- Pediatric Hepatology, Gastroenterology and Nutrition Center, King's College Hospital, London, United Kingdom
It has been 57 years since the first intestinal transplant. An increased incidence of graft rejection has been described compared to other solid organ transplants due to high immunogenicity of the bowel, which in health allows the balance between of dietary antigen with defense against pathogens. Expanding clinical experience, knowledge of gastrointestinal physiology and immunology have progress post-transplant immunosuppressive drug regimens. Current regimes aim to find the window between prevention of rejection and the risk of infection (the leading cause of death) and malignancy. The ultimate aim is to achieve graft tolerance. In this review we discuss advances in mucosal immunology and technologies informing the development of new anti-rejection strategies with the hope of improved survival in the next generation of transplant recipients.
Introduction
For those patients with irreversible intestinal failure, and significant complications including very poor quality of life, bowel transplant offers the only possibility to achieve enteral autonomy (1).
Bowel transplants have been attempted since the 1960's but success was limited by challenges in balancing prevention of rejection with risks of infection and malignancy. Advances in immunosuppression therapies significantly improved outcomes, the introduction of ciclosporin first described for use after bowel transplant by Grant et al. (2), and then more effective calcineurin inhibitors (3). However, survival rates after intestinal transplant currently lag behind those of isolated liver or renal transplant. Better graft survival after isolated small bowel transplant is associated with fewer, less severe, episodes of rejection (4) and liver-inclusive grafts are shown to have reduced rates of rejection compared to bowel transplant alone (though with higher rates of GvHD) (5, 6).
The Intestinal Transplant Registry and published single center experiences describe patient survival at 55–66% at 5 years and graft survival at 48–62%, with rejection (13%) and sepsis (50%) being the biggest contributors to patient death (7, 8). This is compared to a 5 year survival of 95% reported after liver transplant (9). Registry data reports PTLD (post transplant lymphoproliferative disorder) occurrence as 8% in the current era (10).
Can new scientific advances support improved management strategies to allow intestinal transplant survival to match those for other solid organ transplants, particularly in management of rejection, infection and malignancy?
Studies of mucosal immunology in other bowel disorders, such as inflammatory bowel disease (IBD), have revealed a complex network of interactions between immune and GI tract cell types. Developments in molecular and microscopy techniques allow increasingly detailed descriptions of gut specific immune cell subsets. Enhancing those subsets which limit inflammatory responses is advancing, with engineered monoclonal antibody and small molecule therapies already being used to manage other inflammatory disorders of the gut. Research on the gut microbiome has exploded since the first intestinal transplants, however, it remains to be proven whether the microbiome can be manipulated to alter mucosal immune responses. Developing these approaches may allow us to replicate the physiological balance of immunogenicity and tolerogenicity within the bowel after transplant, enhance immune monitoring and immunosuppression and thus improve transplant outcomes. Tissue engineering is also an expanding field, organoids from autologous or animal derived cells could become part of the future of intestinal transplant, potentially preventing the need for immunosuppression altogether.
Immune Cell Modulation in Bowel Transplant
Rejection and infection management strategies can be improved with better understanding of the immune interactions we aim to modulate. Relevant lymphocyte sub sets in the gut are the regulatory T cells (Treg), resident memory (Trm) and effector memory cells (Tem) which themselves can be derived from CD4 or CD8 progenitors. High concentrations of Treg have been demonstrated in the bowel, as their name suggests, to allow tolerogenicity toward nutrient antigen and the healthy microbiome (11). Treg are characterized by the FOXP3 nuclear transcription factor (IPEX is a human disease where there is a mutation in the FOXP3 gene, characterized by immune dysregulation, demonstrating the important role of these cells in regulating or immune system). Treg can also be characterized by cell surface markers CD45 and CD25 (a component of the IL-2 receptor, which is targeted by basilixumab). Treg are shown to suppress CD4 and CD8 T cells, possibly also B cells. Tregs can also produce anti-inflammatory cytokines TGF-beta and IL-10 (12).
There is thought to be a plasticity in phenotype, with peripheral Treg cells migrating to the intestine and via interactions with other immune cell types and antigens being able to shift to express markers more associated with CD4 Tem cells (13). Studies in different solid organ transplant models demonstrate a role for donor specific Treg in promoting graft tolerance.
Studies in Pediatric liver transplant recipients have described a role for Treg in episodes of acute rejection (14).
T Cell Chimerism Role in Graft Rejection
Chimerism after organ transplant refers to the presence of donor/graft and recipient/host immune cells within the organ graft. It is the interaction between the donor and recipient immune systems which modulates between episodes of cellular rejection and tolerance. Populations of distinct tissue resident (rather than circulating) T cells have been demonstrated in the human GI mucosa. The unique environment of bowel transplant, with populations of host and donor cells, has allowed this tissue resident cell type to be directly interrogated. Bartolome-Casado et al. (15) have demonstrated CD8 Trm cells not only persist from the donor in the intestinal mucosa but are also functional. In the parallel paradigm of lung transplant, Snyder et al. (16) have demonstrated that host T cells can migrate into the mucosa to acquire a similar tissue resident phenotype.
