- Hunan Collaborative Innovation Center for Utilization of Botanical Functional Ingredients, College of Animal Science and Technology, Hunan Agricultural University, Changsha, China
Objective: This study aimed to evaluate the effect of an antibiotic cocktail on gut microbiota and provide a reference for establishing an available mouse model for fecal microbiota transplantation (FMT) of specific microbes.
Design: C57BL/6J mice (n = 24) had free access to an antibiotic cocktail containing vancomycin (0.5 g/L), ampicillin (1 g/L), neomycin (1 g/L), and metronidazole (1 g/L) in drinking water for 3 weeks. Fecal microbiota was characterized by 16S rDNA gene sequencing at the beginning, 1st week, and 3rd week, respectively. The mice were then treated with fecal microbiota from normal mice for 1 week to verify the efficiency of FMT.
Results: The diversity of microbiota including chao1, observed species, phylogenetic diversity (PD) whole tree, and Shannon index were decreased significantly (P < 0.05) after being treated with the antibiotic cocktail for 1 or 3 weeks. The relative abundance of Bacteroidetes, Actinobacteria, and Verrucomicrobia was decreased by 99.94, 92.09, and 100%, respectively, while Firmicutes dominated the microbiota at the phylum level after 3 weeks of treatment. Meanwhile, Lactococcus, a genus belonging to the phylum of Firmicutes dominated the microbiota at the genus level with a relative abundance of 80.63%. Further FMT experiment indicated that the fecal microbiota from the receptor mice had a similar composition to the donor mice after 1 week.
Conclusion: The antibiotic cocktail containing vancomycin, ampicillin, neomycin, and metronidazole eliminates microbes belonging to Bacteroidetes, Actinobacteria, and Verrucomicrobia, which can be recovered by FMT in mice.
Introduction
Fecal microbiota transplantation (FMT) has been widely used as an intervention method in the reconstruction of receptor gut microbiota in mice-model experiments against various diseases, such as obesity (1), colitis (2), clostridium difficile infection (3) and spinal cord injury (4). However, donor-derived microbiota transplanted into receptor guts is not easy to achieve colonization since indigenous microbiota is dominant and stable (5). Hence, suitable intestinal preparation is vital in the pretreatment of FMT to attain a susceptible microbiota environment. Antibiotic treatments for mice are more fundamental and economical than for germ-free (GF) mice because GF mice may induce global developmental changes in receptor guts, which can mask disease-specific attributes of donor material (6). However, methods of antibiotic treatments before FMT have been formed diversely, such as composition of antibiotics (7), administration time (8), and concentrations (9).
The antibiotic cocktail containing vancomycin, ampicillin, neomycin, and metronidazole have been adopted widely in mice (10–12). Vancomycin belonging to glycopeptide antibiotics, ampicillin belonging to beta-lactam antibiotics, neomycin belonging to aminoglycoside antibiotics, and metronidazole belonging to nitroimidazoles antibiotics constitute the most common classes of antibiotics against gram-positive bacteria, aerobes or anaerobes (13). The underlying antibacterial mechanisms of these single categories of antibiotics have been well studied (14, 15). However, studies that aim to assess the influence of antibiotic cocktails on different specific microbes are rare, although the antibiotic cocktail may lead to loss of diversity and changes in microbes’ composition (16, 17). Importantly, pieces of evidence are needed to understand the characteristics of microbes after treatment with the antibiotic cocktail to ensure the availability of the antibiotic cocktail and establish an FMT-based non-specific microbe mouse model. Consequently, this study aimed to evaluate the effect of an antibiotic cocktail containing vancomycin, ampicillin, neomycin, and metronidazole in drinking water on the composition of gut microbiota, and further verify the effectiveness of FMT.
Materials and Methods
Diets and Reagents
Mouse feed (D12450J) was purchased from Research Diets Inc. (New Brunswick, NJ, United States), and the diet composition was shown in Supplementary Table 1. Antibiotics including vancomycin, ampicillin, neomycin, and metronidazole were purchased from Shanghai Yuanye Bio-Technology Co., Ltd. (Shanghai, China).
Mouse Model and Experimental Design
The experimental procedures were approved by the Hunan Agricultural University Institutional Animal Care and Use Committee (Permission No. 2020A34). Mice and sterilized poplar bedding were purchased from Hunan Slake Jingda Laboratory Animal Co., Ltd. (License No. SCXK-Xiang 2019-0004, Changsha, Hunan, China). Mice were housed separately in cages with a sterile environment under controlled temperature (23.5°C) and light (12 h light/day) and had free access to feed, drinking water, or an antibiotic cocktail containing vancomycin (0.5 g/L), ampicillin (1 g/L), neomycin (1 g/L), and metronidazole in drinking water.
A total of twenty-four C57BL/6J mice (SPF class, male, and 4 weeks of age) were raised separately with free access to an antibiotic cocktail containing vancomycin (0.5 g/L), ampicillin (1 g/L), neomycin (1 g/L), and metronidazole (1 g/L) in drinking water for consecutive 3 weeks. Then, mice were treated with clean drinking water for 3 days to avoid the interference of antibiotic cocktails on FMT, followed by FMT for 1 week. FMT experiment was conducted with a modified method based on Gong et al. (12). Briefly, fecal samples were collected sterilely through mild stimulation of the anus in mice, and resuspended in sterile phosphate buffer solution (PBS) at 0.125 g/ml, followed by low-speed (800 × g) centrifugation (Low-Speed Bench Centrifuge, TD4 angle rotor, Hunan Michael Laboratory Instrument Co., Ltd., Changsha, China) for 10 min. Ultimately, 0.15 ml of that supernatant was administered to mice by oral gavage once a day for up to consecutive 7 days. Feces of mice at the beginning of the experiment (0 W), the first week (1 W), the third week (3 W), as well as feces from donor mice (dM) and receptor mice (rM) were collected for characterization of microbiota (Figure 1A).
