- 1Department of Animal Production, Faculty of Agriculture, Al-Azhar University, Cairo, Egypt
- 2Department of Dairy Science and Food Technology, Institute of Agricultural Sciences, Banaras Hindu University, Varanasi, India
- 3Department of Animal and Poultry Production, Faculty of Agriculture, Damanhour University, Damanhour, Egypt
- 4Sustainable Agriculture Research Group, Department of Agriculture, Faculty of Environmental Sciences, King Abdulaziz University, Jeddah, Saudi Arabia
- 5Department of Pharmacology, Faculty of Veterinary Medicine, Zagazig University, Zagazig, Egypt
- 6Laboratory of Biotechnology, Faculty of Veterinary Medicine, Zagazig University, Zagazig, Egypt
- 7Biology Department, College of Science, Imam Abdulrahman Bin Faisal University, Dammam, Saudi Arabia
- 8Avian and Rabbit Diseases Department, Faculty of Veterinary Medicine, Benha University, Banha, Egypt
- 9Agricultural Botany Department, Faculty of Agriculture (Saba Basha), Alexandria University, Alexandria, Egypt
- 10Department of Agricultural Microbiology, Faculty of Agriculture, Zagazig University, Zagazig, Egypt
- 11Biochemistry Department, Faculty of Agriculture, Zagazig University, Zagazig, Egypt
- 12Biological Applications Department, Nuclear Research Center, Egyptian Atomic Energy Authority, Abu Zaabal, Egypt
Banning antibiotic growth promoters has negatively impacted poultry production and sustainability, which led to exploring efficient alternatives such as probiotics, probiotics, and synbiotics. Effect of in ovo injection of Bacillus subtilis, raffinose, and their synbiotics on growth performance, cecal microbial population and volatile fatty acid concentration, ileal histomorphology, and ileal gene expression was investigated in broilers (Gallus gallus) raised for 21 days. On 300 h of incubation, a total of 1,500 embryonated eggs were equally allotted into 10 groups. The first was non-injected (NC) and the remaining in ovo injected with sterile distilled water (PC), B. subtilis 4 × 105 and 4 × 106 CFU (BS1 and BS2), Raffinose 2 and 3 mg (R1 and R2), B. subtilis 4 × 105 CFU + raffinose 2 mg (BS1R1), B. subtilis 4 × 105 CFU + raffinose 3 mg (BS1R2), B. subtilis 4 × 106 CFU + raffinose 2 mg (BS2R1), and B. subtilis 4 × 106 CFU + raffinose 3 mg (BS2R2). At hatch, 60 chicks from each group were randomly chosen, divided into groups of 6 replicates (10 birds/replicate), and fed with a corn–soybean-based diet. In ovo inoculation of B. subtilis and raffinose alone or combinations significantly improved body weight, feed intake, and feed conversion ratio of 21-day-old broilers compared to NC. Cecal concentrations of butyric, pentanoic, propionic, and isobutyric acids were significantly elevated in R1, R2, BS2R1, and BS2R2, whereas isovaleric and acetic acids were significantly increased in R1 and BS2R1 compared to NC. Cecal microbial population was significantly altered in treated groups. Ileal villus height was increased (p < 0.001) in BS1, R2, and BS2R2 compared to NC. The mRNA expression of mucin-2 was upregulated (p < 0.05) in synbiotic groups except for BS1R1. Vascular endothelial growth factor (VEGF) expression was increased (p < 0.05) in BS2, R1, BS1R1, and BS1R2 compared to NC. SGLT-1 expression was upregulated (p < 0.05) in all treated birds except those of R1 group compared to NC. The mRNA expressions of interleukin (IL)-2 and toll-like receptor (TLR)-4 were downregulated (p < 0.05) in BS2 and R1 for IL-2 and BS1R1 and BS2R2 for TLR-4. It was concluded that in ovo B. subtilis, raffinose, and synbiotics positively affected growth performance, cecal microbiota, gut health, immune responses, and thus the sustainability of production in 21-day-old broilers.
Introduction
The growing public pressure to ban sub-therapeutic antibiotics from poultry diets has impacted poultry producers by losing profits and seeking alternatives to achieve the same productivity and food quality control (1–4). Therefore, an urgent need exists to understand better the molecular and cellular interaction between the gut microbiota and host that natural compounds may manipulate to maintain gut homeostasis and enhance growth performance and animal productivity (5, 6). In chickens, the gut microbiota is critical to the host’s health, as it affects immunological responses and nutrition utilization and maintains the digestive system in proper working order (7).
Early colonization of the chicken gut by healthy bacteria provides better protection against future environmental and disease threats. The commensal gut microbiota competes with pathogens and assists the host’s intestinal epithelium and immune system maturity (8, 9). A healthy gastrointestinal system with optimal structure and function is required for broiler chickens to achieve rapid growth rates (10). Commercially, first exposure to pathogenic bacteria can occur before hatch due to hatchery or farm contamination, resulting in early chick mortality and severe economic losses (7, 11). Therefore, the early establishment of beneficial bacteria in the chicken gut is critical for preventing pathogen colonization, enhancing the immune system and gastrointestinal development, and overall health (7, 12, 13). Probiotics, prebiotics, and synbiotics are some of the compounds investigated as possible alternatives to antibiotic growth promoters in the poultry industry.
Probiotics are beneficial living bacteria that enhance innate and adaptive immunity and protect against intestinal inflammation (14). It has been found that the majority of probiotic microorganisms are Gram-positive bacteria, such as Bacillus spp., Lactobacillus, Bifidobacteria spp., and Lactococcus spp. (12, 15). Some probiotic bacteria are known to produce bioactive substances such as antimicrobial peptides and bacteriocins that can exert an antimicrobial effect against pathogenic and undesirable bacteria (16). The metabolic slowdown of the spore-forming B. subtilis helps resist severe conditions, including harsh pH and temperature conditions (17, 18). Therefore, these bacteria can benefit the host’s health via decreasing intestinal pH, boosting the immune system, preventing the pathogen growth, enhancing the gut development, and promoting the growth performance (19, 20).
Prebiotics are specialized plant fiber that acts as substrates for beneficial bacteria. Raffinose, as a prebiotic, is a trisaccharide compound found in whole grains, cabbage, beans, brussels sprouts, asparagus, and other vegetables. Prebiotics modulate the gut microbiota by improving the abundance of specific beneficial bacteria. Therefore, it alters the structure of the microbiota community and enhances host’s health. Furthermore, prebiotics can affect nutrient utilization, most likely through prebiotic–microbe interactions (7). Hence, combining probiotics and appropriate prebiotics (synbiotics) is an innovative and revolutionary method to collect the benefits of their biological interactions, which can improve nutrient uptake and host health (21).
Administrating bioactive compounds in poultry feed may encounter obstacles, such as exposure to the high temperature during the manufacturing process, affecting their nutritional value or bioactive functions (12). In addition, the biological value of these substances, when supplemented in drinking water, may be affected by watering devices and water quality. In ovo route is an innovative and effective method, especially as it delivers small amounts of bioactive substances with high efficiency compared to other supplementation routes (12, 22, 23).
Previous studies demonstrated that in ovo inoculation of probiotics (22, 23), prebiotics, and synbiotics (21, 24) maintained the balance of gut microbiota, improved the growth performance, and enhanced the sustainability of broiler chickens. However, limited studies have been conducted on in ovo injection of B. subtilis and their combination with raffinose in broiler chickens. Based on the above considerations, we hypothesized that in ovo injection of B. subtilis, raffinose, and their synbiotics would improve the performance and overall health status of broiler chickens at 21 days of age. Therefore, by using the Gallus gallus in vivo model (7, 25), the current study was conducted to evaluate the effect of in ovo inoculation with different levels of probiotic (B. subtilis PB6), prebiotic (raffinose), and their combinations on growth performance, cecal microbial population, cecal volatile fatty acid (VFA) concentration, ileal histomorphology, and ileal gene expression.
Materials and Methods
Ethics Statement
All animal care procedures were approved by the Central Animal Ethical Committee of Banaras Hindu University (542/GO/ReBi/S/02/CPCSEA 2017)/IAEC/3037.
Incubation and Materials
Fertile eggs were obtained from a local broiler breeder facility (Indian River) at 48 weeks of age. Petersime incubator (Petersime Nv, Zulte, Belgium) was used for egg incubation following the standard commercial conditions (37.5°C and 60% relative humidity). Egg weight was approximately 65.7 g. On day 10 of egg incubation, the eggs were candled, and infertile, non-viable, and contaminated eggs were discarded. In this study, we have used probiotics B. subtilis PB6 (ClOSTAT) provided by Kemin® (Herentals, Belgium) and prebiotic (Raffinose) supplied by Sigma-Aldrich (St. Louis, MO, United States).
In ovo Treatment
After 300 h of incubation, a total of 1,500 embryonated eggs were randomly allotted to 10 groups (n = 150 embryonated eggs per group). The treatment solutions were prepared on the day of injection. Approximately 0.2 ml of treatment solutions were injected into the air cell using a 21-gauge needle on an automatic injector (NJ Phillips Pty Limited, Somersby, Australia). The applied treatments were: (1) non-injected (NC) group was non-injected; (2) sterile distilled water (PC) group was injected with sterile distilled water; (3) in ovo injection with B. subtilis 4 × 105CFU/egg (BS1); (4) in ovo injection with B. subtilis 4 × 106CFU/egg (BS2); (5) in ovo injection with Raffinose 2 mg/egg (R1); (6) in ovo injection with raffinose 3 mg/egg (R2); (7) in ovo injection with B. subtilis 4 × 105CFU + raffinose 2 mg/egg (BS1R1); (8) in ovo injection with B. subtilis 4 × 105CFU + raffinose 3 mg/egg (BS1R2); (9) in ovo injection with B. subtilis 4 × 106CFU + raffinose 2 mg/egg (BS2R1); and (10) in ovo injection with B. subtilis 4 × 106CFU + raffinose 3 mg/egg (BS2R2). The doses of probiotic and synbiotic were previously determined by a preliminary experiment. The effect of different concentrations of both probiotic and synbiotic on embryonic mortality and hatchability was tested. As for prebiotic, concentrations were used, according to previous research (26).
