- 1Lipids and Atherosclerosis Unit, Department of Internal Medicine, Maimonides Biomedical Research Institute of Córdoba (IMIBIC), Reina Sofía University Hospital, University of Córdoba, Córdoba, Spain
- 2Centro de Investigación Biomédica en Red de Fisiología de la Obesidad y Nutricion (CIBERobn), Instituto de Salud Carlos III, Madrid, Spain
- 3Biochemical Laboratory, Reina Sofía University Hospital, Córdoba, Spain
- 4Department of Cell Biology, Physiology, and Immunology, Maimonides Biomedical Research Institute of Córdoba (IMIBIC), Reina Sofía University Hospital, University of Córdoba, Córdoba, Spain
- 5Jean Mayer USDA Human Nutrition Research Center on Aging, Tufts University, Boston, MA, United States
- 6Instituto Madrileño de Estudios Avanzados en Alimentación (IMDEA-Food), Madrid, Spain
- 7International Advisory Board, University Camilo José Cela, Madrid, Spain
Background and Aims: rs964184 variant in the ZPR1 gene has been associated with blood lipids levels both in fasting and postprandial state and with the risk of myocardial infarction in high-risk cardiovascular patients. However, whether this association is modulated by diet has not been studied.
Objective: To investigate whether the type of diet (low-fat or Mediterranean diets) interacts with genetic variability at this loci to modulate fasting and postprandial lipids in coronary patients.
Materials and Methods: The genotype of the rs964184 polymorphism was determined in the Cordioprev Study population (NCT00924937). Fasting and Postprandial triglycerides were assessed before and after 3 years of dietary intervention with either a Mediterranean or a low-fat diet. Postprandial lipid assessment was done by a 4-h oral fat tolerance test (OFTT). Differences in triglycerides levels were identified using repeated-measures ANCOVA.
Results: From 523 patients (85% males, mean age 59 years) that completed the OFTT at baseline and after 3 years of intervention and had complete genotype information, 125 of them were carriers of the risk allele G. At the start of the study, these patients showed a higher fasting and postprandial triglycerides (TG) plasma levels. After 3 years of dietary intervention, G-carriers following a Mediterranean Diet maintained higher fasting and postprandial triglycerides, while those on the low-fat diet reduced their postprandial triglycerides to similar values to the population without the G-allele.
Conclusion: After 3 years of dietary intervention, the altered postprandial triglyceride response induced by genetic variability in the rs964184 polymorphism of the ZPR1 gene can be modulated by a low-fat diet, better than by a Mediterranean diet, in patients with coronary artery disease.
Introduction
The study of the effect of genetic differences between individuals on their response to a specific nutrient or dietary pattern for a specific health outcome (Nutrigenetics), and the study of the effect of foods on gene expression (Nutrigenomics) have enabled the development of the field of personalized nutrition and precision healthcare in the last years (1). Personalized nutritional counseling based on a person’s genetic background may improve the outcomes of a specific dietary intervention and provide a novel therapeutic strategy for better control of cardiovascular disease or its related risk factors, such as diabetes, obesity, or blood lipid levels (2–4).
Recently, there has been a growing recognition of the role of postprandial lipemia and the metabolism of triglycerides-rich proteins in the development and progression of atherosclerosis (5). Thus, postprandial triglyceride levels independently predict the risk of coronary artery disease (CAD), peripheral vascular disease, and cerebrovascular disease and may be stronger predictors of cardiovascular disease (CVD) than fasting triglycerides (6–10). This may be due to the proinflammatory, procoagulant, and prooxidant environment that characterizes the physiological response to dietary intakes, particularly a high-fat meal (11, 12). Specifically, the main mechanisms proposed for atherosclerosis induced by postprandial lipemia include: (1) endothelial dysfunction induced by increased plasma TG with decreased serum nitrite/nitrate levels and flow-mediated dilation, (2) an increase in reactive oxygen species mediated through mitochondrial dissociation and beta-oxidation, (3) increased expression of adhesion factors at the subendothelial level, (4) activation of complement component factor 3, and (5) upregulation of proinflammatory and pro-apoptotic genes in endothelial cells following fat intake (12–15).
Several factors have been described as influencing postprandial lipemia (PPL) response. These include, among others, diet, age, gender and genetics (16–20). Previous studies have evaluated the impact of various nutrients on PPL, indicating that carbohydrate quality, dietary fat content, and polyphenols may be key modulators (21). Among dietary patterns, the Mediterranean diet (MedDiet), with a high proportion of fiber-rich foods, whole grain cereals, vegetables, fruit, polyphenol-rich foods, and a low intake of saturated fatty acids and a high proportion of MUFA, has been proposed as a very attractive option for the prevention and treatment of cardiometabolic risk factors with positive effects on PPL (21). However, very few clinical trials have addressed the long-term effect of other dietary patterns on PPL. Due that the postprandial increase in plasma lipids is related to the amount of ingested fats, reducing fat consumption through a low-fat (LF) diet pattern could be an alternative option that remains to be fully elucidated (22, 23).
Regarding genetics, different common and rare gene variants have been related to plasma triglycerides (TG) (20). Interestingly, a single nucleotide polymorphism (SNP) located in the ZPR1 gene (rs964184) has been identified through genome-wide association study (GWAS) to be associated with the magnitude of postprandial TG response, with G-allele carriers of this SNP at greater risk of high plasma TG values during postprandial state compared to the reference allele carriers (24). The ZPR1 gene, located near the apolipoprotein gene cluster APOA1/C3/A4/A5 on chromosome 11, codes a regulatory protein that binds various transcription factors and interacts with the triglyceride-associated gene APOA5 (25). Its genetic variant rs964184 has been related to fasting TG (26), metabolic syndrome (27, 28), type 2 diabetes mellitus (29, 30), non-alcoholic fatty liver disease (NAFLD) (31), lipid response to fenofibrate (32), subclinical atherosclerosis (33), and coronary artery disease (34, 35). Although this variant has also been involved in plasma fasting lipids’ response to dietary fat (36), to date, there is no information on how different dietary patterns can influence the postprandial lipid response in relation to this SNP.
In our group, we have reported in the population of the CORDIOPREV study (ClinicalTrials.gov Identifier: NCT00924937) how the long-term consumption of a MedDiet may modulate PPL mainly in patients with type 2 diabetes mellitus (37), and the existence of a gene-diet interaction between genetic variants of ApoE and MedDiet (38). The current study aimed to investigate whether long−term consumption of two healthy dietary patterns (LF diet or MedDiet) interacts with rs964184 SNP at the ZPR1 gene to modulate postprandial lipemia in coronary heart disease patients.
Materials and Methods
Study Design and Subjects
The current study was conducted based on the Coronary Diet Intervention With Olive Oil and Cardiovascular Prevention (CORDIOPREV) study, which is a prospective, randomized, controlled trial including 1002 patients with coronary heart disease (CHD) to compare the effects of the consumption of two different dietary patterns (LF diet versus MedDiet) on the incidence of cardiovascular events of patients with coronary disease after 7 years of intervention (ClinicalTrials.gov Identifier: NCT00924937). The design, rationale and baseline characteristics of the CORDIOPREV study have been described elsewhere (39). Inclusion and exclusion criteria are shown in Supplementary Table 1. Briefly, patients were eligible if they were older than 20 but younger than 76 years old, had established CHD, were thought to follow a long-term dietary intervention and had no severe diseases or an expected life expectancy lower than the length of the study. No medication was defined as an exclusion criteria. Details of the dietary intervention have been published previously (40). In summary, the dietary models were as follows: (1) MedDiet, with a minimum of 35% of calories as fat (22% MUFA, 6% PUFA, and <10% saturated), 15% protein, and a maximum of 50% carbohydrates, and (2) LF diet, with high complex carbohydrates, <30% of total fat (<10% saturated fat, 12–14% MUFA and 6–8% PUFA), 15% protein, an a minimum of 55% carbohydrates. The 14-point Mediterranean Diet Adherence Screener (MEDAS) and a 9-point Low-Fat diet adherence were used to assess dietary adherence (41). There were no energy restrictions in place.
