- 1Laboratory of Plant Biochemistry, Institute of Biosciences, São Paulo State University (UNESP), Botucatu, Brazil
- 2Academic Department of Food, Faculty of Technological Sciences, National University of Agriculture, Catacamas, Honduras
- 3Department of Agronomy, Mato Grosso State University (UNEMAT), Nova Mutum, Brazil
- 4Plant Morphogenesis and Biochemistry Laboratory, Federal University of Santa Catarina (UFSC), Florianópolis, Brazil
- 5Department of Horticulture, School of Agriculture, São Paulo State University (UNESP), Botucatu, Brazil
- 6Department of Health Sciences, Universidade Alto Vale do Rio do Peixe (UNIARP), Caçador, Brazil
Tomatoes and their by-products are indisputable sources of substances with antioxidants properties. Several factors limit the production and influence the nutritional and antioxidant quality of tomato fruit. However, consumers can benefit from the effects of environmental factors, such as water and hydric stress, UV radiation, agronomic practices, among others, which lead to changes in the content of secondary metabolites in tomatoes. Molecules as phenolic compounds, carotenoids, and biogenic amines are often formed in response to environmental adversities. In this way, the consumption of tomato fruits or their by-products with higher levels of antioxidants may be important adjuvants in the prevention or reduction of diseases. In this mini-review, we will present how pre- and postharvest conditions may influence the content of some bioactive compounds in tomatoes. Furthermore, we will present how some heat processing methods may change the antioxidant content, as well as, the functional and nutritional properties of the final product.
Introduction
Tomatoes are widely used for food and beverages. The tomato crop is the largest vegetable crop in the world after potatoes and sweet potatoes. Tomatoes are an excellent source of nutrients and bioactive compounds important for human health, including phenolic compounds and carotenoids. Factors, including genetics, environmental conditions, ripeness, and postharvest conditions may influence the chemical composition and levels of phytochemicals (1).
Carotenoids, phenolic compounds and nitrogen-containing compounds are classified as non-nutritive phytochemicals. Carotenoids have a structure consisting of 40-carbon (C-40) isoprene units. Isoprenoids constitute a very representative group, which includes lycopene, β-carotene, g-carotene, z-carotene, among others (2) (Figure 1). Lycopene is responsible for the red color, and it has higher singlet oxygen quenching potential than β-carotene and α-tocopherol (3).
Phenolic compounds have one or more aromatic rings with one or more hydroxyl groups and during ripening, chlorogenic acid and quercetin have been described as major compounds in tomatoes. In ripened tomato fruit, naringenin chalcone was described as the major polyphenol (4), while caffeic acid is present in higher levels in red and yellow tomatoes (5). The content and profile of phenolic compounds are different in relation to tomato morphology, that is, phenolic acids are found in all tissues, while flavonoids and derivatives are described in the epidermis (6). The consumption of phenolic compounds is important for health, as they act as anti-atherogenic, anti-inflammatory, anti-allergenic, cardioprotective compounds (7) (Figure 2).
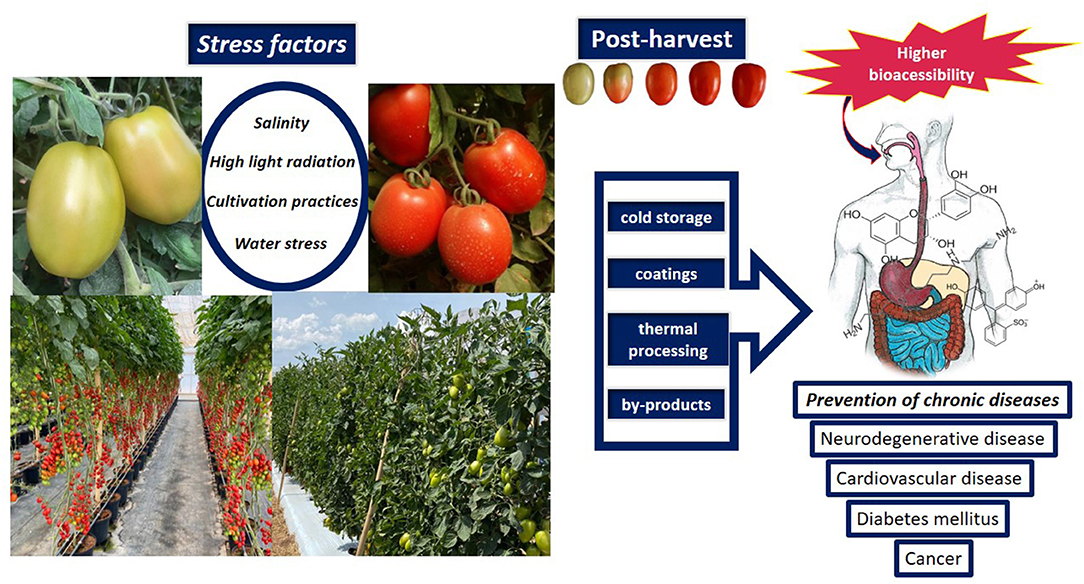
Figure 2. Influence of biotic and abiotic factors on the bioaccessibility and bioavailability of tomato bioactive compounds.
