- 1Facultad de Deportes, Universidad Autónoma de Baja California, Ensenada, Mexico
- 2Department of Health, Sports and Exercise Sciences, University of Kansas, Lawrence, KS, United States
- 3Human Movement Sciences Research Center (CIMOHU), University of Costa Rica, San José, Costa Rica
- 4Exercise and Immunometabolism Research Group, Department of Physical Education, Paulista State University, UNESP, Presidente Prudente, São Paulo, Brazil
Since 2020, the world has been suffering from a pandemic that has affected thousands of people regardless of socio-economic conditions, forcing the population to adopt different strategies to prevent and control the advance of the disease, one of which is social distancing. Even though social distancing is a safe strategy to reduce the spread of COVID-19, it is also the cause of a rising sedentary behavior. This behavior develops an excess of fat tissue that leads to metabolic and inflammatory disruption related to chronic diseases and mental health disorders, such as anxiety, depression, and sleep issues. Furthermore, the adoption of dietary patterns involving the consumption of ultra-processed foods, higher in fats and sugars, and the reduction of fresh and healthy foods may play a role in the progress of the disease. In this perspective, we will discuss how an unhealthy diet can affect brain function and, consequently, be a risk factor for mental health diseases.
Introduction
The COVID-19 outbreak started in March of 2020; this human threat substantially modified the lifestyle of people around the world. Quarantine and social distancing were the two well-known initial preventive care measures imposed by governments worldwide to minimize the spread of infection of COVID-19. Because of the fast virus spread, schools were closed, national and international travel was restricted or forbidden, and other social activities, such as amateur and professional sports tournaments and musicals, were canceled. In addition, hundreds of countries kept their population in lockdown at home in isolation indefinitely to reduce the risk of transmission of the COVID-19. Although social distancing is a safe strategy to reduce the spread of the COVID-19, the lockdown increased sedentary behavior [might be defined as an energy expenditure of ≤1.5 metabolic equivalents of task (METs)] (1), mental and physical health problems (anxiety, depression, and others) (2, 3), and sleep and circadian rhythm disruption in the population (4, 5). The latter impacts body composition by promoting the greater intake of high energy-dense food types (5, 6). On the other hand, circadian misalignment can be achieved by alteration of the sleep and feed patterns (specially the increase in high-fat food intake), and potentially leading to cardiovascular disease (7). Furthermore, circadian clock genes trigger the onset of metabolic disorders, including metabolic syndrome (MetS) (7, 8).
The gain of excessive adipose tissue leads to local and systemic pro-inflammatory conditions, impairing glucose metabolism, and the onset of metabolic disorders (e.g., type-2 diabetes -T2D-), altering the functionality of organs and systems evenly. Moreover, the pro-inflammatory state per se harms the structure of brain topological integration and function. Similarly, poor diet quality, defined as the diet with a reduced variety and nutritional deficiency, does not align with international guidelines (9), represents another factor that generates dysfunctional brain activity (10). Unfortunately, during the COVID-19 lockdown, the population adopted unhealthy diet patterns from previous bad habits or eating behaviors or by emerging social conditions (e.g., reduction of income as a direct consequence of a sharp raising in the unemployment rate) (11, 12). Thereby, scientists and international organizations recommended maintaining a healthy diet focusing on strengthening the immune system and coping with the COVID-19 infection (13–15). However, as mentioned above, an unhealthy diet is a factor that negatively affects brain function. Therefore, the present perspective article briefly discusses how a current poor diet in the population during the COVID-19 lockdown might affect brain health.
Ultra-Processed Food-Based Diet: A Risk Factor for Brain Dysfunction During COVID-19
According to information provided by international organizations and scientists, ultra-processed foods (UPFs) have undergone excessive industrial manufacture. As a result, UPFs are deficient in dietary fiber, protein, and micronutrients, these products contain little to no whole foods, (16–18). Furthermore, UPFs are energy-dense products that contain artificial components that modify textures, flavors, and colors, producing palatable and more attractive foods (17). The UPFs are typically ready for consumption like soft drinks, sugar drinks, fatty or salty snack products, ice cream, French fries, burgers, desserts, and more products offered as a whole variety of fast foods (16, 17, 19).
The excessive consumption of UPFs is considered the primary source of non-communicable diseases (i.e., obesity, MetS, T2D, etc.) (19). In addition, during the COVID-19 lockdown, individuals have reported higher UPFs consumption in contrast to pre-pandemic times (20–24).
Currently, some studies pointed out the excess of dietary fats can promote changes in gut-microbiota and favor augmented lipopolysaccharides (LPS) extravasation to blood (25). Augmented LPS in blood lead to Toll-Like Receptor 4 (TLR-4) activation via binding the cellular membrane, stimulating pro-inflammatory signaling cascades, increasing cytokine synthesis (TNFα, interleukin -IL- 1B, IL-6, and interferon γ –IFNγ-). This constant cycle (higher dietary fat intake and blood LPS) favors the development of chronic metabolic disruptions, like insulin resistance (26). Recently, Teixeira et al. (27) demonstrated an increased microbial translocation and hyper inflammation in patients with severe COVID-19, provoking higher monocyte activation, which may be associated with worsening outcomes, including death.
Linked with the preponderance to UPFs ingestion, the SARS-Cov2 virus directly and indirectly affects at-risk populations (e.g., hypertensive patients, aged people). Social distancing has also caused the world population's physical activity reduction (2) both lifestyle habits induce body weight gain. In agreement, recent work reported that obesity prevalence has raised during the ongoing social distancing (28, 29). In obesity, immune, adipose tissue, skeletal muscle, and liver engage in a particular crosstalk leading to IR (30–38).
There is evidence that IR leads to hyperglycemia and a parallel increase in pancreatic β-cell insulin secretion (i.e., hyperinsulinemia) (39, 40). These conditions often lead to a cascade of metabolic risk factors collectively referred to as MetS, characterized by central obesity, IR, dyslipidemia, and hypertension (41), and it is known to increase T2D risk by over 2-fold (42–44). Contrary to MetS, T2D is mainly impaired insulin secretion resulting from IR (42).