Chicks, Diets, and Experimental Design
At hatch, 60 chicks from each treatment (600 chicks in total) were randomly chosen, allocated to 6 replicates (10 chicks each), and caged in separated metal cages (50 cm × 35 cm × 34 cm) prepared for the newly hatched chicks under controlled environmental conditions and continuous lighting. Feed and drinking water were provided ad libitum. Birds were fed on a crumbled diet (corn- and soybean meal-based diets) for a 21-day post-hatch trial period (starter diet, day 1 to 10; grower, day 11 to 21). Nutrient composition of diets (Table 1) was calculated based on NRC (27) tables of feedstuff analysis to meet the nutrient requirements of the strain, Indian River, Aviagen 2019. All birds were vaccinated against Newcastle disease virus (NDV) and infectious bronchitis virus (IBV) on the 7th day and given a booster against NDV on the 17th day of the experiment. Amprolium 20% (water-soluble powder), 75 g/100 L of drinking water, was used for 3 days during the second week as an anticoccidial drug.
All the experimental groups did not receive any antibiotics.
Growth Performance
The birds of each replicate were weighed on day 21 of age. Feed intake (FI) was recorded, and feed conversion ratio (FCR) was calculated on day 21 of age on a replication basis.
FI = Feed consumption/number of birds.
FCR = Feed consumption/body weight.
Sample Collection
On day 21, 6 birds per treatment (1 bird/replicate) were randomly selected and euthanized by cervical dislocation. Cecal contents were immediately collected into sterile tubes and stored at −20°C for the microbial count and VFA analysis. Ileal samples (approximately 1.5 cm in length of the mid-ileum) were excised and flushed with 0.9% saline to remove all the contents and then fixed in 10% buffered formalin solution for subsequent histomorphological investigations. A section of the mid-ileum (approximately 1.5 cm) was collected, washed with PBS, and immersed in the Trizol reagent for subsequent gene expression investigation.
Histomorphometric Study
Fixed ileal samples were processed, and 4-μm-thick tissue sections were cut out of the paraffin-embedded tissue blocks and stained with hematoxylin and eosin following the protocol of Bancroft and Gamble (28). Stained tissues were examined under a light microscope (Leica DM300 with Leica FLEXACAM C1), whereas representative fields were photographed for morphometrics using Leica LAS X dedicated software. Villus height (VH) and villus width (VW), crypt depth (CD), and muscular thickness were measured. The above-mentioned parameters were measured as the mean of 10 randomly selected parts in each sample. Finally, villus surface area was measured by considering a villus as a cylindrical structure (29) according to the following equation[(2π) × (villus width/2) × (villus height)].
Bacteriological Examination
One gram of each cecal sample was homogenized in 9 ml of sterilized saline peptone solution and stirred for 30 min to obtain 10–1dilution. Decimal serial dilutions were prepared from the previous (10–1) to 10–7. According to Abd El-Hack et al. (30) and Alagawany et al. (31), an aliquot of 0.1 ml of each dilution was spread over different specific media such as plate count agar (PCA) for total bacterial count (TBC) after incubation at 30°C for 48 h (30). Sabouraud Dextrose Agar (SDA) was used to enumerate total yeast and molds count (TYMC) after incubation at 30°C at 24 h for yeasts and 25°C for 5 days for fungi (32). Violet red bile agar, MacConkey agar, and Bacillus cereus agar (Oxoid) were used for total coliforms, Escherichia coli, and B. subtills, respectively, after incubation at 37°C for 24 h. Bacillus cereus agar (Oxoid) was used for counting B. subtills after incubation for 24 h at 37°C. DeManRogosa-Sharpe (MRS) medium and Chromocult enterococci agar were used for lactic acid bacteria and Enterococcus spp., respectively, after incubation at 37°C for 48 h. The microbial counts were converted into log10 CFU/g.
Volatile Fatty Acid Concentration
Cecal content samples were collected and kept frozen until VFA analysis according to the procedures described by Saad et al. (33). The concentrations of VFA were measured using a Mass Spectrometer Agilent 5975C, carrier gas helium, column HP-5ms (30 m × 250 μm × 0.25 μm), and temperature: 35°C/3 min, 5°C/min to 250°C for 3 min, total 49 min, carrier gas helium 1 ml/min constant speed; split ratio 30:1.
Quantitative Real-Time PCR Analyses
Total RNA was extracted from the ileum using Trizol (Invitrogen; Thermo Fisher Scientific, Inc.) and then reverse-transcribed to cDNA using a High-Capacity cDNA Reverse Transcription Kit (Applied Biosystems™, Waltham, MA, United States) following the manufacturer’s protocol. Real-time RT-PCR was performed in an Mx3005P Real-Time PCR System (Agilent Stratagene, United States) using TOPreal™ qPCR 2 × PreMIX (SYBR Green with low ROX) (Enzynomics, Korea) following the manufacturer’s instructions and according to the previous studies (34–36). The PCR cycling conditions included an initial denaturation at 95°C for 12 min followed by 40 cycles of denaturation at 95°C for 20 s, annealing at 60°C for 30 s, and with an extension at 72°C for 30 s. The expression level of the target genes was normalized using the mRNA expression of a known housekeeping gene, B-actin. Results are expressed as fold changes compared to the control groups following the 2–ΔΔCT method (37). The primer sequences used are given in Table 2.
Statistical Analysis
Data were analyzed using one-way ANOVA (SPSS Inc., 2018). The statistical model is given as:
Yij = μ + TRTi + eij
where Yij represents the observation for the dependent variables at the jth replicate in the ith treatment (i = 1 to 10), μ is the overall mean, TRTi is the fixed effect of treatments (i = 1 to 10), and eij is the random residual error.
Normality of the distribution was tested with the Kolmogorov–Smirnov test, whereas the homogeneity of variance in the samples was assessed with Levene’s test. The means were compared using Tukey’s multiple range test. The cage served as the experimental unit for comparing growth performance, whereas individuals’ data served as the experimental units for the remaining parameters. Data are presented as means ± SEM, and the significance was declared at p < 0.05.
Results
Growth Performance
The effects of in ovo inoculation of B. subtilis, raffinose, and their combination on the growth performance of broiler chickens at 21 days of age are presented in Table 3. All treated groups had elevated (p < 0.01) live body weight (BW) compared to NC. BS1R2, BS1R1, and BS2R2, respectively, and recorded the heaviest weight compared to other groups. In ovo supplementation with different levels of B. subtilis, raffinose, and their synbiotics improved (p < 0.01) FI and FCR during the overall period compared to the control groups.
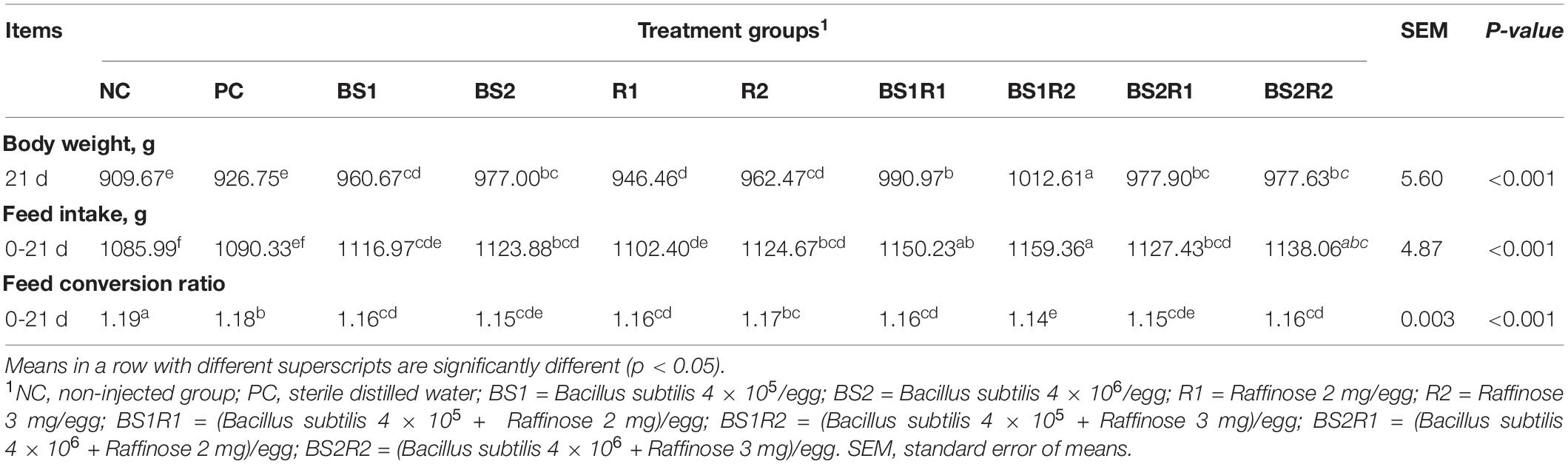
Table 3. Effect of in ovo inclusion of Bacillus subtilis, raffinose, and their synbiotics on growth performance of 21-day-old broilers.
Cecal Volatile Fatty Acid Concentration
Concentrations of major VFA in cecal contents of 21-day-old broilers as influenced by in ovo treatments are presented in Table 4. Concentrations of butyric, pentanoic, propionic, and isobutyric acids were elevated (p < 0.001) in R1, R2, BS2R1, and BS2R2 groups. In contrast, levels of isovaleric and acetic acids were increased (p < 0.001) only in R1 and BS2R1 groups compared to the control groups. R1 group recorded the highest cecal VFA levels, followed by BS2R1.
