- 1Department of Sports Medicine and Psychology, Lobachevsky University, Nizhny Novgorod, Russia
- 2Department of Physiology and Anatomy, Lobachevsky University, Nizhny Novgorod, Russia
- 3Department of Biomedical Sciences, University of Padua, Padua, Italy
Purpose: This study aimed to examine whether oral royal jelly (RJ) and coenzyme Q10 (CoQ10) co-supplementation could improve high-intensity interval exercise (HIIE) performance in runners, reducing exercise-induced lactic acidosis and decreasing elevated sympathetic tone following exercise.
Methods: Thirty regional-level runners (age: 19 ± 1 years; height: 173 ± 2 cm; body mass: 68.9 ± 2 kg; body mass index: 23.1 ± 1 kg/m2) were randomly allocated to receive either 400 mg of RJ and 60 mg of CoQ10 (RJQ) or matching placebo (PLA) once daily for 10 days. Exercise performance expressed as time taken to complete HIIE was evaluated at baseline, and then reassessed at day 10 of intervention. HIIE protocol applied to the runners included three repetitions of 100 m distance at maximum possible speed interspersed with 45 s of recovery periods. Indices of heart rate variability and blood lactate concentration were also measured before and immediately after HIIE in each group.
Results: HIIE performance significantly improved in RJQ group (p = 0.005) compared to PLA group. Blood lactate levels and sympathetic influence on the heart were significantly lower both before and after the HIIE in athletes who received RJQ (p < 0.05) compared to PLA. Regression analysis showed that oral RJQ administration for 10 days was significantly associated with reductions in HIIE-induced increases in blood lactate concentration and enhanced cardiac parasympathetic modulation following exercise compared to PLA. Principal component analysis revealed that runners treated with RJQ are grouped by the first two principal components into a separate cluster compared to PLA. Correlation analysis demonstrated that the improvements in runners’ HIIE performance were due in significant part to RJQ-induced reduction of increment in blood lactate levels in response to exercise in combination with a more rapid shift in autonomic activity toward increased parasympathetic control early at post-exercise.
Conclusion: These findings suggest that RJQ supplementation for 10 days is potentially effective for enhancing HIIE performance and alleviating adverse effects of increased intramuscular acidity and prolonged sympathetic dominance following intense exercise.
Introduction
It is crucial to take into consideration any athlete’s fatigue state and/or performance responses to the training load. Among the methods available for diagnosing non-functional overreaching or overtraining syndrome, the heart rate variability (HRV) is widely used because its alterations depend largely on changes in cardiac autonomic regulation which continuously attempts to adapt cardiovascular function to exercise and training (1–4). The decrease in autonomic nervous system (ANS) activity during intensive training is correlated with the loss in exercise performance, and the rebound in ANS activity with its increase (5). In addition, scientific data have shown that ANS recovery is more rapid in highly trained than in trained subjects following high-intensity exercise (6).
Blood lactate concentration is also one of the most often measured parameters during performance testing of athletes (7). Although lactate is now recognized as an important metabolic intermediate and signaling molecule mediating exercise adaptations and interorgan communication (8–13), its accumulation in muscle and blood at higher levels can lead to acidosis and negatively affect force and power generation, resulting in a reduction in sports performance due to inability to maintain the exercise intensity needed for ultimate success (14–16).
The two major approaches used to enhance fatigue resistance and, thus, sports performance are regular training and various nutritional interventions, and the interactions between them have been recognized (17, 18). Royal jelly (RJ), which is secreted by the cephalic glands of honey bees (Apis mellifera L.) and used as food for young larvae and queen bees, contains many nutrients including vitamins, minerals, fatty acids, carbohydrates, and proteins/amino acids (19). Some ingredients contained in RJ have been suggested to have a potential for increasing ANS activity and energy metabolism in skeletal muscles (20–22). In addition to RJ supplementation, our line of evidence indicates that coenzyme Q10 (CoQ10), which in turn plays a key role in supplying energy to all cells and taking part in redox reactions within the electron transport chain at the mitochondrial level (23–25), may yield synergies toward fatigue resistance and the improvement of athletic performance (26).
Our aim was to conduct a 10-day, randomized, double-blind, placebo-controlled trial to examine whether oral RJ and CoQ10 co-supplementation could improve high-intensity interval exercise (HIIE) performance in runners, reducing exercise-induced lactic acidosis and decreasing elevated sympathetic tone following exercise. We hypothesize that RJ plus CoQ10 (RJQ) supplementation is able to enhance HIIE performance, which can be associated with RJQ-induced neurohumoral and metabolic changes, in particular with a more rapid shift from parasympathetic to sympathetic going from rest to exercise and vice versa, as well as with reducing the increment in blood lactate concentration in response to exercise.
Materials and Methods
Subjects
Thirty male athletes were recruited from a single sports training center (Nizhny Novgorod, Russia) by advertising directly to coaches. Subjects were included in the study if they had at least sport category «Candidate Master of Sports» or sport rank «Master of Sports of Russia» in accordance with the Unified Russian Sports Classification System. Exclusion criteria were the use of any antioxidant supplements, including RJ or/and CoQ10, and/or chronic use of any medication. Two weeks before intervention, the above-mentioned criteria were excluded by coaches’ interview, and all subjects were included in the study. Before any procedures, informed written consent was obtained from each of the study participants. At the end of the first screening visit, subjects were randomly assigned to the groups: RJ plus CoQ10 (RJQ; n = 15; age: 19.40 ± 1.50 years; height: 172.13 ± 2.07 cm; body mass: 68.53 ± 1.96 kg; body mass index: 23.15 ± 0.93 kg/m2) or placebo (PLA; n = 15; age: 19.13 ± 1.06 years; height: 173.00 ± 2.20 cm; body mass: 69.20 ± 2.01 kg; body mass index: 23.13 ± 1.02 kg/m2). The study protocol was approved by the Bioethics Committee of Lobachevsky University (approval number: 43). The study was conducted in accordance with the guidelines laid down in the Declaration of Helsinki (27).
Study Design
This study is a 10-day, randomized, double-blind, placebo-controlled trial. After signing the informed consent and 2 weeks before intervention, the participants were referred to the Integral Human Health Laboratory of the Faculty of Physical Education and Sport of Lobachevsky University. During the visit, the athletes underwent a medical screening to ensure eligibility for the study and food interview to gather information on the subjects’ dietary habits.
Thirty runners were randomized in a double-blind fashion in a 1:1 ratio and assigned to receive either RJQ (n = 15) or PLA (n = 15) once daily for 10 days. To allocate subjects, we applied a computer-generated list of random numbers. Participants were instructed to avoid taking any additional supplements containing RJ and/or CoQ10, as well as any antioxidant supplements during the study. Participants were also instructed to have breakfast 1 h before the training sessions and refrain from consuming alcohol and caffeinated beverages for at least 24 h before exercise testing. Adherence to study medications was assessed daily by a qualified dietician with a face-to-face interview. Athletes randomized to the RJQ group received supplementation daily consisting of active ingredients (60 mg of CoQ10 and 400 mg of RJ) and excipients (10 g of honey). Athletes randomized to PLA group received supplementation daily without active ingredients and containing only excipients (10 g of honey). Both active and placebo supplements were identical in size, color, opacity, shape, presentation, and packaging. The substances were taken sublingually in the morning. CoQ10 was manufactured and donated by OJSC «Kstovo Experimental Pilot Protein-Vitamin Concentrates Plant» (Kstovo, Russia). RJ and honey were manufactured and donated by Federal Beekeeping Research Center (Sochi, Russia). Participants were asked to consume RJQ supplementation 60 min before the training session. This is a sufficient time for RJ-induced activation of 5′-AMP-activated protein kinase (AMPK) and, perhaps, for total coenzyme Q10 level in skeletal muscle cells to become elevated to get an observable ergogenic effect (21, 28–30). One day before the start of the intervention, runners underwent their specific HIIE on a 400 m outdoor track of the stadium. HIIE protocol applied to the runners included three repetitions of 100 m distance at maximum possible speed interspersed with 45 s of recovery periods. Exercise testing was performed in the morning. Subjects were examined before and immediately after HIIE for HRV measures and blood sample collection, followed by determination of lactate levels. Athletes kept a typical training routine, which was the same for all participants. Runners took part in the study in a period away from the competition phase: workouts had a daily schedule and training sessions included exercises mainly aimed at increasing speed endurance. After 10 days, subjects repeated the HIIE and, simultaneously, HRV indices and blood lactate levels were reassessed before and immediately after the exercise.
Investigational Substances
Exogenous CoQ10 is a microbiological synthesis product synthesized at OJSC «Kstovo Experimental Pilot Protein-Vitamin Concentrates Plant» according to the technology developed at the Research Institute «Sintezbelok» of the Russian Academy of Sciences and Research and Production Association «Vitaminy». According to TU—op. 64-12-125-90, CoQ10 is a yellow-orange crystalline powder with the following properties: molecular formula—C59H90O4, molecular weight—863.36 g/mol, and melting point—47.5–49.0°C.