From 1002 patients included in the CORDIOPREV study, a total of 557 patients completed an oral fat test tolerance (OFTT) at baseline and after 3 years of intervention and were selected for genotyping.
The Ethics Committee of Reina Sofía University Hospital approved the trial protocol (n°1496/27/03/2009), which follows the Helsinki declaration and the charter of good clinical practices. The experimental protocol conforms to international ethical standards, and written informed consent was obtained from all the subjects.
Oral Fat Tolerance Tests
At the start of the CORDIOPREV Study and after 3 years of dietary intervention, patients received an OFTT using a weight-adjusted meal (0.7 g fat and 5 mg cholesterol per kg body weight) with 12% saturated fatty acids (SFA), 10% polyunsaturated fatty acids (PUFA), 43% monounsaturated fatty acids (MUFA), 10% protein, and 25% carbohydrates (CHO). The methodology followed for the OFTT has been described in the previous work of our group (42).
The patients fasted before beginning the test (no food or medicines) for 12 h. They were advised to refrain from smoking throughout the fasting period and from consuming alcohol for the previous 7 days. They were also instructed not to engage in any strenuous physical activity the day before the exam.
At 08:00 a.m., the patients arrived at the clinical center and a fasting blood sample and anthropometric measures were taken. Then, they consumed a fat-rich meal challenge under observation during a maximum period of 20 min. The participants were allowed to drink water but were not allowed to eat other foods or drink other beverages during the test period. Blood samples for postprandial biochemical measurements were taken every hour for the following 4 h.
Laboratory Tests and Genotyping
Biochemical measurements and DNA isolation have been previously described (43). 200 uL of DNA (50 ng/uL) isolated from blood samples were sent to the Human Genomics Facility of the Genetic Laboratory of the Department of Internal Medicine at Erasmus MC (Rotterdam, Netherlands), where genotyping was performed using the Illumina GSA beadchip GSA MD v1 (Illumina GSA Arrays “Infinium iSelect 24 × 1 HTS Custom Beadchip Kit”). In this research, we analyzed the rs964184 variation previously reported in the literature linked with lipid metabolism and coronary artery disease (24, 34).
Statistical Analysis
All variables had their distributions checked for normality, and skewed variables were normalized using log10 as needed. Depending on the presence of two or more groups in each comparison, continuous variables were compared using Student’s “t” and analysis of variance (ANCOVA). To quantify the magnitude of change during the postprandial state, we evaluated total (AUC) and incremental (iAUC) area under the curves of the different postprandial parameters using the trapezoid method, as in earlier works by our group (42). The genotype distributions did not differ from those predicted by Hardy-Weinberg (P > 0.05). Due to the low frequencies of homozygous patients for the minor allele (G/G = 7), patients carrying some risk allele (G/G or C/G) were grouped for analysis. Differences in postprandial values were identified using repeated-measures ANOVA, adjusting by age, gender, lipid-lowering drugs, and body mass index (BMI). We calculated the overall gene influence (SNP p-value), the kinetics of the response (Time p-value) and the interaction of both factors (Time × SNP p-value). An SNP-diet interaction term was used to evaluate gene-diet interactions. Bonferroni’s correction was used for multiple comparisons when necessary. Differences were considered to be significant when p < 0.05. All analyses were performed using R software (version 3.6.1).
Results
From 1002 patients included in the CORDIOPREV study, a total of 557 patients completed OFTT at baseline and after 3 years of intervention. Of those, 523 had genotype information and were included in the present analysis (268 in the MedDiet group and 255 in the LF diet group). A flowchart of the participant inclusion is shown in Figure 1.
Baseline characteristics according to genotype rs964184 SNP are shown in Table 1. There were no significant genotype-related differences for the variables examined, except for the higher HDL-cholesterol (HDL-c) and lower fasting triglycerides observed in C/C subjects compared with G-allele carriers (p < 0.01). Baseline and demographic data according to the dietary pattern received are shown in Supplementary Table 2. Changes in adherence to the MedDiet and LF, measured by the scoring scales used in the study (MEDAS and 9-point low-fat adherence) during the follow-up are shown in Supplementary Figure 1.
Table 2 shows the fasting lipid profile and body mass index (BMI) before and after the dietary intervention. No significant changes were observed in BMI, total cholesterol (TC), LDL-c, HDL-c, triglycerides, ApoA1, ApoB or Lp(a) after intervention adjusting by potential confounders (gender, age, BMI, lipid-lowering drugs). After 3 years of intervention, no gene-diet interactions were identified in fasting lipid changes. There were also no differences in physical activity between genotypes after the intervention (p = 0.982).
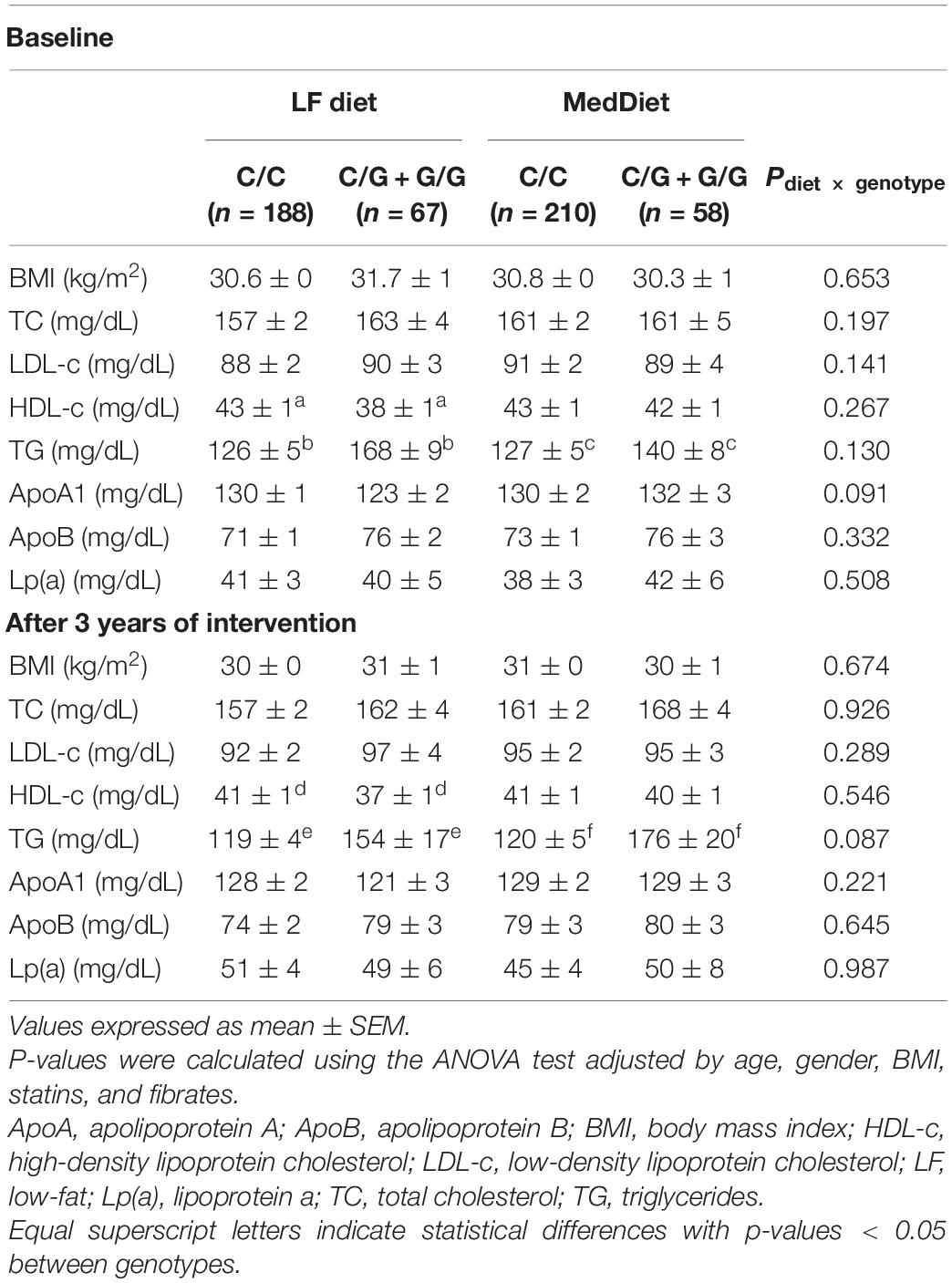
Table 2. Fasting lipid profile, body mass index at baseline, and their changes after 3 years of intervention according to rs964184 SNP and dietary patterns.
