose is much weaker than glucose (30). Thus, the difference between fructose and glucose may suggest the unique role, metabolism, and biological function of fructose.
Fructose functions as a growth-promotor in mouse model
Due to the decreased concentration gradient of fructose in intestine, namely “first-pass” effect, the fructose is potentially related to regulating intestinal growth and development (31). Strikingly, gavaging of 15% fructose for < 3 months will increase the energy intake, weight gain, and growth of mice (32, 33). Similar results are found by feeding mice with 30% fructose solution for 8 weeks (34). However, when mice were fed with 60% fructose for 6 weeks, the incidence of insulin resistance increased (35). Another study reports that 60% of fructose gavage increases cholesterol, triglycerides, blood glucose levels, and liver lipid peroxidation (36). Interestingly, an administration of low concentration of fructose (~15%) gavaging for 30 weeks results in obesity and liver cirrhosis (37). These observations provide evidence that appropriate dose and time of fructose treatment may enhance the length of the small intestine and its absorption of nutrition, indicating the complexity of metabolic flux.
Cellular metabolism of fructose with normal and excessive consumption in intestine and liver
Digestion and absorption of fructose from lumen to intestine
Following oral ingestion, fructose travels through the gastrointestinal tract into the small intestine, where it is passively absorbed from the intestinal lumen mainly by the hexose transporter SLC2A5, also known as GLUT5, and expressed at the brush border of the intestinal epithelium, as shown in Figure 1. GLUT5 is a facilitative transporter, which means that the transport of fructose into intestinal cells is proportional to the concentration gradient across the luminal membrane of intestinal cells (38). Although the expression of GLUT5 is responsible for intestinal fructose absorption, it is also expressed in other tissues and cell types, including skeletal muscle, pre-adipocytes, prostate, spermatozoa, and erythrocytes (39–42). However, the importance of fructose transport to these tissues is poorly understood. Exceeding the clearance capacity of the small intestine, excessive fructose escapes the small intestine and reaches the large intestine and liver (1).
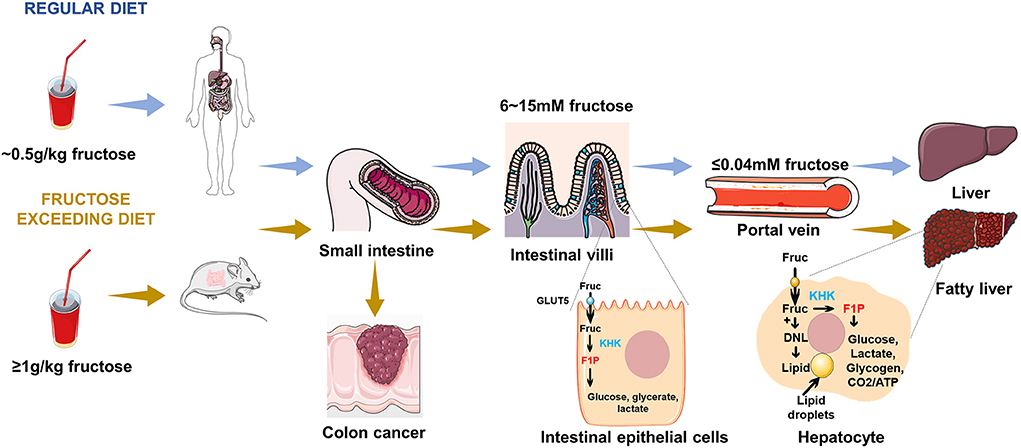
Figure 1. Fructose metabolism in the gut and liver. Following ingestion of fructose, fructose is absorbed into intestinal epithelial enterocytes. Under fasting conditions, the fructose concentration in the small intestine is in the range of 6 ~ 15 mM, while fructose concentration in the portal vein is < 0.04 mM. A portion of this fructose is phosphorylated by KHK within enterocyte and is converted to glucose, lactate, glycerate, and other organic acids, which transport from the portal vein to the liver. Fructose reaching the liver is efficiently extracted by hepatocytes and phosphorylated by KHK, where it can be used for glucose production, lipogenesis, glycogen synthesis, and energetic purposes. An excessive fructose diet can lead to colon cancer, fatty liver, and other related metabolic diseases.
