- 1Department of Pharmacy, Faculty of Science and Engineering, International Islamic University Chittagong, Chittagong, Bangladesh
- 2Gwangju Center, Korea Basic Science Institute, Gwangju, South Korea
- 3Drugs and Toxins Research Divison, BCSIR Laboratories Rajshahi, Bangladesh Council of Scientific and Industrial Research, Rajshahi, Bangladesh
- 4Department of Pharmaceutical Chemistry, Faculty of Pharmacy, University of Dhaka, Dhaka, Bangladesh
- 5Department of Botany, Faculty of Biological Science, University of Chittagong, Chattogram, Bangladesh
- 6Beijing Advanced Innovation Center for Food Nutrition and Human Health, China-Canada Joint Lab of Food Nutrition and Health (Beijing), Beijing Engineering and Technology Research Center of Food Additives, Beijing Technology and Business University, Beijing, China
- 7Department of Pharmacology, Medical School, Jeonbuk National University, Jeonju, South Korea
- 8Molecular Pharmacology Research Center, School of Pharmaceutical Sciences, Wenzhou Medical University, Wenzhou, China
Dillenia pentagyna Roxb. is traditionally used to treat cancer, wound healing, diabetes, and diarrhea in local tribes. This study was designed to evaluate the pharmacological potentiality of this plant. In vivo analgesic, anti-inflammatory, and antipyretic studies of the methanol extracts of D. pentagyna (MEDP) leaves were performed by using acetic acid-induced nociception, formalin-induced paw licking, and yeast-induced pyrexia assay methods, respectively. In vivo antidiarrheal activity was carried out in mice by following castor oil-induced diarrhea and gastrointestinal transit manner. In vitro thrombolytic experiment was performed employing the clot lysis activity. Besides, a molecular docking study was performed by executing the software (PyRx, Discovery Studio, and UCSF Chimera). In the acetic acid-induced writhing study, MEDP possesses significant writhing inhibition in a dose-dependent manner. It showed 50.86% of maximum inhibition of pain in the case of MEDP at a dose of 400 mg/kg body weight. In the anti-inflammatory study, maximum inhibition rate was observed at a value of 59.98 and 41.29% in early and late phases, respectively, at the dose of 400 mg/kg body weight. In the case of yeast-induced hyperpyrexia, MEDP reduced hyperpyrexia in a dose-dependent manner. In the antidiarrheal assay, MEDP moderately inhibited the occurrence of diarrhea in all the experiments. In the thrombolytic study, a moderate (17.76%) clot lysis potency has been yielded by MEDP. Again, the molecular docking simulation revealed strong binding affinities with almost all the targeted proteins. The present study suggests that the MEDP possesses remarkable pharmacological activity and this finding validated the ethnobotanical significance of D. pentagyna as the source of pain, fever, and diarrhea management agent.
Introduction
Pain is referred to as the response to several noxious stimuli in the immune response and is complicatedly structured through various episodes such as vasodilation, plasma extravasation, cell migration, and release of different mediators (1). In addition, bacteria and viruses trigger pyrexia which has been governed by CNS feedback mechanisms including vasodilation or sweat production and is responsible to reduce body temperature (2). Although NSAIDs are vigorously prescribed for pain, inflammation, and fever management, they can cause serious side effects following mucosal disruptions, ulcers, perforation, and blood suppression along with kidney damage, increased blood pressure, and cardiovascular complications (3). Diarrhea is one of the most prevalent diseases associated with altered bowel movement, wet stool, and abdominal pain is caused by multiple factors, namely, infections, food aversion, intestinal disorders, and also symbolic symptoms of multiple diseases like diabetes mellitus, and inflammatory diseases (4). Acute diarrhea in pediatric patients may be caused by bacteria and viruses via self-limiting of fluid and electrolyte replacement. Therapeutic approaches to reducing pain and diarrheal incidence are typically controlled by chemicals or medicines, but the adverse effects of such medicines present a substantial danger (4). Vascular blockage caused by a blood clot (thrombus) developed in the circulatory system due to lack of hemostasis guides to severe cascade sequels in atherothrombotic diseases including myocardial or cerebral infarction followed by death (5). About 1.5 billion (currently about 3.5 billion, i.e., 88%) of the world population are being treated by herbal medicines prepared from medicinal plants which demand new compounds discovery with promising remedial effects and negligible side effects contributing to the treatment of pain, fever, diarrhea, and thrombus (6). In structural molecular biology and computer-assisted drug design, molecular docking is an efficient process. Molecular docking is a structure-based drug design technology that simulates molecular interactions and predicts receptor-ligand binding mechanism and affinity (7). The purpose of ligand-protein docking is to predict the most likely binding modalities of a ligand to a known three-dimensional structure of a protein. Successful docking algorithms may successfully explore high-dimensional regions by using a scoring mechanism that correctly scores dockings. Docking may be used to do virtual screening on vast libraries of compounds, grade the findings, and provide structural insight into how the ligands interact with the target, all of which are immensely advantageous for lead optimization (8). Therefore, molecular docking is becoming more popular as a method for discovering new drug targets because of the growing availability of protein and nucleic acid structures. Docking structure prediction accuracy and screen hit rates have been the subject of a recent study. Docking against homology-modeled targets is now possible for a larger number of proteins as the number of experimentally known structures grows (9). Thus, research should be carried on so that these can lead us to new drug development in the future (10). Dillenia pentagyna Roxb. (family: Dillaneaceae) is an ethnomedicinal plant grown in the different district in Bangladesh. The plant is locally known as Banchalta, Hargaza (Bengali), Ajuli (Dhaka-Mymensingh), Argeza (Chittagong), and Ekush (Sylhet). The tribal and folk people utilize this species for the treatment of numerous diseases, for example, bone fracture (leaf), stomach cancer, pain (root), diarrhea and dysentery (leaf), pain (root), etc. (11, 12). In addition, this plant contains a number of flavonoids and triterpenes, e.g.; kaempferol, quercetin, rhamnetin-3-glucoside, isorhamnetin, and naringenin-7 galactosyl (1-4) glucoside, betulin, malic acid, lupeol, betulinaldehyde, β sitosterol, stigmasterol, and betulinic acid (13–15). Many approaches, namely, agitation, maceration, percolation, and soxhlet extraction for concentrating and extracting bioactive components from D. pentagyna have been demonstrated previously. Finally, based on previous reports and folkloric usages of this plant, it can be hypothesized that D. pentagyna can be a prospective source to develop novel therapeutics in the treatment of pain, fever, inflammation, diarrhea, and thrombosis.
In this study, phytochemical screening has been revealed to predict the elements which could enhance the pharmacological activity of methanol extracts of D. pentagyna (MEDP). Furthermore, we will also report the in vivo antinociceptive, antipyretic, antidiarrheal, and in vitro thrombolytic investigations of this plant extract along with in silico study; hence it was not accelerated previously.
Materials and Methods
Plant Material
In November 2019, plant leaves of the D. pentagyna were obtained from Mirsharai, Chittagong, Bangladesh. The plant was identified by Mr. Sajib Rudra, taxonomist, Department of Botany, University of Chittagong, Chittagong-4331, Bangladesh (Accession number: CTGUH SR 7921).
Drying and Grinding
After the collection and identification, the leaves were washed accurately and dried under natural shade at (23 ± 2)°C for about 14 days. The dried leaves were then ground into powder using a high-capacity grinder. Powdered plant material was stored in a well-closed plastic container for further evaluation.
Extraction of the Plant Material
According to the previously established method, from 400 g dried powder of D. pentagyna, 18 g crude methanol extract was obtained (16).
Drugs and Chemicals
All drugs and chemicals used in this research were of analytical grade. To appliance the analgesic and anti-inflammatory study, acetic acid and formalin were collected from BDH Chemicals Ltd. (Poole, United Kingdom), diclofenac-Na was obtained from Incepta Pharmaceuticals (Dhaka, Bangladesh), and paracetamol and loperamide were from Square Pharmaceuticals Ltd. (Dhaka, Bangladesh). Streptokinase was obtained from Sanofi-aventis Bangladesh Ltd. (Dhaka, Bangladesh). Castor oil was supplied by Well Heath (Madrid, Spain), 10% charcoal in 5% gum acacia from Taj Scientific (Chittagong, Bangladesh), and Tween-80 and ethanol were procured from Sigma-Chemical Co. (St. Louis, MO, United States).
Experimental Animals
To propagate experiments, Swiss albino mice (both male and female sex) aged 7–8 weeks and weighing around 25–30 g were collected from the Venom Research Centre (VRC), Chittagong, Bangladesh. Plastic cases were made to keep all animals under the 20 ± 2°C temperature and serve a 12-h light-dark cycle with the standard provision of water and food. P&D committee (Department of Pharmacy, International Islamic University Chittagong, Bangladesh) sanctioned all study protocols directed in an isolated and silent condition. The animals were acclimatized to laboratory conditions for 10 days before experimentation. The handling and taking care of animals were carried out by universal rules for the utilization and maintenance of experimental animals (17). The principles and guidelines of the Federation of European Laboratory Animal Science Associations (FELASA) were enforced to maintain the mitigation of pain and stress of the experimental models. Throughout the experiments, “3R” (Replace, Reduce, and Refine) was strictly maintained to prevent extreme pain and suffering. Experienced researchers and laboratory assistants handled the total experiment. Finally, after completion of the experiment, an anesthesia overdose [Ketamine HCl (100 mg/kg) and Xylazine (7.5 mg/kg)] through the intraperitoneal route were administered to the laboratory models followed by euthanasia (18).