For the whole population, baseline postprandial triglycerides during the OFTT were higher in G-carriers at all time points (p < 0.05). Also a significant interaction between time × SNP was found (p-value = 0.005) (Figure 2A). Conversely, after 3 years of intervention, there were no differences in postprandial TG during OFTT between genotypes (p-value for SNP = 0.636, p-value for interaction time × SNP = 0.767) (Figure 2B). Changes (3 years–baseline) in postprandial TG AUC and iAUC adjusted by univariate analysis are shown in Supplementary Table 3. We identified a gene-diet interaction for the differences in postprandial TG AUC and iAUC (p-values SNP × diet = 0.006 and 0.031, respectively) (Supplementary Table 3).
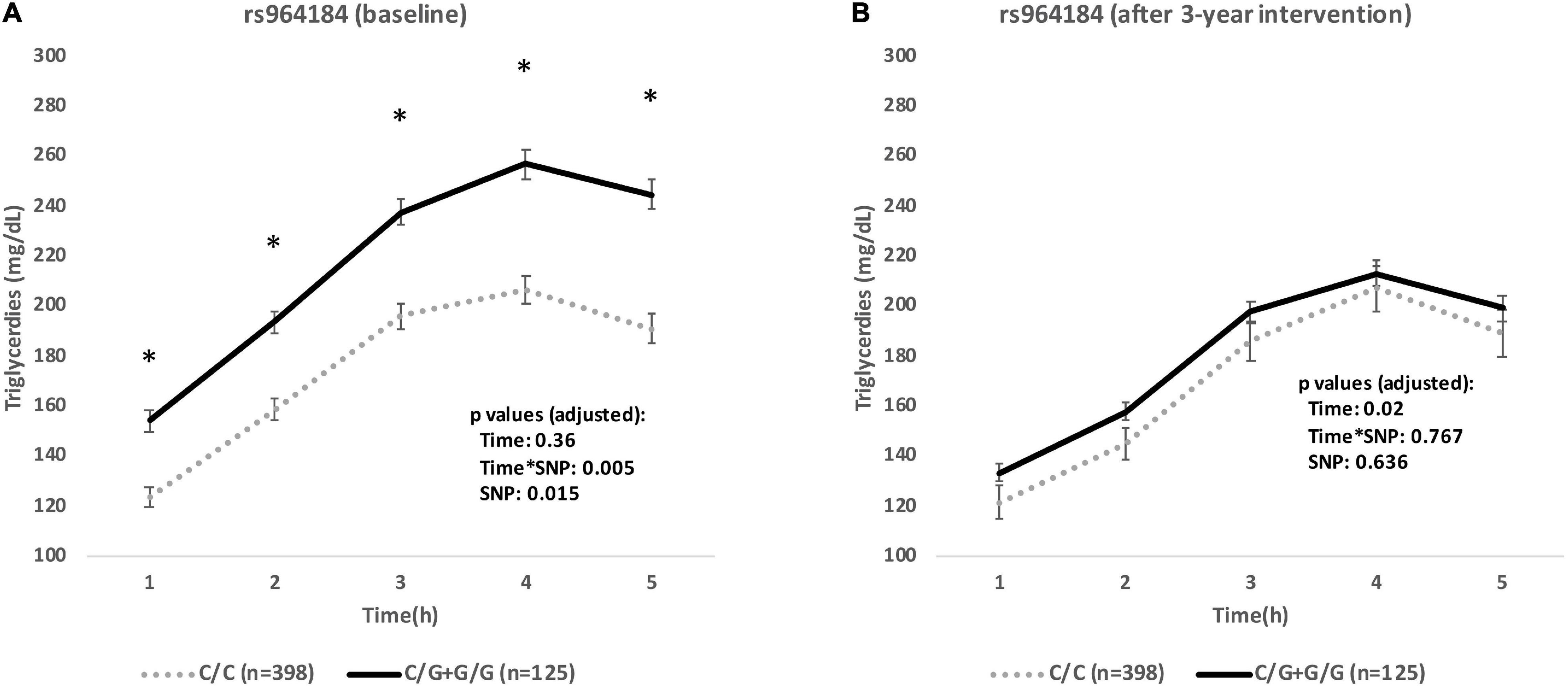
Figure 2. Postprandial triglycerides during baseline oral fat tolerance test (A) and after 3 years (B) of intervention by rs964184 genotypes. Values are means ± SEM. P-values were adjusted for age, gender, lipid-lowering drugs, and body mass index. *P < 0.05.
Low-Fat Intervention
Changes in postprandial TG AUC according to LF diet and genotypes are shown in Figure 3. Carriers of the G allele randomized to the LF diet had a higher magnitude of postprandial TG AUC of TG at baseline compared to C/C patients (p-value = 0.001). No differences were observed in the AUC of TG between genotypes after 3 years of intervention (p-value = 0.744). The p-value for the interaction time × SNP was 0.003.