Donor chimerism in the intestinal mucosa after bowel transplant has been observed to persist up to 5 years post-transplant (17). Zuber et al. (18) found early T cell mediated rejection after bowel transplant demonstrated a higher proportion of host vs. graft T cell clones, which declined after resolution, compared to little difference in late episodes of rejection. They described persistence of a stable repertoire of donor T cells in non-rejection bowel transplant mucosa. Also, a high turnover of recipient T cells migrating into the graft at early time points which then stabilized and replaced the donor T cells. In a separate study Zuber et al. (19) described macrochimerism of immune cells, i.e., >1%, between donor and recipient may be predictive of inducing tolerance. Their study demonstrated chimerism in peripheral blood of intestinal transplant patients, especially T cell chimerism, was associated with fewer/less severe episodes of rejection (similar findings were demonstrated in the lung transplant paradigm by Bartolome-Casado). Lower severity of rejection was also associated with lower levels of pre-formed donor specific antibodies (DSA). Better levels of chimerism were demonstrated after multivisceral rather than isolated small bowel transplant (possibly related to splenectomy and thus reduced host lymphoid compartment in the former). In the future, routinely measuring chimerism within the intestinal graft may become a model for predicting episodes of rejection and altering immunosuppression to prevent it.
Immunosuppression Regimens to Prevent Acute Cellular Rejection
Trafficking signals in the mucosal microenvironment attract effector cells of innate immunity such as neutrophils, circulating lymphocytes and macrophages via cell surface receptors and adhesion molecules. Cytokine intermediaries, such as IL-2, IL-23, TNF-alpha can be released and promote pro-inflammatory signals between tissue resident lymphocyte sub-sets and their neighbors. These pathways can all be targeted to modulate immunosuppression post-transplant.
Initial use of calcineurin inhibitor cyclosporin in the 80's, was replaced by tacrolimus (FK506) in the 90's as primary maintenance due to better outcomes and improved side effect profile. The most recent annual report from the US Organ procurement and transplant network in 2020 states Tacrolimus remains the choice for maintenance immunosuppression after bowel transplant, in combination with corticosteroids (inhibit T cell proliferation, antibody production and neutrophil activity) and/or mycophenolate mofetil (inhibitor of purine synthesis) (20, 21). Tacrolimus targets the intracellular calcineurin signaling pathway (3) to inhibit T cell proliferation. Sirolimus is also used as an adjunct immunosuppressant, but with the disadvantage of delayed wound healing (22, 23), it inhibits T cell proliferation by blocking the action of the intra-cellular signaling enzyme mTOR (mammalian target of rapamycin).
Induction immunosuppression, to deplete T cells pre/intra-operatively, is widely used, with rabbit ATG (anti-thymoglobulin), alemtuzumab (anti-CD52 antibody targeting lymphocyte cell surface receptor), muromonab CD3 (antibody against T cell surface protein CD3) or daclizumab (antibody against cell surface receptor CD25), in order of most to least used as reported in the US in 2008 (21). Basilixumab (antibody against IL-2) is used as an induction agent in some centers (22, 24).
Recent single center experiences describe analysis of induction regimens with the aim to optimize outcomes. Devine et al. (25) have reported a higher occurrence of post-transplant lymphoproliferative disorder (PTLD) in pediatric intestinal transplants recipients receiving basilixumab (anti-IL-2) and alemtuzumab (anti-CD52) compared to rATG (rabbit anti-thymoglobulin). Vianna et al. (26) have reported alemtuzumab induction alone lowered incidence of GvHD (Graft versus Host Disease), but also that intensive induction with combined rATG and rituximab (anti-CD20) was protective against episodes of acute rejection in the early post-transplant period. These studies demonstrate the balance between graft and host that needs to be achieved for graft survival and reduced risk of malignant host immune proliferation (Table 1).
Immunosuppression strategies such as that described by Ceulemans et al. (27) use knowledge of immune responses to optimize outcomes. The Leuven protocol, with donor-specific blood transfusion and lower long-term maintenance immunosuppression aimed to induce Treg cells thus promoting graft tolerance, and indeed had good graft and patient outcomes. Of note in this and other series (28) there was strict donor selection and short cold ischemic time in order to reduce the inflammatory stimulation of ischemia reperfusion injury.