Native fresh royal jelly was extracted in the Federal Beekeeping Research Center. Organoleptic and physicochemical properties of royal jelly complied with the requirements of interstate standard approved by the Interstate Council for Standardization, Metrology and Certification. In accordance with the above-mentioned standard, native fresh royal jelly contained a minimum of 5% decenoic acids (in royal jelly containing no water) where the largest proportion of these acids belonged to 10-hydroxy-2-decenoic acid. Honey was also extracted in the Federal Beekeeping Research Center.
The tested combination is a native fresh royal jelly plus coenzyme Q10 dissolved in a carrier lipid. To determine doses and timing, we were guided by recommended dosages and timing of interventions when using approved biologically active food supplements, in particular «Apitonus» (10–20 g per day) and «Kudesan» (1–2 ml per day). «Apitonus» is a combination of native fresh royal jelly and honey mixed in a 2:100 ratio, respectively. «Kudesan» is a water solution of solubilized coenzyme Q10 (30 mg/mL).
Measurement of Exercise Performance
The HIIE protocol, applied to the runners, consisted of three repetitions of 100 m distance at maximum possible speed, interspersed with 45 s of recovery time. The aim of the test was to complete each set in the shortest possible time. Results were determined by the time taken to complete each set with the subsequent calculation of mean. Timing was recorded using a stopwatch from the start signal until the runner crossed the finish line.
Analysis of Heart Rate Variability
To test general autonomic reactivity using a standard method, heart rate and HRV changes of all participants were measured before and immediately after the exercise. All subjects were instructed to refrain from ingesting beverages containing caffeine or alcohol for 24 h prior to the testing. ECG signal was recorded by a hardware-software complex «Poly-Spectrum-Rhythm» (“Neurosoft,” Ivanovo, Russia) for 5 min in the supine position in a quiet room with normal lighting and room temperature. The participants were asked to remain silent without deep breathing, speaking, or moving at all. The data collection was always performed by the same researcher, who maintained the same conditions for, and gave identical instructions to, all the participants. The signal processing was performed using Neurosoft® HRV analysis software with time and frequency domain analysis. The methodological criteria that were applied were those proposed by the Task Force of the European Society of Cardiology and the North American Society of Pacing and Electrophysiology (31).
In the HRV time domain, the mean RR interval, the SD of NN intervals, the square root of the mean squared difference of successive RR intervals (RMSSD), and the percentage of consecutive RRs that differed by more than 50 ms each were obtained.
A Fast Fourier Transform of the RR signals was used for the HRV frequency domain analysis. The spectral response provided by the system was broken down into three bands: high frequency (0.15–0.4 Hz), low frequency (0.04–0.15 Hz), and very low frequency (0.003–0.04 Hz).
Baevsky’s Stress Index (SI) (32) was calculated according to the formula:
where the mode (Mo) is the most frequent RR interval expressed in seconds. The amplitude of mode (AMo) was calculated, using a 50 ms bin width, as the number of the RR intervals in the bin containing the Mo, expressed as a percentage of the total number of intervals measured. The variability is reflected in MxDMn as the difference between longest (Mx) and shortest (Mn) RR interval values, expressed in seconds. The SI is expressed as s–2.
Measurement of Blood Lactate Concentration
Finger-prick blood samples were collected by a qualified phlebotomist before (at rest) and 7–8 min after the exercise. Lactate concentration was measured by a hand-held point-of-care device “StatStrip®Xpress™” (Nova Biomedical, Waltham, MA, United States) that utilizes 0.7 μL of blood and electrochemical test strips.
Statistical Analysis
A descriptive statistical analysis was carried out for all study variables. Data are expressed as mean ± standard deviation (SD). The sample was checked for normal distribution using the Shapiro–Wilk test. Since all data were normally distributed, comparisons were made using paired Student’s t-test for within-group comparison analysis and using Student’s t-test for independent data for between-group comparison analysis. According to the pre-specified analysis plan, treatment effect was assessed as the mean change in each parameter from pre-exercise to post-exercise for each intervention group. Statistical comparison between the treatment effects on exercise-induced changes in HRV indices and blood lactate concentration was made by regression analysis using a following model:
where Y—response variable; Dummy—dummy variable between 0 and 1 depending on the supplement (0 = PLA, 1 = RJQ); β0 and β1—estimated regression parameters; and ε—modeling error.
The regression model made it possible to determine whether the mean changes in each parameter between the RJQ group and PLA group under HIIE conditions were statistically significant. In addition, principal component analysis (PCA) was applied. PCA made it possible to take into account the variances of all RJQ-dependent variables in the subsequent data analysis in order to establish a causal relationship between formed factor, consisting of HRV indices and blood lactate, and HIIE performance, since PCA is based on the transition from the space of correlated variables to the space of orthogonal variables (principal components). Thus, the use of PCA, on the one hand, solved the arisen problem of multicollinearity of the RJQ-dependent variables. Statistical relationships between exercise-induced changes in HRV indices and blood lactate concentration at day 10 of intervention were evaluated using Pearson’s correlation coefficient (R). On the other hand, PCA provided an opportunity to deviate from direct modeling of HIIE performance, since the inclusion in model specification exclusively investigated parameters and ignoring the rest of the regressors would lead to correlation between model error and independent variable (endogeneity problem). In this regard, we attempted to indirectly explain variation in the completion time by means of explaining variation in changes of the RJQ-dependent variables. Since the set of the analyzed parameters has different units of measurement, it is logical to assume that principal component 1 (PC1) will include variables with the highest sample variance as with the highest weighting coefficients. Thus, in order for the PCA to be applicable, the RJQ-dependent variables were normalized using the following formula:
where aij−j-th observation of the i-th variable; mean of the i-th variable; and SEM(ai)−standard error of the mean of the i-th variable.
For all analyses, a p-value < 0.05 was considered statistically significant. All statistical analyses were performed using RStudio, version 1.3.1093 for macOS (RStudio, PBC).
Results
All the recruited subjects successfully completed the study, and no side effect from RJQ intake was reported. Measurements of HIIE performance (i.e., completion time) in runners at baseline and day 10 of intervention are presented in Figure 1.
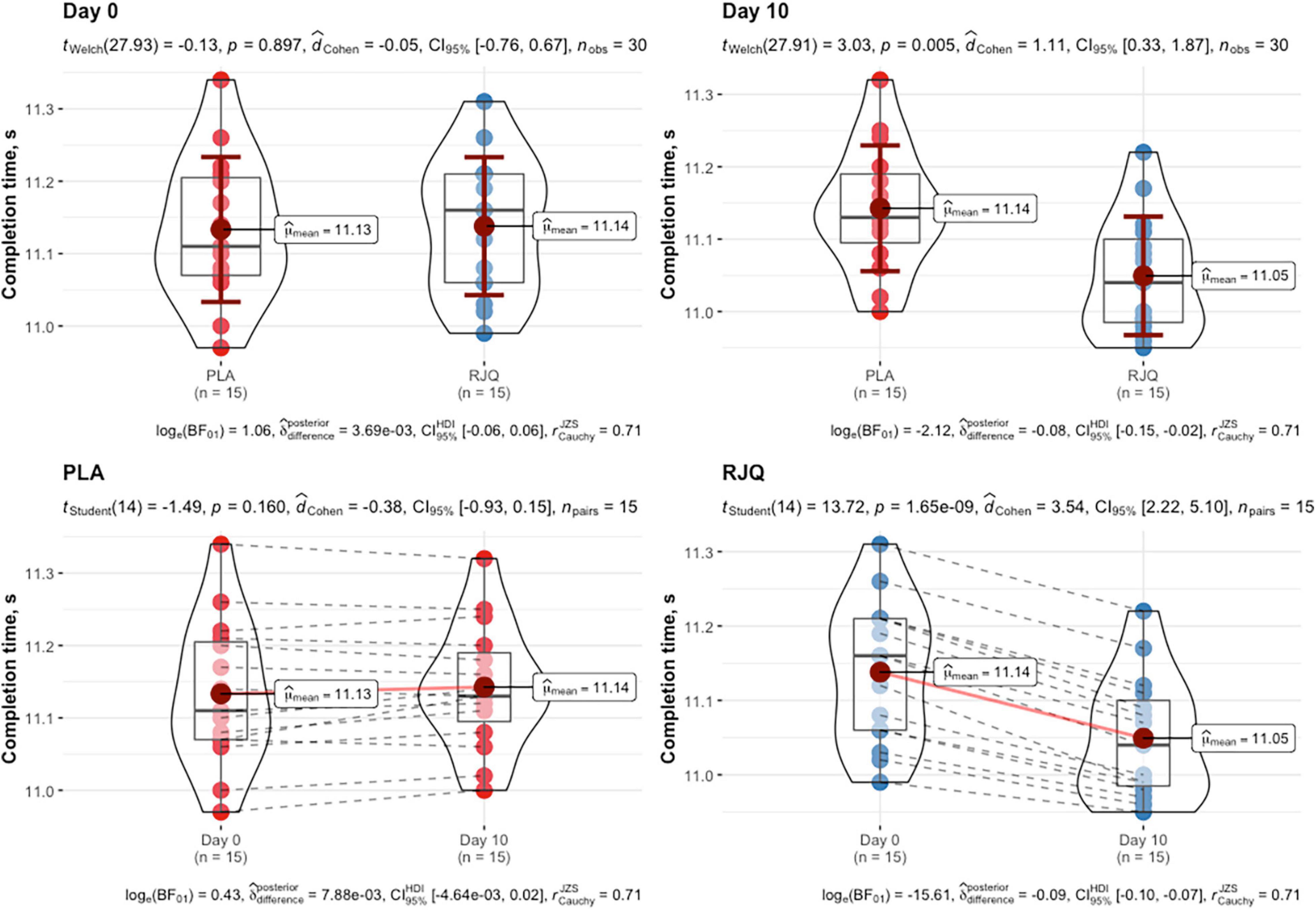
Figure 1. HIIE performance expressed as time to complete exercise at baseline and day 10 of intervention between randomization arms. Data are given as means ± SD and compared by paired Student’s t-test for within-group comparison analysis and by Student’s t-test for independent data for between-group comparison analysis. n = 15 per group.