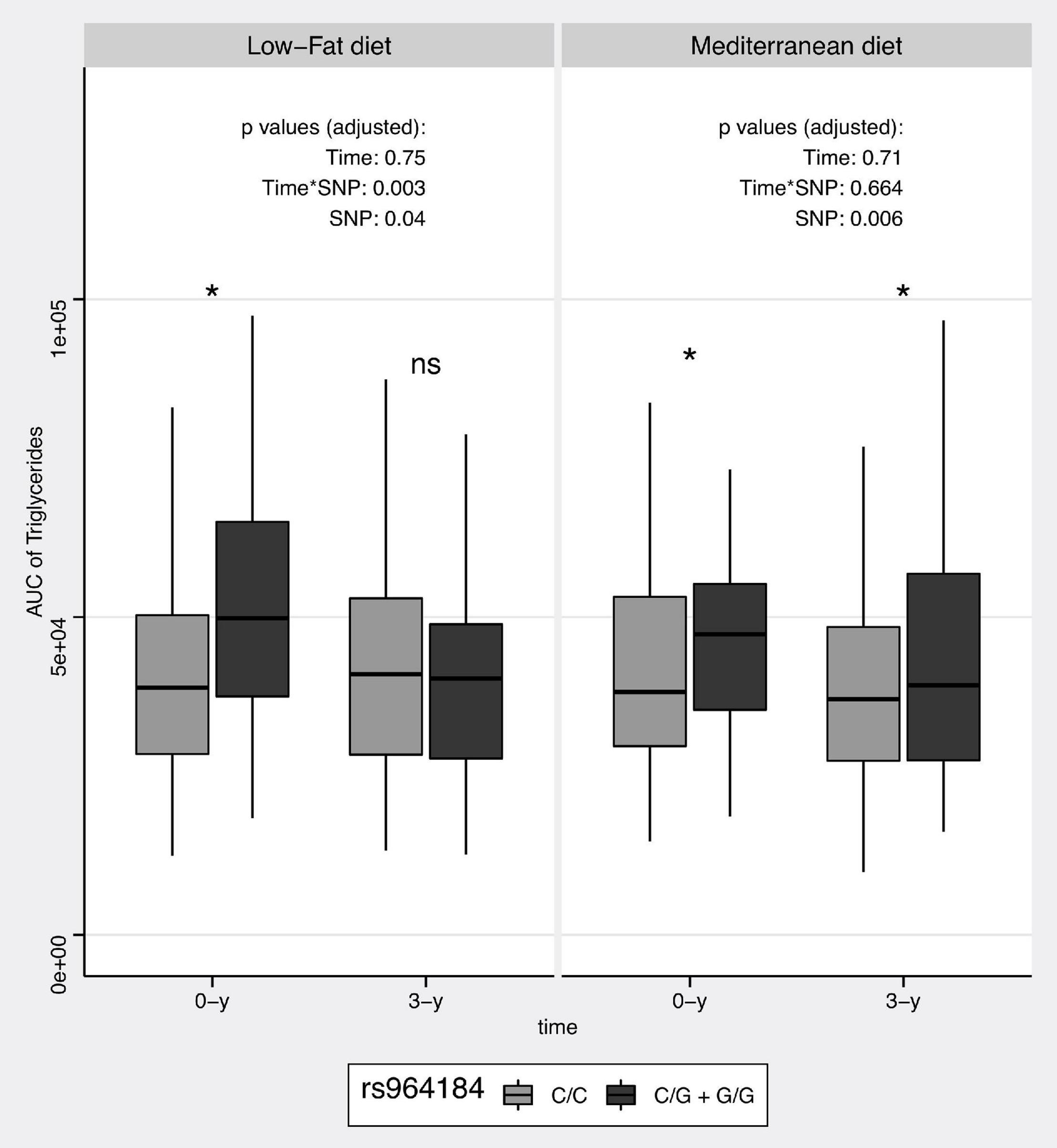
Figure 3. Postprandial AUC of triglycerides according to intervention diet and rs964184 genotypes. Values are shown as box plots, with the median, the approximate quartiles, and the lowest and highest data points. P-values were adjusted for age, gender, lipid-lowering drugs, and body mass index. *, p-value between genotypes < 0.05; ns, p-value between genotypes > 0.05.
Changes in postprandial TG iAUC by genotypes are shown in Supplementary Figure 2, with a p-value for interaction time × SNP = 0.02.
Mediterranean Diet Intervention
Changes in postprandial TG AUC according to MedDiet and genotypes are shown in Figure 3. At baseline, carriers of the G allele had higher AUC of TG compared to C/C patients (p-value = 0.017). After 3 years of Med-diet intervention, this difference remained (p-value = 0.04). The p-value for the interaction time × SNP was 0.664.
Changes in postprandial TG iAUC by genotypes are shown in Supplementary Figure 2, with a p-value for interaction time × SNP = 0.692.
Discussion
In this study, we investigated the effects of a long-term dietary intervention in CAD patients on postprandial triglyceridemia according to the presence or absence of the G allele at the ZPR1 rs964184 SNP. Our results show that the potential TG-rising effect of the G allele can be differentially modulated by diet. Specifically, carriers of the rs964184 allele risk (G/G or C/G), who, on average, had higher TG levels at baseline than CC carriers, decreased their TGs to levels similar to the CC carriers when following an LF diet for 3 years. Conversely, those G-allele carriers who followed a MedDiet intervention maintained their significantly higher TGs during the duration of the follow-up.
Dietetic interventions that encourage high-quality dietary patterns with moderate caloric intake have been linked to improved cardiovascular risk factor control (44). In that way, the MedDiet has been proposed as a compelling option for the prevention and treatment of cardiovascular disease (41), as well as an alternative to other dietary patterns, such as LF diets (22, 39). Thus, in a subgroup of patients with type 2 diabetes mellitus from the CORDIOPREV trial, we previously assessed the favorable effects of the MedDiet compared to the LF diet on PPL, finding that long-term consumption of the MedDiet improves PPL response compared to consumption of a low-fat diet (37). Furthermore, we reported a gene-diet interaction between a variation in the APOE gene (rs439401) and MedDiet, with T-allele carriers in the MedDiet group showing a more significant decrease in postprandial TG compared with CC subject (38).
Given that increased TG levels, both fasting and non-fasting, have been related to higher CVD risk (45, 46), the results of the current study provide evidence that specific nutritional advice can benefit patients who are genetically predisposed to higher fasting and postprandial TGs and supports the notion of Precision Nutrition as the path to promote better health (47).
Previous studies have reported that polymorphisms in or near the ZPR1-APOA5-A4-C3-A1 gene complex are associated with plasma lipids and cardiovascular risk (33, 35, 48, 49). The results of the current study support previous findings, showing that rs964184 within the ZPR1 locus is associated with fasting plasma TG levels and HDL-c. This relation with fasting TG has been well documented (26, 50), and has been replicated recently in a longitudinal multi-ancestry cohort (European, African, and Asian ancestry) (49). In accordance with our results, in a cohort of 734 overweight or obese participants from the Pounds Lost Trial, the carriers of the G allele exhibited higher fasting TG and lower HDL-c values at baseline. Moreover, in that population, and after a 2-year intervention, a significant interaction between the rs964184 SNP and dietary fat intake (low vs. high) was reported in TC, LDL-c, and HDL-c variations (36). Specifically, G carriers benefited from a low-fat diet more than CC carriers in relation to TC and LDL-c changes. However, in our study, no gene-diet interactions were identified in fasting lipid changes after the 3-year intervention, and the significant differences observed between genotypes in HDL-c and TG values at baseline remained unchanged at the end of both interventions. Additionally, we have investigated this reported interaction more deeply by examining the effects of long-term healthy dietary interventions on postprandial TG metabolism. Dietary fat intake in our study ranged from a minimum of 35% of the total calories as fat (22% MUFA) in the MedDiet group to less than 30% of total calories as fat in the LF diet group (39, 40). Our data demonstrate that, in this patient population, G-allele carriers benefit more from an LF diet than from a MedDiet concerning postprandial TG metabolism. As far as we know, no other studies have evaluated the effect of these diets on rs964184 to modulate the postprandial TG response.
