- 1Department of Food Science, Shenyang Agricultural University, Shenyang, China
- 2Institute of Agricultural Facilities and Equipment, Jiangsu Academy of Agricultural Sciences, Nanjing, China
- 3Jiangsu Key Laboratory for Horticultural Crop Genetic Improvement, Nanjing, China
- 4College of Light Industry and Food Engineering, Nanjing Forestry University, Nanjing, China
- 5Nanjing Institute of Vegetable Science, Nanjing, China
The roles of methyl jasmonate (MeJA) and prohydrojasmon (PDJ) in postharvest color development and anthocyanins biosynthesis in the skin of peach fruit remain unclear. In this study, peach fruit were infiltrated with MeJA (200 μM) or PDJ (40 μM) and stored at 22°C for 7 days. The results showed that treatment with MeJA or PDJ had a positive effect on red color formation in peach fruits due to anthocyanins accumulation (∼120% increase). This was attributed to increased enzyme activities, and enhanced transcript abundance of the genes associated with anthocyanins biosynthesis, induced by MeJA or PDJ. Both MeJA and PDJ promoted sucrose biosynthesis, and the subsequently elevated levels of the sucrose during storage were positively correlated with anthocyanins accumulation (0.49) and the activities of key biosynthesis enzymes (0.42–0.79). Based on these findings, we proposed that MeJA or PDJ treatments promote anthocyanins biosynthesis by regulating sucrose metabolism during the postharvest storage of peach fruit.
Introduction
Peach (Prunus Persica L.) is one of the most popular fruit crops due to its attractive color, sweet taste and high nutritional content (1). Between 2015 and 2021, the global production of peach fruit exceeded 20 million tons. Preharvest bagging has become common practice in China and many other countries for the cultivation of commercial fruit such as peach. Some of the advantages of preharvest bagging include reduced pest and mechanical damage, low environmental contamination and maintenance of nutritional properties (2, 3). However, bagging can also block anthocyanins biosynthesis thereby inhibiting the desirable red coloration of peach skin. This is important as the external color of fruit can influence the purchasing behavior of consumers to a certain extent. Anthocyanins are also natural antioxidants which may be beneficial for human health (4). Hence, postharvest treatments that can induce anthocyanins biosynthesis are highly desirable.
Anthocyanins are synthesized by the phenylpropanoid pathway. This transforms phenylalanine into 4-coumaroyl-CoA which then enters the flavonoid pathway to give different classes of flavonoids, including the anthocyanins (5). The central carbon metabolism sugar-related pathway is also responsible for supplying the precursors and energy for the accumulation of anthocyanins. The increase in anthocyanins is believed to depend on sucrose signaling which upregulates key biosynthesis genes in genera such as Arabidopsis (6), Malus (7), and Prunus (8). The accumulation effect was also observed with exogenous sucrose treatments (9).
The biosynthesis and stability of anthocyanins is also determined by responses to various developmental and environmental stimuli (10). Plant growth regulators are likely to be one of the important factors (11). Methyl jasmonate (MeJA), the natural derivative of jasmonic acid (JA), is an important cellular regulator modulating various physiological process in plants including fruit development, ripening and secondary metabolite biosynthesis (12). Preharvest application of MeJA was reported to promote red coloration and anthocyanins accumulation in sweet cheery (13), strawberry (14), and pomegranate (15). Its postharvest application can also induce anthocyanins synthesis in some fruits such as pomegranate (15), blueberry (16), grape (17), apple (18), and raspberry (19). Prohydrojasmon (PDJ) is a synthetic analog of JA, developed for use as a stable, cost-effective and non-toxic plant growth regulator (20). Previous studies have demonstrated that the preharvest application of PDJ can also improve color development by inducing anthocyanins accumulation in the fruit from several species of mandarin orange (21), mango (22), apple (23), and peach (24). Several studies revealed that MeJA promotes anthocyanin accumulation by up-regulating associated gene expression in the flavonoid pathway (25, 26). However, for most species, the underlying mechanism of anthocyanins accumulation by MeJA/PDJ remains obscure.
A few studies of the preharvest application of jasmonates to peach have been reported. MeJA increased expression levels of genes associated with anthocyanins biosynthesis which promoted its accumulation during the early and late development stages (27). A similar result was reported for PDJ which was attributed the reduction of ethylene (28), an inhibitor of anthocyanins biosynthesis in peach (29). Very little information is available for the postharvest effects of MeJA and PDJ on anthocyanins accumulation and coloration in peach. However, both pre- and postharvest MeJA treatments were investigated for red raspberry. Preharvest applications significantly increased the all flavonoids, while postharvest applications enable a natural decline to be avoided (17), suggesting that the different effects induced by pre- and postharvest application of jasmonates might be observed for peach.
The aim of this study was to determine the effectiveness of MeJA and PDJ postharvest treatments on color development and anthocyanins accumulation in peach skin by measuring key enzyme activities and the expression patterns of related genes. The study also explored the relationship between anthocyanins biosynthesis and sucrose metabolism in response to each treatment during postharvest storage.
Materials and Methods
Biological Materials and Treatments
Preharvest bagged (double-layer fruit bags) peach fruit [Prunus persica (L.) Batsch cv. Baifeng] were picked at commercial maturity (approximately 100 d after full bloom) from a commercial orchard in Xinyi City, Jiangsu Province, China. The fruit were placed at 4 ± 2°C for 12 h (precooling). After screening, approximately 500 damage free fruits of uniform size and appearance were selected and randomly divided into three groups for the following treatments: (i) Immersion in a solution of MeJA (200 μM in 0.1% ethanol v/v) for 15 min; (ii) immersion in a solution of PDJ (40 μM in 0.1% ethanol v/v) for 15 min; (iii) immersion in ethanol solution (0.1% v/v) for 15 min (control). Jasmonate treatment concentrations were obtained from preliminary experiments to determine the effects of MeJA (0–800 μM) and PDJ (0–400 μM) solutions on the color formation of peach skin [Prunus persica (L.) Batsch cv. Chunmei]. After treatment, samples were sealed in polyethylene bags (0.2 mm thickness, 50 × 35 cm; 12 samples per bag), each containing four 2 cm diameter holes. All bags were stored at 22 ± 2°C and a light intensity of 300–400 lux, and three bags from each treatment were taken randomly for determination of the key fruit physiological indices after 0, 1, 3, 5, and 7 days of storage. Each determination comprised a minimum of three measurements. Post color evaluation, the peach skins (∼1 mm) from each treatment group were removed with a paring knife, combined, snap-frozen in liquid nitrogen, and stored at –80°C until required for further analysis.
Color Evaluation
Color was evaluated using the CEILAB system at four randomly distributed points on the circumference of each of eight fruits comprising the sample using a CR-400 colorimeter (Konica-Minolta, Tokyo, Japan). L* (lightness and darkness), a* (redness and greenness) and b* (yellowness and blueness) values were used to assess changes in color based on the color index of red grapes (CIRG) according to Eq. (1) (30):
Total Phenolics and Anthocyanins
Peeled tissues were reduced to a powder in liquid nitrogen and mixed thoroughly to obtain a homogeneous sample foreach treatment.
Total phenolic content (TPC) was measured by the Folin-Ciocalteu method (31). Briefly, extractions were performed in darkness for 30 min with ethanol (80% v/v) in an ultrasonic bath. The extracts were then mixed with Folin-Ciocalteu reagent (0.5 mL) and water (1.8 mL). After 5 min, sodium carbonate (15% in water w/v, 1.0 mL) was added, the solution was mixed and maintained in the dark for 1 h before recording the absorbance at 760 nm using a U-5100 UV-Visible spectrophotometer (Tianmei Scientific, Shanghai, China). Gallic acid was used as the calibration standard, and the TPC expressed as gallic acid equivalents (mg/g).
Anthocyanins were determined by the pH differential spectrum method (32) with slight modifications. Sample extracts were diluted in potassium chloride buffer (pH = 1.0) and sodium acetate buffer (pH = 4.5). The absorbance at 520 nm and 700 nm was then recorded at each pH and the differences calculated from Eq. (2):
The absorbance data was converted into cyanidin-3-O-glucoside (mg/kg) peel weight basis using the molar extinction coefficient [26,900 L/(cm⋅mol)]. Final results were expressed as cyanidin-3-O-glucoside equivalents (mg/kg) sample weight basis.
Measurement of Anthocyanins Biosynthesis Enzyme Activities
Phenylalanine ammonia lyase (PAL) activity was determined using the method by Manganaris et al. (33) with slight modifications. Crude enzyme (0.2 mL) and 1-phenylalanine (20 mM, 3 mL) were mixed and incubated at 37°C for 1 h. The reaction was terminated by the addition of hydrochloric acid (6 M, 0.2 mL) and the absorbance was measured at 290 nm. One unit of the enzyme activity was defined as the amount of protein (U/g) giving an increase in absorbance of 0.01 per h.
The activities of chalcone synthase (CHS), chalcone isomerase (CHI), flavanone-3-hydroxylase (F3H), dihydroflavonol 4-reductase (DFR), anthocyanidin synthase (ANS), and UDP-glucose-flavonoid glycosyltransferase (UFGT) were measured using the corresponding ELISA Detection Kits obtained from Jingmei Bio (Yancheng, China) according to the manufacturer’s instruction. The results were expressed as U/g protein.
Sugar (Sucrose, Glucose and Fructose) Contents
Finely powdered samples (0.25 g) were homogenized with ultrapure water (1 mL), centrifuged at 4°C (10,000 g for 20 min), and the supernatant was passed through a 0.45 μm filter membrane prior to analysis. Prepared samples (10 μL) were separated on an Hi-Plex Ca column (300 × 7.7 mm, 8 μm) maintained at 80°C (Agilent, United States) using deionized water at a flow rate 0.6 mL/min on a 1260 HPLC system fitted with a refractive index detector (Agilent, United States). The concentration of each sugar (g/kg) was quantified by the external standard method using corresponding standard solutions of each sugar.