The RJQ supplementation significantly decreased the time taken by runners to complete HIIE, compared to PLA (RJQ group: from 11.14 ± 0.10 s to 11.05 ± 0.08 s; PLA group: from 11.13 ± 0.10 s to 11.14 ± 0.09 s). At day 10 of the intervention, the sympathetic parameter SI and blood lactate levels were significantly lower both before and after the HIIE in RJQ group compared to PLA group (Table 1).
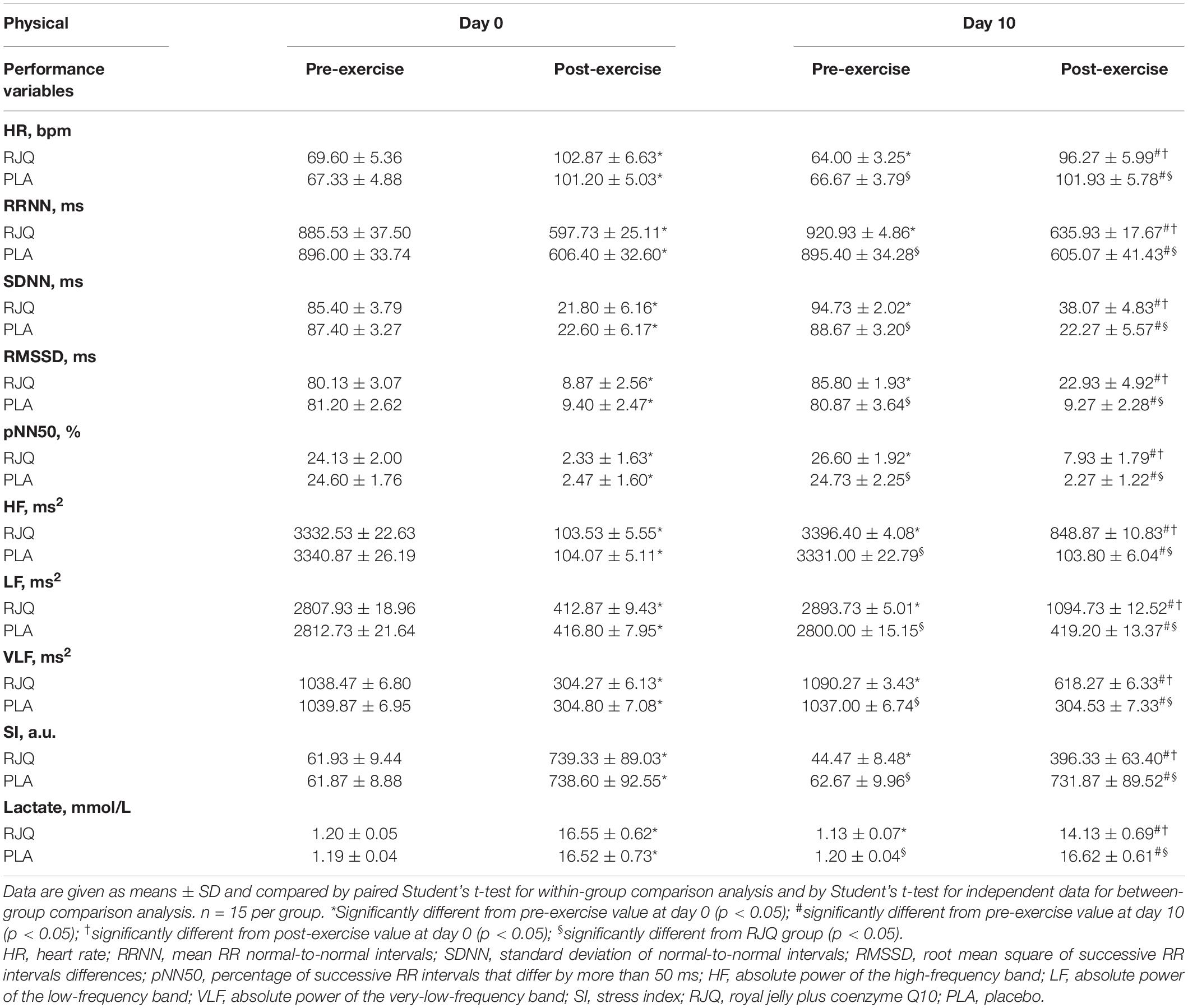
Table 1. Heart rate variability indices and blood lactate concentration before and immediately after high-intensity interval exercise at baseline and day 10 of intervention between randomization arms.
The RJQ supplementation resulted in an increase in RRNN and decrease in heart rate, respectively. The parasympathetic parameters such as SDNN, RMSSD, pNN50, and HF power were significantly higher both at rest and following HIIE in athletes treated for 10 days with RJQ compared to PLA, consistent with an increase in parasympathetic reactivity at post-exercise. In relation to LF power and VLF power, participants who received RJQ also had greater values compared to PLA both at pre-exercise and at post-exercise.
To assess the effect of 10-day RJQ supplementation on HIIE performance (based on the observed completion time) through a combination of decreasing regulation strain (based on the observed HRV measures) and reducing lactic acidosis under HIIE conditions, further correlation and regression analysis using principal components were applied.
A strong positive correlation between the mean changes in HRV indices and blood lactate concentration under HIIE conditions in both intervention groups was observed (Figure 2).