In addition to postprandial metabolism and plasma lipid concentration, different clinical phenomena have been related to the genetic variant studied in the present study. Metabolic syndrome, T2DM, non-alcoholic fatty liver disease (NAFLD), and cardiovascular disease have all been associated with the ZPR1 variation rs964184 among different populations (31, 35, 51). Thus, in a prospective cohort of 185 Spanish patients with primary hypertriglyceridemia, a significant recessive model of risk of NAFLD was reported, with an Odds Ratio of 4.99 in G/G patients vs. C/G + C/C patients (31). Regarding cardiovascular risk, in a study of 725 Caucasian patients with genetically confirmed familial hypercholesterolemia, Paquette et al. (35) found that rs964184 was significantly associated with incident myocardial infarction even after controlling for traditional cardiovascular risk factors. Moreover, in a cohort of 3757 Japanese individuals who either visited outpatient clinics or were admitted to 6 selected hospitals between 2002 and 2012, the G allele of rs964184 SNP was significantly associated with type 2 diabetes prevalence (OR = 1.25) (29), indicating that this SNP may represent a risk factor for all these conditions. In our study, no genotype differences were observed for the risk of diabetes or obesity, as shown in Table 1, probably related to the particular characteristics of this cohort of secondary prevention patients, with a higher prevalence of cardiometabolic abnormalities compared to the general population.
On the other hand, other environmental factors have also been suggested to modulate this genetic variant. Thus, longer sleep duration has been related to the adverse effects of a genetic risk score constructed in 8648 healthy subjects from the Dongfeng-Tongji cohort with a set of 4 polymorphisms in the APOA4-APOA5-ZPR1-BUD13 gene cluster (rs17119975, rs651821, rs7396835, and rs964184) on 5-year triglyceride changes (52). Furthermore, in addition to the influence of genetic background, exercise frequency, hypertension, and education level have been explored as environmental risk factors that may affect serum lipid profiles and diabetes risk. In this way, Li et al. explored in a cohort of 2323 Chinese Han subjects from the Zhejiang Province which genes and environmental factors were associated with type 2 diabetes mellitus risk (30). They reported a set of SNPs and environmental factors that were associated with a higher risk of T2DM, which included rs96418, together with a history of hypertension, regular intake of meat, and waist circumference.
The mechanisms responsible for the reported interaction have not been elucidated. rs964184 is located within the 3-UTR of the ZPR1 gene, which codified a key regulatory protein required for appropriate nucleolar activity in cell proliferation and signal transduction (51). ZPR1’s promoter region has the ability to bind to peroxisome proliferator-activated receptor gamma (PPARG) proteins 1 and 2, which may activate genes involved in glucose and cholesterol metabolism via hepatocyte nuclear factor 4 alpha activation (51). On the other hand, a micro-RNA-related mechanism may also be involved as it has been shown for other TG-related gene-diet interactions. MicroRNAs (miRs) are short nucleotide non-coding RNAs that operate as post-transcriptional inhibitors of gene expression by binding to miR recognition sites within their target mRNAs’ 3-UTRs (53). Previous research has shown that SNPs can cause allele-specific regulation by disrupting crucial areas for binding miRs, known as seed sites, as in the case of the lipoprotein lipase (LPL) variant rs13702 via interfering with the miR-410 seed site (54, 55). Future studies are needed to discover the possible underlying mechanisms responsible for the described interaction between the rs964184 SNP and PPL.
Our study has some limitations. Given the characteristics of the CORDIOPREV study population, most patients were exposed to the effect of lipid-lowering drugs prescribed for their existing CVD. Although no differences were observed in the baseline use of statins or fibrates, their use has been related to changes in postprandial metabolism (56, 57) that might influence our results. Moreover, a previous study has reported a gender effect related to this SNP (58); however, given the small number of women in our patient population, we could not test this gene-diet-sex interaction. Thus, the generalization of our results should be made with caution and would require validation on external populations. Nevertheless, our study has strengths such as the characterization of the modulation of genetic induced effects of coronary patients who have received a long-term nutritional intervention, as well as the performance of dynamic postprandial studies beyond the determination of fasting parameters. Future studies should evaluate whether this gene-diet interaction may be associated with a decrease in the rate of incident vascular events.
In summary, the development of specific nutritional advice based on genetic differences between individuals on their response to specific dietary patterns may promote better health in patients with cardiometabolic risk factors. Postprandial events related to TG metabolism are important factors related to the development and progression of atherosclerotic disease, and the rs964184 variant in the ZRP1 gene has been identified through GWAS studies as one of the most strongly associated with the magnitude of postprandial TG response. In our study, we describe a novel gene-diet interaction between the long-term consumption of two healthy dietary patterns (LF diet and MedDiet) and the ZPR1 rs964184 SNP on postprandial TG in coronary patients. Therefore, patients with the G-allele at this SNP would benefit more from a low-fat diet to reduce their genetically induced postprandial hyperlipemia. Further studies should confirm whether such modulation would lead to a reduction in the risk of developing cardiovascular disease and investigate the underlying mechanisms that explain this interaction.
Data Availability Statement
The original contributions presented in this study are publicly available. This data can be found here: Dryad, Dataset, https://datadryad.org/stash/dataset/doi:10.5061/dryad.9cnp5hqmh.
Ethics Statement
The studies involving human participants were reviewed and approved by the Ethics Committee of Reina Sofía University Hospital (trial protocol 1496/27/03/2009). The patients/participants provided their written informed consent to participate in this study.
Author Contributions
JL-M and JD-L contributed to the study concept. JL-M, JD-L, and JO critically reviewed the manuscript. JA-D and AA-dL contributed to the design of the manuscript, figures preparation, edition, and manuscript drafting. JA-D, AA-dL, JT-P, FR-C, OR-Z, EY-S, FG-M, MC, RL, and PP-M contributed to the acquisition and analysis of data. All authors gave final approval for all aspects of the work, agreed to be fully accountable for ensuring the integrity and accuracy of the work, and read and approved the final manuscript.
Funding
The CORDIOPREV study was supported by the Fundación Patrimonio Comunal Olivarero. The main sponsor agreed to participate in the study because when it started, there were no full-length studies evaluating the effect of an olive oil-based Mediterranean diet on secondary coronary prevention, although there were many observational studies and studies evaluating risk factors that indicated that it could have a favorable effect. The sponsor was not involved in the design or carrying out of the study, and its participation was limited to funding and providing the olive oil used in the study. We also received additional funding from CEAS (Centro de Excelencia en Investigacion sobre Aceite de Oliva), Junta de Andalucia (Consejeria de Salud, Consejeria de Agricultura y Pesca, Consejeria de Innovacion, Ciencia y Empresa), Diputaciones de Jaen y Córdoba, y Salud and Ministerio de Medio Ambiente, Medio Rural y Marino, Spanish Government. It was also partly supported by research grants from the Ministerio de Ciencia e Innovacion (FIS PI10/01041 to PP-M, FIS PI13/00023 to JD-L; PIE14/00005 and PIE 14/00031 to JL-M; PI15/00733 to OR-Z; PI16/01777 to PP-M; PI18/01822 to EY-S; PID2019-104362RB-I00 to JL-M; Ministerio de Economia y Competitividad [AGL2009-122270, AGL2012/39615, PCIN-2016-084 (JPI HDHL) to JL-M, and AGL2015-67896-P to JL-M]; Consejeria de Salud, Junta de Andalucia (PI0193/09 to JL-M, PI-0252/09 to JD-L, and PI-0058/10 to PP-M; PC-0283/2017 to EY-S; PI-0170-2018-FIB to OR-Z); Proyecto de Excelencia, Consejeriía de Economiía, Innovación, Ciencia y Empleo (CVI-7450 to JL-M); and Seventh Framework Programme (Project N° 289511, Acronym NutriTech). This study was also co-financed by the Fondo Europeo de Desarrollo Regional (FEDER). The CIBEROBN and CIBERCV were initiatives of the Instituto de Salud Carlos III, Madrid, Spain. EY-S was the recipient of the Nicolas Monardes Programme from the Servicio Andaluz de Salud, Junta de Andalucia, Spain (C1-0005-2019). JA-D was the recipient of “Action B Clinical Researchers” Programme from the Servicio Andaluz de Salud, Junta de Andalucia, Spain (B-0009-2017).