Besides the pathological effects of MetS and T2D on peripheral organs, recent evidence also suggests a negative impact on brain function and surrounding areas (45–48), such as the blood-brain barrier (BBB) (49–52). The BBB regulates the molecular exchange between the peripheral blood and the brain (53, 54). Conceptual models suggest that chronic peripheral inflammation due to T2D and MetS increases the BBB permeability to leucocytes and external molecules into the brain (41, 42). Thus, the cerebral response begins with an inflammatory response (43, 44), followed by a pro-inflammatory response that alters endothelial cells (ECs), increasing the BBB permeability (55, 56). Other studies show that T2D increases the inflammatory profile of ECs and BBB permeability, a response closely associated with cognitive impairment (57, 58).
In addition, MetS depicts elevated serum triacylglycerol (TGs) and low high-density lipoprotein (HDL-c) concentrations (33). Nevertheless, cross-sectional studies have reported equivocal findings regarding the association between high serum TGs and cognitive function in humans (59, 60). Some authors report an adverse effect of TGs on cognitive function (46, 60), while others suggest a positive outcome on brain function (45). In this regard, it is worth indicating that the former study was in a Chinese sample, whereas the other studies were in the western populations. This evidence suggests racial/ethnic disparities in the effects of TGs on cognitive function. Finally, IR in the brain induced by TGs was also demonstrated (47).
Another concern in MetS is the continuous hyperglycemic state that facilitates the non-enzymatic interaction between glucose and proteins (48, 49). Glycated protein generates advanced glycation end-products (AGEs). These molecules have been associated with reduction in BBB integrity (39, 49). Moreover, AGEs activate the synthesis of pro-inflammatory cytokines in the BBB's ECs, causing a pro-inflammatory feedback loop (42). Chronic hyperglycemia triggers several metabolic signaling mechanisms that induce inflammation, apoptosis, and the synthesis of reactive oxygen species (ROS) (50). Additionally, studies performed in obese rodents show that ROS increases BBB permeability, reducing the expression of proteins associated with tight junctions (37, 51). On the other hand, hyperglycemia per se harms the brain in patients with T2D (31, 52, 53). At least two mechanisms negatively contribute to this: 1) hyperglycemia is associated with brain atrophy (53), 2), hyperglycemia increases the risk for stroke, leading to brain acidosis (52, 54). Furthermore, hyperglycemia increases the activity of excitatory neurotransmitters (e.g., glutamate), resulting in a higher calcium concentration in neural cytosol to induce cell death (54). Finally, hyperglycemia is a factor that reduces the topological integration in brain (45), which possibly contributes to cognitive impairment in T2D (45) (Figure 1).
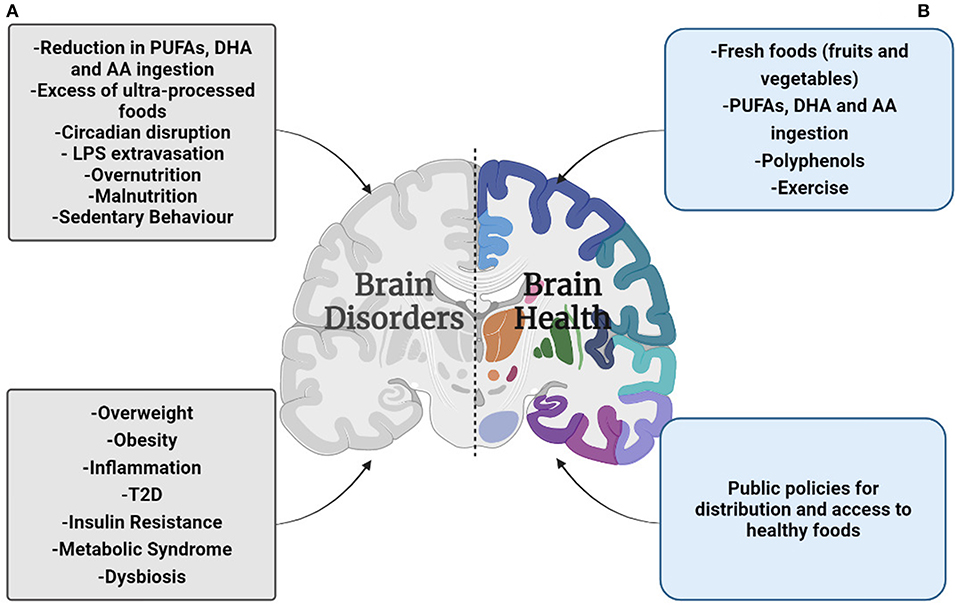
Figure 1. Summary overview of the malnutrition effects on brain health during the COVID-19 pandemic. (A) The preponderance by the UPF's ingestion, circadian disruption, and a sedentary lifestyle will facility the gain of body weight, leading to suffering overweight/obesity, and in a worst-case scenario suffer metabolic syndrome and T2D. Moreover, overweight/obesity increases the risk of low-grade chronic inflammation; the pro-inflammatory cytokines hinder the blood-brain barrier function deteriorating brain health. Additionally, the higher rate of unemployment and the rising price of food have reduced the affordability of fresh products, and protein based-products, resulting in a poor quality diet. This condition is a factor that reduces brain integrity that leads to suffering brain disorders. (B) The public services focused to facilitate the practice of physical exercise during the lockdown, and better distribution and access to healthy food will strengthen the brain health and reduce the risk to suffer brain disorders.
Poor Diet Quality: A Risk Factor for Brain Integrity During COVID-19
The human body is a structure that requires energy for anabolic and catabolic processes. In this sense, ingesting food was initially considered a survival practice. However, anthropologists proved hypotheses concerning the diet role in Hominids evolution (61–65). For instance, cooking foods improved digestion capacity (62, 66). Additionally, cuisine foods increased the availability of the nutrients present in plants and meat (66). The previous conditions and others facilitated the brain evolution (i.e., encephalization) (62, 67). Therefore, the diet components have played a relevant role throughout the Homo evolution in conjunction with food processing. In this sense, fatty acids, mainly the long-chain polyunsaturated fatty acids (PUFAs), docosahexaenoic acid (DHA), and arachidonic acid (AA), have provided essential effects on brain evolution (63, 68). Moreover, AA is a lipid that strengthens synaptic transmission (69, 70). However, the AA is also a precursor of molecules linked with inflammatory responses such as prostaglandins and pro-inflammatory cytokines, such as TNFα and IL-1B (71, 72). Another example of dietary adaptations is the lactose tolerance of some populations. The latter is due to milk consumption after the weaning period, keeping the lactase enzyme active (73). As can be noted, the diet helps humans deal with the context of living, allowing us to say that we are what we eat. Therefore, the population who show a poor-quality diet will have few tools to cover all the surrounding challenges.