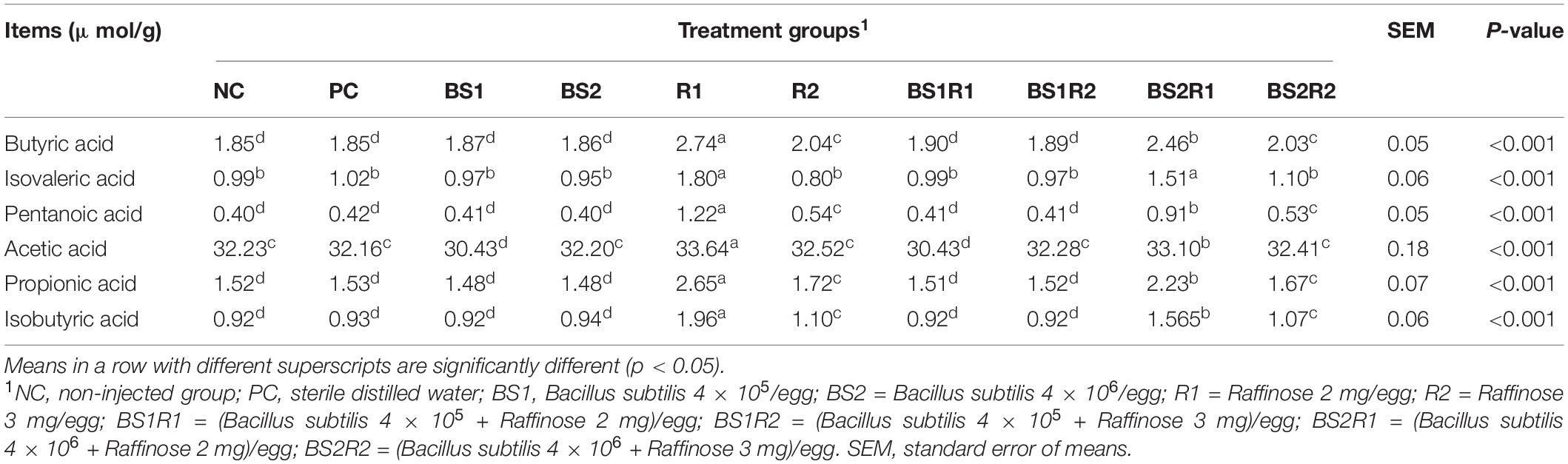
Table 4. Effect of in ovo inclusion of Bacillus subtilis, raffinose, and their synbiotics on cecal volatile fatty acid concentrations at 21-day-old broilers.
Microbial Enumeration
Cecal microbial enumeration of broilers at 21 days of age was remarkably influenced (p < 0.001) by in ovo administration of B. subtilis, raffinose, and their synbiotics (Table 5). Cecal population of B. subtilis and lactic acid bacteria was elevated (p < 0.001) by in ovo probiotics and synbiotics compared to NC. The count of total molds and yeast was not altered among experimental groups. The population of E. coli, Enterococcus spp., total coliform, and the total bacterial count were decreased (p < 0.001) in the cecal contents of all treated birds compared to the control groups.
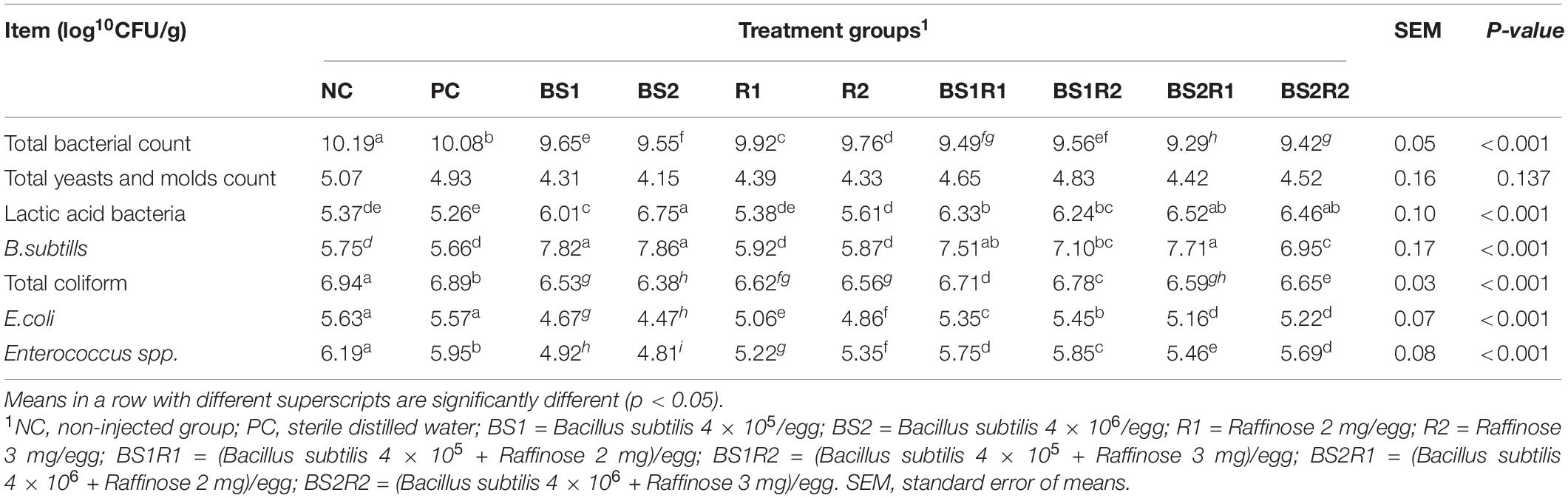
Table 5. Effect of in ovo inclusion of Bacillus subtilis, raffinose, and their synbiotics on cecal microbial population of 21-day-old broilers.
Ileal Histomorphometry
The architecture of ileal samples of 21-day-old broilers in ovo treated with B. subtilis, raffinose, and their synbiotics is presented in Figure 1 and Table 6. Ileal VH was significantly increased (p < 0.001) in BS1, R2, and BS2R2 compared to NC. The highest value of ileal VH has observed in the group that received a high prebiotic level. However, VW and CD were not significantly affected by the in ovo supplements. Significant differences (p < 0.05) were found in the values of muscular thickness among the different groups. BS2R2 group had the highest value compared to the other groups. Values of villus surface area showed an increasing trend compared to the control groups. However, this increase was not statistically significant (p > 0.05).
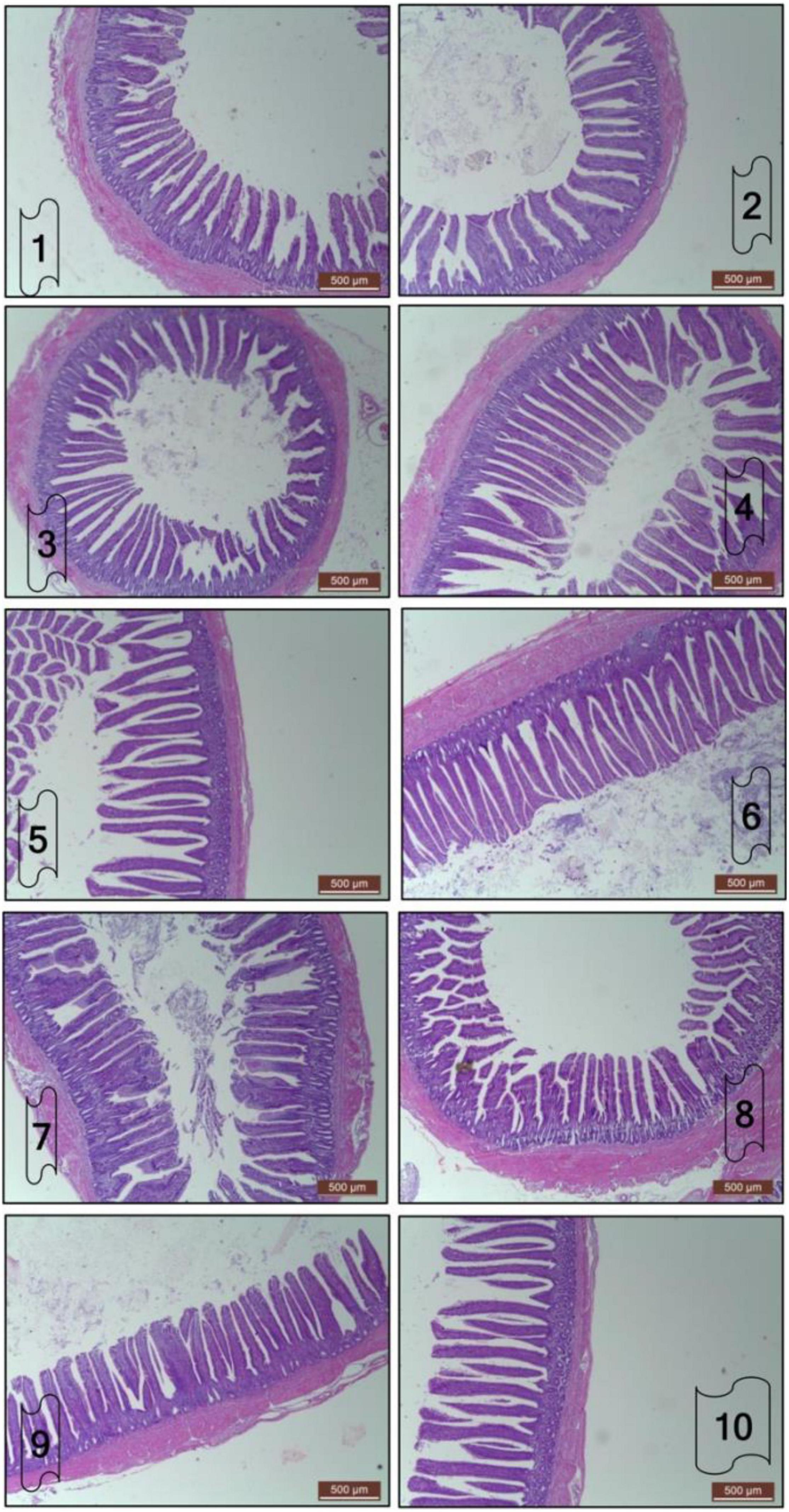
Figure 1. Effect of in ovo inclusion of Bacillus subtilis, raffinose, and their synbiotics on ileal histomorphometry of 21-day-old broilers. Each number on the figure means the following groups: 1 = non-injected group; 2 = sterile distilled water; 3 = Bacillus subtilis 4 × 105/egg; 4 = Bacillus subtilis 4 × 106/egg; 5 = Raffinose 2 mg/egg; 6 = Raffinose 3 mg/egg; 7 = (Bacillus subtilis 4 × 105 + Raffinose 2 mg)/egg; 8 = (Bacillus subtilis 4 × 105 + Raffinose 3 mg)/egg; 9 = (Bacillus subtilis 4 × 106 + Raffinose 2 mg)/egg; 10 = (Bacillus subtilis 4 × 106 + Raffinose 3 mg)/egg. Images were captured with light microscopy. Scale bar indicates 500 μm.
