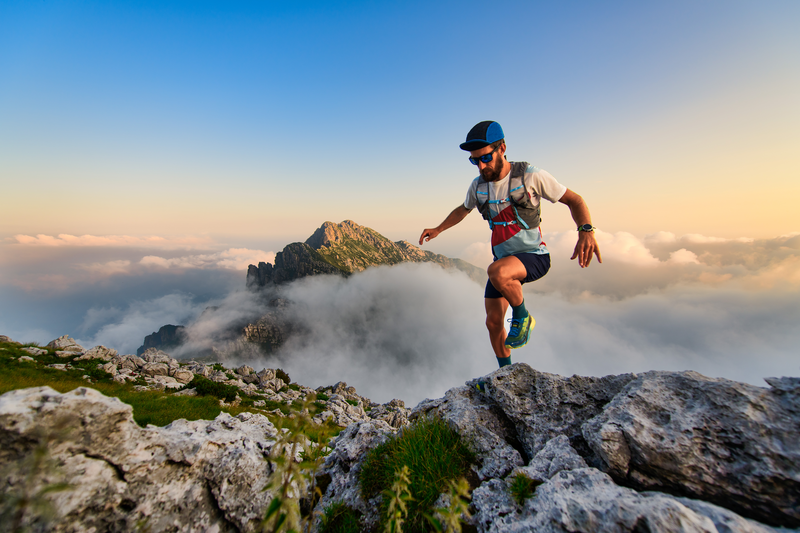
94% of researchers rate our articles as excellent or good
Learn more about the work of our research integrity team to safeguard the quality of each article we publish.
Find out more
ORIGINAL RESEARCH article
Front. Nutr. , 29 April 2022
Sec. Nutrition and Sustainable Diets
Volume 9 - 2022 | https://doi.org/10.3389/fnut.2022.890786
This article is part of the Research Topic The Chemistry of Food in the Advent of Sustainable Diets View all 8 articles
The supplementation of bioactive compounds in astronaut’s diets is undeniable, especially in the extreme and inhospitable habitat of future space settlements. This study aims to enhance the Martian and Lunar regolith fertility (testing two commercial simulants) through the provision of organic matter (manure) as established by in situ resource utilization (ISRU) approach. In this perspective, we obtained 8 different substrates after mixing Mojave Mars Simulant (MMS-1) or Lunar Highlands Simulant (LHS-1), with four different rates of manure (0, 10, 30, and 50%, w/w) from monogastric animals. Then, we assessed how these substrates can modulate fresh yield, organic acid, carotenoid content, antioxidant activity, and phenolic profile of lettuce plants (Lactuca sativa L.). Regarding fresh biomass production, MMS-1-amended substrates recorded higher yields than LHS-1-ones; plants grown on a 70:30 MMS-1/manure mixture produced the highest foliar biomass. Moreover, we found an increase in lutein and β-carotene content by + 181 and + 263%, respectively, when applying the highest percentage of manure (50%) compared with pure simulants or less-amended mixtures. The 50:50 MMS-1/manure treatment also contained the highest amounts of individual and total organic acids, especially malate content. The highest antioxidant activity for the ABTS assay was recorded when no manure was added. The highest content of total hydroxycinnamic acids was observed when no manure was added, whereas ferulic acid content (most abundant compound) was the highest in 70:30 simulant/manure treatment, as well as in pure LHS-1 simulant. The flavonoid content was the highest in pure-simulant treatment (for most of the compounds), resulting in the highest total flavonoid and total phenol content. Our findings indicate that the addition of manure at specific rates (30%) may increase the biomass production of lettuce plants cultivated in MMS-1 simulant, while the phytochemical composition is variably affected by manure addition, depending on the stimulant. Therefore, the agronomic practice of manure amendment showed promising results; however, it must be tested with other species or in combination with other factors, such as fertilization rates and biostimulants application, to verify its applicability in space colonies for food production purposes.
In recent years, there is a growing interest in space exploration and the subsequent establishment of extraterrestrial colonies on the Moon or Mars. In addition to the leading space agencies, such as the National Aeronautics and Space Administration (NASA) and the European Space Agency (ESA), private companies (e.g., SpaceX) are also currently focused on space research (1, 2). The synergistic collaboration of many countries and experts in various disciplines has resulted in the achievement of new technological milestones, which should allow the realization of full-fledged missions aimed at both exploration and colonization of new planets in the coming decades (3, 4). When planning the establishment of a future outpost, either on the Moon or Mars, it is fundamental to consider the self-sufficiency of the colony as the hypothesis of total resources that supply from Earth would be practically unrealistic in regard to both time management and high costs (5–7). In this regard, indigenous soil-based agricultural systems could be an effective solution to relieve the inputs of a bioregenerative life support system (BLSS) (8–11), and meanwhile, the in situ resource utilization (ISRU) approach could provide a valuable contribution to cost reduction using in situ materials and recycling all colony waste products to the maximum extent possible (12–17). Recently, Cannon and Britt (18) evaluated a set of alternatives for the possible development of a self-sufficient community on Mars. The authors assumed the in situ production of basic needs, emphasizing that the diet of colony residents would necessarily have to change by focusing on insect farming for food production rather than plant cultivation, pointing out the differences in terms of growth cycle duration, area devoted, and production costs (18). However, this assessment omits the remarkable ecological role that plants may play, since apart from food production, they are able to strongly support the BLSS through water recycling, CO2 fixation, and oxygen production (5, 8, 19), thus being a pivotal part of a biological loop that is not only focused on food production. In addition, there are nutritional aspects of plant-derived food products that cannot be neglected, such as their content in fundamental macro- and microminerals, bioactive compounds, such as carotenoids and phenolic acids, that are extremely important for balanced human health (20, 21).
The Martian and Lunar surface is composed mainly of basaltic rocks and sediments that include varying amounts of different minerals, such as olivine, pyroxene, plagioclase, anorthosite, vitric and lithic fragments, iron oxides, and sulfates (22–27). Several studies reported that Martian and Lunar soils are not suitable for plant cultivation, as they are poor in nutrients (primarily nitrogen) and organic matter, and also lack proper soil structure (28, 29). However, these shortcomings could be compensated by proper agronomic practices (30, 31). Due to the unavailability of real Martian and Lunar regolith samples for agronomic testing, scientific experiments on space cultivation can be only conducted with commercial simulants derived from crushed terrestrial rocks, which tend to replicate the geotechnical and compositional characteristics of regolith studied during the past manned and unmanned missions to the Moon or Mars, respectively (17). Over the years, various research organizations have developed different versions of extraterrestrial soil simulants (27, 32). To make such media suitable for plant cultivation, the use of organic soil amendments, such as monogastric manure, could be hypothesized. The use of monogastric manure is based on the concept that this organic matter would be more similar to crew excrement, which after being properly treated (33–35) could be adopted to improve the physicochemical characteristics of regolith. As it is well known, the symbiotic relationship created between plants and the soil microbiomes (e.g., fungi and bacteria) is also fundamental for proper soil fertility. In general, rhizosphere interactions occur through different mechanisms, such as the colonization of root surfaces, bacterial movement, and the creation of synergistic interactions with the plant (36). However, the extreme environment of the Moon and Mars is not conventional for life development; for this reason, the relationship between plant–soil microbiome could be not predictable at planet surface. However, the use of regolith as growing media under “controlled conditions” cannot preclude the establishment of microbial relationships that can improve the fertility of these substrates in a long-term cultivation.
Another important aspect is the species selection for food production in BLSS. The selection of candidate crops was usually carried out using specific criteria, such as nutritional value, plant size, adaptability to extreme environmental conditions, low resource requirements, short crop cycle, and high harvest index (37–41). Among the various candidate species, lettuce (Lactuca sativa L.) is highly ranked, as it is a fast-growing leafy vegetable with a high harvest index (> 0.9) (42). In addition, its leaves are rich in mineral elements, dietary fiber, carotenoids, vitamin C, and phenolic compounds (43–47), which are sorely needed especially for space crews subjected to severe oxidative and inflammatory stresses during space missions (48–51).