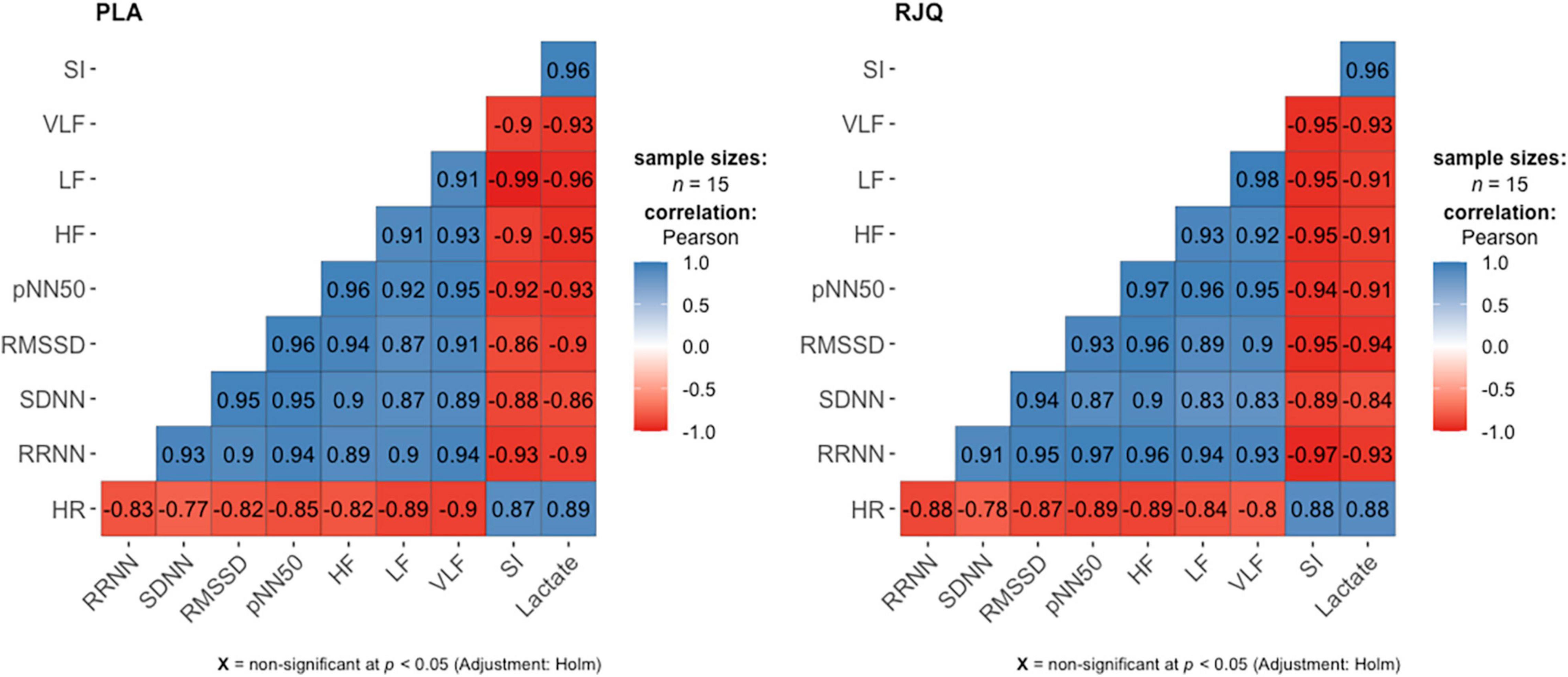
Figure 2. Correlation matrix for displaying the relationship between the changes in HRV parameters and blood lactate levels under HIIE conditions in both intervention groups. n = 15 per group. HR, heart rate; RRNN, mean RR normal-to-normal intervals; SDNN, standard deviation of normal-to-normal intervals; RMSSD, root mean square of successive RR intervals differences; pNN50, percentage of successive RR intervals that differ by more than 50 ms; HF, absolute power of the high-frequency band; LF, absolute power of the low-frequency band; VLF, absolute power of the very-low-frequency band; SI, stress index; RJQ, royal jelly plus coenzyme Q10; PLA, placebo.
Regression analysis showed that oral RJQ administration for 10 days was significantly associated with reductions in HIIE-induced increases in heart rate, SI, and blood lactate concentration compared to PLA (Table 2).
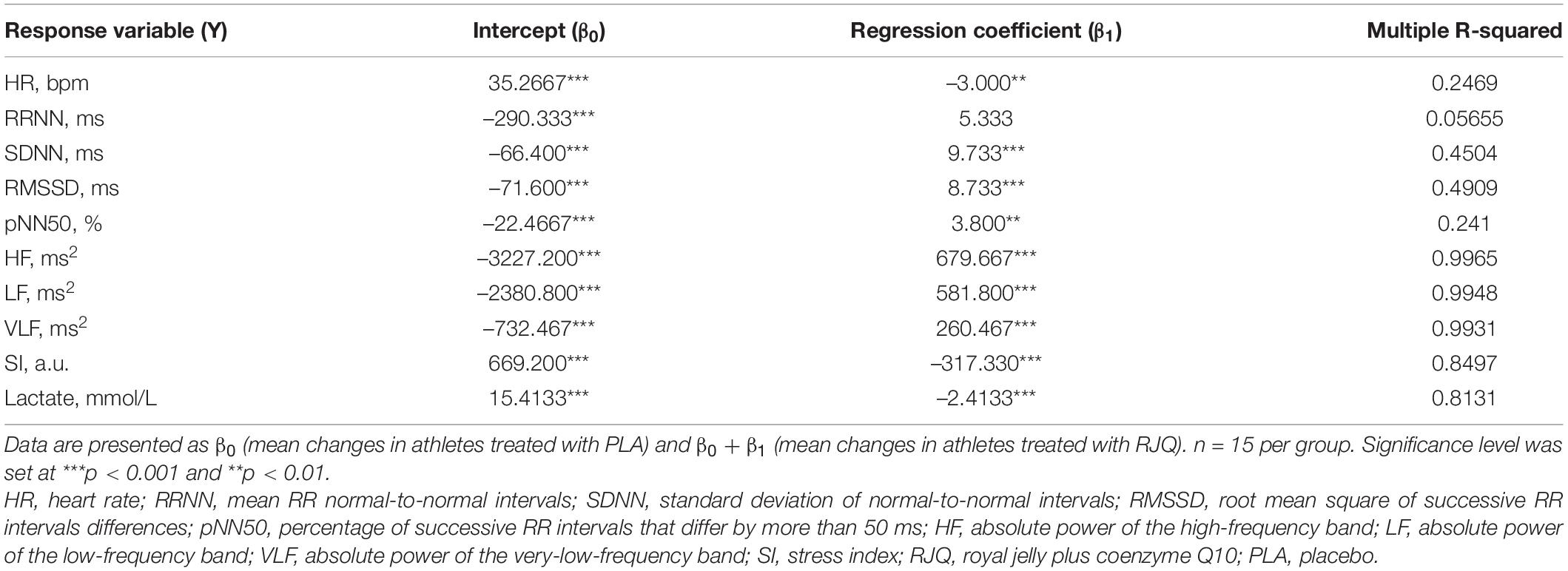
Table 2. Modeling the effects of 10-day RJQ supplementation on changes in HRV parameters and blood lactate levels in response to high-intensity interval exercise.
The marked RJQ-induced reduction in SI following exercise is consistent with a withdrawal of sympathetic activity. Moreover, the more rapid shift in autonomic balance toward a dominant parasympathetic activity was reflected by the RJQ-caused increment in SDNN, RMSSD, pNN50, and HF power immediately after the HIIE. An important finding was that HIIE also induced a smaller decrease in LF power and VLF power in subjects who consumed RJQ compared to PLA. This finding may be considered as additional evidence that the LF power and VLF power are not specific for sympathetic activity.
Principal component analysis revealed that PC1 describes almost 83.23% of the total variance of the changes in HR, SDNN, RMSSD, pNN50, HF, LF, VLF, SI, and blood lactate. In its turn, PC2, PC3, PC4, PC5, PC6, PC7, PC8, and PC9 described only a minor part (12.94%, 2.67%, 0.58%, 0.29%, 0.23%, 0.06%, 0.004%, and 0.002%, respectively) of total variation of the changes in RJQ-dependent variables. Their explanation is too low to take PC 2–9 into consideration in the subsequent analysis. Figure 3 shows that athletes treated with RJQ are grouped by the first two principal components into a separate cluster compared to PLA.
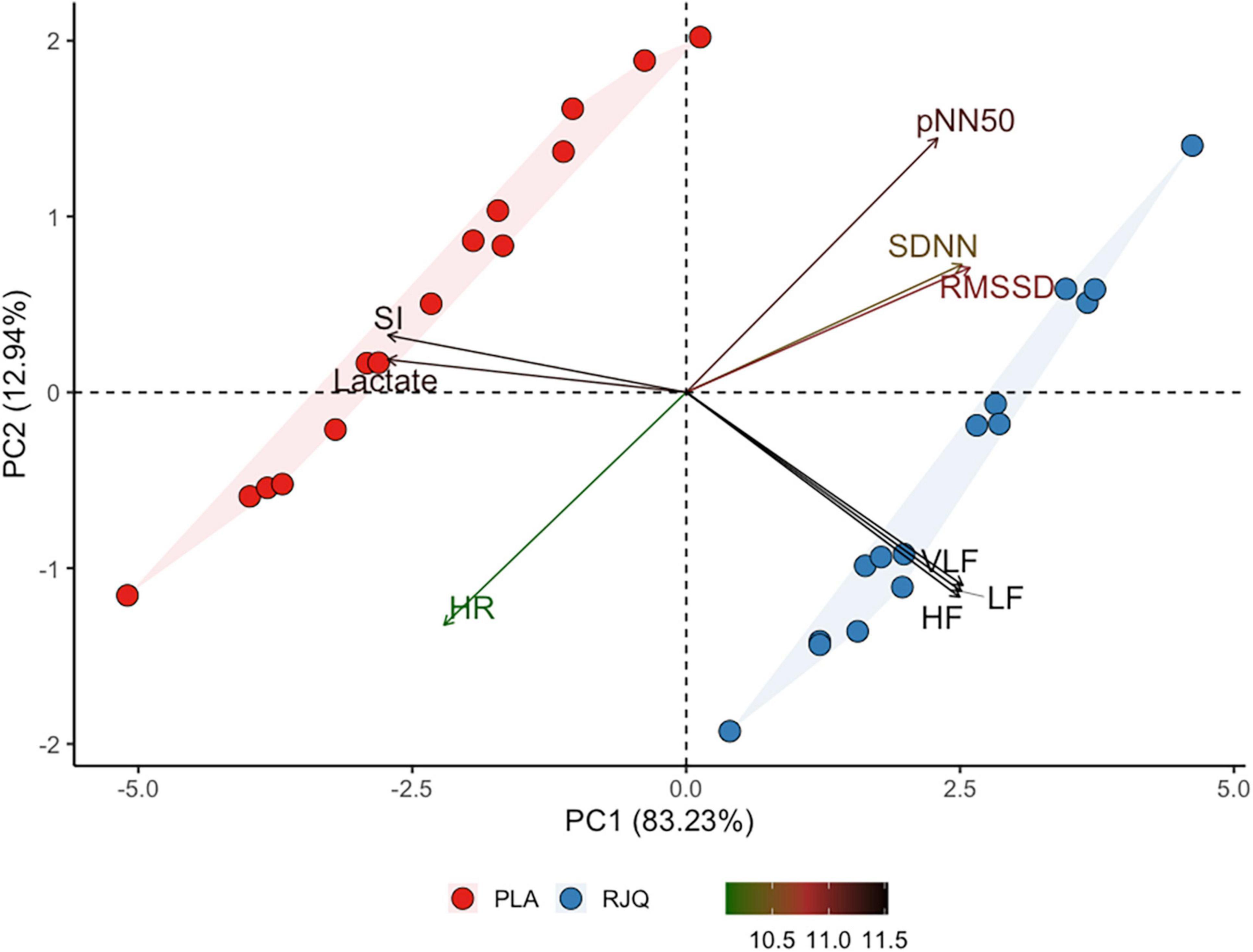
Figure 3. Principal component analysis applied to the changes in RJQ-dependent variables under HIIE conditions. n = 15 per group. PC1, principal component 1; PC2, principal component 2; HR, heart rate; SDNN, standard deviation of normal-to-normal intervals; RMSSD, root mean square of successive RR intervals differences; pNN50, percentage of successive RR intervals that differ by more than 50 ms; HF, absolute power of the high-frequency band; LF, absolute power of the low-frequency band; VLF, absolute power of the very-low-frequency band; SI, stress index; RJQ, royal jelly plus coenzyme Q10; PLA, placebo.