Fructose metabolism in intestinal epithelial cell
The small intestine is the major and first site for fructose metabolism, as shown in Figure 1 (1). Fructose concentration in peripheral plasma is typically about 0.04 mM, which is consistent with the data reported by the previous studies (27, 28, 43–45). By contrast, the concentration of fructose in the intestine (6 to 15 mM) is higher than peripheral plasma (39, 46). This observation provides evidence that dietary fructose metabolism started in small intestinal epithelial cells, and was formed by ketohexokinase (KHK) phosphorylation to form a fructose-1-phosphate (F1P), which accumulated in cells with high levels (1, 47). Much of the fructose is metabolized to lactate, alanine, glycerate, and other organic acids within the intestine. The small intestine fully express glucoisomerase to efficiently convert fructose into circulating glucose (1, 48, 49), which is the mechanism of maintaining liver health. Low doses of fructose(~0.5 g/kg) are 90% cleared by the intestine (1), but high doses of fructose (≥1 g/kg), exceeding intestinal fructose absorption and clearance, results in fructose reaching both the liver and colon, where it may cause disease by impacting hepatic function or microbial composition (1).
Fructose metabolism in hepatocyte
It has been assumed that the liver is another vital site of dietary fructose metabolism (47, 50–52). In a more recent study, it was reported that the liver is able to extract 70% of the oral fructose load, whereas the liver extracts only 10% to 35% of the oral glucose load (53, 54). A small fraction of the carbon in ingested fructose is converted into glucose by the liver and enters the systemic circulation. These observations demonstrate that the rapid clearance of fructose in the blood is largely mediated by the effective extraction of the liver (21). When fructose reaches the liver from the portal vein, fructokinase phosphorylates fructose to fructose-1-phosphate, which can be converted to glycerol-3-phosphate for synthesis of glycerol or metabolized to acetyl-CoA and incorporated into fatty acids through de novo lipogenesis preferentially (55). In fact, fructose is converted to fatty acids at a higher rate in the liver than glucose (56). The entry of fructose into the lipogenic process may contribute to fructose-induced hyperlipidemia, particularly the marked increase in postprandial triglyceride levels (57, 58). Pathologically, recent studies have confirmed that NAFLD is commonly associated with excessive fructose intake (4, 59). Based on the cellular metabolism and function of fructose in the intestine and liver, investigations are required for in-depth study.
Fructose consumption and metabolic roles in pigs
At present, most of the studies on fructose are from mouse model, which is widely used but not well-accorded with human diseases, so we have chosen to focus on another greater model than murine: pigs. Pig is a more suitable biomedical model for humans than mouse (60). The anatomical and physiological similarities between pigs and humans suggests pig model to be an important tool for medical research, providing important models for human therapies (61). In addition, pig production plays an important role in farming systems worldwide (62) and the large-scale pig industry is developing rapidly all over the world to satisfy the growing requirement of consumers (63). However, there are still some problems that are difficult to solve at present, for example, postnatal growth retardation (PGR) is common in piglets, which are prone to developing chronic disease (64). Therefore, we further summarized the roles of fructose in pig production and pig biomedical models for human metabolic diseases.
Fructose in reproductive performance of pigs
Reproductive performance has a great influence on sow productivity and production profit (65). As mentioned in Table 1, McCracken (70) gave pre-pubertal gilts dietary treatments containing 35% fructose for 9 weeks, and found that the size of the reproductive tract had increased but the pregnancy rate decreased. It was also observed that fructose can increase litter size of primiparous sows but had no effect on individual weight and milk yield (67). However, there are different views that the milk yield of sows fed with a fructose diet increased significantly on day 14 and day 21 during a 22-d lactation, implying that fructose might be used to increase total yield of milk during lactation (66), but the dose and concentration need to be further studied. In fact, there is increasing clinical evidence that fructose contributes to elongated estrous cycles and hyperandrogenemia, accompanied by decreased LH concentrations and an increase in the number of follicles and in the level of luteal phase progesterone (68). Mechanically, Ossabaw pigs fed with fructose have increased transcript levels and dysfunctional steroidogenic enzymes in the ovarian delta 4 steroidogenic pathway, leading to hyperandrogenemia (69). However, the detailed mechanism of how fructose affects the reproduction functions of pigs needs further investigation.