Biogenic amines (BAs) stand out for their antioxidant activity (Figure 1). Some Bas, as spermidine (Spd) and spermine (Spm), can play a protective role against different stresses and are related to the shelf life and quality of tomato fruits (8). Monoamines such as serotonin have also been detected in tomatoes and it was shown content ranging from 132.47 to 266.87 mg/kg, depending on the genotype (9). However, BAs in foods can also have negative impacts on health, especially due to histamine (His) and tyramine (Tyr) content. In tomatoes, His and Tyr may occur at levels ranging from not detected to 17.1 mg/kg and not detected to 6.38 mg/kg, respectively (10). Both His and Tyr were detected in cherry tomatoes but at levels below those considered harmful (His: 0.16–2.26 mg/kg; Tyr: 1.13–1.82 mg/kg) (11). Although other BAs, such as putrescine and cadaverine, do not have a direct toxic effect, they can potentiate the effects of tyramine and histamine, competing with detoxifying enzymes, in addition to being related to the formation of nitrosamines (12).
Preharvest Factors
The environment in which the crop grows, the relative humidity, temperature, photoperiod, irradiance, soil, and water in combination with seasonal changes play a definitive role in determining the content and the profile of phytochemicals (Figure 2). Preharvest factor may promote changes in the postharvest quality in tomatoes. The selection of tomato genotypes with higher nutritional and phytochemical quality can be obtained as a function of the genotype-environment interaction (13). In contrast, in “TY Megaton” and “Yureka,” there was a reduction of phenolic compounds at the vine- and postharvest-ripened red stages (14). Variations in the profile of phenolic compounds can also occur under vine- and postharvest-ripening conditions, as well as the concentration of some specific phenolic compounds are dependent on the cultivar (15). In hydroponically grown cherry tomatoes ripened from breaker stage there was a rapid decrease in the flavonoids content (55%) compared to on-vine red ripe (16). Furthermore, the level of antioxidant compounds is different depending on the stage of maturity. After the breaker stage, the lycopene content increases reaching maximum values at the ripe-red stage, depending on the cultivar (17).
UV radiation can influence the content of phytochemicals with high antioxidant potential. UV-B (280–315 nm) induces DNA damage and leads to the production of reactive oxygen species. As a defense, the plant may also trigger the production of antioxidant compounds (18). UV-B can alter the citric acid cycle, an important pathway that provides the substrate for the synthesis of phenolic compounds through the phenylpropanoid pathway (19). In greenhouses, UV-B is usually filtered through plastics (opaque to incident UV-B). UV exclusion under a polyvinylchloride (PVC) cover may be responsible for low levels of phenolic compounds in fruits produced in greenhouses (20). Tomatoes grown under a covering that allowed solar UV transmission accumulated more phenols than those grown under a covering material that excluded solar UV (21). In tomatoes grown with doses of supplemental radiation, there was a stimulation of rutin content. However, the use of UV-A or UV-A+B did not influence other phenolic compounds or carotenoids (22).
The temperature during cultivation is a key factor for the levels of some bio-actives. Temperatures during cultivation above 30°C and below 12°C may influence lycopene levels. At higher temperatures, inhibition of lycopene formation can occur and increase the synthesis of other carotenoids, changing the color of the fruits which become more yellow (21, 22). During tomato fruit ripening in high temperature conditions (>30°C) there is an increase in rutin and caffeic acid glucoside (23). In thermotolerant saladette-type tomatoes cultivated at high temperature and in protected cultivation (24), detected high levels of lycopene (up to 2.73 mg/100 g) and β-carotene (1.33 mg/100 g). The consumption of some genotypes may amount to 54.6 and 66.5% of the daily needs of lycopene and β-carotene, considering the minimum recommended (2 mg/day) (25, 26).
Variations in vapor pressure (VP) can affect the growth, flowering and quality of tomato fruits. Low VP (deficit) results in a decrease in growth, a consequence of increased transpiration, in addition to variations in the water potential of the stem, affecting the xylem influx. VP deficit may induce fruit cracking due to the variations in fruit growth and water influx and high VP reduce fruit cracking and promote blossom-end-rot (26). Water stress also directly influences the content of secondary metabolites. It is well-argued that climate change will contribute to increasing water scarcity, and available water resources will need to be used more efficiently. In water deficit, plants tend to present an increase in reactive oxygen species (ROS) and inactivation of enzymes. In water-stressed tomatoes, it was described a decrease in lycopene and β-carotene levels and an increase in chlorogenic acid (27).
