- 1Aqua Research Lab, Department of Zoology, University of Delhi, New Delhi, India
- 2Department of Biotechnology, Delhi Technological University, New Delhi, India
- 3Institute of Aquaculture, Faculty of Natural Sciences, University of Stirling, Stirling, United Kingdom
- 4Guangdong Provincial Key Laboratory of Marine Biotechnology, Shantou University, Shantou, China
The freshwater macrophytes are abundant in tropical and subtropical climates. These macrophytes may be used as feed ingredients for fish and other animals. The nutritional value of twelve freshwater-cultured macrophytes was evaluated in the present study. Significantly higher crude protein (36.94–36.65%) and lipid (8.13–7.62%) were found in Lemna minor and Spirodela polyrhiza; ash content was significantly higher in Hydrilla verticillata, Wolffia globosa, and Pistia stratiotes (20.69–21.00%) compared with others. The highest levels of sodium, magnesium, chromium, and iron levels were recorded in P. stratiotes. H. verticillata was a rich source of copper, manganese, cobalt, and zinc; the contents of calcium, magnesium, strontium, and nickel were highest in S. polyrhiza. Selenium and potassium contents were higher in Salvinia natans and W. globosa, respectively. The n-6 and n-3 polyunsaturated fatty acids (PUFAs) contents were significantly higher in W. globosa and Ipomoea aquatica, respectively compared with others. Linoleic and α-linolenic acids were dominant n-6 and n-3 PUFAs. The highest value (4.04) of n-3/n-6 was found in I. aquatica. The ratio ranged from 0.61 to 2.46 in other macrophytes. This study reveals that macrophytes are rich sources of minerals, n-6 and n-3 PUFAs.
Introduction
Freshwater macrophytes, the fastest growing aquatic plants, are abundant in tropical and subtropical countries. They grow profusely in nutrient-rich water. These macrophytes are broadly classified into four groups based on their occurrence in the water body: the surface floating (e. g. Azolla spp.), submerged (e. g. Hydrilla spp.), emergent (e. g. Potamogeton spp.), and marginal (e. g. Ipomoea spp.). The nutritional value of freshwater macrophytes has been recognized globally. The unchecked propagation of freshwater macrophytes creates problems in many water bodies. The judicial exploitation of these nutrient-rich plants may open a new avenue from a nutritional view point for humans and animals. The leaf protein extracted from freshwater macrophytes may be used for human or non-ruminant animals (1). Macrophytes are a rich source of protein, lipid, amino acids, fatty acids, and minerals (2). The amino acid and fatty acid profiles of duckweeds Lemna minor and Spirodela polyrhiza have been documented recently (3, 4). The mineral composition of macrophytes is different from the usual terrestrial vegetation. Calcium (Ca), iron (Fe), and manganese (Mn) contents are higher in aquatic plants compared with the terrestrial ones (1). Minerals are important catalysts for various biochemical reactions. These are essential components for metabolism, growth, and development and help the animals to cope with the variable environmental conditions (5). There is an optimum dose for each mineral. Low/high concentrations may affect the physiology of the organisms. Toxic minerals like arsenic (As), mercury (Hg), antimony (Sb), cadmium (Cd) etc., are required by the body in little amounts, whereas excess levels of useful minerals like, sodium (Na), potassium (K), magnesium (Mg), Ca, Fe etc., may be harmful (5). Dietary inclusions of polyunsaturated fatty acids (PUFAs) have several health benefits for humans and other animals. The study of the profiles of fatty acids of feed ingredients ensures the quality of diets. Fish are unable to synthesize two essential fatty acids like n-6 (derived from linoleic acid, LA) and n-3 (derived from alpha-linolenic acid, ALA). So these fatty acids should be supplied to the diets of fishes (6).
The evaluation of minerals and fatty acids' compositions of aquatic macrophytes is essential for their selection as potential feed ingredients for fish and other animals. Some of the commonly occurring freshwater macrophytes are: Azolla microphylla, A. pinnata, Enhydra fluctuans, Hydrilla verticillata, Ipomoea aquatica, Lemna minor, Marsilea quadrifolia, Pistia stratiotes, Salvinia molesta, S. natans, Spirodela polyrhiza, and Wolffia globosa. These macrophytes are distributed throughout the temperate, sub-tropical, and tropical regions of the world. Some of these macrophytes like, E. fluctuans, I. aquatica, and M. quadrifolia, are consumed as vegetables by humans in India and Bangladesh (7), and W. arrhiza has been consumed in Thailand (8). Most of these macrophytes, except H. verticillata (submerged plant), M. quadrifolia, and E. fluctuans (marginal plants) are surface floating macrophytes. All these macrophytes propagate through vegetative reproduction. Mosquito fern Azolla spp. (Azollaceae) are heterosporous free-floating ferns. It lives symbiotically with nitrogen-fixing blue-green algae Anabaena azollae. Watercress Enhydra fluctuans (Asteraceae) is a hydrophytic plant and it grows in canals and marshy places. Waterthyme Hydrilla verticillata (Hydrocharitaceae) is a submerged, rooted aquatic plant. It can grow in water up to a depth of 6 m, and in transparent water it can survive up to a depth of 12 m. The water spinach Ipomoea aquatica (Convolvulaceae) with hollow roots floats in water easily. Three members of the family Lemnaceae, namely Lemna spp., Spirodela spp., and Wolffia spp. are known as duckweeds. The plant consists of a single leaf or frond with one or more roots. Water clover Marsilea quadrifolia (Marsileaceae) is a deciduous, aquatic fern. Each green and thin stalk rises from the rhizome to the water surface; it contains a single shamrock-like leaf with four leaflets. Water cabbage Pistia stratiotes (Araceae) is a perennial monocotyledon with thick, soft, and light green leaves that form a rosette. It floats on the surface of the water and roots are hanging beneath the leaves. The short stolon connects both the mother and daughter plants. Water fern Salvinia spp. (Salviniaceae) is a perennial free-floating macrophyte. During the period of high growth, leaf size decreases and both leaves and stems fold, doubling and layering to cover more of the water surface. The nutritional value of macrophytes in terms of proteins, lipids, ash etc. varies greatly (2). The culture medium influences the mineral contents of the macrophytes (8). The extracts of seven freshwater macrophytes show no cytotoxic and anti-proliferative effects on human cell lines (9). Therefore, macrophytes should be considered as useful feed ingredients. Production of macrophytes using a standard technique may help to maintain the nutritional value of the plant and also maximize the health benefits.