Phytochemical Screening
The phytochemical investigations of MEDP to confirm the existence of carbohydrates, alkaloids, flavonoids, tannins, terpenoids, glycosides, steroids, saponin, resin, phenol, polyphenol, protein, anthocyanin, and cholesterol were defined by the established method (19).
Assesment of Pharmacological Actions
In vivo Bioassay
Preparation of Extract Solution
1% Tween Solution Preparation. In total, 1 ml Tween was taken in a beaker and 99 ml distilled water was added to the beaker to prepare 1% Tween-80.
Dose Preparation. For preparing 200 and 400 mg/kg dose, 200 and 400 mg extract were taken, respectively, and dissolved in 10 ml 1% Tween solution.
Acute Toxicity Test. An acute oral toxicity study was performed according to the OECD guidelines for the testing of chemicals (20). To conduct the toxicity test, a total of five animal models were considered. They received a single oral dose of either 500, 1,000, 1,500, or 2,000 (mg/kg BW) of MEDP followed by suspension of food administration for 3–4 h and then observation of the animals was done carefully for the next 72 h. Other changes, including in skin and fur, eyes and allergic reaction, respiratory and circulatory rate, and autonomic and CNS function (excitability and sedation) were observed (21).
In vivo Analgesic Activity
Acetic Acid-Induced Writhing Test. The acetic acid-induced writhing test was performed by an established protocol mentioned by Emon et al. (22). Four groups of male mice (20–25 g) each having six mice were used in this experiment. MEDP (100, 200, and 400 mg/kg; p.o.) had been administered to the individual mice. After 30 min, an intraperitoneal injection of 0.7% v/v acetic acid solution was administered. In transpicuous cages, mice were positioned individually and 5 min had been allowed to endure. For 20 min, the number of acid-induced writhes had been counted. Control and standard group animals were treated with normal saline (10 ml/kg, i.p.) and diclofenac-Na (10 mg/kg, i.p.), respectively.
Formalin-Induced Licking Test. According to Hunskaar et al. method (23), four groups of mice, each having five mice (20–25 g) were injected 20 μl of 1% formalin prepared in 0.9% saline, subcutaneously into the dorsal hind paw and shifted instantly in a transparent box for observation. The length of response time (paw licking or biting) was once determined between 0–5 min (first phase) and 15–30 min (second phase). Animals had been administered MEDP (100, 200, and 400 mg/kg; p.o.). Diclofenac-Na (10 mg/kg, i.p.) had been given to the standard animals. Control animals had been given normal saline (0.1 ml/10 g).
In vivo Antipyretic Activity
According to Brewer’s yeast-induced fever method with some modifications (24, 25), at 0 h, the basal rectal temperature of each mouse was once recorded using a medical digital thermometer. Pyrexia was triggered with the aid of a subcutaneous injection of 15% w/v suspension of Brewer’s yeast in distilled water at a dose of 10 ml/kg body weight. After 18 h of Brewer’s yeast injection, the rise in rectal temperature used to be recorded, and only animals displaying an increase in temperature of at least 0.6°F (or 1°C) had been chosen for the study (26). The animals had been randomly divided into five groups, each group containing six mice. Group I received 1% Tween-80 in normal saline orally. Group II was given the standard drug paracetamol at the dose of 10 mg/kg orally. Groups III, IV, and V received methanol extract at an oral dose of 100, 200, and 400 (mg/kg; p.o). After the treatment, the temperature of all the mice in each group was recorded at 0, 1, 2, 3, and 4 h.
In vivo Antidiarrheal Activity
Castor Oil-Induced Diarrhea. The related method (27) was followed for this study with slight modification. Mice (20–25 g) fasted for 18 h. The selected mice for diarrheal tests were divided into four groups consisting of 6 mice. Group I was given normal saline (10 ml/kg) orally and designated as the control group. Group II obtained loperamide (5 mg/kg) as a standard group. Groups III–V obtained methanolic extract of MEDP (100, 200, and 400 mg/kg; p.o.), respectively. After 1 h, all groups received castor oil 1 ml each orally. Then they were positioned in cages lined with adsorbent papers and observed for 4 h for the presence of characteristic diarrheal droppings. In total, 100% was regarded as the total number of feces in the control group. The activity was expressed as % inhibition of diarrhea.
Here, Mo = mean number of feces of the control group and M = mean number of feces of the test group.
Castor Oil-Induced Gastrointestinal Motility. This experiment was done with the strategy of a previously established method (28). All mice were divided into five groups of six mice. In total, 0.5 ml of castor oil was given orally to each mouse to produce diarrhea. After 1 h, the group I was given normal saline (10 ml/kg) orally as the control group. Group II received loperamide (5 mg/kg) as a standard group. Groups III, IV, and V received a MEDP (200 and 400 mg/kg; i.p.), respectively. After 1 h, 1 ml charcoal meal (10% charcoal suspension in 5% gum acacia) was given to all mice. The animals were then sacrificed after an hour and the small intestine was dissected from pylorus to cecum. The distance traveled by the charcoal meal from the pylorus was measured and expressed as a percentage of the total length of the small intestine from the pylorus to the cecum.
In vitro Bioassay
In vitro Thrombolytic Activity
This study was conducted by following the method mentioned by Ahmed et al. (29). In total, 5 ml of venous blood had been drawn from wholesome volunteers. A total of 0.5 ml/tube of blood was dispensed in a preweighed sterile Eppendorf tube. Incubation of them at 37°C for 45 min allowed clot formation. The developed serum was eliminated except for disturbing the clot. Each tube was once again weighed to measure the clot weight. A total of 100 μl extract solutions were added separately to the tubes. As a positive control, 100 μl of streptokinase was added separately. A total of 100 μl of water was added separately to the clot of blank tubes. All the tubes were incubated at 37°C for 90 min. After 90-min incubation, the developed fluid from the clot was discarded very carefully and tubes were weighed again.
In silico Studies
Selection of Ligands
The compounds of D. pentagyna such as Kaempferol (PubChem CID: 5280863), Quercetin, (PubChem CID: 5280343), Isorhamnetin (PubChem CID: 5281654), Lupeol (PubChem CID: 259846), and Betulin (PubChem CID: 72326) were loaded in 2DSDF format, and the ligands were then minimized and turned to pdbqt format using PyRx tools to calculate the binding affinity in these targets.
Preparation of Targeted Proteins
The three-dimensional structures of the mu-opioid receptor-Gi protein complex (PDB: 6DDF) (30), the structure of celecoxib bound at the COX-2 active site (PDB: 3LN1) (31), human microsomal prostaglandin E synthase 1 (PDB: 3DWW) (32), kappa-opioid receptor (PDB: 6VI4) (26, 33), and tissue-type plasminogen activator (PDB: 1TPM) (34) were downloaded from the RCSB Protein Data Bank in pdb format. Gasteiger charges and hydrogen atoms were employed in a unique way to synthesize proteins. This procedure also avoided the use of any extraneous solvents. Changes in proteins were yielded in different parameters, for example, selenomethionine (MSE) was replaced by methionine (MET), Bromo UMP was replaced by UMP (U), and methylselenyl-dUMP (UMS) was replaced by UMP (U), methylselenyl-dCMP (CSL). The Dunbrack 2010 rotomer library was used to replace several of the side chains that were yet unfinished. In Chimera, the residues were retained in AMBER ff14sB mode and Gasteiger mode. As a result, all proteins have been shrunk to the least level of energy (35).
Docking Analysis
PyRx Autodock Vina was used to accomplish the binding interaction on the generated protein-ligand complexes (36). Docking experiments were conducted using PyRx’s semiflexible docking device. The phytochemicals were converted to PDBQT formats using PyRx AutoDock tools. The stiffness of proteins and ligands was maintained throughout this investigation. Ligand molecules had provided ten degrees of freedom (37). During the transformation, a grid box with an active site in the center was built. Finally, utilizing the BIOVIA Discovery Studio Visualizer 2020, docking sites for the best connection strategies were examined (24).
Statistical Analysis
Statistical analysis of data is exhibited as mean ± SEM using Graph pad prism version 5.0. Statistical significance was dictated by one-way ANOVA and was accomplished by Dunnett’s multiple comparison test. P-values of less than 0.05, 0.01, and 0.001 were considered statistically significant.
Results
Phytochemical Screening
The qualitative phytochemical screening was performed to ensure the presence or absence of secondary plant metabolites. The phytochemical screening result of MEDP showed in Table 1.