Gene Expression Analysis
Total RNA was isolated with the FastPure®Plant Total RNA Isolation Kit (Vazyme Biotech, Nanjing, China). For each sample, DNA-free RNA (1,000 ng) was reverse-transcribed using the HiScript® III RT SuperMix for qPCR (+gDNA wiper) (Vazyme Biotech, Nanjing, China) according to the protocol supplied by the manufacturer. The expression of 10 genes (including structural genes and transcription factors; TFs) involved in anthocyanins synthesis (PAL, CHS, CHI, F3H, DFR, ANS, UFGT, MYB10, bHLH3, and WD40), as well as genes involved in sugar metabolism (SPS1/2, SS, NI, AI, and G6PDH), were monitored. Real-time quantitative reverse transcription PCR (qRT-PCR) was carried out with the ChamQ Universal SYBR qPCR Master Mix kit (Vazyme Biotech, Nanjing, China) and a CFX Connect™ Real-Time PCR Thermal Cycler (Bio-Rad, United States). Relative changes in gene expression were obtained by 2–ΔΔCt method (actin was used as internal control). The primer sequences are listed in Supplementary Table 1. Actin and TEF2 was used as the internal reference gene (8, 34).
Statistical Analysis
All data were analyzed and plotted using IBM SPSS Statistics for Windows, Version 26.0 (IBM Corp., Armonk, NY, United States) and Prism 8.4.2 (GraphPad, San Diego, United States). Experimental data obtained from each sample were reported as the mean ± SD. Differences between group means were assessed using one-way ANOVA: P-values < 0.05 were considered significant. Pearson’s correlation coefficient was used to identify significant correlations between key physiological indices (anthocyanins content, activities of key biosynthesis enzymes, sugar contents).
Results and Discussion
Effects of Postharvest Jasmonate Treatments on Color Formation and Anthocyanins Biosynthesis
Peach Skin Color Development
The visual images in Figure 1 show that the skin pigmentation of all groups became progressively red during storage. Compared with the control, skin color development in the MeJA/PDJ-treated peaches was significantly different. MeJA and PDJ accelerated the pigmentation process and at the end of storage, the treated fruits exhibited a deeper red color. Interestingly, color development in the PDJ-treated fruit followed that of the control at day three, while the extent of redness was similar to the MeJA group at the end of storage.
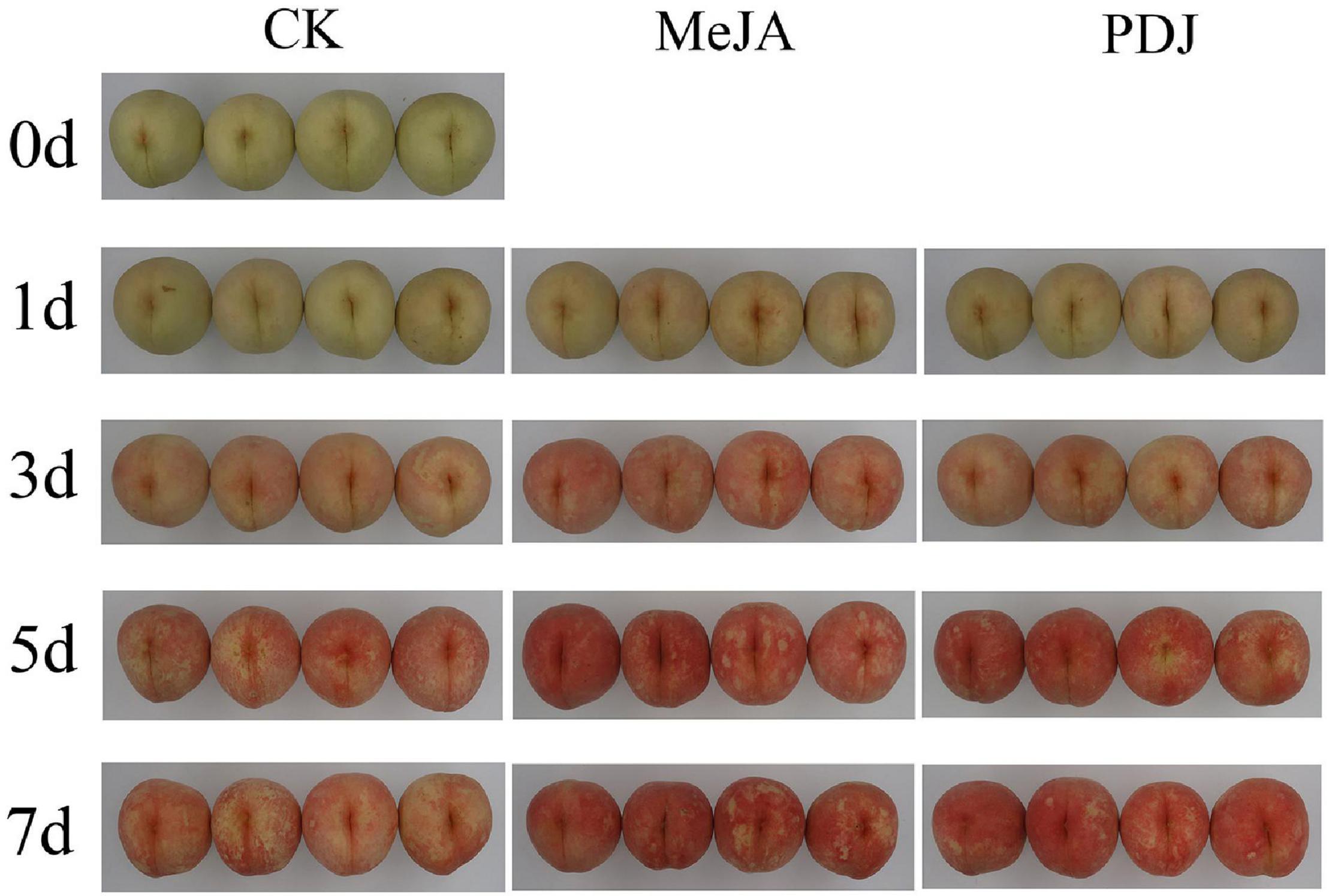
Figure 1. Effects of postharvest jasmonate treatments on visual color development in the skin of peach fruits during storage (22 ± 2°C; light intensity 300–400 lux).
In agreement with visual changes in color, significant variations in a*, b*, CIRG, and L* values were observed in all groups (Figure 2). Figure 2A shows that a* values (redness) for all groups increased with increasing storage time although the rate of increase was significantly greater in the treated fruits. At day three, the a* value of the MeJA-treated fruits was 1.25 time higher than that of the PDJ group, otherwise both groups followed a similar trend. These observations also agreed with the visual changes shown in Figure 1. CIRG values, which are considered to be a good indicators of anthocyanins content (30), exhibited a similar trend to the a* values (Figure 2B). Inspection of b* values (blue/yellow) revealed significant differences between the treated groups and the control during the late stages of storage, indicating a yellowish color in untreated fruits (Figure 2C). As shown in Figure 2D, L* values (perceptual lightness) decreased with increasing storage. Compared with the control, the rate of decrease was greater in the MeJA- and PDJ-treated peaches, which agreed with formation of the deep red color hue (reduced brightness).
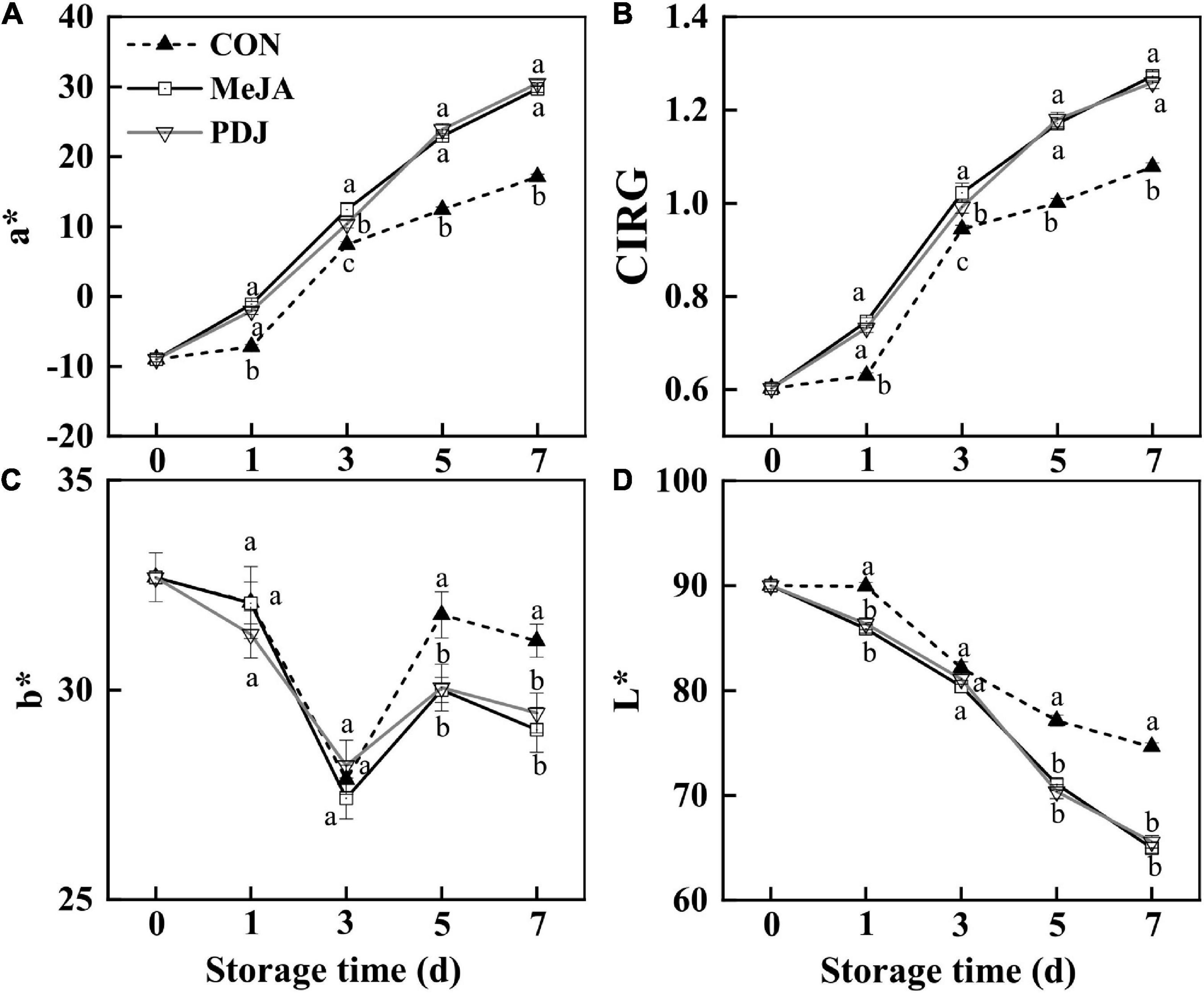
Figure 2. Effects of postharvest jasmonate treatments on a* (A), CIRG* (B), b* (C), and L* (D) values in the skin of peach fruits during storage (22 ± 2°C; light intensity 300–400 lux). Different lower-case letters indicate significant differences (P < 0.05) from three treatments at the same time point.