To date, very few works have evaluated the plant cultivation on Martian or Lunar soil simulants (28, 52, 53). In particular, Gilrain et al. (52) evaluated the Swiss chard (Beta vulgaris) growth for the application in Advanced Life Support (ALS) using Martian regolith simulants (JCS-1A Mars) mixed with leaf compost in the following ratios:1:0, 3:1, 1:1, 1:3, and 0:1 (v/v). Onsay et al. (54) assessed the performance of a full crop cycle (of Extra Dwarf pak choi and red romaine lettuce) in Martian regolith simulants (JCS-1A Mars and MGS-1) amended with mushroom compost, measuring biometric parameters and the quantity of chlorophyll and anthocyanin. Finally, Duri et al. (16) grew in a walk-in growth chamber two varieties of lettuce with different leaf pigmentation on four different mixtures (0:100, 70:30, 30:70, and 100:0, v/v) based on Martian simulant (MMS-1) and green compost, and biometric, physiological, and qualitative parameters were measured at harvest. To the best of our knowledge, only these three experiments have adopted regolith–compost mixtures as plant-growing media, providing a Hoagland solution for all experiments, but none of the abovementioned studies addressed the effect of manure amendment. Therefore, the purpose of this work was to evaluate the response in terms of nutraceutical properties of a widely used crop, such as lettuce to soil amendment, by testing mixtures at different percentages of extraterrestrial simulant and monogastric manure. The main aim was to evaluate whether manure amendment can compensate the defects of extraterrestrial regolith, as established in the ISRU approach, to find the best mix that can maximize the nutritional value of lettuce while ensuring an acceptable yield.
A pot experiment was carried out in an open-gas-exchange growth chamber (28 m2: 7.0 m × 2.1 m × 4.0 m; W × H × D; Process-C5, Spagnol srl, Treviso, Italy), with lettuce plants (Lactuca sativa L. cv. “Grand Rapids”) grown in different mixtures of extraterrestrial soil simulants and monogastric manure. The lighting of the growth chamber was provided by high-pressure sodium lamps (Master SON-T PIA Plus 400W, Philips, Eindhoven, the Netherlands) with a photosynthetic photon flux density (PPFD) of 420 ± 20 μmolm–2 s–1. Air temperature was set at 24–18°C (light/dark) with a 12-h photoperiod and a relative air humidity of 60–80% maintained by a fog system. The experiment was carried out at ambient CO2 concentration (390 ± 20 ppm), while air exchange and dehumidification were guaranteed by two heating, ventilation, and air conditioning (HVAC) systems. Then, two different simulants were tested: the Mojave Mars Simulant (MMS-1), purchased from The Martian Garden (Austin, Texas, United States) and the Lunar Highlands Simulant (LHS-1) purchased from Exolith Lab (University of Central Florida, Orlando, Florida, United States). Both simulants are coarse-textured alkaline (pH 9 to 10) substrates consisting mostly of plagioclase (anorthite) and amorphous Al and Fe minerals. The tested treatments were prepared after mixing each simulant with sieved (2 mm) horse/swine monogastric manure (Agraria Di Vita srl, Pescia, Pistoia, Italy) at doses of 0, 10, 30, and 50% (w/w). Plants were transplanted into 9 cm x 9 cm x 9 cm pots irrigated with only reverse osmosis water throughout the crop cycle (31 days). The experimental design was laid out according to the randomized complete-block factorial design with four manure amendment rates (M) and two extraterrestrial soil simulants (S), with three replicates. Each experimental plot consisted of four plants (total of 96 plants).
At harvest, the fresh biomass (g plant–1) of all plants for each treatment was determined. Fresh samples from each plant were split into two subsamples; one of them was instantly frozen in liquid nitrogen, lyophilized, and stored at –80°C for further phytochemical analyses, whereas the remaining sample was used for water content determination after drying in a forced-air oven at 70°C to constant weight (around 72 h). Oven-dried samples were then ground with a cutting-grinding head mill at 0.5 mm (IKA, MF 10.1, Staufen, Germany) and used for nitrate and organic acid content determinations (malate, tartrate, oxalate, citrate, and isocitrate). In brief, 250 mg of ground leaf tissue was suspended in 50 ml of ultrapure water (Arium® Advance EDI pure water system; Sartorius, Goettingen, Lower Saxony, Germany), stirred in a shaking water bath (ShakeTemp SW22, Julabo, Seelbach, Germany) at 80°C for 10 min, filtered at 0.45 μm, and finally analyzed by ion chromatography (ICS-3000, Dionex, Sunnyvale, CA, United States) as described by Pannico et al. (46).
For antioxidant activity, 200 mg of lyophilized material was extracted with 5 ml of methanol (stored at 4°C) and then centrifuged at 400 rpm for 5 min. The supernatant was collected and re-centrifuged after adding a further 5 ml methanol to the pellet. A total of two different assays were carried out for antioxidant activity determination: the ABTS-scavenging capacity based on the method described by Re et al. (55), and the 1,1-diphenyl-2-picrylhydrazyl (DPPH) free radical scavenging activity using the procedure proposed by Brand-Williams et al. (56) modified, briefly detailed as follows.
For the ABTS assay, a stock solution was incubated at a temperature of 4°C for 16 h (2.5 ml of aqueous ABTS-7 mM and 44 ml of potassium persulfate-2.45 mM). Then, this stock solution was diluted (1:88) with ethanol having an absorbance of 0.700 ± 0.050 at 734 nm. The analysis was performed by combining 0.1 ml of filtered sample and 1 ml of ABTS radical working solution and then monitoring the absorbance after 2.5 min at 734 nm. For the DPPH assay, 1 ml of methanolic DPPH-100 μm was added to 200 μl of diluted lettuce extract. The absorbance of DPPH was 0.90 ± 0.02 at 517 nm, while the decrease in absorbance of the resulting solution was monitored after 10 min of incubation at room temperature in the dark at 517 nm. All determinations were performed in triplicate, and the results were expressed as Trolox® equivalent antioxidant capacity (TEAC, mmol Trolox® equivalents kg–1 dw).