The aim of the present study is to evaluate the nutritional value, viz. proximate composition, minerals and fatty acids profiles of twelve cultured freshwater macrophytes. This study will help to evaluate the suitability of these macrophytes as feed ingredients for fish, poultry, and livestock.
Materials and Methods
Culture of Macrophytes
Freshwater macrophytes were collected from water bodies of Delhi, Uttar Pradesh, and West Bengal and then identified. Macrophytes were cultured in outdoor cemented tanks (1.2 × 0.35 m) with clean dechlorinated tap water (3). A 10-cm layer of soil was used for the culture of H. verticillata, M. quadrifolia, and E. fluctuans. All other macrophytes were cultured without soil base. The depth of water was 30 cm in all culture tanks. A combination of organic manures viz. cattle manure, poultry droppings, and mustard oil cake was used (1:1:1) at the rate of 1.052 kg/m3. All manures were decomposed for 5 days and then macrophytes were introduced individually in the outdoor cemented tanks. Three replicates were used for each macrophyte. For the steady supply of nutrients for the growth of macrophytes, the same combination of manures (at one-fourth dose of the initial one) was applied in the culture tanks. Manures were decomposed for 5 days in separate containers and then applied on day 6. This schedule was followed throughout the culture period. Culture tanks were monitored regularly and macrophytes were harvested when the whole surface of the tank was covered with plants. The freshly harvested macrophytes were washed twice with tap water and then with distilled water. After air drying, macrophytes were kept at 40°C for 3 h. Then the ground, sieved, and fine powders were kept in air-tight containers and stored in a refrigerator at 4°C for further assay.
Proximate Composition Analysis
The proximate composition of the macrophytes was analyzed (10). Three replicates were used for each assay. Moisture content was estimated after drying the sample at 105°C for 24 h. The dried samples were kept in a muffle furnace at 550°C for 8 h for the determination of ash contents. The crude protein contents were analyzed by measuring the nitrogen content (N x 6.25) with an automated micro-Kjeldhal apparatus (Pelican Instruments, Chennai, India). Crude lipid contents of the macrophytes were assayed gravimetrically (11). Carbohydrate contents were estimated by the subtraction method.
Mineral Assay
The mineral compositions of macrophytes were assayed using Inductively-Coupled Plasma Mass Spectrometer (ICP-MS, Agilent 7900, USA) following standard protocol at the Instrumentation Facility of Indian Institute of Technology, New Delhi. The powdered macrophyte sample (150 mg) was taken in a closed digestion vessel and 8 ml of suprapure 69% nitric acid (HNO3, Merck, USA) was added to this. The sample was digested in Microwave digestion system (Multiwave PRO; Anton Paar, Austria). The digested sample was cooled at room temperature and transferred into a measuring cylinder; Milli-Q ultrapure water was added to make the volume 40 ml. Then the sample was filtered through a 0.2 μM syringe filter (Thermo Scientific, USA) and was collected in a glass vial. A 20 μL sample was injected through autosampler in the ICP-MS. The standard solution for each mineral was supplied with the equipment (Agilent Technologies, USA). It was diluted with Milli-Q ultrapure water containing 1% HNO3 to make concentrations of 20, 40, 60, 80, 100, 250, 500, 1000 μg/l. The calibration (standard) curve was prepared. The blank was prepared with Milli-Q ultrapure water containing HNO3 (1%). Minerals are divided into three major groups based on their concentrations in the mammal/human body viz. macro, trace, and ultra-trace minerals (5).
Fatty Acid Analysis
The fatty acid profiles of the macrophytes were analyzed using Gas Chromatograph (GC)–Flame Ionization Detector, Clarus 580 (Perkin Elmer, USA). The total lipid extracted from plants (11) was used to prepare fatty acid methyl esters (FAME) by transesterification using sulfuric acid in methanol at 50°C for 16 h (12). After extraction and purification of FAME (13), 1 ml sample was kept in a glass vial of autosampler of GC. The sample was separated and quantified in a GC column (60 m × 0.32 mm i.d. × 0.25 μm ZB-wax, Phenomenex, UK). The data were collected from pre-installed program software (TotalChrom Workstation Ver6.3, Perkin Elmer). The FAME was identified with the help of standards (Supelco FAME 37 mix, Sigma-Aldrich, USA).
Statistical Analysis
The compositions of twelve macrophytes are given as means ± standard error (SE). The differences in nutritional values of various macrophytes were tested using one-way analysis of variance (ANOVA) and Duncan's multiple range test (14). Statistical analyses were performed using the SPSS program (version 25.0). Statistical significance was accepted at p < 0.05.
Results
Proximate Composition
The moisture content was highest (11.86%) and lowest (6.26%) in E. fluctuans and W. globosa, respectively (Figure 1). Significantly higher crude protein contents were found in two duckweeds, namely L. minor and S. polyrhiza, compared with others. The highest lipid content was also recorded in L. minor, followed by S. polyrhiza. The lipid content was minimum in E. fluctuans. Ash content was significantly higher in H. verticillata, W. globose, and P. stratiotes compared with other macrophytes. The ash content was minimum in M. quadrifolia. Carbohydrates levels were minimum and maximum in L. minor and M. quadrifolia, respectively.