Acetic Acid-Induced Writhing
Methanol extracts of D. pentagyna (MEDP) (100, 200, and 400 mg/kg) produced a dose-dependent analgesic response which moderately reduced acetic acid-induced writhing. Dose 100, 200, and 400 mg/kg produced 19.23 (∗∗p < 0.01), 31.27% (∗∗∗p < 0.001), and 50.86% (∗∗∗p < 0.001) inhibition respectively. Diclofenac-Na formed 64.94% (∗∗∗p < 0.001) of inhibition. The result of acetic acid-induced writhing has been shown in Figure 1.
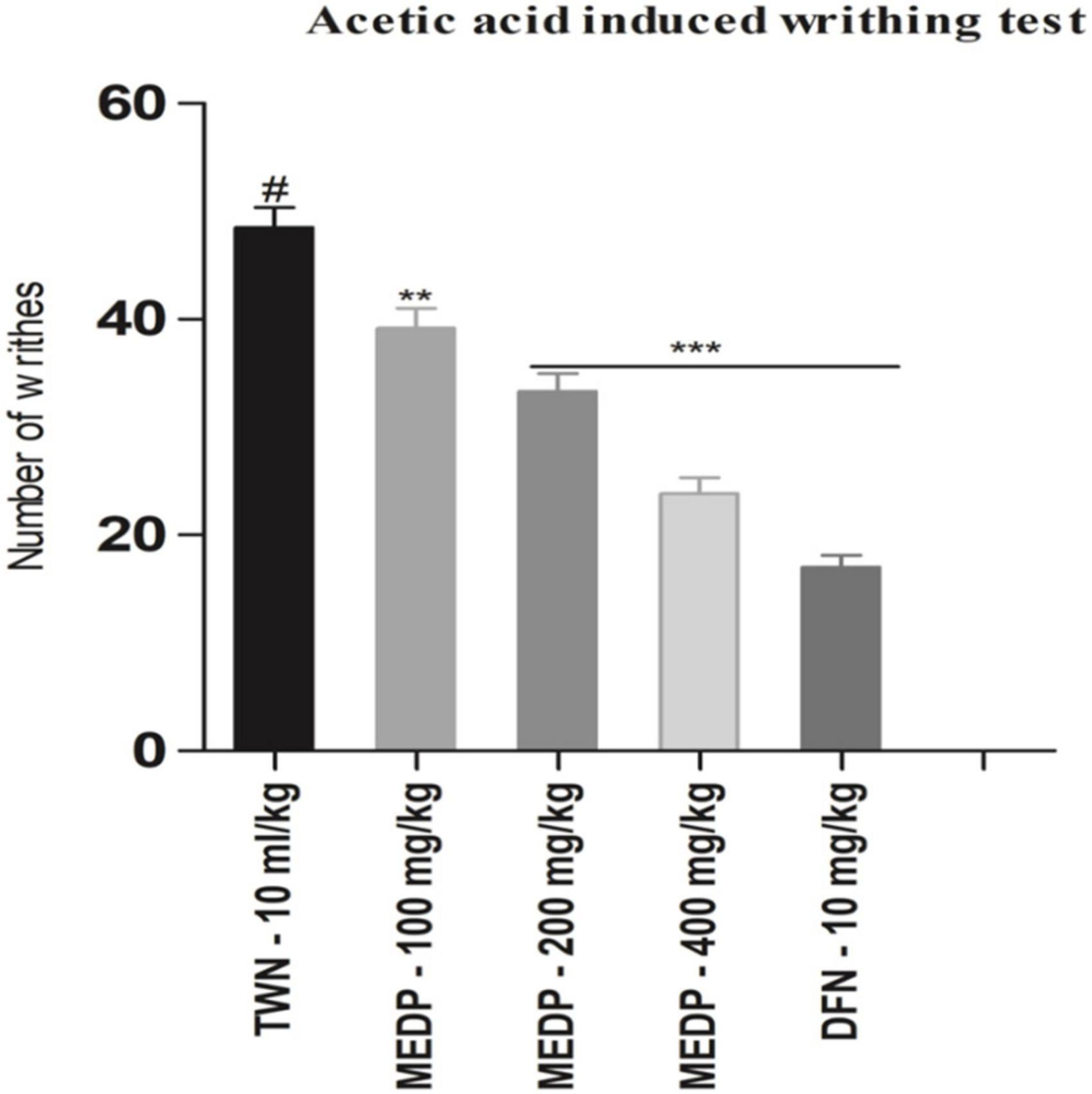
Figure 1. Effects of various test doses of MELM on the acetic acid-induced writhing test in mice (n = 6). Values are presented as mean ± SEM; one-way ANOVA followed by Dunnett’s test. *p < 0.05, **p < 0.01, and ***p < 0.001 were considered as significant compared with the control, where # is designated as control. MEDP = methanol extract of Dillenia pentagyna, TWN = 1% Tween-80 and DFN = diclofenac-Na.
Formalin-Induced Licking
Oral administration of MEDP at different concentrations showed a moderate reduction of paw licking in both early and late phases in formalin-induced pain methods. In the early phase, 18.15%, 47.49% (∗∗p < 0.01), and 59.98% (∗∗∗p < 0.001) had been reported for MEDP 100, 200, and 400 mg/kg, respectively. On the other hand, 14.63%, 29.92% (∗∗p < 0.01), and 41.29% (∗∗∗p < 0.001) licking inhibition was recorded in the late phase for MEDP 100, 200, and 400 mg/kg, respectively. Connecting to the standard drug (diclofenac-Na) showed 76.32% (∗∗∗p < 0.001) and 72.18% (∗∗∗p < 0.001) of inhibition in both the early and late phases. The consequence of the formalin-induced paw licking test has been shown in Figure 2.
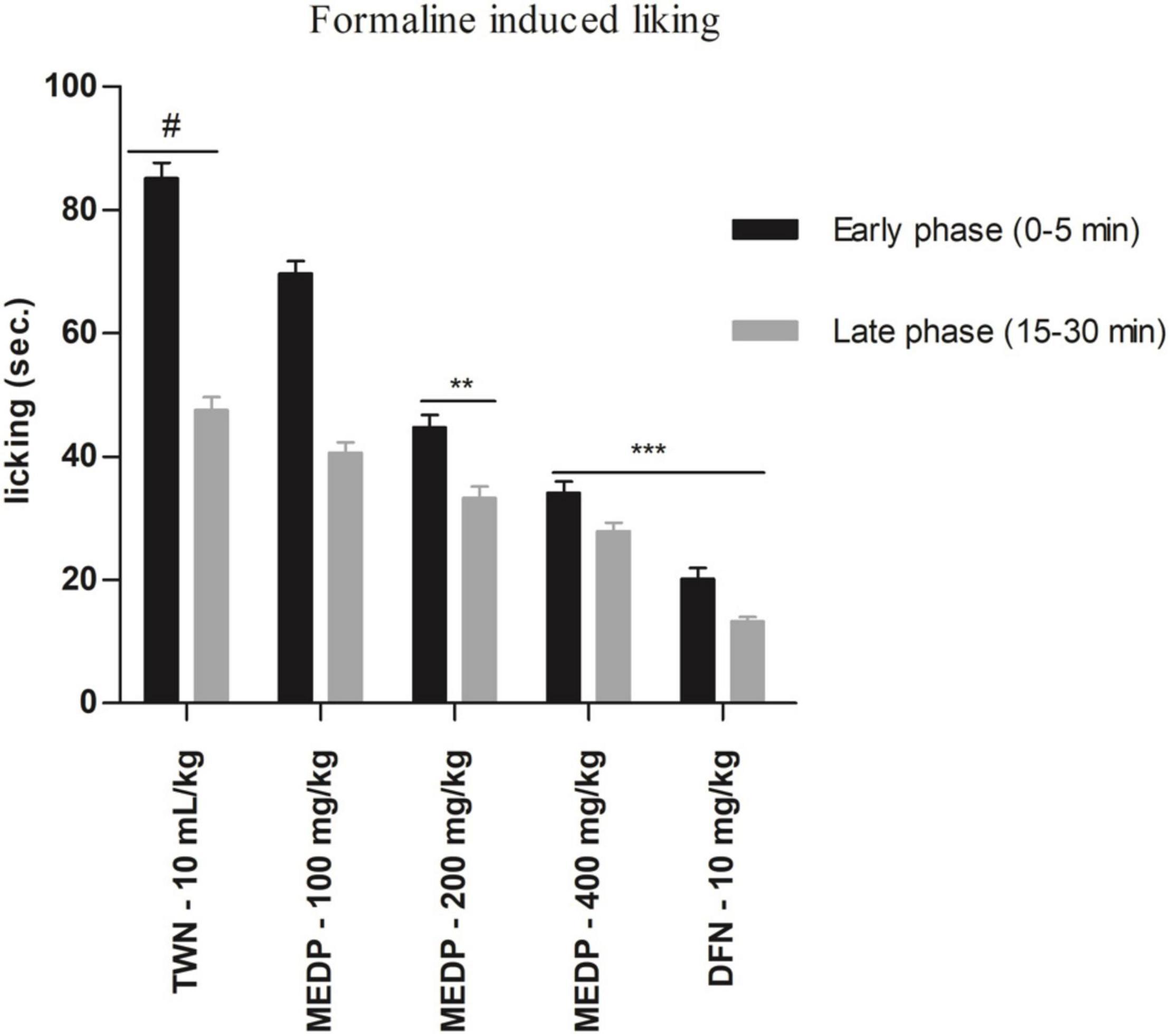
Figure 2. Effects of various test doses of the MELM on the formalin-induced paw licking study in mice (n = 6). Values are presented as mean ± SEM; one-way ANOVA followed by Dunnett’s test. **p < 0.01 and ***p < 0.001 were considered as significant compared with the control, where # is designated as control. MEDP = methanol extract of Dillenia pentagyna TWN = 1% Tween-80 and DFN = diclofenac-Na.