Salt stress is currently one of the biggest problems. This occurs due to a lack of water, and it may be a cause of water deficit. In salinity tolerant tomato landraces, there may be an increase in the content of phenolic compounds and carotenoids, as there was an adaptation to the saline condition (28). In saline stress, the increase in the levels of some compounds is due to the osmoregulatory/osmo-protective effect, in addition to scavenging ROS or reactive nitrogen species (RNS) formed in response to the overproduction of free radicals. BAs can scavenge free radicals, acting as membrane protectors against lipid peroxidation and oxidative stress (29). Tomatoes supplied with 80 or 160 mM NaCl and harvested at the ripe stage showed an increase in serotonin (radical scavenging activity) with average of 6.4 μg/g f.w. (30). In response to water stress, Spd and Spm levels increase in un-grafted, grafted, and self-grafted tomato fruits (31). Depending on the genotype, the content ranged from 0.55 to 2.18 mg/g d.w. Spd and 0.18 to 0.95 mg/g d.w. Spm. In water stress-resistant cherry tomatoes, increase in Spd and Spm levels were related to drought tolerance (32). The increase in Spm may be related to the osmotic potential, stomatal opening and closing, or the stimulation of NO production as a way to mitigate stress (33). Spd is described by having protective effect against cancer and metabolic and neurodegenerative disorders (34) and it has been described as delaying aging in humans (35). It is a “caloric restriction mimetic,” inducing biochemical changes similar to caloric restrictions (34). Also, it competes with acetyl CoA for binding to the catalytic site of EP300, decreasing its activity (36) and demonstrating the anticoagulant action of Spd (37).
The content of Spd, Spm, His, and total BAs content were highest in organically grown tomatoes. Hydroponically cultivated cherry tomatoes showed higher levels of phenolic compounds (817.66 mg/kg), total BAs (415.31 mg/Kg), and BAs index (BAI) (applied to determine the loss in quality) (6.63) when compared to fruits from conventional cultivation (total BA = 409.28 mg/Kg and BAI = 1.20) and to organic cultivation (total BA = 186.62 mg/kg and BAI = 0.73) (11). Regardless of the type of cultivation, serotonin was the major BAs, ranging between 167.41 to 385.96 mg/Kg. In tomato ketchup, histamine levels are slightly elevated, i.e., 22 mg/kg (38). Comparatively, the levels of His (0.16 to 2.26 mg/kg) were below the levels considered harmful to humans (500 ppm) (39) or 25 to 50 mg of His per healthy person per meal, established using non-observed adverse effect level (NOAEL) (12). It is worth noting that food intake increases the bioavailability of His, as endogenous His is synthesized from L-histidine by enzyme-dependent histidine decarboxylation in mast cells and basophils, among others (40). When an immune or non-immune stimulus occurs, promoted by viruses or other pathogens, His is released in the body. Some studies suggest that the use of antihistamines may be useful in the treatment of patients with severe acute respiratory syndrome coronavirus 2 (SARS-CoV-2) (41). The levels of Tyr in tomatoes do not appear to be harmful to human health. Studies describe levels between 0.12 to 0.18 gm/100 g (42), while other describe contents ranging from not detected to 0.31 mg/100 g (43). For a safe diet, Tyr levels should not exceed 600 mg for healthy individuals. For those who are taking a monoamine oxidase inhibitor (MAOI), levels should not exceed 50 mg (MAOI—third generation) and 6 mg for people taking classical MAOI (10).
Postharvest Factors
Storage and Postharvest Treatments
Removal of field heat is important as it allows longer shelf life of tomato fruits (Figure 2), as well as storage at low temperatures (4 to 12oC) enhance shelf-life and maintains the nutritional quality (44). Frost/freezing and chilling injuries should be avoided as they promote damage, such as low lycopene (45) and high putrescine content (46), the formation of ice crystals and damage to cell integrity, blotchy coloration (dependent on temperature and exposure time), in addition to phenolic oxidation induced by the release of polyphenoloxidase of vacuole. Storage at low or room temperatures did not influence the serotonin content (30), while exogenous melatonin in tomatoes confer chilling tolerance (47) through proline activation and NO biosynthesis (47).
The edible chitosan coating on tomato fruits induces changes in the anthocyanins and flavonoids, in addition to modifying the antioxidant capacity (48). Tomatoes treated with different concentrations of gum Arabic used as an edible coating did not show an increase in storage life, but treatment with 10% gum Arabic was efficient in maintaining the levels of phenolic compounds, carotenoids, and antioxidant activity until the 10th day of storage at 20°C (49). Due to the content and diversity of bioactives in tomatoes, they have been used as adjuvants in edible coatings. Gelatin coatings incorporated with tomato oily extracts were efficient for preserving the phytochemicals in garambullo fruits (Myrtillocactus geometrizans). In addition, they delayed weight loss as well as changes in pH and soluble solids, among others (50).