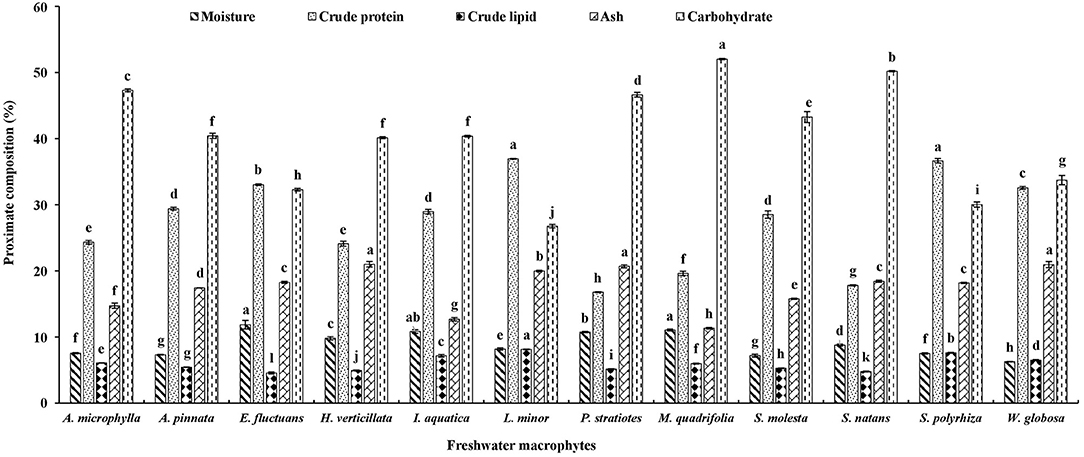
Figure 1. Proximate composition of twelve freshwater macrophytes cultured with organic manures. Bars with different superscripts are significantly different (n = 3).
Mineral Composition
Macrominerals
Among these twelve freshwater macrophytes, Na content was significantly higher in P. stratiotes compared with others (Figure 2A). This group was followed by S. natans and E. fluctuans. A significantly higher K level was found in W. globosa compared with others. This was followed by L. minor, E. fluctuans, H. verticillate, and P. stratiotes. Ca content was highest in S. polyrhiza, followed by P. stratiotes. A significantly higher Mg level was found in P. stratiotes and S. polyrhiza compared with others. The Na, Ca, and Mg contents were minimum in M. quadrifolia compared with other macrophytes. It indicates the nutritional value of the macrophytes.
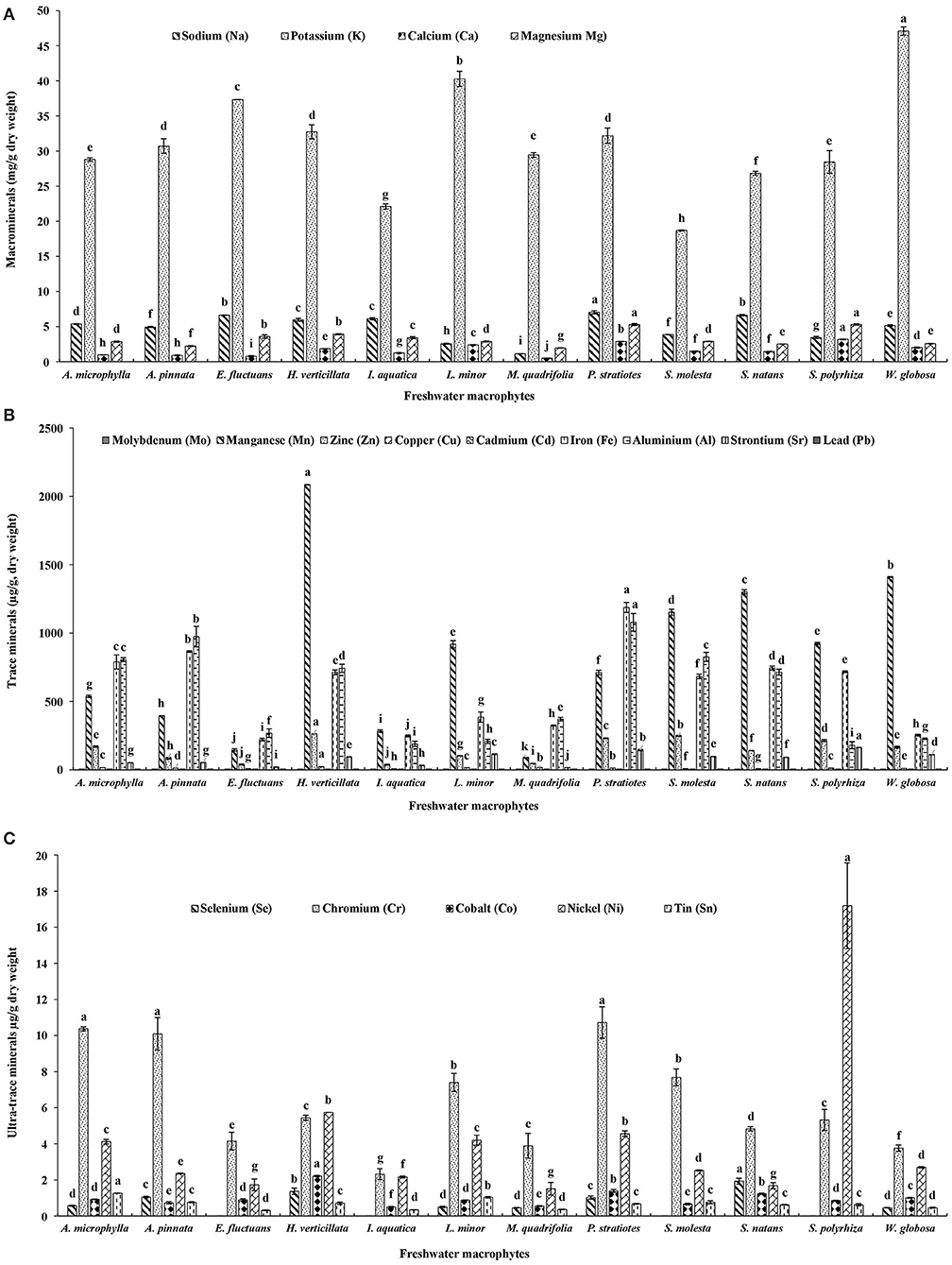
Figure 2. (A) Macrominerals, (B) trace minerals, and (C) ultra-trace minerals contents of twelve freshwater macrophytes cultured with organic manures. Bars with different superscripts are significantly different (n = 3).