Antipyretic Activity
Methanol extracts of D. pentagyna (MEDP) significantly (∗p < 0.05, ∗∗ p < 0.01, and ∗∗∗p < 0.001) reduces the fever at the dose-dependent manner. The body temperature of mice has been decreased with the administration of MEDP (200 and 400 mg/kg). MEDP 400 mg/kg showed almost similar activity to the standard drug paracetamol. The summary of hyperpyrexia reduction has been shown in Table 2.
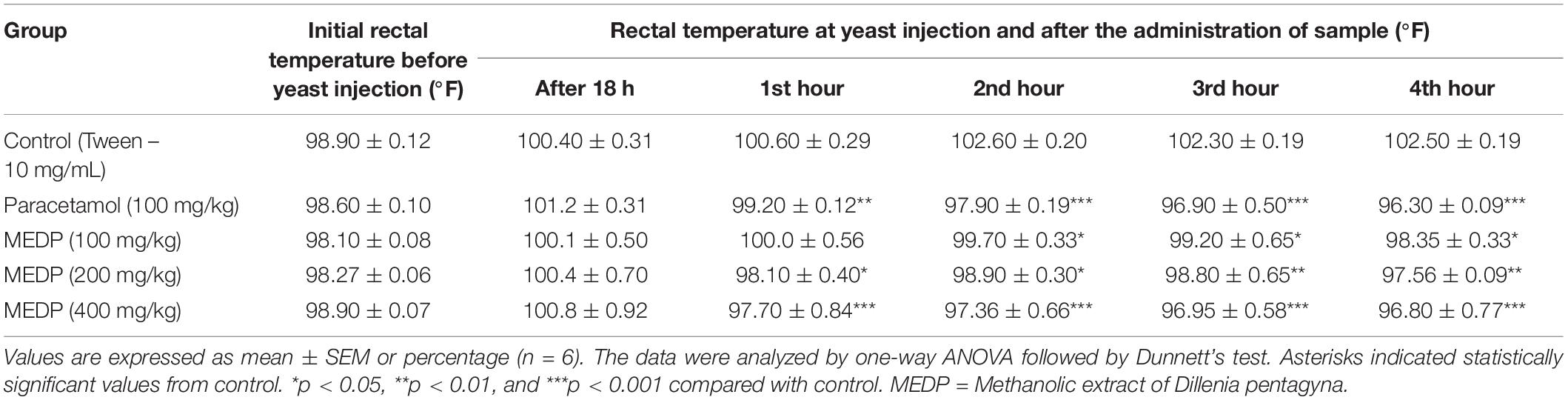
Table 2. Antipyretic activity of the methanol extracts of Dillenia pentagyna in yeast induced pyrexia method.
Castor Oil-Induced Diarrhea
In this experiment, oral administration of MEDP 100, 200, and 400 mg/kg showed significant dose-related inhibition of defecation frequency when compared to loperamide 5 mg/kg. MEDP administered at the dose of 100, 200, and 400 mg/kg showed 29.31%, 51.03% (∗∗p < 0.01), and 60.68% (∗∗∗p < 0.001) reduction, respectively, where, standard drug loperamide (5 mg/kg) yielded 78.13% (∗∗∗p < 0.001) of retardation. Result is shown in Table 3.
Charcoal-Induced Intestinal Transit in Mice
In this study, the results found that, after the administration of MEDP 100 mg/kg, charcoal was traveled in the small intestine up to (31.93 ± 0.83) cm. Besides, charcoal meal has been traveled almost (24.65 ± 0.73) (∗p < 0.05) cm and (18.70 ± 1.10) (∗∗∗p < 0.001) cm for MEDP 200 and 400 mg/kg, respectively. Under similar experimental conditions, only (11.23 ± 1.12) (∗∗∗p < 0.001) cm charcoal meal traveled in the small intestine of mice at a dose of loperamide 5 mg/kg. The peristaltic index and percent of inhibition in castor oil-induced gastrointestinal motility have been shown in Table 4.
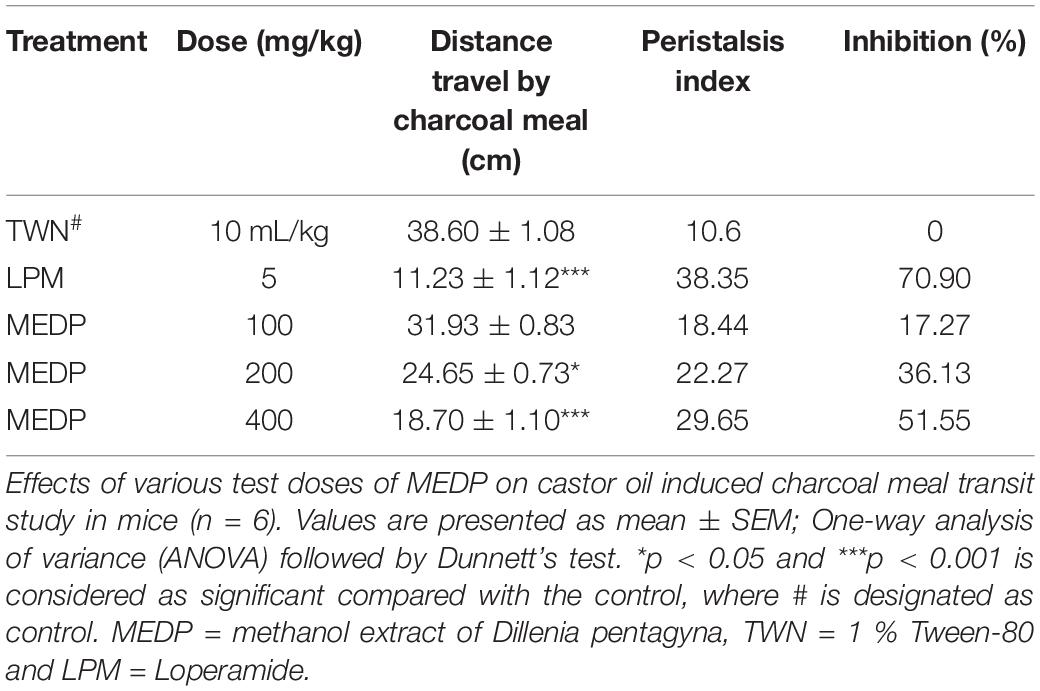
Table 4. Castor oil-induced gastrointestinal motility of the methanol extracts of Dillenia pentagyna.
Anticoagulant Activity
In vitro thrombolytic test, streptokinase (100 μl) showed 71.42 % (∗∗∗p < 0.001) clot lysis. In addition, 4.70% clots lysis was observed when the mice were treated with sterile distilled water where MEDP showed only 17.76% (∗p < 0.05) clot lysis in the clot lysis study model. A statistical representation of clot lysis percentage by negative control, positive control, and MEDP has been shown in Figure 3.