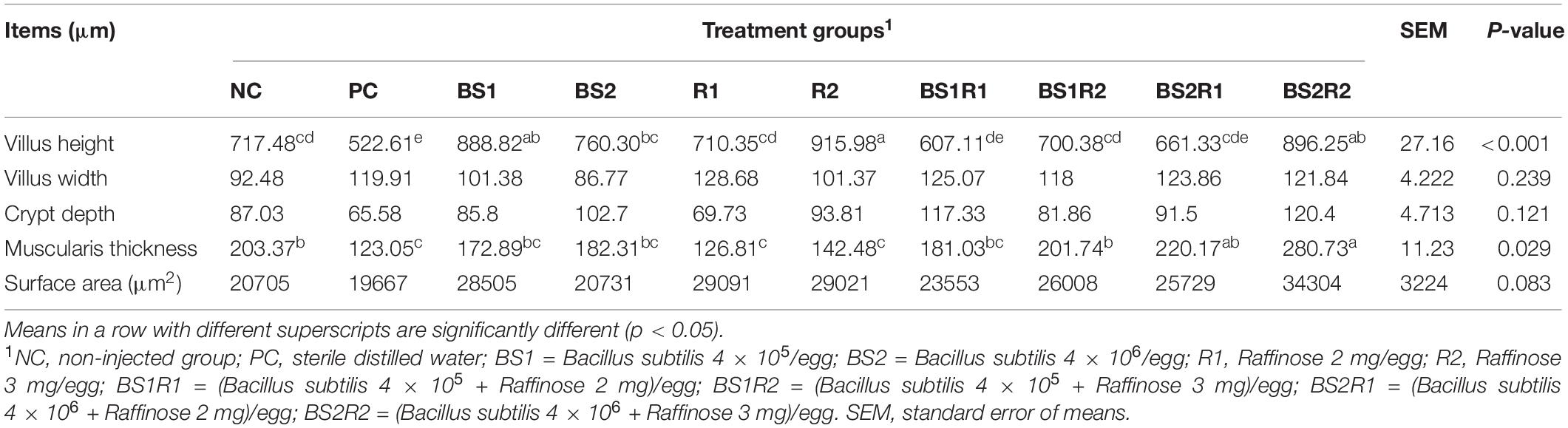
Table 6. Effect of in ovo inclusion of Bacillus subtilis, raffinose, and their synbiotics on ileal morphology of 21-day-old broilers.
Ileal Gene Expression
Relative Expression of Intestinal Function-Related Genes
The mRNA expression of mucin-2 and vascular endothelial growth factor genes in the ileum of broilers in different experimental groups is illustrated in Figures 2A,B. The mRNA expression of mucin-2 was elevated significantly (p < 0.05) in BS1R2, BS2R1, and BS2R2, and numerically in the rest of the groups compared to NC. Vascular endothelial growth factor (VEGF) expression was increased (p < 0.05) in BS2, R1, BS1R1, and BS1R2 compared to NC. BS1R2 recorded the highest mRNA expression of both genes.
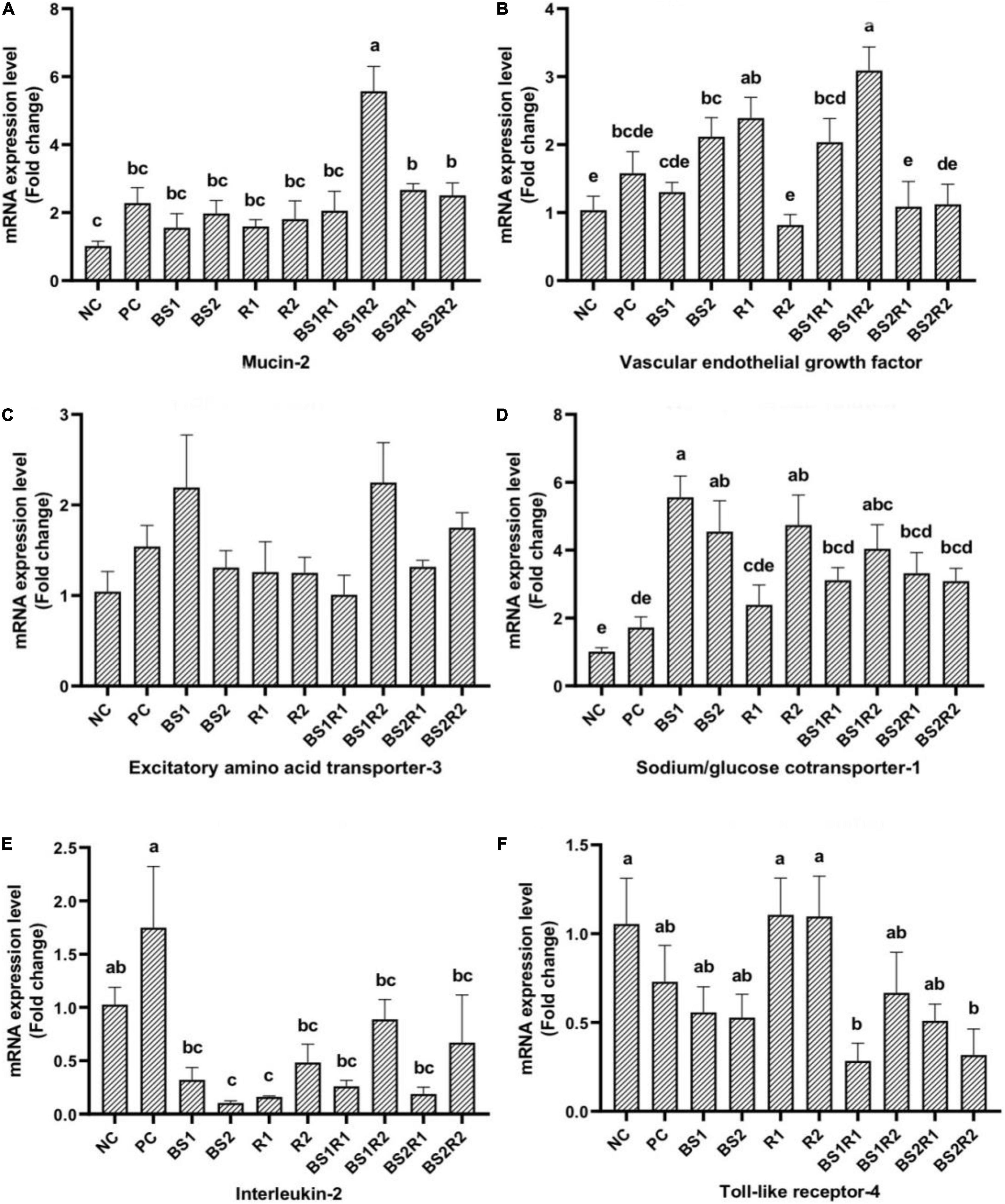
Figure 2. Effect of in ovo inclusion of Bacillus subtilis, raffinose, and their synbiotics on ileal gene expression of 21-day-old broilers. (A,B) Intestinal function-related, (C,D) nutrient transporter genes, and (E,F) immune-related genes. NC, non-injected group; PC, sterile distilled water; BS1 = Bacillus subtilis 4 × 105/egg; BS2 = Bacillus subtilis 4 × 106/egg; R1 = Raffinose 2 mg/egg; R2 = Raffinose 3 mg/egg; BS1R1 = (Bacillus subtilis 4 × 105 + Raffinose 2 mg)/egg; BS1R2 = (Bacillus subtilis 4 × 105 + Raffinose 3 mg)/egg; BS2R1 = (Bacillus subtilis 4 × 106 + Raffinose 2 mg)/egg; BS2R2 = (Bacillus subtilis 4 × 106 + Raffinose 3 mg)/egg. Data are presented as the mean values with their standard errors. Gene expression differences were evaluated using fold changes compared to the non-injected group. The significance was declared at p < 0.05. Bars with different letters represent significant differences among the different groups.
Relative Expression of Nutrient Transporter Genes
The mRNA expression of nutrients transportation-related genes (EAAT-3 and SGLT-1) in the ileum of broilers in ovo treated with B. subtilis, raffinose, and their synbiotics is shown in Figures 2C,D. The gene expression of EAAT-3 was not affected by in ovo supplements with a numerical increase in BS1 and BS1R2 groups compared to the control groups. SGLT-1 expression was upregulated (p < 0.05) in all treated birds except those of the R1 group compared to NC.
Relative Expression of Immune-Related Genes
The ileal mRNA expression of immune-related genes (interleukin (IL)-2 and toll-like receptor (TLR)-4) of broilers in ovo inoculated with B. subtilis, raffinose, and their combination is depicted in Figures 2E,F. The mRNA expressions of IL-2 and TLR-4 were downregulated (p < 0.05) in BS2 and R1 for IL-2 and BS1R1 and BS2R2 for TLR-4 and decreased numerically in the remaining groups compared to the control groups.