These findings were similar to previous studies using apple (18), raspberry (12), mango (16), and pear (24), i.e., the pigmentation process was accelerated by postharvest treatment of the fruits with MeJA or PDJ. A plausible reason for the color changes was stimulation of anthocyanins accumulation by the jasmonate treatments.
Total Phenolic Content and Anthocyanins
TPC in the skin of all peach samples increased over the first 5 days, decreasing slightly thereafter (Figure 3A). Compared with the control group, the TPC of samples from the jasmonate treatments had increased by a factor of ∼1.3 at the end of the storage. No significant differences were observed in TPC between samples from the MeJA and PDJ groups.
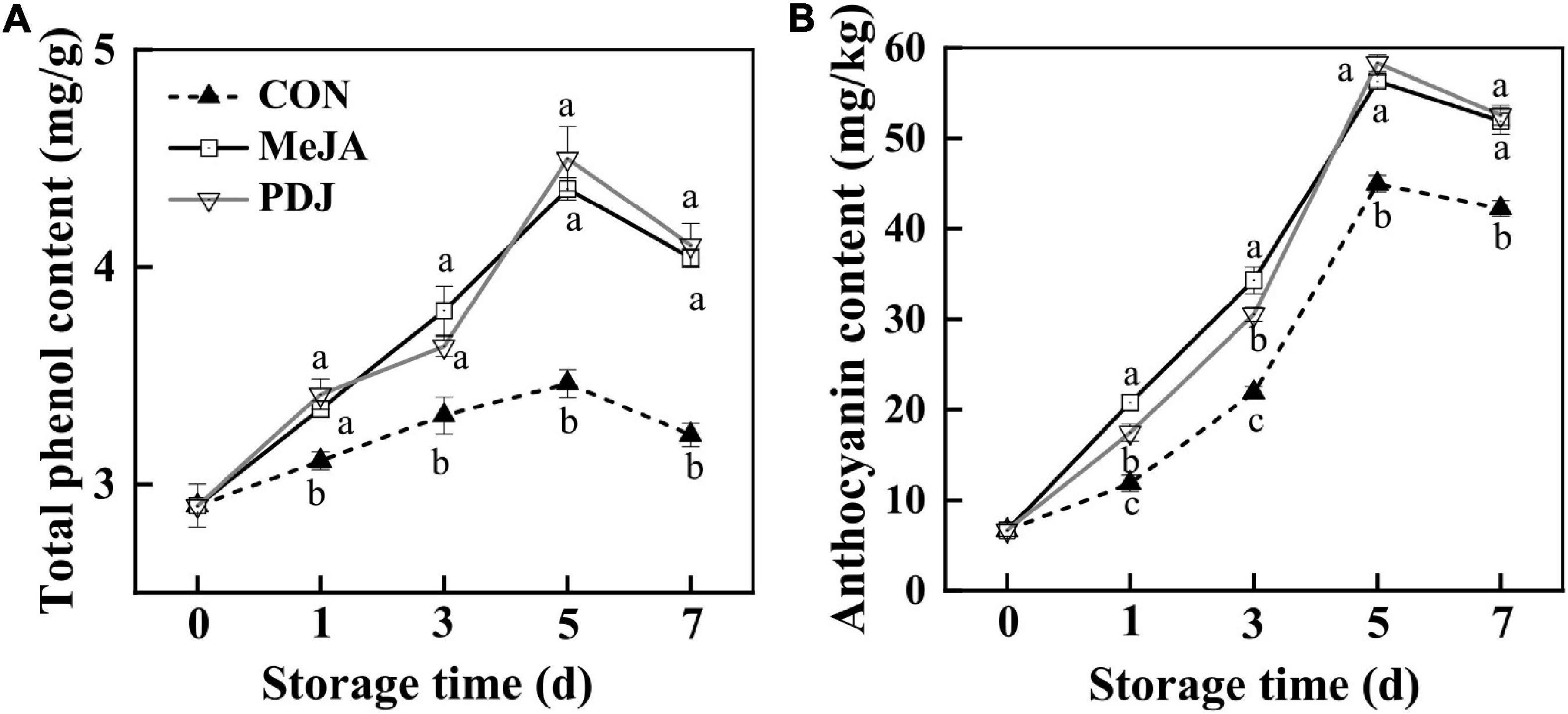
Figure 3. Effects of postharvest jasmonate treatments on TPC (A) and anthocyanins (B) in the skin of peach fruits during storage (22 ± 2°C; light intensity 300–400 lux). Different lower-case letters indicate significant differences (P < 0.05) from three treatments at the same time point.
The changes in total anthocyanins showed a similar trend. Although anthocyanins in the PDJ treated samples were lower than those of MeJA treated fruits at days one and three, they shared similar levels in the late stages of storage, and the overall increase (∼ 1.2 times) was similar to that of the TPC.
These results indicated that both jasmonate treatments could significantly induce the accumulation of TPC and anthocyanins, and this agreed with the reported increases in phenolics and anthocyanins in apple skin following postharvest treatment with MeJA (35). In another study, preharvest spraying of mango fruits with PDJ (0.2–0.4%) induced red blush and increased anthocyanins accumulation (by a factor of three), particularly in those fruits from outer side of tree canopy (22). Since jasmonates play an important role in response to plant stresses such as mechanical damage, the effects of MeJA might be explained by the subsequent changes in metabolism associated with defense and tissue repair (36). Hence, jasmonates stimulate the production and accumulation of secondary metabolites with high antioxidant activities such as terpenoids, phenolics, glucosinolates, and anthocyanins (37). However, the response to exogenous MeJA or its analogs might vary with species, cultivar, environmental conditions, cultural practices or treatment dose and timing (38). For example, MeJA was found to have a negative effect on total phenolics and total monomeric anthocyanins in sweet cherry fruits (13), while higher concentrations of MeJA repressed phenolics and anthocyanins accumulation in Thevetia peruviana and cotton (39, 40). Supplementary Figure 1 shows the results obtained from the preliminary experiments used to determine the optimum treatment concentrations of MeJA and PDJ in this study. At concentrations above the optimum values [(MeJA) = 200 μM] and [(PDJ) = 40 μM], a* values for the skin of treated peaches decreased significantly. This suggests that the mechanism involving negative feedback loops for JA signaling in Arabidopsis (41) may also act to reduce the accumulation of anthocyanins at higher concentrations of MeJA and PDJ in the postharvest treatment of peach fruit.
Activities of Anthocyanins Biosynthesis Enzymes
The significant increases in TPC and anthocyanins following the application of jasmonate suggested that these treatments could up-regulate the phenylpropanoid and anthocyanins pathways. In this instance, MeJA and PDJ may preferentially activate the key enzymes in these pathways (42). Hence the activities of enzymes associated with anthocyanins biosynthesis were investigated.
The activity of PAL, the upstream enzyme of the phenylpropanoid pathway, is shown in Figure 4 and Supplementary Table 2. Compared with the other enzymes, the level of PAL in untreated fruits was closer to the levels in the MeJA and PDJ groups, although, it always exhibited lower levels except for day three. This indicated that MeJA and PDJ might promote the conversion and usage of phenylalanine into phenolic compounds including anthocyanins. The results also agreed with the studies of the effects of MeJA treatments on strawberry, sweet potato, and sweet cherry (13, 43, 44).
