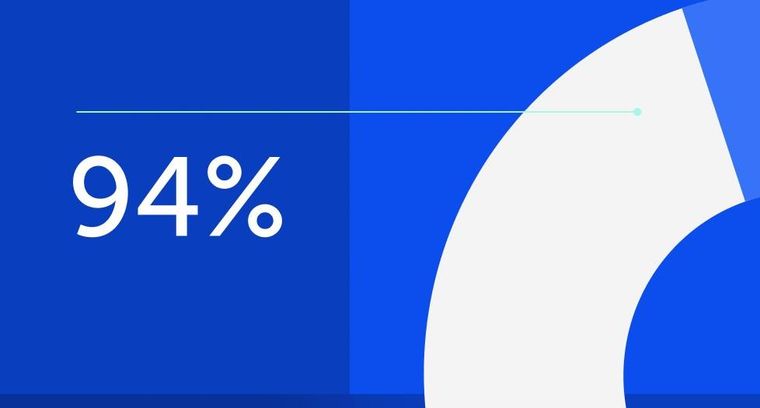
94% of researchers rate our articles as excellent or good
Learn more about the work of our research integrity team to safeguard the quality of each article we publish.
Find out more
ORIGINAL RESEARCH article
Front. Nutr., 09 June 2022
Sec. Nutrition and Food Science Technology
Volume 9 - 2022 | https://doi.org/10.3389/fnut.2022.918696
This article is part of the Research TopicNovel Technologies in Whole-Grain Processing and its Global Nutritional BenefitsView all 5 articles
Adzuki bean is widely consumed in East Asia. Although the positive effects of its biologically active ingredients on obesity have been confirmed, the role of whole cooked adzuki bean in preventing obesity and the relationship between the effects and gut microbiota remain unclear. Mice were fed either a low-fat diet (LFD) or high-fat diet (HFD) with or without 15% cooked adzuki bean for 12 weeks. Cooked adzuki bean significantly inhibited weight gain and hepatic steatosis, reduced high levels of serum triacylglycerol (TG), alanine aminotransferase (ALT), and aspartate aminotransferase (AST), and alleviated systemic inflammation and metabolic endotoxemia in mice fed a HFD. Importantly, cooked adzuki bean regulated gut microbiota composition, decreased the abundance of lipopolysaccharide (LPS)-producing bacteria (Desulfovibrionaceae,Helicobacter,and Bilophila), and HFD-dependent taxa (Deferribacteraceae, Ruminiclostridium_9, Ruminiclostridium, Mucispirillum, Oscillibacter, Enterorhabdus, Tyzzerella, Anaerotruncus, Intestinimonas, unclassified_f_Ruminococcaceae, Ruminiclostridium_5, and Ruminococcaceae), and enriched Muribaculaceae, norank_f_Muribaculaceae, Anaeroplasma, Lachnospiraceae_NK4A136_group, and Lachnospiraceae to alleviate inflammation and metabolic disorders induced by HFD. These findings provide new evidence for understanding the anti-obesity effect of cooked adzuki bean.
Obesity is becoming a worldwide health problem (1). The main feature of obesity is excessive fat accumulation, accompanied by dyslipidemia, liver injury, and hepatic steatosis. Excessive fat accumulation leads to adipocyte hypertrophy and the production of pro-inflammatory cytokines (tumor necrosis factor-α and interleukin-6). Therefore, obesity is also considered a type of chronic low-grade inflammation (2). The current treatment of obesity is often inefficient and accompanied by side effects, so effective and safe anti-obesity methods are needed. Dietary recommendations, such as legumes consumption, have received increasing attention because they prevent obesity by alleviating dyslipidemia, suppressing inflammatory signals, and reshaping gut microbiota (3).
Gut microbiota regulates many physiological processes through interaction with the host, and the abnormal function or composition of gut microbiota contribute to the development of metabolic diseases (4). Studies have shown that HFDs not only significantly increase body fat mass, but also reshape the gut microbiota (5). Gut microbiota dysbiosis induced by HFD increase energy collection and storage, destroy the intestinal barrier, cause inflammation, and lead to obesity (6). In addition, LPS is an important metabolite of gut microbiota, and its release is related to systemic inflammation and obesity (7). HFD feeding impairs intestinal integrity, promotes the transfer of LPS from intestinal microbiota to blood, and causes metabolic endotoxemia (8). Emerging evidence shows that diets can quickly and reproducibly change the structure of gut microbiota (9). Therefore, dietary intervention is considered to be an effective way to alleviate gut microbiota dysbiosis.
Adzuki bean is commonly consumed in East Asia and is treated as a diuretic and an antidote (10). Adzuki bean is rich in biologically active ingredients, such as flavonoids, proanthocyanidins, saponins, and functional polypeptide (11, 12). Previous studies have shown that saponins and flavonoids from adzuki bean prevent HFD-induced obesity in mice (13). Adzuki bean resistant starch reduced serum cholesterol level in rats fed a cholesterol diet (14). Adzuki bean dietary fiber reduced visceral fat accumulation in rats fed a normal diet (15). The biologically active peptides in adzuki bean exhibited significant anti-inflammatory activity by inhibiting the expression of tumor necrosis factor-α (TNF-α) and interleukin-6 (IL-6) (16). In addition, adzuki bean water extract containing polyphenols attenuated obesity by regulating gut microbiota (17). Most adzuki bean studies on reducing obesity induced by HFD focused mainly either on the effects of bean extracts or on single chemicals. However, people in daily life usually consume adzuki bean as whole beans and make it into porridge, bean paste, and soup. Beans are usually not consumed raw and need to be processed before consumption. Processing can promote protein digestion by reducing the level of antinutritional factors in beans (18). Importantly, Processing not only significantly decreased the content of total polyphenols and total flavonoids in adzuki bean, but also significantly reduced the bioavailability of total phenols and antioxidant activity (19–21). Compared with the peptides obtained from raw adzuki bean protein, the thermally processed peptides showed significantly reduced inhibition of 3-hydroxy-3-methylglutaryl coenzyme-A reductase catalytic activity (22). Our previous study has found that 15% raw adzuki bean supplementation significantly alleviated obesity in HFD-fed mice (23). We also found that heat-treated adzuki bean protein hydrolysates reduced obesity in mice fed a HFD via remodeling gut microbiota (12). But it is of more practical importance for dietary guidance to understand if cooked adzuki bean can prevent obesity. Therefore, it is also necessary to clarify the relationship between the effects of cooked adzuki bean on preventing obesity and gut microbiota.
Thus, we assessed the effects of cooked adzuki bean on the body weight gain, serum lipids, and systemic inflammation of mice fed a HFD, and further analyzed the changes in gut microbiota composition. The present study reveals the link between the gut microbiota and cooked adzuki bean-mediated metabolic improvement. Results may help to understand the mechanism of cooked adzuki bean in preventing obesity, especially the role of the gut microbiota.