Proposed New Drug Therapies
Cytokine Directed Therapies
Increased expression of TNF alpha has been described in episodes of small bowel transplant rejection (29). Pech et al. have described use of anti-TNF therapy to overcome episodes of acute rejection in a rat model of bowel transplant (30) and also reduce inflammatory infiltrate and dysmotility in the same rat model (31). Case reports and cohort studies describe beneficial use of anti-TNF to treat patients with acute rejection resistant to conventional therapy, and one report describing use for mucosal inflammation in an intestinal graft (32–35). Kroemer et al. (36) have demonstrated a good response to infliximab therapy in episodes of rejection unresponsive to ATG. When immune cells from the intestinal mucosa of these patients were interrogated, non-responders to ATG were shown to have a higher proportion of IL-17 and TNF-alpha producing CD4 T cells. The authors hypothesize this is why targeted therapy with anti-TNF therapy infliximab showed endoscopic and histological resolution of rejection.
Kodama et al. (37) used a rat model of intestinal transplant to investigate the novel immunosuppressive compound Prenylated Quinolinecarboxylic Acid compound 18 (PQA-18). The compound inhibits p21-activated kinase 2, reducing cytokine production of IL-2, IL-6 and TNF-alpha. The study showed better graft survival associated with use of PQA-18 likely secondary to suppressed T cells and macrophage differentiation.
Anti-cell Adhesion Molecules
β2-integrins form leukocyte adhesion molecules on the surface of lymphocytes, and could be linked to promoting inflammation in a transplant graft via their previously described roles in lymphocyte trafficking and disease activity in IBD. Antibodies against cell adhesion molecules, such as the α4β7 integrin blocker vedolizumab are already used in IBD. Trentadue et al. (38) describe a case of acute rejection after bowel transplant being successfully treated with vedolizumab. Fitzpatrick et al. (17) used single cell RNA sequencing to demonstrate transcriptionally distinct tissue resident T cell subtypes from the donor after bowel transplant. One of these sub-populations highly expressed β2-integrin, the study suggests this may be used to identify tissue resident CD8 cells. Anti-adhesion molecules may be useful in bowel transplant if future studies confirm a role for β2-integrins in the development of graft rejection.
Proteosome Inhibitors and Purine Analogs
Proteosome inhibition has been shown to reduce incidence of antibody mediated rejection (AMR) (39). AMR is a process of graft rejection driven by plasma cells (derived from B cells) which generate and release immunoglobulin antibodies. The proteosome complex exists in cells to degrade damaged proteins, proteosome inhibitor bortezomib inhibits chymotrypsin activity within the proteosome complex. Inhibition of the proteasome can induce apoptosis of plasma cells, and bortezomib is shown to be effective in clinical studies of AMR treatment post-renal transplant (40). There are case reports describing use bortezomib as a rescue treatment for AMR after bowel transplant (41). Purine analog MMF is already used for long term immunosuppression post-intestinal transplant due to it's ability to inhibit T cell proliferation. Vela et al. (42) described a rat model of intestinal transplant where purine analog fludarabine is used as an induction agent infused into the graft ex-vivo. Fudarabine is a purine analog with T cell toxicity currently used in chemotherapy for leukemia. The study concludes this treatment reduced donor cell chimerism, severity of GvHD and improved survival.
Microbiome Manipulation
The gut microbiome is extensively studied, including profiles in intestinal failure and subsequent gut adaptation alongside parenteral nutrition (43). No therapeutic microbiome strategies have yet been proposed for management of intestinal failure or transplant. Communication between the microbiome and the immune system can modulate immune responses, therefore, microbiome manipulation may have a therapeutic role in intestinal transplant management.
Hartman et al. (44) described that the microbiome in stoma effluent obtained post-small bowel transplant was similar to their control cohort (patients who had ileostomy for other reasons), with a predominance of lactobacilli and enterobacteria. This then reverted to “normal” (as compared to published normative data) after reconnection. Oh et al. (45) subsequently described the microbiota composition of ileal effluent associated with rejection episodes post-small bowel transplant. They demonstrated a different microbial community to Hartman, dominated by Streptococcus, Escherichia and Klebsiella (as well as Lactobacillus and Enterococcus described by Hartman), and that this significantly changed in episodes of acute rejection with expansion of the E.coli and Klebsiella species.
Weber et al. (46) undertook a prospective study to measure L-tryptophan metabolites of gut microbiota in urine as a predictor of intestinal GvHD in patients after stem cell transplant. They found that low levels of the metabolite 3-indoxyl sulfate was associated with poor outcomes, hypothesizing that the microbial signature associated with high urinary levels of 3-indoxyl sulfate is suggestive of a more diverse microbiome, and had a bacteriostatic effect on enterococci. The study also showed that higher, possibly protective, levels of metabolite were seen in patients treated with rifaximin for gut decontamination vs. ciprofloxin/metronidazole. Together, these studies suggest microbiome profiling may be developed for use as a marker of allograft rejection in the future.