PC1 values in the RJQ group were more than those in the PLA group, which corresponded to a much lower increase in HR, SI, and blood lactate concentration in response to HIIE, as well as to the higher SDNN, RMSSD, pNN50, HF, LF, and VLF following exercise. It should be noted that there was a strong negative correlation between the time taken to complete HIIE and PC1 values in both intervention groups (Figure 4).
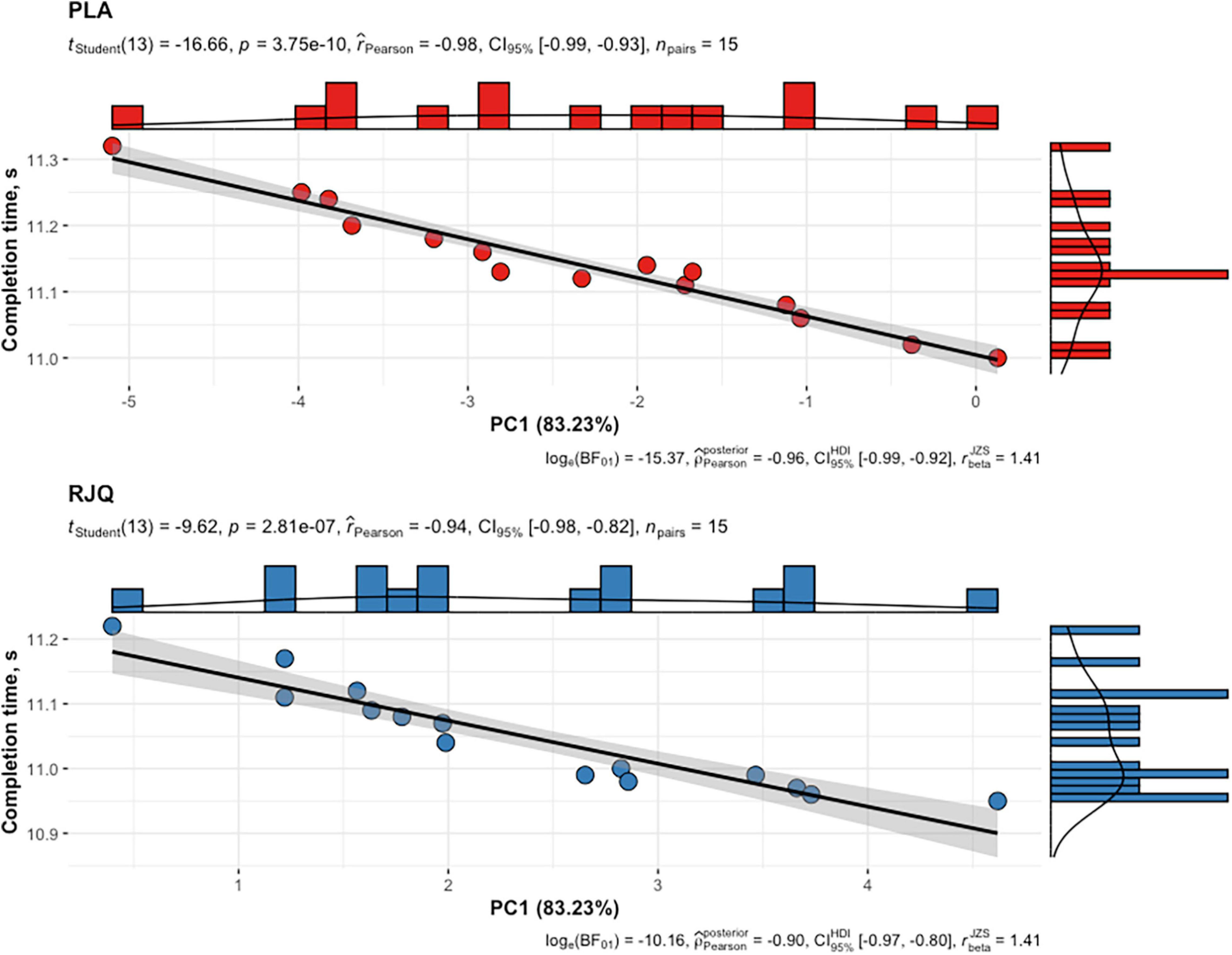
Figure 4. Scattergram of PC1 values and time taken by athletes to complete HIIE in both intervention groups. n = 15 per group. PC1, principal component 1; RJQ, royal jelly plus coenzyme Q10; PLA, placebo.
Discussion
To our knowledge, this is one of the first randomized, placebo-controlled, double-blind trials to determine whether RJQ supplementation exerts beneficial effects on energetic metabolism and autonomic nervous system in athletes under HIIE conditions, and also on their exercise performance. The results of this study have shown that oral RJQ administration for 10 days was associated with a much lower increase in blood lactate concentration in response to the HIIE. The RJQ supplementation also induced a shift toward greater parasympathetic tone early at post-exercise, as indicated by lower HR and higher SDNN, RMSSD, and pNN50 compared to PLA. In addition, HRV frequency-domain parameters, such as HF power, LF power, and VLF power, in the RJQ group were higher than those in the PLA group, reflecting not only enhanced parasympathetic and sympathetic modulation but also increase in favor of baroreflex input and humoral influence on the heart. Moreover, the RJQ-induced changes in HRV and blood lactate concentration led to a shorter time taken by athletes to complete HIIE compared to PLA. Direction and strength of statistical relationship between the completion time and PC1 values demonstrate that the improvements in runners’ HIIE performance were due in significant part to RJQ-induced reduction of increment in blood lactate levels in response to exercise in combination with a more rapid shift in autonomic activity toward increased parasympathetic control early following exercise. Our prior work partially confirms these findings where 10-day RJQ ingestion increased HIIE performance in swimmers while reducing the increment in SI and blood lactate levels in response to exercise (33). And, unlike the present study, specific exercise protocol related to swimming was applied. Thereby, developing new pharmacological strategies that include RJQ supplementation could be potentially beneficial for improving HIIE performance in athletes and reducing the risk of adverse effects of increased intramuscular acidity and prolonged sympathetic dominance following intense exercise.