Fructose metabolism in growth and development of pigs
Despite the controversial role of fructose in reproduction, fructose is the most abundant hexose sugar in pig embryos, indicating a specific function of fructose in embryo development. It has also been shown to be the principal blood sugar in the ungulate fetus (81–83). In fetal pig blood, the concentration of fructose is higher than glucose (84). Gluconeogenesis rarely occurs in the placenta and embryo of pigs (85). It means that glucose and fructose in maternal blood must be transported to the uterine cavity to ensure the use of glucose and fructose in the placenta and fetus. In pigs, glucose and fructose are transported from the maternal vascular system to the placental vascular system through multiple cell layers in a SLC2A-dependent manner (74, 75). Notably, intraperitoneal injection of sugars containing uniformly labeled carbon (UL14C) into pigs shows that glucose may act as a precursor of fructose and convert to fructose in porcine endometrium and placenta (71, 74). Due to the high concentration of fructose, a partial amount of fructose is involved in the synthesis of nucleic acids, thereby providing substrate for anabolic functions necessary for fetal growth and development (72). In a more recent study, it is reported that fructose can be used as an alternative energy source by embryos that express enzymes to promote fructose to enter the glycolysis pathway for metabolism (74). Moreover, it was observed that fructose is mediated via hexosamine biosynthesis pathway to stimulate mTOR cell signaling, which can promote embryonic/fetal growth and development (73). Thus, it is of importance to better understand the critical role of fructose in the placenta and embryo.
Pig acts as a human biomedical model for fructose-induced metabolic disorders
Animal biomedical models play a central role in human medical research and developing new therapeutical strategies (61). Pig biomedical models for human reproduction have greatly advanced our understanding of the basic science of fertilization and pregnancy, as well as metabolic diseases including NAFLD (61). Although there are several factors that may lead to NAFLD, excessive consumption of fructose is considered to be a key factor in the formation of the disease (3–5). A recent study reported that juvenile Ossabaw swine fed a high-fat, high-fructose, high-cholesterol diet, containing 17.8% HFCS, can develop obesity and have serious effects on liver, blood lipids, microflora, and a NAFLD phenotype (76). Furthermore, a high-fructose and high-fat diet (HFF) interfered with the skeletal muscle metabolism of Iberian piglets after feeding for 10 weeks (78). Mechanically, there is increasing clinical evidence to show that fructose produces potential lipogenesis through precursors that can be used for de novo lipogenesis (DNL), leading to fat accumulation and liver steatosis (77, 86).
However, it has been reported recently that a high-fructose diet does not induce NAFLD. Some studies demonstrate that, compared with a sucrose-enriched diet, histological markers of NAFLD in Iberian pigs showed no significant increase in the fructose group (80). Feeding castrated male Danish Landrace-Yorkshire-Duroc pigs with 60% fructose for 4 weeks showed no steatosis or hepatocyte ballooning in the liver, providing evidence that short-term feeding with a high-fructose diet may not induce NAFLD in pigs (79). Collectively, breed and age of pigs might be factors that protect pigs from the development of steatosis.
Conclusion and perspective
Over the last decade, studies have illustrated the role of fructose and its metabolic mechanism. However, the functional effect of fructose in vivo remains controversial, and there is no conclusion on the specific healthy use of fructose. Moreover, its different roles in pigs are challenging and worthy of exploration. In piglets, PGR is also prevalent in pig production (64). From the perspective of the effect of fructose, it indicates that fructose might have a potential growth-promoting effect. Besides, taking the safe thresholds for sugar consumption into account will be a matter of concern in nutrition and the detailed mechanism of how fructose affects the reproduction functions of pigs warrants further investigation. Hopefully, better understanding of the complex role of fructose may open new avenues for curbing human metabolic diseases and improve pig production.
Author contributions
TS designed this review, helped with writing and revising of the manuscript, and provided critical feedback. JX and SS conceptualized the topic, researched and analyzed the literature, and composed and revised the manuscript. YL, SW, and SR helped draft the manuscript. All authors approve the final version of the manuscript, ensure the accuracy and integrity of the work, and agree to be accountable for all aspects of the work.
Funding
This work was supported by grants from the National Natural Science Foundation of China (32102561), the Chinese Fundamental Research Funds for the Central Universities (2662019QD022), the Student Research Funds of Huazhong Agricultural University (S202110504033), National Innovation and Entrepreneurship Training Program for Undergraduate (202210504008), and the State Key Laboratory for Managing Biotic and Chemical Threats to the Quality and Safety of Agro-products (2021DG700024-KF202214).
Acknowledgments
We would like to thank all the members of Adipo-mystery group.
Conflict of interest
The authors declare that the research was conducted in the absence of any commercial or financial relationships that could be construed as a potential conflict of interest.
Publisher's note
All claims expressed in this article are solely those of the authors and do not necessarily represent those of their affiliated organizations, or those of the publisher, the editors and the reviewers. Any product that may be evaluated in this article, or claim that may be made by its manufacturer, is not guaranteed or endorsed by the publisher.