The COVID-19 lockdown disrupted the dietary patterns in the world population (74, 75), affecting low-and-middle-income countries (11, 12, 76, 77). Therefore, besides the enhancing effect on the UPFs consumption, the COVID-19 outbreak reduced food security, and consequently, the dietary quality (11, 12, 75, 78, 79). Food security is a complex phenomenon that implicates time, physical and economic access to sufficient healthy food to satisfy the nutritional needs and food preferences for a healthy lifestyle (80). The opposite condition is known as food insecurity (FI) (80, 81), which is related to malnutrition (i.e., undernutrition and micronutrient deficiency) (81). During the COVID-19 lockdown, unemployment growth and increased food prices were the main factors reducing food affordability (11). In addition, the lockdown restrictions reduced the food supply chain (78), which in turn reduced the ingestion of fresh products such as fruit and vegetables (75). Together with the prior information, other authors have reported that the population with high FI scores showed higher anxiety levels (78), independent of the socio-economic factors (82). Besides the FI, an inverse relationship is reported between quality diet and anxiety levels in individuals undergoing lockdown (29).
The nutrient deficiency intake might impact brain function. For instance, the PUFAs role on neural membrane integrity, gray matter, and hippocampal volume (83), makes them an important nutrient whose low ingestion contributes to a reduced brain plasticity (83–85). Together with lipid actions, other molecules are also essential to strengthen brain function. Concretely, polyphenols found in fruits and vegetables also have positive effects (86). Resveratrol for example, a phytoalexin present in grapes, berries, tomatoes, nuts, and cocoa (87), demonstrated positive effects on brain function and structure (88, 89). Chronic consumption of resveratrol led to a better cognitive performance (i.e., improving memory) and mood in postmenopausal women (89), the hypothetical mechanism explaining these responses was a better cerebral perfusion modulation in the participants (89). A similar effect was reported in healthy men (90); moreover, this polyphenol enhances the functional connectivity from the hippocampus to frontal, parietal, and occipital areas, improvement in the memory retention correlated with a topological shift in brain, and glucose metabolism in healthy older adults (91).
Conversely, protein malnutrition (PMN) is a risk factor for neuroinflammation and oxidative stress (10, 92, 93). Moreover, the PMN in pregnant women affects brain development and cognition considerably in the offspring (10). The previous findings emphasize the impact of the diet on brain integrity during the COVID-19 outbreak (Figure 1).
Poor diet, nutrient availability, and quality will also impact the gut microbiome and, eventually, brain health. Intestinal content and the brain represent a dynamic bidirectional communication described as the “gut (microbiota)-brain axis” (94). The human gut microbiome includes different types of bacteria responsible for several functions such as energy metabolism, immunity, vitamin synthesis, hormone, and neurotransmitter production, and it also influences human behavior (94–96). Environmental factors (e.g., diet changes caused by the COVID-19 pandemic, medication, exercise) can potentially change the gut microbiome rapidly. In addition, special conditions might lead to a microbial imbalance (i.e., dysbiosis), a factor contributing or associated to the development of some diseases like inflammatory bowel disease (97), atopic diseases (e.g., eczema, asthma, food allergies) (98), type-1 diabetes (99), schizophrenia, and other cognitive disorders (94, 100, 101). Changes in the gut microbiome have shown concomitant changes in brain structure, function, and behaviors (e.g., stress, anxiety, depression) (94, 101). Indeed, the effect of gut microbiome diversity on brain function is partially accounted for by vitamin-mediated neuronal function, neurotransmitter composition, and short-chain fatty acid (sCFas) metabolites (94, 102).
Previous evidence suggests that vagal afferent sensory neurons are microbiota-mediated, regulating information transmission through the kynurenine pathway (103). Probiotic supplementation impacts the central nervous system, and research has shown its effects on anxiety disorders (i.e., anxiolytic effect) (102, 104). Although specific probiotic species affecting brain health are currently under study, recent evidence suggests that anxiety and depressive disorders correlate to higher pro-inflammatory species and lower abundance of sCFas-producing species (105).
Conclusions and Final Remarks
Social distancing was a strategy implemented worldwide by several governments to reduce the risk of COVID-19 infection. However, this outbreak has impacted the household economy considerably, reducing food affordability and, consequently, the food quality. Although different documents highlight the diet's relevance to strengthening the immune system, there is a lack of emphasis on the diet's role in maintaining brain integrity and functionality during the COVID-19 outbreak. In the current work, we discussed how overweight and obesity impact brain function. Even though this effect is widely reported, the social distancing during COVID-19 increased the risk of suffering obesity. We also discussed how undernutrition is a condition with deleterious effects on brain integrity. Although the mobility restriction is less severe today, the economic impact of the COVID-19 pandemic is still present in society; furthermore, it is projected to reach the pre-pandemic levels until 2023 (106). This scenario impairs individuals' availability to secure adequate nutrients and causes changes in the gut microbiome, resulting in vulnerable brain health and increasing the risk of suffering anxiety, cognitive deficiency, mental disorders, and impaired mood. Finally, different authors indicated that the brain is directly and indirectly affected by COVID-19 (107–109). Therefore, we believe that a fragile brain resulting from malnutrition (i.e., over-nutrition and undernutrition) could worsen the consequences after the COVID-19 infection. Consequently, we consider that governments worldwide must develop strategies to improve the diet quality in the population, mainly during the COVID-19 outbreak. If fulfilled, the possibility of increasing brain health in children, adults, and the elderly is nigh.
Data Availability Statement
The original contributions presented in the study are included in the article/supplementary material, further inquiries can be directed to the corresponding author.