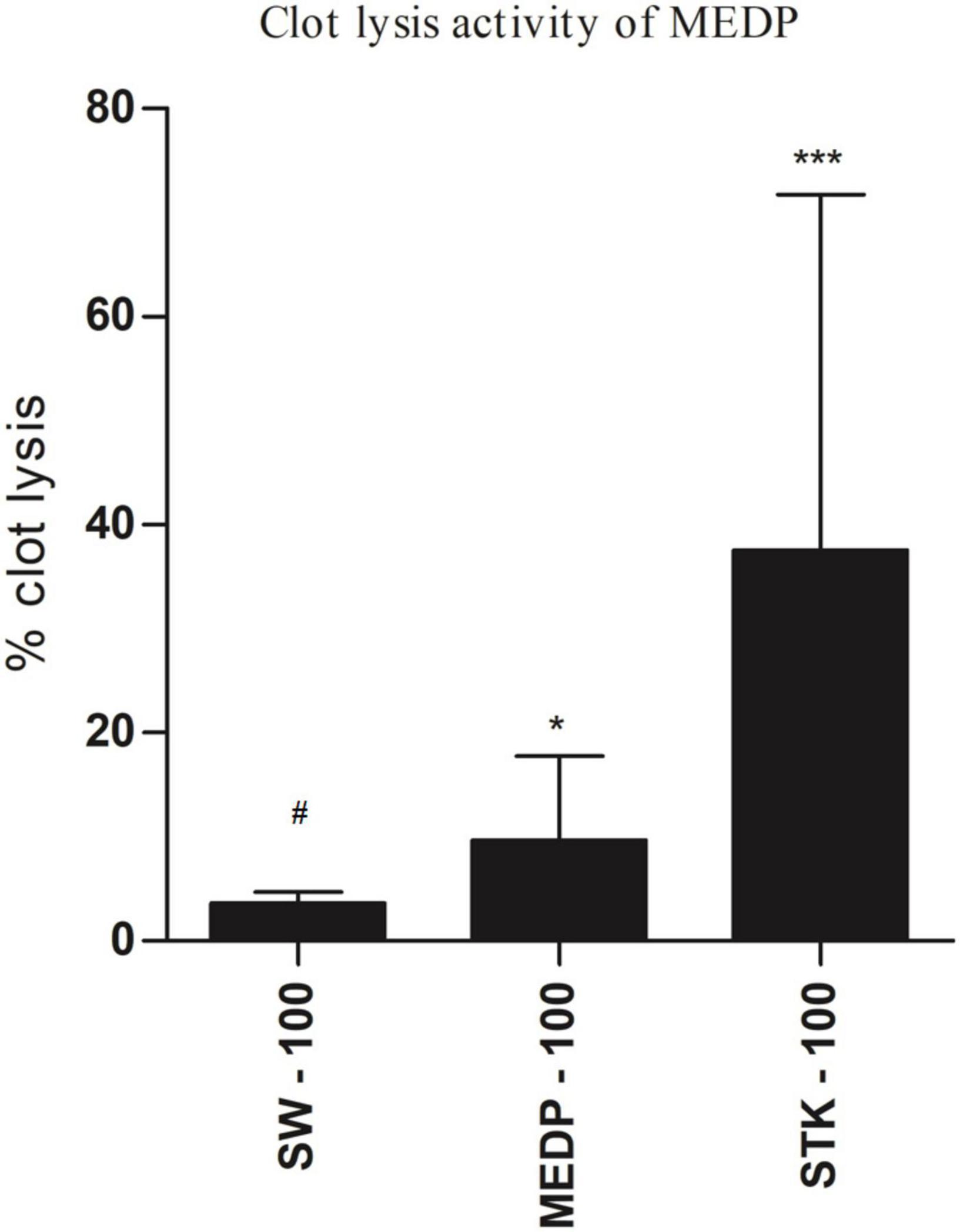
Figure 3. Clot lysis effects by saline water, streptokinase, and MEDP. Anticoagulant values are presented as mean ± SEM (n = 5); One-way ANOVA was followed by Dunnett’s test. *p < 0.05 and ***p < 0.001 were considered as significant compared with the control, where # is designated as control. MEDP = methanol extract of Dillenia pentagyna leaves, SW = saline water, SPK = streptokinase.
Molecular Docking Analysis
The molecular interactions of 6DDF and components of D. pentagyna ranked as Lupeol > Betulin > Quercetin > Isorhamnetin > Kaempferol. The docking rank for the 3LN1 receptor and selected elements is as follows: Betulin > Lupeol > Quercetin > Isorhamnetin > Kaempferol. The best hit of 3DWW with the Quercetin was possessed via a series of residues (glu77, met76, arg73, his72, and arg73). Besides, betulin and lupeol possessed the best binding affinity to the 6VI4 and 1TPM receptors. Betulin binds to the kappa-opioid receptor through pro238 and phe147 residues where lupeol interacted with the tissue-type plasminogen activator through his18 and ser20 residues and the binding scores of these complexes are –9.2 and 7.0 (kcal/mol), respectively. The overall interactions have been pointed out in Table 5 and Figure 4.
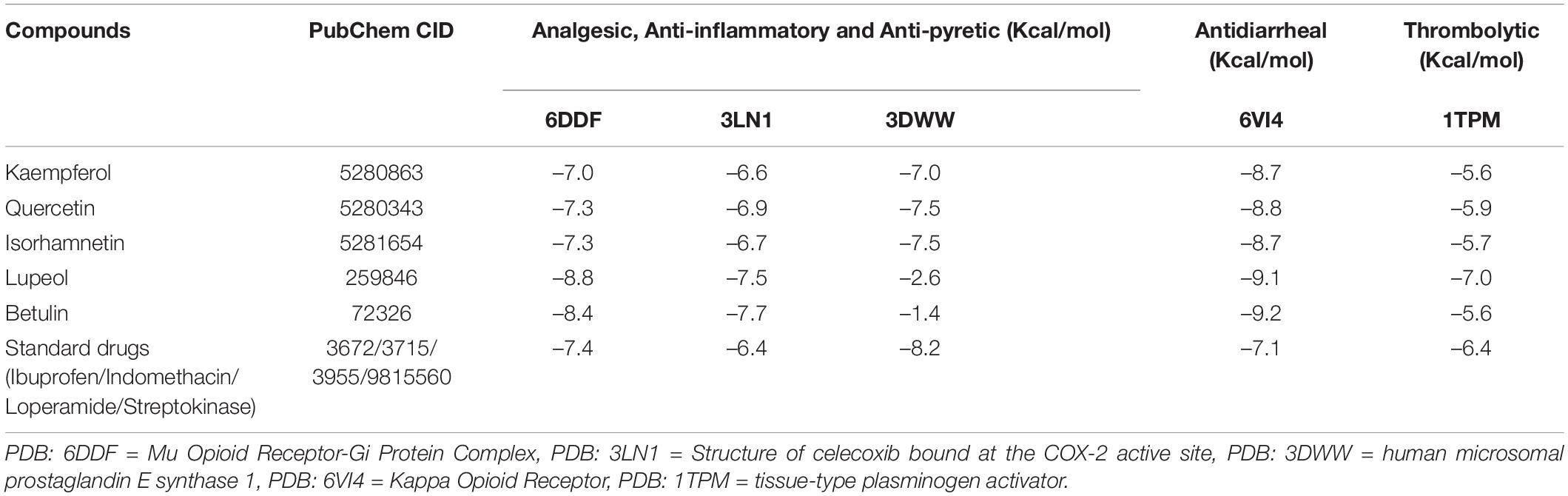
Table 5. The docking score of screened phytochemical’s binding at the active site of the selected proteins.
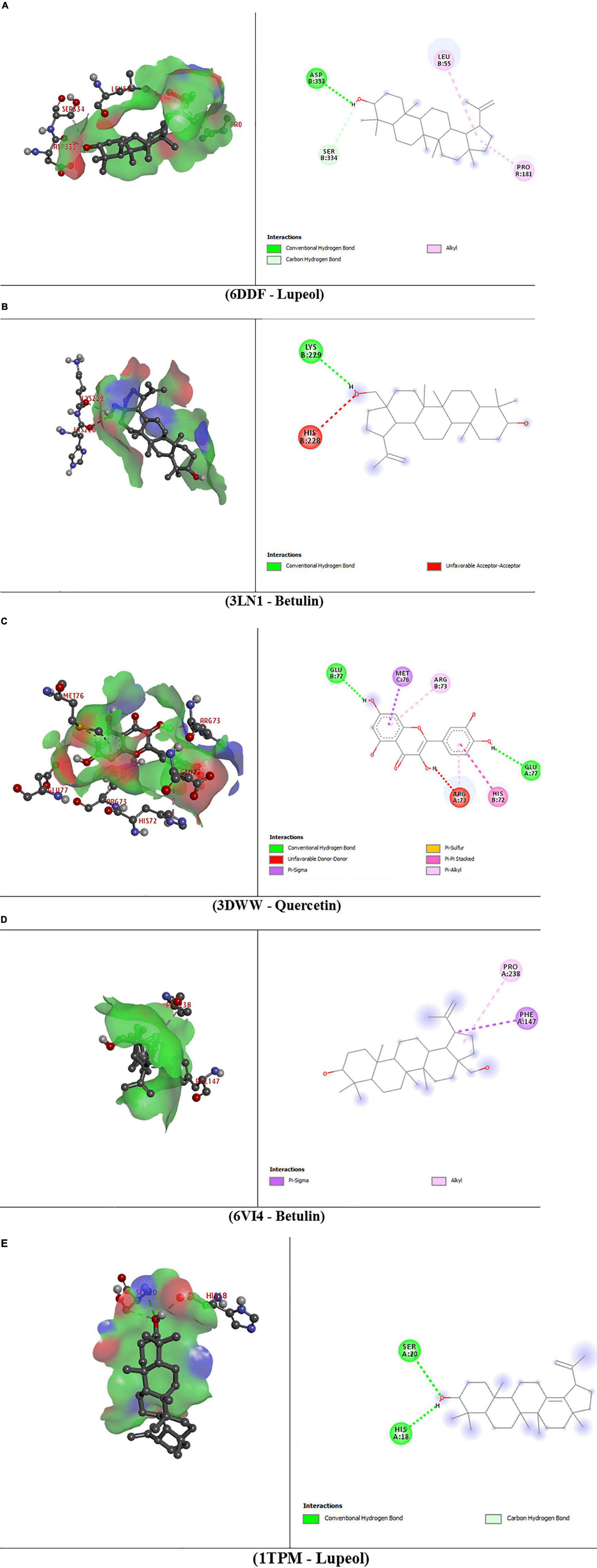
Figure 4. 3D and 2D presentations of the best key interactions in the binding pocket for selected ligands and receptors where panel (A) represents the interaction of mu-opioid receptor-Gi protein complex and lupeol, panel (B) denotes celecoxib bound at the COX-2 active site and betulin, panel (C) denotes microsomal prostaglandin E synthase 1 and quercetin, panel (D) denotes kappa-opioid receptor and betulin, panel (E) denotes the interaction of tissue plasminogen activator and lupeol, respectively.