References
1. Jang C, Hui S, Lu W, Cowan AJ, Morscher RJ, Lee G, et al. The small intestine converts dietary fructose into glucose and organic acids. Cell Metab. (2018) 27:351–61. e3. doi: 10.1016/j.cmet.2017.12.016
2. Adams LA, Lindor KD. Nonalcoholic fatty liver disease. Ann Epidemiol. (2007) 17:863–9. doi: 10.1016/j.annepidem.2007.05.013
3. Ishimoto T, Lanaspa MA, Rivard CJ, Roncal-Jimenez CA, Orlicky DJ, Cicerchi C, et al. High-Fat and high-sucrose (Western) diet induces steatohepatitis that is dependent on fructokinase. Hepatology. (2013) 58:1632–43. doi: 10.1002/hep.26594
4. Lim JS, Mietus-Snyder M, Valente A, Schwarz J-M, Lustig RH. The role of fructose in the pathogenesis of Nafld and the metabolic syndrome. Nat Rev Gastroenterol Hepatol. (2010) 7:251–64. doi: 10.1038/nrgastro.2010.41
5. Basaranoglu M, Basaranoglu G, Bugianesi E. Carbohydrate intake and nonalcoholic fatty liver disease: fructose as a weapon of mass destruction. Hepatobiliary Surg Nutr. (2015) 4:109.
6. Taylor SR, Ramsamooj S, Liang RJ, Katti A, Pozovskiy R, Vasan N, et al. Dietary fructose improves intestinal cell survival and nutrient absorption. Nature. (2021) 597:263–7. doi: 10.1038/s41586-021-03827-2
7. Joh H-K, Lee DH, Hur J, Nimptsch K, Chang Y, Joung H, et al. simple sugar and sugar-sweetened beverage intake during adolescence and risk of colorectal cancer precursors. Gastroenterology. (2021) 161:128–42. e20. doi: 10.1053/j.gastro.2021.03.028
8. Tasevska N, Jiao L, Cross AJ, Kipnis V, Subar AF, Hollenbeck A, et al. Sugars in diet and risk of cancer in the Nih-Aarp diet and health study. Int J cancer. (2012) 130:159–69. doi: 10.1002/ijc.25990
9. Bostick RM, Potter JD, Kushi LH, Sellers TA, Steinmetz KA, McKenzie DR, et al. Sugar, meat, and fat intake, and non-dietary risk factors for colon cancer incidence in iowa women (United States). Cancer Cause Control. (1994) 5:38–52. doi: 10.1007/BF01830725
10. Meyerhardt JA, Sato K, Niedzwiecki D, Ye C, Saltz LB, Mayer RJ, et al. Dietary glycemic load and cancer recurrence and survival in patients with stage iii colon cancer: findings from Calgb 89803. J Natl Cancer Inst. (2012) 104:1702–11. doi: 10.1093/jnci/djs399
11. Jang C, Wada S, Yang S, Gosis B, Zeng X, Zhang Z, et al. The small intestine shields the liver from fructose-induced steatosis. Nature Metab. (2020) 2:586–93. doi: 10.1038/s42255-020-0222-9
12. Goncalves MD, Lu C, Tutnauer J, Hartman TE, Hwang S-K, Murphy CJ, et al. High-Fructose corn syrup enhances intestinal tumor growth in mice. Science. (2019) 363:1345–9. doi: 10.1126/science.aat8515
13. Deol P, Evans JR, Dhahbi J, Chellappa K, Han DS, Spindler S, et al. Soybean oil is more obesogenic and diabetogenic than coconut oil and fructose in mouse: potential role for the liver. PLoS ONE. (2015) 10:e0132672. doi: 10.1371/journal.pone.0132672
14. Lozano I, Van der Werf R, Bietiger W, Seyfritz E, Peronet C, Pinget M, et al. High-Fructose and high-fat diet-induced disorders in rats: impact on diabetes risk, hepatic and vascular complications. Nutr Metab. (2016) 13:1–13. doi: 10.1186/s12986-016-0074-1
15. Aigner B, Renner S, Kessler B, Klymiuk N, Kurome M, Wünsch A, et al. Transgenic pigs as models for translational biomedical research. J Mol Med. (2010) 88:653–64. doi: 10.1007/s00109-010-0610-9
16. VanderWaal K, Deen J. Global trends in infectious diseases of swine. Proc Nat Acad Sci. (2018) 115:11495–500. doi: 10.1073/pnas.1806068115
17. Herman MA, Birnbaum MJ. Molecular aspects of fructose metabolism and metabolic disease. Cell Metab. (2021) 33:2329–54. doi: 10.1016/j.cmet.2021.09.010