Adzuki bean from Dongfangliang Life Technology Co., Ltd., (Datong, China) was soaked for 12 h, steamed for 2 h, and dried for 12 h at 40°C. Cooked beans were pulverized into 80-mesh powders. The basic nutritional composition of cooked adzuki bean was showed in Supplementary Table 1.
All experimental design was approved by the Animal Care Committee of China Agricultural University (AW09089102-4). Four-week-old male C57BL/6 mice (Vital River Laboratory Animal Technology Co., Ltd., Beijing, China) were housed in a Specific-Pathogen-Free facility with controlled conditions (55 ± 5% humidity, 24 ± 2°C, 12 h light-dark cycle) with free access to water and food. After 1 week of adaptive feeding, mice were randomly divided into three groups (n = 7 per group) and housed at 2 or 3 per cage. The design of the 12-week animal experiment (Figure 1A) was as follows: (1) low-fat diet (LFD, 3.85 total kcal/g, 10% kcal from fat), (2) high-fat diet (HFD, 5.24 total kcal/g, 60% kcal from fat), and (3) HFD supplemented with 15% cooked adzuki bean powder (HFD-CAB, 5.24 total kcal/g, 60% kcal from fat). The detailed diet energy densities and compositions were determined (Supplementary Table 2). The LFD (D12450J) and HFD (D12492) diets were obtained from Research Diets Inc. (New Brunswick, NJ, United States). Daily intake of 200–300 g of cereal-grain, including 50–150 g of whole grain and pulses, is recommended according to the Dietary Guidelines for Chinese Residents (24). The dietary guidelines suggest a 50% maximum intake level for pulses. However, it is difficult for people to consume so many legumes daily, so a more practical low intake level needs to be recommended. The 15% cooked adzuki bean supplementation level refers to previous studies (15, 23, 25) and is an physiologically relevant and achievable level of pulse intake in humans (∼1 cup/day) (26). When whole grain and pulses are added to animal feed, the diet must be adjusted to maintain the original composition of whole grain and pulses and ensure the consistency of energy intake (27). The composition of HFD-CAB diet was matched to the HFD diet based on previous studies (26). Importantly, the HFD-CAB and HFD diets did not differ in macronutrient composition and were both isocaloric, so each macronutrient contributed the same to the caloric density of the diet. Body weight and food intake was measured once a week. After 12-weeks, fecal samples were collected and the mice were sacrificed after a 12-h overnight fast. Epididymal, perirenal, and retroperitoneal fat were rapidly removed and weighed.
Figure 1. Cooked adzuki bean reduces HFD-induced body weight gain and lipid accumulation in mice. (A) Experimental protocol and design, (B) Body weight time courses, (C) Weight gain, (D) Adipose tissue weight, (E) Adipose cell size, (F) H&E staining of Epi-WAT sections, (G) Food intake, and (H) Energy intake. Data presented as mean ± SEM, n = 7 per group. **P < 0.01 vs. LFD; #P < 0.05 and ##P < 0.01 vs. HFD. Epi-WAT epididymal fat; Per-WAT perirenal fat; Retro-WAT retroperitoneal fat; LFD, low-fat diet; HFD, high-fat diet; HFD-CAB, high-fat diet supplemented with cooked adzuki bean.
Blood samples were collected from the orbital vascular plexus and centrifuged at 3,000 rpm at 4°C for 10 min to obtain serum. The TG, total cholesterol (TC), high-density lipoprotein cholesterol (HDL-C), low-density lipoprotein cholesterol (LDL-C), ALT, and AST were measured with an automatic biochemistry analyzer (Hitachi Ltd., Tokyo, Japan).
Samples of adipose and liver tissue were fixed in 4% paraformaldehyde, and then stained with hematoxylin and eosin (H&E). The liver samples were also stained with Oil Red O solution.
The serum concentration of TNF-α and IL-6 were measured with enzyme-linked immunosorbent assay kits from Nanjing Jiancheng Bioengineering Institute. According to the manufacturer’s protocol, LPS was measured with enzyme-linked immunosorbent assay kit from Shanghai Enzyme-linked Biotechnology Co., Ltd.
According to the manufacturer’s protocol, microbial community genomic DNA was extracted from fecal samples using the E.Z.N.A. soil DNA Kit. The DNA purity and concentration were determined with a NanoDrop 2000 UV-vis spectrophotometer. The hypervariable region V3–V4 of the bacterial 16S rRNA gene was amplified with primer pairs 338F (5′-ACTCCTACGGGAGGCAGCAG-3′) and 806R (5′-GGACTACHVGGGTWTCTAAT-3′) with an ABI GeneAmp 9700 PCR thermocycler. The PCR product was extracted from 2% agarose gel, then purified and quantified. High-throughput sequencing was done by Majorbio (Shanghai Majorbio Bio-pharm Technology Co., Ltd., China).
The data were shown as mean ± SEM. Group differences were determined by One-Way ANOVA followed by Duncan test using SPSS 22.0 (SPSS Inc., Chicago, IL, United States) where P < 0.05 was considered statistically significant. Prism 5 (La Jolla, CA, United States) was used to prepare graphs.
At week 0, no significant difference occurred in body weight among the three groups (Figure 1B). After 12-weeks, mice in HFD group showed higher body weight compared with the LFD group, and mice in the HFD-CAB group had significantly lower body weight than the HFD group (Figure 1B, P < 0.01). Consistently, the weight gain of HFD-CAB group mice was significantly lower than that in the HFD group (Figure 1C, P < 0.01). Weight gain is usually accompanied by fat accumulation. HFD feeding led to a significant increase in white adipose tissue (Per-WAT, Epi-WAT, and Retro-WAT) weight, while cooked adzuki bean significantly reduced the weight of Epi-WAT and Per-WAT compared to the HFD group (Figure 1D, P < 0.05). In addition, HFD feeding significantly promoted adipocyte hypertrophy and increased adipocyte area, while cooked adzuki bean effectively inhibited the increase of adipocyte size compared with the HFD group (Figures 1E,F, P < 0.01). These results indicate that cooked adzuki bean reduces fat accumulation and body weight gain in HFD-fed mice. The inhibitory effects of cooked adzuki bean are not due to reduced food consumption, because no significant differences occurred in energy intake and food intake between the HFD-CAB and HFD groups (Figures 1G,H, P > 0.05).
As expected, HFD feeding induced dyslipidemia, manifested by significantly increased serum TC, TG, HDL-C, and LDL-C levels, while cooked adzuki bean led to a significant decrease in serum TG level (Figure 2A, P < 0.01). The liver injury of HFD group mice was indicated by significantly elevated serum AST and ALT levels. In contrast, compared with the HFD group cooked adzuki bean significantly reduced serum AST and ALT levels (Figure 2B, P < 0.05). These results indicate that cooked adzuki bean significantly ameliorates the high level of serum TG and liver injury in HFD-fed mice.