Discussion
In this study, the analgesic, anti-inflammatory, antipyretic, and antidiarrheal activity of MEDP were evaluated to identify the claims which were made in traditional medicine. The results obtained from the study revealed that the MEDP produced a moderate dose-dependent inhibition of pain response. The standard drugs, however, showed a greater effect than the extract. The acetic acid-induced writhing is a manifestation of peripheral pain (38). Induction of several endogenous biochemical pain mediators like PGE2, PGI2, PGF2α (39), etc. are responsible for further stimulation of nociceptive neurons and an additional amplification of pain sensation takes place through capillary permeability (40). The pain inhibitory capability showed by the extract is a potential reflection of the apprehension of prostaglandins release mimicking the same mechanism followed by aspirin and other non-steroidal anti-inflammatory drugs (NSAIDs). A moderate exhibitory activity showed by MEDP in the formalin-induced licking test can be differentiated between the central and peripheral pain components. Pain induced by formalin is biphasic having an early phase (0–5 min) and the other late phase (15–20 min) (41). Activation of C-fiber neurons potentiates the early phase followed by the release of inflammatory mediators causing the late phase (42). A satisfactory response in both phases is reflected by centrally acting drugs. On the other hand, a peripherally acting drug is only effective in the late phase by inhibiting prostaglandin synthesis. The study result of MEDP reveals a very significant antipyretic effect in Brewer’s yeast-induced elevation of body temperature in a dose-dependent way. It is already documented that elevation of body temperature occurs by the proinflammatory cytokines productions like interleukin-1β (IL-1β) and IL-6, interferon-α (IFN-α), and tumor necrosis factor-α (TNF-α), and prostaglandins like PGE2 and PGI2 by acting on the brain (43, 44). Paracetamol and other antipyretics used in fever management work by reducing prostaglandin levels and acting on cyclooxygenase enzymes, enhancing antipyretic messages within the brain and stimulating anti-inflammatory signals at the injury site (45). A significant drop in temperature in yeast-induced pyrexia is noticed in methanol and petroleum ether fractions of D. pentagyna which is higher than even paracetamol and suggests that the plant possesses a significant antipyretic property. Prostaglandins produced in pyrexia are also an easy target of flavonoids (46). Several studies have confirmed the use of medicinal plants against diarrhea, i.e., antispasmodic activity slows intestinal movement, suppresses gut motility, promotes water adsorption, or decreases intraluminal fluid aggregation (47). Previously, numerous mechanisms were suggested to understand the diarrheal action of castor oil, including suppression of gastrointestinal Na+-K+ ATPase response to minimize normal fluid accumulation, amplification of adenylate cyclase, or effective secretion facilitated by mucosal cAMP (48), enhancement of prostaglandin production, platelet stimulation factor and currently, nitric oxide was believed to lead to the production of prostaglandin (49). Flavonoids and carbohydrates collected from selective conventional medicinal plants in Bangladesh have been claimed to have antidiarrheal capabilities (50). Recently, a variety of medicinal plants had been examined and it was found that the antidiarrheal action of these plants was attributed to flavonoids, alkaloids, tannins, triterpenes, saponins, reducing sugar, and sterols, existing in them (51). Again, the thrombosis or formation of the blood clot is a result of summative cascade action where damaged regions of the endothelial cell surface or blood vessel are barricaded by the platelets deposition, tissue factor, and fibrin (52) where the foundational stage is governed by platelets when the activated platelets form platelets to platelets bonds followed by further binding to the leucocytes and bringing them into a complex process of plaque formation and growth (53). So a thrombolytic agent should possess the aptness to lyse clot by disrupting the fibrinogen and fibrin and plasmin is one of the natural antithrombotic agents (54). Fibrinolysis results from the activation of cell surface-bound plasminogen to plasmin. Streptokinase, which is a bacterial plasminogen activator and a widely used thrombolytic agent, can convert additional plasminogen to plasmin (55). Phytochemicals can be prospective agents in blood clot lysis. Studies have shown that secondary phenolic compounds, such as flavonoids, have a wide range of pharmacological properties (56). Many studies are being done on flavonoids to see if they help fight free radicals, prevent heart disease and cancer, and even protect the liver from damage and inflammation (57). The immune system and inflammatory cells are significantly impacted by certain flavonoids. Flavonoids such as keampferol, quercetin, apigenin, luteolin, and hesperidin have been found to have anti-inflammatory and analgesic activities (56). Inflammation-inducing enzyme systems, such as serine-threonine protein kinases and tyrosine may be affected directly by the antioxidant capabilities of flavonoids (58). Kaempferol’s anti-inflammatory properties were attributed to a slew of mechanisms. This drug inhibits the release of IL-6, IFN-a, and TNF-a (59). The transcriptional activation of Nrf2-regulated genes and the reduction of the DNA-NF-B binding activity of the myeloid differentiation factor 88 (MDF88) are likewise reduced by Kaempferol (60, 61). Quercetin’s anti-inflammatory effects also include its capacity to prevent the synthesis of TNF-a in macrophages and the creation of IL-8 in lung cells (A549). It has been shown that quercetin reduces apoptotic neuronal cell death by inhibiting TNF-1 and IL-1 mRNA expression levels in LPS-stimulated glial cells. Quercetin also inhibits inflammation-causing enzymes [cyclo-oxygenase (COX) and lipoxygenase (LOX)] in RAW 264.7 cells and restricts LPS-induced inflammation by inhibiting Src and Syk in RAW 264.7 cells-mediated tyrosine phosphorylation of PI3K-(p85) and subsequent activation of TLR4/MyD88/PI3K (62). Lupeol’s anti-inflammatory activities were also demonstrated in studies using A23187-stimulated macrophages and triterpenes such pretreatment with lupeol to reduce PGE2 production (63). In the perspective of these observations, flavonoids and triterpenes are critical for the treatment of a wide range of health conditions, namely, chronic pain, acute inflammation, fever, diarrhea, thrombosis, and more. On the other hand, molecular docking is the process of finding the lead compound and hit compound from molecular databases using a scoring function, which has greatly increased the screening efficiency over the classic screen approach. Virtual screening has a wide range of uses. The integrated approach thrives swiftly, especially considering the exponential rise of high-throughput high-performance computing machine learning and deep learning methods (7). Considering this concept, this study also included a molecular docking study and some of the previously identified compounds of D. pentagyna have been docked to the mu-opioid receptor-Gi protein complex, COX-2 receptor, microsomal prostaglandin E synthase 1, and kappa-opioid receptor and the results showed prominent binding affinity of receptors and ligands. Among all compounds, Lupeol, Betulin, and Quercetin yielded the best docking scores which means these compounds have many capabilities of being the best lead compounds and better possibilities of being a good drug for the treatment of prospective diseases. With further research on cell viability tests and in vivo studies, this finding may have important implications in the treatment of cardiovascular diseases which is increasing at an alarming rate. Since the drugs used for cardiovascular diseases are not economical and not accessible to the greater section of society, the application of this study may be a boon for them.
Conclusion
According to the experimental results, MEDP possessing an elevated amount of bioactive phytoconstituents can be a remarkable wellspring of analgesic and antipyretic activities with moderate anti-inflammatory, antidiarrheal, and anticoagulant therapies. The study attempts to evaluate its traditional uses in the management of pain, fever, and diarrhea though further studies are still recommended to ascertain its absolute safety and efficacy profile in the long term use and the establishment of a proper mechanism of action for exerted pharmacological activities. Future prospects of MEDP can be evaluated properly if the plant extract/respective phytochemicals can be subjected to extensive preclinical studies followed by clinical trials. Thus, for the drug-development process, future researchers can give the COX inhibitory potentials of MEDP an exclusive focus.
Data Availability Statement
The original contributions presented in this study are included in the article/supplementary material, further inquiries can be directed to the corresponding authors.
Ethics Statement
The animal study was reviewed and approved by the all biological activity screenings were conducted according to the ethical standards laid down in the Declaration of Helsinki 2013 (64). Animal models were handled and treated according to the principles of the Swiss Academy of Medical Sciences and Swiss Academy of Sciences and were euthanized following the Guidelines for the Euthanasia of Animals: 2013 edition.
Author Contributions
NS, NUE, H-JC, and FA conceptualized and designed the study protocol. NS, NUE, and MTIT prepared the plant extract, designed protocols, conducted the investigations, and collected data. NUE, SA, SR, and AAM calculated the data. NUE and SA revised the manuscript. SA, NUE, NS, and SR wrote the manuscript. SA, SR, and FA supervised and monitored the research. All authors read and approved the final manuscript for publication.