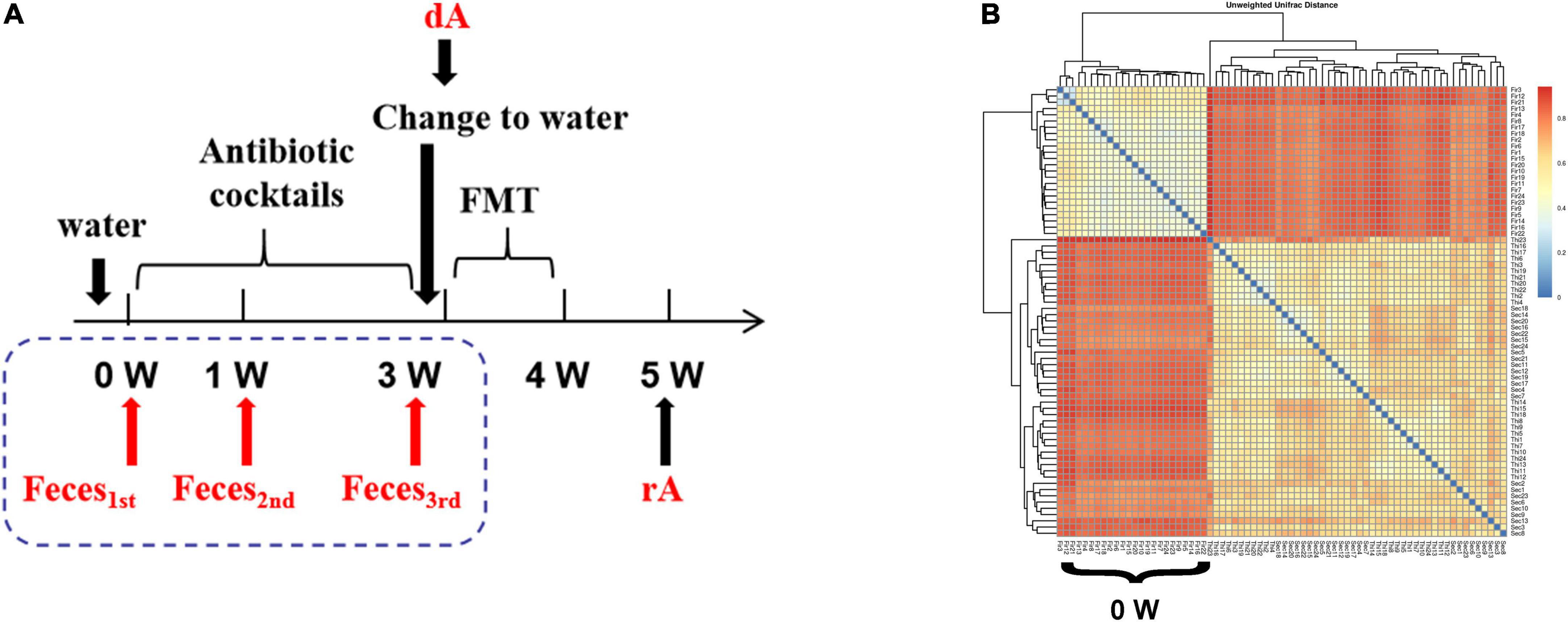
Figure 1. The cluster of fecal microbiota. (A) Experimental design drawing. Receptor mice had free access to antibiotic cocktails containing vancomycin (0.5 g/L), ampicillin (1 g/L), neomycin (1 g/L), and metronidazole (1 g/L) in drinking water. Feces were collected on the first day of the experiment (0 W), the first week of the experiment (1 W), the third week of the experiment (3W), as well as feces from donor mice (dM) fed with normal diet and feces from receptor mice (rM) when FMT ended over another 1 week. All mice were fed a normal diet. (B) β-diversity indices of unweighted_unifrac_distance. The redder color is, the farther distance is. dM, donor mice fed with a normal diet; FMT, fecal microbiota transplantation; PLS-DA, partial least squares discriminant analysis; rM, receptor mice transplanted with microbiota from dM.
Analysis of Gut Microbiota
Total DNA of feces was extracted by using a Stool DNA Isolation Kit (Tiangen Biotech Co., Ltd., Beijing, China). DNA quality was detected and controlled by Nanodrop (Thermo Fisher Scientific Inc., Rockford, IL, United States), and then 30 ng DNA was used for PCR amplification. The V4 hypervariable region of the bacterial 16S rRNA gene was amplified by PCR, where the forward primer was 515a: 5′–GTGCCAGCMGCCGCGGTAA-3′ and the reverse primer was 806: 5′-GGACTACHVGGGTWTCTAAT-3′. For each sample, a 10-digit barcode sequence was added to the 5′ end of the forward and reverse primers (provided by Allwegene Technology Inc., Beijing, China), and each sample was carried out by three same replications to mitigate reaction-level PCR biases. The volume of PCR reaction was 25 μL, containing 12.5 μL of 2xTaq PCR MasterMix, 1 μL of forward and 1 μL of reverse primers at the concentration of 5 μM, respectively, 3 μL BSA at the concentration of 2 ng/μL, 3 μL DNA samples (30 ng), and 4.5 μL double-distilled H2O (ddH2O). Cycling parameters were 95°C for 5 min, followed by 25 cycles at 95°C for 45 s, 50°C for 50 s, and 72°C for 45 s, and a final extension at 72°C for 10 min. Detection parameters of agarose gel electrophoresis were referred to as follows: concentration of gel with 1%, voltage with 170V, and electrophoretic time of 30 min. Three PCR products from the same sample were mixed in equidensity ratios and purified with a GeneJET Gel Extraction Kit (Thermo Fisher Scientific Inc., Rockford, IL, United States), quantified using real-time PCR, and sequenced at Allwegene Technology Inc. (Beijing, China).