Author Contributions
IR, PG-S, and JM-P reviewed the literature, wrote the first draft, and finalized the manuscript. JM-J, BA, and FL finalized the manuscript, BA conceived and designed Figure 1. AJ-M conceived the article focus, reviewed the literature, and wrote the first draft. All authors approved the final version of the manuscript.
Conflict of Interest
The authors declare that the research was conducted in the absence of any commercial or financial relationships that could be construed as a potential conflict of interest.
Publisher's Note
All claims expressed in this article are solely those of the authors and do not necessarily represent those of their affiliated organizations, or those of the publisher, the editors and the reviewers. Any product that may be evaluated in this article, or claim that may be made by its manufacturer, is not guaranteed or endorsed by the publisher.
References
1. Thivel D, Tremblay A, Genin PM, Panahi S, Rivière D, Duclos M. Physical activity, inactivity, and sedentary behaviors: definitions and implications in occupational health. Front Public Health. (2018) 6:288. doi: 10.3389/fpubh.2018.00288
2. Tison GH, Avram R, Kuhar P, Abreau S, Marcus GM, Pletcher MJ, et al. Worldwide effect of COVID-19 on physical activity: a descriptive study. Ann Intern Med. (2020) 173:767–70. doi: 10.7326/M20-2665
3. Balanzá-Martínez V, Kapczinski F, de Azevedo Cardoso T, Atienza-Carbonell B, Rosa AR, Mota JC, et al. The assessment of lifestyle changes during the COVID-19 pandemic using a multidimensional scale. Rev Psiquiatr Salud Ment. (2021) 14:1. doi: 10.1016/j.rpsm.2020.07.003
4. Morin CM, Carrier J, Bastien C, Godbout R. Sleep and circadian rhythm in response to the COVID-19 pandemic. Can J Public Heal. (2020) 111:654–7. doi: 10.17269/s41997-020-00382-7
5. Romdhani M, Rae DE, Nédélec M, Ammar A, Chtourou H, Al Horani R, et al. COVID-19 lockdowns: a worldwide survey of circadian rhythms and sleep quality in 3911 athletes from 49 countries, with data-driven recommendations. Sport Med. (2021) 8:1–16. doi: 10.1007/s40279-021-01601-y
6. Broussard JL, Cauter E Van. Disturbances of sleep and circadian rhythms: novel risk factors for obesity. Curr Opin Endocrinol Diabetes Obes. (2016) 23:353–9. doi: 10.1097/MED.0000000000000276
7. Machado RM, Koike MK. Circadian rhythm, sleep pattern, and metabolic consequences: an overview on cardiovascular risk factors. Horm Mol Biol Clin Investig. (2014) 18:47–52. doi: 10.1515/hmbci-2013-0057
8. Škrlec I, Talapko J, DŽijan S, Cesar V, Lazić N, Lepeduš H. The association between circadian clock gene polymorphisms and metabolic syndrome: a systematic review and meta-analysis. Biology (Basel) (2022) 11:1–14. doi: 10.3390/biology11010020
9. Wirt A, Collins CE. Diet quality - what is it and does it matter? Public Health Nutr. (2009) 12(12). doi: 10.1017/S136898000900531X
10. Sinha S, Patro N, Patro IK. Maternal protein malnutrition: current and future perspectives of spirulina supplementation in neuroprotection. Front Neurosci. (2018) 12: 966. doi: 10.3389/fnins.2018.00966
11. Madzorera I, Ismail A, Hemler EC, Korte ML, Olufemi AA, Wang D, et al. Impact of COVID-19 on nutrition, food security, and dietary diversity and quality in Burkina Faso, Ethiopia, and Nigeria. Am J Trop Med Hyg. (2021) 105:2. doi: 10.4269/ajtmh.20-1617
12. Picchioni F, Goulao LF, Roberfroid D. The impact of COVID-19 on diet quality, food security and nutrition in low and middle income countries: a systematic review of the evidence. Clin Nutr. (2021) 1532–983. doi: 10.1016/j.clnu.2021.08.015
13. Eiser AR. Could dietary factors reduce COVID-19 mortality rates? moderating the inflammatory state. J Altern Complement Med. (2021):27. doi: 10.1089/acm.2020.0441
14. Calder PC. Nutrition, immunity and COVID-19. BMJ Nutr Prev Heal. (2020) 3:1. doi: 10.1136/bmjnph-2020-000085
15. Arshad MS, Khan U, Sadiq A, Khalid W, Hussain M, Yasmeen A, et al. Coronavirus disease (COVID-19) and immunity booster green foods: a mini review. Food Sci Nutr. (2020) 8. 3971–976. doi: 10.1002/fsn3.1719
16. Monteiro CA, Cannon G, Moubarac JC, Levy RB, Louzada MLC, Jaime PC. The un decade of Nutrition, the NOVA food classification and the trouble with ultra-processing. Public Health Nutr. (2018) 21: 5–17. doi: 10.1017/S1368980017000234
17. Monteiro CA, Cannon G, Lawrence M, Costa Louzada ML, Machado PP. The NOVA Food Classification System and Its Four Food Groups. Ultra-Processed Foods, Diet Quality, and Health Using the NOVA Classification System. Rome: FAO (2019) p. 6–9.
18. Fardet A, Rock E. Ultra-processed foods and food system sustainability: what are the links? Sustainability (Switzerland). (2020) 12:6280. doi: 10.3390/su12156280
19. Pan American Health Organization. Ultra-Processed Food and Drink Products in Latin America: Sales, Sources, Nutrient Profiles and Policy Implications. Washington, DC: PAHO (2019) p. 72.