Figure 2. Cooked adzuki bean alleviates dyslipidemia and liver injury in HFD-fed mice. (A) Serum lipid profile, and (B) Serum ALT and AST levels. Data presented as mean ± SEM, n = 7 per group. **P < 0.01 vs. LFD; #P < 0.05 and ##P < 0.01 vs. HFD. TC total cholesterol; TG triacylglycerol; HDL-C high-density lipoprotein cholesterol; LDL-C low-density lipoprotein cholesterol; AST aspartate aminotransferase; ALT alanine aminotransferase; LFD, low-fat diet; HFD, high-fat diet; HFD-CAB, high-fat diet supplemented with cooked adzuki bean.
Histological analysis showed that HFD feeding caused lipid accumulation in mouse liver compared with the LFD group, accompanied by a large number of cytoplasmic vacuoles and hepatocyte swelling, whereas cooked adzuki bean reduced lipid accumulation and effectively inhibited hepatic steatosis (Figure 3).
Figure 3. Cooked adzuki bean limits HFD-induced hepatic steatosis. (A) H&E staining of liver tissue sections, and (B) Liver Oil Red O staining. n = 7 per group. LFD, low-fat diet; HFD, high-fat diet; HFD-CAB, high-fat diet supplemented with cooked adzuki bean.
As expected, compared with the LFD group HFD feeding caused significant increase in serum IL-6, TNF-α, and LPS in mice. In contrast, cooked adzuki bean maintained normal levels of IL-6, TNF-α, and LPS (Figure 4, P < 0.01). These results indicate that cooked adzuki bean significantly reduced HFD-induced inflammation and metabolic endotoxemia in mice.
Figure 4. Cooked adzuki bean ameliorates inflammation and metabolic endotoxemia in HFD-fed mice. (A) Serum tumor necrosis factor-α (TNF-α) concentrations, (B) Serum interleukin-6 (IL-6) concentrations, and (C) Serum lipopolysaccharide (LPS) levels. Data presented as mean ± SEM, n = 7 per group. *P < 0.05 and **P < 0.01 vs. LFD; ##P < 0.01 vs. HFD. LFD, low-fat diet; HFD, high-fat diet; HFD-CAB, high-fat diet supplemented with cooked adzuki bean.
More and more studies show that the gut microbiota is involved in the development of obesity and related diseases (28). Therefore, 16S rRNA high-throughput sequencing was applied to explore the effects of cooked adzuki bean on gut microbiota of mice fed a HFD. Alpha diversity is represented by the community richness (Chao and ACE indexes) and community diversity (Simpson and Shannon indexes). HFD feeding resulted in significantly decreased ACE, Chao, and Simpson indexes, and an increased Shannon index compared with the LFD group (Figures 5A,B, P < 0.05). In contrast, cooked adzuki bean significantly modulated the decline of ACE and Chao indexes induced by HFD, indicating that cooked adzuki bean significantly improved the community richness. To learn more about the influence of cooked adzuki bean on the structure and composition of gut microbiota, β diversity based on the NMDS score plot was performed. The more similar the gut microbiota composition of samples, the closer the distance in the NMDS diagram. The results showed that cooked adzuki bean had a significant effect on the gut microbiota composition and modulated the overall structure of gut microbiota damaged by HFD (Figure 5C). Venn diagram analysis was performed to understand the differences of gut microbiota in all groups of mice (Figure 5D). In the three groups of mice, 364 identical OTUs were identified. The LFD, HFD, and HFD-CAB groups showed 66, 17, and 89 unique OTUs, respectively.
Figure 5. Cooked adzuki bean modulates α and β diversity of gut microbiota in HFD-fed mice. (A) The community richness accessed by the ACE and Chao indexes, (B) The community diversity accessed by the Shannon and Simpson indexes, (C) Non-metric multidimensional scaling (NMDS) score plot based on Bray–Curtis, and (D) Venn diagram showing the OTUs shared by the mice from each group. Data presented as mean ± SEM, n = 7 per group. *P < 0.05 and **P < 0.01 vs. LFD; ##P < 0.01 vs. HFD. LFD, low-fat diet; HFD, high-fat diet; HFD-CAB, high-fat diet supplemented with cooked adzuki bean.
At the phylum level, the gut microbiota mainly includes Firmicutes, Bacteroidetes, Verrucomicrobia, Actinobacteria, and Proteobacteria (Figure 6A). At the family level, HFD feeding significantly enriched Desulfovibrionaceae and Deferribacteraceae, and decreased the abundance of Muribaculaceae (Figure 6B, P < 0.05). However, cooked adzuki bean significantly alleviated the changes in gut microbiota induced by HFD. At the genus level, the HFD group showed significant enrichment of Bilophila, Ruminiclostridium_9, Ruminiclostridium, unclassified_f_Ruminococcaceae, Mucispirillum, Oscillibacter, Enterorhabdus, Tyzzerella, Anaerotruncus, Intestinimonas, Ruminiclostridium_5, and a reduction in norank_f_Muribaculaceae and Anaeroplasma (Figure 6C). Cooked adzuki bean not only alleviated these changes, but also significantly increased the abundance of Lachnospiraceae_NK4A136_group. In short, cooked adzuki bean relieved the disorders of gut microbiota composition induced by HFD, contributing to the anti-obesity effect.
Figure 6. Cooked adzuki bean modulates microbial taxonomic profiles in HFD-fed mice. (A) Bacterial profile at the phylum level, (B) Relative abundance of Muribaculaceae, Desulfovibrionaceae, and Deferribacteraceae at the family level, and (C) Mean proportions of key genera in different groups. Data presented as mean ± SEM, n = 7 per group. *P < 0.05 and **P < 0.01 vs. LFD; ##P < 0.01 vs. HFD (B). *P < 0.05 and **P < 0.01 (C). LFD, low-fat diet; HFD, high-fat diet; HFD-CAB, high-fat diet supplemented with cooked adzuki bean.
LEfSe analysis was used to further screen bacteria enriched in different dietary groups to obtain a clade map and an LDA value distribution histogram. The levels of specific bacterial taxa varied from phylum to genus in each group (Figure 7A). LEfSe analysis indicated that Ruminococcaceae and Helicobacter had higher abundance in HFD group mice. The HFD-CAB group was characterized by a higher abundance of Lachnospiraceae, norank_f_Muribaculaceae, Muribaculaceae, and Lachnospiraceae_NK4A136_group (Figure 7B).
Figure 7. Effect of cooked adzuki bean supplementation on key phylotypes. (A) Cladogram generated from LEfSe analysis showing the relationship between taxon (the levels represent, from the inner to outer rings, phylum, class, order, family, and genus), and (B) linear discriminant analysis (LDA) scores derived from LEfSe analysis (the length of the bar represents the LDA score).
Spearman correlation analysis was used to identify the main gut microbiota affecting obesity-related indicators (Figure 8). The heat map shows that TG was positively correlated with Ruminiclostridium_5, Ruminiclostridium_9, Ruminiclostridium, Tyzzerella, Anaerotruncus, and Intestinimonas. ALT was positively correlated with Ruminiclostridium, Tyzzerella, and Anaerotruncus. IL-6 was negatively correlated with Muribaculaceae and norank_f_Muribaculaceae (Figure 8). LPS was negatively correlated with Muribaculaceae, norank_f_Muribaculaceae, Anaeroplasma, and Lachnospiraceae_NK4A136_group, and was positively correlated with Enterorhabdus. These bacteria may be critical to the development of obesity and its complications.