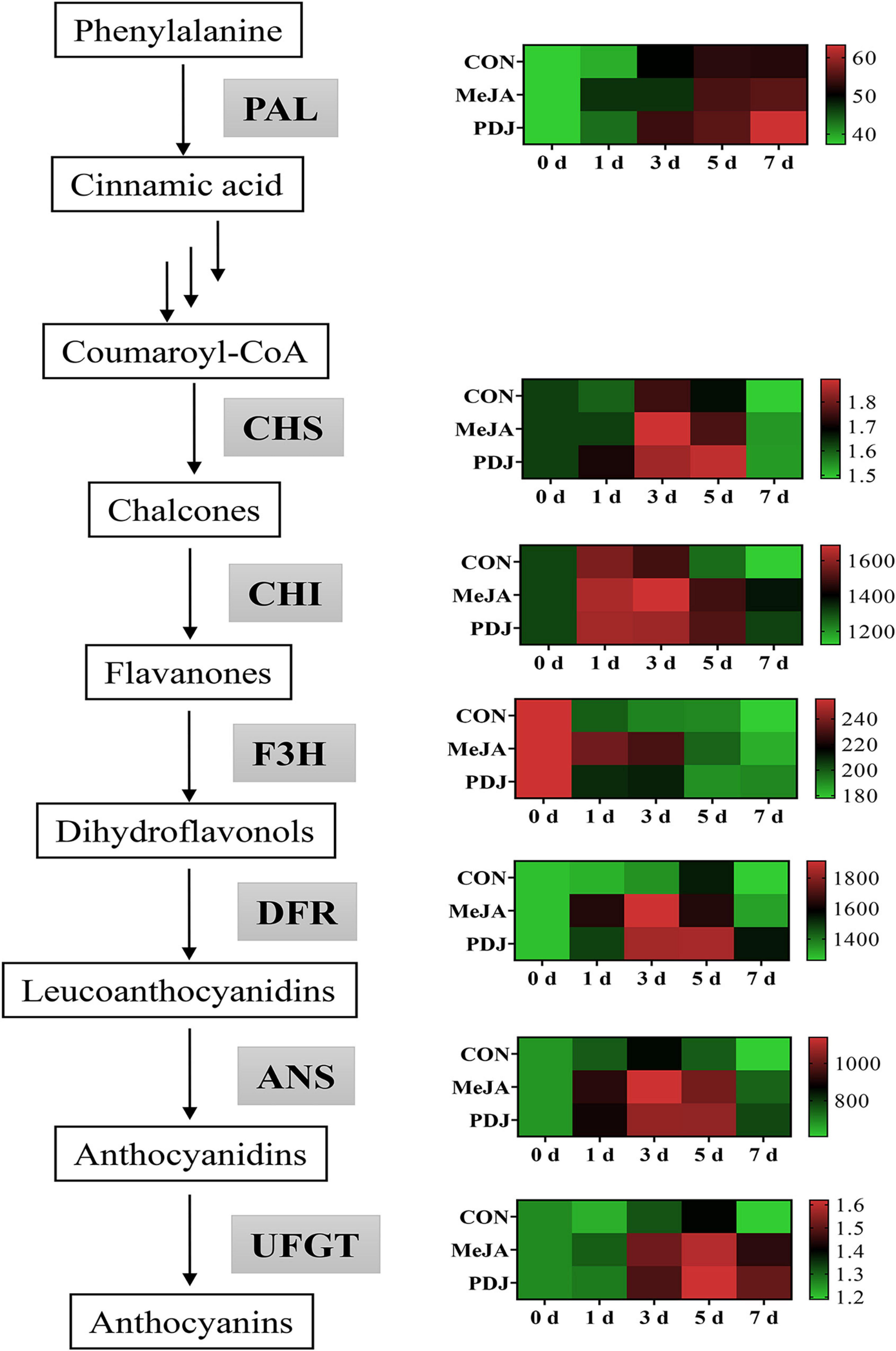
Figure 4. Heatmap showing the effects of postharvest jasmonate treatments on the of the activities of anthocyanins biosynthesis enzymes in peach skin during storage (22 ± 2°C; light intensity 300–400 lux). The color gradient scale to the right of each chart (green-black-red), represents low, middle, and high values of enzyme activity.
CHS, CHI, F3H are responsible for the transformation of coumaroyl-CoA into dihydroflavonols (45). MeJA and PDJ both displayed higher CHS activities than the control over the first 5 d, and there were no significant differences between either treatment. Untreated fruits showed the lowest CHI activity from day three onwards, ranging from 3.4 to 22.3% lower than the MeJA and PDJ groups. Like the results obtained for CHS activity, the MeJA and PDJ treatments had the same influence on CHI. However, MeJA treatment appeared to increase the activity of F3H more than PDJ. At day three, the activities of the control and PDJ-treated peaches were 38.17 and 18.58 U/g lower than the MeJA treated samples. In an investigation of the effects of the ethylene action inhibitor 1-methylcyclopropene (1-MCP) on anthocyanins biosynthesis, hot air and ultraviolet C treatments positively correlated with anthocyanins accumulation in peach (29). An increase in the catalytic activities of CHS, CHI and F3H were also observed, indicating that these enzymes were essential for provision of the dihydroflavonol precursors of anthocyanins formation.
DFR, ANS, and UFGT are the key enzymes associated with the synthesis and stability of anthocyanins in most plant species (46), and their activities in the peach samples exhibited similar trends. Enzyme activities were significantly higher in the MeJA and PDJ-treated fruits, especially for DFR and ANS, which showed maximum increases of ∼1.4 times between days three and five. Notably, the activity of DFR was the highest of all the enzymes, which suggested that it played a vital role in converting the dihydroflavonol precursors into the intermediates required for the anthocyanins pathway. Interestingly, DFR activity decreased in grape berry cell cultures when MeJA was used to stimulated anthocyanins production (47). This implied differences in the anthocyanins promotion mechanism by MeJA, necessitating further investigation. Both jasmonate treatments gave similar increases in UFGT activity ranging from 4.7 to 26.9% during storage. Positive responses in UFGT activity were also observed in MeJA-treated grape berry cell cultures (47). These results demonstrated that MeJA and PDJ both had a positive effect on most enzymes in the anthocyanins pathway, which agreed with the measured increases in anthocyanins of the treated fruits (Figure 3B).
Expression of Genes Associated With Anthocyanins Biosynthesis
To gain insight into the MeJA and PDJ elicited red color development and anthocyanins accumulation in peach skin, the relative expressions of genes associated with anthocyanins biosynthesis were investigated.
As shown in Figure 5, the relative expressions of most structural genes associated with anthocyanins biosynthesis differed from the control in jasmonate-treated peaches. Expression of the PAL gene was significantly enhanced by MeJA or PDJ, especially during early storage (Figure 5A). The genes encoding the enzymes responsible for converting coumaroyl-CoA into flavanones (CHS, CHI and F3H) were affected by MeJA and PDJ to different extents (Figures 5B–D). Gene encoding CHS was upregulated by MeJA during the first 3 days, while gene expressing CHI was stimulated by both treatments before day three. Compared with the control fruit, the expression levels of F3H gene was upregulated in MeJA-treated fruit during storage, and an obvious upregulation was observed on days one and five. Exogenous jasmonates treatment significantly upregulated the transcript levels of DFR and ANS during 1–3 d (Figures 5E,F). With the exception of MeJA treatments at day five, expression of UFGT gene was significantly induced by both jasmonates (Figure 5G), there were no significant differences between treatments at most time points, and the trends were similar to the observed catalytic activities. The upregulation of phenylpropanoid-related genes in PDJ-treated lettuce after 48 h was reported (48). Highly significant correlations were also found among flavonoids, anthocyanins, PAL and genes expression of ANS, DFR, and UFGT, which were involved in the metabolism of anthocyanin, quercitrin, and epicatechin (49).
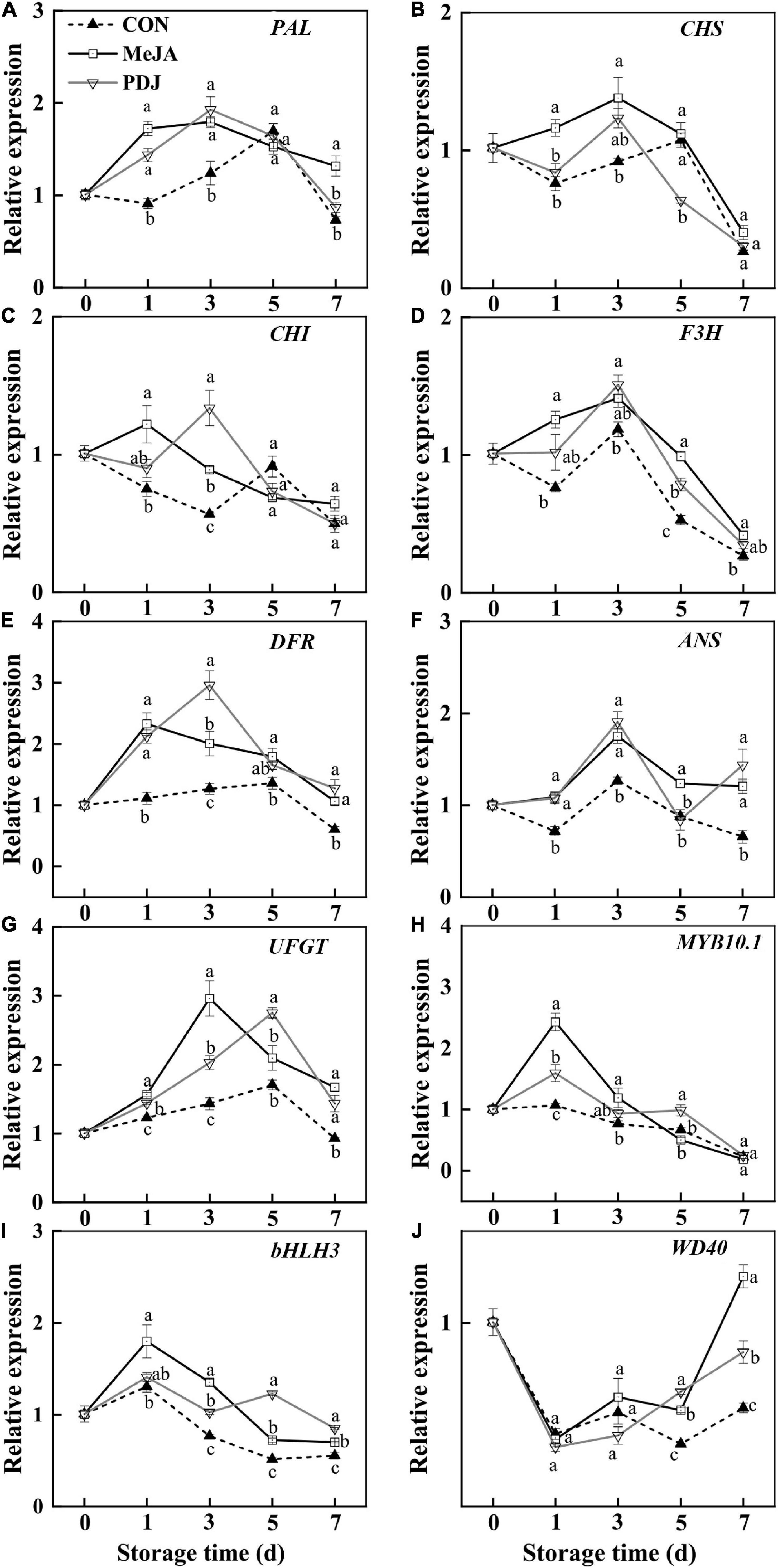
Figure 5. Effects of postharvest jasmonate treatments on the expressions of genes associated with anthocyanins biosynthesis during storage (22 ± 2°C; light intensity 300–400 lux; different lower-case letters indicate significant differences (P < 0.05) from three treatments at the same time point). (A–J) PAL, CHS, CHI, F3H, DFR, ANS, UFGT, MYB10.1, bHLH3, WD40.