Discussion
Early colonization by beneficial bacteria or the inclusion of vital nutrients that encourage the growth of these beneficial microorganisms may play a major role in improving growth performance and reducing the occurrence of infections in chickens fed with no antibiotic growth promoters and enhancing the sustainability of broiler production (38). In a research review, Shehata et al. (7) discussed how in ovo administration of probiotics and prebiotics alters the growth performance, gut microbiota, and gut health of broiler chickens (7). In the present study, in ovo inoculation of B. subtilis, raffinose, and their combination improved BW, FI, and FCR compared to the untreated group. The improvement effects of these supplements could be due to their role in enhancing the health status of the intestine via increasing the population of beneficial bacteria, decreasing the population of harmful bacteria, regulating the expression of several ileal genes, and improving intestinal morphology (22, 26). B. subtilis is spore-forming bacteria that can tolerate harsh environmental conditions, colonize birds’ gut, and increase the activities of exogenous digestive enzymes, including lipase, protease, and amylase, which contribute to increasing nutrients digestion and absorption (18, 39). Tavaniello et al. (40) revealed that the positive impact of raffinose-injected in ovo on growth performance might be attributed to their ability to stimulate the early development of intestinal microbiota via increasing the population of beneficial bacteria such as lactobacilli and bifidobacteria and preventing colonization of pathogens. The proteome and cellular adhesion analyses showed improved adhesive properties of the beneficial bacteria (Lactobacillus acidophilus) grown on raffinose. This improvement was positively associated with differential protein abundance (elongation factor G and myosin cross-reactive antigen), indicating the positive interaction with mucin and host intestinal epithelial cells (41). Moreover, a previous study confirmed the prebiotic properties of raffinose family oligosaccharides via promoting the growth of Bifidobacterium bifidum and L. acidophilus (42). Additionally, administration of B. subtilis, prebiotics, or synbiotics caused an increase in the activity of pancreatic enzymes and thyroid hormones, which enhance the utilization of nutrients to maintain optimum growth performance of treated chickens (18, 43). Earlier investigations have demonstrated that Bacillus spp.-based probiotic is resistant to intestinal biochemical conditions in vitro and in vivo in chickens (44, 45). The ability of Bacillus-based probiotic to improve gut morphology and the gut microbiota structure may effectively contribute to increasing nutrient utilization and enhancing the immune responses, leading to improved growth performance. A previous study showed that Bacillus spp.-based probiotic produces various enzymes and antibacterial substances, which can enhance growth performance by improving nutrient digestibility, modulating gut viscosity, and enhancing intestinal integrity (46). In conformation with our results, Sen et al. (47) documented that BWG and FCR were increased linearly by increasing the dietary level of B. subtilis. Abdel-Moneim et al. (18) and Jeong and Kim (48) reported that the inclusion of B. subtilis spores improved weight gain of broilers. Additionally, Tavaniello et al. (40) and Bednarczyk et al. (49) reported that in ovo inoculation of raffinose improved the growth performance of Ross-308 chickens. Furthermore, chickens in ovo injected with prebiotics and synbiotics recorded heavier weights than the control groups (21). On the contrary, other studies reported non-significant effects of in ovo supplementation with B. subtilis and raffinose on the growth performance of broiler chickens (12, 16, 26, 50). These inconsistencies among studies might be attributed to various factors, including dosage of bioactive substances, sources, viability, administration route, environmental stressors, or sample size (12, 21). Intra-amniotic administration of B. subtilis fermentation extract (10 × 106 CFU) on day 18.5 of incubation did not affect the growth performance of Cobb 500 broiler chickens (12). Likewise, B. subtilis (107 CFU) injected on day 18 of incubation into the amnion had no significant effect on the growth performance of Cobb 500 broilers (16). In a previous study, inoculation of raffinose (1.5, 3.0, and 4.5 mg/egg) showed a numerical improvement in growth performance of broilers without any significant differences between the different groups (26). In ovo injection of 1.9 mg/egg raffinose (extracted from the seeds of Lupinus luteus L) did not affect the growth performance of broilers on the slaughter age (50).
The short-chain fatty acid (SCFA) and VFA are the by-products of cecal microbial fermentation and play vital roles in reducing gut pathogens, the functionality of the intestinal tract, and birds’ energy metabolism (51). In the present study, in ovo supplements elevated the levels of butyric, pentanoic, propionic, isobutyric, isovaleric, and acetic acids. These results are in agreement with those obtained recently by Oladokun et al. (12), who reported, for the first time, the effect of in ovo-delivered B. subtilis on concentrations of SCFA in broilers’ cecum. The authors found a consistent increase in VFA concentrations due to in ovo probiotics treatment. Moreover, dietary supplementation of soybean fermented with B. subtilis var. natto increased acetic acid and the total VFA concentrations (52). Similarly, administration of L. agilis and L. salivarius ssp. salicinius increased butyrate and propionate concentrations (51). Adding B. subtilis or B. licheniformis to chicken feed enhanced SCFA production via altering the microbiota structure in the chicken’s gut (53). SCFAs, in addition to being the primary source of energy for colonocytes, play a crucial role in maintaining health and modulating immunological and inflammatory responses (54). Nevertheless, further studies are needed to fully understand the effect of in ovo inoculation of probiotics and prebiotics due to the limited studies investigating this point (12).
The antimicrobial effects of B. subtilis, raffinose, and their synbiotics are well-presented in the current study. The inhibitory activity of these supplements is attributed to their ability to produce antibacterial and antifungal substances, including bacteriocins, bacteriocin-like substances, acetic acid, hydrogen peroxide, carbon dioxide, diacetyl, and lactic acid (7, 55). Intestinal immunity modulation (7), fat storage regulation (56), dietary fiber utilization (57), and competitive insularity of pathogenic bacteria (58) are other functional properties of the antimicrobial role of the in ovo supplements. The impacts of probiotics and prebiotics on modifying the intestinal microbiota composition by suppressing pathogen numbers and elevating counts of beneficial microorganisms were previously documented (14). Oh et al. (59) and Li et al. (8) reported the potential of B. subtilis to improve the richness of bacterial species, particularly from phylum Bacteroidetes. Our results are in agreement with those of Abdel-Moneim et al. (23), who noticed a reduction in total coliform and total bacterial counts and increased counts of bifidobacteria and lactic acid bacteria in ileal contents due to the in ovo injection of four bifidobacteria strains. Dietary supplementation of B. toyonensis and Bifidobacterium bifidum depressed the population of intestinal coliform, E. coli, and total bacterial count. Serafini et al. (60) also reported the inhibitory activity of probiotics on pathogenic bacteria such as E. coli and Cronobacter sakazakii. Furthermore, Pacifici et al. (61) and Stadnicka et al. (50) reported that in ovo administration of raffinose reduced pathogenic bacteria like Clostridia and E. coli and increased the population of beneficial bacteria like bifidobacteria and lactobacilli. Davani-Davari et al. (62) stated in their recent review on prebiotic that more study is needed to understand the impact of raffinose on gut flora fully. The crucial role of raffinose in enhancing the fermentation by Lactobacillus and Bifidobacteria and the production of SCFAs improves the competition with the pathogens. It improves the intestinal histomorphology and immune-related genes. This has been demonstrated before; for instance, treatment with beneficial bacteria reduced the virulence of pathogenic bacteria by promoting the formation of SCFAs in the gut (63). We propose that a similar mode of action may explain the results given here, with the resulting improvement in broiler chicken health and performance.
The gastrointestinal tract is the main interface between the birds and their nutritional environment, which allows it to play a pivotal role in the development and growth of hatchlings. Improving the intestinal microarchitecture of broiler chicks enhances feed utilization and nutrient uptake. The roles of probiotics and prebiotics in improving gut health and structure have been well-documented in previous studies (9, 50, 64–66). In the present study, ileal VH was improved in broilers in ovo treated with B. subtilis, raffinose, and their synbiotic. These results support the previous study of Berrocoso et al. (26) who noticed an improvement in the ileum mucosa development by in ovo injection of raffinose in the air sac. The same observations after in ovo inoculation of raffinose were reported in a recent study (50). Furthermore, Elbaz et al. (9), Abdel-Moneim et al. (23), and Abou-Kassem et al. (67) reported that administration of probiotics strains increased VH and reduced CD regardless of the route of administration. According to Abdel-Moneim et al. (18), the elevation in VH is accompanied by a tendency to reduce CD, higher activity of the digestive enzymes, and better absorption.
Additionally, longer lifespan and faster healing of enterocytes are associated with longer villi and shallower crypts, contributing to better nutrient utilization and improved growth performance (68, 69). Commensal bacteria can ferment raffinose, and the generated VFAs lower the pH of the intestines. Butyrate, one of the SCFAs produced during this fermentation, promotes the development of intestinal epithelial cells, which enhances nutrient uptake (70).
Maintaining gut integrity and mucin secretion is crucial to reducing pathogenic invasion and improving nutrient digestion and absorption. Mucins are mainly glycoproteins that lubricate and protect the intestinal epithelial surface from pathogens’ adhesion and invasion. Mucin production is encoded by the mucin-2 gene, which plays an important immunological role and is mediated by Th2 cytokines and T lymphocytes (71). Mucin dynamics are greatly influenced by intestinal microbiota and probiotic bacteria (7, 72). Mucin biosynthesis, turnover, and secretion are affected by intestinal microbiota and their metabolites, as they stimulate mucin gene expression via prostaglandin production (73). VEGF gene is essential in regulating tissue capillarity, improving their endogenous regeneration and vascularity, and lowering tissue fibrosis (74). The better expression of VEGF gene enhances blood supply to the intestine, leading to better nutrient absorption. Our results revealed upregulation in the expression of mucin-2 and VEGF genes in in ovo-treated birds. In line with our findings, Pender et al. (75) demonstrated that in ovo supplementation of probiotics elevates the expression of mucin-2 at 22 days post-hatch. Similarly, Majidi-Mosleh et al. (16) revealed that in ovo inoculation of B. subtilis into the amniotic fluid upregulated the intestinal mucin-2 gene expression in broilers at 3 days of age. Tsirtsikos et al. (76) reported a linear increase in mucus layer thickness with the addition of probiotics on day 12 and 42 of age.
Brush-border membrane genes are functional genes found on the brush border of enterocytes and used as biomarkers of intestinal capability for digestion and absorption and general tissue functionality (77, 78). SGLT1 is a glucose transporter, whereas EAAT3 is a glutamate and aspartate transporter, and both are located in the intestinal enterocytes. Prior to hatch, because of the low functional gene expression of brush-border membrane genes, such as SGLT1 and EAAT3, chicken embryos have a low ability to digest and absorb nutrients (79). Yalcin et al. (80) reported the upregulation of SGLT1 and PepT1 expression after hatch in the first 30 h. Therefore, in ovo and early nutrition are crucial for the intestinal development. Our results revealed non-significant alteration in EAAT3 expression, whereas SGLT1 expression was elevated in all treated birds. These results agreed with those of Pacifici et al. (61), who reported that intra-amniotic administration of raffinose upregulated SGLT1 expression significantly. Similarly, dietary supplementation of multi-strain probiotics upregulated the expression of SGLT1 in broilers at 14 days post-hatch (81). The upregulated expression of brush-border membrane functional genes indicates the higher function and development of the small intestine and better nutrient absorption, leading to improving the growth performance of birds.