The success of manipulation of the microbiome to prevent or treat rejection episodes is difficult to assess. Clinical studies have difficulty in demonstrating whether the dysbiosis associated with rejection is cause or consequence. Krams et al. (47) have studied a mouse model of small bowel transplant to demonstrate that TLR4 (Toll-like receptor 4, a cellular receptor known to interact with bacterial lipopolysaccharide to induce inflammatory responses) knock out mice have longer graft survival than their wild type controls. A rat model of intestinal transplant demonstrated a similar rejection-associated dysbiosis to the human studies, and showed that dietary fish oil can normalize this dysbiosis and improve tight junction integrity; potentially resulting in reduced inflammatory cell infiltrate into the graft (48).
There are case reports of the safety of fecal microbiota transplant (FMT) to treat refractory Clostridium difficile colitis in immunosuppressed renal and lung transplant patients (49). A recent multicenter retrospective review including a small number of bowel transplant patients receiving FMT for management of C. difficile colitis also demonstrated safety and efficacy but with a risk of CMV reactivation (50).
Whilst studies suggest that profiling the microbiome may be a useful non-invasive marker for rejection, manipulating it to prevent or treat rejection episodes is more difficult to assess, but offers potential for future therapies.
Tissue Engineering
The holy grail of transplant management is graft tolerance without the need for immunosuppression. Patient-derived grafts would be a way to achieve this. In the meantime, autologous immune cells have the potential to allow reduced immunosuppression by inducing tolerance.
CAR-T Cell Therapy
Chimeric Antigen Receptor (CAR) T cell therapy is a personalized T cell therapy that has been approved for use in the UK to treat leukemia. T cells are engineered for each patient individually to target cancer cells. Initial studies have been undertaken with autologous regulatory T cell therapy (using similar methodology to CAR T cell expansion) as a possible mechanism to induce tolerance post-liver transplant (51). The studies have described a safe protocol for ongoing research to explore personalized cell based immunomodulation in solid organ transplant.
Intestinal Organoids
Tissue engineering at an organ level is fast advancing. Intestinal organoids are derived from isolation of stem cells from intestinal crypts using ex-vivo intestinal tissue. The stem cells are then cultured in-vitro within a matrix and a mixture of growth factors to develop into 3D organoids representative of intestinal epithelium with villi, crypts, functional enterocytes, as well as goblet, Paneth and enteroendocrine cells (52).
Grant et al. have developed tissue engineered small intestine (TESI) derived from human and mouse organoids. These were then implanted into a mouse model where they demonstrated good cell differentiation and polarization within a crypt structure analogous to that of native tissue (53). They were also able to demonstrate expression of secretory and absorptive cell transporters, and ex-vivo functional brush border enzymes, suggesting the possibility to develop absorptive capacity. Workman et al. (54) have engineered human stem cell derived intestinal tissue with a functional enteric nervous system in vitro, using co-culture of epithelial organoids with neurospheres; potentially a future treatment for long-segment Hirschprung's disease.
Engineered intestinal grafts have been transplanted into rats and demonstrated functionality in vivo. Griksheit et al. undertook massive small bowel resection in their rat model and then transplanted tissue engineered small intestine. The transplanted rats showed more rapid post-operative weight gain and serum B12 levels compared to the control group (55). Meran et al. have described the use of patient intestinal mucosa biopsies to develop an organoid, which demonstrated subsequent cell proliferation with maintenance of an intestinal signature and seeding on to a human scaffold. Pig and mouse models were used to demonstrate functional capacity and in-vivo survival (56).
Summary
Progress in management of intestinal transplant with the novel therapies discussed offers the exciting prospect of improved graft tolerance and survival with less immunosuppressive drug side effects. With appropriate patient selection, we hope this allows intestinal transplant to be offered pre-emptively, with curative intent for irreversible intestinal failure, rather than after complications of long-term parenteral nutrition have already set in (Figure 1).
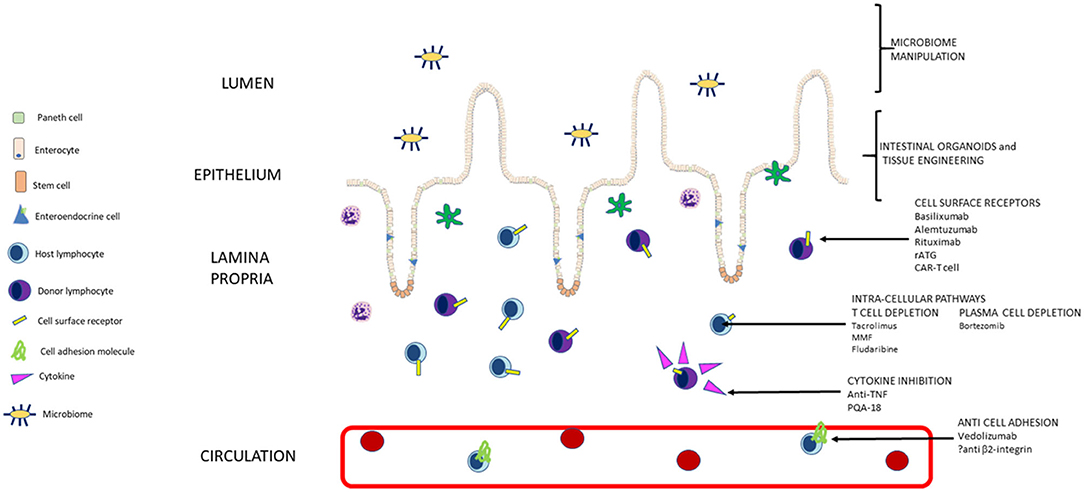
Figure 1. Summary of pathways targeted by current and new approaches to immunosuppression in intestinal transplant (not to scale).