Trace Minerals
A total of nine trace minerals were found in these macrophytes (Figure 2B). Molybdenum (Mo) content was significantly higher in A. microphylla, A. pinnata, and P. stratiotes compared with others. Mn, zinc (Zn), copper (Cu), and Cd contents were significantly higher in H. verticillata compared with others. In P. stratiotes, significantly higher levels of Fe and aluminum (Al) were found compared with others. Among these macrophytes, A. pinnata ranked second for both Fe and Al. A. microphylla ranked third for Fe and fourth for Al contents. Maximum strontium (Sr) level was recorded in S. polyrhiza followed by P. stratiotes. In all these macrophytes lead (Pb) was found.
Ultra-Trace Minerals
Five ultra-trace minerals were found in these macrophytes (Figure 2C). A significantly higher level of selenium (Se) was found in S. natans compared with others. This plant was followed by H. verticillata and P. stratiotes. Se was absent in S. molesta, E. fluctuans, I. aquatica, and S. polyrhiza. Chromium (Cr) content was significantly higher in P. stratiotes, A. microphylla, and A. pinnata compared with others. Cobalt (Co) content was significantly higher in H. verticillata compared with others. This was followed by P. stratiotes and S. natans. Nickel (Ni) and tin (Sn) levels were significantly higher in S. polyrhiza and A. microphylla, respectively compared with others. Among these macrophytes, P. stratiotes ranked third for Ni content.
Fatty Acid Profile
The fatty acid profiles of twelve freshwater macrophytes were documented in the present study (Tables 1–3). The saturated fatty acids (SFAs) content was significantly higher in W. globosa compared with others. This was followed by A. pinnata, L. minor, and I. aquatica. SFA content was minimum in P. stratiotes. Among, SFA, palmitic acid (C16:0) was the dominant one in all these plants. Monounsaturated fatty acids (MUFA) content was significantly higher in M. quadrifolia compared with others. Among various MUFAs, oleic acid (C18:1n-9) was present in most of the plants and the amount was also higher compared with the others (Supplementary Tables 1A–C). MUFA content was also minimum in P. stratiotes. Though in small amounts two other monounsaturated fatty acids like, palmitoleic acid (C16:1n-9) and nervonic acid (C24:1), were present in all macrophytes, except E. fluctuans and A. pinnata. Another isomer of palmitoleic acid (C16:1n-7) was absent in two species of Azolla and S. natans.
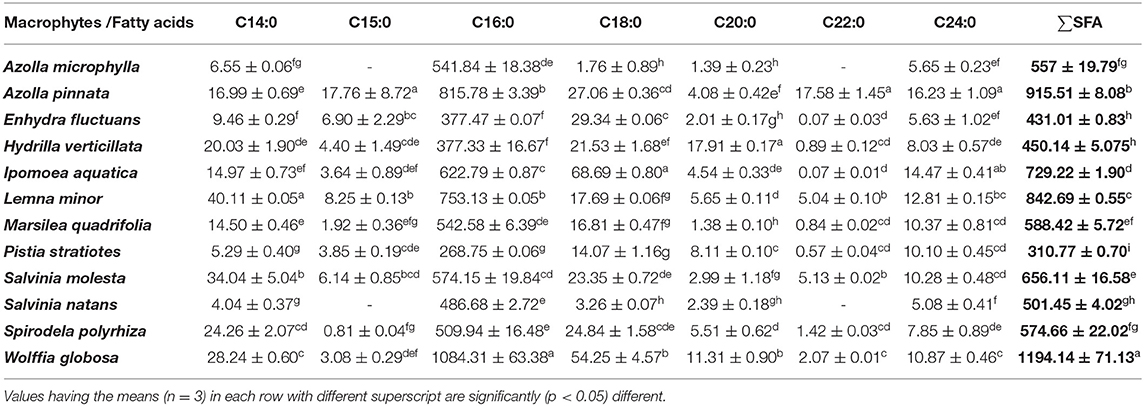
Table 1. Saturated fatty acids (SFA) profiles of freshwater macrophytes cultured with organic manures (mg/100 g, dry weight).
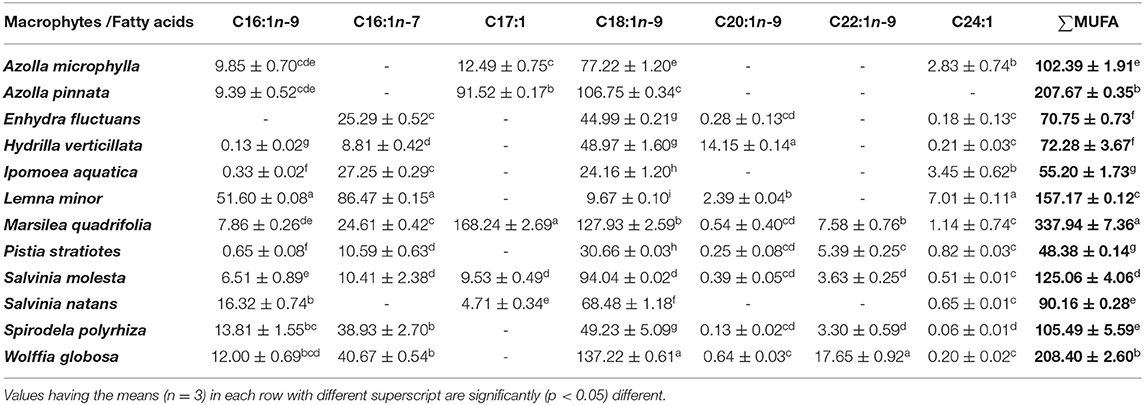
Table 2. Monounsaturated fatty acids (MUFA) profiles of freshwater macrophytes cultured with organic manures (mg/100 g, dry weight).