Early establishment of beneficial microbiota and modulation of the immune system in poultry by in ovo administration of probiotics, prebiotics, or synbiotics contribute to increasing general health, well-being, and performance of birds. These supplements also contribute to eliminating the use of prophylactic drugs. The immunomodulating capabilities of these supplements are represented in several reports (9, 75, 81). TLR-2 is a pathogen recognition receptor expressed on infectious agents to recognize the molecular patterns associated with microbes and play a vital role in initiating and regulating the innate immune response. IL-2 plays a fundamental role in stimulating the proliferation of T and B lymphocytes and natural killer cells as a pro-inflammatory cytokine. In this study, we evaluated the expression of TLR-2 and IL-2 as indicators of the effect of in ovo supplements on innate and adaptive immune responses, respectively. Both TLR-4 and IL-2 were downregulated in the present study by in ovo-delivered supplements. The downregulation of the expression of these genes could be a result of the inhibitory impacts of in ovo supplements to pathogen colonization, which eliminates the need for TLR-4 and IL-2 induction. Correlating with our findings, Pender et al. (75) reported that in ovo injection of probiotics mixture of Lactobacilli, Enterococci, and Bifidobacteria strains suppressed the intestinal expression of TLR-2, TLR-4, IL-4, and IL-13 in broilers. Berrocoso et al. (26) revealed that in ovo injection of raffinose upregulated the expression of chB6 and CD3 in the gut of broiler chickens, whereas expressions of TLR-4, IL-1β, and IL-10 were not altered. The down-regulated expression of innate immunity-related genes might be attributed to the absence of pathogenic infections in treated groups.
Conclusion
In ovo inoculation of different levels of B. subtilis (4 × 105 and 4 × 106/egg), raffinose (2 and 3 mg), and their combinations on 300 h of incubation can improve the growth performance, intestinal morphology, cecal microbial population, and cecal VFA of broiler chickens, enhancing the sustainability of broiler production up to 21 days. In ovo supplementation plays an immunomodulatory role via modulating the expression of some ileal immune-related genes. Furthermore, our data showed that in ovo supplementation of B. subtilis, raffinose, and their combinations can upregulate the mRNA expression of intestinal function-related genes and nutrient transporter-related genes. In conclusion, in ovo supplementation with the B. subtilis, raffinose, or their combinations provided beneficial changes to growth performance and gut health of broiler chickens.
Data Availability Statement
The datasets presented in this study can be found in online repositories. The names of the repository/repositories and accession number(s) can be found in the article/supplementary material.
Ethics Statement
The animal study was reviewed and approved by the Central Animal Ethical Committee of Banaras Hindu University, Varanasi, India.
Author Contributions
ASh and VP designed the experiment. ASh conducted the animal experiment. ASh, TK, MAA, ES, ME-S, and ASa conducted the detection and analysis works. ASh and A-MA-M prepared the manuscript with input obtained from YA, AA, MMA, and RE. All authors participated in the discussion and editing of the manuscript.
Conflict of Interest
The authors declare that the research was conducted in the absence of any commercial or financial relationships that could be construed as a potential conflict of interest.
Publisher’s Note
All claims expressed in this article are solely those of the authors and do not necessarily represent those of their affiliated organizations, or those of the publisher, the editors and the reviewers. Any product that may be evaluated in this article, or claim that may be made by its manufacturer, is not guaranteed or endorsed by the publisher.
Acknowledgments
We thank and acknowledge the support of their respective universities and institutes. ASh was grateful for receiving a scholarship under the joint executive program between the Ministry of Higher Education of the Arab Republic of Egypt and the Indian Council for Cultural Relations (ICCR). ASh and VP are also grateful to Banaras Hindu University for partial financial assistance in the form of Institute of Imminence (IoE) Research Incentive Grant to carry out this research work.
References
1. Abdel-Moneim A-ME, Shehata AM, Selim DA, El-Saadony MT, Mesalam NM, Saleh AA. Spirulina platensis and biosynthesized selenium nanoparticles improve performance, antioxidant status, humoral immunity and dietary and ileal microbial populations of heat-stressed broilers. J Therm Biol. (2022) 104:103195. doi: 10.1016/j.jtherbio.2022.103195
2. Abdel-Moneim A-ME, El-Saadony MT, Shehata AM, Saad AM, Aldhumri SA, Ouda SM, et al. Antioxidant and antimicrobial activities of Spirulina platensis extracts and biogenic selenium nanoparticles against selected pathogenic bacteria and fungi. Saudi J Biol Sci. (2021) 29:1197–209. doi: 10.1016/j.sjbs.2021.09.046
3. Elnesr SS, Elwan HAM, El Sabry MI, Shehata AM, Alagawany M. Impact of chitosan on productive and physiological performance and gut health of poultry. Worlds Poult Sci J. (2022) 1–16. doi: 10.1080/00439339.2022.2041992
4. MacDonald JM, Wang S. Foregoing sub-therapeutic antibiotics: the impact on broiler grow-out operations. Appl Econ Perspect Policy. (2011) 33:79–98.
5. Abdel-Moneim AE, Shehata AM, Alzahrani SO, Shafi ME, Mesalam NM, Taha AE, et al. The role of polyphenols in poultry nutrition. J Anim Physiol Anim Nutr (Berl). (2020) 104:1851–66. doi: 10.1111/jpn.13455
6. Abd El-Hack ME, El-Saadony MT, Shehata AM, Arif M, Paswan VK, Batiha GE, et al. Approaches to prevent and control Campylobacter spp. colonization in broiler chickens: a review. Environ Sci Pollut Res. (2020) 28:4989–5004. doi: 10.1007/s11356-020-11747-3
7. Shehata AM, Paswan VK, Attia YA, Abdel-Moneim A-ME, Abougabal MS, Sharaf M, et al. Managing gut microbiota through in ovo nutrition influences early-life programming in broiler chickens. Animals. (2021) 11:3491. doi: 10.3390/ani11123491
8. Li Y, Xu Q, Huang Z, Lv L, Liu X, Yin C, et al. Effect of Bacillus subtilis CGMCC 1.1086 on the growth performance and intestinal microbiota of broilers. J Appl Microbiol. (2016) 120:195–204. doi: 10.1111/jam.12972
9. Elbaz AM, Ibrahim NS, Shehata AM, Mohamed NG, Abdel-Moneim A-ME. Impact of multi-strain probiotic, citric acid, garlic powder or their combinations on performance, ileal histomorphometry, microbial enumeration and humoral immunity of broiler chickens. Trop Anim Health Prod. (2021) 53:115. doi: 10.1007/s11250-021-02554-0
10. Lilburn MS, Loeffler S. Early intestinal growth and development in poultry. Poult Sci. (2015) 94:1569–76. doi: 10.3382/ps/pev104
11. van der Eijk JAJ, Rodenburg TB, de Vries H, Kjaer JB, Smidt H, Naguib M, et al. Early-life microbiota transplantation affects behavioural responses, serotonin and immune characteristics in chicken lines divergently selected on feather pecking. Sci Rep. (2020) 10:1–13. doi: 10.1038/s41598-020-59125-w
12. Oladokun S, Koehler A, MacIsaac J, Ibeagha-Awemu EM, Adewole DI. Bacillus subtilis delivery route: effect on growth performance, intestinal morphology, cecal short-chain fatty acid concentration, and cecal microbiota in broiler chickens. Poult Sci. (2021) 100:100809. doi: 10.1016/j.psj.2020.10.063
13. Zhang J, Cai K, Mishra R, Jha R. In ovo supplementation of chitooligosaccharide and chlorella polysaccharide affects cecal microbial community, metabolic pathways, and fermentation metabolites in broiler chickens. Poult Sci. (2020) 99:4776–85. doi: 10.1016/j.psj.2020.06.061
14. Mountzouris KC, Tsitrsikos P, Palamidi I, Arvaniti A, Mohnl M, Schatzmayr G, et al. Effects of probiotic inclusion levels in broiler nutrition on growth performance, nutrient digestibility, plasma immunoglobulins, and cecal microflora composition. Poult Sci. (2010) 89:58–67. doi: 10.3382/ps.2009-00308
15. Bilal M, Si W, Barbe F, Chevaux E, Sienkiewicz O, Zhao X. Effects of novel probiotic strains of Bacillus pumilus and Bacillus subtilis on production, gut health, and immunity of broiler chickens raised under suboptimal conditions. Poult Sci. (2021) 100:100871. doi: 10.1016/j.psj.2020.11.048
16. Majidi-Mosleh A, Sadeghi AA, Mousavi SN, Chamani M, Zarei A. Ileal MUC2 gene expression and microbial population, but not growth performance and immune response, are influenced by in ovo injection of probiotics in broiler chickens. Br Poult Sci. (2017) 58:40–5. doi: 10.1080/00071668.2016.1237766
17. Gauvry E, Mathot A-G, Couvert O, Leguérinel I, Coroller L. Effects of temperature, pH and water activity on the growth and the sporulation abilities of Bacillus subtilis BSB1. Int J Food Microbiol. (2021) 337:108915. doi: 10.1016/j.ijfoodmicro.2020.108915
18. Abdel-Moneim A-ME, Selim DA, Basuony HA, Sabic EM, Saleh AA, Ebeid TA. Effect of dietary supplementation of Bacillus subtilis spores on growth performance, oxidative status, and digestive enzyme activities in Japanese quail birds. Trop Anim Health Prod. (2020) 52:671–80. doi: 10.1007/s11250-019-02055-1
19. Park I, Zimmerman NP, Smith AH, Rehberger TG, Lillehoj E, Lillehoj HS. Dietary supplementation with Bacillus subtilis direct-fed microbials alters chicken intestinal metabolite levels. Front Vet Sci. (2020) 7:123. doi: 10.3389/fvets.2020.00123
20. Ruiz Sella SRB, Bueno T, de Oliveira AAB, Karp SG, Soccol CR. Bacillus subtilis natto as a potential probiotic in animal nutrition. Crit Rev Biotechnol. (2021) 41:355–69. doi: 10.1080/07388551.2020.1858019
21. Dankowiakowska A, Bogucka J, Sobolewska A, Tavaniello S, Maiorano G, Bednarczyk M. Effects of in ovo injection of prebiotics and synbiotics on the productive performance and microstructural features of the superficial pectoral muscle in broiler chickens. Poult Sci. (2019) 98:5157–65. doi: 10.3382/ps/pez202
22. El-Moneim AEEA, El-Wardany I, Abu-Taleb AM, Wakwak MM, Ebeid TA, Saleh AA. Assessment of in ovo administration of Bifidobacterium bifidum and Bifidobacterium longum on performance, ileal histomorphometry, blood hematological, and biochemical parameters of broilers. Probiotics Antimicrob Proteins. (2020) 12:439–50. doi: 10.1007/s12602-019-09549-2
23. Abdel-Moneim A-ME, Elbaz AM, Khidr RE-S, Badri FB. Effect of in ovo inoculation of Bifidobacterium spp. on growth performance, thyroid activity, ileum histomorphometry, and microbial enumeration of broilers. Probiotics Antimicrob Proteins. (2020) 12:873–82. doi: 10.1007/s12602-019-09613-x
24. Siwek M, Slawinska A, Stadnicka K, Bogucka J, Dunislawska A, Bednarczyk M. Prebiotics and synbiotics–in ovo delivery for improved lifespan condition in chicken. BMC Vet Res. (2018) 14:402. doi: 10.1186/s12917-018-1738-z
25. Gomes MJ, Kolba N, Agarwal N, Kim D, Eshel A, Koren O, et al. Modifications in the intestinal functionality, morphology and microbiome following intra-amniotic administration (Gallus gallus) of grape (Vitis vinifera) stilbenes (resveratrol and pterostilbene). Nutrients. (2021) 13:3247. doi: 10.3390/nu13093247
26. Berrocoso JD, Kida R, Singh AK, Kim YS, Jha R. Effect of in ovo injection of raffinose on growth performance and gut health parameters of broiler chicken. Poult Sci. (2017) 96:1573–80. doi: 10.3382/ps/pew430
28. Bancroft JD, Gamble M. Theory and practice of histological techniques. 5th. Edinburgh Churchill Livingstone Pub. (2002) 172:593–620.