20. Lopez KV, Garduño AMJ, Regules AEO, Romero LMI, Martinez OAG, Pereira TSS. Lifestyle and nutrition changes during the SARS-CoV-2 (COVID-19) lockdown in México: an observational study. Rev Esp Nutr Humana y Diet. (2021) 25:1–21. doi: 10.14306/renhyd.25.S2.1099
21. Ruiz-Roso MB, Padilha P de C, Mantilla-Escalante DC, Ulloa N, Brun P, Acevedo-Correa D, et al. Covid-19 confinement and changes of adolescent's dietary trends in Italy, Spain, Chile, Colombia and Brazil. Nutrients. (2020) 12:6. doi: 10.3390/nu12061807
22. Clemente-Suárez VJ, Ramos-Campo DJ, Mielgo-Ayuso J, Dalamitros AA, Nikolaidis PA, Hormeño-Holgado A, et al. Nutrition in the actual covid-19 pandemic. a narrative review. Nutrients. (2021) 13:1924. doi: 10.3390/nu13061924
23. Zupo R, Castellana F, Sardone R, Sila A, Giagulli VA, Triggiani V, et al. Preliminary trajectories in dietary behaviors during the COVID-19 pandemic: a public health call to action to face obesity. Int J Environ Res. (2020) 17:7073. doi: 10.3390/ijerph17197073
24. Smaira FI, Mazzolani BC, Esteves GP, André HCS, Amarante MC, Castanho DF, et al. Poor eating habits and selected determinants of food choice were associated with ultraprocessed food consumption in Brazilian women during the COVID-19 pandemic. Front Nutr. (2021) 8:672372. doi: 10.3389/fnut.2021.672372
25. Lancaster GI, Langley KG, Berglund NA, Kammoun HL, Reibe S, Estevez E, et al. Evidence that TLR4 is not a receptor for saturated fatty acids but mediates lipid-induced inflammation by reprogramming macrophage metabolism. Cell Metab. (2018) 27:5. doi: 10.1016/j.cmet.2018.03.014
26. Lira FS, Rosa JC, Pimentel GD, Santos R V, Carnier J, Sanches PL, et al. Long-term interdisciplinary therapy reduces endotoxin level and insulin resistance in obese adolescents. Nutr J. (2012) 11:1. doi: 10.1186/1475-2891-11-74
27. Teixeira PC, Dorneles GP, Santana Filho PC, da Silva IM, Schipper LL, Postiga IAL, et al. Increased LPS levels coexist with systemic inflammation and result in monocyte activation in severe COVID-19 patients. Int Immunopharmacol. (2021) 100:108125. doi: 10.1016/j.intimp.2021.108125
28. Lange SJ, Kompaniyets L, Freedman DS, Kraus EM, Porter R, Blanck HM, et al. Morbidity and Mortality Weekly Report Longitudinal Trends in Body Mass Index Before and During the Covid-19 Pandemic Among Persons Aged 2–19 Years-United States, 2018–2020. Washington, DC: Morbidity and Mortality Weekly Reports (2018).
29. Kaufman-Shriqui V, Navarro DA, Raz O, Boaz M. Multinational dietary changes and anxiety during the coronavirus pandemic-findings from Israel. Isr J Health Policy Res. (2021) 10:1. doi: 10.1186/s13584-021-00461-1
30. Lorenzo C, Okoloise M, Williams K, Stern MP, Haffner SM. The metabolic syndrome as predictor of type 2 diabetes: the san antonio heart study. Diabetes Care. (2003) 26:11. doi: 10.2337/diacare.26.11.3153
31. Kim DJ, Yu JH, Shin MS, Shin YW, Kim MS. Hyperglycemia reduces efficiency of brain networks in subjects with type 2 diabetes. PLoS ONE. (2016) 11:1–14. doi: 10.1371/journal.pone.0157268
32. Nägga K, Gustavsson A-M, Stomrud E, Lindqvist D, van Westen D, Blennow K, et al. Increased midlife triglycerides predict brain β-amyloid and tau pathology 20 years later. Neurology. (2018) 90:e73–81. doi: 10.1212/WNL.0000000000004749
33. Banks WA, Coon AB, Robinson SM, Moinuddin A, Shultz JM, Nakaoke R, et al. Triglycerides induce leptin resistance at the blood-brain barrier. Diabetes. (2004) 53:1253–60. doi: 10.2337/diabetes.53.5.1253
34. Moheet A, Mangia S, Seaquist ER. Impact of diabetes on cognitive function and brain structure. Ann N Y Acad Sci. (2015) 1353:60–71. doi: 10.1111/nyas.12807
35. Rutkowsky JM, Lee LL, Puchowicz M, Golub MS, Befroy DE, Wilson DW, et al. Reduced cognitive function, increased bloodbrain-barrier transport and inflammatory responses, and altered brain metabolites in LDLr-/-And C57BL/6 mice fed a western diet. PLoS ONE. (2018) 13:191909. doi: 10.1371/journal.pone.0191909
36. Hargrave SL, Davidson TL, Zheng W, Kinzig KP. Western diets induce blood-brain barrier leakage and alter spatial strategies in rats. Behav Neurosci. (2016) 130:123–35. doi: 10.1037/bne0000110
37. Salameh TS, Mortell WG, Logsdon AF, Butterfield DA, Banks WA. Disruption of the hippocampal and hypothalamic blood-brain barrier in a diet-induced obese model of type II diabetes: prevention and treatment by the mitochondrial carbonic anhydrase inhibitor, topiramate. Fluids Barriers CNS. (2019) 16:1–17. doi: 10.1186/s12987-018-0121-6
38. Yoo DY, Yim HS, Jung HY, Nam SM, Kim JW, Choi JH, et al. Chronic type 2 diabetes reduces the integrity of the blood-brain barrier by reducing tight junction proteins in the hippocampus. J Vet Med Sci. (2016) 78:957–62. doi: 10.1292/jvms.15-0589
