- 1Food Science and Technology Programme, National University of Singapore, Singapore, Singapore
- 2Nestlé Research, Singapore, Singapore
- 3Nestlé Research, Beijing, China
- 4Nestlé Institute of Health Sciences, Nestlé Research, Société des Produits Nestlé S.A., Lausanne, Switzerland
The human intestinal microbiota has been shown to be modulated during inflammatory conditions. Probiotic administration has been shown to affect the immune system and cytokine expression which can affect inflammation and health outcomes. There seems to be an association between the mother's intestinal microbiota and inflammation biomarkers, both of which may contribute to newborn early life immune and metabolic programming and impact short and long-term health outcomes. Probiotic supplementation during pregnancy has been shown to influence metabolic health, immunity, and gastrointestinal health of the mother, and can also have carry-over benefits to infants such as infant allergy risk reduction. Therefore, this review focuses on the evidence of probiotic administration in women of reproductive age, including during pregnancy and its impact on inflammatory markers and on maternal and infant health. We performed a PubMed search for articles published in English in the last 20 years. Immune markers were narrowed to serum and breast milk levels of TNF-α, IL-6 and TGF-β, IgA, and IL-10. Studies that investigated the beneficial effects of interventions in women with gestational diabetes mellitus, polycystic ovarian syndrome, and infant allergy management are summarized. These results show a beneficial or neutral effect on selected health outcomes and that it is safe for woman and their infants. The effect of probiotics on modulation of inflammatory markers was probiotic specific. More research is needed to further our understanding of the mechanisms underlying the effects of probiotics on inflammation and how these effects improve health outcomes.
Introduction
Globally, women suffer from autoimmune diseases and inflammatory diseases more often than men; level of estrogen, puberty, pregnancy, and menopause are associated with the increased risk (1). During pregnancy, immunological changes can occur at the placental interface to inhibit the rejection of the fetus. Simultaneously, at the mother's mucosal surface, there can be elevated inflammatory responses which can result in autoimmune diseases (2). The mother's intestinal microbiota, body weight and metabolic biomarkers appear to be interlinked and these can contribute to the seeding of a healthy microbiota of the newborn at birth and may affect the infant's health (3). In women of reproductive age, probiotics have been hypothesized to be beneficial for non-pregnancy related diseases linked to an inflammatory milieu such as Polycystic Ovarian Syndrome (PCOS) (1).
Successful pregnancies require a robust, dynamic and responsive immune system and are primarily dependent on the coordinated balance between the invading trophoblast and receptive maternal decidua (4). Consequently, the decidual immune cells must be optimized to support the development of the fetal-placental unit. These immune cells including macrophages, Natural Killer (NK) cells and T cells, contribute to the establishment of an anti-inflammatory microenvironment and play their respective roles to modulate the adaptive and innate immune system (5). Hence, pregnancy begins in a pro-inflammatory environment that allows for implantation and placentation and subsequently, shifts to an anti-inflammatory stage that facilitates fetal growth and lastly, back to a pro-inflammatory state that ends with labor and delivery (4). The change in states is primarily due to the balance of T helper cells shifting from a pro-inflammatory Th1 profile to a Th2 profile. These responses are also antagonistic to each other which means that a strong Th1 response would tend to have a low Th2 response and vice versa (6). Disruptions in these responses, such as a sudden alteration in cytokine levels and profiles, have the potential to cause spontaneous births or abortions (7).
In recent years, there has been increasing interest in the concept of modulating the gut microbiota to contribute to improving inflammatory states, immunity, and autoimmune diseases. One way to modulate the gut microbiota is through the administration of specific probiotic strains associated with benefits on immunity and overall health. Probiotics are defined by the Food and Agriculture Organization (FAO) of the United Nations as live microorganisms that, when administered in sufficient amounts, can confer a health benefit to the host. Probiotics interact with the Gastrointestinal (GI) mucosa and Gut Associated Lymphoid Tissue (8). These interactions can evoke immune activation signaling or tolerance signaling through the stimulation of cytokine production.
This paper aims to review the evidence about how probiotic administration can help with autoimmune diseases in women of reproductive age, including pregnant and lactating women in relation to changes in cytokine levels and profiles, and the potential effects on the health of women and their children.
Probiotics and Their Immunomodulatory Properties
Probiotic benefits are strain-specific which means that different strains and dosages elicit different effects on health outcomes, and that there is no universal strain that can address all health outcomes. Among other probiotics, specific Lactobacillus, Lacticaseibacilus and Bifidobacterium strains have been well-documented in terms of their safety and conferred health benefits. However, modulation of the gut microbiome for health improvement is not always straightforward, as supplementation with one or two probiotic strains is not always associated with detectable changes in microbial composition or activity. Yet, supplementation with probiotics has been suggested to evoke numerous immune benefits through signaling pathways, cytokine and anti-oxidative stress marker expression.
Probiotic bacteria can help induce the secretion of cytokines from intestinal epithelial cells in a strain-specific and dose-dependent manner (9). Ingested probiotics have been reported to interact with enterocytes and dendritic cells, Th1, Th2, and regulatory T cells (Treg) in the intestine. These probiotics must encounter macrophages and dendritic cells to induce the production of anti- or pro-inflammatory cytokines which can evoke subsequent immune signaling pathways. It has been suggested that probiotics can reduce inflammation by stimulating anti-inflammatory cytokines and decreasing pro-inflammatory cytokines which can, in turn, modulate NK cells' activity, inhibit Toll-Like Receptors (TLR) and subsequently, the Nuclear Factor- Kappa B (NF-κB) pathway (10).
While the mechanism of probiotics and their effects on the immune system are not entirely understood, this section aims to summarize the suggested mechanisms and their immunomodulatory effects (Figure 1).
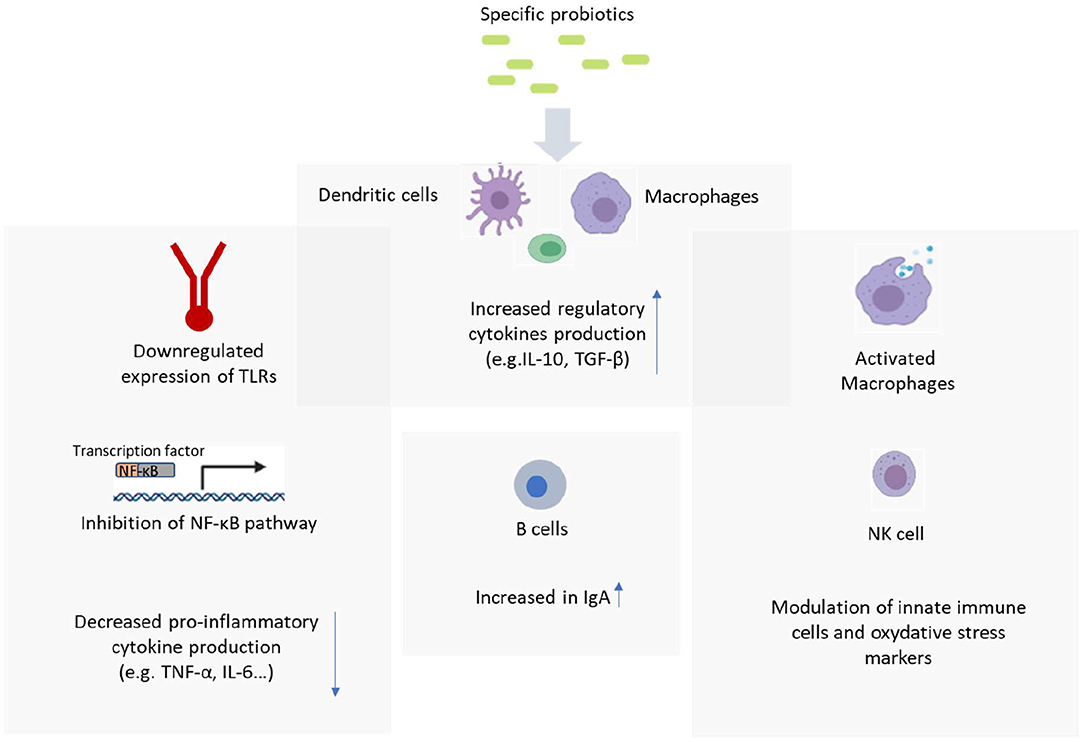
Figure 1. Probiotic mechanism of action. Figure created with BioRender.com.