It is known that RJ and CoQ10 are able to promote ATP synthesis via glycolysis and oxidative phosphorylation (OXPHOS) (24, 25), respectively, suggesting that RJQ may play an important role in enhancing exercise performance and reductions in lactic acidosis. 10-hydroxy-2-decenoic acid (10H2DA), which is a unique fatty acid specifically found in RJ, has been reported to induce AMPK activation in skeletal muscle, leading to insulin-independent translocation of glucose transporter (GLUT) 4 to the plasma membrane and greater glucose uptake (21). In addition to 10H2DA, leucine and casein peptide found in RJ have been shown to also activate AMPK in skeletal muscles (23–25). Furthermore, AMPK, known to be activated not only by RJ but also by CoQ10, is responsible for the stimulation of glycolysis through phosphorylation of 6-phosphofructo-2-kinase that catalyzes the synthesis and degradation of fructose-2,6-bisphosphate, which in turn regulates the activity of 6-phosphofructo-1-kinase, thereby controlling the glycolysis rate (34–37). During very intense efforts lasting seconds, such as the 100-m sprint, most ATP is derived from the breakdown of phosphocreatine (PCr) and glycogen to lactate (16). OXPHOS is also activated but its contribution to ATP turnover during maximal exercise lasting about 12 s is low and may be highest after the duration of high-intensity interval exercise (repeated bouts of 100-m sprint with active recovery of 45 s between sets) extends beyond approximately 1 min (16, 38, 39). Despite the activation of the oxidative pathways in skeletal muscle during HIIE, the rates of ATP provision from the anaerobic sources (PCr and anaerobic glycolysis) are much more rapid than those from aerobic pathways, which resulted in the production of lactate and its subsequent accumulation in muscle and blood (16, 40). In this situation, CoQ10 contained in RJQ could stimulate mitochondrial OXPHOS through activation of complex I that regulates the rate in overall respiration (41). CoQ10, an essential cofactor in OXPHOS, is able to increase cellular ATP production, carrying the electrons from complexes I and II to complex III of the mitochondrial respiratory chain (23–25). On the other hand, CoQ10 might also indirectly increase the flux of glucose through pyruvate dehydrogenase (PDH) into the tricarboxylic acid cycle, in part via a decrease in NADH and possibly acetyl-CoA, which would keep PDH active during HIIE (42, 43). Therefore, glycolytic flux, activity of complex I and NAD+-dependent enzymes, and ultimately aerobic ATP production might be higher during exercise in athletes treated with RJQ compared to PLA. Perhaps, RJQ supplementation links the glycolytic pathway and OXPHOS in muscle cells, leading to greater ability to rapidly resynthesize PCr when the exercise intensity falls and athletes rest.
Interestingly, phosphorylation and activation of AMPK by 10H2DA are mediated via extracellular Ca2+/calmodulin-dependent protein kinase β (CaMKKβ) independently of changes in adenine nucleotides and liver kinase B1 pathway (21). Thus, 10H2DA-induced AMPK activation in response to a transient elevation of intracellular Ca2+ caused by muscle contractions appears likely to play an important role in regulating AMPK under exercise conditions. Despite this activation of AMPK in skeletal muscle, CaMKKβ-induced activation of AMPK is suggested to be a key pathway in neural tissue because expression of CaMKKβ is much higher in the brain than in other organs (44, 45). Novel biological contexts in which AMPK activation by calcium might be relevant are constantly being reported (46). One such notable context may be an activation of transient receptor potential ankyrin 1 (TRPA1) and vanilloid 1 (TRPV1), both of which are Ca2+-permeable non-selective cation channels (47, 48). Specific hydroxy fatty acids in RJ, such as 10H2DA, 10-hydroxydecanoic acid, have been shown to activate both TRPA1 and TRPV1, but TRPA1 more strongly than TRPV1 (20). Additionally, dicarboxylic acids showed equal efficacy for both channels, but they are present in only small amounts in RJ (20). The RJQ-induced activation of TRPA1 and TRPV1 appears to have a role in the regulation of both sympathetic and parasympathetic branches of the autonomic nervous system, likely in a biphasic manner characterized by early sympathetic activation, when exercise begins, followed by a shift toward greater vagal dominance as rapidly as needed, when transitioning from exercise to rest occurs or the exercise intensity falls to low levels. Activation of TRPA1 in muscle afferent nerves has been shown to amplify sympathetic responsiveness via the metabolic component of the exercise pressor reflex when blood supply to the muscles is insufficient, which occurs during HIIE (49). In contrast, blocking TRPA1 has been reported to attenuate sympathetic nerve activity response evoked by muscle contraction and not to affect sympathetic modulation during passive tendon stretch (49). Similarly, TRPV1 channel is involved in the skeletal muscle exercise pressor reflex (50). Smith et al. found that TRPV1 blockade alleviates the increase in heart rate and blood pressure during muscle contraction (51). However because we did not measure HRV parameters during transitions from rest to exercise in the present study, we cannot confirm the presence of enhanced sympathetic drive during exercise in the RJQ group compared to the PLA group. Instead, we observed opposite effects of RJQ supplementation on HRV during the transition from HIIE to recovery and concluded that the parasympathetic dominance (i.e., increased SDNN, RMSSD, pNN50, and HF power) may be in part due to RJQ-induced reductions in oxidative stress caused by exercise (26). Our previous study has suggested that improved high-intensity interval exercise performance in swimmers is partially associated with the RJQ-induced reducing in lipid peroxidation and muscle damage during exercise (52). It should be noted that exposure to exogenous activators of TRPA1 and TRPV1 has been shown to cause a slight increase in parasympathetic influence on heart rate in healthy subjects at rest (53). Importantly, TRPV1 activation improves baroreflex sensitivity and energy metabolism (50, 54). Since LF power and VLF power reflect baroreflex function and energy metabolic regulation, respectively (53, 55), the possible activation of TRPV1 by RJQ may be an explanation for significantly higher values of these HRV frequency-domain parameters in athletes treated with RJQ compared to PLA under exercise conditions. Besides, Luo et al. revealed that chronic TRPV1 activation induces expression of the transcriptional cofactor peroxisome proliferator-activated receptor-γ coactivator-1α (PGC-1α) and enhances mitochondrial function in a Ca2+-dependent manner in the skeletal muscle, leading to greater exercise endurance (56). Increased HIIE performance in participants who received RJQ compared to PLA for 10 days is likely to be TRPA1/TRPV1-mediated Ca2+-signaling mechanisms that involve CaMKKβ and AMPK. Of note, RJQ-induced AMPK activation may be functionally more important for the post-exercise changes in muscle metabolism and insulin sensitivity, and for mediating some of the key adaptive responses to exercise in skeletal muscle, such as mitochondrial biogenesis, enhanced antioxidant protection, and increased GLUT4 expression. AMPK increases mitochondrial biogenesis in skeletal muscle via activation of PGC-1α that enhances mitochondrial tissue content and subsequently the capacity of the cell to respond to future energetic challenges (46, 57). Therefore, the long-term changes induced by RJQ supplementation may be associated with improved skeletal muscle fiber transformation from glycolytic type II fibers to more oxidative type I fibers and, consequently, increased respiratory function. It is known that type I fibers have more mitochondrial mass and produce more ATP from lipid oxidation but less from glycolysis than type II fibers, thus providing stable energy state for a longer time with less lactate accumulation (56, 58–60). A recent study demonstrated that RJ treatment with endurance training induced mitochondrial adaptation through activation of AMPK in mice soleus muscle (22), which predominantly consists of type I and type IIA muscle fibers (61–63). These findings support the hypothesis that oral ingestion of RJQ for 10 days in conjunction with regular exercise might yield synergies toward greater HIIE performance through multiple complementary pathways, as stated above.
This study has some limitations that should be noted. First, the CoQ10 levels in blood plasma and skeletal muscle were not quantified. The second limitation derives from the origin of the sample. The fact that all athletes were associated with sprinting and recruited from a single sports center prompts us to be cautious in generalizing the results to athletes who perform HIIE and participate in other sports. Additional context that may be considered as important for the RJQ supplementation is its dose and timing, which were pre-established, and the dose-response effect could not be analyzed in the present study. It may be useful to consider higher dosages and longer interventions to determine their potential benefit. Nevertheless, our study had important strengths because there are no studies, excluding our findings, which have analyzed RJQ as a nutritional supplement in athletes. Further studies with athletes who perform various types of muscular exercise are now needed to determine whether RJQ supplementation may be effective not just in increasing runners’ HIIE performance but also in other sport-specific performances.
Conclusion
The study reveals that RJQ supplementation significantly reduced the exercise-induced increment in blood lactate concentration in combination with a more rapid shift in autonomic balance toward increased parasympathetic control early following exercise compared to PLA. We also found a strong negative correlation between the time taken to complete HIIE and PC1 values, which described more than 83% of the total variance in HR, SDNN, RMSSD, pNN50, HF, LF, VLF, SI, and blood lactate. An important point to note here is that PC1 values in the RJQ group were more than those in the PLA group, which corresponded to a much lower increase in HR, SI, and blood lactate concentration in response to HIIE, as well as to higher SDNN, RMSSD, pNN50, HF, LF, and VLF following exercise. Thus, we demonstrated that RJQ-induced changes in cardiac autonomic regulation and blood lactate levels in runners under HIIE conditions enhance their exercise performance.
Data Availability Statement
The raw data supporting the conclusions of this article will be made available by the authors, without undue reservation.