Author Contributions
All authors listed have made a substantial, direct, and intellectual contribution to the work and approved it for publication.
Conflict of Interest
The authors declare that the research was conducted in the absence of any commercial or financial relationships that could be construed as a potential conflict of interest.
Publisher's Note
All claims expressed in this article are solely those of the authors and do not necessarily represent those of their affiliated organizations, or those of the publisher, the editors and the reviewers. Any product that may be evaluated in this article, or claim that may be made by its manufacturer, is not guaranteed or endorsed by the publisher.
References
1. NHS Blood Transplant. POL194/5 – Intestinal Transplantation : Patient Selection. Bristol (2021). Available online at: https://nhsbtdbe.blob.core.windows.net/umbraco-assets-corp/23344/pol194.pdf
2. Grant D, Wall W, Mimeault R, Zhong R, Ghent C, Garcia B, et al. Successful small-bowel/liver transplantation. Lancet. (1990) 335:181–4. doi: 10.1016/0140-6736(90)90275-A
3. Todo S, Reyes J, Furukawa H, Abu-Elmagd K, Lee RG, Tzakis A, et al. Outcome analysis of 71 clinical intestinal transplantations. Ann Surg. (1995) 222:270–82. doi: 10.1097/00000658-199509000-00006
4. Selvaggi G, Gaynor JJ, Moon J, Kato T, Thompson J, Nishida S, et al. Analysis of acute cellular rejection episodes in recipients of primary intestinal transplantation: a single center, 11-year experience. Am J Transplant. (2007) 7:1249–57. doi: 10.1111/j.1600-6143.2007.01755.x
5. Abu-Elmagd KM, Kosmach-Park B, Costa G, Zenati M, Martin L, Koritsky DA, et al. Long-term survival, nutritional autonomy, and quality of life after intestinal and multivisceral transplantation. Ann Surg. (2012) 256:494–508. doi: 10.1097/SLA.0b013e318265f310
6. Crispe IN, Giannandrea M, Klein I, John B, Sampson B WS. Cellular and molecular mechanisms of liver tolerance. Immunol Rev. (2006) 213:101–18. doi: 10.1111/j.1600-065X.2006.00435.x
7. Grant D, Abu-Elmagd K, Mazariegos G, Vianna R, Langnas A, Mangus R, et al. Intestinal transplant registry report: global activity and trends. Am J Transplant. (2015) 15:210–9. doi: 10.1111/ajt.12979
8. Kaufman SS, Avitzur Y, Beath S V, Ceulemans LJ, Gondolesi GE, Mazariegos GV, et al. Consensus in the year 2019. Transplantation. (2020) 104:937–46. doi: 10.1097/TP.0000000000003065
9. Ekong UD, Gupta NA, Urban R, Andrews WS. 20- to 25-year patient and graft survival following a single pediatric liver transplant—analysis of the United Network of organ sharing database: where to go from here. Pediatr Transplant. (2019) 23:1–10. doi: 10.1111/petr.13523
10. Raghu VK, Beaumont JL, Everly MJ, Venick RS, Lacaille FMG. Pediatric intestinal transplantation: analysis of the intestinal transplant registry. Pediatr Transpl. (2019) 23:e13580. doi: 10.1111/petr.13580
11. Jacobse J, Li J, Rings EHHM, Samsom JN, Goettel JA. Intestinal regulatory T Cells as specialized tissue-restricted immune cells in intestinal immune homeostasis and disease. Front Immunol. (2021) 12:1–23. doi: 10.3389/fimmu.2021.716499
12. Harrison OJ, Powrie FM. Regulatory T cells and immune tolerance in the intestine. Cold Spring Harb Perspect Biol. (2013) 5:1–17. doi: 10.1101/cshperspect.a018341
13. Sujino T, London M, Hoytema van Konijnenburg DP, Rendon T, Buch T, Silva HM, et al. Tissue adaptation of regulatory and intraepithelial CD4+ T cells controls gut inflammation. Science. (2016) 352:1581–6. doi: 10.1126/science.aaf3892
14. Stenard F, Nguyen C, Cox K, Kambham N, Umetsu DT, Krams SM, et al. Decreases in circulating CD4+CD25hiFOXP3+ cells and increases in intragraft FOXP3+ cells accompany allograft rejection in pediatric liver allograft recipients. Pediatr Transplant. (2009) 13:70–80. doi: 10.1111/j.1399-3046.2008.00917.x
15. Bartolome-Casado R, Landsverk OJB, Chauhan SK, Richter L, Phung D, Greiff V, et al. Resident memory CD8 T cells persist for years in human small intestine. J Exp Med. (2019) 216:2412–26. doi: 10.1084/jem.20190414