Inhibition of TLR
Toll-Like Receptors (TLR) are a family of Pattern Recognition Receptors (PRRs) that can recognize a wide range of microbial components. They consist of 11 different proteins (TLR 1—TLR11). The binding of TLR ligands to TLRs can activate the TLR signaling pathway which under certain conditions can exert pro-inflammatory effects (11). TLRs can recognize molecular patterns and activate inflammatory mediators which include the proteins involved in the transcription and signaling of NF-kB and the expression of other TLR ligands (12). TLR ligands are specific in their interaction with TLRs. For example, Lipopolysaccharide (LPS) is specific to TLR 2/4 whereas Flagellin is specific to TLR 5 found on the plasma membrane. TLRs can also be located intracellularly such as TLR 9 in the endosome which is responsive to the DNA as ligands (13). The gut epithelial lining must have a high tolerance to TLR ligands as epithelial cells express minimal TLRs (14), but in states of inflammation and altered gut microbiota, TLRs can be activated and responsive to such ligands to promote further inflammation and oxidative stress and lead to diseases.
Probiotics have been shown to suppress intestinal inflammation via the downregulation of TLR expression. Depending on the type of TLR, the reduced expression of TLR can lead to various benefits such as reduced NF-κB activity and other pro-inflammatory expressions (11). Although not yet fully understood, the probiotic(s) used have different interactions in the activity and expression of TLRs. For example, Rautava et al. found that the gene expression of TLRs 1 and 7 was significantly decreased in the placenta whereas TLR3 increased when women received B. lactis. However, supplementation with B.lactis combined with L. rhamnosus GG resulted in a significant decrease only in TLR1 mRNA. There were also carry-over effects to the infants where there was reduced TLR7 mRNA in those whose mothers received B. lactis whereas the combination of B. lactis and L. rhamnosus GG saw a decrease in TLR6 mRNA in the infant intestine (15). Hence, it was concluded that probiotics could develop and strengthen the mucosal immune system managing the inflammatory response. Another study showed an interplay between TLR Single Nucleotide Polymorphisms (SNPs) and the efficacy of L. rhamnosus HN001 or B. animalis subsp. lactis HN019 at reducing the risk of eczema in children compared to placebo (16).
Inhibition of the NF-κβ Pathway
NF-κB is a family of transcription factors composed of five members—p65, REL-B, cytoplasmic REL, p50, and p52. Activation of NF-κB involves the phosphorylation and proteolysis of the IκB proteins and the concomitant release and nuclear translocation of the NF-κB factors (17). NF-κB is paramount in controlling the innate and adaptive immune system and is responsive to a wide range of pathogenic stimuli and pro-inflammatory cytokines such as Tumor Necrosis Factor (TNF) and Interleukin-1 (IL-1). These cytokines are highly activated at sites of inflammation in inflammatory bowel disease and metabolic diseases. Activation of NF-κB proteins induces the transcription of more pro-inflammatory cytokines such as IL-6, chemokines, and adhesion molecules which can potentially lead to the onset of inflammatory diseases. IL-6 is a key cytokine of the acute inflammatory response (18). Probiotics can downregulate inflammation by inhibiting various signaling pathways such as the NF-κB pathway which also relates to mitogen-activated protein kinases (MAPK) and PRR pathways (19). Specific strains of probiotics can reduce the binding of NF-κB to the DNA by inhibiting Ikb-βα or ubiquitination phosphorylation and NF-κβ inhibitor degradation and reduce the nuclear translocation of p65. Furthermore, certain probiotics can also inhibit the binding of the LPS to the CD14 receptor, leading to the overall reduction in NF-κβ activity and pro-inflammatory cytokines (20).
Modulation of Innate Immune Cells
Natural Killer cells are key cells of the innate immune system and are made up of both NK and NK T cells. NKs can distinguish between healthy and abnormal cells and upon recognition, can elicit the secretion of immune mediators such as cytokine IFN-γ for direct cytolysis of the abnormal cells (21). Together with other phagocytic cells, they prevent infections by forming a barrier against pathogenic microorganisms (22). Being innate, they are non-specific in their recognition of antigens. They also have an innate memory (immune training) and can remember previous pathogenic encounters when attacking again. During pregnancy, decidua NK cells (dNK) dominate the uterine lining and produce a range of angiogenic factors to facilitate vascular stability and function, probably to guarantee arterial remodeling toward the growing fetus (23). These dNKs beyond their role on immune function can also promote a healthy pregnancy and prevent spontaneous abortions. Probiotic strains can increase the cytotoxic potential, proliferation and activation of the NK cells via interactions with dendritic cells (24). In a study by Ortiz-Andrellucchi et al., the administration of L. casei DN for 6 weeks significantly increased Absolute Variation (AV) of NK cells (CD3−CD56+) when measured at 10 and 45 days postpartum in peripheral blood treatment (AV: 18.5 cells/μl and −13 cells/μl for L. casei and control groups, respectively; P = 0.026) (22).
Antigen Presenting Cells (APC) are responsible for the maintenance of immune homeostasis in the GI tract. They are typically made up of Dendritic Cells (DCs), macrophages and other monocytes. APCs produce cytokines and chemokines required for T cell replication, differentiation and response which in turn, initiate antigen-specific immune responses toward potential dangers (25). Such cytokines include IL-12 which causes the differentiation and polarization of Th1 responses and induces cell-mediated immunity such as phagocytosis. Dendritic cells exist throughout the intestine and allow antigen internalization and presentation (25). It has been suggested that probiotics are internalized into APCs to induce maturation which allows them to secrete cytokines for T-cell activation (26). More recently, it has been demonstrated that probiotics (and heat-treated probiotics) could modulate monocyte micro- RNA expression, as a mechanism by which the immunomodulatory cytokine IL-10 was overexpressed through stabilization of the mRNA (27). IL-10 is a regulatory cytokine that has been shown to be modulated by probiotic intervention. Another regulatory cytokine includes TGF-beta. These regulatory cytokines help reduce the initiation or the chronicity of the inflammation by downregulating pro-inflammatory cytokine production.
Impact on Oxidative Stress Markers
Oxidative stress can be defined as the imbalance between oxidants and antioxidants in favor of oxidants which leads to subsequent disruptions in redox signaling, modulation and molecular damage. Oxidative stress has been associated with a wide variety of non-communicable diseases and is dependent on oxidation-reduction (redox) homeostasis. Oxidative stress has been shown to play a role in the pathogenesis of autoimmune diseases by downregulating immune tolerance cell number and activity. It is induced by a range of intrinsic factors, foods and environmental factors which generates reactive species such as reactive oxygen species (ROS) and reactive nitrogen species (RNS). Typically, the body can regulate this via transcription factors or through reversible protein oxidation reactions, creating a balance between eustress and distress signals. Oxidative stress can be measured using stress markers such as Nitric Oxide (NO), C Reactive Protein (hs-CRP), Glutathione (GSH), Malondialdehyde (MDA), or by measuring the Total Antioxidant Capacity (TAC) which measures the amount of free radicals scavenged.
Nitric Oxide (NO) mediates the relaxation of blood vessels and in mediating oxidative eustress. NO can improve blood pressure, endothelial function and provide other cardiovascular health benefits (28). However, excessive NO production becomes noxious and can induce oxidative damage by forming RNS that can subsequently result in DNA and cellular damage, axonal degeneration and other neurogenerative disorders (29). Probiotics have been suggested to improve endothelial function by modulating the intestinal microbiota and the generation of reactive species and NO bioavailability. This can reduce the risk of cardiovascular diseases and other metabolic dysfunctions (30).
CRP is another marker of systemic inflammation playing a role in innate immunity. They are primarily synthesized in the liver but can also be found in various other cells such as smooth muscle cells, endothelial cells and macrophages. There is growing evidence that CRP plays a role in the inflammatory process through the production of NO and pro-inflammatory cytokines such as IL-6 and TNF-α. Elevated levels of CRP have been found in pathophysiological complications such as insulin resistance, metabolic dysfunctions and chronic inflammatory diseases such as cardiovascular diseases, diabetes and aspects of metabolic syndrome. Probiotics have been suggested to modulate CRP levels via an increase in production of Short Chain Fatty Acids (SCFA) in the colon, decreased expression of pro-inflammatory cytokines such as IL-6, and increasing levels of antioxidants and scavengers such as glutathione (GSH) (31, 32).
Glutathione is an antioxidant scavenger that can prevent damage to cellular components such as DNA and lipids by rendering reactive species such as ROS and RNS inactive. They protect such components against free radicals, peroxides, and heavy metals. GSH is known as a master antioxidant and participates in both the antioxidant defense system and metabolic processes (33). Hence, GSH deficiency or imbalance leads to a wide range of pathological and non-communicable diseases such as diabetes. It has been shown that synbiotics and probiotics can increase the synthesis and secretion of GSH through the production of SCFAs (34) and reduced expression of pro-inflammatory cytokines and activity of TLRs (35).