39. Bogush M, Heldt NA, Persidsky Y. Blood brain barrier injury in diabetes: unrecognized effects on brain and cognition. J Neuroimmune Pharmacol. (2017) 12:593–601. doi: 10.1007/s11481-017-9752-7
40. Shalev H, Serlin Y, Friedman A. Breaching the blood-brain barrier as a gate to psychiatric disorder. Cardiovasc Psychiatry Neurol. (2009) 2009:1–7. doi: 10.1155/2009/278531
41. Mauro C, De Rosa V, Marelli-Berg F, Solito E. Metabolic syndrome and the immunological affair with the blood-brain barrier. Front Immunol. (2015) 6:677. doi: 10.3389/fimmu.2014.00677
42. Van Dyken P, Lacoste B. Impact of metabolic syndrome on neuroinflammation and the blood–brain barrier. Front Neurosci. (2018) 12:930. doi: 10.3389/fnins.2018.00930
43. Takechi R, Lam V, Brook E, Giles C, Fimognari N, Mooranian A, et al. Blood-brain barrier dysfunction precedes cognitive decline and neurodegeneration in diabetic insulin resistant mouse model: an implication for causal link. Front Aging Neurosci. (2017) 9:399. doi: 10.3389/fnagi.2017.00399
44. Hwang IK, Choi JH, Nam SM, Park OK, Yoo DY, Kim W, et al. Activation of microglia and induction of proinflammatory cytokines in the hippocampus of type 2 diabetic rats. Neurol Res. (2014) 36:824–32. doi: 10.1179/1743132814Y.0000000330
45. Yin ZX, Shi XM, Kraus VB, Fitzgerald SM, Qian HZ, Xu JW, et al. High normal plasma triglycerides are associated with preserved cognitive function in chinese oldest-old. Age Ageing. (2012) 41:600–6. doi: 10.1093/ageing/afs033
46. Parthasarathy V, Frazier DT, Bettcher BM, Jastrzab L, Chao L, Reed B, et al. Triglycerides are negatively correlated with cognitive function in non-demented aging adults. Neuropsychology. (2017) 31:682–8. doi: 10.1037/neu0000335
47. Banks WA, Farr SA, Salameh TS, Niehoff ML, Rhea EM, Morley JE, et al. Triglycerides cross the blood – brain barrier and induce central leptin and insulin receptor resistance. Nat Publ Gr. (2017) 42:391–7. doi: 10.1038/ijo.2017.231
48. Singh VP, Bali A, Singh N, Jaggi AS. Advanced glycation end products and diabetic complications. Korean J Physiol Pharmacol. (2014) 18:1–14. doi: 10.4196/kjpp.2014.18.1.1
49. Ferreira LSS, Fernandes CS, Vieira MNN, De Felice FG. Insulin resistance in Alzheimer ' s disease. Front Neurosci. (2018) 12:830. doi: 10.3389/fnins.2018.00830
50. de Oliveira Sá G, dos Santos Neves V, de Oliveira Fraga SR, Souza-Mello V, Barbosa-da-Silva S. High-intensity interval training has beneficial effects on cardiac remodeling through local renin-angiotensin system modulation in mice fed high-fat or high-fructose diets. Life Sci. (2017) 189:8–17. doi: 10.1016/j.lfs.2017.09.012
51. Salameh TS, Shah GN, Price TO, Hayden MR, Banks WA. Blood-brain barrier disruption and neurovascular unit dysfunction in diabetic mice: protection with the mitochondrial carbonic anhydrase inhibitor topiramate. J Pharmacol Exp Ther. (2016) 359:452–9. doi: 10.1124/jpet.116.237057
52. Sommerfield AJ, Deary IJ, Frier BM. Acute hyperglycemia alters mood state and impairs cognitive performance in people with type 2 diabetes. Diabetes Care. (2004) 27:2335–40. doi: 10.2337/diacare.27.10.2335
53. Tiehuis AM, van der Graaf Y, Visseren FL, Vincken KL, Biessels GJ, Appelman APA, et al. Diabetes increases atrophy and vascular lesions on brain MRI in patients with symptomatic arterial disease. Stroke. (2008) 39:1600–3. doi: 10.1161/STROKEAHA.107.506089
54. Kagansky N, Levy S, Knobler H. The role of hyperglycemia in acute stroke. Arch Neurol. (2001) 58:1209–12. doi: 10.1001/archneur.58.8.1209
55. Boulé NG, Haddad E, Kenny GP, Wells GA, Sigal RJ. Effects of exercise on glycemic control and body mass in type 2 diabetes mellitus: a meta-analysis of controlled clinical trials. JAMA. (2001) 286:1218–27. doi: 10.1001/jama.286.10.1218
56. Nathan DM, Buse JB, Davidson MB, Ferrannini E, Holman RR, Sherwin R, et al. Medical management of hyperglycemia in type 2 diabetes: a consensus algorithm for the initiation and adjustment of therapy. Diabetes Care. (2009) 32:1. doi: 10.2337/dc08-9025
57. Colberg SR, Sigal RJ, Yardley JE, Riddell MC, Dunstan DW, Dempsey PC, et al. Physical activity/exercise and diabetes: a position statement of the American Diabetes Association. Diabetes Care. (2016) 39:2065–79. doi: 10.2337/dc16-1728
58. Johnson NA, Sachinwalla T, Walton DW, Smith K, Armstrong A, Thompson MW, et al. Aerobic exercise training reduces hepatic and visceral lipids in obese individuals without weight loss. Hepatology. (2009) 50:4. doi: 10.1002/hep.23129
59. Fisher G, Brown AW, Bohan Brown MM, Alcorn A, Noles C, Winwood L, et al. High intensity interval- vs moderate intensity- training for improving cardiometabolic health in overweight or obese males: a randomized controlled trial. PLoS ONE. (2015) 10:138853. doi: 10.1371/journal.pone.0138853