Ethics Statement
The studies involving human participants were reviewed and approved by the Bioethics Committee of Lobachevsky University (approval number: 43). The participants provided their written informed consent to participate in this study.
Author Contributions
AO: conceptualization and visualization. AO and AD: formal analysis, investigation, resources, and data curation. AO and AP: writing-original draft preparation. AO, AD, and AP: writing-review and editing. All authors have read and agreed to the published version of the manuscript.
Conflict of Interest
The authors declare that the research was conducted in the absence of any commercial or financial relationships that could be construed as a potential conflict of interest.
Publisher’s Note
All claims expressed in this article are solely those of the authors and do not necessarily represent those of their affiliated organizations, or those of the publisher, the editors and the reviewers. Any product that may be evaluated in this article, or claim that may be made by its manufacturer, is not guaranteed or endorsed by the publisher.
Acknowledgments
We thank the athletes for their participation and their coaches for help in conducting this research.
References
2. Meeusen R, Duclos M, Foster C, Fry A, Gleeson M, Nieman D, et al. Prevention, diagnosis, and treatment of the overtraining syndrome: joint consensus statement of the European college of sport science and the American college of sports medicine. Med Sci Sports Exerc. (2013) 45:186–205. doi: 10.1249/MSS.0b013e318279a10a
3. Schmitt L, Regnard J, Millet GP. Monitoring fatigue status with HRV measures in elite athletes: an avenue beyond RMSSD? Front Physiol. (2015) 6:343. doi: 10.3389/fphys.2015.00343
4. Perrone MA, Volterrani M, Manzi V, Barchiesi F, Iellamo F. Heart rate variability modifications in response to different types of exercise training in athletes. J Sports Med Phys Fitness. (2021) 61:1411–5. doi: 10.23736/S0022-4707.21.12480-6
5. Garet M, Tournaire N, Roche F, Laurent R, Lacour JR, Barthélémy JC, et al. Individual Interdependence between nocturnal ANS activity and performance in swimmers. Med Sci Sports Exerc. (2004) 36:2112–8. doi: 10.1249/01.mss.0000147588.28955.48
6. Seiler S, Haugen O, Kuffel E. Autonomic recovery after exercise in trained athletes: intensity and duration effects. Med Sci Sports Exerc. (2007) 39:1366–73. doi: 10.1249/mss.0b013e318060f17d
7. Goodwin ML, Harris JE, Hernández A, Gladden LB. Blood lactate measurements and analysis during exercise: a guide for clinicians. J Diabetes Sci Technol. (2007) 1:558–69. doi: 10.1177/193229680700100414
8. Brooks GA. The lactate shuttle during exercise and recovery. Med Sci Sports Exerc. (1986) 18:360–8. doi: 10.1249/00005768-198606000-00019
9. Miller BF, Fattor JA, Jacobs KA, Horning MA, Navazio F, Lindinger MI, et al. Lactate and glucose interactions during rest and exercise in men: effect of exogenous lactate infusion. J Physiol. (2002) 544:963–75. doi: 10.1113/jphysiol.2002.027128
10. Medbø JI, Jebens E, Noddeland H, Hanem S, Toska K. Lactate elimination and glycogen resynthesis after intense bicycling. Scand J Clin Lab Invest. (2006) 66:211–26. doi: 10.1080/00365510600570599
11. Hashimoto T, Hussien R, Oommen S, Gohil K, Brooks GA. Lactate sensitive transcription factor network in L6 cells: activation of MCT1 and mitochondrial biogenesis. FASEB J. (2007) 21:2602–12. doi: 10.1096/fj.07-8174com
12. Takahashi H, Alves CRR, Stanford KI, Middelbeek RJW, Nigro P, Ryan RE, et al. TGF-β2 is an exercise-induced adipokine that regulates glucose and fatty acid metabolism. Nat Metab. (2019) 1:291–303. doi: 10.1038/s42255-018-0030-7
13. Scheiman J, Luber JM, Chavkin TA, MacDonald T, Tung A, Pham LD, et al. Meta-omics analysis of elite athletes identifies a performance-enhancing microbe that functions via lactate metabolism. Nat Med. (2019) 25:1104–9. doi: 10.1038/s41591-019-0485-4
14. Gaitanos GC, Williams C, Boobis LH, Brooks S. Human muscle metabolism during intermittent maximal exercise. J Appl Physiol. (1993) 75:712–9. doi: 10.1152/jappl.1993.75.2.712
15. Sahlin K, Ren JM. Relationship of contraction capacity to metabolic changes during recovery from a fatiguing contraction. J Appl Physiol. (1989) 67:648–54. doi: 10.1152/jappl.1989.67.2.648
16. Hargreaves M, Spriet LL. Skeletal muscle energy metabolism during exercise. Nat Metab. (2020) 2:817–28.
17. Burke LM, Hawley JA. Swifter, higher, stronger: what’s on the menu? Science. (2018) 362:781–7. doi: 10.1126/science.aau2093
18. Hawley JA, Burke LM, Phillips SM, Spriet LL. Nutritional modulation of training-induced skeletal muscle adaptations. J Appl Physiol. (2011) 110:834–45. doi: 10.1152/japplphysiol.00949.2010
19. Ramadan MF, Al-Ghamdi A. Bioactive compounds and health-promoting properties of royal jelly: a review. J Funct Foods. (2012) 4:39–52. doi: 10.3390/molecules26123770
20. Terada Y, Narukawa M, Watanabe T. Specific hydroxy fatty acids in royal jelly activate TRPA1. J Agric Food Chem. (2011) 59:2627–35. doi: 10.1021/jf1041646
21. Takikawa M, Kumagai A, Hirata H, Soga M, Yamashita Y, Ueda M, et al. 10-Hydroxy-2-decenoic acid, a unique medium-chain fatty acid, activates 5’-AMP-activated protein kinase in L6 myotubes and mice. Mol Nutr Food Res. (2013) 57:1794–802. doi: 10.1002/mnfr.201300041
22. Takahashi Y, Hijikata K, Seike K, Nakano S, Banjo M, Sato Y, et al. Effects of royal jelly administration on endurance training-induced mitochondrial adaptations in skeletal muscle. Nutrients. (2018) 10:1735. doi: 10.3390/nu10111735
24. Haas RH. The evidence basis for coenzyme Q therapy in oxidative phosphorylation disease. Mitochondrion. (2007) 7:S136–45. doi: 10.1016/j.mito.2007.03.008
25. Testai L, Martelli A, Flori L, Cicero AFG, Colletti A. Coenzyme Q10: clinical applications beyond cardiovascular diseases. Nutrients. (2021) 13:1697. doi: 10.3390/nu13051697
26. Ovchinnikov AN. The Effect of the Combination of Royal Jelly and Exogenous Coenzyme Q10 on the Indicators of Functional Status Among Athletes Under Exercise. Dissertation/PhD Thesis. Nizhny Novgorod: Lobachevsky University (2019).
27. World medical association declaration of Helsinki. Recommendations guiding physicians in biomedical research involving human subjects. JAMA. (1997) 277:925–6. doi: 10.1001/jama.1997.03540350075038
28. Kon M, Tanabe K, Akimoto T, Kimura F, Tanimura Y, Shimizu K, et al. Reducing exercise-induced muscular injury in kendo athletes with supplementation of coenzyme Q10. Br J Nutr. (2008) 100:903–9. doi: 10.1017/S0007114508926544
29. Shimomura Y, Suzuki M, Sugiyama S, Hanaki Y, Ozawa T. Protective effect of coenzyme Q10 on exercise-induced muscular injury. Biochem Biophys Res Commun. (1991) 176:349–55. doi: 10.1016/0006-291x(91)90931-v
30. Takahashi K, Takahashi M. Exogenous administration of coenzyme Q10 restores mitochondrial oxygen consumption in the aged mouse brain. Mech Ageing Dev. (2013) 134:580–6. doi: 10.1016/j.mad.2013.11.010
31. Heart rate variability: standards of measurement, physiological interpretation and clinical use. Task Force of the European Society of Cardiology and the North American Society of Pacing and Electrophysiology. Circulation. (1996) 93:1043–65.
32. Baevsky RM, Chernikova AG. Heart rate variability analysis: physiological foundations and main methods. Cardiometry. (2017) 10:66–7.
33. Ovchinnikov AN, Deryugina AV. Effects of composition of ubiquinone-10 and royal jelly on physiological and biochemical correlates of improved physical activity rates in highly qualified athletes. Teoriya i Praktika Fizicheskoy Kultury. (2020) 2020:36–8.