Malondialdehyde (MDA) is a lipid oxidative stress marker and can be formed through lipid peroxidation reactions. Free radicals can oxidize linoleic or arachidonic acid (AA) found to form aldehydes such as MDA. Hence, elevated levels of MDA occur in disease and complications due to oxidative stress (36). The effects of probiotic and synbiotic administration can decrease MDA levels and these changes might be linked to the improved serum lipid profiles. This can include cholesterol-lowering effects, reduced absorption of intestinal cholesterol and modulation of lipid metabolism (37).
Methods
This study aims to investigate the effects of the probiotic intervention on inflammation markers in women and its impact on health outcomes in women and their children. We assessed papers reporting pro-inflammatory and anti-inflammatory cytokines measured in the mother. These indicators were typically measured in the serum and plasma found in the blood and maternal milk during lactation. To narrow the scope of the study, we selected the markers TNF-α, IL-6 but also IgA, and the regulatory cytokines TGF- β, and IL-10 (Figure 2).
All identified English articles published in PubMed on human intervention with probiotics were reviewed. We used a search strategy in accordance with MeSH terminology and used the following search strategy (probiotic* OR synbiotic* OR symbiotic* OR lactobacilli* OR streptococci* OR bifidobacteria* OR saccharum* OR yeast OR yogurt OR bacteria* OR acidophilus OR ferment* OR microorganism*) AND (diet OR supplement OR intake OR consume) AND (preconcept* OR pregnant* OR gestation* OR matern* OR obstetric* OR expectant* OR women) AND (random* OR trial* OR placebo OR blind*). There was a total of 1,931 search results between the search period of 2001 to 2020 where 342 were deemed relevant after reading the abstract. Upon further filtering of the results, 20 publications were focused on probiotic usages and their effects on maternal inflammation markers.
This study reviewed all randomized controlled trials studying the effects of probiotics in pregnant women or women of reproductive ages (15–49 years old) that were published in English. All formats of probiotic intakes, such as product supplementation or dietary fortification were included. We excluded articles that included solely prebiotic consumption and women of menopausal ages (>49 years old; Figure 3).
Results on probiotic administration in women of reproductive age or during pregnancy are shown for inflammatory markers (Table 1) and on maternal and infant health and reported across the studies for benefits related to gestational diabetes mellitus, polycystic ovarian syndrome, and infant allergy management.
Probiotic Administration In Women Of Reproductive Age
Clinical Evidence on Inflammatory Markers
Meyer et al. administered a probiotic yogurt enriched with L. casei DN 114 001 at 3.7 × 108/ml and a conventional yogurt on healthy women between 22 and 29 years old for 4 weeks (n = 33) (49). Participants were asked to consume 100 g of yogurt for the first 2 weeks then 200 g of yogurt for the next 2 weeks. Here, there was a significant increase in TNF-α levels during the first 2 weeks of intake in the conventional and probiotic yogurt group and remained higher than baseline in the next 2 weeks. IL-10 showed a significant decrease after the probiotic yogurt consumption during the first 2 weeks but was not significantly different from the conventional yogurt group at the final evaluation. IL-6 showed no significant difference during the study period. Results concluded that both conventional and probiotic enriched yogurt enhanced the stimulated production of pro-inflammatory cytokines by myeloid cells such as macrophages and NK cells. Asemi et al. administered a probiotic yogurt enriched with L. acidophilus La5 and B. lactis Bb12 a total of 200 × 107 CFU/day on pregnant women (n = 70) for 9 weeks during the 3rd trimester of pregnancy (57). Results showed that the probiotic yogurt had no significant effects on serum TNF-α. Here results are thus conflicting, and more study are needed to reach a conclusion.
Despite probiotic yogurts exhibiting minimal changes in immunomodulatory markers, it still appears useful in terms of immune function. A study by Meyer et al. has shown that higher cytolytic activity of NK cells has been exhibited after yogurt consumption which can explain better defense against pathogenic microorganisms, protection against infections and acute inflammation (58).
Lastly, studies showed that oral probiotic administration may be ineffective in modulating vaginal cytokine profiles. Yang et al. administered L. rhamnosus GR-1 and L. reuteri RC-14 at 5.0 × 109 CFU each for 12 weeks on asymptomatic pregnant women (before 17 weeks of gestation) who had an Intermediate or Bacterial Vaginosis Nugent score at 13 weeks (n = 86) (51). Results showed that there were no significant differences in levels of IL-10, TNF-α, and IL-6 at the end of the intervention in vaginal samples. It was hypothesized that this could have been due to insufficient dosages of probiotics to displace the indigenous lactobacilli, resulting in minimal changes in microbiota function and potentially no changes in the inflammation markers. A study in Italy conducted for 4 weeks from week 33–37 weeks of gestation also administered VSL#3 at 9 × 1011 CFU/capsule per day on healthy women (n = 27) and saw no significant differences in TNF-α, IL-6, and IL-10 at the end of the intervention in vaginal samples (53). One reason suggested was that VSL#3 could have contained too many species of organisms and in turn, canceled the stimulatory effect of other constituents (59). Another study by Singh et al. also administered VSL#3 at 112.5 × 109 CFU/capsule twice a day in healthy non-pregnant women (n = 22) for 4 weeks results showed that there were no significant differences in levels of TNF-α, IL-6, and IL-10 at the end of the intervention (52).
Results in health and disease for the modulation of inflammatory markers are still highly conflicting. Modulation of TNF-α and IL-6 or the regulatory cytokine IL-10 may depend on the specificity of the probiotics, the health or disease state and the timing of the intervention. Further studies are thus needed to confirm the relevance of these markers as a proxy for probiotic clinical response in human clinical trials.
Polycystic Ovarian Syndrome and Gestational Diabetes Mellitus
PCOS affects both reproductive and metabolic functions, causing infertility, obesity and insulin resistance in women. Similar to metabolic syndrome, it is characterized by a constellation of symptoms which consist of hyperandrogenism, ovarian dysfunction, and polycystic ovarian morphology (60). It is also associated with metabolic disorders such as insulin resistance (61), type 2 diabetes, and other cardiovascular diseases (62). PCOS is caused by the interaction between numerous genes which affect neuroendocrine and metabolic functions, inducing metabolic endotoxemia and circulation of LPS, causing low-grade chronic inflammation, cardiometabolic changes, increased NF-KB activation, and oxidative stress (63). PCOS has been shown to chronically activate the immune system, regardless of obesity and recent studies have suggested that the gut microbiota can be a potential pathogenic factor in the development of PCOS.
A randomized, double-blinded and placebo-controlled conducted in Iran in 2018 administered L. acidophilus, L. plantarum, L. fermentum, and L. gasseri at 2 × 109 CFU of each strain per day for 12 weeks to women who suffered from PCOS (n = 60) (48). Results showed that serum IL-10 significantly increased in the group with probiotic supplementation. However, serum TNF-α showed no significant differences and IL-6 showed a significant decrease in both groups. IL-10 showed a significant increase in the probiotics group as compared to the placebo group. Another trial with the same combination of probiotics at 2 × 109 CFU per strain, co-administered with 200 μg/day selenium as selenium yeast, showed significant reductions in weight, serum insulin levels and homeostatic model of assessment for insulin resistance and a significant increase in glucose and insulin (64). It also significantly decreased serum triglycerides and LDL levels. While more research is needed, evidence may suggest that these 4 lactobacilli probiotic strains can be useful in modulating inflammation markers and help with metabolic dysfunctions but other studies need to confirm these findings.
Similar to other chronic diseases, it is unclear whether the gut microbiota dysbiosis causes PCOS or the other way round (65). However, the intestinal microbiota plays a role in PCOS pathogenesis reason why, probiotic usage could be a nutritional intervention to help prevent or manage PCOS. Evidence has shown to be useful in managing the metabolic profiles in women with PCOS. A meta-analysis in 2020 concluded that synbiotics and probiotics can improve hormonal and inflammatory indices in this population (66). Such inflammatory indices typically contribute to impaired glucose tolerance, insulin sensitivity and other complications. Synbiotic and probiotic administration can modulate glucotoxicity and manage the pathophysiological complications that come with PCOS.
GDM is defined as any form of glucose intolerance during pregnancy. During pregnancy, metabolic changes occur that cause adipose tissue accretion and the gradual development of insulin resistance to facilitate fetal nutrition (67). It has become a rising concern as GDM is a risk factor for developing other complications such as hypertension, preeclampsia, type 2 diabetes, and other cardiometabolic diseases. High glucose levels stimulate pro-inflammatory effects by inducing TLR activation by causing an increase in TLR2 and TLR4 expression, which ultimately results in the NF-kB activation and further pro-inflammatory cytokine secretion (12). The increased TLR4 expression also activates NF-κB and mitogen-activated protein kinase (MAPK) signaling pathway, leading to the increased transcription of genes involved in inflammation and this results in the feed-forward loop of inflammation levels. This elevated level of inflammation can result in insulin secretion impairment and insulin resistance, leading to metabolic inefficiencies and ultimately, the development of GDM. GDM results in elevated glucose levels and often remains unregulated. Hence, it has recently been of interest to investigate strategies to bring down these elevated levels of inflammation to help with GDM risk and management. Probiotics can regulate the secretion of pro-inflammatory mediators bringing down the risk of insulin resistance and thus the onset of GDM (68).