16. Snyder ME, Finlayson MO, Connors TJ, Dogra P, Bush E, Carpenter D, et al. Generation and persistence of human tissue-resident memory T cells in lung transplantation. Sci Immunol. (2019) 4, aav5581. doi: 10.1126/sciimmunol.aav5581
17. Fitzpatrick MEB, Provine NM, Garner LC, Soilleux E, Klenerman P, Allan PJ, et al. Article human intestinal tissue-resident memory T cells comprise transcriptionally and functionally distinct subsets ll Human intestinal tissue-resident memory T cells comprise transcriptionally and functionally distinct subsets. Cell Rep. (2021) 34:108661. doi: 10.1016/j.celrep.2020.108661
18. Zuber J, Shonts B, Lau S, Obradovic A, Yang S, Lambert M, et al. Bidirectional intragraft alloreactivity drives the repopulation of human intestinal allografts and correlates with clinical outcome. Sci Immunol. (2016) 1:1–22. doi: 10.1126/sciimmunol.aah3732
19. Zuber J, Rosen S, Shonts B, Sprangers B, Savage TM, Richman S, et al. Macrochimerism in intestinal transplantation: association with lower rejection rates and multivisceral transplants, without GVHD. Am J Transplant. (2015) 15:2691–703. doi: 10.1111/ajt.13325
20. US Department of Health and Human Services. Annual Report of the U.S. Organ Procurement and Transplantation Network and the Scientific Registry of Transplant Recipients. US Department of Health and Human Services, Health Resources and Services Administration, Health Care Systems Bur 2020. 2020 OPTN/SRTR annual report: transplant data 2020 (2020).
21. US Department of Health and Human Services. Annual report of the U.S. Organ Procurement and Transplantation Network and the Scientific Registry of Transplant Recipients. US Department of Health and Human Services, Health Resources and Services Administration, Health Care Systems Bur D of T. 2009 OPTN/SRTR Annual Report: transplant data 2009 (2009).
22. Fishbein TM, Florman S, Gondolesi G, Schiano T, Leleiko N, Tschernia A, et al. Intestinal transplantation before and after the introduction of sirolimus. Transplantation. (2002) 73:1538–42. doi: 10.1097/00007890-200205270-00004
23. Lauro A, Dazzi A, Ercolani G, Zanfi C, Golfieri L, Amaduzzi A, et al. Rejection episodes and 3-year graft survival under sirolimus and tacrolimus treatment after adult intestinal transplantationtle. Transpl Proc. (2007) 39:1629–31. doi: 10.1016/j.transproceed.2007.02.067
24. Goulet O, Sauvat F, Ruemmele F, Caldari D, Damotte D, Cezard JP, et al. Results of the Paris program: ten years of pediatric intestinal transplantation. Transplant Proc. (2005) 37:1667–70. doi: 10.1016/j.transproceed.2005.03.153
25. Devine K, Ranganathan S, Mazariegos G, Bond G, Soltys K, Ganoza A, et al. Induction regimens and post-transplantation lymphoproliferative disorder after pediatric intestinal transplantation: single-center experience. Pediatr Transplant. (2020) 24:1–8. doi: 10.1111/petr.13723
26. Vianna R, Farag A, Gaynor JJ, Selvaggi G, Tekin A, Garcia J, et al. Association of alemtuzumab induction with a significantly lower incidence of GVHD following intestinal transplantation: results of 445 consecutive cases from a single center. Transplantation. (2020) 104:2179–88. doi: 10.1097/TP.0000000000003111
27. Ceulemans LJ, Braza F, Monbaliu D, Jochmans I, De Hertogh G, Du Plessis J, et al. The Leuven immunomodulatory protocol promotes T-regulatory cells and substantially prolongs survival after first intestinal transplantation. Am J Transplant. (2016) 16:2973–85. doi: 10.1111/ajt.13815
28. Elsabbagh AM, Hawksworth J, Khan KM, Kaufman SS, Yazigi NA, Kroemer A, et al. Long-term survival in visceral transplant recipients in the new era: a single-center experience. Am J Transplant. (2019) 19:2077–91. doi: 10.1111/ajt.15269
29. Farmer DG, McDiarmid SV, Kuniyoshi J, Robert ME, Shaked A, Busuttil RW. Intragraft expression of messenger RNA for interleukin-6 and tumor necrosis factor-α is a predictor of rat small intestine transplant rejection. J Surg Res. (1994) 57:138–42. doi: 10.1006/jsre.1994.1121