18. Krause N, Wegner A. Fructose metabolism in cancer. Cells. (2020) 9:2635. doi: 10.3390/cells9122635
19. Nakagawa T, Lanaspa MA, San Millan I, Fini M, Rivard CJ, Sanchez-Lozada LG, et al. Fructose contributes to the warburg effect for cancer growth. Cancer Metab. (2020) 8:1–12. doi: 10.1186/s40170-020-00222-9
20. Febbraio MA, Karin M. “Sweet Death”: fructose as a metabolic toxin that targets the gut-liver axis. Cell Metab. (2021) 33:2316–28. doi: 10.1016/j.cmet.2021.09.004
21. Hannou SA, Haslam DE, McKeown NM, Herman MA. Fructose metabolism and metabolic disease. J Clin Invest. (2018) 128:545–55. doi: 10.1172/JCI96702
22. Gonzalez JT, Betts JA. Dietary fructose metabolism by splanchnic organs: size matters. Cell Metab. (2018) 27:483–5. doi: 10.1016/j.cmet.2018.02.013
23. Federico A, Rosato V, Masarone M, Torre P, Dallio M, Romeo M, et al. The role of fructose in non-alcoholic steatohepatitis: old relationship and new insights. Nutrients. (2021) 13:1314. doi: 10.3390/nu13041314
24. Jiang H, Lin Q, Ma L, Luo S, Jiang X, Fang J, et al. Fructose and fructose kinase in cancer and other pathologies. J Genetics Genomics. (2021) 48:531–9. doi: 10.1016/j.jgg.2021.06.006
25. Park YK, Yetley EA. Intakes and food sources of fructose in the United States. Am J Clin Nutr. (1993) 58:737S−47S. doi: 10.1093/ajcn/58.5.737S
26. Wang P, Wu T, Fu Q, Liao Q, Li Y, Huang T, et al. Maternal high-fructose intake activates myogenic program in fetal brown fat and predisposes offspring to diet-induced metabolic dysfunctions in adulthood. Front Nutr. (2022) 9:848983. doi: 10.3389/fnut.2022.848983
27. Chen Y, Lin H, Qin L, Lu Y, Zhao L, Xia M, et al. Fasting serum fructose levels are associated with risk of incident type 2 diabetes in middle-aged and older Chinese population. Diabetes Care. (2020) 43:2217–25. doi: 10.2337/dc19-2494
28. Francey C, Cros J, Rosset R, Crézé C, Rey V, Stefanoni N, et al. the extra-splanchnic fructose escape after ingestion of a fructose–glucose drink: an exploratory study in healthy humans using a dual fructose isotope method. Clinical Nutr ESPEN. (2019) 29:125–32. doi: 10.1016/j.clnesp.2018.11.008
29. Klein AV, Kiat H. The mechanisms underlying fructose-induced hypertension: a review. J Hypertens. (2015) 33:912. doi: 10.1097/HJH.0000000000000551
30. Lee BM, Lee SK, Kim HS. Inhibition of oxidative DNA damage, 8-Ohdg, and carbonyl contents in smokers treated with antioxidants (Vitamin E, Vitamin C, B-Carotene and Red Ginseng). Cancer Lett. (1998) 132:219–27. doi: 10.1016/S0304-3835(98)00227-4
31. Schaefer EJ, Gleason JA, Dansinger ML. Dietary fructose and glucose differentially affect lipid and glucose homeostasis. J Nutr. (2009) 139:1257S−62S. doi: 10.3945/jn.108.098186
32. Jürgens H, Haass W, Castaneda TR, Schürmann A, Koebnick C, Dombrowski F, et al. Consuming fructose-sweetened beverages increases body adiposity in mice. Obes Res. (2005) 13:1146–56. doi: 10.1038/oby.2005.136
33. Nunes PM, Anastasiou D. Fructose in the Diet Expands the Surface of the Gut and Promotes Nutrient Absorption. Nature Publishing Group. (2021) 597:180–2. doi: 10.1038/d41586-021-02195-1
34. Volynets V, Spruss A, Kanuri G, Wagnerberger S, Bischoff SC, Bergheim I. Protective effect of bile acids on the onset of fructose-induced hepatic steatosis in mice. J Lipid Res. (2010) 51:3414–24. doi: 10.1194/jlr.M007179
35. Mellor K, Ritchie RH, Meredith G, Woodman OL, Morris MJ, Delbridge LM. High-fructose diet elevates myocardial superoxide generation in mice in the absence of cardiac hypertrophy. Nutrition. (2010) 26:842–8. doi: 10.1016/j.nut.2009.08.017