TNF-α is a pro-inflammatory cytokine that is a major regulator of the NF-kB and MAPK pathways. These cytokines are capable of promoting the proliferation of several cells and signals apoptosis, inevitably playing a part in immunity (69). Interleukin-6 (IL-6) is another pro-inflammatory cytokine that mediates interactions between the immune system and the Central Nervous System (CNS) and is disruptive to its functions (70). It has been suggested to be associated with GDM due to its diabetogenic actions and can interfere with normal insulin resistance (71). Lastly, IL-10 is regarded as an anti-inflammatory cytokine that is essential in maintaining the immune integrity and homeostasis of tissue epithelial layers. Other suppressive cytokines that inhibit pro-inflammatory responses such as lesion production for cardiovascular health. These cytokines are produced by a wide variety of cells including leukocytes, macrophages, dendritic cells, NK cells and T cells to help control acute and chronic inflammation either by regulating other immune responses such as T cell response or by modulating secretion and expression of pro-inflammatory cytokines (72).
As the development of PCOS and GDM involves a multitude of factors such as insulin resistance and chronic low-grade inflammation, one can hypothesize that pro-inflammatory cytokines play a role in the onset of metabolic diseases (73). Several studies have shown that probiotics can regulate TNF-α, IL-6, and IL-10 which are associated with metabolic dysfunctions.
Administration of L. acidophilus La5, B. lactis Bb12, S. thermophilus STY-31, and L. delbrueckii bulgaricus LBY-27 at a sum of at least 4 × 109 CFU/day in women diagnosed with GDM, for 8 weeks during pregnancy (n = 64) (56) showed significantly lower serum TNF-α in the probiotic group as compared to the placebo. However, there were no significant differences between the 2 groups in serum IL-6 levels. Another study in 2016 administered VSL#3 containing 112.5 × 109 CFU/capsule of eight strains; S. thermophilus, B. breve, B. longum, B. infantis, L. acidophilus, L. plantarum, L. paracasei, and L. delbrueckii subsp. bulgaricus (50). The administration of VSL#3 was conducted in women with GDM for 8 weeks (n = 82). Here, the probiotic administration resulted in a significant decrease in levels of TNF-α (−0.62 ± 1.0 vs. 0.45 ± 0.8 pg/mL; p = 0.04) and IL-6 (−0.44 ± 0.5 vs. 0.33 ± 0.42 pg/mL; p = 0.04) as compared to the placebo. However, IL-10 showed no significant difference between the probiotic group and the placebo group (0.74 ± 4.4 vs. −0.4 ± 5.1; p = 0.54).
Despite observed inconsistencies in changes in the cytokine profiles after probiotic administration, the evidence suggests that probiotic administration is safe and as far as efficacy, it is either neutral or beneficial. Furthermore, it is probably not just the changes in cytokine levels that contribute to these beneficial changes. A meta-analysis in 2020 showed that the reduction of fasting glucose in pregnant women without GDM using a range of probiotics is very small and by itself may not be clinically relevant. Furthermore, probiotic administration did not reduce the incidence of GDM (65). However, it has been shown that probiotics can modulate gestational glucose haemostasis and reduce glucotoxicity by increasing insulin sensitivity (74). Hence, probiotics as a unique approach may not be a meaningful strategy in the prevention of GDM but could be considered as part of more holistic approaches to improve glycemic response and glucose metabolism in women. We hypothesize that probiotics may be useful to reduce the risk of GDM when used in conjunction with other strategies such as dietary interventions, obesity management or when used in combination with other bioactives. Lastly, a recent meta-analysis showed that probiotic intervention in GDM women can improve maternal HDL-cholesterol and markers of inflammation and oxidative stress, and can reduce the incidence of hyperbilirubinemia in the newborn (75). Hence, probiotic administration may be beneficial in pregnant women with GDM to manage GDM complications and improve some infant outcomes, however further research is needed.
While not many studies have investigated probiotics for GDM or PCOS prevention, probiotic/synbiotic administration seems to benefit glycemic profiles, thus reducing a risk factor for metabolic complications and potentially related diseases such as GDM and PCOS while proving safe for consumption even during pregnancy. As the onset of GDM or PCOS typically includes a multitude of factors, managing one risk factor through probiotic administration may still be beneficial.
Infant Allergy Risk Reduction
Breast milk composition can help with infant intestinal inflammatory conditions and the reduction of risk of allergic diseases (76). Secretory Immunoglobulin A (IgA) can help maintain humoral immunity by binding to antigens, thereby limiting their access to the epithelium (77). It has been shown that infants are unable to produce their protective levels of IgA until almost 30 days after birth and hence, are reliant on other factors such as maternal breastfeeding to attain adequate levels of IgA (78). Probiotics have been shown to stimulate the production of secretory IgA (19) in human milk or the intestinal tract of infants. Hence, the stimulated production and higher levels of IgA antibodies in human milk may have transferred over to the infants during breastfeeding and can help with antigen-epithelium related diseases such as allergy management.
Other inflammatory markers often discussed in human milk are also transforming growth factor-beta (TGF-β) and IL-10. TGF-β suppresses inflammatory responses by inducing Tregs and promoting B-cell IgA production (79). Rigotti et al. demonstrated allergic mothers have a reduced TGF-β1 in breast milk and colostrum that may affect the modulation of the mucosal immune system and facilitate the development of allergy (80). IL-10 negatively regulates cell-mediated immunity directly or via suppression of antigen-presenting cell functions including IL-12 production (9) and can also inhibit NF-κB (81). It has also been suggested that IL-10 can suppress IL-12 and consequently IFN-γ production which leads to a Th2 or T regulatory response (82). Niers et al. suggested that the prevention of atopic eczema by probiotics could be through TLRs which have a key role to play in inhibiting the NF-κB and maintaining mucosal and intestinal homeostasis (83).
Prescott et al. suggested that the prenatal/postnatal administration of L. rhamnosus HN001 can reduce the rate of eczema by increasing the level of cord blood interferon-γ and consequently, change the composition of intestinal flora in children, thus modulating the immune system and allergy risk. A study conducted in New Zealand in 2008 administered L. rhamnosus HN001 at 6 × 109 CFU/day or B. animalis subsp lactis HN019 at 9 × 109 CFU/day from 35th week of gestation up to 6 months postpartum and subsequently, up to 2 years in the infant via formula or solid food. Breast milk samples were analyzed at 1 week, 3 months, and 6 months of lactation. Results showed that the probiotic administration resulted in significantly higher levels of TGF-β1 (p = 0.028) in breast milk (week 1) as compared to the placebo group. Effects of this were more pronounced in the B. lactis HN019 group (P = 0.041) with a similar trend in the L. rhamnosus HN001 group (P = 0.075). However, such differences were no longer observed in mature breast milk (3 and 6 months). Furthermore, there were no significant differences observed in breast milk TNF-α, IL-6, IL-10, and IgA throughout the administration period (40). Interestingly, the L. rhamnosus group supplementation group showed a significantly reduced risk of eczema at 2 years (HR 0.51; 95% CI, 0.30–0.85) compared to the placebo which was not observed in the B. lactis group (HR 0.90; 95% CI, 0.58–1.41) (74) despite showing similar changes in breast milk cytokine levels. It may suggest there may be a multitude of other factors linked to L. rhamnosus immunoregulation of infant allergy that B. lactis HN019 does not have and confirm that infant atopic dermatitis risk reduction is not solely reliant on breast milk cytokine profiles.
Another study found that L. rhamnosus may be ineffective in affecting breast milk anti-inflammatory markers such as TGF-β levels. A study administered L. rhamnosus GG at 1010 on mothers with a history of atopic disease for 4 weeks before expected delivery (42). Results showed that mature breast milk (3 months) TGF-β1 showed no significant differences compared with the placebo group. However, TGF-β2 in the breast milk of mothers receiving the probiotics was higher than that in the breast milk of mothers receiving placebo. Probiotic administration was still effective in the prevention of early atopic diseases in children at high risk (84). Even at higher dosages of L. rhamnosus GG, this probiotic strain may prove ineffective in modulating breast milk inflammatory markers. One study administered L. rhamnosus GG at 1.8 × 1010 CFU/day on pregnant women with a high allergic disease risk from 36 weeks of gestation to delivery (n = 73) (44) and found a lower level of total IgA seen in the 28-day mature milk as compared to the placebo group but no significant differences were seen in total IgA in the 7-day breast milk samples and no significant difference in breast milk TGF-β1 compared with the placebo group at 7 or 28 days.