In addition to the structural genes, various TFs, including MYB, bHLH, and WD40, also participate in anthocyanins biosynthesis (50). TFs bind to specific DNA sequences to regulate the transcription of genetic information, such as the expression of structural genes in the anthocyanins pathway. In peach, the MBW complex (PpMYB10.1, bHLH3, and WD40) was reported to activate anthocyanins biosynthesis (51, 52). Here, the expression of MYB10.1 and bHLH3 in the MeJA and PDJ-treated fruits alternated to present maximum values at most time points, similar to the expression patterns of the structural genes (Figures 5H–J). On the other hand, the stimulated expression of WD40 by MeJA and PDJ was not observed until day five, reaching a maximum value at the end of storage. A similar effect was observed in 1-MCP treated peaches (29), suggesting that both jasmonates upregulate the structural genes of anthocyanins biosynthesis by promoting expression of the upstream MBW complex.
Differential Effects of Methyl Jasmonate and Prohydrojasmon
Although the effects of MeJA and PDJ on gene expression appeared to mirror enzyme activities, anthocyanins accumulation, and hence red color development, there were small differences between the effects of the two jasmonates. Compared with MeJA, PDJ showed delayed red color development in peach skin, and the peak values of various indicators including a* and CIRG, anthocyanins content, enzyme activities and gene expression always occurred at later time points. Otherwise, the influence of PDJ on some indicators was weaker than MeJA. This might be due to different biochemical transformations for PDJ and MeJA or the complex transcriptional framework resulting from the overlapping defense responses of fruits (53). Although the PDJ treatment group elicited delayed effects, the final indices of anthocyanins accumulation induced by both treatments were comparable at the end of storage. Notably, the working concentration of PDJ was one fifth that of MeJA, and this stronger biological activity might be due to its greater chemical stability (28). Elsewhere, the application of PDJ at concentrations of one half that of MeJA gave equivalent results for improvements in fruit setting, growth, ripening and cold-resistance (53). However, information involving PDJ metabolism in plants is scarce, and little is known about the mechanism influencing plant physiology which appears to differ from MeJA. Hence, further studies are required to provide the scientific basis necessary to develop the more cost-effective synthetic JA.
Anthocyanins Biosynthesis and Sugar Metabolism
Plants are autotrophs, and the sugars generated via photosynthesis serve as energy sources, carbon skeletons, as well as signal molecules (54). During fruit development, the production of anthocyanins is always accompanied by sugar accumulation. Based on this, studies have shown that exogenous sugar treatment can activate anthocyanins biosynthesis by upregulating the expression of structural genes in Arabidopsis (55), mango (56), strawberry (57), grape (58), and other species (59). The amount of endogenous sugar was also positively associated with anthocyanins accumulation (8, 27). Here, the contents of sucrose, glucose and fructose, as well as the expression of genes involved in sugar metabolism, were measured in postharvest jasmonate treated peach (Figure 6).
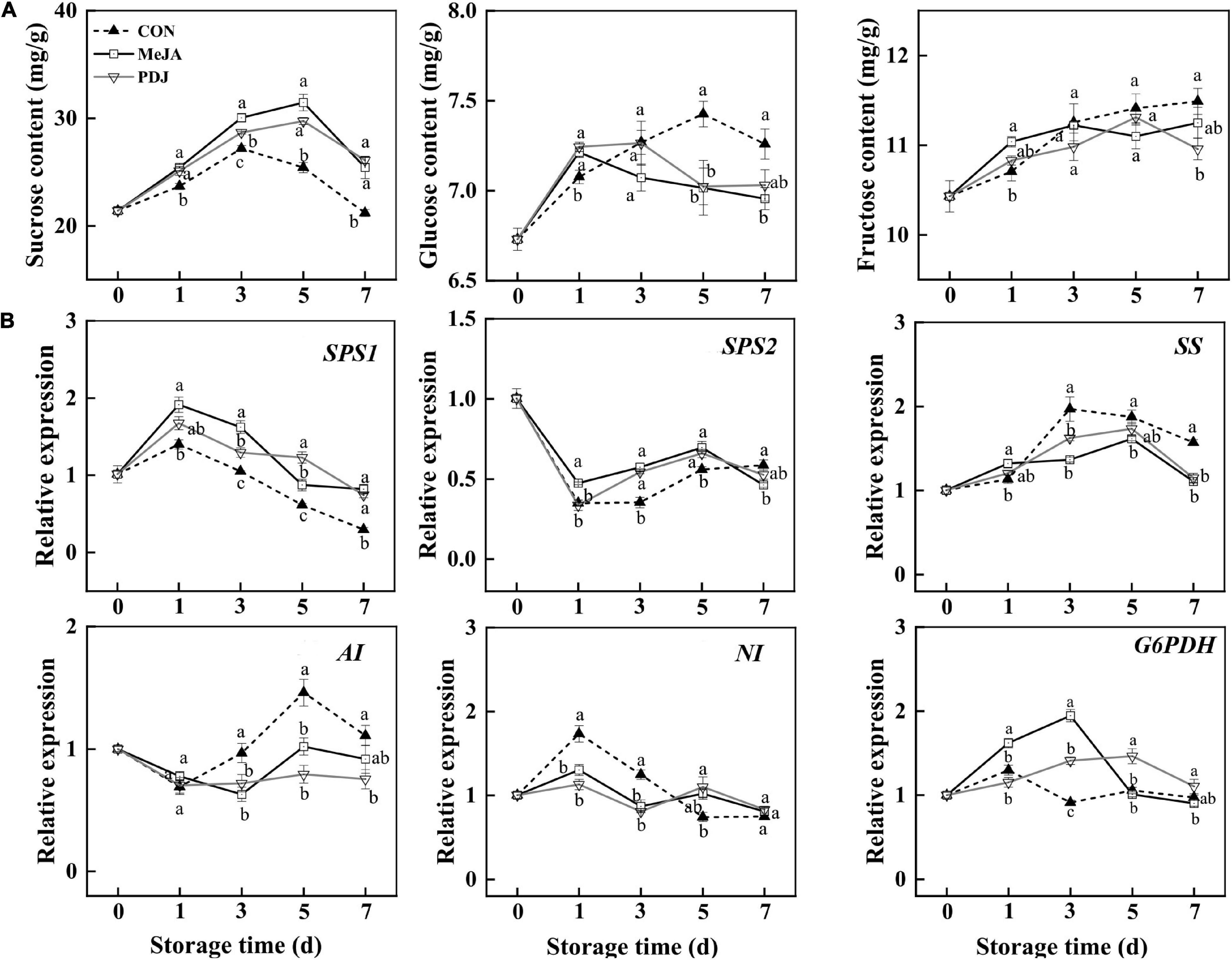
Figure 6. Effects of postharvest jasmonate treatments on sugar contents and the expressions of associated genes during storage (22 ± 2°C; light intensity 300–400 lux; different lower-case letters indicate significant differences (P < 0.05) from three treatments at the same time point): (A) Sucrose, glucose and fructose contents; (B) expressions of genes in the sucrose metabolism pathway.
Changes in sucrose contents followed a similar trend in all three groups, initially increasing and then decreasing at the end of storage. The sucrose contents of both treated groups were similar and significantly higher than the control throughout storage. On day one of the treatments, MeJA promoted both glucose and fructose while PDJ promoted glucose only. From day three onwards, the amounts of both glucose and fructose were higher in the control. Overall, the results indicated that both jasmonate treatments could elicit glucose and fructose production in the early stages of storage, and sucrose production throughout. Exogenous methyl jasmonate treatment resulted in a significantly higher sucrose content and lower glucose and fructose contents, which were also found in tomato during postharvest ripening (60). Similar response patterns were reported for postharvest MeJA treated peach fruit during cold storage (34). Pre-harvest application of MJ increased sucrose, glucose and fructose concentrations in mango during storage (61). A recent study into the effects of growth regulators on potato growth and tuberization indicated that PDJ treatment similarly increased sucrose content (62). Hence, these findings suggested that sucrose metabolism is a complicated process, which may be an important component of the response to jasmonate treatment in multiple plant species including peach.