36. Rivera EC, Yamakawaa CK, Garciaa MH, Geraldoa VC, Rossella CE, Filhob RM, et al. A procedure for estimation of fermentation kinetic parameters in fed-batch bioethanol production process with cell recycle. Chemical Eng. (2013) 32:1369–74. doi: 10.3303/CET1332229
37. Mastrocola R, Collino M, Rogazzo M, Medana C, Nigro D, Boccuzzi G, et al. Advanced Glycation end products promote hepatosteatosis by interfering with scap-srebp pathway in fructose-drinking mice. Am J Physiol Gastrointest Liver Physiol. (2013) 305:G398–407. doi: 10.1152/ajpgi.00450.2012
38. Cori CF. The rate of absorption of hexoses and pentoses. Proceedings Soc Experiment Biol Med. (1925) 22:497–9. doi: 10.3181/00379727-22-239
39. Burant C, Takeda J, Brot-Laroche E, Bell G, Davidson N. Fructose transporter in human spermatozoa and small intestine is glut5. J Biol Chem. (1992) 267:14523–6. doi: 10.1016/S0021-9258(18)42067-4
40. Concha II, Velásquez FV, Martinez JM, Angulo C, Droppelmann A, Reyes AM, et al. Human erythrocytes express glut5 and transport fructose. Blood. (1997) 89:4190–5. doi: 10.1182/blood.V89.11.4190
41. Kayano T, Burant C, Fukumoto H, Gould G, Fan Y, Eddy R, et al. Human facilitative glucose transporters isolation, functional characterization, and gene localization of cdnas encoding an isoform (glut5) expressed in small intestine, kidney, muscle, and adipose tissue and an unusual glucose transporter pseudogene-like sequence (glut6). J Biol Chem. (1990) 265:13276–82. doi: 10.1016/S0021-9258(19)38295-X
42. Reinicke K, Sotomayor P, Cisterna P, Delgado C, Nualart F, Godoy A. Cellular distribution of glut-1 and glut-5 in benign and malignant human prostate tissue. J Cell Biochem. (2012) 113:553–62. doi: 10.1002/jcb.23379
43. Sugimoto K, Kawasaki T, Tomoda M, Nakagawa K, Hayashi S, Inui H, et al. Lowering of postprandial hyperfructosemia in humans by eucalyptus leaf extract: a randomized, double-blind, placebo-controlled crossover study. Food Sci Technol Res. (2010) 16:509–12. doi: 10.3136/fstr.16.509
44. Wahjudi PN, Patterson ME, Lim S, Yee JK, Mao CS, Lee W-NP. Measurement of glucose and fructose in clinical samples using gas chromatography/mass spectrometry. Clin Biochem. (2010) 43:198–207. doi: 10.1016/j.clinbiochem.2009.08.028
45. Preston GM, Calle RA. Elevated serum sorbitol and not fructose in type 2 diabetic patients. Bio Insights. (2010) 5:S4530. doi: 10.4137/BMI.S4530
46. Douard V, Ferraris RP. Regulation of the fructose transporter glut5 in health and disease. Am J Physiol Endocrinol Metab. (2008) 295:E227–37. doi: 10.1152/ajpendo.90245.2008
47. Miller M, Craig JW, Drucker WR, Woodward H Jr. The metabolism of fructose in man. Yale J Biol Med. (1956) 29:335.
48. BISMUT H, HERS HG, Van Schaftingen E. Conversion of fructose to glucose in the rabbit small intestine: a reappraisal of the direct pathway. Eur J Biochem. (1993) 213:721–6. doi: 10.1111/j.1432-1033.1993.tb17812.x
49. Ginsburg V, Hers H. On the Conversion of Fructose to Glucose by Guinea Pig Intestine. Biochim Biophys Acta. (1960) 38:427–34. doi: 10.1016/0006-3002(60)91278-6
50. Herman RH, Stifel FB, Greene HL, Herman YF. Intestinal metabolism of fructose. Acta Med Scand. (1972) 192:19–25. doi: 10.1111/j.0954-6820.1972.tb05315.x
51. Froesch E. Fructose metabolism in adipose tissue. Acta Med Scand. (1972) 192:37–46. doi: 10.1111/j.0954-6820.1972.tb05317.x
52. Mirtschink P, Krishnan J, Grimm F, Sarre A, Hörl M, Kayikci M, et al. Hif-Driven Sf3b1 induces Khk-C to enforce fructolysis and heart disease. Nature. (2015) 522:444–9. doi: 10.1038/nature14508
53. Lam P. Effects of consuming dietary fructose versus glucose on de novo lipogenesis in overweight and obese human subjects. Berkeley Sci J. (2011) 15:1–3. doi: 10.5070/BS3152011589