Figure 8. Heatmap of Spearman’s correlation between the gut microbiota and obesity-related indices. The intensity of the colors represented the degree of association (red, positive correlation; blue, negative correlation). Significant correlations are marked by *P < 0.05; **P < 0.01; ***P < 0.001.
At present, the incidence of obesity is increasing worldwide (1). HFDs promote the development of obesity, yet there is growing evidence that legume consumption can alleviate obesity (29). In this study, cooked adzuki bean significantly inhibited body weight gain, reduced serum TG and proinflammatory cytokines (IL-6, TNF-α, and LPS) levels, and alleviated obesity-related hepatic steatosis and liver injury. Importantly, the beneficial effects of cooked adzuki bean may be closely related to the improvement of gut microbiota imbalances. Evidence shows that legume consumption alleviates obesity by reducing weight gain and serum lipid levels (23). Consistent with previous studies, cooked adzuki bean significantly inhibited weight gain and reduced serum TG (Figures 1B, 2A). Interestingly, cooked adzuki bean had no effect on TC, HDL-C, and LDL-C (Figure 2A). A previous study found that heat treatment resulted in a significant reduction in the cholesterol-lowering properties of adzuki bean peptides (22). Long-term lipid metabolism disorders lead to liver injury. AST and ALT are likely the most sensitive indicators of liver function. It was shown previously that adzuki bean hot water extract containing polyphenols significantly alleviated HFD-induced liver injury (17). Similarly, cooked adzuki bean significantly reduced the levels of serum AST and ALT (Figure 2B). Due to extensive lipid accumulation in hepatocytes, obesity is often accompanied by hepatic steatosis, which also promotes the development of nonalcoholic fatty liver disease (NAFLD) (30). Previous studies found that adzuki bean supplementation attenuated lipid accumulation by inhibiting the expression of mRNA related to adipogenesis in the liver of NAFLD model mice (25). As expected, cooked adzuki bean also alleviated hepatic steatosis induced by HFD (Figure 3). These important beneficial effects of cooked adzuki bean on obese mice were not due to reduced food consumption, since no significant differences occurred in energy intake and food intake between the HFD and HFD-CAB groups (Figures 1G,H).
HFDs lead to hyperlipidemia, which in turn promotes the occurrence of inflammation. Inflammation is one of the main mechanisms affecting the development of obesity. TNF-α can be produced by adipocytes, and promote the production of IL-6 by activating the MAPK and NF-κB signaling pathways (31). IL-6 induces inflammation by affecting the secretory function of intestinal epithelial cells (32). It was shown previously that adzuki bean supplementation relieved inflammation by inhibiting the expression of proinflammatory mediators (TNF-α, caspase-3, and nuclear factor κB) in the liver of NAFLD model mice (25). In addition, adzuki bean peptides exhibited significant anti-inflammatory activity by inhibiting the expression of TNF-α and IL-6 (16). Adzuki bean extract containing polyphenols also led to a reduction in proinflammatory adipocytokines in human adipocytes (33). In this study, cooked adzuki bean significantly reduced serum IL-6 and TNF-α (Figures 4A,B). LPS is connected with elevated levels of TNF-α and IL-6, and can increase intestinal permeability and induce inflammation through the Toll-like receptor 4 signaling pathway (34, 35). HFDs lead to the increased serum LPS in human (36). Cooked adzuki bean significantly inhibited the high level of LPS induced by HFD, which may contribute to the alleviation of inflammation (Figure 4C). Previous studies have found that adzuki bean ethanol extract decreased the concentrations of LPS and various circulating proinflammatory cytokines such as IL-1β, TNF-α, and IL-6 in HFD-fed mice (37).
Many studies have shown that HFDs promote the development of obesity by inducing gut microbiota dysbiosis (38). As a shared substrate between the host and gut microbiota, diets have been reported to manage obesity by regulating the composition of the gut microbiota (39). Microbial diversity, including α diversity and β diversity, is an important biomarker in the obesity development (40). Cooked adzuki bean significantly inhibited the HFD-mediated decrease in ACE and Chao indexes (Figure 5A). Obese individuals with lower community richness have more weight gain over time (41). It has been reported that adzuki bean dietary fiber significantly increased the number of observed species (15). β diversity analysis also confirmed that cooked adzuki bean changed the overall structure of gut microbiota (Figure 5C). Previous studies have shown that both adzuki bean polyphenolic extract and heat-treated protein hydrolysates have significant effects on gut microbial community composition (12, 17).
Ruminococcaceae promotes fat synthesis (42). Ruminiclostridium_9 leads to abnormal lipid metabolism (43). In contrast, Lachnospiraceae_NK4A136_group is involved in the regulation of body weight (44). Norank_f_Muribaculaceae relieves abnormal lipid metabolism (23). In the present study, TG was positively correlated with Ruminiclostridium_5, Ruminiclostridium_9, Ruminiclostridium, Tyzzerella, Anaerotruncus, and Intestinimonas (Figure 8). Cooked adzuki bean significantly reduced the abundance of Ruminococcaceae, Ruminiclostridium_5, Ruminiclostridium_9, Ruminiclostridium, Tyzzerella, Anaerotruncus, and Intestinimonas, and increased the abundance of Lachnospiraceae_NK4A136_group and norank_f_Muribaculaceae, which may contribute to the alleviation of body weight gain and reduction in the level of serum TG (Figures 1B–F, 2A). It has been found that soybean insoluble dietary fiber significantly increased the abundance of Lachnospiraceae_NK4A136_group (45). Previous studies have also shown that raw adzuki bean significantly enriched the occurrence of norank_f_Muribaculaceae and Lachnospiraceae_NK4A136_group, and returned HFD-dependent taxa (Ruminiclostridium_9 and Ruminiclostridium) back to normal status (23). Interestingly, raw adzuki bean supplementation enriched the abundance of Bifidobacterium in obese mice, but this effect did not appear after cooked adzuki bean supplementation (23). Studies have found that polyphenols significantly increase the abundance of Bifidobacterium, because polyphenols are beneficial to the growth, proliferation or survival of gut microbiota (46, 47). A previous study found that processing caused a significant decrease in the adzuki bean polyphenols, which may be due to high temperature and the destruction of bean tissues during cooking (19, 48). Therefore, the difference of Bifidobacterium between raw adzuki bean and cooked adzuki bean supplementation may be due to the loss of adzuki bean polyphenols after processing.