Conflict of Interest
The authors declare that the research was conducted in the absence of any commercial or financial relationships that could be construed as a potential conflict of interest.
Publisher’s Note
All claims expressed in this article are solely those of the authors and do not necessarily represent those of their affiliated organizations, or those of the publisher, the editors and the reviewers. Any product that may be evaluated in this article, or claim that may be made by its manufacturer, is not guaranteed or endorsed by the publisher.
Acknowledgments
We especially want to thank the participants of the CORDIOPREV Study.
Supplementary Material
The Supplementary Material for this article can be found online at: https://www.frontiersin.org/articles/10.3389/fnut.2022.885256/full#supplementary-material
Abbreviations
ApoA1, apolipoprotein A1; ApoB, apolipoprotrein B; AUC, total area under the curve; CAD, coronary artery disease; CVD, cardiovascular disease; CHD, coronary heart disease; CHO, carbohydrates; GWAS, genome-wide association study; HDL-c, high-density lipoprotein cholesterol; Hs-CRP, high sensitive C-reactive protein; iAUC, incremental area under the curve; LDL-c, low-density lipoprotein cholesterol; LF, low-fat; Lp(a), lipoprotein(a); MedDiet, Mediterranean diet; miRs, microRNAs; MUFA, monounsaturated fatty acids; NAFLD, non-alcoholic fatty liver disease; OFTT, oral fat tolerance test; PPL, postprandial lipemia; PUFA, polyunsaturated fatty acids; SFA, saturated fatty acids; SNP, single nucleotide polymorphism; T2DM, type 2 diabetes mellitus; TG, triglycerides; TRLs, triglycerides-rich-lipoproteins.
References
1. Marcum JA. Nutrigenetics/nutrigenomics, personalized nutrition, and precision healthcare. Curr Nutr Rep. (2020) 9:338–45. doi: 10.1007/s13668-020-00327-z
2. Perez-Beltran YE, Rivera-Iniguez I, Gonzalez-Becerra K, Perez-Naitoh N, Tovar J, Sayago-Ayerdi SG, et al. Personalized dietary recommendations based on lipid-related genetic variants: a systematic review. Front Nutr. (2022) 9:830283. doi: 10.3389/fnut.2022.830283
3. Barrea L, Annunziata G, Bordoni L, Muscogiuri G, Colao A, Savastano S, et al. Nutrigenetics-personalized nutrition in obesity and cardiovascular diseases. Int J Obes Suppl. (2020) 10:1–13. doi: 10.1038/s41367-020-0014-4
4. Franzago M, Santurbano D, Vitacolonna E, Stuppia L. Genes and diet in the prevention of chronic diseases in future generations. Int J Mol Sci. (2020) 21:2633. doi: 10.3390/ijms21072633
5. Boren J, Taskinen MR, Bjornson E, Packard CJ. Metabolism of triglyceride-rich lipoproteins in health and dyslipidaemia. Nat Rev Cardiol. (2022). doi: 10.1038/s41569-022-00676-y [Epub ahead of print].
6. Freiberg JJ, Tybjaerg-Hansen A, Jensen JS, Nordestgaard BG. Nonfasting triglycerides and risk of ischemic stroke in the general population. JAMA. (2008) 300:2142–52. doi: 10.1001/jama.2008.621
7. Bansal S, Buring JE, Rifai N, Mora S, Sacks FM, Ridker PM. Fasting compared with nonfasting triglycerides and risk of cardiovascular events in women. JAMA. (2007) 298:309–16. doi: 10.1001/jama.298.3.309
8. Langsted A, Freiberg JJ, Nordestgaard BG. Fasting and nonfasting lipid levels: influence of normal food intake on lipids, lipoproteins, apolipoproteins, and cardiovascular risk prediction. Circulation. (2008) 118:2047–56. doi: 10.1161/CIRCULATIONAHA.108.804146
9. Nordestgaard BG, Freiberg JJ. Clinical relevance of non-fasting and postprandial hypertriglyceridemia and remnant cholesterol. Curr Vasc Pharmacol. (2011) 9:281–6. doi: 10.2174/157016111795495585
10. Mora S, Rifai N, Buring JE, Ridker PM. Fasting compared with nonfasting lipids and apolipoproteins for predicting incident cardiovascular events. Circulation. (2008) 118:993–1001. doi: 10.1161/CIRCULATIONAHA.108.777334
11. Klop B, Proctor SD, Mamo JC, Botham KM, Castro Cabezas M. Understanding postprandial inflammation and its relationship to lifestyle behaviour and metabolic diseases. Int J Vasc Med. (2012) 2012:947417. doi: 10.1155/2012/947417
12. Zhao Y, Liu L, Yang S, Liu G, Pan L, Gu C, et al. Mechanisms of atherosclerosis induced by postprandial lipemia. Front Cardiovasc Med. (2021) 8:636947. doi: 10.3389/fcvm.2021.636947
13. Johnson BD, Padilla J, Harris RA, Wallace JP. Vascular consequences of a high-fat meal in physically active and inactive adults. Appl Physiol Nutr Metab. (2011) 36:368–75. doi: 10.1139/H11-028
14. Meessen ECE, Warmbrunn MV, Nieuwdorp M, Soeters MR. Human postprandial nutrient metabolism and low-grade inflammation: a narrative review. Nutrients. (2019) 11:3000. doi: 10.3390/nu11123000
15. Spallarossa P, Garibaldi S, Barisione C, Ghigliotti G, Altieri P, Tracchi I, et al. Postprandial serum induces apoptosis in endothelial cells: role of polymorphonuclear-derived myeloperoxidase and metalloproteinase-9 activity. Atherosclerosis. (2008) 198:458–67. doi: 10.1016/j.atherosclerosis.2007.11.030
16. Rosenson RS, Davidson MH, Hirsh BJ, Kathiresan S, Gaudet D. Genetics and causality of triglyceride-rich lipoproteins in atherosclerotic cardiovascular disease. J Am Coll Cardiol. (2014) 64:2525–40. doi: 10.1016/j.jacc.2014.09.042
17. Perez-Martinez P, Delgado-Lista J, Perez-Jimenez F, Lopez-Miranda J. Update on genetics of postprandial lipemia. Atheroscler Suppl. (2010) 11:39–43. doi: 10.1016/j.atherosclerosissup.2010.03.002
18. Parnell LD, Ordovas JM, Lai CQ. Environmental and epigenetic regulation of postprandial lipemia. Curr Opin Lipidol. (2018) 29:30–5. doi: 10.1097/MOL.0000000000000469
19. Perez-Martinez P, Ordovas JM, Garcia-Rios A, Delgado-Lista J, Delgado-Casado N, Cruz-Teno C, et al. Consumption of diets with different type of fat influences triacylglycerols-rich lipoproteins particle number and size during the postprandial state. Nutr Metab Cardiovasc Dis. (2011) 21:39–45. doi: 10.1016/j.numecd.2009.07.008