54. Tappy L, Lê K-A. Does fructose consumption contribute to non-alcoholic fatty liver disease? Clin Res Hepatol Gastroenterol. (2012) 36:554–60. doi: 10.1016/j.clinre.2012.06.005
55. Frayn KN, Kingman SM. Dietary sugars and lipid metabolism in humans. Am J Clin Nutr. (1995) 62:250S−61S. doi: 10.1093/ajcn/62.1.250S
56. Aoyama Y, Yoshida A, Ashida K. Effect of dietary fats and fatty acids on the liver lipid accumulation induced by feeding a protein-repletion diet containing fructose to protein-depleted rats. J Nutr. (1974) 104:741–6. doi: 10.1093/jn/104.6.741
57. Imamura F, O'Connor L, Ye Z, Mursu J, Hayashino Y, Bhupathiraju SN, et al. Consumption of sugar sweetened beverages, artificially sweetened beverages, and fruit juice and incidence of type 2 diabetes: systematic review, meta-analysis, and estimation of population attributable fraction. BMJ. (2015) 351:h3576. doi: 10.1136/bmj.h3576
58. de Koning L, Malik VS, Kellogg MD, Rimm EB, Willett WC, Hu FB. Sweetened beverage consumption, incident coronary heart disease, and biomarkers of risk in men. Circulation. (2012) 125:1735–41. doi: 10.1161/CIRCULATIONAHA.111.067017
59. Jensen VS, Hvid H, Damgaard J, Nygaard H, Ingvorsen C, Wulff EM, et al. Dietary fat stimulates development of nafld more potently than dietary fructose in sprague–dawley rats. Diabetol Metab Syndr. (2018) 10:1–13. doi: 10.1186/s13098-018-0307-8
60. Bassols A, Costa C, Eckersall PD, Osada J, Sabria J, Tibau J. The pig as an animal model for human pathologies: a proteomics perspective. PROTEOMICS–Clin Appli. (2014) 8:715–31. doi: 10.1002/prca.201300099
61. Lunney JK, Van Goor A, Walker KE, Hailstock T, Franklin J, Dai C. Importance of the pig as a human biomedical model. Science Trans Med. (2021) 13:eabd5758. doi: 10.1126/scitranslmed.abd5758
62. Zhang Y-g, Yin Y-l, Fang J, Wang Q. Pig production in subtropical agriculture. J Sci Food Agric. (2012) 92:1016–24. doi: 10.1002/jsfa.4679
63. Thomas R, Singh V, Gupta VK. Current status and development prospects of India's pig industry. Indian J Animal Sci. (2021) 91:255–68.
64. Qi M, Tan B, Wang J, Li J, Liao S, Yan J, et al. Small intestinal transcriptome analysis revealed changes of genes involved in nutrition metabolism and immune responses in growth retardation piglets. J Anim Sci. (2019) 97:3795–808. doi: 10.1093/jas/skz205
65. Engblom L, Stalder KJ, Mabry JW. Culling, Mortality and Lifetime Production among Us Sows. Iowa State University Animal Industry Report (2010).
66. White C, Head H, Bachman K, Bazer F. Yield and composition of milk and weight gain of nursing pigs from sows fed diets containing fructose or dextrose. J Anim Sci. (1984) 59:141–50. doi: 10.2527/jas1984.591141x
67. Campbell W, Brendemuhl J, Bazer F. Effect of fructose consumption during lactation on sow and litter performance and sow plasma constituents. J Anim Sci. (1990) 68:1378–88. doi: 10.2527/1990.6851378x
68. Newell-Fugate AE, Taibl JN, Clark SG, Alloosh M, Sturek M, Krisher RL. Effects of diet-induced obesity on metabolic parameters and reproductive function in female ossabaw minipigs. Comp Med. (2014) 64:44–9.
69. Newell-Fugate AE, Taibl JN, Alloosh M, Sturek M, Bahr JM, Nowak RA, et al. Effects of obesity and metabolic syndrome on steroidogenesis and folliculogenesis in the female ossabaw mini-pig. PLoS ONE. (2015) 10:e0128749. doi: 10.1371/journal.pone.0128749
70. McCracken VL. The Effects of Dietary Fructose and Fat on the Reproductive Parameters of Prepubertal and Pregnant Gilts. Virginia Tech (2015) 1–106.