HFDs can increase intestinal permeability and promote the entrance of inflammatory mediators into systemic circulation, thereby inducing systemic inflammation (49). Deferribacteraceae, Enterorhabdus, Anaerotruncus, Intestinimonas, Ruminiclostridium_5, and Ruminiclostridium_9 promote the development of inflammation (43, 50–54). In addition, Tyzzerella and unclassified_f_Ruminococcaceae are positively correlated with pro-inflammatory cytokines (55, 56). In contrast, Lachnospiraceae_NK4A136_group, Lachnospiraceae, norank_f_Muribaculaceae, and Anaeroplasma have anti-inflammatory effects (57–60). In this study, IL-6 was negatively correlated with Muribaculaceae and norank_f_Muribaculaceae (Figure 8). Cooked adzuki bean significantly decreased the abundance of Deferribacteraceae, Enterorhabdus, Anaerotruncus, Intestinimonas, Ruminiclostridium_5, Ruminiclostridium_9, Tyzzerella, and unclassified_f_Ruminococcaceae, and enriched the Lachnospiraceae_NK4A136_group, Lachnospiraceae, norank_f_Muribaculaceae, Anaeroplasma, and Muribaculaceae, all of which may contribute to the alleviation of inflammation (Figures 4A,B). It has been reported that heat-treated adzuki bean protein hydrolysates significantly increased the abundance of Anaeroplasma in mice fed a HFD (12). Mung bean protein supplementation can significantly enrich Muribaculaceae (61).
Gut microbiota is the part of intestinal barrier and plays a key role in maintaining intestinal health. When leaky gut occurs, harmful bacteria and their metabolites (e.g., LPS) invade the intestinal and circulatory system, causing the release of proinflammatory cytokines and leading to metabolic endotoxemia (62). HFD feeding increases the circulating LPS concentration, which is closely related to obesity-related inflammation (63). Desulfovibrionaceae, Helicobacter, and Bilophila produce LPS (64–67). In addition, Mucispirillum and Ruminococcaceae damage the intestinal mucosa (68, 69). Oscillibacter and Ruminiclostridium are related to intestinal dysfunction (70–73). In contrast, Muribaculaceae is beneficial to the health of the intestinal epithelium (74). In this study, LPS was negatively correlated with Muribaculaceae, norank_f_Muribaculaceae, Anaeroplasma, and Lachnospiraceae_NK4A136_group, and was positively correlated with Enterorhabdus (Figure 8). Cooked adzuki bean significantly reduced the abundance of LPS-producing bacteria (Desulfovibrionaceae,Helicobacter,Bilophila), Mucispirillum, Ruminococcaceae, Oscillibacter, Ruminiclostridium, and Enterorhabdus, and enriched Muribaculaceae, norank_f_ Muribaculaceae, Anaeroplasma, and Lachnospiraceae_NK4A136_ group, which have contributed to the reduction of serum LPS and the maintenance of intestinal health (Figure 4C). Similarly, heat-treated adzuki bean protein hydrolysates significantly decreased the abundance of Bilophila and Mucispirillum in HFD-fed mice (12).
In conclusion, cooked adzuki bean showed beneficial effects on HFD-induced obesity in mice by inhibiting body weight gain, reducing the levels of serum TG, ALT, and AST, and limiting hepatic steatosis (Figure 9). Of particular significance, cooked adzuki bean also improved systemic inflammation and metabolic endotoxemia, and maintained intestinal health, indicating that adzuki bean is an effective functional food for preventing obesity and related complications. Yet to be clarified is the relationship between the positive effects of cooked adzuki bean on obesity and the adzuki bean-mediated changes of gut microbiota composition. Consequently, further work is needed to elucidate the specific mechanisms of the beneficial effects of cooked adzuki bean supplementation.
Figure 9. Schematic representation of the anti-obesity effect of cooked adzuki bean via gut microbiota.
The original contributions presented in this study are included in the article/Supplementary Material, further inquiries can be directed to the corresponding author.
The animal study was reviewed and approved by the Animal Care Committee of China Agricultural University (AW09089102-4).
QZ: conceptualization, methodology, investigation, data curation, and writing – original draft. ZL, YZ, HW, ZD, XY, XR, and YX: supervision. QS: writing – review and editing and funding acquisition. All authors contributed to the article and approved the submitted version.
This work was financially supported by the China key research and development program under Grant No. 2018YFE0206300-02 and cooperation project between China Agricultural University and Datong City.
XY was employed by the Cofco Nutrition and Health Research Institute Co., Ltd.
The remaining authors declare that the research was conducted in the absence of any commercial or financial relationships that could be construed as a potential conflict of interest.
All claims expressed in this article are solely those of the authors and do not necessarily represent those of their affiliated organizations, or those of the publisher, the editors and the reviewers. Any product that may be evaluated in this article, or claim that may be made by its manufacturer, is not guaranteed or endorsed by the publisher.
The Supplementary Material for this article can be found online at: https://www.frontiersin.org/articles/10.3389/fnut.2022.918696/full#supplementary-material
1. Choi WJ, Dong HJ, Jeong HU, Ryu DW, Song SM, Kim YR, et al. Lactobacillus plantarum LMT1-48 exerts anti-obesity effect in high-fat diet-induced obese mice by regulating expression of lipogenic genes. Sci Rep. (2020) 10:869. doi: 10.1038/s41598-020-57615-5
2. Lumeng CN, Saltiel AR. Inflammatory links between obesity and metabolic disease. J Clin Invest. (2011) 121:2111–7. doi: 10.1172/jci57132
3. McGinley JN, Fitzgerald VK, Neil ES, Omerigic HM, Heuberger AL, Weir TL, et al. Pulse crop effects on gut microbial populations, intestinal function, and adiposity in a mouse model of diet-induced obesity. Nutrients. (2020) 12:593. doi: 10.3390/nu12030593
4. Canfora EE, Meex RCR, Venema K, Blaak EE. Gut microbial metabolites in obesity, NAFLD and T2DM. Nat Rev Endocrinol. (2019) 15:261–73. doi: 10.1038/s41574-019-0156-z
5. Requena T, Martinez-Cuesta MC, Pelaez C. Diet and microbiota linked in health and disease. Food Funct. (2018) 9:688–704. doi: 10.1039/c7fo01820g
6. Xu JL, Liu TT, Li YY, Liu W, Ding ZJ, Ma H, et al. Jamun (Eugenia jambolana Lam.) fruit extract prevents obesity by modulating the gut microbiome in high-fat-diet-fed mice. Mol Nutr Food Res. (2019) 63:1801307. doi: 10.1002/mnfr.201801307
7. Daskalopoulou SS, Mikhailidis DP, Elisaf A. Prevention and treatment of the metabolic syndrome. Angiology. (2004) 55:589–612. doi: 10.1177/000331970405500601
8. Cani PD, Bibiloni R, Knauf C, Neyrinck AM, Neyrinck AM, Delzenne NM, et al. Changes in gut microbiota control metabolic endotoxemia-induced inflammation in high-fat diet-induced obesity and diabetes in mice. Diabetes. (2008) 57:1470–81. doi: 10.2337/db07-1403
9. David LA, Maurice CF, Carmody RN, Gootenberg DB, Button JE, Wolfe BE, et al. Diet rapidly and reproducibly alters the human gut microbiome. Nature. (2014) 505:559. doi: 10.1038/nature12820
10. Itoh T, Furuichi Y. Lowering serum cholesterol level by feeding a 40% ethanol-eluted fraction from HP-20 resin treated with hot water extract of adzuki beans (Vigna angularis) to rats fed a high-fat cholesterol diet. Nutrition. (2009) 25:318–21. doi: 10.1016/j.nut.2008.08.011
11. Ha TJ, Park JE, Lee KS, Seo WD, Song SB, Lee MH, et al. Identification of anthocyanin compositions in black seed coated Korean adzuki bean (Vigna angularis) by NMR and UPLC-Q-Orbitrap-MS/MS and screening for their antioxidant properties using different solvent systems. Food Chem. (2021) 346:128882. doi: 10.1016/j.foodchem.2020.128882
12. Zhao QY, Fu YX, Zhang F, Wang C, Yang XH, Bai SQ, et al. Heat-treated adzuki bean protein hydrolysates reduce obesity in mice fed a high-fat diet via remodeling gut microbiota and improving metabolic function. Mol Nutr Food Res. (2022) 66:e2100907. doi: 10.1002/mnfr.202100907
13. Liu R. Anti-Obesity Effects of Flavonoids and Saponins From Adzuki Bean. Hong Kong: Hong Kong Baptist University (2014).