The freeze-dried lettuce samples (100 mg) were extracted by modifying the method of Kim et al. (57). The samples were mixed in 6 ml of ethanol containing 0.1% BHT and placed in a water bath for 5 min at 85°C. Then, 120 μl of 80% KOH was added to the samples, and subsequently, they were vortexed and returned to the water bath for 10 min at the same temperature. In the end, they were placed in ice to stop the reaction and in each solution were added 3 ml of distilled water and 3 ml of hexane. Subsequently, centrifugation was applied to collect the hexane layer, and the pellet was re-extracted two times more using hexane. Finally, after all the extraction procedures, the hexane layers were combined and dried with nitrogen gas. About 1 ml of chloroform was added to recover the residue and filtered with a 0.2-μm nylon filter before the quantification by HPLC-DAD. For the quantification, a Shimadzu HPLC Model LC 10 (Shimadzu, Osaka, Japan) was used, equipped with a reverse-phase 250 mm × 4.6 mm, 5 μm Gemini C18 column (Phenomenex, Torrance, CA, United States), after injecting 20 μl of sample. The following A:B gradient: 0–8 min (82:18); 8–12 min (76:24); 12–18 min (39:61); and 18–25 min, a linear gradient to equilibration (82:18), was prepared using acetonitrile and ethanol/n-hexane/dichloromethane (1:1:1) (respectively, for mobile phases A and B). The absorbance measurements were recorded at 450 nm and expressed in mg kg–1 dw. To perform the quantification, a linear calibration curve was carried out using lutein and β-carotene standards at 6 levels of concentration (from 5 up to 100 μg ml–1). About 100 mg of lyophilized sample was used for polyphenol quantification (expressing it as μg g–1 dw). The extraction procedure involved the use of 5 ml of methanol and water solution (60:40, v/v), which was sonicated with the sample for 30 min. Then, the suspension was centrifuged (400 rpm) for 15 min and filtered with filter paper (0.45 μm) using 10 μl for mass spectrometry (HRMS-Orbitrap) analysis. UHPLC system (UHPLC, Thermo Fisher Scientific, Waltham, MA, United States) equipped with a Dionex Ultimate 3000 Quaternary pump performing at 1,250 bar and a thermostated (25°C) Kinetex 1.7 μm biphenyl (100 mm × 2.1 mm) column (Phenomenex, Torrance, CA, United States) was used to carry out the polyphenol determination assay. A volume of 2 μl was injected, using a flow rate of 0.2 ml min–1 to elute and using 0.1% formic acid in water and 0.1% formic acid in methanol, respectively, to prepare a gradient of A and B in the following way: 0 min – 5% B, 1.3 min – 30% B, 9.3 min – 100% B, 11.3 min – 100% B, 13.3 min – 5% B, and 20 min – 5% B. A Q Exactive Orbitrap LC-MS/MS (Thermo Fisher Scientific, Waltham, MA, United States) was involved in mass spectrometry analysis. An ESI source (HESI II, Thermo Fischer Scientific, Waltham, MA, United States) in negative ion mode (ESI-) was used. The following ion source parameters were applied: −2.8 kV spray voltage, sheath gas (N2 > 95%) 45, auxiliary gas (N2 > 95%) 10, capillary temperature 275°C, S-lens RF level 50, and auxiliary gas heater temperature 305°C. The polyphenolic compound targeted acquisition was carried out on parallel reaction monitoring (PRM) mode, set as follows: microscans at 1, resolution at 35.000, AGC target at 5e5, maximum ion time at 100 ms, MSX count at 1, and isolation window at 1.0 m/z. The input time frame for elution and collision energy (CE) were optimized for each polyphenolic compound. The accuracy and calibration of the Q Exactive Orbitrap LC-MS/MS were checked using a Thermo Fisher Scientific reference standard mixture and setting the mass tolerance window for the two analysis modes at 5 ppm. The linearity of the method for both low and high (5 mg kg–1–120 mg kg–1) concentration ranges was assessed using six concentration levels in each calibration range. The low limit of detection (LOD) and low limit of quantitation (LOQ) values for HPLC-DAD analysis of carotenoids were determined for β-carotene, while in the case of LC-MS/MS analysis of polyphenols were based on chlorogenic acid and rutin signal-to-noise levels. LOD and LOQ for each compound were obtained by serial dilutions of stock solution, and the analysis and processing of data were performed using the Xcalibur software, v. 3.0.63 (Thermo Fisher Scientific, Waltham, MA, United States).
Data were subjected to two-way ANOVA using the IBM SPSS software package (SPSS Inc., Chicago, Illinois, United States). The mean effects of simulants (S) and manure amendment (M) factors were compared according to the unpaired Student’s t-test and one-way analysis of variance, respectively. Significant statistical differences were determined by Tukey–Kramer HSD test for the S factor and the S × M interaction at the level of p ≤ 0.05.
A statistically significant interaction was observed between simulant (S) and manure percentage (M) factors, with the highest value recorded in the treatment where MMS-1 was combined with 30% manure, while the Lunar simulant (LHS-1) had the highest yields in the intermediate treatments (10 and 30% of manure) (Figure 1). Moreover, regardless of the amendment treatment, lettuce fresh biomass produced on the MMS-1 simulant was on average of 3.7-folds higher than on LHS-1, indicating that the specific simulant is more appropriate for lettuce cultivation than the LHS-1 substrate.
Figure 1. Fresh biomass of lettuce grown in different mixtures of MMS-1 or LHS-1 simulants and manure (simulant/manure rates: 100:0, 90:10, 70:30, 50:50; w/w).
The simulant mean effect showed significant differences for the content of water, nitrate, malate, citrate, and isocitrate, with the highest values obtained in MMS-1 except for nitrate content which was significantly lower compared to lunar simulant (−22%) (Table 1). The water content showed a direct correlation with the percentage of amendment (R = 0.89) presenting a 24% increase in the 50% manure treatments compared to the non-amended simulants (Table 1).
Table 1. Water, nitrate, and organic acid contents of grown lettuce in different mixtures of MMS-1 or LHS-1 simulants and manure (simulant/manure rates: 100:0, 90:10, 70:30, 50:50; w/w).
A statistically significant interaction of the two factors (S × M) was observed in the content of all detected organic acids. There was a significantly higher content of malate, tartrate, and oxalate in the 50% MMS-1 mixture compared to the rest of the treatments where an increase by 486, 217, and 67% was recorded, respectively; however, the citrate content did not differ between the various combinations of simulants and manure rates (Table 1). Regarding the Lunar simulant, malate and tartrate contents were significantly higher (on average of + 275 and + 130%, respectively) in the amended mixtures than in the pure LHS-1. On the other hand, the isocitrate content was significantly higher in the non-amended simulant and 50% amendment than in the 10 and 30% manure treatment; in contrast, the citrate content was significantly lower in the non-amended LHS-1 compared to the 30% amended mixture (Table 1). Finally, the highest content of individual and total organic acids (except for the case of citrate where no significant differences were observed) was recorded for the highest rate (50%) of manure, regardless of the simulant, whereas MMS-1 had higher amounts of malate, citrate, and isocitrate than the LSH-1 simulant, regardless of the manure rate (Table 1).
A significant interaction of the two factors (S × M) was observed for ABTS and DPPH assays, where the non-amended simulants had the highest Trolox content in the case of ABTS, whereas the non-amended simulants and the combination of MMS-1 × 50% of manure and LSH-1 × 30% of manure recorded the highest activity in DPPH assay (Table 2). The simulant mean effect (S) showed significantly higher ABTS and DPPH assays in lettuce grown on LHS-1 (+ 3.2 and + 10.7%, respectively) compared with those on MMS-1 (Table 2). Similarly, the non-amended simulants showed the highest activity in all the studied assays.
Table 2. Antioxidant activity of lettuce grown in different mixtures of MMS-1 or LHS-1 simulants and manure (simulant/manure rates: 100:0, 90:10, 70:30, 50:50; w/w).
The mean effect of simulants (S) showed a significantly higher concentration of lutein in LHS-1 (236 mg kg–1) compared to MMS-1 (200 mg kg–1), whereas no significant difference was detected in β-carotene content. For both carotenoids, a direct correlation was observed between their content and the amendment percentage of the different mixtures (R > 0.97). Moreover, a significant interaction of S × M was found in both carotenoids reaching the highest content at the 50% manure dose (Figure 2). Specifically, the Martian simulant recorded an increase in lutein and β-carotene content at 30 (+ 78.7 and + 141%, respectively) and 50% (+ 181 and + 263%, respectively) amendment compared to non-amended MMS-1 (Figure 2). Regarding LHS-1, the lutein content is on average of 132% higher at the two intermediate mixtures and 245% higher at the maximum manure dose with respect to the non-amended simulant. Similarly, the β-carotene content in the lunar simulant was significantly higher by 206 and 287% at 10 and 50% amendment treatments, respectively, whereas its concentration at the 30% manure dose was assessed between the latter two mixtures (Figure 2).
Figure 2. Lutein and β-carotene concentration of lettuce grown in different mixtures of MMS-1 or LHS-1 simulants and manure (simulant/manure rates: 100:0, 90:10, 70:30, 50:50; w/w).