Funding
This research was funded by the Korea Basic Science Institute (KBSI), grant C220000, C280320, and C210500.
Conflict of Interest
The authors declare that the research was conducted in the absence of any commercial or financial relationships that could be construed as a potential conflict of interest.
Publisher’s Note
All claims expressed in this article are solely those of the authors and do not necessarily represent those of their affiliated organizations, or those of the publisher, the editors and the reviewers. Any product that may be evaluated in this article, or claim that may be made by its manufacturer, is not guaranteed or endorsed by the publisher.
References
1. Florentino IF, Silva DP, Galdino PM, Lino RC, Martins JL, Silva DM, et al. Antinociceptive and anti-inflammatory effects of Memora nodosa and allantoin in mice. J Ethnopharmacol. (2016) 186:298–304. doi: 10.1016/j.jep.2016.04.010
2. Ahmad S, Akram M, Ali Shah SM, Sultana S. Evaluation of antipyretic activity of hydroalcoholic extract of Corchorus depressus Linn. in Escherichia coli–induced pyretic rabbits. Eur J Inflamm. (2018) 16:2058739218792958.
3. Hajhashemi V, Sajjadi SE, Heshmati M. Anti-inflammatory and analgesic properties of Heracleum persicum essential oil and hydroalcoholic extract in animal models. J Ethnopharmacol. (2009) 124:475–80. doi: 10.1016/j.jep.2009.05.012
4. Shang X, Miao X, Yang F, Li B, Guo X, Pan H, et al. The anti-diarrheal activity of the non-toxic Dihuang powder in mice. Front Pharmacol. (2018) 9:1037. doi: 10.3389/fphar.2018.01037
5. Lee HS. How safe is the readministration of streptokinase? Drug Saf. (1995) 13:76–80. doi: 10.2165/00002018-199513020-00002
6. Shahidullah A, Haque CE. Linking medicinal plant production with livelihood enhancement in Bangladesh: implications of a vertically integrated value chain. J Transdiscip Environ Stud. (2010) 9:1–18.
8. Morris GM, Lim-Wilby M. Molecular docking. In: A Kukol editor. Molecular modeling of proteins. Berlin: Springer (2008). p. 365–82.
9. Shoichet BK, McGovern SL, Wei B, Irwin JJ. Lead discovery using molecular docking. Curr Opin Chem Biol. (2002) 6:439–46. doi: 10.1016/S1367-5931(02)00339-3
10. Pattanayak P, Behera P, Das D, Panda SK. Ocimum sanctum Linn. A reservoir plant for therapeutic applications: an overview. Pharmacogn Rev. (2010) 4:95–105. doi: 10.4103/0973-7847.65323
11. Dubey P, Sikarwar R, Khanna K, Tiwari AP. Ethnobotany of Dillenia pentagyna Roxb. in vindhya region of Madhya Pradesh, India. Nat Prod Rad. (2009) 8:546–8.
12. Prasad SB, Rosangkima G, Rongpi T. Studies on the ethnomedical value of Dillenia pentagyna Roxb. in murine model. J Pharm Res. (2009) 2:243–9.
13. Abigail EA, Salam JA, Das N. Atrazine degradation in liquid culture and soil by a novel yeast pichia kudriavzevii strain Atz-EN-01 and its potential application for bioremediation. J Appl Pharm Sci. (2013) 3:35.
14. Alam S, Emon NU, Hasib MS, Rashid MA, Soma MA, Saha T, et al. Computer-aided approaches to support the ethnopharmacological importance of Dillenia pentagyna Roxb: an in silico study. Bangladesh Pharm J. (2021) 24:125–32.
15. Gandhi D, Mehta P. Dillenia indica Linn. and Dillenia pentagyna Roxb: pharmacognostic, phytochemical and therapeutic aspects. J Appl Pharm Sci. (1930) 3:134–42.
16. Alam S, Emon NU, Shahriar S, Richi FT, Haque MR, Islam MN, et al. Pharmacological and computer-aided studies provide new insights into Millettia peguensis Ali (Fabaceae). Saudi Pharm J. (2020) 28:1777–90. doi: 10.1016/j.jsps.2020.11.004
17. Care, Animals, Resources. Guide for the Care and Use of Laboratory Animals. Washington, DC: National Academies (1985).
18. Alam S, Rashid MA, Sarker MMR, Emon NU, Arman M, Mohamed IN, et al. Antidiarrheal, antimicrobial and antioxidant potentials of methanol extract of Colocasia gigantea Hook. f. leaves: evidenced from in vivo and in vitro studies along with computer-aided approaches. BMC Complement Med Ther. (2021) 21:11. doi: 10.1186/s12906-021-03290-6
19. Edeoga HO, Okwu D, Mbaebie B. Phytochemical constituents of some nigerian medicinal plants. Afr J Biotechnol. (2005) 4:685–8.
20. Toxic OAOT-A. Class Method-SERIES on Testing and Assessment No. 423. Paris: Organisation for Economic Co-Operation and Development (2001).
21. Zaoui A, Cherrah Y, Mahassini N, Alaoui K, Amarouch H, Hassar M. Acute and chronic toxicity of Nigella sativa fixed oil. Clin Phytosci. (2002) 9:69–74. doi: 10.1078/0944-7113-00084
22. Emon NU, Jahan I, Sayeed MA. Investigation of antinociceptive, anti-inflammatory and thrombolytic activity of Caesalpinia digyna (Rottl.) leaves by experimental and computational approaches. Adv Tradit Med. (2020) 20:451–9. doi: 10.1007/s13596-020-00429-7
23. Hunskaar S, Hole K. The formalin test in mice: dissociation between inflammatory and non-inflammatory pain. Pain. (1987) 30:103–14. doi: 10.1016/0304-3959(87)90088-1
24. Emon NU, Alam S, Rudra S, Al Haidar IK, Farhad M, Rana MEH, et al. Antipyretic activity of the leaves extract of Caesalpinia digyna Rottl along with phytoconstituent’s binding affinity to COX-1, COX-2 and mPGES-1 receptors: an in vivo and in silico approaches (Antipyretic activity of Caesalpinia digyna Rottl). Saudi J Biol Sci. (2021) 28:5302–9. doi: 10.1016/j.sjbs.2021.05.050
25. Rauf A, Uddin G, Siddiqui BS, Muhammad N, Khan H. Antipyretic and antinociceptive activity of Diospyros lotus L. in animals. Asian Pac J Trop Biomed. (2014) 4:S382–6. doi: 10.12980/APJTB.4.2014C1020
26. Emon NU, Alam MM, Sawon MSU, Rana EH, Afroj M, Tanvir MMH. Biological and computational studies provide insights into Caesalphinia digyna Rottler stems. Biochem Biophys Rep. (2021) 26:100994. doi: 10.1016/j.bbrep.2021.100994
27. Shoba FG, Thomas M. Study of antidiarrhoeal activity of four medicinal plants in castor-oil induced diarrhoea. J Ethnopharmacol. (2001) 76:73–6. doi: 10.1016/s0378-8741(00)00379-2
28. Rahman M, Chowdhury M, Uddin A, Islam MT, Uddin ME, Sumi CD. Evaluation of antidiarrheal activity of methanolic extract of Maranta arundinacea Linn. leaves. Adv Pharmacol Sci. (2015) 2015:257057. doi: 10.1155/2015/257057
29. Ahmed F, Rahman MS. Preliminary assessment of free radical scavenging, thrombolytic and membrane stabilizing capabilities of organic fractions of Callistemon citrinus (Curtis.) skeels leaves. BMC Complement Alternat Med. (2016) 16:247. doi: 10.1186/s12906-016-1239-1
30. Koehl A, Hu H, Maeda S, Zhang Y, Qu Q, Paggi JM, et al. Structure of the μ-opioid receptor-G(i) protein complex. Nature. (2018) 558:547–52. doi: 10.1038/s41586-018-0219-7
31. Wang JL, Limburg D, Graneto MJ, Springer J, Hamper JR, Liao S, et al. The novel benzopyran class of selective cyclooxygenase-2 inhibitors. Part 2: the second clinical candidate having a shorter and favorable human half-life. Bioorg Med Chem Lett. (2010) 20:7159–63. doi: 10.1016/j.bmcl.2010.07.054
32. Jegerschöld C, Pawelzik SC, Purhonen P, Bhakat P, Gheorghe KR, Gyobu N, et al. Structural basis for induced formation of the inflammatory mediator prostaglandin E2. Proc Natl Acad Sci USA. (2008) 105:11110–5. doi: 10.1073/pnas.0802894105
33. Che T, English J, Krumm BE, Kim K, Pardon E, Olsen RHJ, et al. Nanobody-enabled monitoring of kappa opioid receptor states. Nat Commun. (2020) 11:1145. doi: 10.1038/s41467-020-14889-7
34. Downing AK, Driscoll PC, Harvey TS, Dudgeon TJ, Smith BO, Baron M, et al. Solution structure of the fibrin binding finger domain of tissue-type plasminogen activator determined by 1H nuclear magnetic resonance. J Mol Biol. (1992) 225:821–33. doi: 10.1016/0022-2836(92)90403-7
35. Hossain MS, Barua A, Tanim MAH, Hasan MS, Islam MJ, Hossain MR, et al. Ganoderma applanatum mushroom provides new insights into the management of diabetes mellitus, hyperlipidemia, and hepatic degeneration: a comprehensive analysis. Food Sci Nutr. (2021) 9:4364–74. doi: 10.1002/fsn3.2407
36. Emon NU, Rudra S, Alam S, Haidar IKA, Paul S, Richi FT, et al. Chemical, biological and protein-receptor binding profiling of Bauhinia scandens L. stems provide new insights into the management of pain, inflammation, pyrexia and thrombosis. Biomed Pharmacother. (2021) 143:112185. doi: 10.1016/j.biopha.2021.112185
37. Emon NU, Alam S, Rudra S, Riya SR, Paul A, Hossen SMM, et al. Antidepressant, anxiolytic, antipyretic, and thrombolytic profiling of methanol extract of the aerial part of Piper nigrum: in vivo, in vitro, and in silico approaches. Food Sci Nutr. (2021) 9:833–46. doi: 10.1002/fsn3.2047
38. Banerjee S, Mukherjee A, Chatterjee TK. Evaluation of analgesic activities of methanolic extract of medicinal plant Juniperus communis Linn. Int J Pharm Pharm Sci. (2012) 4:547–50.