Statistical Analysis
Results are expressed as means ± SD. Significant differences between groups were determined using one-way ANOVA tests, followed by Fisher’s least significant difference (LSD) and Duncan’s Multiple Range test (SPSS21, IBM Corp., Armonk, NY, United States). A probability of P < 0.05 was considered significant.
Results
The Effect of Antibiotic Cocktail on a Cluster of Fecal Microbiota
As shown in Figure 1B, treatment with the antibiotic cocktail for 1 or 3 weeks induced obvious changes in microbiota clusters, and the evolutionary tree between 1 and 3 weeks suggested a similar sample composition.
The Effect of Antibiotic Cocktail on α Diversity of Microbiota
The abundance of gut microbiota is associated with the stability of the micro-ecological environment. As shown in Figure 2, the antibiotic cocktail led to a significant decrease in α diversity of the microbiota, including Chao 1 (A), observed species (B), phylogenetic diversity (PD) whole tree (C), and Shannon index (D). Especially, observed species were decreased by 71.94% (556 to 156) and 76.08% (556 to 133) by administration with the antibiotic cocktail for 1 or 3 weeks (P < 0.05), respectively.
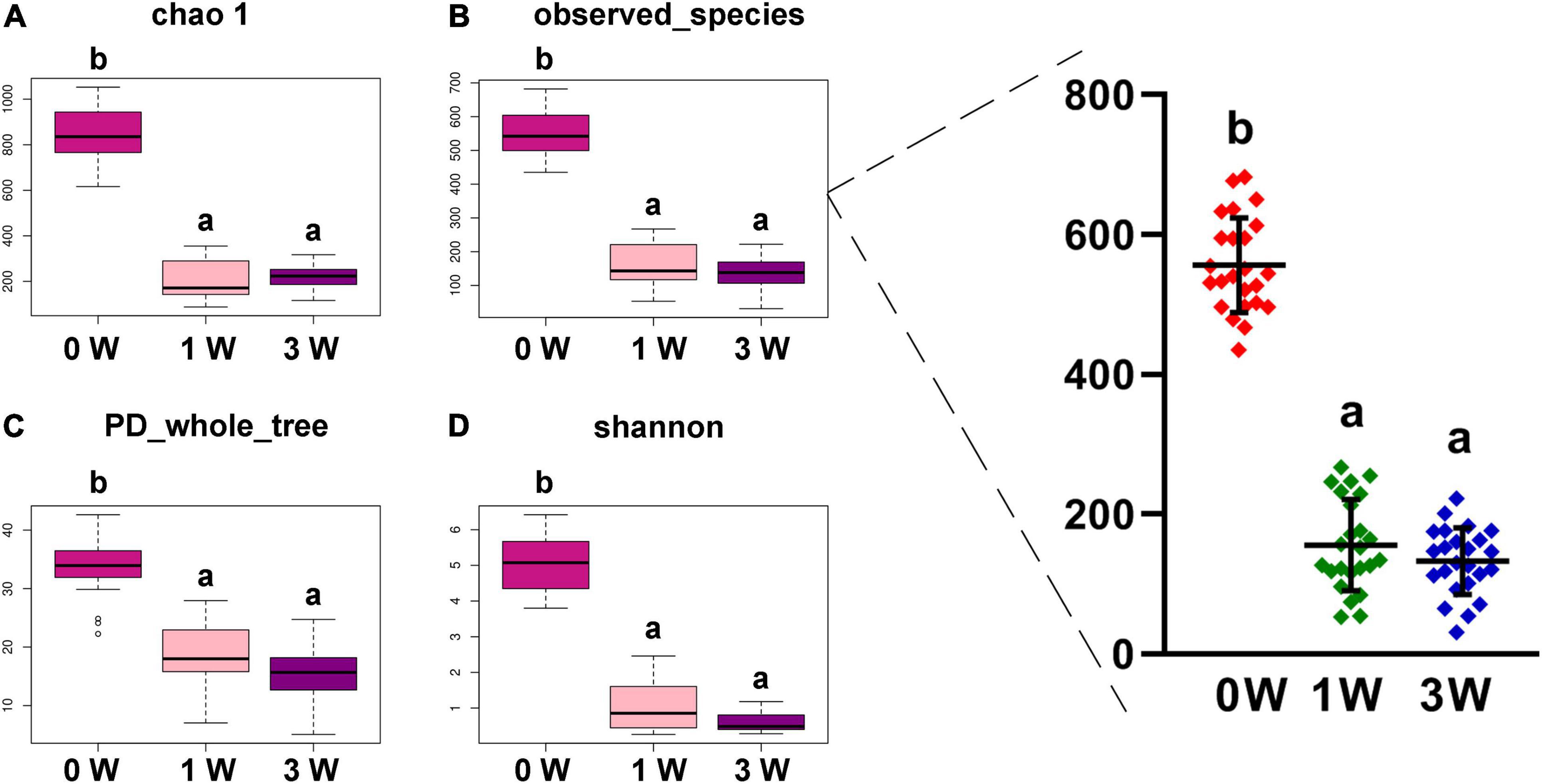
Figure 2. The α diversity of fecal microbiota. (A) Chao 1. (B) Observed species. (C) PD whole tree. (D) Shannon. Data represented as mean ± SD (n = 24). Bars with different letters differ significantly (P < 0.05). Chao1, observed species, and PD whole tree are species richness indices, and the Shannon index reflects the diversity of gut microbiota. PD, phylogenetic diversity.
The Effect of Antibiotic Cocktail on Microbiota Composition at the Phylum Level
To make an insight into the alteration of gut microbiota after treatment with an antibiotic cocktail, the relative abundance of microbes at the phylum level in different periods was analyzed. The relative abundance of 3 kinds of phyla including Bacteroidetes, Actinobacteria, and Verrucomicrobia was decreased significantly by the antibiotic cocktail (P < 0.05). In detail, Bacteroidetes was decreased by 98.75 and 99.94%, Actinobacteria was decreased by 38.05 and 92.09%, while Verrucomicrobia was decreased by 99.71 and 100%, respectively, after treatment with the cocktail for 1 or 3 weeks (Figure 3A). Firmicutes dominated the gut microbiota, and the ratio of Firmicutes to Bacteroidetes was increased by the antibiotic cocktail in a dose-dependent manner (Figure 3B).