Chlorogenic acid was the most prevalent compound among all detected hydroxycinnamic acids, followed by ferulic acid, feruloyl-disinapoyl-gentiobiose, and synapoyl-hexose (Table 3A). A significant interaction of S × M factors was found for coumaroyl-diglucoside, ferulic acid, feruloyl-disinapolyl-gentiobiose, and synapoyl-hexose. Specifically, coumaroyl-diglucoside was the highest in the non-amended MMS-1 simulant, whereas the content of feruloyl-disinapolyl-gentiobiose results higher in both pure simulants. Instead, ferulic acid and feruloyl-disinapoyl-gentiobiose varied among the treatments. Ferulic acid was significantly higher in the pure LHS-1 compared to the 10 and 50% manure mixtures. In contrast, synapoyl-hexose was significantly higher in the 30 and 50% manure Martian simulant than in pure MMS-1. The mean effect of simulants (S) was significant for coumaroyl-diglucoside and synapoyl-hexose content which was the highest in MMS-1, whereas the opposite trend was found for ferulic acid (highest content in LSH-1 simulant). The mean effect of the amendment (M) revealed an inverse correlation between chlorogenic acid concentration and manure dose in the different mixtures, reaching a 20.7% reduction at the highest amendment percentage compared to the non-amended simulant. The same trend was also observed for total hydroxycinnamic acids, whereas the opposite trend was recorded for synapoyl-hexose which increased in the amended simulant compared to the non-amended one (Table 3A).
Table 3A. Phenolic profiles and total phenolic composition of lettuce grown in different mixtures of MMS-1 or LHS-1 simulants and manure (simulant/manure rates: 100:0, 90:10, 70:30, 50:50; w/w).
Regarding the flavonoid profile, no significant interactions S x M were found in flavonoids as well as the content of total flavonoids and total phenols. Moreover, the mean effect of simulants (S) showed a significantly higher concentration in the case of kaempferol-3-diglucoside, quercetin 3-sophoroside-7-glucoside, and rutin in LHS-1 compared to MMS-1 simulant (Table 3B). Interestingly, the mean effect of the amendment (M) revealed a progressive decrease in the content of most of the detected compounds when manure was added in the tested simulants. The same trend was also observed for the content of total flavonoids and total phenols, resulting in a reduction at the maximum manure dose of 53.0 and 23.3%, respectively, compared to the non-amended simulant (Table 3B).
Table 3B. Phenolic profiles and total phenolic composition of lettuce grown in different mixtures of MMS-1 or LHS-1 simulants and manure (simulant/manure rates: 100:0, 90:10, 70:30, 50:50; w/w).
This study evaluates the possibility of using human excreta (replaced by a surrogate derived from monogastric farm animals) as a soil amendment to improve the physicochemical and structural characteristics of Martian or Lunar regolith for plant production, in the preparation for a future manned mission to Mars or Moon, respectively. To improve ISRU protocols and minimize procurement from the Earth, the impact of the amendment rate on the nutritional and functional characteristics of lettuce was evaluated. Although this vegetable provides only a limited amount of calories, it is nevertheless a rich source of bioactive compounds, such as carotenoids (mainly lutein and β-carotene) and polyphenols, which counteract the development of chronic diseases and could be helpful to maintain health in space missions (58). A long-term space mission inevitably results in a progressive decline in astronauts’ mental and physical performances (59). In this context, dietary supplementation with fresh vegetables rich in nutraceuticals possessing high antioxidant activity could, on the one hand, minimize pathophysiological effects (59, 60) and, on the other hand, help maintain mental well-being of individuals forced to live in isolated or extreme environments (17, 50). Considering the above, two commercial simulants (a Martian and a Lunar simulant) were amended with different ratios of manure from monogastric animals aiming to evaluate the possible effects on lettuce cultivation. Although these simulants can be a source of available nutrients, such as Ca, Mg, and K, they lack organic C, N, and available P and S, essential for plant growth. As well, they easily release soluble Na, which can induce salt stress in the plants [(61), submitted]. Whereas the manure was characterized by a low C/N atomic ratio (i.e., 11.0 ± 0.4), it can provide a significant amount of potentially available N for rhizosphere microorganisms and plant roots. At the same time, according to the mediumlow H/C atomic ratio (i.e., 1.5 ± 0.1), it would comprise a significant aromatic moiety, ensuring good stability of the organic matter over time. It is also an important source of nutrients; however, it contains significant amounts of Na, which negatively raise its pH to 9.0 and E.C. to ∼7 dS m–1 [(61), submitted]. According to the literature, the previous experiment with human excreta as plant fertilizers gave promising results in terms of crop performance and acceptability from farmers (33). Therefore, the goal of this experiment was to test whether it is possible to improve the physicochemical parameters of Martian and Lunar simulants through the manure amendment and extrapolate the obtained results to the cultivation of lettuce in space conditions using human excreta as a soil amendment.
Regardless of treatments, the low yield recorded was attributable to a severe nutritional deficiency as excluding the limited input by the manure, both regolith simulants were highly lacking in key macronutrients and organic matter (61, 62). The higher fresh biomass obtained in plants grown on the Martian simulant could be due to the worse physicochemical characteristics of the Lunar substrate, such as the lower water retention capacity and higher pH, and/or the higher ammonium nitrate content of the Martian regolith, as assessed in a complementary study – part 1 [(61), submitted] and discussed as well by Wamelink et al. (28). By analyzing the interaction between the tested factors (S x M), the yield reduction observed in plants grown on the substrates containing 50% of manure, especially in the case of LHS-1 simulant, could be due to the increase in electrical conductivity of the substrates by the higher manure content (63). This trend was also analyzed and discussed in a complementary study – part 2 [(64), submitted], which assessed the suitability of these eight MMS-1 or LHS-1/manure mixtures for space food production, by matching their physicochemical and hydraulic characteristics with the lettuce growth performance (biometric and physiological parameters), soil enzymatic activity, and nutrient bioavailability in the growth media at plant harvest time.
According to Wamelink et al. (28), the better performance of the Martian simulant compared to the Lunar one could be due to better water-holding capacity; however, the increased rates of manure (> 30%) probably exaggerated the water content and had negative effects on lettuce plants grown in both simulants. Similar results were also observed by Petropoulos et al. (65) who also associated the differences in water content of lettuce plants to the differences in water-holding capacity of the substrates tested. The results of our study are in accordance with the findings of Duri et al. (16) who also suggested that the amendment of Martian regolith with 30% of compost was the most realistic in terms of crop performance and compost availability in space conditions, whereas the 30:70 (Martian regolith/compost) gave the best overall results. Caporale et al. (29) also suggested the use of 30% of green compost due to larger leaf area and better pore size distribution. However, Duri et al. (16) also suggested that a genotype-dependent response was observed, which could justify the differences with our study. Moreover, the abovementioned studies used composts as soil amendments instead of manure, and this could also explain the differences in observed results due to the difference in the physicochemical properties of the tested amendments. Therefore, it seems that the intermediate amounts of manure are the most beneficial since they not only increase the amounts of water that simulants may hold but also increase nutrient availability and retain pH and EC values at acceptable levels for efficient plant growth.
The percentage of manure amendment in the substrates also affected the plant antioxidant activity, showing a clear response to nutritional stress, confirming the findings observed in previous work (16). The non-amended simulants showed the highest antioxidant activity in the case of ABTS assay, whereas the response to DPPH varied among the tested treatments. Moreover, in all the assays, the non-amended simulants had the highest antioxidant activity, regardless of the simulant which further justifies our previous argument regarding the stressful conditions that lettuce plants were subjected to when grown in non-amended substrates. Our hypothesis was also supported by the content of phenolic acid, flavonoid, and total phenol, since the biosynthesis of these secondary metabolites tends to increase as a response to plant stressors (66), while their content is strongly dependent on genotype and agronomic conditions (67, 68). Moreover, the higher ABTS and DPPH values recorded in lettuces grown on LHS-1 mixtures were consistent with the lower fresh biomass yield for the same treatment, confirming the high stress exerted by the lunar simulant.