Natural preservative agents can be a safe option against the harmful effects of chemical residues applied in postharvest. Exogenous melatonin in postharvest may inhibit the senescence and be related to anthocyanin accumulation in ripened tomato (51), besides from inducing the endogenous melatonin levels (49, 52) and phenolic compounds (52, 53).
Thermal Processing
Heat processing increases the bioavailability and bio-accessibility of carotenoids because, as already mentioned, there is a disintegration of the cell wall and organelle membranes where carotenoids are located (54). Heat denatures the protein-carotenoid complexes that limit the bio-accessibility of carotenoids, favoring their release from the food matrix (55). Thus, thermal processing has a direct effect on the profile and amount of carotenoids in tomatoes. The content of lycopene in raw tomatoes is ~2 mg of t-lycopene/g, and heating (88°C) can induce increases above 150% (52). An increase in cis-lycopene levels has also been described by the authors, although in a lower percentage and varying as a function of cooking time at temperatures below 100°C. At temperatures between 130 and 140°C, a decrease in t-lycopene content occur due to its degradation (53). It is noteworthy that in tomatoes, the bioavailability of lycopene in processed products is higher than in fresh (56).
Tomato By-Products
Agriculture is one of the biggest generators of waste, representing millions of tons of lost and wasted food resulting from the production and sales for consumption in natura (57). Every year, tomato production generates a considerable amount of vegetable by-products of no commercial value, resulting from several stages which include tomato field waste before harvest and postharvest and from home or industrial processes. It is currently unthinkable that such losses could occur, given the current and future needs of the human population and the finite resources our planet has. In this sense, the use of by-products is fundamental. This is especially true for tomatoes due to their nutritional and phytochemical importance. The residues could be used for the elaboration of new products, such as flours, or be used for the production of new polysaccharides with antioxidant and anticytotoxic activity and for production of biofilms (58).
For the flour, the ideal temperature vs. time must be used for the drying process so that the nutritional and phytochemical quality of the product is preserved. Using field waste and tomato fruit in the late stage of production, it was found that the best drying time and temperature was 55°C for 120 min using forced convection oven (59). The study also analyzed the lyophilized product. Authors detected 11.26 μg/mg of lycopene and 162.82 l μg/mg of phenolic compounds. In the flour, kaempferol and lycopene were the major compounds detected (1.09 and 11.26 μg/mg, respectively). Among the phenolic compounds, those that appeared at the highest levels were naringerin (90.04 μg/mg) from oven-dried and catechin (255.03 μg/mg) from lyophilized by-product. In dried tomato waste, (60) detected ellagic and chlorogenic acids (143.4 and 76.3 mg/kg), and lycopene (510.6 mg/kg) and β-carotene (95.6 mg/kg) were the most abundant phenolic compounds and carotenoids. Syringic acid has been described in tomato processing by-products. It has antimicrobial activity against Staphylococcus aureus (61).
Phytochemicals may be unstable in response to processing conditions such as high temperatures, acidity, oxidation, light, solubility, among others that are used and it is important to study ways to protect the extraction or incorporation of bio-actives in foods to avoid loss of functionality (62). Microencapsulation has shown positive effects for incorporation of bioactive by-products in food. The addition of lycopene in spices increases the antioxidant activity and improves the stability of the bioactive (63).
Conclusion
The beneficial effects of tomato and tomato-based products are closely related to the presence and abundance of various biologically active compounds. Agricultural practices may favor the accumulation of phytochemicals. Cultivation techniques, such as the selection of genotypes with target metabolites (e.g. high lycopene or serotonin content), temperature and vapor pressure control and water stress could be used for the production of tomatoes with superior quality and, consequently, for the development of derived products with superior amounts of beneficial compounds. Simple techniques (e.g., cooking) may facilitate the extraction and increase the bio-accessibility and bioavailability of compounds related to the reduction and/or prevention of metabolic and cardiovascular diseases and improve the functioning of the immune system. The study of management techniques in the pre- and postharvest of tomatoes, as well as the by-products generated during the productive system of the culture, becomes essential to guarantee for the population safe food with ideal quality for consumption, in addition to helping to avoid and/or reduce the frequency of food and health crises.
Author's Note
The mechanisms involved in tomato fruit quality have been extensively investigated by physiologists and geneticists, and the responses to climatic and cultural practices have been widely described. Yet, our ability to manage and improve fruit quality in a context of global change will rely on our capacity to integrate knowledge's and anticipate interactions among genotype, environment and cultural practices.
Author Contributions
GL and CB contributed to the conception, writing and editing of the manuscript. HG, SS, MM, and MT contributed to the writing. All authors contributed to the article and approved the submitted version.
Conflict of Interest
The authors declare that the research was conducted in the absence of any commercial or financial relationships that could be construed as a potential conflict of interest.
Publisher's Note
All claims expressed in this article are solely those of the authors and do not necessarily represent those of their affiliated organizations, or those of the publisher, the editors and the reviewers. Any product that may be evaluated in this article, or claim that may be made by its manufacturer, is not guaranteed or endorsed by the publisher.