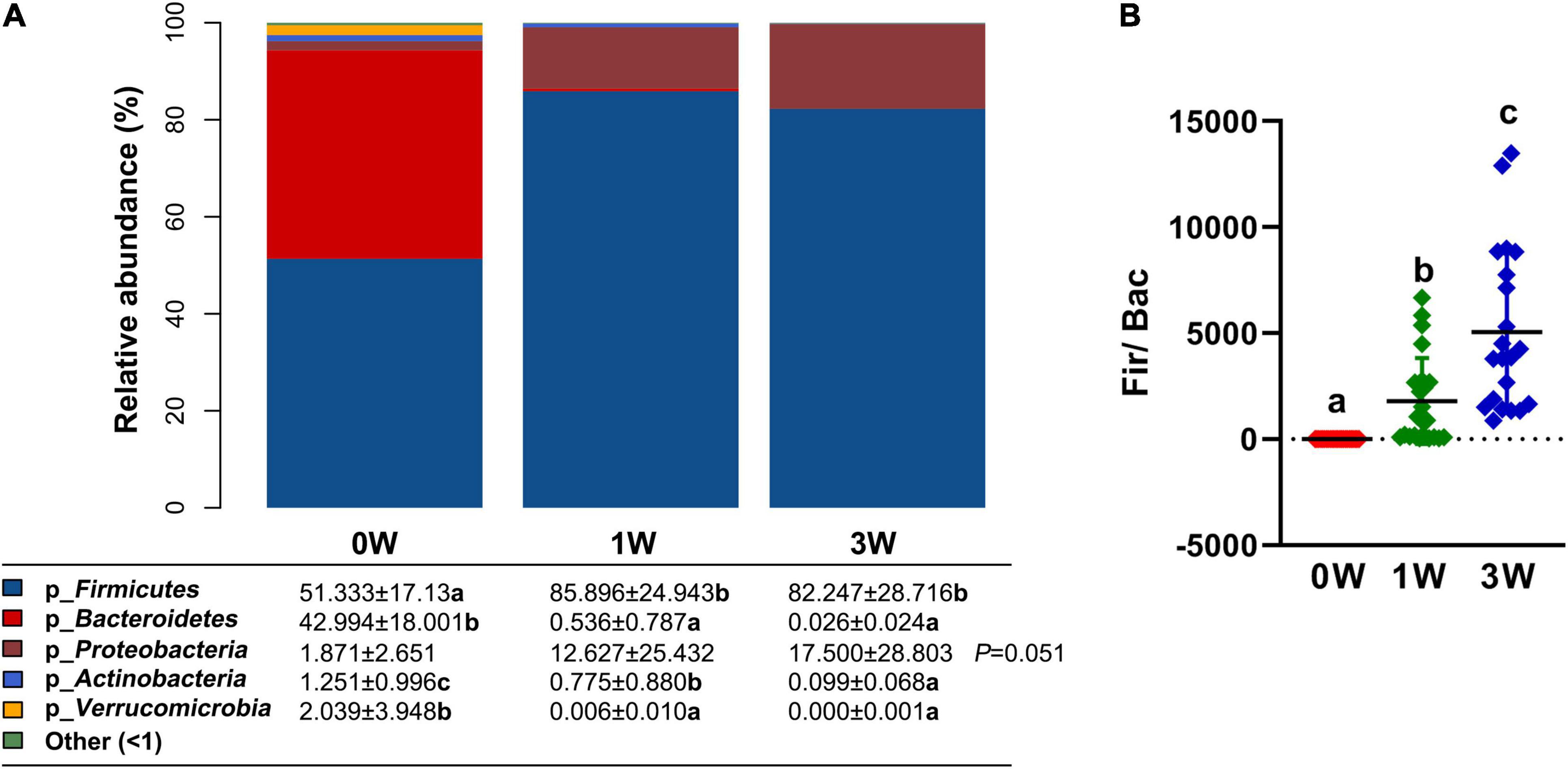
Figure 3. The relative abundance of fecal microbiota at the phylum level. (A) The relative abundance of microbes. (B) The ratio of Firmicutes to Bacteroidetes. Data represented as mean ± SD (n = 24). Bars with different letters differ significantly (P < 0.05). p_ represents microbial phylum.
The Effect of Antibiotic Cocktail on Microbiota Composition at the Genus Level
Further analysis of the relative abundance of microbes at the genus level revealed that Lactococcus, a genus belonging to the phylum of Firmicutes, was increased significantly after being treated with the antibiotic cocktail for 1 or 3 weeks (P < 0.05), and became the dominant genus (80.63%) in the microbial community (Figure 4). On the other hand, Lactobacillus, Faecalibaculum, and Lachnospiraceae_ NK4A136_group belonging to the phylum of Firmicutes, Helicobacter belonging to the phylum of Proteobacteria, Alloprevotella, and Bacteroides belonging to the phylum of Bacteroidetes, and Akkermansia belonging to the phylum of Verrucomicrobia were decreased significantly by the antibiotic cocktail (P < 0.05).