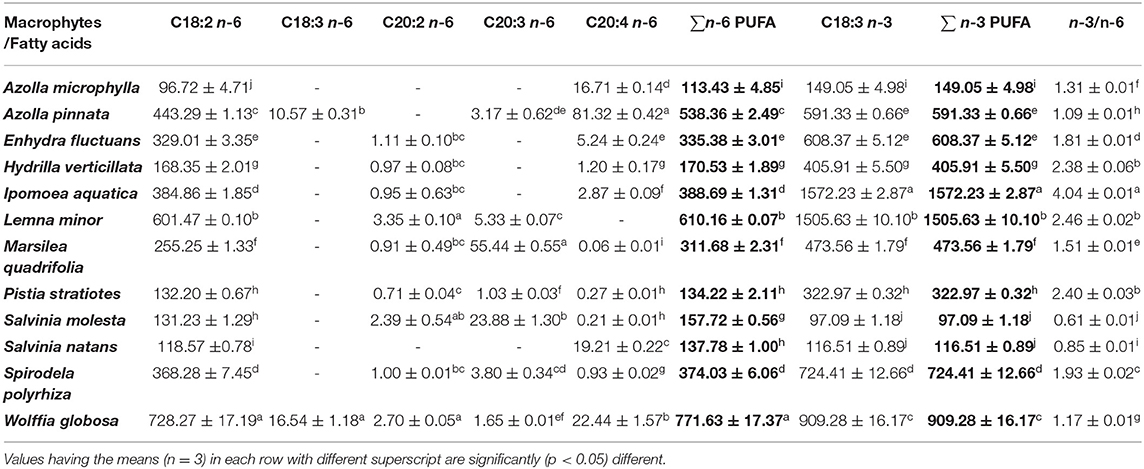
Table 3. Polyunsaturated fatty acids (PUFA) profiles of freshwater macrophytes cultured with organic manures (mg/100 g, dry weight).
The n-6 PUFA content was significantly higher in W. globosa compared with others. This was followed by L. minor and A. pinnata. The minimum level was found in A. microphylla. Among n-6 PUFA, LA (C18:2n-6) was the dominant one and was present in all macrophytes. Arachidonic acid (C20:4n-6) was the second dominant n-6 PUFA found in all macrophytes, except in L. minor. ALA (C18:3n-3) was the only member of n-3 PUFA present in all these macrophytes. ALA content was significantly higher in I. aquatica compared with others. This was followed by L. minor and W. globosa. The highest (4.04) n-3/n-6 was found in I. aquatica (Supplementary Table 2). The ratio ranged from 0.61 (S. molesta)−2.46 (L. minor) in other macrophytes.
Discussion
A wide variation in the composition of freshwater macrophytes was recorded in the present study. The advantage of this study is that plants were cultured in the outdoor systems following a standard protocol (3). Therefore, almost the same quality of products is expected in a further study. There is scope for improvement in the nutritional value as the quality of the culture medium influences the composition of the plants.
In the present study, crude protein levels in three members of Lemnaceae family and E. fluctuans were above 30%, and protein contents of other macrophytes (except P. stratiotes, S. natans, and M. quadrifolia) were above 20%. The present study confirms the previous finding that macrophytes are rich sources of protein. The protein contents of L. minor and S. polyrhiza were 36.07 and 35.82%, respectively (3, 4). A previous study in Bangladesh reported that the protein contents of E. fluctuans and I. aquatica were 16.69 and 21.45%, respectively; macrophytes were collected from natural water bodies (15). In the present study, protein contents of E. fluctuans and I. aquatica were 16.35 and 7.51% higher compared with the same macrophytes studied in Bangladesh. Lipid contents of I. aquatica, S. polyrhiza, and L. minor ranged from 7.16 to 8.13% in the present study. The lipid contents of E. fluctuans and I. aquatica were 1.90 and 3.82% higher in the present study compared with the previous study (15). Ash contents of these two macrophytes were also higher in the present study compared with the previous one. Higher levels of ash contents of H. verticillata, W. globosa, and P. stratiotes compared with other macrophytes enhanced the nutritional value of these plants as feed ingredients for fish, poultry, and livestock. In the present study, lower levels of carbohydrates were observed in macrophytes compared with the plants harvested from the wild (15). Culture of macrophytes with organic manures enhanced the nutritional value of plants.
Among these macrophytes, highest levels of macrominerals, Na and Mg, were found in P. stratiotes. K and Ca were highest in W. globosa and S. polyrhiza, respectively. In the present study, among various macrophytes, P. stratiotes ranked second and fifth for Ca and K, respectively. A previous study reported the highest Ca level in Hydrilla sp., followed by P. stratiotes and E. crassipes. There was no variation in Mg level among these three macrophytes (16). Macromineral profile of leaves and roots of P. stratiotes collected from a natural water body of Nigeria was documented (17). This study showed that Na, K, Ca, and Mg contents were 3.73, 32.83, 2.30, and 3.70 g/kg of leaves, respectively. In the present study, Na, Ca, and Mg contents were 47, 20, and 30%, respectively, higher in P. stratiotes compared with the plants studied in Nigeria. K content was almost the same in the plants grown in two different conditions. The Na, Ca, Zn, and Cu contents were higher in I. aquatica grown in Bangladesh compared with the macrophytes assayed in the present study (15). Although, Mg, K, and Fe contents were higher in the I. aquatica assayed in the present study compared with the plants studied in Bangladesh, Na, Mg, and K contents were higher in E. fluctuans evaluated in the present study compared with the previous study in Bangladesh. The Na, K, Mg, and Ca contents were higher in A. filiculoides and S. molesta grown in swine lagoons compared with the present study (18). In A. filiculoides, Na, K, Mg, and Ca contents were 2.77, 22.5, 5.04, and 9.3 g/kg (dry matter), respectively. In S. molesta Na, K, Mg, and Ca contents were 4.44, 34.7, 5.18 and 10.6 g/kg (dry matter), respectively.