Furthermore, results vary when L. rhamnosus GG is consumed in conjunction with other probiotic strains. A similar finding by Hurree et al. also administered L. rhamnosus GG and B. lactis Bb12 at 1010 CFU/day in pregnant women with an allergic history from the first trimester of pregnancy to the end of exclusive breastfeeding (n = 140) and found no significant differences in TGF-β1, TNF-α, IL-10, and IL-6 on the colostrum or mature (1 month) breast milk samples compared with the placebo group (46).
Kuitunen et al. administered L. rhamnosus GG (ATCC 53103) 1010 CFU, L. rhamnosus LC705 1010 CFU, B. breve Bb99 1010 CFU and P. freudenreichii ssp shermanii JS 4 × 109 CFU in mothers from 36 weeks of gestation until birth and to infants during the first 6 months of life (with 0.8 g of prebiotics with infants; n = 1,223) (45). Colostrum milk and mature breast milk (3 months) were analyzed. Results showed that probiotic intervention showed no significant differences compared to the placebo group in colostrum TGF-β2 levels and mature breast milk TGF-β2. However, the probiotic intervention increased IL-10 levels in the mature breast milk but did not influence their levels in colostrum. Lastly, probiotic intervention showed no differences as compared to the placebo group in total IgA in colostrum milk and mature milk. An interesting finding was that high TGF-β2 in mature BM was associated with more allergic disease and eczema at 2 years and was independent of probiotic treatment. As mentioned above, it appears that immunological development of infant allergy and the protective effect of probiotics are not necessarily mediated by immunomodulatory factors in breast milk but may be attributed to other components.
Apart from the administration of L. rhamnosus GG, other probiotic combinations have been used to investigate its effects on breast milk inflammatory markers. Baldassarre et al. administered Vivomixx® in healthy pregnant women from 4 weeks before the expected delivery date until 4 weeks after delivery (n = 66) (39). Vivomixx® contained 8 strains at a sum of 900 × 109 CFU of S. thermophilus DSM 24731, B. breve DSM 24732, B. longum DSM 24736, B. infantis DSM 24737, L. acidophilus DSM 24735, L. plantarum DSM 24730, L. paracasei DSM 24733, and L. delbrueckii subsp. bulgaricus DSM 24734. Results showed that breast milk TGF-β1, IL-6, and IL-10 were significantly higher in colostrum and mature (30 days) breast milk of the probiotic group compared to the control group but there were no significant differences observed for levels of IgA. One interesting observation was that IgA was significantly higher in newborn stools in the probiotic group compared to the control group. This may suggest that the increase in TGF-β1 in the breast milk of the probiotic group could result in higher IgA levels but is instead found in amniotic fluid as opposed to breast milk to create a more effective mucosal immune system. Hence, this shows probiotic supplementation has the potential to confer its health benefits to the infant despite certain breast milk inflammation markers showing no significant differences.
Another study conducted in Iran in 2013 administered a synbiotic combination of L. casei PXN 37, L. PXN 54, S. thermophilus PXN 66, B. breve PXN 25, L. acidophilus PXN 35, B. longum PXN 30, L. bulgaricus PXN 39 at a mixture of 2 × 108 CFU, and fructo-oligosaccharide (394 mg) daily for 30 days in lactating women who breastfed exclusively for 3 months (41). Results showed that there was an increase in TGF-β2 levels of breast milk and IgA while no significant differences were seen in the placebo group. However, no significant differences were observed in breast milk TGF-β1 levels. An open-label pilot trial administered 5 × 109 CFU of L. casei LC5, 5 × 109 CFU of B. longum BG7, and 2 × 108 CFU of B. coagulans SANK70258 per day for 2 months from 1 to 3 months postpartum (85). Results showed that there were no significant differences in median TGF-β1 and TGF-β2 from the 1 to 3 months postpartum. IgA levels decreased in both the probiotic and the placebo groups. One notable difference was that the median TGF-β1 decreased from 1 to 2 months postpartum followed by an increase from 2 to 3 months. To the best of our knowledge, the studies by Nikniaz et al. (41) and Takahashi et al. (38) were the only two studies that focused on probiotic administration solely during the lactation phase These 2 studies further highlight that probiotics act in a strain-specific manner and the discrepancy in results was likely due to the different probiotic strains, dosages and intervention formats used.
Lastly, few studies showed a decrease in anti-inflammatory markers. One study in Sweden found that L. reuteri ATCC 55730 at 1 × 108 CFU/day was supplemented through coconut and peanut oil drops. Supplementation was done from 36 weeks of gestation until delivery and inflammation markers were measured form colostrum and mature milk (1 month) (n = 109) (43). Results showed that there was a significantly lower amount of TGF- β2 and a significantly higher amount of IL-10 in colostrum milk as compared to placebo. However, there were no significant differences found in mature milk. Furthermore, there were no significant differences in levels of TGF- β1, IgA, and TNF-α in either colostrum or mature milk.
Research has remained inconsistent on how probiotic administration can affect the cytokine profile in breast milk, the fetus and their translated effects on infant outcomes. Ultimately, this depends on the probiotic strain, dosages, and period of intervention used. Despite similar changes in breast milk cytokine profile using two different probiotic strains, as demonstrated by Wickens et al. (86), there were not always associated with an allergy risk reduction in infants. Even if there were differences in breast milk cytokine profile in some studies, it does not seems to associate with infant outcomes if we compare Wickens et al. (86) and Kalliomäki et al. (84). Hence, it may be more meaningful to understand the strain specificity of probiotics and how it impacts allergy risk reduction than use cytokine as surrogate marker management in terms of immunological pathways. Even if infant allergy prevention is associated with the breast milk inflammatory markers, it appears to not always be associated with TGF-β, IL-10, or IgA and could perhaps be related more closely to fatty acids and/or human milk oligosaccharides that were not discussed (86).
However, one strategy that could be an administration started during pregnancy and be continued during lactation if possible. Despite the differences in breast milk immunomodulatory factors, one consistent result from these studies is that administration should start during late pregnancy, continued postnatally and should be fed to the infant even after breastfeeding for allergy management. We also recommend a probiotic species of L. rhamnosus HN001 or L. rhamnosus GG to be used as results may show its better competency in being an immunomodulatory agent as compared to other species and better effects on infant allergy risk management. Furthermore, a systematic review and meta-analysis conducted by Garcia-Larsen et al. concluded that probiotics supplements such as L. rhamnosus administered at a dosage between 1 and 10 × 109 CFU per day taken from 36 to 38 weeks gestation and through the first 3 to 6 months of lactation may reduce the risk of eczema in children. One precautionary measure is to avoid administration during early pregnancy as it has been associated with adverse effects such as intestinal disorders or immune deficiency (85).
Conclusion, Limitations, And Future Directions
We cannot strongly conclude on the impact of probiotic interventions on markers of inflammation and health outcomes in women of reproductive age and their children, due to the heterogeneity of the study results. Further studies should be done to focus on the contribution of the immunomodulatory markers and their association with health impacts on the subject. Our focus was maternal inflammation markers, and we did not explore the carry-over effects to the infant inflammatory markers due to the absence of data on infant inflammatory markers in cord blood.
Lastly, we recognize that inflammation markers may also be representative of a current symptomatic or asymptomatic infection and not always linked to the observed clinical outcome of diseases. These and other confounding factors such as lifestyle modifications, age, diet and the environment can contribute heavily to the observed changes, and/or offset the effects of probiotic administration. The assessment of probiotic efficacy in well-designed randomized placebo control trials is thus essential.
Observation made in this paper should be taken with caution and should not be generalized to all populations or all probiotics. The study designs mentioned in the paper are inherently different, making it difficult to determine if the evidence presents a global representation of the effects of probiotic administration. Current observations tend to conclude toward probiotic strain-specific effects.
Research remains inconsistent on the changes in immunomodulatory properties through probiotic administration. However, probiotic administration has been shown to evoke beneficial or neutral effects on non-communicable diseases and some of their risk factors. Beyond non-communicable diseases and cytokine profiles, specific probiotic strains appear to reduce the risk or duration of infections, but this was not discussed in this paper. In conclusion, specific probiotic strain or specific probiotic mix administration may provide beneficial effects in premenopausal women. However, proper assessment of probiotic strains, dosages, and intervention duration is desirable to allow a good interpretation of the results of future intervention studies. Future studies need to further evaluate the mechanisms of probiotics and their impact on inflammatory markers.