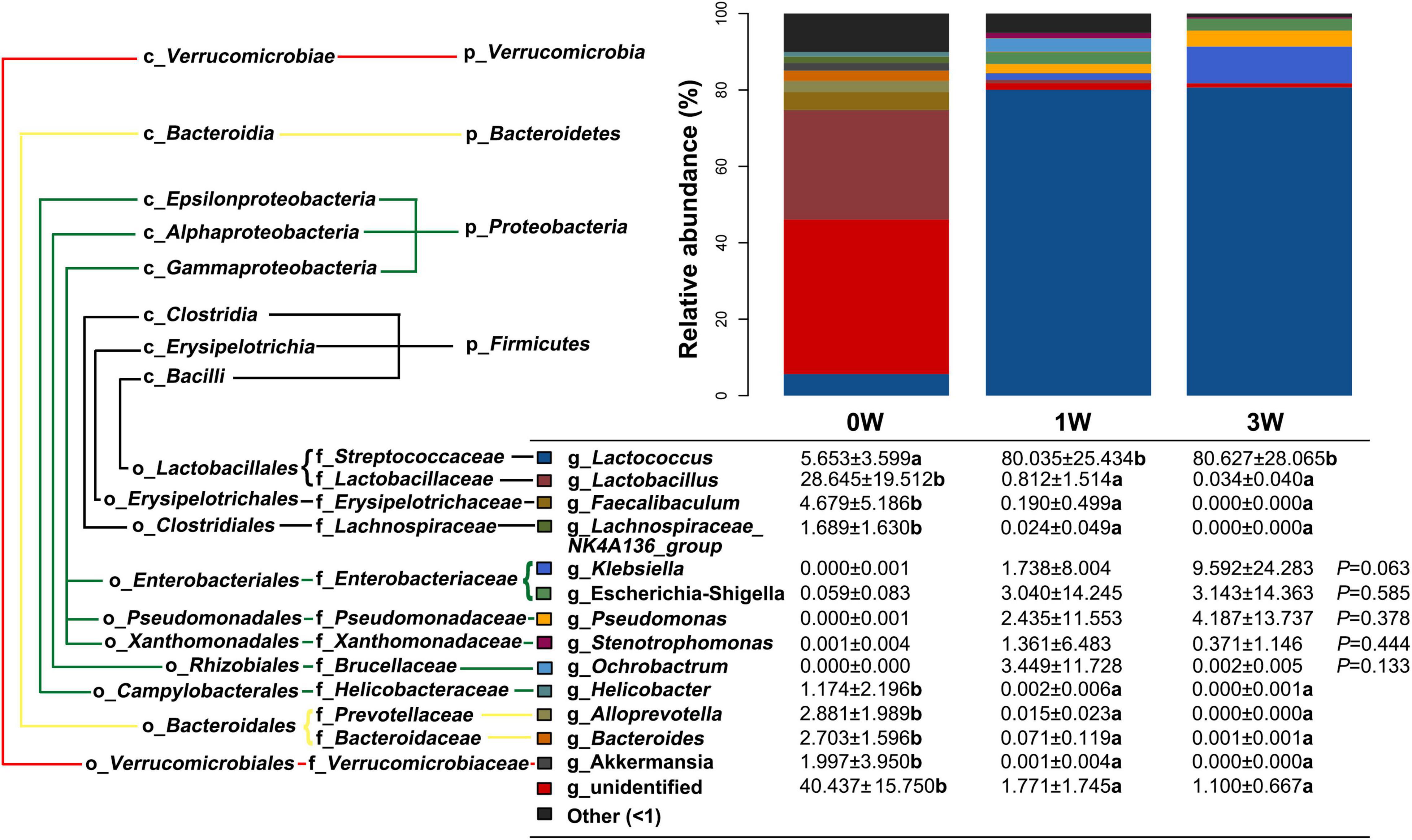
Figure 4. The relative abundance of fecal microbiota at the genus level. Data represented as mean ± SD (n = 24). Bars with different letters differ significantly (P < 0.05). g_ represents microbial genus.
Efficiency of Fecal Microbiota Transplantation on Antibiotics-Pretreated Mice
To verify whether the pretreatment of the antibiotic cocktail is effective, a further FMT experiment was conducted. As shown in Figure 5, the observed species in receptor mice (rM) were elevated obviously as compared with antibiotics-pretreated mice at the 3rd week (3 W). Meanwhile, the number of observed species in rM was similar to that in donor mice (dM) (Figure 5A). Further analysis of the composition of relative gut microbiota at phylum or genus level revealed that FMT reversed relative gut microbiota composition, with more abundant taxon as well as similar relative gut microbiota composition to dM (Figures 5B,C).
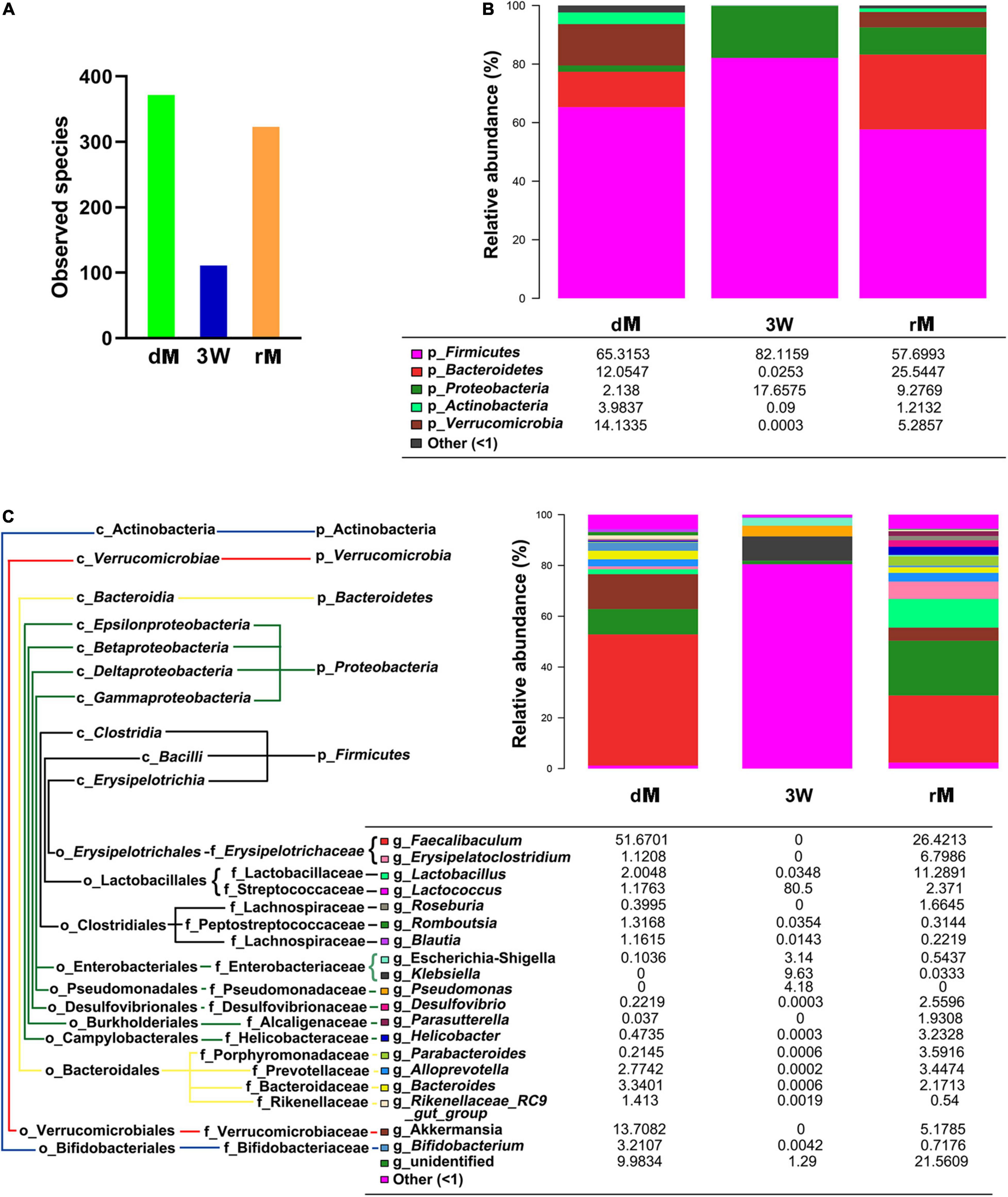
Figure 5. The diversity and relative abundance of fecal microbiota after FMT. (A) Observed species of fecal microbiota among donor mice (dM), mice treated with the antibiotic cocktail for 3 weeks (3W), and receptor mice (rM). (B) Effect of FMT on the relative abundance of fecal microbiota at the phylum level. (C) Effect of FMT on the relative abundance of fecal microbiota at the genus level. dM: donor mice fed with normal diet; FMT, fecal microbiota transplantation; rM, receptor mice with FMT from dM; p_ represents microbial phylum, g_ represents microbial genus.
Discussion
Driven by its amenable property, the antibiotic cocktail serves as the conventionally applicable method to deplete indigenous microbiota to make receptors obtain a susceptible status. In the present study, an antibiotic cocktail containing vancomycin (0.5 g/L), ampicillin (1 g/L), neomycin (1 g/L), and metronidazole (1 g/L) was used in the mouse model for FMT. Vancomycin and ampicillin mainly suppress gram-positive bacteria by inhibiting the synthesis of cell walls (18). Neomycin can inhibit the synthesis of protein in aerobes (19), and metronidazole can inhibit the synthesis of DNA in anaerobes (20). In this study, the observed species of indigenous microbiota were depleted by 71.94% (from 556 to 156) and 76.08% (from 556 to 133), mainly including Bacteroidetes, Actinobacteria, and Verrucomicrobia, after being treated with the antibiotic cocktail for 1 and 3 weeks, respectively, but recovered by the subsequent FMT with fecal microbiota from normal mice. Moreover, treatment with the cocktail