In agreement with Kim et al. (58), the most abundant phenolic acid in lettuce in our study was chlorogenic acid, which indicates that the phenolic profile is highly associated with the genotype (45, 69). Moreover, the content of total phenolic acids, total flavonoids, and total phenols was the highest in non-amended simulants which was also reported by Duri et al. (16) for red Salanova lettuce plants, whereas the same authors did not record significant differences in total phenol content for green Salanova plants at the tested rates of simulant/compost. This finding indicates that genotype is also important for plant response to abiotic stressors, and various lettuce genotypes should be tested in future studies to make safe conclusions about the potential of cultivating lettuce in Martian or Lunar soils and the possibility to use human excreta as soil amendments.
Nitrate content in leaf tissues is strongly influenced by soil nitrate and ammonium levels (70); nevertheless, in our experiment, the amendment dose did not significantly affect the foliar concentration of this anion. Regarding the effect of the simulant, two hypotheses can explain the lower nitrate content recorded in MMS-1. The first is a probable dilution effect triggered by both the higher fresh biomass and the greater water content of plants grown on this simulant compared to LHS-1; the second hypothesis considers a lower accumulation due to a higher rate of assimilation into organic compounds (such as proteins and nucleic acids). Very low nitrate levels (on a fresh basis) have also been found in lettuce (71) and Brassicaceae (72) grown under severe nutrient deficit. However, it seems that nitrate content is also highly depended on the genotype since according to El-Nakhel et al. (37), significant differences between two Salanova lettuce cultivars (green and red Salanova) showed a different response to nitrate accumulation when grown under controlled conditions. It is also important to facilitate optimum growth conditions, especially regarding light intensity, since leafy vegetables tend to increase its content when grown under suboptimal light conditions (11, 73).
Manures have been shown to improve the water retention capacity of substrates (17, 74). These findings corroborate the higher water content of lettuce plants recorded at high manure doses, probably due to increased water availability as the percentage of amendment increases [(54), submitted]. The dose of manure in regolith mixtures also induced an adaptive response by the plants, which resulted in an accumulation of organic acids. These metabolites are involved in different biochemical pathways at the cell level, as the intermediates of photosynthesis and amino acid biosynthesis (75, 76) or as osmoregulators and cell protectors against stress conditions (77). In our experiment, the increased content of malate, tartrate, oxalate, and isocitrate in the 50% substrates is likely due to higher salt stress, as observed in previous work on lettuce (78) and other leafy vegetables (79). Moreover, the content of specific organic acids, such as oxalates, is also associated with nitrogen availability and nitrogen form, and an increase of oxalates should be expected with increasing nitrogen availability (77, 80), as was also the case of high amendment rates in our study. The content of organic acids in vegetables not only affects their taste, but also their acceptability, nutraceutical value, and shelf life (81–83). In addition, they are beneficial to human health by acting as antioxidants due to their ability to chelate metals (84).
The increase in lutein and β-carotene achieved in plants grown on the 50:50 mixtures was consistent with findings on lettuce by Kim et al. (58) following increasing doses of soil amendment. In the same line, Hernández et al. (85) suggested that reduced nitrogen availability resulted in reduced amounts of carotenoids, whereas high salinity induced the biosynthesis of these compounds. High amounts of carotenoids, such as lutein and β-carotene, may play a protective role against oxidative stress since they act as reactive oxygen species scavengers and quenchers of free radicals (11). Although carotenoid content is highly associated with light conditions and light intensity in particular (86, 87), the application of soil amendments from different sources may also increase their content and improve the quality of lettuce plants (88) and their health beneficial effects (58). However, considering the genotypic variation in carotenoid content among the various lettuce genotypes, further studies with multiple genotypes are needed to identify those cultivars that could be used in space colonies as health-promoting food sources (89).
The supplementation of bioactive compounds in astronaut’s diets is undeniable, especially in the extreme and inhospitable habitat of future space settlements. The carotenoid content was positively correlated with the increment of monogastric manure in the growth substrate (+ 210% of lutein and + 273% of β-carotene). In contrast, the content of total phenols was lower in amended simulants than in pure ones, whereas the antioxidant activity was shown to be mainly related to the phenolic content. Our results indicate that the lettuce yields observed in the tested growth substrates are still not sufficient to ensure the self-sustainability of future space settlements. Exclusive input of pure water and manure does not appear to meet the minimum soil fertility requirements that are necessary to guarantee optimal crop development. However, it must be considered that pedogenesis is regulated by long processes that cannot be accomplished in a single lettuce cycle since the formation of fertile soil from disintegrated parental rock requires several chemical and physical alterations and continuous inputs of organic matter. Similarly, in the future extraterrestrial outpost, it will be feasible to gradually improve the regolith fertility with repeated cropping cycles and continuous inputs of organic substances composed partly by crew excrements and partly by the residues of previous crops. In addition, a start-up phase involving minimal nutrient supplementation and inoculation of plant growth-promoting rhizobacteria (PGPR) should be also investigated in future studies. In this regard, we can consider our results promising, as they demonstrated that by adding 30% of manure to pure regolith, it is possible to complete a lettuce cycle by feeding the plants with only pure water. However, further studies are needed with more lettuce genotypes to explore genotypic variation and make safe conclusions about the potential cultivation of the species in regolith.
The raw data supporting the conclusions of this article will be made available by the authors, without undue reservation.
YR contributed to conceptualization, visualization, supervision, and project administration. LD, AP, SP, AC, PA, GG, AR, and SD contributed to methodology, validation, formal analysis, investigation, and writing—original draft preparation. YR and SD contributed to funding acquisition and resources. LD and AP contributed to software and data curation. LD, AP, SP, AC, PA, GG, AR, SD, and YR contributed to writing, reviewing, and editing the manuscript. All authors contributed to the article and approved the submitted version.
This research was supported by the project in situ REsource Bio-Utilization for life Support system (ReBUS), unique project code (CUP) F74I16000000005 financed by the Italian Space Agency.
The authors declare that the research was conducted in the absence of any commercial or financial relationships that could be construed as a potential conflict of interest.
All claims expressed in this article are solely those of the authors and do not necessarily represent those of their affiliated organizations, or those of the publisher, the editors and the reviewers. Any product that may be evaluated in this article, or claim that may be made by its manufacturer, is not guaranteed or endorsed by the publisher.
1. Shammas VL, Holen TB. One giant leap for capitalistkind: private enterprise in outer space. Palgrave Commun. (2019) 5:10. doi: 10.1057/s41599-019-0218-9
2. Sarang M, Robinson C, Ekblaw A. Towards a lunar open architecture: facilitating transparency and collaboration in the new era of lunar exploration. In: Proceedings of the 71st International Astronautical Congress. New York, NY: IAC (2006). doi: 10.1016/s0262-4079(08)62732-3
3. Verseux C, Baqué M, Lehto K, de Vera JPP, Rothschild LJ, Billi D. Sustainable life support on Mars – the potential roles of cyanobacteria. Int J Astrobiol. (2016) 15:65–92. doi: 10.1017/S147355041500021X
5. Llorente B, Williams T, Goold H. The multiplanetary future of plant synthetic biology. Genes (Basel). (2018) 9:348. doi: 10.3390/genes9070348
6. Massa GD, Emmerich JC, Morrow RC, Bourget CM, Mitchell CA. Plant-growth lighting for space life support: a review. Gravitational Sp Biol. (2006) 19:19–30.