39. Zahid H, Rizwani GH, Shareef H, Ahmed M, Hina B. Analgesic and antipyretic activities of Hibiscus schizopetalus (Mast.) hook. Int J Pharm Pharm Sci. (2012) 4:218–21.
40. Amico-Roxas M, Caruso A, Trombadore S, Scifo R, Scapagnini U. Gangliosides antinociceptive effects in rodents. Arch Int Pharm Ther. (1984) 272:103–17.
41. Kolesnikov Y, Cristea M, Oksman G, Torosjan A, Wilson R. Evaluation of the tail formalin test in mice as a new model to assess local analgesic effects. Brain Res. (2004) 1029:217–23. doi: 10.1016/j.brainres.2004.09.058
42. Shibata M, Ohkubo T, Takahashi H, Inoki R. Modified formalin test: characteristic biphasic pain response. Pain. (1989) 38:347–52. doi: 10.1016/0304-3959(89)90222-4
43. Luheshi GN. Cytokines and fever: mechanisms and sites of action. Ann N Y Acad Sci. (1998) 856:83–9. doi: 10.1111/j.1749-6632.1998.tb08316.x
44. Veale W, Cooper K, Pittman Q. Role of prostaglandins in fever and temperature regulation. In: PW Ramwell editor. The Prostaglandins. Heidelberg: Springer (1977). p. 145–67.
45. Aronoff DM, Neilson EG. Antipyretics: mechanisms of action and clinical use in fever suppression. Am J Med. (2001) 111:304–15. doi: 10.1016/S0002-9343(01)00834-8
46. Narayana KR, Reddy MS, Chaluvadi M, Krishna D. Bioflavonoids classification, pharmacological, biochemical effects and therapeutic potential. Indian J Pharmacol. (2001) 33:2–16.
47. Atta AH, Mouneir SM. Evaluation of some medicinal plant extracts for antidiarrhoeal activity. Phytother Res. (2005) 19:481–5. doi: 10.1002/ptr.1639
48. Capasso F, Mascolo N, Izzo AA, Gaginella TS. Dissociation of castor oil-induced diarrhoea and intestinal mucosal injury in rat: effect of NG-nitro-L-arginine methyl ester. Br J Pharmacol. (1994) 113:1127–30. doi: 10.1111/j.1476-5381.1994.tb17113.x
49. Mascolo N, Izzo AA, Gaginella TS, Capasso F. Relationship between nitric oxide and platelet-activating factor in castor-oil induced mucosal injury in the rat duodenum. Naunyn-Schmiedeberg’s Arch Pharmacol. (1996) 353:680–4. doi: 10.1007/BF00167187
50. Islam MM, Pia RS, Sifath-E-Jahan K, Chowdhury J, Akter F, Parvin N, et al. Antidiarrheal activity of Dillenia indica bark extract. Int J Pharm Sci Res. (2013) 4:682.
51. Longanga Otshudi A, Vercruysse A, Foriers A. Contribution to the ethnobotanical, phytochemical and pharmacological studies of traditionally used medicinal plants in the treatment of dysentery and diarrhoea in Lomela area, Democratic Republic of Congo (DRC). J Ethnopharmacol. (2000) 71:411–23. doi: 10.1016/S0378-8741(00)00167-7
53. Das A, Dewan SMR, Ali MR, Debnath PC, Billah MM. Investigation of in vitro thrombolytic potential of ethanolic extract of Momordica charantia fruits: an anti-diabetic medicinal plant. Der Pharm Sin. (2013) 4:104–8.
54. Pantzar M, Ljungh Å, Wadström T. Plasminogen binding and activation at the surface of Helicobacter pylori CCUG 17874. Infect Immu. (1998) 66:4976–80. doi: 10.1128/IAI.66.10.4976-4980.1998
55. Bhowmick R, Sarwar MS, RahmanDewan SM, Das A, Das B, NasirUddin MM, et al. In vivo analgesic, antipyretic, and anti-inflammatory potential in Swiss albino mice and in vitro thrombolytic activity of hydroalcoholic extract from Litsea glutinosa leaves. Biol Res. (2014) 47:56. doi: 10.1186/0717-6287-47-56
56. Kumar S, Pandey AK. Chemistry and biological activities of flavonoids: an overview. Sci World J. (2013) 2013:162750. doi: 10.1155/2013/162750
57. Dias MC, Pinto DCGA, Silva AMS. Plant flavonoids: chemical characteristics and biological activity. Molecules. (2021) 26:5377. doi: 10.3390/molecules26175377
58. Nishizuka Y. The molecular heterogeneity of protein kinase C and its implications for cellular regulation. Nature. (1988) 334:661–5. doi: 10.1038/334661a0
59. Tang X.-l, Liu J-X, Dong W, Li P, Li L, Hou J-C, et al. Protective Effect of kaempferol on LPS plus ATP-induced inflammatory response in cardiac fibroblasts. Inflammation. (2015) 38:94–101. doi: 10.1007/s10753-014-0011-2
60. Alam W, Khan H, Shah MA, Cauli O, Saso L. Kaempferol as a dietary anti-inflammatory agent: current therapeutic standing. Molecules. (2020) 25:4073. doi: 10.3390/molecules25184073
61. Zhang R, Ai X, Duan Y, Xue M, He W, Wang C, et al. Kaempferol ameliorates H9N2 swine influenza virus-induced acute lung injury by inactivation of TLR4/MyD88-mediated NF-κB and MAPK signaling pathways. Biomed Pharmacother. (2017) 89:660–72. doi: 10.1016/j.biopha.2017.02.081
62. Li Y, Yao J, Han C, Yang J, Chaudhry MT, Wang S, et al. Quercetin. Inflammation and Immunity. Nutrients. (2016) 8:167. doi: 10.3390/nu8030167
63. Saleem M. Lupeol, a novel anti-inflammatory and anti-cancer dietary triterpene. Cancer Lett. (2009) 285:109–15. doi: 10.1016/j.canlet.2009.04.033
Keywords: Dillenia pentagyna, pyrexia, antidiarrheal, antipyretic, thrombolytic, anti-inflammatory, molecular docking, ethnomedicinal plant
Citation: Sultana N, Chung H-J, Emon NU, Alam S, Taki MTI, Rudra S, Tahamina A, Alam R, Ahmed F and Mamun AA (2022) Biological Functions of Dillenia pentagyna Roxb. Against Pain, Inflammation, Fever, Diarrhea, and Thrombosis: Evidenced From in vitro, in vivo, and Molecular Docking Study. Front. Nutr. 9:911274. doi: 10.3389/fnut.2022.911274
Received: 02 April 2022; Accepted: 06 June 2022;
Published: 12 July 2022.
Edited by:
Yasmina Sultanbawa, The University of Queensland, AustraliaReviewed by:
Katsuyoshi Matsunami, Hiroshima University, JapanMd Saquib Hasnain, Palamau Institute of Pharmacy, India
Copyright © 2022 Sultana, Chung, Emon, Alam, Taki, Rudra, Tahamina, Alam, Ahmed and Mamun. This is an open-access article distributed under the terms of the Creative Commons Attribution License (CC BY). The use, distribution or reproduction in other forums is permitted, provided the original author(s) and the copyright owner(s) are credited and that the original publication in this journal is cited, in accordance with accepted academic practice. No use, distribution or reproduction is permitted which does not comply with these terms.
*Correspondence: Safaet Alam, c2FmYWV0LmR1QGdtYWlsLmNvbQ==; Sajib Rudra, cnVkcmFzYWppYmN1ODlAZ21haWwuY29t
†ORCID: Safaet Alam, orcid.org/0000-0002-1831-2278; Sajib Rudra, orcid.org/0000-0003-1030-1379
‡These authors have contributed equally to this work