Figure 6B shows the expression of genes associated with sugar metabolism in the peach samples subjected to the different treatments. Relative to the control, MeJA and PDJ significantly enhanced the expression levels of SPS1 and SPS2 at most of time points during storage, although their trends were slightly different and agreed with the higher sucrose contents of the treated fruits. Similar observations were reported for MeJA treated peach (34) and tomato (60). The expression of SS in all three groups showed similar increasing trends, followed by a decline toward the end of storage. From day three onwards, the control fruits displayed higher SS expression levels than the treatment groups. The downregulation of SS in peach was similar to that in tomato (60), strawberry (63), and peach fruit treated with MeJA (34); however, the reasons for the downregulation of SS need to be investigated. Similarly, MeJA and PDJ inhibited the expression of AI from day three onward, and no significant differences were observed between the two groups. The expression of NI followed a different pattern as it was suppressed by both jasmonate treatments before the day three. G6PDH is the key regulatory enzyme in the pentose phosphate pathway which works in parallel with glycolysis (64). Here, MeJA upregulated G6PDH immediately after treatment to reach a maximum at day three, while PDJ increased expression more slowly, peaking at day five. A similar phenomenon was observed in peach fruit, of which the anthocyanin content significantly increased subjected to UV and hot air treatments (8). Overall, the observed expression of genes responsible for sugar metabolism broadly followed the contents of sucrose, glucose and fructose in the postharvest jasmonate treated peaches.
Correlation Analysis
Since the jasmonate treatments elicited anthocyanins accumulation and expression of their related enzymes, we investigated the statistical relationships between these factors, and their association with sugar contents. Figure 7 shows the Pearson’s correlation coefficients for the associations between anthocyanins, its key biosynthesis enzyme activities, and sugar contents in peach skin exposed to MeJA and PDJ.
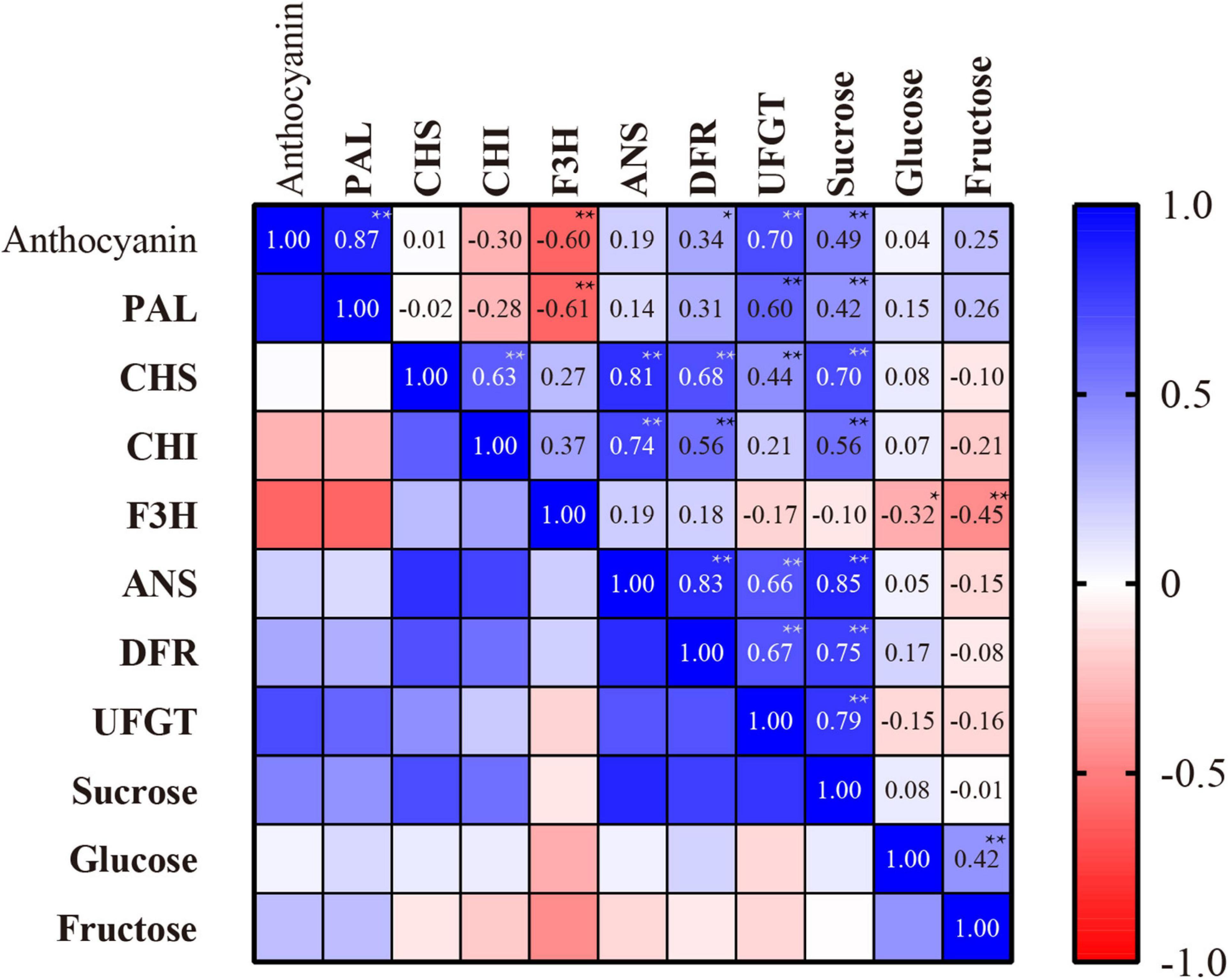
Figure 7. Pearson’s correlation coefficients for the associations between anthocyanins content, anthocyanins biosynthesis enzyme activities, and sugar contents in peach skin exposed to MeJA and PDJ. *Significant at P < 0.05; **Significant at P < 0.01.
The key enzymes PAL, DFR, and UFGT showed strong positive associations with anthocyanins content (0.34–0.87), further confirming the key roles of these enzymes in peach anthocyanins biosynthesis in response to the jasmonate treatments.
Sucrose content showed a significant association with anthocyanins content (0.49) as well as the activities of most biosynthesis enzymes including PAL, DFR, and UFGT (0.42–0.79). Glucose and fructose were also found to stimulate the expression of anthocyanins biosynthesis genes in Arabidopsis (55), radish (52), and grape (47). However, no significant correlations were observed between glucose, fructose and anthocyanins content in this research. It is known that sucrose can act as a signaling molecule to upregulate a diverse range of biosynthesis genes, and its promotion of anthocyanins biosynthesis in peach was recently confirmed using in vitro experiments (8). This was subsequently confirmed in vivo in our lab by the application of exogenous sucrose (200 mM) to peach fruits, leading to significant increases in endogenous sucrose and hence increases in anthocyanins contents of up to 1.85 times in the skin tissues (27). In this study, MeJA and PDJ promoted the biosynthesis of sucrose, leading to significantly higher levels during storage. These findings implicate sucrose as a signaling molecule for anthocyanins biosynthesis by activating key enzymes in the jasmonate-treated peaches. Based on this, we proposed that the promotion of sucrose biosynthesis by MeJA and PDJ during storage increased anthocyanins accumulation, which agreed with the results obtained for peach exposed to postharvest hot air and UV-C treatments (8, 27).
Conclusion
This study has demonstrated that postharvest applications of MeJA or PDJ could improve the appearance of peaches by inducing red color development in the skin. The red color was attributed to anthocyanins accumulation, which correlated with the increased activities of PAL, DFR, and UFGT, while expression of the related structural genes and TFs was also upregulated. Both jasmonate treatments ptomoted the biosynthesis of sucrose. The resulting increased concentrations of sucrose during storage were positively correlated with anthocyanins accumulation and the activities of the related enzymes PAL, DFR, and UFGT. Hence, we proposed that MeJA or PDJ treatments could promote anthocyanins biosynthesis by increasing amounts of the signal molecule sucrose during postharvest storage. Notably, PDJ exhibited similar activity to MeJA at one fifth of its concentration, although there was an initial delayed effect, and further studies are required to understand these differences. The results presented here demonstrate that the application of these two hormones, especially PDJ could provide a simple strategy to improve the commercial value of peach.
Data Availability Statement
The raw data supporting the conclusions of this article will be made available by the authors, without undue reservation.
Author Contributions
TT: lab. experiment and writing. HZ: conceptualization, design, and data curation. LW, JL, and WH: methodology. JZ and LM: lab. experiment. GL: project administration. PL: funding acquisition and supervision. YZ: investigation, reviewing, and editing. All authors contributed to the article and approved the submitted version.
Funding
This work was funded by the National Natural Science Foundation of China (Grant No. 31901746), the Natural Science Foundation of Jiangsu Province (Grant No. BK20190265), the Earmarked Fund for Jiangsu Agriculture Industry Technology System [Grant No. JATS(2021)446], the Earmarked Fund for China Agriculture Research System (Grant No. CARS-30-5-03), and Key Research and Development Program of Kunshan (Grant No. KN202104).
Conflict of Interest
The authors declare that the research was conducted in the absence of any commercial or financial relationships that could be construed as a potential conflict of interest.
Publisher’s Note
All claims expressed in this article are solely those of the authors and do not necessarily represent those of their affiliated organizations, or those of the publisher, the editors and the reviewers. Any product that may be evaluated in this article, or claim that may be made by its manufacturer, is not guaranteed or endorsed by the publisher.
Acknowledgments
We are grateful to Jialei Wang for their technical assistance with HPLC analysis.