7. Zabel P, Zeidler C, Vrakking V, Dorn M, Schubert D. Biomass production of the EDEN ISS space greenhouse in Antarctica during the 2018 experiment phase. Front Plant Sci. (2020) 11:656. doi: 10.3389/fpls.2020.00656
8. Maggi F, Pallud C. Martian base agriculture: the effect of low gravity on water flow, nutrient cycles, and microbial biomass dynamics. Adv Sp Res. (2010) 46:1257–65. doi: 10.1016/j.asr.2010.07.012
9. Nelson M, Dempster WF, Allen JP. Integration of lessons from recent research for “Earth to Mars” life support systems. Adv Sp Res. (2008) 41:675–83. doi: 10.1016/j.asr.2007.02.075
10. Silverstone S, Nelson M, Alling A, Allen J. Development and research program for a soil-based bioregenerative agriculture system to feed a four person crew at a Mars base. Adv Sp Res. (2003) 31:69–75. doi: 10.1016/S0273-1177(02)00661-0
11. Rouphael Y, Petropoulos SA, El-Nakhel C, Pannico A, Kyriacou MC, Giordano M, et al. Reducing energy requirements in future bioregenerative life support systems (BLSSs): performance and bioactive composition of diverse lettuce genotypes grown under optimal and suboptimal light conditions. Front Plant Sci. (2019) 10:1305. doi: 10.3389/fpls.2019.01305
12. Sridhar KR, Finn JE, Kliss MH. In-situ resource utilization technologies for Mars life support systems. Adv Sp Res. (2000) 25:249–55. doi: 10.1016/S0273-1177(99)00955-2
13. O’Handley D, Rice E, Gustafson R. ISRU support for a self-sustaining lunar colony. In: Proceedings of the 39th Aerospace Sciences Meeting and Exhibit. Reston: American Institute of Aeronautics and Astronautics (2001). doi: 10.2514/6.2001-937
14. Rygalov VY, Bucklin RA, Drysdale AE, Fowler PA, Wheeler RM. The potential for reducing the weight of a Martian greenhouse. In: Proceedings of the International Conference on Environmental Systems. Warrendale, PA: SAE (2001). doi: 10.4271/2001-01-2360
15. Benaroya H, Metzger P, Muscatello A. Special issue on in situ resource utilization. J Aerosp Eng. (2013) 26:1–4. doi: 10.1061/(ASCE)AS.1943-5525.0000282
16. Duri LG, El-Nakhel C, Caporale AG, Ciriello M, Graziani G, Pannico A, et al. Mars Regolith simulant ameliorated by compost as in situ cultivation substrate improves lettuce growth and nutritional aspects. Plants. (2020) 9:628. doi: 10.3390/plants9050628
17. Duri LG, Caporale AG, Rouphael Y, Vingiani S, Palladino M, De Pascale S, et al. The potential for lunar and Martian Regolith simulants to sustain plant growth: a multidisciplinary overview. Front Astron Sp Sci. (2022) 8:747821. doi: 10.3389/fspas.2021.747821
18. Cannon KM, Britt DT. Feeding one million people on mars. New Sp. (2019) 7:245–54. doi: 10.1089/space.2019.0018
19. Wheeler RM. Horticulture for Mars. Acta Hortic. (2004) 642:201–15. doi: 10.17660/ActaHortic.2004.642.22
20. Yoon GA, Yeum KJ, Cho YS, Chen CYO, Tang G, Blumberg JB, et al. Carotenoids and total phenolic contents in plant foods commonly consumed in Korea. Nutr Res Pract. (2012) 6:481. doi: 10.4162/nrp.2012.6.6.481
21. Lima GPP, Vianello F, Corrêa CR, Campos RA, da S, Borguini MG. Polyphenols in fruits and vegetables and its effect on human health. Food Nutr Sci. (2014) 05:1065–82. doi: 10.4236/fns.2014.511117
22. Kayama M, Nagaoka H, Niihara T. Lunar and Martian silica. Minerals. (2018) 8:267. doi: 10.3390/min8070267
23. Taylor LA, Pieters C, Patchen A, Taylor DHS, Morris RV, Keller LP, et al. Mineralogical and chemical characterization of lunar highland soils: insights into the space weathering of soils on airless bodies. J Geophys Res. (2010) 115:E02002. doi: 10.1029/2009JE003427
24. Ohtake M, Matsunaga T, Haruyama J, Yokota Y, Morota T, Honda C, et al. The global distribution of pure anorthosite on the Moon. Nature. (2009) 461:236–40. doi: 10.1038/nature08317
25. Grotzinger JP, Sumner DY, Kah LC, Stack K, Gupta S, Edgar L, et al. A habitable fluvio-lacustrine environment at Yellowknife Bay, gale crater, mars. Science. (2014) 343:1242777–1242777. doi: 10.1126/science.1242777
26. Zeng X, Li X, Wang S, Li S, Spring N, Tang H, et al. JMSS-1: a new Martian soil simulant. Earth Planets Sp. (2015) 67:72. doi: 10.1186/s40623-015-0248-5
27. Cannon KM, Britt DT, Smith TM, Fritsche RF, Batcheldor D. Mars global simulant MGS-1: a rocknest-based open standard for basaltic Martian Regolith simulants. Icarus. (2019) 317:470–8. doi: 10.1016/j.icarus.2018.08.019
28. Wamelink GWW, Frissel JY, Krijnen WHJ, Verwoert MR, Goedhart PW. Can plants grow on mars and the moon: a growth experiment on mars and moon soil simulants. PLoS One. (2014) 9:e103138. doi: 10.1371/journal.pone.0103138
29. Caporale AG, Vingiani S, Palladino M, El-Nakhel C, Duri LG, Pannico A, et al. Geo-mineralogical characterisation of Mars simulant MMS-1 and appraisal of substrate physico-chemical properties and crop performance obtained with variable green compost amendment rates. Sci Total Environ. (2020) 720:137543. doi: 10.1016/j.scitotenv.2020.137543
30. De Micco V, Aronne G, Colla G, Fortezza R, De Pascale S. Agro-biology for bioregenerative life support systems in long-term space missions: general constraints and the Italian efforts. J Plant Interact. (2009) 4:241–52. doi: 10.1080/17429140903161348
31. Ming DW. Manufactured soils for plant growth at a lunar base. In: Proceedings of the Lunar Base Agriculture: Soils for Plant Growth. Madison: American Society of Agronomy (2015). p. 93–105. doi: 10.2134/1989.lunarbaseagriculture.c7
32. Rickman D, Edmunson J, McLemore C. Functional comparison of lunar regoliths and their simulants. J Aerosp Eng. (2013) 26:176–82. doi: 10.1061/(ASCE)AS.1943-5525.0000223
33. Moya B, Parker A, Sakrabani R, Mesa B. Evaluating the efficacy of fertilisers derived from human excreta in agriculture and their perception in Antananarivo, Madagascar. Waste Biomass Valoriz. (2019) 10:941–52. doi: 10.1007/s12649-017-0113-9
34. Harder R, Wielemaker R, Larsen TA, Zeeman G, Öberg G. Recycling nutrients contained in human excreta to agriculture: pathways, processes, and products. Crit Rev Environ Sci Technol. (2019) 49:695–743. doi: 10.1080/10643389.2018.1558889
35. Krounbi L, Enders A, van Es H, Woolf D, von Herzen B, Lehmann J. Biological and thermochemical conversion of human solid waste to soil amendments. Waste Manag. (2019) 89:366–78. doi: 10.1016/j.wasman.2019.04.010
36. Romano I, Ventorino V, Pepe O. Effectiveness of plant beneficial microbes: overview of the methodological approaches for the assessment of root colonization and persistence. Front Plant Sci. (2020) 11:6. doi: 10.3389/fpls.2020.00006
37. El-Nakhel C, Giordano M, Pannico A, Carillo P, Fusco GM, De Pascale S, et al. Cultivar-specific performance and qualitative descriptors for butterhead salanova lettuce produced in closed soilless cultivation as a candidate salad crop for human life support in space. Life. (2019) 9:61. doi: 10.3390/life9030061
38. Chunxiao X, Hong L. Crop candidates for the bioregenerative life support systems in China. Acta Astronaut. (2008) 63:1076–80. doi: 10.1016/j.actaastro.2008.02.003
39. Wheeler RM. Agriculture for space: people and places paving the way. Open Agric. (2017) 2:14–32. doi: 10.1515/opag-2017-0002
40. Kuang A, Xiao Y, McClure G, Musgrave ME. Influence of microgravity on ultrastructure and storage reserves in seeds of Brassica rapa L. Ann Bot. (2000) 85:851–9. doi: 10.1006/anbo.2000.1153
41. Meinen E, Dueck T, Kempkes F, Stanghellini C. Growing fresh food on future space missions: environmental conditions and crop management. Sci Hortic (Amsterdam). (2018) 235:270–8. doi: 10.1016/j.scienta.2018.03.002
42. Dueck T, Kempkes F, Meinen E, Stanghellini C. Choosing crops for cultivation in space. In: Proceedings of the 46Th International Conference on Environmental Systems. Vienna: (2016).