20. Dron JS, Hegele RA. Genetics of triglycerides and the risk of atherosclerosis. Curr Atheroscler Rep. (2017) 19:31. doi: 10.1007/s11883-017-0667-9
21. Bozzetto L, Della Pepa G, Vetrani C, Rivellese AA. Dietary impact on postprandial lipemia. Front Endocrinol (Lausanne). (2020) 11:337. doi: 10.3389/fendo.2020.00337
22. Prentice RL, Aragaki AK, Van Horn L, Thomson CA, Beresford SA, Robinson J, et al. Low-fat dietary pattern and cardiovascular disease: results from the women’s health initiative randomized controlled trial. Am J Clin Nutr. (2017) 106:35–43. doi: 10.3945/ajcn.117.153270
23. Teng KT, Chang CY, Kanthimathi MS, Tan AT, Nesaretnam K. Effects of amount and type of dietary fats on postprandial lipemia and thrombogenic markers in individuals with metabolic syndrome. Atherosclerosis. (2015) 242:281–7. doi: 10.1016/j.atherosclerosis.2015.07.003
24. Wojczynski MK, Parnell LD, Pollin TI, Lai CQ, Feitosa MF, O’Connell JR, et al. Genome-wide association study of triglyceride response to a high-fat meal among participants of the NHLBI genetics of lipid lowering drugs and diet network (GOLDN). Metabolism. (2015) 64:1359–71. doi: 10.1016/j.metabol.2015.07.001
25. Read RW, Schlauch KA, Lombardi VC, Cirulli ET, Washington NL, Lu JT, et al. Genome-wide identification of rare and common variants driving triglyceride levels in a nevada population. Front Genet. (2021) 12:639418. doi: 10.3389/fgene.2021.639418
26. Teslovich TM, Musunuru K, Smith AV, Edmondson AC, Stylianou IM, Koseki M, et al. Biological, clinical and population relevance of 95 loci for blood lipids. Nature. (2010) 466:707–13. doi: 10.1038/nature09270
27. Kraja AT, Vaidya D, Pankow JS, Goodarzi MO, Assimes TL, Kullo IJ, et al. A bivariate genome-wide approach to metabolic syndrome: STAMPEED consortium. Diabetes. (2011) 60:1329–39. doi: 10.2337/db10-1011
28. Mirhafez SR, Avan A, Pasdar A, Khatamianfar S, Hosseinzadeh L, Ganjali S, et al. Zinc finger 259 gene polymorphism rs964184 is associated with serum triglyceride levels and metabolic syndrome. Int J Mol Cell Med. (2016) 5:8–18.
29. Tokoro F, Matsuoka R, Abe S, Arai M, Noda T, Watanabe S, et al. Association of a genetic variant of the ZPR1 zinc finger gene with type 2 diabetes mellitus. Biomed Rep. (2015) 3:88–92. doi: 10.3892/br.2014.379
30. Li Z, Ye CY, Zhao TY, Yang L. Model of genetic and environmental factors associated with type 2 diabetes mellitus in a Chinese Han population. BMC Public Health. (2020) 20:1024. doi: 10.1186/s12889-020-09130-5
31. Esteve-Luque V, Padro-Miquel A, Fanlo-Maresma M, Corbella E, Corbella X, Pinto X, et al. Implication between genetic variants from APOA5 and ZPR1 and NAFLD severity in patients with hypertriglyceridemia. Nutrients. (2021) 13:552. doi: 10.3390/nu13020552
32. Aslibekyan S, Goodarzi MO, Frazier-Wood AC, Yan X, Irvin MR, Kim E, et al. Variants identified in a GWAS meta-analysis for blood lipids are associated with the lipid response to fenofibrate. PLoS One. (2012) 7:e48663. doi: 10.1371/journal.pone.0048663
33. Vargas JD, Manichaikul A, Wang XQ, Rich SS, Rotter JI, Post WS, et al. Common genetic variants and subclinical atherosclerosis: the multi-ethnic study of atherosclerosis (MESA). Atherosclerosis. (2016) 245:230–6. doi: 10.1016/j.atherosclerosis.2015.11.034
34. Schunkert H, Konig IR, Kathiresan S, Reilly MP, Assimes TL, Holm H, et al. Large-scale association analysis identifies 13 new susceptibility loci for coronary artery disease. Nat Genet. (2011) 43:333–8. doi: 10.1038/ng.784
35. Paquette M, Fantino M, Bernard S, Baass A. The ZPR1 genotype predicts myocardial infarction in patients with familial hypercholesterolemia. J Clin Lipidol. (2020) 14:660–6. doi: 10.1016/j.jacl.2020.07.008
36. Zhang X, Qi Q, Bray GA, Hu FB, Sacks FM, Qi L. APOA5 genotype modulates 2-y changes in lipid profile in response to weight-loss diet intervention: the pounds lost trial. Am J Clin Nutr. (2012) 96:917–22. doi: 10.3945/ajcn.112.040907
37. Gomez-Marin B, Gomez-Delgado F, Lopez-Moreno J, Alcala-Diaz JF, Jimenez-Lucena R, Torres-Pena JD, et al. Long-term consumption of a Mediterranean diet improves postprandial lipemia in patients with type 2 diabetes: the Cordioprev randomized trial. Am J Clin Nutr. (2018) 108:963–70. doi: 10.1093/ajcn/nqy144
38. Gomez-Delgado F, Alcala-Diaz JF, Leon-Acuna A, Lopez-Moreno J, Delgado-Lista J, Gomez-Marin B, et al. Apolipoprotein E genetic variants interact with Mediterranean diet to modulate postprandial hypertriglyceridemia in coronary heart disease patients: CORDIOPREV study. Eur J Clin Invest. (2019) 49:e13146. doi: 10.1111/eci.13146
39. Delgado-Lista J, Perez-Martinez P, Garcia-Rios A, Alcala-Diaz JF, Perez-Caballero AI, Gomez-Delgado F, et al. CORonary Diet Intervention with Olive oil and cardiovascular PREVention study (the CORDIOPREV study): rationale, methods, and baseline characteristics: a clinical trial comparing the efficacy of a Mediterranean diet rich in olive oil versus a low-fat diet on cardiovascular disease in coronary patients. Am Heart J. (2016) 177:42–50. doi: 10.1016/j.ahj.2016.04.011
40. Quintana-Navarro GM, Alcala-Diaz JF, Lopez-Moreno J, Perez-Corral I, Leon-Acuna A, Torres-Pena JD, et al. Long-term dietary adherence and changes in dietary intake in coronary patients after intervention with a Mediterranean diet or a low-fat diet: the CORDIOPREV randomized trial. Eur J Nutr. (2020) 59:2099–110. doi: 10.1007/s00394-019-02059-5
41. Estruch R, Ros E, Salas-Salvado J, Covas MI, Corella D, Aros F, et al. Primary prevention of cardiovascular disease with a Mediterranean diet supplemented with extra-virgin olive oil or nuts. N Engl J Med. (2018) 378:e34. doi: 10.1056/NEJMoa1800389