34. Tsai KL, Chen LH, Chiou SH, Chiou GY, Chen YC, Chou HY, et al. Coenzyme Q10 suppresses oxLDL-induced endothelial oxidative injuries by the modulation of LOX-1-mediated ROS generation via the AMPK/PKC/NADPH oxidase signaling pathway. Mol Nutr Food Res. (2011) 55:S227–40. doi: 10.1002/mnfr.201100147
35. Langsjoen PH, Langsjoen JO, Langsjoen AM, Lucas LA. Treatment of statin adverse effects with supplemental coenzyme Q10 and statin drug discontinuation. BioFactors. (2005) 25:147–52. doi: 10.1002/biof.5520250116
36. Rider MH, Bertrand L, Vertommen D, Michels PA, Rousseau GG, Hue L. 6-phosphofructo-2-kinase/fructose-2,6-bisphosphatase: head-to-head with a bifunctional enzyme that controls glycolysis. Biochem J. (2004) 381(Pt 3):561–79. doi: 10.1042/BJ20040752
37. Marsin AS, Bertrand L, Rider MH, Deprez J, Beauloye C, Vincent MF, et al. Phosphorylation and activation of heart PFK-2 by AMPK has a role in the stimulation of glycolysis during ischaemia. Curr Biol. (2000) 10:1247–55. doi: 10.1016/s0960-9822(00)00742-9
38. Parolin ML, Chesley A, Matsos MP, Spriet LL, Jones NL, Heigenhauser GJ. Regulation of skeletal muscle glycogen phosphorylase and PDH during maximal intermittent exercise. Am J Physiol. (1999) 277:E890–900. doi: 10.1152/ajpendo.1999.277.5.E890
39. Medbø JI, Tabata I. Relative importance of aerobic and anaerobic energy release during short-lasting exhausting bicycle exercise. J Appl Physiol. (1989) 67:1881–6. doi: 10.1152/jappl.1989.67.5.1881
40. Spriet LL, Howlett RA, Heigenhauser GJ. An enzymatic approach to lactate production in human skeletal muscle during exercise. Med Sci Sports Exerc. (2000) 32:756–63. doi: 10.1097/00005768-200004000-00007
41. Sharma LK, Lu J, Bai Y. Mitochondrial respiratory complex I: structure, function and implication in human diseases. Curr Med Chem. (2009) 16:1266–77. doi: 10.2174/092986709787846578
42. Patel MS, Roche TE. Molecular biology and biochemistry of pyruvate dehydrogenase complexes. FASEB J. (1990) 4:3224–33. doi: 10.1096/fasebj.4.14.2227213
43. Prentki M, Matschinsky FM, Madiraju SR. Metabolic signaling in fuel-induced insulin secretion. Cell Metab. (2013) 18:162–85. doi: 10.1016/j.cmet.2013.05.018
44. Hawley SA, Pan DA, Mustard KJ, Ross L, Bain J, Edelman AM, et al. Calmodulin-dependent protein kinase kinase-beta is an alternative upstream kinase for AMP-activated protein kinase. Cell Metab. (2005) 2:9–19. doi: 10.1016/j.cmet.2005.05.009
45. Woods A, Dickerson K, Heath R, Hong SP, Momcilovic M, Johnstone SR, et al. Ca2+/calmodulin-dependent protein kinase kinase-beta acts upstream of AMP-activated protein kinase in mammalian cells. Cell Metab. (2005) 2:21–33. doi: 10.1016/j.cmet.2005.06.005
46. Garcia D, Shaw RJ. AMPK: Mechanisms of cellular energy sensing and restoration of metabolic balance. Mol Cell. (2017) 66:789–800. doi: 10.1016/j.molcel.2017.05.032
47. Ching LC, Chen CY, Su KH, Hou HH, Shyue SK, Kou YR, et al. Implication of AMP-activated protein kinase in transient receptor potential vanilloid type 1-mediated activation of endothelial nitric oxide synthase. Mol Med. (2012) 18:805–15. doi: 10.2119/molmed.2011.00461
48. Maiese K. Warming up to new possibilities with the capsaicin receptor TRPV1: mTOR, AMPK, and erythropoietin. Curr Neurovasc Res. (2017) 14:184–9. doi: 10.2174/1567202614666170313105337
49. Xing J, Lu J, Li J. TRPA1 mediates amplified sympathetic responsiveness to activation of metabolically sensitive muscle afferents in rats with femoral artery occlusion. Front Physiol. (2015) 6:249. doi: 10.3389/fphys.2015.00249
50. Hudson AS, Kunstetter AC, Damasceno WC, Wanner SP. Involvement of the TRPV1 channel in the modulation of spontaneous locomotor activity, physical performance and physical exercise-induced physiological responses. Braz J Med Biol Res. (2016) 49:e5183. doi: 10.1590/1414-431X20165183
51. Smith SA, Leal AK, Williams MA, Murphy MN, Mitchell JH, Garry MG. The TRPv1 receptor is a mediator of the exercise pressor reflex in rats. J Physiol. (2010) 588(Pt 7):1179–89. doi: 10.1113/jphysiol.2009.184952
52. Ovchinnikov A, Deryugina A, Kontorschikova C, Okrut I. Effects of 10-day royal jelly and coenzyme Q10 supplementation on functional condition in highly qualified athletes. Clin Chim Acta. (2019) 493(Suppl. 1):S494.
53. Michlig S, Merlini JM, Beaumont M, Ledda M, Tavenard A, Mukherjee R, et al. Effects of TRP channel agonist ingestion on metabolism and autonomic nervous system in a randomized clinical trial of healthy subjects. Sci Rep. (2016) 6:20795. doi: 10.1038/srep20795
54. Sun H, Li DP, Chen SR, Hittelman WN, Pan HL. Sensing of blood pressure increase by transient receptor potential vanilloid 1 receptors on baroreceptors. J Pharmacol Exp Ther. (2009) 331:851–9. doi: 10.1124/jpet.109.160473
55. Rahman F, Pechnik S, Gross D, Sewell L, Goldstein DS. Low frequency power of heart rate variability reflects baroreflex function, not cardiac sympathetic innervation. Clin Auton Res. (2011) 21:133–41.
56. Luo Z, Ma L, Zhao Z, He H, Yang D, Feng X, et al. TRPV1 activation improves exercise endurance and energy metabolism through PGC-1α upregulation in mice. Cell Res. (2012) 22:551–64. doi: 10.1038/cr.2011.205
57. Steinberg GR, Carling D. AMP-activated protein kinase: the current landscape for drug development. Nat Rev Drug Discov. (2019) 18:527–51. doi: 10.1038/s41573-019-0019-2
58. Wang YX, Zhang CL, Yu RT, Cho HK, Nelson MC, Bayuga-Ocampo CR, et al. Regulation of muscle fiber type and running endurance by PPARdelta. PLoS Biol. (2004) 2:e294. doi: 10.1371/journal.pbio.0020294
59. Joyner MJ, Coyle EF. Endurance exercise performance: the physiology of champions. J Physiol. (2008) 586:35–44. doi: 10.1113/jphysiol.2007.143834
60. Jeukendrup AE, Saris WH, Wagenmakers AJ. Fat metabolism during exercise: a review–part II: regulation of metabolism and the effects of training. Int J Sports Med. (1998) 19:293–302. doi: 10.1055/s-2007-971921
61. Augusto V, Padovani CR, Rocha Campos G. Skeletal muscle fiber types in C57Bl6J mice. Braz J Morphol Sci. (2004) 21:89–94.
62. Denies MS, Johnson J, Maliphol AB, Bruno M, Kim A, Rizvi A, et al. Diet-induced obesity alters skeletal muscle fiber types of male but not female mice. Physiol Rep. (2014) 2:e00204. doi: 10.1002/phy2.204
Keywords: royal jelly, coenzyme Q10, athletic performance, running, autonomic nervous system, heart rate variability, energetic metabolism, lactate
Citation: Ovchinnikov AN, Deryugina AV and Paoli A (2022) Royal Jelly Plus Coenzyme Q10 Supplementation Enhances High-Intensity Interval Exercise Performance via Alterations in Cardiac Autonomic Regulation and Blood Lactate Concentration in Runners. Front. Nutr. 9:893515. doi: 10.3389/fnut.2022.893515
Received: 10 March 2022; Accepted: 19 May 2022;
Published: 23 June 2022.
Edited by:
Mark Elisabeth Willems, University of Chichester, United KingdomReviewed by:
Patrick Orlando, Marche Polytechnic University, ItalyAlenka Nemec Svete, University of Ljubljana, Slovenia
Copyright © 2022 Ovchinnikov, Deryugina and Paoli. This is an open-access article distributed under the terms of the Creative Commons Attribution License (CC BY). The use, distribution or reproduction in other forums is permitted, provided the original author(s) and the copyright owner(s) are credited and that the original publication in this journal is cited, in accordance with accepted academic practice. No use, distribution or reproduction is permitted which does not comply with these terms.
*Correspondence: Aleksandr N. Ovchinnikov, YWxleGFuZGVyX292Y2hpbm5pa292OTFAbWFpbC5ydQ==