60. Weston M, Taylor KL, Batterham AM, Hopkins WG. Effects of low-volume high-intensity interval training (HIT) on fitness in adults: a meta-analysis of controlled and non-controlled trials. Sports Med. (2014) 44:1005–17. doi: 10.1007/s40279-014-0180-z
61. Laland KN, Odling-Smee J, Myles S. How culture shaped the human genome: Bringing genetics and the human sciences together. Nat Rev Genet. (2010) 11:137–48. doi: 10.1038/nrg2734
62. Wrangham RW, Jones JH, Laden G, Pilbeam D, Conklin-Brittain N. The raw and the stolen. Curr Anthropol. (1999) 40:5. doi: 10.1086/300083
63. Leonard WR, Snodgrass JJ, Robertson ML. Effects of brain evolution on human nutrition and metabolism. Annu Rev Nutr. (2007) 27:311–27. doi: 10.1146/annurev.nutr.27.061406.093659
64. James WPT, Johnson RJ, Speakman JR, Wallace DC, Frühbeck G, Iversen PO, et al. Nutrition and its role in human evolution. J Intern Med. (2019) 285:533–49. doi: 10.1111/joim.12878
65. Luca F, Perry GH, Di Rienzo A. Evolutionary adaptations to dietary changess. Annu Rev Nutr. (2010) 30:291–314. doi: 10.1146/annurev-nutr-080508-141048
66. Wrangham R. The evolution of human nutrition. Curr Bio. (2013) 23:354–5. doi: 10.1016/j.cub.2013.03.061
67. Burini RC, Leonard WR. The evolutionary roles of nutrition selection and dietary quality in the human brain size and encephalization. Nutrire. (2018) 43:19. doi: 10.1186/s41110-018-0078-x
68. Cordain L, Watkins BA, Mann NJ. Fatty acid composition and energy density of foods available to African hominids. evolutionary implications for human brain development. World Rev Nutr Diet. (2001) 90:144–61. doi: 10.1159/000059813
69. Nishizaki T, Nomura T, Matsuoka T, Enikolopov G, Sumikawa K. Arachidonic acid induces a long-lasting facilitation of hippocampal synaptic transmission by modulating PKC activity and nicotinic ACh receptors. Mol Brain Res. (1999) 69:263–72. doi: 10.1016/S0169-328X(99)00117-5
70. Rickman C, Davletov B. Arachidonic acid allows SNARE complex formation in the presence of munc18. Chem Biol. (2005) 12:545–53. doi: 10.1016/j.chembiol.2005.03.004
71. Anthonsen MW, Solhaug A, Johansen B. Functional coupling between secretory and cytosolic phospholipase a 2 modulates tumor necrosis factor-α- and interleukin-1β -induced nf-κb activation. J Biol Chem [Internet]. (2001) 276:30527–36. doi: 10.1074/jbc.M008481200
72. Song C, Manku MS, Horrobin DF. Long-chain polyunsaturated fatty acids modulate interleukin-1β-induced changes in behavior, monoaminergic neurotransmitters, and brain inflammation in rats. J Nutr. (2008) 138:954–63. doi: 10.1093/jn/138.5.954
73. Armelagos GJ. Brain evolution, the determinates of food choice, and the omnivore's dilemma. Crit Rev Food Sci Nutr. (2014) 54:10. doi: 10.1080/10408398.2011.635817
74. Kaku K, Kadowaki T, Seino Y, Okamoto T, Shirakawa M, Sato A, et al. Efficacy and safety of ipragliflozin in Japanese patients with type 2 diabetes and inadequate glycaemic control on sitagliptin. Diabetes Obes Metab. (2021) 23:2099–108. doi: 10.1111/dom.14448
75. Bennett G, Young E, Butler I, Coe S. The impact of lockdown during the covid-19 outbreak on dietary habits in various population groups: a scoping review. Front Nutr. (2021) 8:626432. doi: 10.3389/fnut.2021.626432
76. Laborde D, Herforth A, Headey D, de Pee S. COVID-19 pandemic leads to greater depth of unaffordability of healthy and nutrient-adequate diets in low- and middle-income countries. Nat Food. (2021) 2:7. doi: 10.1038/s43016-021-00323-8
77. Merino J, Joshi AD, Nguyen LH, Leeming ER, Mazidi M, Drew DA, et al. Diet quality and risk and severity of COVID-19: a prospective cohort study. Gut. (2021) 70:11. doi: 10.1136/gutjnl-2021-325353
78. Gaitán-Rossi P, Vilar-Compte M, Teruel G, Pérez-Escamilla R. Food insecurity measurement and prevalence estimates during the COVID-19 pandemic in a repeated cross-sectional survey in Mexico. Public Health Nutr. (2021) 24:3. doi: 10.1017/S1368980020004000
79. Headey D, Heidkamp R, Osendarp S, Ruel M, Scott N, Black R, et al. Impacts of COVID-19 on childhood malnutrition and nutrition-related mortality. Lancet. (2020) 396:513–82. doi: 10.1016/S0140-6736(20)31647-0
80. Jones AD, Ngure FM, Pelto G, Young SL. What are we assessing when we measure food security? a compendium and review of current metrics. Adv Nutr. (2013) 4:481–505. doi: 10.3945/an.113.004119
81. FAO, IFAD, UNICEF W. The State of Food Security and Nutrition in the World (2020) Transforming Food Systems for Affordable Healthy Diets. IEEE Journal of Selected Topics in Applied Earth Observations and Remote Sensing. (2020).