42. Alcala-Diaz JF, Delgado-Lista J, Perez-Martinez P, Garcia-Rios A, Marin C, Quintana-Navarro GM, et al. Hypertriglyceridemia influences the degree of postprandial lipemic response in patients with metabolic syndrome and coronary artery disease: from the CORDIOPREV study. PLoS One. (2014) 9:e96297. doi: 10.1371/journal.pone.0096297
43. Gomez-Delgado F, Alcala-Diaz JF, Garcia-Rios A, Delgado-Lista J, Ortiz-Morales A, Rangel-Zuniga O, et al. Polymorphism at the TNF-alpha gene interacts with Mediterranean diet to influence triglyceride metabolism and inflammation status in metabolic syndrome patients: from the CORDIOPREV clinical trial. Mol Nutr Food Res. (2014) 58:1519–27. doi: 10.1002/mnfr.201300723
44. Yu E, Malik VS, Hu FB. Cardiovascular disease prevention by diet modification: JACC health promotion series. J Am Coll Cardiol. (2018) 72:914–26. doi: 10.1016/j.jacc.2018.02.085
45. Eberly LE, Stamler J, Neaton JD, Multiple Risk Factor Intervention Trial Research Group. Relation of triglyceride levels, fasting and nonfasting, to fatal and nonfatal coronary heart disease. Arch Intern Med. (2003) 163:1077–83. doi: 10.1001/archinte.163.9.1077
46. Nordestgaard BG, Benn M, Schnohr P, Tybjaerg-Hansen A. Nonfasting triglycerides and risk of myocardial infarction, ischemic heart disease, and death in men and women. JAMA. (2007) 298:299–308. doi: 10.1001/jama.298.3.299
47. Ferguson LR, De Caterina R, Gorman U, Allayee H, Kohlmeier M, Prasad C, et al. Guide and position of the international society of nutrigenetics/nutrigenomics on personalised nutrition: part 1 - fields of precision nutrition. J Nutrigenet Nutrigenomics. (2016) 9:12–27. doi: 10.1159/000445350
48. Cui G, Tian M, Hu S, Wang Y, Wang DW. Identifying functional non-coding variants in APOA5/A4/C3/A1 gene cluster associated with coronary heart disease. J Mol Cell Cardiol. (2020) 144:54–62. doi: 10.1016/j.yjmcc.2020.05.003
49. Rosenthal EA, Crosslin DR, Gordon AS, Carrell DS, Stanaway IB, Larson EB, et al. Association between triglycerides, known risk SNVs and conserved rare variation in SLC25A40 in a multi-ancestry cohort. BMC Med Genomics. (2021) 14:11. doi: 10.1186/s12920-020-00854-2
50. Weissglas-Volkov D, Aguilar-Salinas CA, Nikkola E, Deere KA, Cruz-Bautista I, Arellano-Campos O, et al. Genomic study in Mexicans identifies a new locus for triglycerides and refines European lipid loci. J Med Genet. (2013) 50:298–308. doi: 10.1136/jmedgenet-2012-101461
51. Guan F, Niu Y, Zhang T, Liu S, Ma L, Qi T, et al. Two-stage association study to identify the genetic susceptibility of a novel common variant of rs2075290 in ZPR1 to type 2 diabetes. Sci Rep. (2016) 6:29586. doi: 10.1038/srep29586
52. Yang L, Ma L, Guo W, Fang Q, Lai X, Zhang X. Interaction of polymorphisms in APOA4-APOA5-ZPR1-BUD13 gene cluster and sleep duration on 5-year lipid changes in middle aged and older Chinese. Sleep. (2019) 42:zsz115. doi: 10.1093/sleep/zsz115
53. Saliminejad K, Khorram Khorshid HR, Soleymani Fard S, Ghaffari SH. An overview of microRNAs: biology, functions, therapeutics, and analysis methods. J Cell Physiol. (2019) 234:5451–65. doi: 10.1002/jcp.27486
54. Corella D, Sorli JV, Estruch R, Coltell O, Ortega-Azorin C, Portoles O, et al. MicroRNA-410 regulated lipoprotein lipase variant rs13702 is associated with stroke incidence and modulated by diet in the randomized controlled PREDIMED trial. Am J Clin Nutr. (2014) 100:719–31. doi: 10.3945/ajcn.113.076992
55. Richardson K, Nettleton JA, Rotllan N, Tanaka T, Smith CE, Lai CQ, et al. Gain-of-function lipoprotein lipase variant rs13702 modulates lipid traits through disruption of a microRNA-410 seed site. Am J Hum Genet. (2013) 92:5–14. doi: 10.1016/j.ajhg.2012.10.020
56. Boquist S, Karpe F, Danell-Toverud K, Hamsten A. Effects of atorvastatin on postprandial plasma lipoproteins in postinfarction patients with combined hyperlipidaemia. Atherosclerosis. (2002) 162:163–70. doi: 10.1016/s0021-9150(01)00689-x
57. Reyes-Soffer G, Ngai CI, Lovato L, Karmally W, Ramakrishnan R, Holleran S, et al. Effect of combination therapy with fenofibrate and simvastatin on postprandial lipemia in the ACCORD lipid trial. Diabetes Care. (2013) 36:422–8. doi: 10.2337/dc11-2556
Keywords: postprandial triglycerides, diet, coronary artery disease, single nucleotide polymorphism, SNP, nutrigenomics, nutrigenetics
Citation: Alcala-Diaz JF, Arenas-de Larriva AP, Torres-Peña JD, Rodriguez-Cantalejo F, Rangel-Zuñiga OA, Yubero-Serrano EM, Gutierrez-Mariscal FM, Cardelo MP, Luque RM, Ordovas JM, Perez-Martinez P, Delgado-Lista J and Lopez-Miranda J (2022) A Gene Variation at the ZPR1 Locus (rs964184) Interacts With the Type of Diet to Modulate Postprandial Triglycerides in Patients With Coronary Artery Disease: From the Coronary Diet Intervention With Olive Oil and Cardiovascular Prevention Study. Front. Nutr. 9:885256. doi: 10.3389/fnut.2022.885256
Received: 27 February 2022; Accepted: 09 May 2022;
Published: 17 June 2022.
Edited by:
Jarlei Fiamoncini, University of São Paulo, BrazilReviewed by:
Gabriela Laiber Pascoal, University of São Paulo, BrazilThomas Skurk, Technical University of Munich, Germany
Copyright © 2022 Alcala-Diaz, Arenas-de Larriva, Torres-Peña, Rodriguez-Cantalejo, Rangel-Zuñiga, Yubero-Serrano, Gutierrez-Mariscal, Cardelo, Luque, Ordovas, Perez-Martinez, Delgado-Lista and Lopez-Miranda. This is an open-access article distributed under the terms of the Creative Commons Attribution License (CC BY). The use, distribution or reproduction in other forums is permitted, provided the original author(s) and the copyright owner(s) are credited and that the original publication in this journal is cited, in accordance with accepted academic practice. No use, distribution or reproduction is permitted which does not comply with these terms.
*Correspondence: Jose Lopez-Miranda, bWQxbG9taWpAdWNvLmVz
†These authors have contributed equally to this work and share first authorship
‡These authors share senior authorship