Author Contributions
ST, IS-Z, and LF initiated the project. KK and CB gathered the data and prepared the publication. KK, CB, LF, IS-Z, AI, and ST drafted, reviewed, and accepted the last version of the documents. All authors contributed to the article and approved the submitted version.
Conflict of Interest
KK, LF, IS-Z, ST, AI, and CB were employed by Nestlé Research during the conduct of the study.
Publisher's Note
All claims expressed in this article are solely those of the authors and do not necessarily represent those of their affiliated organizations, or those of the publisher, the editors and the reviewers. Any product that may be evaluated in this article, or claim that may be made by its manufacturer, is not guaranteed or endorsed by the publisher.
Abbreviations
CFU, Colony Forming Unit; CRP, C-Reactive Protein; GDM, Gestational Diabetes Mellitus; GSH, Glutathione; HDL, High-Density Lipoprotein; IgA, Immunoglobulin A; IL, Interleukine; NK, Natural Killer; TLR, Toll-Like Receptors; GSH, Glutathione; LPS, Lipopolysaccharide; MAPK, mitogen-activated protein kinases; NF-κB, Nuclear Factor-Kappa B; PCOS, Polycystic Ovarian Syndrome; PRRs, Pattern Recognition Receptors; ROS, Reactive Oxygen Species; SCFA, Short Chain Fatty Acids; SNPs, Single Nucleotide Polymorphisms; TGF, Transforming Growth Factor; TNF, Tumor Necrosis Factor.
References
1. Klein SL, Flanagan KL. Sex differences in immune responses. Nat Rev Immunol. (2016) 16:626–38. doi: 10.1038/nri.2016.90
2. Koren O, Goodrich JK, Cullender TC, Spor A, Laitinen K, Bäckhed HK, et al. Host remodeling of the gut microbiome and metabolic changes during pregnancy. Cell. (2012) 150:470–80. doi: 10.1016/j.cell.2012.07.008
3. Sanz Y. Gut microbiota and probiotics in maternal and infant health. Am J Clin Nutr. (2011) 94(Suppl.6):2000S. doi: 10.3945/ajcn.110.001172
4. Mor G, Aldo P, Alvero AB. The unique immunological and microbial aspects of pregnancy. Nat Rev Immunol. (2017) 17:469. doi: 10.1038/nri.2017.64
5. Faas MM, De Vos P. Uterine NK cells and macrophages in pregnancy. Placenta. (2017) 56:44–52. doi: 10.1016/j.placenta.2017.03.001
6. Raghupathy R, Kalinka J. Cytokine imbalance in pregnancy complications and its modulation. Front Biosci. (2008) 13:985–94. doi: 10.2741/2737
7. Romero R, Espinoza J, Gonçalves LF, Kusanovic JP, Friel L, Hassan S. The role of inflammation and infection in preterm birth. Semin Reprod Med. (2007) 25:21–39. doi: 10.1055/s-2006-956773
8. Kang H-J, Im S-H. Probiotics as an immune modulator. J Nutr Sci Vitaminol. (2015) 61:S103–5. doi: 10.3177/jnsv.61.S103
9. Ashraf R, Shah NP. Immune system stimulation by probiotic microorganisms. Crit Rev Food Sci Nutr. (2014) 54:938–56. doi: 10.1080/10408398.2011.619671
10. Pourrajab B, Fatahi S, Sohouli MH, Găman M-A, Shidfar F. The effects of probiotic/synbiotic supplementation compared to placebo on biomarkers of oxidative stress in adults: a systematic review and meta-analysis of randomized controlled trials. Crit Rev Food Sci Nutr. (2020) 2020:1–18. doi: 10.1080/10408398.2020.1821166
11. Plaza-Diaz J, Ruiz-Ojeda FJ, Gil-Campos M, Gil A. Mechanisms of action of probiotics. Adv Nutr. (2019) 10(Suppl.1):S49–66. doi: 10.1093/advances/nmy063
12. Medzhitov R. Toll-like receptors and innate immunity. Nat Rev Immunol. (2001) 1:135–45. doi: 10.1038/35100529
13. Lee CC, Avalos AM, Ploegh HL. Accessory molecules for Toll-like receptors and their function. Nat Rev Immunol. (2012) 12:168–79. doi: 10.1038/nri3151
14. Kubinak JL, Round JL. Toll-like receptors promote mutually beneficial commensal-host interactions. PLoS Pathog. (2012) 8:e1002785. doi: 10.1371/journal.ppat.1002785
15. Rautava S, Collado MC, Salminen S, Isolauri E. Probiotics modulate host-microbe interaction in the placenta and fetal gut: a randomized, double-blind, placebo-controlled trial. Neonatology. (2012) 102:178–84. doi: 10.1159/000339182
16. Marlow G, Han DY, Wickens K, Stanley T, Crane J, Mitchell EA, et al. Differential effects of two probiotics on the risks of eczema and atopy associated with single nucleotide polymorphisms to Toll-like receptors. Pediatr Allergy Immunol. (2015) 26:262–71. doi: 10.1111/pai.12371
17. Akira S, Takeda K. Toll-like receptor signalling. Nat Rev Immunol. (2004) 4:499–511. doi: 10.1038/nri1391
18. Li Q, Verma IM. NF-κB regulation in the immune system. Nat Rev Immunol. (2002) 2:725–34. doi: 10.1038/nri910
19. Yousefi B, Eslami M, Ghasemian A, Kokhaei P, Salek Farrokhi A, Darabi N. Probiotics importance and their immunomodulatory properties. J Cell Physiol. (2019) 234:8008–18. doi: 10.1002/jcp.27559
20. Mykhal'chyshyn H, Bodnar P, Kobyliak N. Effect of probiotics on proinflammatory cytokines level in patients with type 2 diabetes and nonalcoholic fatty liver disease. Likars' ka sprava. (2013) 2:56–62.
21. Di Santo JP, Vosshenrich CA, Satoh-Takayama N. A 'natural' way to provide innate mucosal immunity. Curr Opin Immunol. (2010) 22:435–41. doi: 10.1016/j.coi.2010.05.004
22. Ortiz-Andrellucchi A, Sánchez-Villegas A, Rodríguez-Gallego C, Lemes A, Molero T, Soria A, et al. Immunomodulatory effects of the intake of fermented milk with Lactobacillus casei DN114001 in lactating mothers and their children. Br J Nutr. (2008) 100:834–45. doi: 10.1017/S0007114508959183
23. Lash GE, Schiessl B, Kirkley M, Innes BA, Cooper A, Searle RF, et al. Expression of angiogenic growth factors by uterine natural killer cells during early pregnancy. J Leukoc Biol. (2006) 80:572–80. doi: 10.1189/jlb.0406250
24. Rizzello V, Bonaccorsi I, Dongarrà ML, Fink LN, Ferlazzo G. Role of natural killer and dendritic cell crosstalk in immunomodulation by commensal bacteria probiotics. J Biomed Biotechnol. (2011) 2011:473097. doi: 10.1155/2011/473097
25. Guermonprez P, Valladeau J, Zitvogel L, Théry C, Amigorena S. Antigen presentation and T cell stimulation by dendritic cells. Annu Rev Immunol. (2002) 20:621–67. doi: 10.1146/annurev.immunol.20.100301.064828
26. Banchereau J, Steinman RM. Dendritic cells and the control of immunity. Nature. (1998) 392:245–52. doi: 10.1038/32588
27. Demont A, Hacini-Rachinel F, Doucet-Ladeveze R, Ngom-Bru C, Mercenier A, Prioult G, et al. Live and heat-treated probiotics differently modulate IL10 mRNA stabilization and microRNA expression. J Allergy Clin Immunol. (2016) 137:1264–7e10. doi: 10.1016/j.jaci.2015.08.033
28. Bondonno CP, Croft KD, Hodgson JM. Dietary nitrate, nitric oxide, and cardiovascular health. Crit Rev Food Sci Nutr. (2016) 56:2036–52. doi: 10.1080/10408398.2013.811212
29. Tse JK. Gut microbiota, nitric oxide, and microglia as prerequisites for neurodegenerative disorders. ACS Chem Neurosci. (2017) 8:1438–47. doi: 10.1021/acschemneuro.7b00176
30. Vasquez EC, Pereira T, Peotta VA, Baldo MP, Campos-Toimil M. Probiotics as beneficial dietary supplements to prevent and treat cardiovascular diseases: uncovering their impact on oxidative stress. Oxid Med Cell Longevity. (2019) 2019:3086270. doi: 10.1155/2019/3086270
31. Asemi Z, Zare Z, Shakeri H, Sabihi SS, Esmaillzadeh A. Effect of multispecies probiotic supplements on metabolic profiles, hs-CRP, and oxidative stress in patients with type 2 diabetes. Ann Nutr Metab. (2013) 63:1–9. doi: 10.1159/000349922