71. White C, Piper E, Noland P. Conversion of glucose to fructose in the fetal pig. J Anim Sci. (1979) 48:585–90. doi: 10.2527/jas1979.483585x
72. White C, Piper E, Noland P, Daniels L. Fructose utilization for nucleic acid synthesis in the fetal pig. J Anim Sci. (1982) 55:73–6. doi: 10.2527/jas1982.55173x
73. Kim J, Song G, Wu G, Bazer FW. Functional roles of fructose. Proc Nat Acad Sci. (2012) 109:E1619–E28. doi: 10.1073/pnas.1204298109
74. Steinhauser CB, Landers M, Myatt L, Burghardt RC, Vallet JL, Bazer FW, et al. Fructose synthesis and transport at the uterine-placental interface of pigs: cell-specific localization of Slc2a5, Slc2a8, and components of the polyol pathway. Biolo Reproduct. (2016) 95:108. doi: 10.1095/biolreprod.116.142174
75. Kramer AC, Steinhauser CB, Gao H, Seo H, McLendon BA, Burghardt RC, et al. Steroids regulate Slc2a1 and Slc2a3 to deliver glucose into trophectoderm for metabolism via glycolysis. Endocrinology. (2020) 161:bqaa098. doi: 10.1210/endocr/bqaa098
76. Panasevich MR, Meers GM, Linden MA, Booth FW, Perfield JW, Fritsche KL, et al. High-Fat, high-fructose, high-cholesterol feeding causes severe nash and cecal microbiota dysbiosis in juvenile ossabaw swine. Am J Physiol Endocrinol Metab. (2018) 314:E78–92. doi: 10.1152/ajpendo.00015.2017
77. Curtasu MV, Tafintseva V, Bendiks ZA, Marco ML, Kohler A, Xu Y, et al. Obesity-Related metabolome and gut microbiota profiles of juvenile göttingen minipigs—long-term intake of fructose and resistant starch. Metabolites. (2020) 10:456. doi: 10.3390/metabo10110456
78. Spooner HC, Derrick SA, Maj M, Manjarín R, Hernandez GV, Tailor DS, et al. High-Fructose, high-fat diet alters muscle composition and fuel utilization in a juvenile iberian pig model of non-alcoholic fatty liver disease. Nutrients. (2021) 13:4195. doi: 10.3390/nu13124195
79. Schmidt NH, Svendsen P, Albarrán-Juárez J, Moestrup SK, Bentzon JF. High-Fructose feeding does not induce steatosis or non-alcoholic fatty liver disease in pigs. Sci Rep. (2021) 11:1–10. doi: 10.1038/s41598-021-82208-1
80. Maj M, Harbottle B, Thomas PA, Hernandez GV, Smith VA, Edwards MS, et al. Consumption of high-fructose corn syrup compared with sucrose promotes adiposity and increased triglyceridemia but comparable nafld severity in juvenile iberian pigs. J Nutr. (2021) 151:1139–49. doi: 10.1093/jn/nxaa441
81. Bacon J, Bell D. Fructose and Glucose in the Blood of the Foetal Sheep. Biochem J. (1948) 42:397. doi: 10.1042/bj0420397
82. Bacon J, Bell D. The identification of fructose as a constituent of the foetal blood of the sheep. Biochem J. (1946) 40:xlii.
83. Barklay H, Haas P, Huggett ASG, King G, Rowley D. The Sugar of the foetal blood, the amniotic and allantoic fluids. J Physiol. (1949) 109:98. doi: 10.1113/jphysiol.1949.sp004373
84. Huggett ASG. Carbohydrate metabolism in the placenta and foetus. Br Med Bull. (1961) 17:122–6. doi: 10.1093/oxfordjournals.bmb.a069885
85. Fowden AL, Forhead AJ, Silver M, MacDonald A. Glucose, lactate and oxygen metabolism in the fetal pig during late gestation. Exp Physiol. (1997) 82:171–82. doi: 10.1113/expphysiol.1997.sp004006
Keywords: fructose, pig production, biomedical model, intestine, liver
Citation: Xie J, Shi S, Liu Y, Wang S, Rajput SA and Song T (2022) Fructose metabolism and its role in pig production: A mini-review. Front. Nutr. 9:922051. doi: 10.3389/fnut.2022.922051
Received: 17 April 2022; Accepted: 06 June 2022;
Published: 29 July 2022.
Edited by:
Ju-Sheng Zheng, Westlake University, ChinaReviewed by:
Jie Yin, Hunan Agricultural University, ChinaXin Zhang, China Agricultural University, China
Copyright © 2022 Xie, Shi, Liu, Wang, Rajput and Song. This is an open-access article distributed under the terms of the Creative Commons Attribution License (CC BY). The use, distribution or reproduction in other forums is permitted, provided the original author(s) and the copyright owner(s) are credited and that the original publication in this journal is cited, in accordance with accepted academic practice. No use, distribution or reproduction is permitted which does not comply with these terms.
*Correspondence: Tongxing Song, songtongxing@mail.hzau.edu.cn
†These authors have contributed equally to this work and share first authorship