30. Pech T, Finger T, Fujishiro J, Praktiknjo M, Ohsawa I, Abu-Elmagd K, et al. Perioperative infliximab application ameliorates acute rejection associated inflammation after intestinal transplantation. Am J Transplant. (2010) 10:2431–41. doi: 10.1111/j.1600-6143.2010.03279.x
31. Pech T, Fujishiro J, Finger T, Ohsawa I, Praktiknjo M, Abu-Elmagd K, et al. Combination therapy of tacrolimus and infliximab reduces inflammatory response and dysmotility in experimental small bowel transplantation in rats. Transplantation. (2012) 93:249–56. doi: 10.1097/TP.0b013e31823e7abb
32. De Greef E, Avitzur Y, Grant D, De-Angelis M, Ng V, Jones N, et al. Infliximab as salvage therapy in paediatric intestinal transplant with steroid- and thymoglobulin-resistant late acute rejection. J Pediatr Gastroenterol Nutr. (2012) 54:565–7. doi: 10.1097/MPG.0b013e3182293d73
33. Pascher A, Radke C, Dignass A, Schulz RJ, Veltzke-Schlieker W, Adler A, et al. Successful infliximab treatment of steroid and OKT3 refractory acute cellular rejection in two patients after intestinal transplantation. Transplantation. (2003) 76:615–8. doi: 10.1097/01.TP.0000072804.41125.82
34. Pascher A, Klupp J, Langrehr JM, Neuhaus P. Anti-TNF-alpha therapy for acute rejection in intestinal transplantation. Transplant Proc. (2005) 37:1635–6. doi: 10.1016/j.transproceed.2004.09.023
35. Gerlach UA, Koch M, Müller HP, Veltzke-Schlieker W, Neuhaus P, Pascher A. Tumor necrosis factor alpha inhibitors as immunomodulatory antirejection agents after intestinal transplantation. Am J Transplant. (2011) 11:1041–50. doi: 10.1111/j.1600-6143.2011.03497.x
36. Kroemer A, Belyayev L, Khan K, Loh K, Kang J, Duttargi A, et al. Rejection of intestinal allotransplants is driven by memory T helper type 17 immunity and responds to infliximab. Am J Transplant. (2021) 21:1238–54. doi: 10.1111/ajt.16283
37. Kodama T, Maeda A, Lo PC, Noguchi Y, Toyama C, Takama Y, et al. The effect of a novel immunosuppressive drug, a PAK-2 inhibitor, on macrophage differentiation/polarization in a rat small intestinal transplantation model. Transpl Immunol. (2019) 57:101246. doi: 10.1016/j.trim.2019.101246
38. Trentadue G, Kats-Ugurlu G, Blokzijl T, Diercks GFH, Haveman JW, Faber KN, et al. Safe and successful treatment of acute cellular rejection of an intestine and abdominal wall transplant with vedolizumab. Transplant Direct. (2020) 17:3–8. doi: 10.1097/TXD.0000000000000973
39. Woodle ES, Walsh RC, Alloway RR, Girnita A, Brailey P. Proteasome inhibitor therapy for antibody-mediated rejection. Pediatr Transplant. (2011) 15:548–56. doi: 10.1111/j.1399-3046.2011.01543.x
40. Flechner SM, Fatica R, Askar M, Stephany BR, Poggio E, Koo A, et al. The role of proteasome inhibition with bortezomib in the treatment of antibody-mediated rejection after kidney-only or kidney-combined organ transplantation. Transplantation. (2010) 90:1486–92. doi: 10.1097/TP.0b013e3181fdd9b0
41. Fujiwara S, Wada M, Kudo H, Yamaki S, Fujishima F, Ishida K, et al. Effectiveness of bortezomib in a patient with acute rejection associated with an elevation of donor-specific HLA antibodies after small-bowel transplantation: case report. Transplant Proc. (2016) 48:525–7. doi: 10.1016/j.transproceed.2015.09.073
42. Vela M, Stringa P, González-Navarro P, Machuca M, Pascual-Miguel B, Mestre C, et al. Donor's graft ex vivo T-cell depletion with fludarabine reduces graft-vs.-host disease signs and improves survival after intestinal transplantation – an experimental study. Transpl Int. (2020) 33:1302–11. doi: 10.1111/tri.13672
43. Neelis E, de Koning B, Rings E, Wijnen R, Nichols B, Hulst J, et al. The gut microbiome in patients with intestinal failure: current evidence and implications for clinical practice. J Parenter Enter Nutr. (2019) 43:194–205. doi: 10.1002/jpen.1423
44. Hartman AL, Lough DM, Barupal DK, Fiehn O, Fishbein T, Zasloff M, et al. Human gut microbiome adopts an alternative state following small bowel transplantation. Proc Natl Acad Sci USA. (2009) 106:17187–92. doi: 10.1073/pnas.0904847106