increased significantly the ratio of Firmicutes to Bacteroidetes, which was similar to the results of an antibiotic cocktail containing ampicillin (1 g/L) and neomycin (0.5 g/L) for 2 weeks (21). Unexpectedly, Lactococcus, a genus belonging to the phylum of Firmicutes, dominated the gut microbiota of mice at the genus level after treating with the antibiotic cocktail either for 1 or 3 weeks. The relative abundance of Lactobacillus and Akkermansia were also decreased significantly by administrating with the antibiotic cocktail for 1 or 3 weeks, which was in accordance with previous studies (14, 22). However, the relative abundance of Proteobacteria had large individual differences in this study, while another study reported that the alpha subclass of Proteobacteria can be decreased significantly by the antibiotic cocktail (23).
Antibiotics directly target antibiotic-sensitive bacterial species and then affect other microbes owing to the dysbiosis (24). Recent studies have suggested that Lactococcus (such as Lactococcus lactis subsp. lactis) may act as the predator bacterial to exert their antibacterial property via the generation of bacteriocins (25), and an antibiotic cocktail of ciprofloxacin and metronidazole in drinking water ad libitum to mice for 2 weeks can enhance the relative abundance of Lactococcus lactis (26). Potentially, the antibiotic cocktail containing vancomycin, ampicillin, neomycin, and metronidazole may cause a relative increase in predators bacterial such as Lactococcus (25) and pro-inflammatory bacteria such as Escherichia-Shigella and Klebsiella (27), but decrease the relative abundance of anti-inflammatory bacteria such as Akkermansia (28) and autoimmunity-driven bacteria such as Lactobacillus (29), which further affect the capability of microbiota in controlling intestinal inflammation and immunity (30), with a susceptible micro-environment (31) ready for FMT.
Collectively, the antibiotic cocktail effectively depleted indigenous microbiota, and is available for the pretreatment of FMT. Nevertheless, considering the dominant genus Lactococcus after treatment with the antibiotic cocktail, extra data in different situations are needed to ensure disease-specific attributes of donors.
Conclusion
Treatment with an antibiotic cocktail containing vancomycin (0.5 g/L), ampicillin (1 g/L), neomycin (1 g/L), and metronidazole (1 g/L) in drinking water for 3 weeks eliminated microbes belonging to Bacteroidetes, Actinobacteria, and Verrucomicrobia in the gut microbiota of mice, and those microbes can be recovered by FMT.