29. Sakamoto K, Hirose H, Onizuka A, Hayashi M, Futamura N, Kawamura Y, et al. Quantitative study of changes in intestinal morphology and mucus gel on total parenteral nutrition in rats. J Surg Res. (2000) 94:99–106. doi: 10.1006/jsre.2000.5937
30. El-Hack A, Mohamed E, Alaidaroos BA, Farsi RM, Abou-Kassem DE, El-Saadony MT, et al. Impacts of supplementing broiler diets with biological curcumin, zinc nanoparticles and Bacillus licheniformis on growth, carcass traits, blood indices, meat quality and cecal microbial load. Animals. (2021) 11:1878. doi: 10.3390/ani11071878
31. Alagawany M, Madkour M, El-Saadony MT, Reda FM. Paenibacillus polymyxa (LM31) as a new feed additive: antioxidant and antimicrobial activity and its effects on growth, blood biochemistry, and intestinal bacterial populations of growing Japanese quail. Anim Feed Sci Technol. (2021) 276:114920.
32. Abou-Kassem DE, Mahrose KM, El-Samahy RA, Shafi ME, El-Saadony MT, Abd El-Hack ME, et al. Influences of dietary herbal blend and feed restriction on growth, carcass characteristics and gut microbiota of growing rabbits. Ital J Anim Sci. (2021) 20:896–910.
33. Saad AM, Mohamed AS, Ramadan MF. Storage and heat processing affect flavors of cucumber juice enriched with plant extracts. Int J Veg Sci. (2020) 27:277–87.
34. Khamis T, Fouad A, Abdallah S, Saeed A, Edress N, Hamed A. Breast milk MSCs transplantation attenuates male diabetic infertility via immunomodulatory mechanism in rats. Adv Anim Vet Sci. (2019) 7:145–53.
35. Khamis T, Abdelalim AF, Abdallah SH, Saeed AA, Edress NM, Arisha AH. Early intervention with breast milk mesenchymal stem cells attenuates the development of diabetic-induced testicular dysfunction via hypothalamic Kisspeptin/Kiss1r-GnRH/GnIH system in male rats. Biochim Biophys Acta (BBA) Mol Basis Dis. (2020) 1866:165577. doi: 10.1016/j.bbadis.2019.165577
36. Khamis T, Abdelalim AF, Saeed AA, Edress NM, Nafea A, Ebian HF, et al. Breast milk MSCs upregulated β-cells PDX1, Ngn3, and PCNA expression via remodeling ER stress/inflammatory/apoptotic signaling pathways in type 1 diabetic rats. Eur J Pharmacol. (2021) 905:174188.
37. Livak KJ, Schmittgen TD. Analysis of Relative Gene Expression Data Using Real-Time Quantitative PCR and the 2−ΔΔCT Method. Methods. (2001) 25:402–8.
38. Shehata AA, Yalçın S, Latorre JD, Basiouni S, Attia YA, Abd El-Wahab A, et al. Probiotics, Prebiotics, and Phytogenic Substances for Optimizing Gut Health in Poultry. Microorganisms. (2022) 10:395. doi: 10.3390/microorganisms10020395
39. Li W, Bai J, Li Y, Qin Y, Yu D. Effects of Bacillus subtilis on meat quality, nutrient digestibility and serum biochemical parameters of broilers. Chinese J Vet Sci. (2014) 34:1682–5.
40. Tavaniello S, Maiorano G, Stadnicka K, Mucci R, Bogucka J, Bednarczyk M. Prebiotics offered to broiler chicken exert positive effect on meat quality traits irrespective of delivery route. Poult Sci. (2018) 97:2979–87. doi: 10.3382/ps/pey149
41. Celebioglu HU, Ejby M, Majumder A, Købler C, Goh YJ, Thorsen K, et al. Differential proteome and cellular adhesion analyses of the probiotic bacterium Lactobacillus acidophilus NCFM grown on raffinose – an emerging prebiotic. Proteomics. (2016) 16:1361–75. doi: 10.1002/pmic.201500212
42. Bednarczyk M, Urbanowski M, Gulewicz P, Kasperczyk K, Maiorano G, Szwaczkowski T. Field and in vitro study on prebiotic effect of raffinose family oligosaccharides in chickens. Bull Vet Inst Pulawy. (2011) 55:465–9.
43. Pruszynska-Oszmalek E, Kolodziejski PA, Stadnicka K, Sassek M, Chalupka D, Kuston B, et al. In ovo injection of prebiotics and synbiotics affects the digestive potency of the pancreas in growing chickens. Poult Sci. (2015) 94:1909–16. doi: 10.3382/ps/pev162
44. Latorre JD, Hernandez-Velasco X, Kuttappan VA, Wolfenden RE, Vicente JL, Wolfenden AD, et al. Selection of Bacillus spp. for cellulase and xylanase production as direct-fed microbials to reduce digesta viscosity and Clostridium perfringens proliferation using an in vitro digestive model in different poultry diets. Front Vet Sci. (2015) 2:25. doi: 10.3389/fvets.2015.00025
45. Latorre JD, Hernandez-Velasco X, Wolfenden RE, Vicente JL, Wolfenden AD, Menconi A, et al. Evaluation and selection of Bacillus species based on enzyme production, antimicrobial activity, and biofilm synthesis as direct-fed microbial candidates for poultry. Front Vet Sci. (2016) 3:95. doi: 10.3389/fvets.2016.00095
46. Adhikari B, Hernandez-Patlan D, Solis-Cruz B, Kwon YM, Arreguin MA, Latorre JD, et al. Evaluation of the antimicrobial and anti-inflammatory properties of Bacillus-DFM (NorumTM) in broiler chickens infected with Salmonella enteritidis. Front Vet Sci. (2019) 6:282. doi: 10.3389/fvets.2019.00282
47. Sen S, Ingale SL, Kim YW, Kim JS, Kim KH, Lohakare JD, et al. Effect of supplementation of Bacillus subtilis LS 1-2 to broiler diets on growth performance, nutrient retention, caecal microbiology and small intestinal morphology. Res Vet Sci. (2012) 93:264–8. doi: 10.1016/j.rvsc.2011.05.021
48. Jeong JS, Kim IH. Effect of Bacillus subtilis C-3102 spores as a probiotic feed supplement on growth performance, noxious gas emission, and intestinal microflora in broilers. Poult Sci. (2014) 93:3097–103. doi: 10.3382/ps.2014-04086
49. Bednarczyk M, Stadnicka K, Kozłowska I, Abiuso C, Tavaniello S, Dankowiakowska A, et al. Influence of different prebiotics and mode of their administration on broiler chicken performance. Animal. (2016) 10:1271–9. doi: 10.1017/S1751731116000173
50. Stadnicka K, Bogucka J, Stanek M, Graczyk R, Krajewski K, Maiorano G, et al. Injection of raffinose family oligosaccharides at 12 days of egg incubation modulates the gut development and resistance to opportunistic pathogens in broiler chickens. Animals. (2020) 10:592. doi: 10.3390/ani10040592
51. Meimandipour A, Shuhaimi M, Soleimani AF, Azhar K, Hair-Bejo M, Kabeir BM, et al. Selected microbial groups and short-chain fatty acids profile in a simulated chicken cecum supplemented with two strains of Lactobacillus. Poult Sci. (2010) 89:470–6. doi: 10.3382/ps.2009-00495
52. Fujiwara K, Yamazaki M, Abe H, Nakashima K, Yakabe Y, Otsuka M, et al. Effect of Bacillus subtilis var. natto fermented soybean on growth performance, microbial activity in the caeca and cytokine gene expression of domestic meat type chickens. J Poult Sci. (2009) 46:116–22.