Acknowledgments
GL, MM, and MT would to thank National Council for Scientific and Technological Development, Brazil (CNPq) (grants 307571/2019-0, 304657/2019-0 and 305724/2018-5) and PROPG UNESP (Edital 01/2022).
References
1. Coyago-Cruz E, Corell M, Moriana A, Hernanz D, Benítez-González AM, Stinco CM, et al. Antioxidants (carotenoids and phenolics) profile of cherry tomatoes as influenced by deficit irrigation, ripening and cluster. Food Chem. (2018) 240:870–84. doi: 10.1016/j.foodchem.2017.08.028
2. Salehi B, Sharifi-Rad R, Sharopov F, Namiesnik J, Roointan A, Kamle M, et al. Beneficial effects and potential risks of tomato consumption for human health: an overview. Nutrition. (2019) 62:201–8. doi: 10.1016/j.nut.2019.01.012
3. Chaudhary P, Sharma A, Singh B, Nagpal AK. Bioactivities of phytochemicals present in tomato. J Food Sci Technol. (2018) 55:2833–49. doi: 10.1007/s13197-018-3221-z
4. Martí R, Roselló S, Cebolla-Cornejo J. Tomato as a source of carotenoids and polyphenols targeted to cancer prevention. Cancers (Basel). (2016) 8:1–28. doi: 10.3390/cancers8060058
5. Georgé S, Tourniaire F, Gautier H, Goupy P, Rock E, Caris-Veyrat C. Changes in the contents of carotenoids, phenolic compounds and vitamin C during technical processing and lyophilisation of red and yellow tomatoes. Food Chem. (2011) 124:1603–11. doi: 10.1016/j.foodchem.2010.08.024
6. Moco S, Capanoglu E, Tikunov Y, Bino RJ, Boyacioglu D, Hall RD, et al. Tissue specialization at the metabolite level is perceived during the development of tomato fruit. J Exp Bot. (2007) 58:4131–46. doi: 10.1093/jxb/erm271
7. Shahidi F, Varatharajan V, Oh WY, Peng H. Phenolic compounds in agri-food by-products, their bioavailability and health effects. J Food Bioact. (2019) 5:57–119. doi: 10.31665/JFB.2019.5178
8. Kamiab F, Tavassolian I, Hosseinifarahi M. Biologia futura: the role of polyamine in plant science. Biol Futur. (2020) 71:183–94. doi: 10.1007/s42977-020-00027-3
9. Ibrahim AMH, Quick JS, Kaya R, Grandgirard J, Poinsot D, Krespi L, et al. Evaluation of spring wheat genotypes for heat tolerance using cell membrane thermostability. Crop Pasture Sci. (2017) 2:291–6. doi: 10.1501/Tarimbil
10. Sánchez-Pérez S, Comas-Basté O, Rabell-González J, Veciana-Nogués MT, Latorre-Moratalla ML, Vidal-Carou MC. Biogenic amines in plant-origin foods: are they frequently underestimated in low-histamine diets? Foods. (2018) 7:1–17. doi: 10.3390/foods7120205
11. Rapa M, Ciano S, Ruggieri R, Vinci G. Bioactive compounds in cherry tomatoes (Solanum Lycopersicum var. Cerasiforme): cultivation techniques classification by multivariate analysis. Food Chem. (2021) 355:1–7. doi: 10.1016/j.foodchem.2021.129630
12. EFSA. Scientific Opinion on risk based control of biogenic amine formation in fermented foods. EFSA J. (2011) 9:2393–486. doi: 10.2903/j.efsa.2011.2393
13. Asensio E, Sanvicente I, Mallor C, Menal-Puey S. Spanish traditional tomato. Effects of genotype, location and agronomic conditions on the nutritional quality and evaluation of consumer preferences. Food Chem. (2019) 270:452–8. doi: 10.1016/j.foodchem.2018.07.131
14. Tilahun S, Do SP, Mu HS, Cheon SJ. Review on factors affecting the quality and antioxidant properties of tomatoes. Afr J Biotechnol. (2017) 16:1678–87. doi: 10.5897/AJB2017.16054
15. Tilahun S, Park DS, Taye AM, Jeong CS. Effect of ripening conditions on the physicochemical and antioxidant properties of tomato (Lycopersicon esculentum Mill). Food Sci Biotechnol. (2017) 26:473–9. doi: 10.1007/s10068-017-0065-7
16. Tsakiri S, Sofia T, Nifakos K, Tsaniklidis G, Vakros J, Delis C, et al. Comparison of on-vine and post-harvest ripening on antioxidant compounds and antioxidant activities of hydroponically grown cherry tomatoes. Eur J Horotultural Sci. (2020) 85:422–9. doi: 10.17660/eJHS.2020/85.6.6
17. Tilahun S, Park DS, Solomon T, Choi HR, Jeong CS. Maturity stages affect nutritional quality and storability of tomato cultivars. CYTA - J Food. (2019) 17:87–95. doi: 10.1080/19476337.2018.1554705