In the present study, Na to K ratio ranged from 0.038 (M. quadrifolia) −0.276 (I. aquatica). The ratio was 0.063, 0.109, 0.121, 0.160, 0.177, 0.182, 0.185, 0.205, 0.218, and 0.238 in L. minor, W. globosa, S. polyrhiza, A. pinnata, E. fluctuans, H. verticillata, A. microphylla, S. molesta, P. stratiotes, and S. natans, respectively. In all these macrophytes, the ratio of Na to K is less than the WHO/FAO-recommended ratio for an adult human, i. e., <0.49 (19). Various studies showed the effect of culture medium on the mineral profile of macrophytes (8, 20, 21). In different species of duckweeds Na: K varied from 0.027–1.49 (K: Na = 0.67–37). In Wolffia, the ratio was 0.025 (K: Na = 40) and in another species, W. microscopica it was 0.003 (K: Na = 276).
In the present study, the Mg: Ca varied from 1.20 (L. minor)−4.65 in (E. fluctuans). Ca has been serving as the main structural mineral and helps in metabolism. It serves as a signal for vital physiological processes. Mg, the fourth most abundant cation in the body, is a co-factor for 350 cellular enzymes, most of which are involved in energy metabolism (22), hence, the ratio of Mg: Ca should be maintained. The Mg: Ca ratio was 0.4 in duckweed (21) and 0.5 in other species, W. microscopica (8). In the present study, the ratio was 1.28 for W. globosa.
The trace minerals analysis showed that among these macrophytes, P. stratiotes was a rich source for Mo, Fe, and Al. This macrophyte also has considerable amounts of Mn, Zn, and Sr. A. microphylla and A. pinnata were also rich sources of Fe and Mo. In a different strain of W. arrhiza, Fe contents ranged from 0.16–0.29 μg/g freeze-dried sample (23). The Fe content of W. globosa was 254.12 μg/g in the present study. Higher levels of Zn and Cu were found in I. aquatica grown in Bangladesh compared with the present study; Fe content was higher in the present study compared with the previous one (15). Fe content of E. fluctuans grown in two different environments was the same. Zn and Cu contents were lower in the plants assayed in the present study compared with the plants studied in Bangladesh. The Cu content of S. molesta grown in swine lagoons was 13 g/kg, dry weight (18). In the present study, Cu content of S. molesta was less compared with the previous study.
In the present study, the highest level of ultra-trace mineral Se was found in S. natans. This important mineral was also present in H. verticillata and P. stratiotes. It was interesting to record that Se was absent in S. molesta, S. polyrhiza, E. fluctuans, and I. aquatica. The Se content of freeze-dried W. arrhiza was <0.03 μg/g (23). In the present study, Se content of W. globosa was higher compared with the previous study. Significantly higher Cr levels were found in P. stratiotes, A. microphylla, and A. pinnata compared with other macrophytes. A significantly higher Co level was found in H. verticillata compared with the others. This macrophyte was followed by P. stratiotes and S. natans. Co content in all these macrophytes was >0.50 μg/g (dry weight). Among these macrophytes, the highest Ni content was found in S. polyrhiza, and this macrophyte was followed by H. verticillata and P. stratiotes. In the present study, the contents of heavy metals viz. Cd, Cu, Pb, and Sn of macrophytes were within the permissible limits (Cd: 0.2, Cu: 73.3, Pb: 0.3, Sn: 250 Zn: 99.40; mg/kg of wet weight) of WHO/FAO (24). In the present study, the mineral composition was evaluated in the dry sample. Therefore, the moisture (minimum 90%) contents of the samples should be considered at the time of comparison with the permissible limit of WHO/FAO for humans (where fresh plants were considered). In seaweeds, there is no regulation on the maximum heavy metals contents (25).
Various studies showed the dietary requirements of different macro, trace, and ultra-trace minerals for different animals (Supplementary Tables 3A,B). Na requirements of grass carp (Ctenopharyngodon idella), poultry, cattle, and humans are 2, 0.012–0.200, 0.96 g/kg diet, and 2.4 g/day, respectively. Among various fishes and prawns (Pinneaus indicus), Mg requirements vary from 0.4 to 0.946 g/kg of diets. K requirements recorded for common carp (Cyprinus carpio), grass carp, and Nile tilapia (Orechromis niloticus) are as follows: 0.9–12.4, 4.6, and 2.1–3.3 g/kg diets. K requirements for poultry, cattle, and humans are 0.3 and 2.4 g/kg diet and 3.5 g/day, respectively. Among different groups of fishes, rohu (Labeo rohita), common carp, grass carp, catla (Catla catla), and Nile tilapia require 1.9, 0.1, 2, 1.9 and 7 g Ca/kg diet, respectively. Ca requirements for poultry, cattle, and humans are 8 and 5.12 g/kg diet and 1.0 g/day, respectively. Among various fishes, Mn, Fe, Zn, and Co requirements vary from 12–25, 30–200, 15–79, and 0.01–0.5 mg/kg diet, respectively. Nile tilapia requires Se and Cr at the rate of 0.4 and 139.6 mg/kg diet, respectively. Fe, Zn, Cu, Se, Cr, and Co requirements are also evaluated for poultry, cattle, and humans. In channel catfish Ictaluraus punctatus, Fe, Cu, Mn, Zn, Se, and Co requirements were 30, 5, 25, 200, 0.1, and 0.05 mg/kg feed, respectively (26). In fish, Fe deficiency causes hypochromic microcytic anemia, Co and Mn deficiencies result in poor growth; Zn deficiency causes growth depression, cataract, and caudal fin and skin erosion; Se deficiency results in muscular dystrophy. In fish nutrition, Co plays a significant role. In common carp, the addition of cobalt chloride/cobalt nitrate enhanced the growth and hemoglobin formation (27). Therefore, supplementation of freshwater macrophytes may help to overcome the mineral deficiency in fish and other animals without showing any negative impact (9).