53. Xu Y, Yu Y, Shen Y, Li Q, Lan J, Wu Y, et al. Effects of Bacillus subtilis and Bacillus licheniformis on growth performance, immunity, short chain fatty acid production, antioxidant capacity, and cecal microflora in broilers. Poult Sci. (2021) 100:101358. doi: 10.1016/j.psj.2021.101358
54. Yosi F, Sharma S, Sener-Aydemir A, Koger S, Baskara AP, Metzler-Zebeli BU. Short-chain fatty acids promote jejunal barrier function and caecal muscle contractibility in laying hens ex vivo. Br Poult Sci. (2021) 1–8. doi: 10.1080/00071668.2021.2008312
55. Mandal SM, Silva ON, Franco OL. Recombinant probiotics with antimicrobial peptides: a dual strategy to improve immune response in immunocompromised patients. Drug Discov Today. (2014) 19:1045–50. doi: 10.1016/j.drudis.2014.05.019
56. DiBaise JK, Frank DN, Mathur R. Impact of the gut microbiota on the development of obesity: current concepts. Am J Gastroenterol Suppl. (2012) 1:22.
57. Slavin J. Fiber and prebiotics: mechanisms and health benefits. Nutrients. (2013) 5:1417–35. doi: 10.3390/nu5041417
58. Yu Q, Wang Z, Yang Q. Ability of Lactobacillus to inhibit enteric pathogenic bacteria adhesion on Caco-2 cells. World J Microbiol Biotechnol. (2011) 27:881–6.
59. Oh JK, Pajarillo EAB, Chae JP, Kim IH, Yang DS, Kang D-K. Effects of Bacillus subtilis CSL2 on the composition and functional diversity of the faecal microbiota of broiler chickens challenged with Salmonella gallinarum. J Anim Sci Biotechnol. (2017) 8:1–9. doi: 10.1186/s40104-016-0130-8
60. Serafini F, Strati F, Ruas-Madiedo P, Turroni F, Foroni E, Duranti S, et al. Evaluation of adhesion properties and antibacterial activities of the infant gut commensal Bifidobacterium bifidum PRL2010. Anaerobe. (2013) 21:9–17. doi: 10.1016/j.anaerobe.2013.03.003
61. Pacifici S, Song J, Zhang C, Wang Q, Glahn RP, Kolba N, et al. Intra amniotic administration of raffinose and stachyose affects the intestinal brush border functionality and alters gut microflora populations. Nutrients. (2017) 9:304. doi: 10.3390/nu9030304
62. Davani-Davari D, Negahdaripour M, Karimzadeh I, Seifan M, Mohkam M, Masoumi SJ, et al. Prebiotics: definition, types, sources, mechanisms, and clinical applications. Foods. (2019) 8:92. doi: 10.3390/foods8030092
63. Arreguin-Nava MA, Graham BD, Adhikari B, Agnello M, Selby CM, Hernandez-Velasco X, et al. Evaluation of in ovo Bacillus spp. based probiotic administration on horizontal transmission of virulent Escherichia coli in neonatal broiler chickens. Poult Sci. (2019) 98:6483–91. doi: 10.3382/ps/pez544
64. Abdel-Moneim AE, Shehata AM, Khidr RE, Paswan VK, Ibrahim NS, El-Ghoul AA, et al. Nutritional manipulation to combat heat stress in poultry – a comprehensive review. J Therm Biol. (2021) 98:102915. doi: 10.1016/j.jtherbio.2021.102915
65. Abdel-Moneim AE, Shehata AM, Mohamed NG, Elbaz AM, Ibrahim NS. Synergistic effect of Spirulina platensis and selenium nanoparticles on growth performance, serum metabolites, immune responses, and antioxidant capacity of heat-stressed broiler chickens. Biol Trace Elem Res. (2021) 200:768–79. doi: 10.1007/s12011-021-02662-w
66. Saleh AA, Shukry M, Farrag F, Soliman MM, Abdel-Moneim A-ME. Effect of feeding wet feed or wet feed fermented by Bacillus licheniformis on growth performance, histopathology and growth and lipid metabolism marker genes in broiler chickens. Animals. (2021) 11:83.
67. Abou-Kassem DE, Elsadek MF, Abdel-Moneim AE, Mahgoub SA, Elaraby GM, Taha AE, et al. Growth, carcass characteristics, meat quality, and microbial aspects of growing quail fed diets enriched with two different types of probiotics (Bacillus toyonensis and Bifidobacterium bifidum). Poult Sci. (2021) 100:84–93. doi: 10.1016/j.psj.2020.04.019
68. Rebolé A, Ortiz LT, Rodríguez M, Alzueta C, Treviño J, Velasco S. Effects of inulin and enzyme complex, individually or in combination, on growth performance, intestinal microflora, cecal fermentation characteristics, and jejunal histomorphology in broiler chickens fed a wheat-and barley-based diet. Poult Sci. (2010) 89:276–86. doi: 10.3382/ps.2009-00336
69. Awad WA, Ghareeb K, Abdel-Raheem S, Böhm J. Effects of dietary inclusion of probiotic and synbiotic on growth performance, organ weights, and intestinal histomorphology of broiler chickens. Poult Sci. (2009) 88:49–56. doi: 10.3382/ps.2008-00244
70. Bogusławska-Tryk M, Ziółkowska E, Sławińska A, Siwek M, Bogucka J. Modulation of intestinal histology by probiotics, prebiotics and synbiotics delivered in ovo in distinct chicken genotypes. Animals. (2021) 11:3293. doi: 10.3390/ani11113293
71. Beum PV, Basma H, Bastola DR, Cheng P-W. Mucin biosynthesis: upregulation of core 2 β1, 6 N-acetylglucosaminyltransferase by retinoic acid and Th2 cytokines in a human airway epithelial cell line. Am J Physiol Cell Mol Physiol. (2005) 288:L116–24. doi: 10.1152/ajplung.00370.2003
72. Abd El-Hack ME, El-Saadony MT, Shafi ME, Qattan SYA, Batiha GE, Khafaga AF, et al. Probiotics in poultry feed: a comprehensive review. J Anim Physiol Anim Nutr (Berl). (2020) 104:1835–50.
73. Shibolet O, Karmeli F, Eliakim R, Swennen E, Brigidi P, Gionchetti P, et al. Variable response to probiotics in two models of experimental colitis in rats. Inflamm Bowel Dis. (2002) 8:399–406. doi: 10.1097/00054725-200211000-00004
74. Hotowy A, Sawosz E, Pineda L, Sawosz F, Grodzik M, Chwalibog A. Silver nanoparticles administered to chicken affect VEGFA and FGF2 gene expression in breast muscle and heart. Nanoscale Res Lett. (2012) 7:1–8. doi: 10.1186/1556-276X-7-418
75. Pender CM, Kim S, Potter TD, Ritzi MM, Young M, Dalloul RA. In ovo supplementation of probiotics and its effects on performance and immune-related gene expression in broiler chicks. Poult Sci. (2017) 96:1052–62. doi: 10.3382/ps/pew381
76. Tsirtsikos P, Fegeros K, Balaskas C, Kominakis A, Mountzouris KC. Dietary probiotic inclusion level modulates intestinal mucin composition and mucosal morphology in broilers. Poult Sci. (2012) 91:1860–8. doi: 10.3382/ps.2011-02005
77. Reed S, Neuman H, Moscovich S, Glahn RP, Koren O, Tako E. Chronic zinc deficiency alters chick gut microbiota composition and function. Nutrients. (2015) 7:9768–84. doi: 10.3390/nu7125497
78. Hou T, Tako E. The in ovo feeding administration (Gallus gallus)—an emerging in vivo approach to assess bioactive compounds with potential nutritional benefits. Nutrients. (2018) 10:418. doi: 10.3390/nu10040418
79. Uni Z, Ferket PR. Enhancement of Development of Oviparous Species by in ovo Feeding. U.S. Patent No. 6,592,878. Washington, DC: U.S. Patent and Trademark Office (2003).
80. Yalcin S, Gursel I, Bilgen G, Izzetoglu GT, Horuluoglu BH, Gucluer G. Egg storage duration and hatch window affect gene expression of nutrient transporters and intestine morphological parameters of early hatched broiler chicks. Animal. (2016) 10:805–11. doi: 10.1017/S175173111500261X
Keywords: bioactive compounds, in ovo feeding, gut microbiota, volatile fatty acid, ileal architecture, gene expression, sustainability, broiler chickens
Citation: Shehata AM, Paswan VK, Attia YA, Abougabal MS, Khamis T, Alqosaibi AI, Alnamshan MM, Elmazoudy R, Abaza MA, Salama EAA, El-Saadony MT, Saad AM and Abdel-Moneim A-ME (2022) In ovo Inoculation of Bacillus subtilis and Raffinose Affects Growth Performance, Cecal Microbiota, Volatile Fatty Acid, Ileal Morphology and Gene Expression, and Sustainability of Broiler Chickens (Gallus gallus). Front. Nutr. 9:903847. doi: 10.3389/fnut.2022.903847
Received: 24 March 2022; Accepted: 04 May 2022;
Published: 31 May 2022.
Edited by:
J. Fernando Ayala-Zavala, Centro de Investigación en Alimentación y Desarrollo, Consejo Nacional de Ciencia y Tecnología (CONACYT), MexicoReviewed by:
Henny Akit, Putra Malaysia University, MalaysiaCristóbal González-Pérez, University of Sonora, Mexico
Yessica Enciso-Martínez, University of Sonora, Mexico
Copyright © 2022 Shehata, Paswan, Attia, Abougabal, Khamis, Alqosaibi, Alnamshan, Elmazoudy, Abaza, Salama, El-Saadony, Saad and Abdel-Moneim. This is an open-access article distributed under the terms of the Creative Commons Attribution License (CC BY). The use, distribution or reproduction in other forums is permitted, provided the original author(s) and the copyright owner(s) are credited and that the original publication in this journal is cited, in accordance with accepted academic practice. No use, distribution or reproduction is permitted which does not comply with these terms.
*Correspondence: Abdelrazeq M. Shehata, abdelrazeq@azhar.edu.eg; Youssef A. Attia, yaattia@gmail.com