Supplementary Material
The Supplementary Material for this article can be found online at: https://www.frontiersin.org/articles/10.3389/fnut.2022.871467/full#supplementary-material
References
1. Zhou Y, Zhou H, Wang KL, Vimolmangkang S, Espley RV, Wang L, et al. Transcriptome analysis and transient transformation suggest an ancient duplicated MYB transcription factor as a candidate gene for leaf red coloration in peach. BMC Plant Biol. (2014) 14:388. doi: 10.1186/s12870-014-0388-y
2. Zhu M, Fang WC, Chen CW, Wang LR, Cao K. Effects of shading by bagging on carotenoid accumulation in peach fruit flesh. J Plant Growth Regul. (2020) 40:1912–21. doi: 10.1007/s00344-020-10227-9
3. Li Q, Cheng CX, Zhang XF, Wang CH, Yang SL. Preharvest bagging and postharvest calcium treatment affects superficial scald incidence and calcium nutrition during storage of ‘Chili’ pear (Pyrus bretschneideri) fruit. Postharvest Biol Technol. (2020) 163:111149. doi: 10.1016/j.postharvbio.2020.111149
4. Wang YC, Wang N, Xu HF, Jiang SH, Fang HC, Su MY, et al. Auxin regulates anthocyanin biosynthesis through the Aux/IAA-ARF signaling pathway in apple. Hortic Res. (2018) 1:5–59. doi: 10.1038/s41438-018-0068-4
5. Duan YJ, Zhang LG, Qiong HE, Zhang MK, Jiang-Chao AS. Expression of transcriptional factors and structural genes of anthocyanin biosynthesis in purple-heading Chinese Cabbage. Acta Hortic Sin. (2012) 39:2159–67. doi: 10.16420/j.issn.0513-353x.2012.11.012
6. Solfanelli C, Poggi A, Loreti E, Alpi A, Perata P. Sucrose-specific induction of the anthocyanin biosynthetic pathway in Arabidopsis. Plant Physiol. (2006) 140:637–46. doi: 10.1104/pp.105.072579
7. Hu D, Sun CH, Zhang QY, An JP, Hao YJ. Glucose sensor MdHXK1 phosphorylates and stabilizes MdbHLH3 to promote anthocyanin biosynthesis in apple. PLoS Genet. (2016) 12:e1006273. doi: 10.1371/journal.pgen.1006273
8. Zhou DD, Li R, Zhang H, Chen SX, Tu K. Hot air and UV-C treatments promote anthocyanin accumulation in peach fruit through their regulations of sugars and organic acids. Food Chem. (2020) 309:125726. doi: 10.1016/j.foodchem.2019.125726
9. Dong L, Zhang X, Xu Y, Aghdam MS, Luo ZS. Effect of exogenous sucrose on anthocyanin synthesis in postharvest strawberry fruit. Food Chem. (2019) 289:112–20.
10. Nathalie K, Le G, Wu DZ, Ben-Hong W, Virgine L, Eric G, et al. Berry ripening: recently heard through the grapevine. J Exp Bot. (2014) 65:4543–59. doi: 10.1093/jxb/ert395
11. Siatka T. Effects of growth regulators on production of anthocyanins in callus cultures of Angelica archangelica. Nat Prod Commun. (2019) 14:1–4. doi: 10.1177/1934578X19857344
12. Cheong JJ, Choi YD. Methyl jasmonate as a vital substance in plants. Trends Genet. (2003) 19:409–13. doi: 10.1016/S0168-9525(03)00138-0
13. Saracoglu O, Ozturk B, Yildiz K, Kucuker E. Pre-harvest methyl jasmonate treatments delayed ripening and improved quality of sweet cherry fruits. Sci Hortic. (2017) 226:19–23. doi: 10.1016/j.scienta.2017.08.024
14. Saba MK, Zarei L. Preharvest methyl jasmonate’s impact on postharvest chilling sensitivity, antioxidant activity, and pomegranate fruit quality. J Food Biochem. (2019) 43:e12763. doi: 10.1111/jfbc.12763
15. Sayyari M, Babalar M, Kalantari S, Martínez-Romero D, Guillén F, Serrano M, et al. Vapour treatments with methyl salicylate or methyl jasmonate alleviated chilling injury and enhanced antioxidant potential during postharvest storage of pomegranates. Food Chem. (2011) 124:964–9. doi: 10.1016/j.foodchem.2010.07.036
16. Wang HB, Wu Y, Yu RP, Wu CE, Fan GJ, Li TT. Effects of postharvest application of methyl jasmonate on physicochemical characteristics and antioxidant system of the blueberry fruit. Sci Hortic. (2019) 258:108785. doi: 10.1016/j.scienta.2019.108785
17. Flores G, Del Castillo MLRP. Influence of preharvest and postharvest methyl jamonate treatments on flavonoid content and metabolomic enzymes in red raspberry. Postharvest Biol Technol. (2014) 97:77–82. doi: 10.1016/j.postharvbio.2014.06.009
18. Rudell DR, Mattheis JP, Fan X, Fellman JK. Methyl jasmonate enhances anthocyanin accumulation and modifies production of phenolics and pigments in ‘Fuji’ apples. J Am Soc Hortic Sci. (2002) 127:435–441. doi: 10.1103/PhysRevLett.84.2792
19. Chanjirakul K, Wang SY, Wang CY, Siriphanich J. Effect of natural volatile compounds on antioxidant capacity and antioxidant enzymes in raspberries. Postharvest Biol Technol. (2006) 40:106–15. doi: 10.1016/j.postharvbio.2006.01.004
20. Uefune M, Ozawa R, Takabayashi J. Prohydrojasmon treatment of lima bean plants reduces the performance of two-spotted spider mites and induces volatiles. J Plant Interact. (2014) 9:69–73. doi: 10.1080/17429145.2012.763146
21. Sato K, Ikoma Y. Improvement in handpicking efficiency of satsuma mandarin fruit with combination treatments of gibberellin, prohydrojasmon and ethephon. Hortic J. (2016) 86:283–90. doi: 10.2503/hortj.OKD-003
22. Sudheeran PK, Love C, Feygenberg O, Maurer D, Ovadia R, Oren-Shamir M, et al. Induction of red skin and improvement of fruit quality in ‘Kent’, ‘Shelly’ and ‘Maya’ mangoes by preharvest spraying of prohydrojasmon at the orchard. Postharvest Biol Technol. (2019) 149:18–26. doi: 10.1016/j.postharvbio.2018.11.014
23. Atay E. Effect of application time of prohydrojasmon on fruit coloration of ‘Gala’ and ‘Braeburn’ apples. Bulgarian J Agric Sci. (2015) 21:1242–7.
24. Wang XQ, Cao XY, Shang Y, Bu HD, Wang TY, Lyu DG, et al. Preharvest application of prohydrojasmon affects color development, phenolic metabolism, and pigment-related gene expression in red pear (Pyrus ussuriensis). J Sci Food Agric. (2020) 100:4766–75. doi: 10.1002/jsfa.10535
25. Wang YC, Liu WJ, Jiang HY, Mao ZL, Wang N, Jiang SH, et al. The R2R3-MYB transcription factor MdMYB24-like is involved in methyl jasmonate-induced anthocyanin biosynthesis in apple. Plant Physiol Biochem. (2019) 139:273–82. doi: 10.1016/j.plaphy.2019.03.031
26. Park WT, Kim YB, Seo JM, Kim SJ, Chung E, Lee JH, et al. Accumulation of anthocyanin and associated gene expression in radish sprouts exposed to light and methyl jasmonate. J Agric Food Chem. (2013) 61:4127–32. doi: 10.1021/jf400164g
27. Tian MY, Zhou HS, Tang TT, Zhang YT, Ling J, Luo SF. Effects of exogenous sucrose treatment on the color formation of postharvest peach peel. Food Sci. (2022) 43:177–83. doi: 10.7506/spkx1002-6630-20201112-135
28. Ziosi V, Bregoli AM, Fregola F, Costa G, Torrigiani P. Jasmonate-induced ripening delay is associated with up-regulation of polyamine levels in peach fruit. J Plant Physiol. (2009) 166:938–46. doi: 10.1016/j.jplph.2008.11.014
29. Zhang YT, Ling J, Zhou HS, Tian MY, Huang W, Luo SF, et al. 1-Methylcyclopropene counteracts ethylene inhibition of anthocyanin accumulation in peach skin after harvest. Postharvest Biol Technol. (2022) 183:111737. doi: 10.1016/j.postharvbio.2021.111737
30. Vergara AE, Diaz K, Carvajal R, Espinoza L, Alcalde JA, Perez-Donoso AG. Exogenous applications of brassinosteroids improve color of red table grape (Vitis vinifera L. Cv. “Redglobe”) berries. Front Plant Sci. (2018) 9:363. doi: 10.3389/fpls.2018.00363
31. Nemenyi A, Stefanovitsne-Banyai E, Pek Z, Hegedus A, Gyuricza C, Barocsi Z, et al. Total antioxidant capacity and total phenolics content of phyllostachys taxa shoots. Not Bot Horti Agrobot Cluj Napoca. (2015) 43:64–9. doi: 10.15835/nbha4319586
32. Cheng GW, Breen PJ. Activity of phenylalanine ammonialyase (PAL) and concentrations of anthocyanins and phenolics in developing strawberry fruit. J Am Soc Hortic Sci. (1991) 116:865–9. doi: 10.21273/JASHS.116.5.865
33. Manganaris GA, Vicente AR, Crisosto CH, Labavitch JM. Effect of dips in a 1-methylcyclopropene-generating solution on ‘Harrow Sun’ plums stored under different temperature regimes. J Agric Food Chem. (2007) 55:7015–20. doi: 10.1021/jf071065p
34. Yu LN, Liu HX, Shao XF, Yu F, Wei YZ, Ni ZM, et al. Effects of hot air and methyl jasmonate treatment on the metabolism of soluble sugars in peach fruit during cold storage. Postharvest Biol Technol. (2016) 113:8–16. doi: 10.1016/j.postharvbio.2015.10.013
35. Shafiq M, Singh Z, Khan AS. Time of methyl jasmonate application influences the development of ‘Cripps Pink’ apple fruit colour. J Sci Food Agric. (2013) 93:611–8. doi: 10.1002/jsfa.5851