43. Caporale AG, Sommella A, Lorito M, Lombardi N, Azam SMGG, Pigna M, et al. Trichoderma spp. alleviate phytotoxicity in lettuce plants (Lactuca sativa L.) irrigated with arsenic-contaminated water. J Plant Physiol. (2014) 171:1378–84. doi: 10.1016/j.jplph.2014.05.011
44. El-Nakhel C, Pannico A, Kyriacou MC, Giordano M, De Pascale S, Rouphael Y. Macronutrient deprivation eustress elicits differential secondary metabolites in red and green-pigmented butterhead lettuce grown in a closed soilless system. J Sci Food Agric. (2019) 99:6962–72. doi: 10.1002/jsfa.9985
45. El-Nakhel C, Petropoulos SA, Pannico A, Kyriacou MC, Giordano M, Colla G, et al. The bioactive profile of lettuce produced in a closed soilless system as configured by combinatorial effects of genotype and macrocation supply composition. Food Chem. (2020) 309:125713. doi: 10.1016/j.foodchem.2019.125713
46. Pannico A, El-Nakhel C, Kyriacou MC, Giordano M, Stazi SR, De Pascale S, et al. Combating micronutrient deficiency and enhancing food functional quality through selenium fortification of select lettuce genotypes grown in a closed soilless system. Front Plant Sci. (2019) 10:1495. doi: 10.3389/fpls.2019.01495
47. Chutichude B, Chutichude P, Kaewsit S. Influence of developmental stage on activities of polyphenol oxidase, internal characteristics and colour of lettuce cv. Grand Rapids. Am J Food Technol. (2011) 6:215–25. doi: 10.3923/ajft.2011.215.225
48. Goodwin TJ, Christofidou-Solomidou M. Oxidative stress and space biology an organ-based approach. Int J Mol Sci. (2018) 19:959. doi: 10.3390/books978-3-03842-904-3
49. Kyriacou MC, De Pascale S, Kyratzis A, Rouphael Y. Microgreens as a component of space life support systems: a cornucopia of functional food. Front Plant Sci. (2017) 8:1587. doi: 10.3389/fpls.2017.01587
50. Bates S, Gushin V, Bingham G, Vinokhodova A, Marquit J, Sychev V. Plants as countermeasures: a review of the literature and application to habitation systems for humans living in isolated or extreme environments. Habitation. (2009) 12:33–40. doi: 10.3727/154296610X12686999887201
52. Gilrain MR, Hogan JA, Cowan RM, Finstein MS, Logendra LS. Preliminary Study of Greenhouse Grown Swiss Chard in Mixtures of Compost and Mars Regolith Simulant. Warrendale, PA: SAE Technical Paper (1999). doi: 10.4271/1999-01-2021
53. Mortley DG, Aglan HA, Bonsi CK, Hill WA. Growth of Sweetpotato in Lunar and Mars Simulants. Warrendale, PA: SAE Technical Paper (2000). doi: 10.4271/2000-01-2289
54. Onsay D, Massa GD, Smith T. Researching Plant Growth in Amended Martian Regolith Simulant, Photosynthetic Rates of plants, Seed Surface Decontamination by Plasma methods, New Crop Development, and Porous Concrete Media. Florida, FL: NTRS – NASA Technical Reports Server. (2019).
55. Re R, Pellegrini N, Proteggente A, Pannala A, Yang M, Rice-Evans C. Antioxidant activity applying an improved ABTS radical cation decolorization assay. Free Radic Biol Med. (1999) 26:1231–7. doi: 10.1016/S0891-5849(98)00315-3
56. Brand-Williams W, Cuvelier ME, Berset C. Use of a free radical method to evaluate antioxidant activity. LWT Food Sci Technol. (1995) 28:25–30. doi: 10.1016/S0023-6438(95)80008-5
57. Kim HJ, Fonseca JM, Choi JH, Kubota C, Kwon DY. Salt in irrigation water affects the nutritional and visual properties of romaine lettuce (Lactuca sativa L.). J Agric Food Chem. (2008) 56:3772–6. doi: 10.1021/jf0733719
58. Kim MJ, Moon Y, Tou JC, Mou B, Waterland NL. Nutritional value, bioactive compounds and health benefits of lettuce (Lactuca sativa L.). J Food Compos Anal. (2016) 49:19–34. doi: 10.1016/j.jfca.2016.03.004
59. Bychkov A, Reshetnikova P, Bychkova E, Podgorbunskikh E, Koptev V. The current state and future trends of space nutrition from a perspective of astronauts’ physiology. Int J Gastron Food Sci. (2021) 24:100324. doi: 10.1016/j.ijgfs.2021.100324
60. Smith SM, Lane HW, Zwart SR. Spaceflight metabolism and nutritional support. In: Barratt MR, Baker ES, Pool SL editors. Principles of Clinical Medicine for Space Flight. (New York, NY: Springer New York) (2019). p. 413–39. doi: 10.1007/978-1-4939-9889-0_13
61. Caporale AG, Palladino M, De Pascale S, Duri LG, Rouphael Y, Adamo P. The suitability of lunar and martian soils for food plant growth. Part 1: the benefits of monogastric-based manure fertilization on chemical and physico-hydraulic properties. Submitt to Agric Ecosyst Environ. (2022).
62. Seiferlin K, Ehrenfreund P, Garry J, Gunderson K, Hütter E, Kargl G, et al. Simulating Martian Regolith in the laboratory. Planet Space Sci. (2008) 56:2009–25. doi: 10.1016/j.pss.2008.09.017
63. Duggan T, Jones P. Lettuce (Lactuca sativa ‘webb’s wonderful’) shoot and root growth in different grades of compost and vermicomposted compost. Acta Hortic. (2016) 1146:33–40. doi: 10.17660/ActaHortic.2016.1146.4
64. Caporale AG, Amato M, Duri LG, Bochicchio R, De Pascale S, Di Rauso Simeone G, et al. The suitability of Lunar and Martian soils for food plant growth. Part 2: the effects of monogastric-based manure fertilization on lettuce growth and physiology, soil enzymatic activity and nutrient bioavailability. Submitt Agric Ecosyst Environ. (2022).