14. Han KH, Iijuka M, Shimada K, Sekikawa M, Kuramochi K, Ohba K, et al. Adzuki resistant starch lowered serum cholesterol and hepatic 3-hydroxy-3-methylglutaryl-CoA mRNA levels and increased hepatic LDL-receptor and cholesterol 7 alpha-hydroxylase mRNA levels in rats fed a cholesterol diet. Brit J Nutr. (2005) 94:902–8. doi: 10.1079/bjn20051598
15. Han K-H, Ohashi S, Sasaki K, Nagata R, Pelpolage S, Fukuma N, et al. Dietary adzuki bean paste dose-dependently reduces visceral fat accumulation in rats fed a normal diet. Food Res Int. (2020) 130:108890. doi: 10.1016/j.foodres.2019.108890
16. Shi Z, Dun B, Wei Z, Liu C, Tian J, Ren G, et al. Peptides released from extruded adzuki bean protein through simulated gastrointestinal digestion exhibit anti-inflammatory activity. J Agric Food Chem. (2021) 69:7028–36. doi: 10.1021/acs.jafc.1c01712
17. Lee PS, Teng CY, Hsieh KF, Chiou YS, Wu JC, Lu TJ, et al. Adzuki bean water extract attenuates obesity by modulating M2/M1 macrophage polarization and gut microbiota composition. Mol Nutr Food Res. (2019) 63:1900626. doi: 10.1002/mnfr.201900626
18. Shi L, Arntfield SD, Nickerson M. Changes in levels of phytic acid, lectins and oxalates during soaking and cooking of Canadian pulses. Food Res Int. (2018) 107:660–8. doi: 10.1016/j.foodres.2018.02.056
19. Jung IC, Lee Y-S, Kang D-K, Sohn HY. Evaluation of useful biological activities of hot-water extracts of raw-red bean and boiled-red bean (Phaseolus radiatus L.). J East Asian Soc Diet Life. (2015) 25:451–9.
20. Luo Y, Li J, Xu C, Hao Z, Jin X, Wang Q. Impact of processing on in vitro bioavailability of phenols and flavonodis and antioxidant activities in faba bean (Vicia faba L.) andazuki bean (Vigna angularis L.). Legume Res. (2014) 37:492–9. doi: 10.5958/0976-0571.2014.00665.1
21. Zhao QY, Hou DZ, Laraib Y, Xue Y, Shen Q. Comparison of the effects of raw and cooked adzuki bean on glucose/lipid metabolism and liver function in diabetic mice. Cereal Chem. (2021) 98:1081–90. doi: 10.1002/cche.10456
22. Ashraf J, Awais M, Liu LY, Khan MI, Tong LT, Ma YL, et al. Effect of thermal processing on cholesterol synthesis, solubilisation into micelles and antioxidant activities using peptides of Vigna angularis and Vicia faba. LWT Food Sci Technol. (2020) 129:109504. doi: 10.1016/j.lwt.2020.109504
23. Zhao QY, Hou DZ, Fu YX, Xue Y, Guan X, Shen Q. Adzuki bean alleviates obesity and insulin resistance induced by a high-fat diet and modulates gut microbiota in mice. Nutrients. (2021) 13:3240. doi: 10.3390/nu13093240
24. Chinese Nutrition Society. Dietary Guidelines for Chinese Residents. Beijing: People’s Medical Publishing House (2022).
25. Kim S, Hong J, Jeon R, Kim HS. Adzuki bean ameliorates hepatic lipogenesis and proinflammatory mediator expression in mice fed a high-cholesterol and high-fat diet to induce nonalcoholic fatty liver disease. Nutr Res. (2016) 36:90–100. doi: 10.1016/j.nutres.2015.11.002
26. Monk JM, Wu W, Lepp D, Wellings HR, Hutchinson AL, Liddle DM, et al. Navy bean supplemented high-fat diet improves intestinal health, epithelial barrier integrity and critical aspects of the obese inflammatory phenotype. J Nutr Biochem. (2019) 70:91–104. doi: 10.1016/j.jnutbio.2019.04.009
27. Liu J, Wang Y, Xue L, Nie C, Sun J, Fan M, et al. Novel metabolic regulation of bile acid responses to low cholesterol in whole-grain-diet-fed mice. J Agr Food Chem. (2021) 69:8440–7. doi: 10.1021/acs.jafc.1c02662
28. Dalby MJ, Ross AW, Walker AW, Morgan PJ. Dietary uncoupling of gut microbiota and energy harvesting from obesity and glucose tolerance in mice. Cell Rep. (2017) 21:1521–33. doi: 10.1016/j.celrep.2017.10.056
29. Moreno-Valdespino CA, Luna-Vital D, Camacho-Ruiz RM, Mojica L. Bioactive proteins and phytochemicals from legumes: mechanisms of action preventing obesity and type-2 diabetes. Food Res Int. (2020) 130:108905. doi: 10.1016/j.foodres.2019.108905
30. Friedman SL, Neuschwander-Tetri BA, Rinella M, Sanyal AJ. Mechanisms of NAFLD development and therapeutic strategies. Nat Med. (2018) 24:908–22. doi: 10.1038/s41591-018-0104-9
31. McArdle MA, Finucane OM, Connaughton RM, McMorrow AM, Roche HM. Mechanisms of obesity-induced inflammation and insulin resistance: insights into the emerging role of nutritional strategies. Front Endocrinol. (2013) 4:52. doi: 10.3389/fendo.2013.00052
32. Liu JL, Gao YY, Zhou J, Tang XY, Wang P, Shen LW, et al. Changes in serum inflammatory cytokine levels and intestinal flora in a self-healing dextran sodium sulfate-induced ulcerative colitis murine model. Life Sci. (2020) 263:118587. doi: 10.1016/j.lfs.2020.118587
33. Kitano-Okada T, Ito A, Koide A, Nakamura Y, Han KH, Shimada K, et al. Anti-obesity role of adzuki bean extract containing polyphenols: in vivo and in vitro effects. J Sci Food Agric. (2012) 92:2644–51. doi: 10.1002/jsfa.5680
34. Ghanim H, Abuaysheh S, Sia CL, Korzeniewski K, Chaudhuri A, Fernandez-Real JM, et al. Increase in plasma endotoxin concentrations and the expression of toll-like receptors and suppressor of cytokine signaling-3 in mononuclear cells after a high-fat, high-carbohydrate meal implications for insulin resistance. Diabetes Care. (2009) 32:2281–7. doi: 10.2337/dc09-0979
35. Guo SH, Nighot M, Al-Sadi R, Alhmoud T, Nighot P, Ma TY. Lipopolysaccharide regulation of intestinal tight junction permeability is mediated by TLR4 signal transduction pathway activation of FAK and MyD88. J Immunol. (2015) 195:4999–5010. doi: 10.4049/jimmunol.1402598