45. Oh PL, Martínez I, Sun Y, Walter J, Peterson DA, Mercer DF. Characterization of the ileal microbiota in rejecting and non-rejecting recipients of small bowel transplants. Am J Transplant. (2012) 12:753–62. doi: 10.1111/j.1600-6143.2011.03860.x
46. Weber D, Oefner PJ, Hiergeist A, Koestler J, Gessner A, Weber M, et al. Low urinary indoxyl sulfate levels early after transplantation reflect a disrupted microbiome and are associated with poor outcome. Blood. (2015) 126:1723–8. doi: 10.1182/blood-2015-04-638858
47. Krams SM, Wang M, Castillo RO, Ito T, Phillips L, Higgins J, et al. Toll-like receptor 4 contributes to small intestine allograft rejection. Transplantation. (2010) 90:1272–7. doi: 10.1097/TP.0b013e3181fdda0d
48. Li Q, Zhang Q, Wang C, Tang C, Zhang Y, Li N, et al. Fish oil enhances recovery of intestinal microbiota and epithelial integrity in chronic rejection of intestinal transplant. PLoS ONE. (2011) 6:e20460. doi: 10.1371/journal.pone.0020460
49. Friedman-Moraco RJ, Mehta AK, Lyon GM, Kraft CS. Fecal microbiota transplantation for refractory Clostridium difficile colitis in solid organ transplant recipients. Am J Transplant. (2014) 14:477–80. doi: 10.1111/ajt.12577
50. Cheng Y-W, Phelps E, Ganapini V, Khan N, Ouyang F, Xu H, et al. Fecal microbiota transplantation for the treatment of recurrent and severe Clostridium difficile infection in solid organ transplant recipients: a multicenter experience. Am J Transplant. (2019) 19:501–11. doi: 10.1111/ajt.15058
51. Sánchez-Fueyo A, Whitehouse G, Grageda N, Cramp ME, Lim TY, Romano M, et al. Applicability, safety, and biological activity of regulatory T cell therapy in liver transplantation. Am J Transplant. (2020) 20:1125–36. doi: 10.1111/ajt.15700
52. Spence JR, Mayhew CN, Rankin SA, Kuhar MF, Vallance JE, Tolle K, et al. Directed differentiation of human pluripotent stem cells into intestinal tissue in vitro. Nature. (2011) 470:105–10. doi: 10.1038/nature09691
53. Grant CN, Mojica SG, Sala FG, Ryan Hill J, Levin DE, Speer AL, et al. Human and mouse tissue-engineered small intestine both demonstrate digestive and absorptive function. Am J Physiol Gastrointest Liver Physiol. (2015) 308:G664–77. doi: 10.1152/ajpgi.00111.2014
54. Workman MJ, Mahe MM, Trisno S, Poling HM, Watson CL, Sundaram N, et al. Engineered human pluripotent-stem-cell-derived intestinal tissues with a functional enteric nervous system. Nat Med. (2017) 23:49–59. doi: 10.1038/nm.4233
55. Grikscheit TC, Siddique A, Ochoa ER, Srinivasan A, Alsberg E, Hodin RA, et al. Tissue-engineered small intestine improves recovery after massive small bowel resection. Ann Surg. (2004) 240:748–54. doi: 10.1097/01.sla.0000143246.07277.73
Keywords: intestinal failure, bowel transplant, parenteral nutrition, immunosuppression, tissue engineering
Citation: Dogra H and Hind J (2022) Innovations in Immunosuppression for Intestinal Transplantation. Front. Nutr. 9:869399. doi: 10.3389/fnut.2022.869399
Received: 04 February 2022; Accepted: 03 May 2022;
Published: 15 June 2022.
Edited by:
Jutta Koeglmeier, Great Ormond Street Hospital for Children NHS Foundation Trust, United KingdomReviewed by:
Lorenzo Norsa, Papa Giovanni XXIII Hospital, ItalyKadiyala Ravindra, Duke University, United States
Jeremy Mark Woodward, Cambridge University Hospitals NHS Foundation Trust, United Kingdom
Copyright © 2022 Dogra and Hind. This is an open-access article distributed under the terms of the Creative Commons Attribution License (CC BY). The use, distribution or reproduction in other forums is permitted, provided the original author(s) and the copyright owner(s) are credited and that the original publication in this journal is cited, in accordance with accepted academic practice. No use, distribution or reproduction is permitted which does not comply with these terms.
*Correspondence: Jonathan Hind, amhpbmQmI3gwMDA0MDtuaHMubmV0
†These authors have contributed equally to this work