65. Petropoulos SA, Fernandes Â, Plexida S, Pereira C, Dias MI, Calhelha R, et al. The sustainable use of cotton, hazelnut and ground peanut waste in vegetable crop production. Sustainability. (2020) 12:8511. doi: 10.3390/su12208511
66. Dai J, Mumper RJ. Plant phenolics: extraction, analysis and their antioxidant and anticancer properties. Molecules. (2010) 15:7313–52. doi: 10.3390/molecules15107313
67. Ordidge M, García-Macías P, Battey NH, Gordon MH, Hadley P, John P, et al. Phenolic contents of lettuce, strawberry, raspberry, and blueberry crops cultivated under plastic films varying in ultraviolet transparency. Food Chem. (2010) 119:1224–7. doi: 10.1016/j.foodchem.2009.08.039
68. Zhao XM, Wu YP, Wei R, Cai HX, Tornoe I, Han JJ, et al. Plasma surfactant protein d levels and the relation to body mass index in a Chinese population. Scand J Immunol. (2007) 66:71–6. doi: 10.1111/j.1365-3083.2007.01943.x
69. El-Nakhel C, Pannico A, Kyriacou MC, Petropoulos SA, Giordano M, Colla G, et al. Dataset on the organic acids, sulphate, total nitrogen and total chlorophyll contents of two lettuce cultivars grown hydroponically using nutrient solutions of variable macrocation ratios. Data Br. (2020) 29:105135. doi: 10.1016/j.dib.2020.105135
70. Nazaryuk VM, Klenova MI, Kalimullina FR. Ecoagrochemical approaches to the problem of nitrate pollution in agroecosystems. Russ J Ecol. (2002) 33:392–7. doi: 10.1023/A:1020995329784
71. Pannico A, Graziani G, El-Nakhel C, Giordano M, Ritieni A, Kyriacou M, et al. Nutritional stress suppresses nitrate content and positively impacts ascorbic acid concentration and phenolic acids profile of lettuce microgreens. Italus Hortus. (2020) 27:41–52. doi: 10.26353/j.itahort/2020.3.4152
72. El-Nakhel C, Pannico A, Graziani G, Kyriacou MC, Gaspari A, Ritieni A, et al. Nutrient supplementation configures the bioactive profile and production characteristics of three Brassica L. microgreens species grown in peat-based media. Agronomy. (2021) 11:346. doi: 10.3390/agronomy11020346
73. Petropoulos SA, Constantopoulou E, Karapanos I, Akoumianakis CA, Passam HC. Diurnal variation in the nitrate content of parsley foliage. Int J Plant Prod. (2011) 5:431–8.
74. Zhang J, Amonette JE, Flury M. Effect of biochar and biochar particle size on plant-available water of sand, silt loam, and clay soil. Soil Tillage Res. (2021) 212:104992. doi: 10.1016/j.still.2021.104992
75. López-Bucio J, Nieto-Jacobo MF, Ramıìrez-Rodrıìguez V, Herrera-Estrella L. Organic acid metabolism in plants: from adaptive physiology to transgenic varieties for cultivation in extreme soils. Plant Sci. (2000) 160:1–13. doi: 10.1016/S0168-9452(00)00347-2
76. Osmolovskaya N, Viet Vu D, Kuchaeva L. The role of organic acids in heavy metal tolerance in plants. Biol Commun. (2018) 63:9–16. doi: 10.21638/spbu03.2018.103
77. Petropoulos SA, Fernandes Â, Dias MI, Pereira C, Calhelha R, Gioia FD, et al. Wild and cultivated Centaurea raphanina subsp. Mixta: a valuable source of bioactive compounds. Antioxidants. (2020) 9:1–23. doi: 10.3390/antiox9040314
78. El-Nakhel C, Geelen D, De Paepe J, Clauwaert P, De Pascale S, Rouphael Y. An appraisal of urine derivatives integrated in the nitrogen and phosphorus inputs of a lettuce soilless cultivation system. Sustainability. (2021) 13:4218. doi: 10.3390/su13084218
79. Petropoulos SA, Fernandes Â, Dias MI, Pereira C, Calhelha RC, Chrysargyris A, et al. Chemical composition and plant growth of Centaurea raphanina subsp. Mixta plants cultivated under saline conditions. Molecules. (2020) 25:1–26. doi: 10.3390/molecules25092204
80. Petropoulos S, Fernandes Â, Karkanis A, Antoniadis V, Barros L, Ferreira I. Nutrient solution composition and growing season affect yield and chemical composition of Cichorium spinosum plants. Sci Hortic (Amsterdam). (2018) 231:97–107. doi: 10.1016/j.scienta.2017.12.022
81. Ayaz FA, Glew RH, Millson M, Huang HS, Chuang LT, Sanz C, et al. Nutrient contents of kale (Brassica oleraceae L. var. acephala DC.). Food Chem. (2006) 96:572–9. doi: 10.1016/j.foodchem.2005.03.011
82. Poyrazoǧlu E, Gökmen V, Artιk N. Organic acids and phenolic compounds in pomegranates (Punica granatum L.) grown in Turkey. J Food Compos Anal. (2002) 15:567–75. doi: 10.1006/jfca.2002.1071
83. Rodríguez-Bernaldo de Quirós A, Frecha-Ferreiro S, Vidal-Pérez AM, López-Hernández J. Antioxidant compounds in edible brown seaweeds. Eur Food Res Technol. (2010) 231:495–8. doi: 10.1007/s00217-010-1295-6
84. Sánchez-Mata MC, Cabrera Loera RD, Morales P, Fernández-Ruiz V, Cámara M, Díez Marqués C, et al. Wild vegetables of the Mediterranean area as valuable sources of bioactive compounds. Genet Resour Crop Evol. (2012) 59:431–43. doi: 10.1007/s10722-011-9693-6
85. Hernández V, Ángeles Botella M, Hellín P, Cava J, Fenoll J, Mestre T, et al. Phenolic and carotenoid profile of lamb’s lettuce and improvement of the bioactive content by preharvest conditions. Foods. (2021) 10:188. doi: 10.3390/foods10010188
86. Ouzounis T, Razi Parjikolaei B, Fretté X, Rosenqvist E, Ottosen C-O. Predawn and high intensity application of supplemental blue light decreases the quantum yield of PSII and enhances the amount of phenolic acids, flavonoids, and pigments in Lactuca sativa. Front Plant Sci. (2015) 6:19. doi: 10.3389/fpls.2015.00019
87. Kołton A, Wojciechowska R, Długosz-Grochowska O, Grzesiak W. The storage ability of lamb’s lettuce cultivated in the greenhouse under led or HPS lamps. J Hortic Res. (2014) 22:159–65. doi: 10.2478/johr-2014-0033
88. Cruz R, Baptista P, Cunha S, Pereira JA, Casal S. Carotenoids of lettuce (Lactuca sativa L.) grown on soil enriched with spent coffee grounds. Molecules. (2012) 17:1535–47. doi: 10.3390/molecules17021535
Keywords: in situ resource utilization (ISRU), space farming, mars and lunar simulants, organic amendment, antioxidant activity, carotenoids, phenolic compounds, Orbitrap LC-MS/MS
Citation: Duri LG, Pannico A, Petropoulos SA, Caporale AG, Adamo P, Graziani G, Ritieni A, De Pascale S and Rouphael Y (2022) Bioactive Compounds and Antioxidant Activity of Lettuce Grown in Different Mixtures of Monogastric-Based Manure With Lunar and Martian Soils. Front. Nutr. 9:890786. doi: 10.3389/fnut.2022.890786
Received: 06 March 2022; Accepted: 31 March 2022;
Published: 29 April 2022.
Edited by:
Kathleen L. Hefferon, Cornell University, United StatesReviewed by:
Ki-Ho Son, Gyeongsang National University, South KoreaCopyright © 2022 Duri, Pannico, Petropoulos, Caporale, Adamo, Graziani, Ritieni, De Pascale and Rouphael. This is an open-access article distributed under the terms of the Creative Commons Attribution License (CC BY). The use, distribution or reproduction in other forums is permitted, provided the original author(s) and the copyright owner(s) are credited and that the original publication in this journal is cited, in accordance with accepted academic practice. No use, distribution or reproduction is permitted which does not comply with these terms.
*Correspondence: Youssef Rouphael, youssef.rouphael@unina.it
Disclaimer: All claims expressed in this article are solely those of the authors and do not necessarily represent those of their affiliated organizations, or those of the publisher, the editors and the reviewers. Any product that may be evaluated in this article or claim that may be made by its manufacturer is not guaranteed or endorsed by the publisher.
Research integrity at Frontiers
Learn more about the work of our research integrity team to safeguard the quality of each article we publish.