36. Harte AL, Varma MC, Tripathi G, McGee KC, Al-Daghri NM, Al-Attas OS, et al. High fat intake leads to acute postprandial exposure to circulating endotoxin in type 2 diabetic subjects. Diabetes Care. (2012) 35:375–82. doi: 10.2337/dc11-1593
37. Yook JS, Kim KA, Kim M, Cha YS. Black adzuki bean (Vigna angularis) attenuates high-fat diet-induced colon inflammation in mice. J Med Food. (2017) 20:367–75. doi: 10.1089/jmf.2016.3821
38. Li YY, Cui Y, Hu XS, Liao XJ, Zhang Y. Chlorophyll supplementation in early life prevents diet-induced obesity and modulates gut microbiota in mice. Mol Nutr Food Res. (2019) 63:1801219. doi: 10.1002/mnfr.201801219
39. Nogacka AM, de los Reyes-Gavilan CG, Martinez-Faedo C, Ruas-Madiedo P, Suarez A, Mancabelli L, et al. Impact of extreme obesity and diet-induced weight loss on the fecal metabolome and gut microbiota. Mol Nutr Food Res. (2021) 65:2000030. doi: 10.1002/mnfr.202000030
40. Ley RE, Turnbaugh PJ, Klein S, Gordon JI. Microbial ecology – human gut microbes associated with obesity. Nature. (2006) 444:1022–3. doi: 10.1038/4441022a
41. Le Chatelier E, Nielsen T, Qin JJ, Prifti E, Hildebrand F, Falony G, et al. Richness of human gut microbiome correlates with metabolic markers. Nature. (2013) 500:541. doi: 10.1038/nature12506
42. Gong S, Ye T, Wang M, Wang M, Li Y, Ma L, et al. Traditional Chinese medicine formula Kang Shuai Lao Pian improves obesity, gut dysbiosis, and fecal metabolic disorders in high-fat diet-fed mice. Front Pharmacol. (2020) 11:297. doi: 10.3389/fphar.2020.00297
43. Wang K, Jin X, Li Q, Sawaya ACHF, Le Leu RK, Conlon MA, et al. Propolis from different geographic origins decreases intestinal inflammation and Bacteroides spp. Populations in a model of DSS-induced colitis. Mol Nutr Food Res. (2018) 62:e1800080. doi: 10.1002/mnfr.201800080
44. Wang P, Li DT, Ke WX, Liang D, Hu XS, Chen F. Resveratrol-induced gut microbiota reduces obesity in high-fat diet-fed mice. Int J Obesity. (2020) 44:213–25. doi: 10.1038/s41366-019-0332-1
45. Wang BX, Yu HS, He Y, Wen LK, Gu JD, Wang XY, et al. Effect of soybean insoluble dietary fiber on prevention of obesity in high-fat diet fed mice via regulation of the gut microbiota. Food Funct. (2021) 12:7923–37. doi: 10.1039/d1fo00078k
46. Huang JC, Chen L, Xue B, Liu QY, Ou SY, Wang Y, et al. Different flavonoids can shape unique gut microbiota profile in vitro. J Food Sci. (2016) 81:H2273–9. doi: 10.1111/1750-3841.13411
47. Hervert-Hernandez D, Goni I. Dietary polyphenols and human gut microbiota: a review. Food Rev Int. (2011) 27:154–69. doi: 10.1080/87559129.2010.535233
48. Kebe M, Renard C, El Maataoui M, Amani GNG, Maingonnat JF. Leaching of polyphenols from apple parenchyma tissue as influenced by thermal treatments. J Food Eng. (2015) 166:237–46. doi: 10.1016/j.jfoodeng.2015.05.037
49. Cani PD, Possemiers S, Van de Wiele T, Guiot Y, Everard A, Rottier O, et al. Changes in gut microbiota control inflammation in obese mice through a mechanism involving GLP-2-driven improvement of gut permeability. Gut. (2009) 58:1091–103. doi: 10.1136/gut.2008.165886
50. Zhang H, Zhang Z, Song G, Tang X, Song H, Deng A, et al. Development of an XBP1 agonist, HLJ2, as a potential therapeutic agent for ulcerative colitis. Eur J Pharm Sci. (2017) 109:56–64. doi: 10.1016/j.ejps.2017.07.028
51. Yusufu I, Ding KH, Smith K, Wankhade UD, Sahay B, Patterson GT, et al. A tryptophan-deficient diet induces gut microbiota dysbiosis and increases systemic inflammation in aged mice. Int J Mol Sci. (2021) 22:5005. doi: 10.3390/ijms22095005
52. Li XQ, Chen Y, Dai GC, Zhou BB, Yan XN, Tan RX. Abietic acid ameliorates psoriasis -like inflammation and modulates gut microbiota in mice. J Ethnopharmacol. (2021) 272:113934. doi: 10.1016/j.jep.2021.113934
53. Wu Y, Chen Y, Li Q, Ye X, Guo X, Sun L, et al. Tetrahydrocurcumin alleviates allergic airway inflammation in asthmatic mice by modulating the gut microbiota. Food Funct. (2021) 12:6830–40. doi: 10.1039/d1fo00194a
54. Wu R, Ma L, Li P. Effect of chondroitin sulfate from sturgeon on intestinal flora of mice with colorectal cancer. Food Sci. (2020) 41:216–23. doi: 10.7506/spkx1002-6630-20200209-065
55. Huang J, Huang JL, Li Y, Wang YL, Wang FH, Qiu X, et al. Sodium alginate modulates immunity, intestinal mucosal barrier function, and gut microbiota in cyclophosphamide-induced immunosuppressed BALB/c mice. J Agric Food Chem. (2021) 69:7064–73. doi: 10.1021/acs.jafc.1c02294
56. Tang C, Sun J, Zhou B, Jin C, Liu J, Kan J, et al. Effects of polysaccharides from purple sweet potatoes on immune response and gut microbiota composition in normal and cyclophosphamide treated mice. Food Funct. (2018) 9:937–50. doi: 10.1039/c7fo01302g
57. Xia T, Duan W, Zhang Z, Li S, Zhao Y, Geng B, et al. Polyphenol-rich vinegar extract regulates intestinal microbiota and immunity and prevents alcohol-induced inflammation in mice. Food Res Int. (2021) 140:110064. doi: 10.1016/j.foodres.2020.110064
58. Berger K, Burleigh S, Lindahl M, Bhattacharya A, Patil P, Stalbrand H, et al. Xylooligosaccharides increase bifidobacteria and lachnospiraceae in mice on a high-fat diet, with a concomitant increase in short-chain fatty acids, especially butyric acid. J Agric Food Chem. (2021) 69:3617–25. doi: 10.1021/acs.jafc.0c06279