Data Availability Statement
The datasets presented in this study can be found in online repositories. The names of the repository/repositories and accession number(s) can be found in the article/Supplementary Material.
Ethics Statement
The animal study was reviewed and approved by the Hunan Agricultural University Institutional Animal Care and Use Committee.
Author Contributions
JT, JG, FL, BL, ZL, and JY: experimental execution. JT and SW: writing – original draft preparation. SW and JH: writing – review and editing and supervision. All authors contributed to the article and approved the submitted version.
Funding
We gratefully acknowledge the support from the National Natural Science Foundation of China (32102578), Key R&D Program of Hunan Province (2021NK2010), and Fellowship of China Postdoctoral Science Foundation (2021T140715 and 2021M703545), and Huxiang High-Level Talent Gathering Project of HUNAN Province (2019RS1053).
Conflict of Interest
The authors declare that the research was conducted in the absence of any commercial or financial relationships that could be construed as a potential conflict of interest.
Publisher’s Note
All claims expressed in this article are solely those of the authors and do not necessarily represent those of their affiliated organizations, or those of the publisher, the editors and the reviewers. Any product that may be evaluated in this article, or claim that may be made by its manufacturer, is not guaranteed or endorsed by the publisher.
Supplementary Material
The Supplementary Material for this article can be found online at: https://www.frontiersin.org/articles/10.3389/fnut.2022.918098/full#supplementary-material
Supplementary Table 1 | Diet composition of mouse feed.
References
1. Zhang XY, Chen J, Yi K, Peng L, Xie J, Gou X, et al. Phlorizin ameliorates obesity-associated endotoxemia and insulin resistance in high-fat diet-fed mice by targeting the gut microbiota and intestinal barrier integrity. Gut Microbes. (2020) 12:1–18. doi: 10.1080/19490976.2020.1842990
2. Zhang L, Ma X, Liu P, Ge W, Hu L, Zuo Z, et al. Treatment and mechanism of fecal microbiota transplantation in mice with experimentally induced ulcerative colitis. Exp Biol Med. (2021) 246:1563–75. doi: 10.1177/15353702211006044
3. Littmann ER, Lee JJ, Denny JE, Alam Z, Maslanka JR, Zarin I, et al. Host immunity modulates the efficacy of microbiota transplantation for treatment of Clostridioides difficile infection. Nat Commun. (2021) 12:755. doi: 10.1038/s41467-020-20793-x
4. Jing Y, Yu Y, Bai F, Wang L, Yang D, Zhang C, et al. Effect of fecal microbiota transplantation on neurological restoration in a spinal cord injury mouse model: involvement of brain-gut axis. Microbiome. (2021) 9:59. doi: 10.1186/s40168-021-01007-y
5. Liu L, Kirst ME, Zhao L, Li E, Wang GP. Microbiome resilience despite a profound loss of minority microbiota following clindamycin challenge in humanized gnotobiotic mice. Microbiol Spectr. (2022) 10:e0196021. doi: 10.1128/spectrum.01960-21
6. Staley C, Kaiser T, Beura LK, Hamilton MJ, Weingarden AR, Bobr A, et al. Stable engraftment of human microbiota into mice with a single oral gavage following antibiotic conditioning. Microbiome. (2017) 5:87. doi: 10.1186/s40168-017-0306-2
7. Xu J, Xu H, Peng Y, Zhao C, Zhao H, Huang W, et al. The effect of different combinations of antibiotic cocktails on mice and selection of animal models for further microbiota research. Appl Microbiol Biotechnol. (2021) 105:1669–81. doi: 10.1007/s00253-021-11131-2
8. Huang CE, Feng SY, Huo FJ, Liu HL. Effects of four antibiotics on the diversity of the intestinal microbiota. Microbiol Spectr. (2022) 10:e0190421. doi: 10.1128/spectrum.01904-21
9. Liu J, Miyake H, Zhu H, Li B, Alganabi M, Lee C, et al. Fecal microbiota transplantation by enema reduces intestinal injury in experimental necrotizing enterocolitis. J Pediatr Surg. (2020) 55:1094–8. doi: 10.1016/j.jpedsurg.2020.02.035
10. Luna M, Guss JD, Vasquez-Bolanos LS, Castaneda M, Rojas MV, Strong JM, et al. Components of the gut microbiome that influence bone tissue-level strength. J Bone Miner Res. (2021) 36:1823–34. doi: 10.1002/jbmr.4341
11. Su H, Liu J, Wu G, Long Z, Fan J, Xu Z, et al. Homeostasis of gut microbiota protects against polychlorinated biphenyl 126-induced metabolic dysfunction in liver of mice. Sci Total Environ. (2020) 720:137597. doi: 10.1016/j.scitotenv.2020.137597
12. Gong S, Yan Z, Liu Z, Niu M, Fang H, Li N, et al. Intestinal microbiota mediates the susceptibility to polymicrobial sepsis-induced liver injury by granisetron generation in mice. Hepatology. (2019) 69:1751–67. doi: 10.1002/hep.30361
13. Duan H, Yu L, Tian F, Zhai Q, Fan L, Chen W. Antibiotic-induced gut dysbiosis and barrier disruption and the potential protective strategies. Crit Rev Food Sci Nutr. (2020) 62:1427–52. doi: 10.1080/10408398.2020.1843396
14. Shi Y, Kellingray L, Zhai Q, Gall GL, Narbad A, Zhao J, et al. Structural and functional alterations in the microbial community and immunological consequences in a mouse model of antibiotic-induced dysbiosis. Front Microbiol. (2018) 9:1948. doi: 10.3389/fmicb.2018.01948