18. Huché-Thélier L, Crespel L, Gourrierec J. Le, Morel P, Sakr S, Leduc N. Light signaling and plant responses to blue and UV radiations-Perspectives for applications in horticulture. Environ Exp Bot. (2016) 121:22–38. doi: 10.1016/j.envexpbot.2015.06.009
19. Cavalcanti JHF, Esteves-Ferreira AA, Quinhones CGS, Pereira-Lima IA, Nunes-Nesi A, Fernie AR, et al. Evolution and functional implications of the tricarboxylic acid cycle as revealed by phylogenetic analysis. Genome Biol Evol. (2014) 6:2830–48. doi: 10.1093/gbe/evu221
20. Patanè C, Malvuccio A, Saita A, Rizzarelli P, Siracusa L, Rizzo V, et al. Nutritional changes during storage in fresh-cut long storage tomato as affected by biocompostable polylactide and cellulose based packaging. Lwt. (2019) 101:618–24. doi: 10.1016/j.lwt.2018.11.069
21. Luthria DL, Mukhopadhyay S, Krizek DT. Content of total phenolics and phenolic acids in tomato (Lycopersicon esculentum Mill) fruits as influenced by cultivar and solar UV radiation. J Food Compos Anal. (2006) 19:771–7. doi: 10.1016/j.jfca.2006.04.005
22. Dzakovich MP, Ferruzzi MG, Mitchell CA. Manipulating sensory and phytochemical profiles of greenhouse tomatoes using environmentally relevant doses of ultraviolet radiation. J Agric Food Chem. (2016) 64:6801–8. doi: 10.1021/acs.jafc.6b02983
23. Gautier H, Diakou-Verdin V, Bénard C, Reich M, Buret M, Bourgaud F, et al. How does tomato quality (sugar, acid, and nutritional quality) vary with ripening stage, temperature, and irradiance? J Agric Food Chem. (2008) 56:1241–50. doi: 10.1021/jf072196t
24. Junior SS, Casagrande JG, Toledo CA de L, Ponce F da S, Ferreira F da S, Zanuzo MR, et al. Selection of thermotolerant Italian tomato cultivars with high fruit yield and nutritional quality for the consumer taste grown under protected cultivation. Sci Hortic (Amsterdam). (2022) 291:110559. doi: 10.1016/j.scienta.2021.110559
25. Stinco CM, Pumilia G, Giuffrida D, Dugo G, Meléndez-Martínez AJ, Vicario IM. Bioaccessibility of carotenoids, vitamin A and α-tocopherol, from commercial milk-fruit juice beverages: contribution to the recommended daily intake. J Food Compos Anal. (2019) 78:24–32. doi: 10.1016/j.jfca.2019.01.019
26. Bertin N, Génard M. Tomato quality as influenced by preharvest factors. Sci Hortic (Amsterdam). (2018) 233:264–76. doi: 10.1016/j.scienta.2018.01.056
27. Atkinson NJ, Dew TP, Orfila C, Urwin PE. Influence of combined biotic and abiotic stress on nutritional quality parameters in tomato (Solanum lycopersicum). J Agric Food Chem. (2011) 59:9673–82. doi: 10.1021/jf202081t
28. Sumalan RM, Ciulca SI, Poiana MA, Moigradean D, Radulov I, Negrea M, et al. The antioxidant profile evaluation of some tomato landraces with soil salinity tolerance correlated with high nutraceuticaland functional value. Agronomy. (2020) 10:500. doi: 10.3390/agronomy10040500
29. Toscano S, Trivellini A, Cocetta G, Bulgari R, Francini A, Romano D, et al. Effect of preharvest abiotic stresses on the accumulation of bioactive compounds in horticultural produce. Front Plant Sci. (2019) 10:1212. doi: 10.3389/fpls.2019.01212
30. Hano S, Shibuya T, Imoto N, Ito A, Imanishi S, Aso H, et al. Serotonin content in fresh and processed tomatoes and its accumulation during fruit development. Sci Hortic (Amsterdam). (2017) 214:107–13. doi: 10.1016/j.scienta.2016.11.009
31. Sánchez-Rodríguez E, Romero L, Ruiz JM. Accumulation of free polyamines enhances the antioxidant response in fruits of grafted tomato plants under water stress. J Plant Physiol. (2016) 190:72–8. doi: 10.1016/j.jplph.2015.10.010
32. Montesinos-Pereira D, Barrameda-Medina Y, Romero L, Ruiz JM, Sánchez-Rodríguez E. Genotype differences in the metabolism of proline and polyamines under moderate drought in tomato plants. Plant Biol. (2014) 16:1050–7. doi: 10.1111/plb.12178
33. Hasan MM, Skalicky M, Jahan MS, Hossain MN, Anwar Z, Nie Z, et al. Spermine: its emerging role in regulating drought stress responses in plants. Cells. (2021) 10:1–15. doi: 10.3390/cells10020261