59. Huang H, Li M, Wang Y, Wu X, Shen J, Xiao Z, et al. Excessive intake of longan arillus alters gut homeostasis and aggravates colitis in mice. Front Pharmacol. (2021) 12:640417. doi: 10.3389/fphar.2021.640417
60. Beller A, Kruglov A, Durek P, von Goetze V, Hoffmann U, Maier R, et al. Anaeroplasma, a potential anti-inflammatory probiotic for the treatment of chronic intestinal inflammation. Ann Rheum Dis. (2019) 78:A45–6. doi: 10.1136/annrheumdis-2018-EWRR2019.92
61. Zhang S, Ma YT, Feng YC, Wang CY, Zhang DJ. Potential effects of mung bean protein and a mung bean protein-polyphenol complex on oxidative stress levels and intestinal microflora in aging mice. Food Funct. (2022) 13:186–97. doi: 10.1039/d1fo03058b
62. Upadhyaya S, Banerjee G. Type 2 diabetes and gut microbiome: at the intersection of known and unknown. Gut Microbes. (2015) 6:85–92. doi: 10.1080/19490976.2015.1024918
63. Cani PD, Jordan BF. Gut microbiota-mediated inflammation in obesity: a link with gastrointestinal cancer. Nat Rev Gastro Hepat. (2018) 15:671–82. doi: 10.1038/s41575-018-0025-6
64. Liu JH, Hao WJ, He ZY, Kwek E, Zhao YM, Zhu HY, et al. Beneficial effects of tea water extracts on the body weight and gut microbiota in C57BL/6J mice fed with a high-fat diet. Food Funct. (2019) 10:2847–60. doi: 10.1039/c8fo02051e
65. Tian BM, Zhao JH, Zhang M, Chen ZF, Ma QY, Liu HC, et al. Lycium ruthenicum anthocyanins attenuate high-fat diet-induced colonic barrier dysfunction and inflammation in mice by modulating the gut microbiota. Mol Nutr Food Res. (2021) 65:2000745. doi: 10.1002/mnfr.202000745
66. Al-odaini A, Singh N. Induction of IL in bone marrow derived macrophages by Helicobacter hepaticus supernatant is mediated by Gpr109a. FASEB J. (2019) 33:lb6. doi: 10.1096/fasebj.2019.33.1_supplement.lb6
67. Zhuang P, Zhang Y, Shou QY, Li HY, Zhu YE, He LL, et al. Eicosapentaenoic and docosahexaenoic acids differentially alter gut microbiome and reverse high-fat diet-induced insulin resistance. Mol Nutr Food Res. (2020) 64:e1900946. doi: 10.1002/mnfr.201900946
68. Xie Z, Bai Y, Chen G, Rui Y, Chen D, Sun Y, et al. Modulation of gut homeostasis by exopolysaccharides from Aspergillus cristatus (MK346334), a strain of fungus isolated from Fuzhuan brick tea, contributes to immunomodulatory activity in cyclophosphamide-treated mice. Food Funct. (2020) 11:10397–412. doi: 10.1039/d0fo02272a
69. Yang H, Pan RH, Wang J, Zheng LZ, Li ZJ, Guo QB, et al. Modulation of the gut microbiota and liver transcriptome by red yeast rice and monascus pigment fermented by purple monascus SHM1105 in rats fed with a high-fat diet. Front Pharmacol. (2021) 11:14. doi: 10.3389/fphar.2020.599760
70. Wu MN, Li PZ, An YY, Ren J, Yan D, Cui JZ, et al. Phloretin ameliorates dextran sulfate sodium-induced ulcerative colitis in mice by regulating the gut microbiota. Pharmacol Res. (2019) 150:104489. doi: 10.1016/j.phrs.2019.104489
71. Lam YY, Ha CWY, Campbell CR, Mitchell AJ, Dinudom A, Oscarsson J, et al. Increased gut permeability and microbiota change associate with mesenteric fat inflammation and metabolic dysfunction in diet-induced obese mice. PLoS One. (2012) 7:e34233. doi: 10.1371/journal.pone.0034233
72. Jia Y, Liao SI, He Y, Shan W, Zhou HE. Dysbiosis of gut microbiota with reduced trimethylamine-N-oxide level in patients with large-artery atherosclerotic stroke or transient ischemic attack. J Am Heart Assoc. (2015) 4:e002699. doi: 10.1161/JAHA.115.002699
73. Ran BB, Guo CE, Li WD, Li WS, Wang Q, Qian JX, et al. Sea buckthorn (Hippophae rhamnoides L.) fermentation liquid protects against alcoholic liver disease linked to regulation of liver metabolome and the abundance of gut microbiota. J Sci Food Agric. (2021) 101:2846–54. doi: 10.1002/jsfa.10915
Keywords: cooked adzuki bean, obesity, inflammation, metabolic endotoxemia, gut microbiota
Citation: Zhao Q, Liu Z, Zhu Y, Wang H, Dai Z, Yang X, Ren X, Xue Y and Shen Q (2022) Cooked Adzuki Bean Reduces High-Fat Diet-Induced Body Weight Gain, Ameliorates Inflammation, and Modulates Intestinal Homeostasis in Mice. Front. Nutr. 9:918696. doi: 10.3389/fnut.2022.918696
Received: 12 April 2022; Accepted: 16 May 2022;
Published: 09 June 2022.
Edited by:
Mohammad Moniruzzaman, Jeju National University, South KoreaReviewed by:
Lina Zhang, Jiangnan University, ChinaCopyright © 2022 Zhao, Liu, Zhu, Wang, Dai, Yang, Ren, Xue and Shen. This is an open-access article distributed under the terms of the Creative Commons Attribution License (CC BY). The use, distribution or reproduction in other forums is permitted, provided the original author(s) and the copyright owner(s) are credited and that the original publication in this journal is cited, in accordance with accepted academic practice. No use, distribution or reproduction is permitted which does not comply with these terms.
*Correspondence: Qun Shen, c2hlbnF1bkBjYXUuZWR1LmNu
Disclaimer: All claims expressed in this article are solely those of the authors and do not necessarily represent those of their affiliated organizations, or those of the publisher, the editors and the reviewers. Any product that may be evaluated in this article or claim that may be made by its manufacturer is not guaranteed or endorsed by the publisher.
Research integrity at Frontiers
Learn more about the work of our research integrity team to safeguard the quality of each article we publish.