82. Jones AD. Food insecurity and mental health status: a global analysis of 149 countries. Am J Prev Med. (2017) 53:2. doi: 10.1016/j.amepre.2017.04.008
83. Witte AV, Kerti L, Hermannstädter HM, Fiebach JB, Schreiber SJ, Schuchardt JP, et al. Long-chain omega-3 fatty acids improve brain function and structure in older adults. Cereb Cortex. (2014) 24:11. doi: 10.1093/cercor/bht163
84. Nurk E, Drevon CA, Refsum H, Solvoll K, Vollset SE, Nygård O, et al. Cognitive performance among the elderly and dietary fish intake: the hordaland health study. Am J Clin Nutr. (2007) 86:5. doi: 10.1093/ajcn/86.5.1470
85. Bos DJ, van Montfort SJT, Oranje B, Durston S, Smeets PAM. Effects of omega-3 polyunsaturated fatty acids on human brain morphology and function: what is the evidence? Eur Neuropsychopharmacol. (2016) 26:3. doi: 10.1016/j.euroneuro.2015.12.031
86. Duthie GG, Gardner PT, Kyle JAM. Plant polyphenols: are they the new magic bullet? Proc Nutr Soc. (2003) 62:3. doi: 10.1079/PNS2003275
87. Sebastià N, Montoro A, León Z, Soriano JM. Searching trans-resveratrol in fruits and vegetables: a preliminary screening. J Food Sci Technol. (2017) 54:3. doi: 10.1007/s13197-016-2474-7
88. Köbe T, Witte AV, Schnelle A, Tesky VA, Pantel J, Schuchardt JP, et al. Impact of resveratrol on glucose control, hippocampal structure and connectivity, and memory performance in patients with mild cognitive impairment. Front Neurosci. (2017) 11:105. doi: 10.3389/fnins.2017.00105
89. Evans HM, Howe PRC, Wong RHX. Effects of resveratrol on cognitive performance, mood and cerebrovascular function in post-menopausal women; a 14-week randomised placebo-controlled intervention trial. Nutrients. (2017) 9:1. doi: 10.3390/nu9010027
90. Kennedy DO, Wightman EL, Reay JL, Lietz G, Okello EJ, Wilde A, et al. Effects of resveratrol on cerebral blood flow variables and cognitive performance in humans: a double-blind, placebo-controlled, crossover investigation. Am J Clin Nutr. (2010) 91:6. doi: 10.3945/ajcn.2009.28641
91. Veronica Witte A, Kerti L, Margulies DS, Flöel A. Effects of resveratrol on memory performance, hippocampal functional connectivity, and glucose metabolism in healthy older adults. J Neurosci. (2014) 34:23. doi: 10.1523/JNEUROSCI.0385-14.2014
92. Khare M, Mohanty C, Das BK, Jyoti A, Mukhopadhyay B, Mishra SP. Free radicals and antioxidant status in protein energy malnutrition. Int J Pediatr. (2014) 6:254396. doi: 10.1155/2014/254396
93. Verma A, Saini T, Meena K. Evaluation of oxidative stress in severe acute malnourished children at malnutrition treatment centre of Sardar Patel Medical College, Bikaner, Rajasthan, India. Int J Res Med Sci. (2016) 4:6. doi: 10.18203/2320-6012.ijrms20161796
94. Morais LH, Schreiber HL, Mazmanian SK. The gut microbiota–brain axis in behaviour and brain disorders. Nat Rev Microbiol. (2021) 19:241–55. doi: 10.1038/s41579-020-00460-0
95. Amon P, Sanderson I. What is the microbiome? Arch Dis Child Educ Pract Ed. (2017) 102:5. doi: 10.1136/archdischild-2016-311643
96. Galland L. The gut microbiome and the brain. J Med Food. (2014) 17:1261–72. doi: 10.1089/jmf.2014.7000
97. Manichanh C, Rigottier-Gois L, Bonnaud E, Gloux K, Pelletier E, Frangeul L, et al. Reduced diversity of faecal microbiota in Crohn's disease revealed by a metagenomic approach. Gut. (2006) 55:2. doi: 10.1136/gut.2005.073817
98. Riiser A. The human microbiome, asthma, and allergy. Allergy Asthma Clin Immunol. (2015) 11:35. doi: 10.1186/s13223-015-0102-0
99. Charbonneau MR, Blanton L V, Digiulio DB, Relman DA, Lebrilla CB, Mills DA, et al. A microbial perspective of human developmental biology. Nature. (2016) 535:48–55. doi: 10.1038/nature18845
100. Ding HT, Taur Y, Walkup JT. Gut microbiota and autism: key concepts and findings. J Autism Dev Disord. (2017) 47:480–9. doi: 10.1007/s10803-016-2960-9
101. Li S, Song J, Ke P, Kong L, Lei B, Zhou J, et al. The gut microbiome is associated with brain structure and function in schizophrenia. Sci Rep. (2021) 11:1. doi: 10.1038/s41598-021-89166-8
102. Hasan Mohajeri M, La Fata G, Steinert RE, Weber P. Relationship between the gut microbiome and brain function. Nutr Rev. (2018) 76:7. doi: 10.1093/nutrit/nuy009
103. Kennedy PJ, Cryan JF, Dinan TG, Clarke G. Kynurenine pathway metabolism and the microbiota-gut-brain axis. Neuropharmacology. (2017) 112:399–12. doi: 10.1016/j.neuropharm.2016.07.002
104. Kane L, Kinzel J. The effects of probiotics on mood and emotion. JAAPA. (2018) 3:1–3. doi: 10.1097/01.JAA.0000532122.07789.f0
105. Simpson CA, Diaz-Arteche C, Eliby D, Schwartz OS, Simmons JG, Cowan CSM. The gut microbiota in anxiety and depression – asystematic review. Clin Psychol Rev. (2021) 83:101943. doi: 10.1016/j.cpr.2020.101943
106. OECD. Real GDP Forecast (Indicator). Paris: Organisation for Economic Co-operation and Development (2022).
107. Kaushik P, Kaushik M, Parveen S, Tabassum H, Parvez S. Cross-talk between key players in patients with covid-19 and ischemic stroke: a review on neurobiological insight of the pandemic. Mol Neurobiol. (2020) 57:4921–8. doi: 10.1007/s12035-020-02072-4
108. Ganti L, Serrano E, Toklu HZ. Can neuron specific enolase be a diagnostic biomarker for neuronal injury in COVID-19? Cureus. (2020) 12:e11033. doi: 10.7759/cureus.11033
Keywords: COVID-19 pandemic, mental health, brain function, nutrition, brain
Citation: Rentería I, García-Suárez PC, Moncada-Jiménez J, Machado-Parra JP, Antunes BM, Lira FS and Jiménez-Maldonado A (2022) Unhealthy Dieting During the COVID-19 Pandemic: An Opinion Regarding the Harmful Effects on Brain Health. Front. Nutr. 9:876112. doi: 10.3389/fnut.2022.876112
Received: 15 February 2022; Accepted: 29 March 2022;
Published: 28 April 2022.
Edited by:
Andrew Scholey, Swinburne University of Technology, AustraliaReviewed by:
Michael W. Greene, Auburn University, United StatesCopyright © 2022 Rentería, García-Suárez, Moncada-Jiménez, Machado-Parra, Antunes, Lira and Jiménez-Maldonado. This is an open-access article distributed under the terms of the Creative Commons Attribution License (CC BY). The use, distribution or reproduction in other forums is permitted, provided the original author(s) and the copyright owner(s) are credited and that the original publication in this journal is cited, in accordance with accepted academic practice. No use, distribution or reproduction is permitted which does not comply with these terms.
*Correspondence: Alberto Jiménez-Maldonado, amltZW5lei5hbGJlcnRvODYmI3gwMDA0MDt1YWJjLmVkdS5teA==