34. Madeo F, Pietrocola F, Eisenberg T, Kroemer G. Caloric restriction mimetics: towards a molecular definition. Nat Rev Drug Discov. (2014) 13:727–40. doi: 10.1038/nrd4391
35. Yang X, Zhang M, Dai Y, Sun Y, Aman Y, Xu Y, et al. Spermidine inhibits neurodegeneration and delays aging via the PINK1-PDR1-dependent mitophagy pathway in C. elegans Aging (Albany NY). (2020) 12:16852–66. doi: 10.18632/aging.103578
36. Pietrocola F, Lachkar S, Enot DP, Niso-Santano M, Bravo-San Pedro JM, Sica V, et al. Spermidine induces autophagy by inhibiting the acetyltransferase EP300. Cell Death Differ. (2015) 22:509–16. doi: 10.1038/cdd.2014.215
37. Madeo F, Carmona-Gutierrez D, Kepp O, Kroemer G. Spermidine delays aging in humans. Aging. (2018) 10:2209–11. https://www.ncbi.nlm.nih.gov/pmc/articles/PMC6128428/
38. Maintz L, Novak N. Histamine and histamine intolerance. Am J Clin Nutr. (2007) 85:1185–96. doi: 10.1093/ajcn/85.5.1185
39. Papageorgiou M, Lambropoulou D, Morrison C, Kłodzińska E, Namieśnik J, Płotka-Wasylka J. Literature update of analytical methods for biogenic amines determination in food and beverages. TrAC Trends Anal Chem. (2018) 98:128–42. doi: 10.1016/j.trac.2017.11.001
40. Khan MA, Khan ZA, Naeem A, Naqvi N, Srivast S, Bhargava A, et al. Effect of Dietary Modification for Targeting Histamine Activity in Patients of Allergic Rhinitis: a Randomised Open Label Study. Lucknow: Research Square (2020). p. 1–17. doi: 10.21203/rs.3.rs-25717/v1
41. Ennis M, Tiligada K. Histamine receptors and COVID-19. Inflamm Res. (2021) 70:67–75. doi: 10.1007/s00011-020-01422-1
42. Pinho L de, Almeida AC, Costa CA, Paes MCD, Glória MBA, Souza RM. Nutritional properties of cherry tomatoes harvested at different times and grown in an organic cropping. Hortic Bras. (2011) 29:205–11. doi: 10.1590/S0102-05362011000200012
43. Dala-Paula BM, Starling M de F V, Gloria MBA. Vegetables consumed in Brazilian cuisine as sources of bioactive amines. Food Biosci. (2021) 40:100856. doi: 10.1016/j.fbio.2020.100856
44. Chomchalow S, El Assi NM, Sargent SA, Brecht JK. Fruit maturity and timing of ethylene treatment affect storage performance of green tomatoes at chilling and nonchilling temperatures. Horttechnology. (2002) 12:271–83. doi: 10.21273/HORTTECH.12.1.104
45. Rai A, Kumari K, Vashistha P. Umbrella review on chilling injuries: post-harvest issue, cause, and treatment in tomato. Sci Hortic (Amsterdam). (2022) 293:110710. doi: 10.1016/j.scienta.2021.110710
46. Tsaniklidis G, Charova SN, Fanourakis D, Tsafouros A, Nikoloudakis N, Goumenaki E, et al. The role of temperature in mediating postharvest polyamine homeostasis in tomato fruit. Postharvest Biol Technol. (2021) 179:111586. doi: 10.1016/j.postharvbio.2021.111586
47. Jannatizadeh A, Aghdam MS, Luo Z, Razavi F. Impact of exogenous melatonin application on chilling injury in tomato fruits during cold storage. Food Bioprocess Technol. (2019) 12:741–50. doi: 10.1007/s11947-019-2247-1
48. Ortega-Ortiz H, Benavides-Mendoza A, Mendoza-Villarreal R, Ramírez-rodríguez H, Romenus KDA. Enzymatic activity in tomato fruits as a response to chemical elicitors. J Mex Chem Soc. (2007) 51:141–4. https://www.redalyc.org/pdf/475/47551303.pdf
49. Ali A, Maqbool M, Alderson PG, Zahid N. Effect of gum arabic as an edible coating on antioxidant capacity of tomato (Solanum lycopersicum L.) fruit during storage. Postharvest Biol Technol. (2013) 76:119–24. doi: 10.1016/j.postharvbio.2012.09.011
50. López-Palestina CU, Aguirre-Mancilla CL, Raya-Pérez JC, Ramírez-Pimentel JG, Gutiérrez-Tlahque J, Hernández-Fuentes AD. The effect of an edible coating with tomato oily extract on the physicochemical and antioxidant properties of garambullo (Myrtillocactus geometrizans) fruits. Agronomy. (2018) 8:1–14. doi: 10.3390/agronomy8110248