The fatty acid compositions of the two duckweeds L. minor and S. polyrhiza showed similarity with the previous study (3, 4). In the present study, palmitic acid and oleic acid were the dominant SFA and MUFA, respectively. Similar results were also found in four duckweeds, Landoltia, Lemna, Wolffiella, and Wolffia (8). The fatty acid compositions of four aquatic plants S. cuculata, Trapa natans, L. minor, and I. reptans showed that cis-15 tetracosenoic acid and 9-hexadecenoic acid were the dominant fatty acids, and highly unsaturated fatty acids contents were higher compared with the saturated fatty acids (28). In the present study, LA was the major contributor for n-6 PUFA in all plants, and except in L. minor, arachidonic acid was also found in all macrophytes. ALA was the only member of n-3 PUFA present in these macrophytes. The presence of LA and ALA were recorded in duckweeds (8). The freshwater teleosts are capable of converting ALA to long-chain polyunsaturated fatty acids (LC-PUFA) like eicosapentaenoic acid (EPA; 20:5n-3) and docosahexaenoic acid (DHA; 22:6n-3) (29–31). Therefore, the feeding of fish with freshwater macrophytes-based diets helps to fulfill the LC-PUFA requirements of cultured fish (32, 33). The n-6/n-3 PUFA was always <1; it ranged from 0.48–0.94 in different Wolffia species (23). A similar result was also found in the present study, except in two species of Salvania, where the ratio was >1.0.
Conclusion
Among these macrophytes, Na, Mg, Cr, and Fe contents were maximum in P. stratiotes; this macrophyte ranked second for Co, Sr, and Ca. H. verticillata was the richest source for Cu, Mn, Co, and Zn, and it ranked second for Se. Ca, Mg, Sr, and Ni contents were higher in S. polyrhiza compared with the others. S. natans and W. globosa were rich sources for Se and K, respectively. All these macrophytes were rich sources of n-6 and n-3 fatty acids. This study shows that macrophytes have an immense potential to be used as rich sources of minerals, as well as n-6 and n-3 PUFA for fish, poultry, and livestock.
Data Availability Statement
The original contributions presented in the study are included in the article/Supplementary Material, further inquiries can be directed to the corresponding author/s.
Author Contributions
JS, RC, and DRT designed the study. GK, RG, AS, NK, JS, and RC grown the macrophytes and analyzed samples. RC, DRT, GK, and JS wrote the manuscript. GK, AS, RG, NK, RC, DRT, and JS prepared tables. All authors contributed to the article and approved the submitted version.
Funding
This study has been supported by the Department of Biotechnology, DBT, New Delhi, India (Dy. No.102/IFD/SAN/4678/2015-2016) and Biotechnology and Biological Science Research Council, BBSRC (BB/N005031/1). GK is thankful to the Indian Council of Medical Research (3/1/3/JRF-2017/HRD-LS/55340/01).
Conflict of Interest
The authors declare that the research was conducted in the absence of any commercial or financial relationships that could be construed as a potential conflict of interest.
Publisher's Note
All claims expressed in this article are solely those of the authors and do not necessarily represent those of their affiliated organizations, or those of the publisher, the editors and the reviewers. Any product that may be evaluated in this article, or claim that may be made by its manufacturer, is not guaranteed or endorsed by the publisher.
Acknowledgments
We are thankful to Prof. Shailendra Goel, Department of Botany, University of Delhi for helping in the identification of macrophytes.
Supplementary Material
The Supplementary Material for this article can be found online at: https://www.frontiersin.org/articles/10.3389/fnut.2022.869425/full#supplementary-material
References
1. Boyd CE. Evaluation of some common aquatic weeds as possible feedstuffs. Hyacinth Control J. (1968) 7:26–27.
2. Hasan MR, Chakrabarti R. Use of algae and aquatic macrophytes as feed in small-scale aquaculture: a review. Fisheries Aquaculture Technical Paper 531. Rome, Italy: Food and Agricultural Organization, FAO (2009). p. 123. Available online at: http://www.fao.org/docrep/012/i1141e/i1141e.pdf
3. Chakrabarti R, Clark WD, Sharma JG, Goswami RK, Shrivastav AK, Tocher DR. Mass production of Lemna minor and its amino acid and fatty acid profiles. Front Chem. (2018) 6:479. doi: 10.3389/fchem.2018.00479
4. Sharma JG, Clark WD, Shrivastav AK, Goswami RK, Tocher DR, Chakrabarti R. Production potential of greater duckweed Spirodela polyrhiza (L. Schleiden) and its biochemical composition evaluation. Aquaculture. (2019) 513:734419. doi: 10.1016/j.aquaculture.2019.734419
5. Skalnaya MG, Skalny AV. Essential Trace Elements in Human Health: A Physician's View. Tomsk, Russia: Publishing House of Tomsk State University. (2018).
6. Horrobin DF, Manku MS. Clinical biochemistry of essential fatty acids. In: Horrobin DF, editor. Omega-6 Essential Fatty Acids: Pathophysiology and Roles in Clinical Medicine. New York: Wiley Liss (1990). p. 21–54.
7. Dewanji A, Matai S, Si L, Barik S, Nag A. Chemical composition of two semi-aquatic plants for food use. Plant Food Hum Nutr. (1993) 44:11–6. doi: 10.1007/BF01088478
8. Appenroth KJ, Sree KS, Böhm V, Hammann S, Vetter W, Leiterer M, et al. Nutritional value of duckweeds (Lemnaceae) as human food. Food Chem. (2017) 217:266–273. doi: 10.1016/j.foodchem.2016.08.116
9. Sree KS, Dahse H-M, Chandran JN, Schneider B, Jahreis G, Appenroth KJ. Duckweed for human nutrition: no cytotoxic and no anti-proliferative effects on human cell lines. Plant Food Hum Nutr. (2019) 74:223–4. doi: 10.1007/s11130-019-00725-x
10. Association of Official Analytical Chemists (AOAC). Official Methods of Analysis. AOAC International, seventeenth ed. Washington DC, USA: AOAC (2000).