32. Hegazy SK, El-Bedewy MM. Effect of probiotics on pro-inflammatory cytokines and NF-kappaB activation in ulcerative colitis. World J Gastroenterol. (2010) 16:4145–51. doi: 10.3748/wjg.v16.i33.4145
33. Teskey G, Abrahem R, Cao R, Gyurjian K, Islamoglu H, Lucero M, et al. Glutathione as a marker for human disease. Adv Clin Chem. (2018) 87:141–59. doi: 10.1016/bs.acc.2018.07.004
34. Asemi Z, Khorrami-Rad A, Alizadeh S-A, Shakeri H, Esmaillzadeh A. Effects of synbiotic food consumption on metabolic status of diabetic patients: a double-blind randomized cross-over controlled clinical trial. Clinical nutrition. (2014) 33:198–203. doi: 10.1016/j.clnu.2013.05.015
35. Taghizadeh M, Hashemi T, Shakeri H, Abedi F, Sabihi S-S, Alizadeh S-A, et al. Synbiotic food consumption reduces levels of triacylglycerols and VLDL, but not cholesterol, LDL, or HDL in plasma from pregnant women. Lipids. (2014) 49:155–61. doi: 10.1007/s11745-013-3867-2
36. Tsikas D. Assessment of lipid peroxidation by measuring malondialdehyde (MDA) and relatives in biological samples: analytical and biological challenges. Anal Biochem. (2017) 524:13–30. doi: 10.1016/j.ab.2016.10.021
37. Reis S, Conceição L, Rosa D, Siqueira N, Peluzio M. Mechanisms responsible for the hypocholesterolaemic effect of regular consumption of probiotics. Nutr Res Rev. (2017) 30:36. doi: 10.1017/S0954422416000226
38. Takahashi T, Fukudome H, Ueno HM, Watanabe-Matsuhashi S, Nakano T, Kobayashi T, et al. Effects of probiotic supplementation on TGF-β1, TGF-β2, and IgA levels in the milk of Japanese women: an open-label pilot study. Front Nutr. (2019) 6:128. doi: 10.3389/fnut.2019.00128
39. Baldassarre ME, Di Mauro A, Mastromarino P, Fanelli M, Martinelli D, Urbano F, et al. Administration of a multi-strain probiotic product to women in the perinatal period differentially affects the breast milk cytokine profile and may have beneficial effects on neonatal gastrointestinal functional symptoms. A randomized clinical trial. Nutrients. (2016) 8:nu8110677. doi: 10.3390/nu8110677
40. Prescott S, Wickens K, Westcott L, Jung W, Currie H, Black P, et al. Supplementation with Lactobacillus rhamnosus or Bifidobacterium lactis probiotics in pregnancy increases cord blood interferon-γ and breast milk transforming growth factor-β and immunoglobin A detection. Clin Exp Allergy. (2008) 38:1606–14. doi: 10.1111/j.1365-2222.2008.03061.x
41. Nikniaz L, Ostadrahimi A, Mahdavi R, Hejazi MA, Hosseini Salekdeh G. Effects of synbiotic supplementation on breast milk levels of IgA, TGF-β1, and TGF-β2. J Hum Lactation. (2013) 29:591–6. doi: 10.1177/0890334413490833
42. Rautava S, Kalliomäki M, Isolauri E. Probiotics during pregnancy and breast-feeding might confer immunomodulatory protection against atopic disease in the infant. J Allergy Clin Immunol. (2002) 109:119–21. doi: 10.1067/mai.2002.120273
43. Böttcher MF, Abrahamsson TR, Fredriksson M, Jakobsson T, Björkstén B. Low breast milk TGF-beta2 is induced by Lactobacillus reuteri supplementation and associates with reduced risk of sensitization during infancy. Pediatr Allergy Immunol. (2008) 19:497–504. doi: 10.1111/j.1399-3038.2007.00687.x
44. Boyle R, Ismail I, Kivivuori S, Licciardi P, Robins-Browne R, Mah LJ, et al. Lactobacillus GG treatment during pregnancy for the prevention of eczema: a randomized controlled trial. Allergy. (2011) 66:509–16. doi: 10.1111/j.1398-9995.2010.02507.x
45. Kuitunen M, Kukkonen AK, Savilahti E. Impact of maternal allergy and use of probiotics during pregnancy on breast milk cytokines and food antibodies and development of allergy in children until 5 years. Int Arch Allergy Immunol. (2012) 159:162–70. doi: 10.1159/000336157
46. Huurre A, Laitinen K, Rautava S, Korkeamäki M, Isolauri E. Impact of maternal atopy and probiotic supplementation during pregnancy on infant sensitization: a double-blind placebo-controlled study. Clin Exp Allergy. (2008) 38:1342–8. doi: 10.1111/j.1365-2222.2008.03008.x
47. Hoppu U, Isolauri E, Laakso P, Matomaki J, Laitinen K. Probiotics and dietary counselling targeting maternal dietary fat intake modifies breast milk fatty acids and cytokines. Eur J Nutr. (2012) 51:211–9. doi: 10.1007/s00394-011-0209-0
48. Ghanei N, Rezaei N, Amiri GA, Zayeri F, Makki G, Nasseri E. The probiotic supplementation reduced inflammation in polycystic ovary syndrome: a randomized, double-blind, placebo-controlled trial. J Funct Foods. (2018) 42:306–11. doi: 10.1016/j.jff.2017.12.047
49. Meyer AL, Elmadfa I, Herbacek I, Micksche M. Probiotic, as well as conventional yogurt, can enhance the stimulated production of proinflammatory cytokines. J Hum Nutr Diet. (2007) 20:590–8. doi: 10.1111/j.1365-277X.2007.00807.x
50. Jafarnejad S, Saremi S, Jafarnejad F, Arab A. Effects of a multispecies probiotic mixture on glycemic control and inflammatory status in women with gestational diabetes: a randomized controlled clinical trial. J Nutr Metabol. (2016) 2016:5190846. doi: 10.1155/2016/5190846
51. Yang S, Reid G, Challis JRG, Gloor GB, Asztalos E, Money D, et al. Effect of oral probiotic Lactobacillus rhamnosus GR-1 and Lactobacillus reuteri RC-14 on the vaginal microbiota, cytokines and chemokines in pregnant women. Nutrients. (2020) 12:20368. doi: 10.3390/nu12020368
52. Singh A, Sarangi AN, Goel A, Srivastava R, Bhargava R, Gaur P, et al. Effect of administration of a probiotic preparation on gut microbiota and immune response in healthy women in India: an open-label, single-arm pilot study. BMC Gastroenterol. (2018) 18:85. doi: 10.1186/s12876-018-0819-6
53. Vitali B, Cruciani F, Baldassarre ME, Capursi T, Spisni E, Valerii MC, et al. Dietary supplementation with probiotics during late pregnancy: outcome on vaginal microbiota and cytokine secretion. BMC Microbiol. (2012) 12:236. doi: 10.1186/1471-2180-12-236
54. Lorea Baroja M, Kirjavainen PV, Hekmat S, Reid G. Anti-inflammatory effects of probiotic yogurt in inflammatory bowel disease patients. Clin Exp Immunol. (2007) 14:470–9. doi: 10.1111/j.1365-2249.2007.03434.x
55. Kabeerdoss J, Devi RS, Mary RR, Prabhavathi D, Vidya R, Mechenro J, et al. Effect of yoghurt containing Bifidobacterium lactis Bb12(R) on faecal excretion of secretory immunoglobulin A and human beta-defensin 2 in healthy adult volunteers. Nutr J. (2011) 10:138. doi: 10.1186/1475-2891-10-138
56. Hajifaraji M, Jahanjou F, Abbasalizadeh F, Aghamohammadzadeh N, Abbasi MM, Dolatkhah N. Effect of probiotic supplements in women with gestational diabetes mellitus on inflammation and oxidative stress biomarkers: a randomized clinical trial. Asia Pac J Clin Nutr. (2018) 27:581. doi: 10.6133/apjcn.082017.03
57. Asemi Z, Jazayeri S, Najafi M, Samimi M, Mofid V, Shidfar F, et al. Effects of daily consumption of probiotic yoghurt on inflammatory factors in pregnant women: a randomized controlled trial. Pak J Biol Sci. (2011) 14:476–82. doi: 10.3923/pjbs.2011.476.482
58. Meyer AL, Micksche M, Herbacek I, Elmadfa I. Daily intake of probiotic as well as conventional yogurt has a stimulating effect on cellular immunity in young healthy women. Ann Nutr Metabol. (2006) 50:282–9. doi: 10.1159/000091687
59. Kekkonen RA, Lummela N, Karjalainen H, Latvala S, Tynkkynen S, Jarvenpaa S, et al. Probiotic intervention has strain-specific anti-inflammatory effects in healthy adults. World J Gastroenterol. (2008) 14:2029–36. doi: 10.3748/wjg.14.2029