51. Sun Q, Zhang N, Wang J, Cao Y, Li X, Zhang H, et al. A label-free differential proteomics analysis reveals the effect of melatonin on promoting fruit ripening and anthocyanin accumulation upon postharvest in tomato. J Pineal Res. (2016) 61:138–53. doi: 10.1111/jpi.12315
52. Dewanto V, Xianzhong W, Adom KK, Liu RH. Thermal processing enhances the nutritional value of tomatoes by increasing total antioxidant activity. J Agric Food Chem. (2002) 50:3010–4. doi: 10.1021/jf0115589
53. Colle I, Van Buggenhout S, Van Loey A, Hendrickx M. High pressure homogenization followed by thermal processing of tomato pulp: Influence on microstructure and lycopene in vitro bioaccessibility. Food Res Int. (2010) 43:2193–200. doi: 10.1016/j.foodres.2010.07.029
54. Cilla A, Bosch L, Barberá R, Alegría A. Effect of processing on the bioaccessibility of bioactive compounds – A review focusing on carotenoids, minerals, ascorbic acid, tocopherols and polyphenols. J Food Compos Anal. (2018) 68:3–15. doi: 10.1016/j.jfca.2017.01.009
55. Colle I, Lemmens L, Van Buggenhout S, Van Loey A, Hendrickx M. Effect of thermal processing on the degradation, isomerization, and bioaccessibility of lycopene in tomato pulp. J Food Sci. (2010) 75:C753–9. doi: 10.1111/j.1750-3841.2010.01862.x
56. Shi J, Le Maguer M. Lycopene in tomatoes: Chemical and physical properties affected by food processing. Crit Rev Biotechnol. (2000) 20:293–334. doi: 10.1080/07388550091144212
57. Dahiya S, Kumar AN, Shanthi Sravan J, Chatterjee S, Sarkar O, Mohan SV. Food waste biorefinery: sustainable strategy for circular bioeconomy. Bioresour Technol. (2018) 248:2–12. doi: 10.1016/j.biortech.2017.07.176
58. Tommonaro G, Poli A, De Rosa S, Nicolaus B. Tomato derived polysaccharides for biotechnological applications: chemical and biological approaches. Molecules. (2008) 13:1384–98. doi: 10.3390/molecules13061384
59. Paulino SLJ, Adrián ÁTG, Gabriela EAL, Maribel VM, Sergio MG. Nutraceutical potential of flours from tomato by-product and tomato field waste. J Food Sci Technol. (2020) 57:3525–31. doi: 10.1007/s13197-020-04585-1
60. Nour V, Panaite TD, Ropota M, Turcu R, Trandafir I, Corbu AR. Nutritional and bioactive compounds in dried tomato processing waste. CYTA J Food. (2018) 16:222–9. doi: 10.1080/19476337.2017.1383514
61. Szabo K, Dulf FV, Diaconeasa Z, Vodnar DC. Antimicrobial and antioxidant properties of tomato processing byproducts and their correlation with the biochemical composition. LWT. (2019) 116:108558. doi: 10.1016/j.lwt.2019.108558
62. Araújo-Rodrigues H, Santos D, Campos DA, Ratinho M, Rodrigues IM, Pintado ME. Development of frozen pulps and powders from carrot and tomato by-products: Impact of processing and storage time on bioactive and biological properties. Horticulturae. (2021) 7:185. doi: 10.3390/horticulturae7070185
Keywords: carotenoids, phenolic compounds, biogenic amines, postharvest, waste, by-products
Citation: Lima GPP, Gómez HAG, Seabra Junior S, Maraschin M, Tecchio MA and Borges CV (2022) Functional and Nutraceutical Compounds of Tomatoes as Affected by Agronomic Practices, Postharvest Management, and Processing Methods: A Mini Review. Front. Nutr. 9:868492. doi: 10.3389/fnut.2022.868492
Received: 02 February 2022; Accepted: 08 March 2022;
Published: 06 April 2022.
Edited by:
José Pinela, Polytechnic Institute of Bragança (IPB), PortugalReviewed by:
Shimeles Tilahun, Kangwon National University, South KoreaLászló Csambalik, Hungarian University of Agricultural and Life Sciences, Hungary
Copyright © 2022 Lima, Gómez, Seabra Junior, Maraschin, Tecchio and Borges. This is an open-access article distributed under the terms of the Creative Commons Attribution License (CC BY). The use, distribution or reproduction in other forums is permitted, provided the original author(s) and the copyright owner(s) are credited and that the original publication in this journal is cited, in accordance with accepted academic practice. No use, distribution or reproduction is permitted which does not comply with these terms.
*Correspondence: Giuseppina Pace Pereira Lima, pace.lima@unesp.br