15. Isaac S, Scher JU, Djukovic A, Jimenez N, Littman DR, Abramson SB, et al. Short- and long-term effects of oral vancomycin on the human intestinal microbiota. J Antimicrob Chemother. (2017) 72:128–36. doi: 10.1093/jac/dkw383
16. Saxena A, Moran RRM, Bullard MR, Bondy EO, Smith MF, Morris L, et al. Sex differences in the fecal microbiome and hippocampal glial morphology following diet and antibiotic treatment. PLoS One. (2022) 17:e0265850. doi: 10.1371/journal.pone.0265850
17. Wu Q, Xu ZY, Song SY, Zhang H, Zhang WY, Liu LP, et al. Gut microbiota modulates stress-induced hypertension through the HPA axis. Brain Res Bull. (2020) 162:49–58. doi: 10.1016/j.brainresbull.2020.05.014
18. Antonoplis A, Zang X, Wegner T, Wender PA, Cegelski LA. Vancomycin-arginine conjugate inhibits growth of carbapenem-resistant e. coli and targets cell-wall synthesis. ACS Chem Biol. (2019) 14:2065–70. doi: 10.1021/acschembio.9b00565
19. Zuo PX, Yu PF, Alvarez Pedro JJ. Aminoglycosides antagonize bacteriophage proliferation, attenuating phage suppression of bacterial growth, biofilm formation, and antibiotic resistance. Appl Environ Microbiol. (2021) 87:e0046821. doi: 10.1128/AEM.00468-21
20. Meng L, Yin J, Yuan Y, Xu N. Near-infrared fluorescence probe: BSA-protected gold nanoclusters for the detection of metronidazole and related nitroimidazole derivatives. Anal Methods. (2017) 9:768–73. doi: 10.1039/C6AY03280J
21. Schepper JD, Collins FL, Rios-Arce ND, Raehtz S, Schaefer L, Gardinier JD, et al. Probiotic Lactobacillus reuteri prevents postantibiotic bone loss by reducing intestinal dysbiosis and preventing barrier disruption. J Bone Miner Res. (2019) 34:681–98. doi: 10.1002/jbmr.3635
22. Yin J, Wang PM, Liao SSX, Peng X, He Y, Chen YR, et al. Different dynamic patterns of b-lactams, quinolones, glycopeptides and macrolides on mouse gut microbial diversity. PLoS One. (2015) 10:e0126712. doi: 10.1371/journal.pone.0126712
23. Morgun A, Dzutsev A, Dong X, Greer RL, Sexton DJ, Ravel J, et al. Uncovering effects of antibiotics on the host and microbiota using transkingdom gene networks. Gut. (2015) 64:1732–43. doi: 10.1136/gutjnl-2014-308820
24. Croswell A, Amir E, Teggatz P, Barman M, Salzman NH. Prolonged impact of antibiotics on intestinal microbial ecology and susceptibility to enteric Salmonella infection. Infect Immun. (2009) 77:2741–53. doi: 10.1128/iai.00006-09
25. Juturu V, Wu JC. Microbial production of bacteriocins: latest research development and applications. Biotechnol Adv. (2018) 36:2187–200. doi: 10.1016/j.biotechadv.2018.10.007
26. Suez J, Zmora N, Zilberman-Schapira G, Mor U, Dori-Bachash M, Bashiardes S, et al. Post-antibiotic gut mucosal microbiome reconstitution is impaired by probiotics and improved by autologous FMT. Cell. (2018) 174:1406.e–23.e. doi: 10.1016/j.cell.2018.08.047
27. Trevejo-Nuñez G, Lin B, Fan L, Aggor FEY, Biswas PS, Chen K, et al. Regnase-1 deficiency restrains Klebsiella pneumoniae infection by regulation of a type i interferon response. mBio. (2022) 13:e0379221. doi: 10.1128/mbio.03792-21
28. Grajeda-Iglesias C, Durand S, Daillère R, Iribarren K, Lemaitre F, Derosa L, et al. Oral administration of Akkermansia muciniphila elevates systemic antiaging and anticancer metabolites. Aging. (2021) 13:6375–405. doi: 10.18632/aging.202739
29. Zegarra-Ruiz DF, ElBeidaq A, Iniguez AJ, Lubrano Di Ricco M, Manfredo Vieira S, Ruff WE, et al. A diet-sensitive commensal Lactobacillus strain mediates TLR7-dependent systemic autoimmunity. Cell Host Microbe. (2019) 25:113.e–27.e. doi: 10.1016/j.chom.2018.11.009
30. Strati F, Pujolassos M, Burrello C, Giuffre MR, Lattanzi G, Caprioli F, et al. Antibiotic-associated dysbiosis affects the ability of the gut microbiota to control intestinal inflammation upon fecal microbiota transplantation in experimental colitis models. Microbiome. (2021) 9:39. doi: 10.1186/s40168-020-00991-x
Keywords: antibiotic cocktail, pretreatment, gut microbiota, mouse model, fecal microbiota transplantation
Citation: Tan J, Gong J, Liu F, Li B, Li Z, You J, He J and Wu S (2022) Evaluation of an Antibiotic Cocktail for Fecal Microbiota Transplantation in Mouse. Front. Nutr. 9:918098. doi: 10.3389/fnut.2022.918098
Received: 12 April 2022; Accepted: 04 May 2022;
Published: 03 June 2022.
Edited by:
Hui Han, Chinese Academy of Sciences (CAS), ChinaReviewed by:
Zhouzheng Ren, Northwest A&F University, ChinaNa Dong, Northeast Agricultural University, China
Baichuan Deng, South China Agricultural University, China
Copyright © 2022 Tan, Gong, Liu, Li, Li, You, He and Wu. This is an open-access article distributed under the terms of the Creative Commons Attribution License (CC BY). The use, distribution or reproduction in other forums is permitted, provided the original author(s) and the copyright owner(s) are credited and that the original publication in this journal is cited, in accordance with accepted academic practice. No use, distribution or reproduction is permitted which does not comply with these terms.
*Correspondence: Jianhua He, amlhbmh1YWh5QGh1bmF1Lm5ldA==; Shusong Wu, d3VzaDY4OEBodWFudS5lZHUuY24=