60. Rotterdam E. ASRM SPONSORED PCOS CONCENSUS WORKSHOP GROUP. Revised 2003 consensus on diagnostic criteria and long-term health risks related to polycystic ovary syndrome. Fertil Steril. (2004) 81:19–25. doi: 10.1016/j.fertnstert.2003.10.004
61. Gilbert EW, Tay CT, Hiam DS, Teede HJ, Moran LJ. Comorbidities and complications of polycystic ovary syndrome: an overview of systematic reviews. Clin Endocrinol. (2018) 89:683–99. doi: 10.1111/cen.13828
62. Bednarska S, Siejka A. The pathogenesis and treatment of polycystic ovary syndrome: what's new. Adv Clin Exp Med. (2017) 26:359–67. doi: 10.17219/acem/59380
63. Yurtdaş G, Akdevelioglu Y. A new approach to polycystic ovary syndrome: the gut microbiota. J Am Coll Nutr. (2020) 39:371–82. doi: 10.1080/07315724.2019.1657515
64. Shabani A, Noshadian M, Jamilian M, Chamani M, Mohammadi S, Asemi Z. The effects of a novel combination of selenium and probiotic on weight loss, glycemic control and markers of cardio-metabolic risk in women with polycystic ovary syndrome. J Funct Foods. (2018) 46:329–34. doi: 10.1016/j.jff.2018.04.071
65. Masulli M, Vitacolonna E, Fraticelli F, Della Pepa G, Mannucci E, Monami M. Effects of probiotic supplementation during pregnancy on metabolic outcomes: a systematic review and meta-analysis of randomized controlled trials. Diabetes Res Clin Pract. (2020) 162:108111. doi: 10.1016/j.diabres.2020.108111
66. Shamasbi SG, Ghanbari-Homayi S, Mirghafourvand M. The effect of probiotics, prebiotics, and synbiotics on hormonal and inflammatory indices in women with polycystic ovary syndrome: a systematic review and meta-analysis. Eur J Nutr. (2020) 59:433–50. doi: 10.1007/s00394-019-02033-1
67. Buchanan TA, Xiang A, Kjos SL, Watanabe R. What is gestational diabetes? Diabetes Care. (2007) 30(Suppl.2):S105–11. doi: 10.2337/dc07-s201
68. Isolauri E, Rautava S, Collado M, Salminen S. Role of probiotics in reducing the risk of gestational diabetes. Diabet Obesity Metabol. (2015) 17:713–9. doi: 10.1111/dom.12475
69. Vincenzi A, Goettert MI, de Souza CFV. An evaluation of the effects of probiotics on tumoral necrosis factor (TNF-α) signaling and gene expression. Cytokine Growth Factor Rev. (2020) 10:4. doi: 10.1016/j.cytogfr.2020.10.004
70. Reiter A, Bengesser SA, Hauschild A-C, Birkl-Töglhofer A-M, Fellendorf FT, Platzer M, et al. Interleukin-6 gene expression changes after a 4-week intake of a multispecies probiotic in major depressive disorder—preliminary results of the PROVIT study. Nutrients. (2020) 12:2575. doi: 10.3390/nu12092575
71. Kuzmicki M, Telejko B, Szamatowicz J, Zonenberg A, Nikolajuk A, Kretowski A, et al. High resistin and interleukin-6 levels are associated with gestational diabetes mellitus. Gynecol Endocrinol. (2009) 25:258–63. doi: 10.1080/09513590802653825
72. Ouyang W, Rutz S, Crellin NK, Valdez PA, Hymowitz SG. Regulation and functions of the IL-10 family of cytokines in inflammation and disease. Annu Rev Immunol. (2011) 29:71–109. doi: 10.1146/annurev-immunol-031210-101312
73. Rostamtabar M, Esmaeilzadeh S, Tourani M, Rahmani A, Baee M, Shirafkan F, et al. Pathophysiological roles of chronic low-grade inflammation mediators in polycystic ovary syndrome. J Cell Physiol. (2021) 236:824–38. doi: 10.1002/jcp.29912
74. Fuller M, Priyadarshini M, Gibbons SM, Angueira AR, Brodsky M, Hayes MG, et al. The short-chain fatty acid receptor, FFA2, contributes to gestational glucose homeostasis. Am J Physiol Endocrinol Metabol. (2015) 309:E840–51. doi: 10.1152/ajpendo.00171.2015
75. Zhang J, Ma S, Wu S, Guo C, Long S, Tan H. Effects of probiotic supplement in pregnant women with gestational diabetes mellitus: a systematic review and meta-analysis of randomized controlled trials. J Diabetes Res. (2019) 2019:5364730. doi: 10.1155/2019/5364730
76. Iyengar SR, Walker W. Immune factors in breast milk and the development of atopic disease. J Pediatr Gastroenterol Nutr. (2012) 55:641–7. doi: 10.1097/MPG.0b013e3182617a9d
77. Delcenserie V, Martel D, Lamoureux M, Amiot J, Boutin Y, Roy D. Immunomodulatory effects of probiotics in the intestinal tract. Curr Issues Mol Biol. (2008) 10:37.
78. Selner J, Merrill D, Claman H. Salivary immunoglobulinand albumin: development during the newborn period. J Pediatr. (1968) 72:685–9. doi: 10.1016/S0022-3476(68)80014-9
79. Cazac BB, Roes J. TGF-β receptor controls B cell responsiveness and induction of IgA in vivo. Immunity. (2000) 13:443–51. doi: 10.1016/S1074-7613(00)00044-3
80. Rigotti E, Piacentini G, Ress M, Pigozzi R, Boner A, Peroni D. Transforming growth factor-β1 and interleukin-10 in breast milk and development of atopic diseases in infants. Clin Exp Allergy. (2006) 36:614–8. doi: 10.1111/j.1365-2222.2006.02483.x
81. Driessler F, Venstrom K, Sabat R, Asadullah K, Schottelius A. Molecular mechanisms of interleukin-10-mediated inhibition of NF-κB activity: a role for p50. Clin Exp Immunol. (2004) 135:64–73. doi: 10.1111/j.1365-2249.2004.02342.x
82. Sabat R, Grütz G, Warszawska K, Kirsch S, Witte E, Wolk K, et al. Biology of interleukin-10. Cytokine Growth Factor Rev. (2010) 21:331–44. doi: 10.1016/j.cytogfr.2010.09.002
83. Niers L, Martín R, Rijkers G, Sengers F, Timmerman H, Van Uden N, et al. The effects of selected probiotic strains on the development of eczema (the PandA study). Allergy. (2009) 64:1349–58. doi: 10.1111/j.1398-9995.2009.02021.x
84. Kalliomäki M, Salminen S, Arvilommi H, Kero P, Koskinen P, Isolauri E. Probiotics in primary prevention of atopic disease: a randomised placebo-controlled trial. Lancet. (2001) 357:1076–9. doi: 10.1016/S0140-6736(00)04259-8
85. Garcia-Larsen V, Ierodiakonou D, Jarrold K, Cunha S, Chivinge J, Robinson Z, et al. Diet during pregnancy and infancy and risk of allergic or autoimmune disease: a systematic review and meta-analysis. PLoS Med. (2018) 15:e1002507. doi: 10.1371/journal.pmed.1002507
Keywords: probiotics, inflammatory markers, gestational diabetes, polycystic ovarian syndrome (PCOS), gestational diabetes (GDM), atopic dermatitis (AD)
Citation: Kwok KO, Fries LR, Silva-Zolezzi I, Thakkar SK, Iroz A and Blanchard C (2022) Effects of Probiotic Intervention on Markers of Inflammation and Health Outcomes in Women of Reproductive Age and Their Children. Front. Nutr. 9:889040. doi: 10.3389/fnut.2022.889040
Received: 03 March 2022; Accepted: 03 May 2022;
Published: 06 June 2022.
Edited by:
Christelle Guillet, University of Auvergne, FranceReviewed by:
Feiyan Zhao, Inner Mongolia Agricultural University, ChinaSeyed Mehdi Kalantar, Yazd University, Iran
Copyright © 2022 Kwok, Fries, Silva-Zolezzi, Thakkar, Iroz and Blanchard. This is an open-access article distributed under the terms of the Creative Commons Attribution License (CC BY). The use, distribution or reproduction in other forums is permitted, provided the original author(s) and the copyright owner(s) are credited and that the original publication in this journal is cited, in accordance with accepted academic practice. No use, distribution or reproduction is permitted which does not comply with these terms.
*Correspondence: Carine Blanchard, carinegaelle.blanchard@rdls.nestle.com