11. Folch J, Lees M, Sloane-Stanley GH. A simple method for the isolation and purification of total lipids from animal tissues. J Biol Chem. (1957) 226:497–509. doi: 10.1016/S0021-9258(18)64849-5
13. Tocher DR, Harvie DG. Fatty acid compositions of the major phosphoglycerides from fish neural tissues; (n-3) and (n-6) polyunsaturated fatty acids in rainbow trout (Salmo gairdneri) and cod (Gadus morhua) brains and retinas. Fish Physiol Biochem. (1988) 5:229–239. doi: 10.1007/BF01874800
15. Satter MMA, Khan MMRL, Jabin SA, Abedin N, Islam MF, Shaha B. Nutritional quality and safety aspects of wild vegetables consume in Bangladesh. Asian Pac J Trop Biomed. (2016) 6:125–31. doi: 10.1016/j.apjtb.2015.11.004
16. Boyd CE. Freshwater plants: a potential source of protein. Econ Bot. (1968) 22:359–68. doi: 10.1007/BF02908132
17. Wasagu RS, Lawal M, Shehu S, Alfa HH, Muhammad C. Nutritive values, mineral and antioxidant properties of Pistia stratiotes (water lettuce). Nig J Basic Appl Sci. (2013) 21:253–7. doi: 10.4314/njbas.v21i4.2
18. Leterme P, Londono AM, Munoz JE, Súarez J, Bedoya CA, Souffrant WB, et al. Nutritional value of aquatic ferns (Azolla filiculoides Lam. and Salvinia molesta Mitchell) in pigs. Anim Feed Sci Tech. (2009) 149:135–48. doi: 10.1016/j.anifeedsci.2008.04.013
19. Bailey RL, Parker EA, Rhodes DG, Goldman JD, Clemens JC, Moshfegh AJ, et al. Estimating sodium and potassium intakes and their ratio in the American diet: data from the 2011–2012 NHANES. J Nutr. (2015) 146:745–50. doi: 10.3945/jn.115.221184
20. Landolt E, Kandeler R. The Family of Lemnaceae - A Monographic Study: Phytochemistry, Physiology, Application, and Bibliography. (Vol 2 of Monograph) Stiftung Rubel: Geobotanical Institute of the ETH. (1987).
21. Edelman M, Colt M. Nutrient value of leaf vs. seed. Front Chem. (2016) 4:32. doi: 10.3389/fchem.2016.00032
22. World Health Organization (WHO). Calcium and Magnesium in Drinking-Water: Public Health Significance. Geneva, Switzerland: World Health Organization, WHO Press (2009).
23. Appenroth KJ, Sree KS, Bog M, Ecker J, Seeliger C, Böhm V, et al. Nutritional value of the duckweed species of the genus Wolffia (Lemnaceae) as human food. Front Chem. (2018) 6:483. doi: 10.3389/fchem.2018.00483
24. Odai SN, Mensah E, Sipitey D, Ryo S, Awuah E. Heavy metals uptake by vegetables cultivated on urban waste dumpsites: case study of Kumasi, Ghana. Res J Environ Toxicol. (2008) 2:92–9. doi: 10.3923/rjet.2008.92.99
25. Chen Q, Pan XD, Huang BF, Han JL. Distribution of metals and metalloids in dried seaweeds and health risk to population in southeastern China. Sci Rep. (2018) 8:3578. doi: 10.1038/s41598-018-21732-z
26. Li MH, Robinson EH. Complete feeds - intensive systems. In: Davis DA, editor. Feed and Feeding Practices in Aquaculture. United Kingdom: Woodhead Publishing (2015). p. 111–126. doi: 10.1016/B978-0-08-100506-4.00004-0
27. Pillay TVR. Aquaculture: Principles and Practices. second ed. United States: Blackwell Publishing (2005).
28. Mukherjee AK, Kalita P, Unni BG, Wann SB, Saikia D, Mukhopadhyay PK. Fatty acid composition of four potential aquatic weeds and their possible use as fish-feed neutraceuticals. Food Chem. (2010) 123:1252–4. doi: 10.1016/j.foodchem.2010.05.057
29. Cook HW. Fatty acid Desaturation and Chain Elongation in Eukaryote. In: Vance DE, Vance JE editor. Biochemistry of Lipids, Lipoproteins and Membranes. Amsterdam, Netherlands: Elsevier (1996). p. 129–152. doi: 10.1016/S0167-7306(08)60512-8
30. Buzzi M, Henderson RJ, Sargent JR. Biosynthesis of docosahexaenoic acid in trout hepatocytes proceeds via 24-carbon intermediates. Comp Biochem Physiol B Biochem Mol Biol. (1997) 116:263–7. doi: 10.1016/S0305-0491(96)00210-6
31. Glencross BD. Exploring the nutritional demand for essential fatty acids by aquaculture species. Rev Aquacult. (2009) 1:71–124. doi: 10.1111/j.1753-5131.2009.01006.x
32. Tocher DR, Agaba M, Hastings N, Bell JG, Dick JR, Teale AJ. Nutritional regulation of hepatocyte fatty acid desaturation and polyunsaturated fatty acid composition in zebrafish (Danio rerio) and tilapia (Oreochromis niloticus). Fish Physiol Biochem. (2002) 24:309–20. doi: 10.1023/A:1015022406790
Keywords: alpha-linolenic acid, linoleic acid, n-3 polyunsaturated fatty acids, sodium, iron, freshwater macrophytes
Citation: Kumar G, Sharma JG, Goswami RK, Shrivastav AK, Tocher DR, Kumar N and Chakrabarti R (2022) Freshwater Macrophytes: A Potential Source of Minerals and Fatty Acids for Fish, Poultry, and Livestock. Front. Nutr. 9:869425. doi: 10.3389/fnut.2022.869425
Received: 04 February 2022; Accepted: 14 March 2022;
Published: 11 April 2022.
Edited by:
Lidia Montero, University of Duisburg-Essen, GermanyReviewed by:
Vishal Mishra, Indian Institute of Technology (BHU), IndiaSapna Langyan, National Bureau of Plant Genetic Resources (ICAR), India
Copyright © 2022 Kumar, Sharma, Goswami, Shrivastav, Tocher, Kumar and Chakrabarti. This is an open-access article distributed under the terms of the Creative Commons Attribution License (CC BY). The use, distribution or reproduction in other forums is permitted, provided the original author(s) and the copyright owner(s) are credited and that the original publication in this journal is cited, in accordance with accepted academic practice. No use, distribution or reproduction is permitted which does not comply with these terms.
*Correspondence: Rina Chakrabarti, YXF1YXJlc2VhcmNobGFiQHlhaG9vLmNvLmlu