36. Azis HR, Etteieb S, Takahashi S, Koshiyama M, Fujisawa H, Isoda H. Effect of prohydrojasmon on total phenolic content, anthocyanin accumulation and antioxidant activity in komatsuna and lettuce. Biosci Biotechnol Biochem. (2020) 84:178–86. doi: 10.1080/09168451.2019.1673146
37. Shabani L, Ehsanpour AA, Asghari G, Emami J. Glycyrrhizin production by in vitro cultured Glycyrrhiza glabra elicited by methyl jasmonate and salicylic acid. Russ J Plant Physiol. (2009) 56:621–6. doi: 10.1134/S1021443709050069
38. Rohwer CL, Erwin JE. Horticultural applications of jasmonates: a review. J Hortic Sci Biotechnol. (2008) 83:283–304. doi: 10.1080/14620316.2008.11512381
39. Konan YKF, Kouassi KM, Kouakou KL, Koffi E, Kouassi KN, Sekou D, et al. Effect of methyl jasmonate on phytoalexins biosynthesis and induced disease resistance to Fusarium oxysporum f. sp. Vasinfectum in cotton (Gossypium hirsutum L.). Int J Agron. (2014) 2014:806439. doi: 10.1155/2014/806439
40. Mendoza D, Cuaspud O, Arias JR, Ruiz O, Arias M. Effect of salicylic acid and methyl jasmonate in the production of phenolic compounds in plant cell suspension cultures of Thevetia peruviana. Biotechnol Rep. (2018) 19:e00273. doi: 10.1016/j.btre.2018.e00273
41. Pauwels L, Barbero GF, Geerinck J, Tilleman S, Grunewald W, Perez AC, et al. NINJA connects the co-repressor TOPLESS to jasmonate signalling. Nature. (2010) 464:788–91. doi: 10.1038/nature08854
42. Ruiz-Garcia Y, Romero-Cascales I, Bautista-Ortin AB, Gil-Munoz R, Martinez-Cutillas A, Gomez-Plaza E. Increasing bioactive phenolic compounds in grapes: response of six monastrell grape clones to benzothiadiazole and methyl jasmonate treatments. Am J Enol Vitic. (2013) 64:459–65. doi: 10.5344/ajev.2013.13038
43. Zhang FS, Wang XQ, Ma SJ, Cao SF, Li N, Wang XX, et al. Effects of methyl jasmonate on postharvest decay in strawberry fruit and the possible mechanisms involved. Acta Hortic. (2006) 712:693–8. doi: 10.17660/ActaHortic.2006.712.87
44. Yao H, Tian S. Effects of pre- and post-harvest application of salicylic acid or methyl jasmonate on inducing disease resistance of sweet cherry fruit in storage. Postharvest Biol Technol. (2005) 35:253–62. doi: 10.1016/j.postharvbio.2004.09.001
45. Ni JB, Zhao Y, Tao RY, Yin L, Gao L, Strid A, et al. Ethylene mediates the branching of the jasmonate-induced flavonoid biosynthesis pathway by suppressing anthocyanin biosynthesis in red Chinese pear fruits. Plant Biotechnol J. (2020) 18:1223–40. doi: 10.1111/pbi.13287
46. Jaakola L. New insights into the regulation of anthocyanin biosynthesis in fruits. Trends Plant Sci. (2013) 18:477–83. doi: 10.1016/j.tplants.2013.06.003
47. Martins V, Garcia A, Costa C, Sottomayor M, Geros H. Calcium- and hormone-driven regulation of secondary metabolism and cell wall enzymes in grape berry cells. J Plant Physiol. (2018) 231:57–67. doi: 10.1016/j.jplph.2018.08.011
48. Takahashi S, Namioka Y, Azis H, Sano T, Aono M, Koshiyama M, et al. Prohydrojasmon promotes the accumulation of phenolic compounds in red leaf lettuce. Plants. (2021) 10:1920. doi: 10.3390/plants10091920
49. Zhang Q, Yang WB, Liu JC, Liu H, Lv ZZ, Zhang CL, et al. Postharvest UV-C irradiation increased the flavonoids and anthocyanins accumulation, phenylpropanoid pathway gene expression, and antioxidant activity in sweet cherries (Prunus avium L.). Postharvest Biol Technol. (2021) 175:111490. doi: 10.1016/j.postharvbio.2021.111490
50. Ravaglia D, Espley RV, Henry-Kirk RA, Andreotti C, Ziosi V, Hellens RP, et al. Transcriptional regulation of flavonoid biosynthesis in nectarine (Prunus persica) by a set of R2R3 MYB transcription factors. BMC Plant Biol. (2013) 13:68. doi: 10.1186/1471-2229-13-68
51. Rahim MA, Busatto N, Trainotti L. Regulation of anthocyanin biosynthesis in peach fruits. Planta. (2014) 240:913–29. doi: 10.1007/s00425-014-2078-2
52. Tuan PA, Bai S, Yaegaki H, Tamura T, Hihara S, Moriguchi T, et al. The crucial role of PpMYB10.1 in anthocyanin accumulation in peach and relationships between its allelic type and skin color phenotype. BMC Plant Biol. (2015) 15:280. doi: 10.1186/s12870-015-0664-5
53. Koshiyama M, Watanabe K, Fujisawa H, Mitomi M, Imamura K. Development of a new plant growth regulator, prohydrojasmon. Regul Plant Growth Dev. (2006) 41:24–33.
54. Couée I, Sulmon C, Gouesbet G, El Amrani A. Involvement of soluble sugars in reactive oxygen species balance and responses to oxidative stress in plants. J Exp Bot. (2006) 57:449–59. doi: 10.1093/jxb/erj027
55. Li Y, Van den Ende W, Rolland F. Sucrose induction of anthocyanin biosynthesis is mediated by DELLA. Mol Plant. (2014) 7:570–2. doi: 10.1093/mp/sst161
56. Lueangprasert K, Uthaibutra J, Saengnil K, Arakawa O. The effects of sugar application on the concentrations of anthocyanin and flavonol of ‘Mahajanaka’ mango (Mangifera indica Linn. cv. Mahajanaka) Fruit. Chiang Mai J Sci. (2010) 37:355–62.
57. Li D, Zhang X, Xu YQ, Li L, Aghdam MS, Luo ZS. Effect of exogenous sucrose on anthocyanin synthesis in postharvest strawberry fruit. Food Chem. (2019) 289:112–20. doi: 10.1016/j.foodchem.2019.03.042
58. Olivares D, Contreras C, Munoz V, Rivera S, Gonzalez-Aguero M, Retamales J, et al. Relationship among color development, anthocyanin and pigment-related gene expression in ‘Crimson Seedless’ grapes treated with abscisic acid and sucrose. Plant Physiol Biochem. (2017) 115:286–97. doi: 10.1016/j.plaphy.2017.04.007
59. Hara M, Oki K, Hoshino K, Kuboi T. Enhancement of anthocyanin biosynthesis by sugar in radish (Raphanus sativus) hypocotyl. Plant Sci. (2003) 164:259–65. doi: 10.1016/S0168-9452(02)00408-9
60. Tao XY, Wu Q, Li JY, Cai LY, Mao LC, Luo ZS, et al. Exogenous methyl jasmonate regulates sucrose metabolism in tomato during postharvest ripening. Postharvest Biol Technol. (2021) 181:111639. doi: 10.1016/j.postharvbio.2021.111639
61. Muengkaew R, Chaiprasart P, Warrington I. Changing of physiochemical properties and color development of mango fruit sprayed methyl Jasmonate. Sci Hortic. (2016) 198:70–7. doi: 10.1016/j.scienta.2015.11.033
62. Jeong EJ, Imran M, Kang SM, Khan MA, Lee IJ. The application of diniconazole and prohydrojasmon as plant growth regulators to induce growth and tuberization of potato. J Appl Bot Food Qual. (2021) 94:39–46. doi: 10.5073/JABFQ.2021.094.005
63. Han YL, Chen C, Yan ZM, Li Y, Wan YH. The methyl jasmonate accelerates the strawberry fruits ripening process. Sci Hortic. (2019) 249:250–6. doi: 10.1016/j.scienta.2019.01.061
64. Wurtele ES, Nikolau BJ. Enzymes of glucose oxidation in leaf tissues: the distribution of the enzymes of glycolysis and the oxidative pentose phosphate pathway between epidermal and mesophyll tissues of C(3)-plants and epidermal, mesophyll, and bundle sheath tissues of C(4)-Plants. Plant Physiol. (1986) 82:503–10. doi: 10.2307/4270216
Keywords: color development, sugar metabolism, peach [Prunus persica (L.) Batsch], methyl jasmonate (MeJA), prohydrojasmon (PDJ), sucrose metabolism
Citation: Tang T, Zhou H, Wang L, Zhao J, Ma L, Ling J, Li G, Huang W, Li P and Zhang Y (2022) Post-harvest Application of Methyl Jasmonate or Prohydrojasmon Affects Color Development and Anthocyanins Biosynthesis in Peach by Regulation of Sucrose Metabolism. Front. Nutr. 9:871467. doi: 10.3389/fnut.2022.871467
Received: 08 February 2022; Accepted: 14 March 2022;
Published: 05 April 2022.
Edited by:
Daming Fan, Jiangnan University, ChinaReviewed by:
Leiqing Pan, Nanjing Agricultural University, ChinaTripti Vashisth, University of Florida, United States
Shuhua Zhu, Shandong Agricultural University, China
Copyright © 2022 Tang, Zhou, Wang, Zhao, Ma, Ling, Li, Huang, Li and Zhang. This is an open-access article distributed under the terms of the Creative Commons Attribution License (CC BY). The use, distribution or reproduction in other forums is permitted, provided the original author(s) and the copyright owner(s) are credited and that the original publication in this journal is cited, in accordance with accepted academic practice. No use, distribution or reproduction is permitted which does not comply with these terms.
*Correspondence: Pengxia Li, guoshubaoxian@163.com; Yingtong Zhang, ytzhang@jaas.ac.cn
†These authors have contributed equally to this work