- 1School of Life Sciences, Shaoxing University, Shaoxing, China
- 2National Engineering Research Center for Cereal Fermentation and Food Biomanufacturing, Jiangnan University, Wuxi, China
Pueraria lobata, an edible food and medicinal plant, is a rich source of bioactive components. In this study, a polyphenol-rich extract was isolated from P. lobata. Puerarin was identified, and the high antioxidant bioactivity of the P. lobata extract was evaluated using the methods of 2,2-diphenyl-1-picrylhydrazyl (DPPH), 2,2′-azinobis-(3-ethylbenzthiazoline-6-sulphonate) (ABTS), and hydroxyl free radical scavenging ratio. Additionally, the IC50 values of DPPH, ABTS, and hydroxyl radical scavenging activities were 50.8, 13.9, and 100.4 μg/ml, respectively. Then, the P. lobata extract was administered to C57Bl/6J mice and confirmed to have a superior effect on enhancing the antioxidant status including improving superoxide dismutase activity, glutathione peroxidase peroxide activity, total antioxidant capacity activity, and malondialdehyde contents in vivo. Furthermore, the P. lobata extract had beneficial and prebiotic effects on the composition and structure of gut microbiota. Results showed that the P. lobata extract significantly increased the abundance of beneficial bacteria, involving Lactobacillaceae and Bacteroidetes, and decreased the abundance of Ruminococcaceae, Prevotellaceae, and Burkholderiaceae. Overall, our results provided a basis for using the P. lobata extract as a promising and potential functional ingredient for the food industry.
Introduction
Pueraria is a typical representative genus comprising over 20 species of herbs, which are highly found in China, Japan, and Korea. Among them, kudzu [Pueraria montana var. lobata (Willd.)] was first documented in a Chinese Materia Medica named Shen Nong Ben Cao Jing written in the Western Han Dynasty, and its medical properties have a long history of wide application in traditional Chinese medicine, as well as being developed as a royal special food for edible uses in Japanese cuisine (1, 2). Pueraria lobata has also been utilized to manage cardiovascular diseases, alcohol-induced liver injury, and many chronic diseases. Likewise, being an herbal, foodstuff could be widely used in nutritious foods and dietary supplements. According to preliminary statistics, the total area of cultivated and wild P. lobata in China is 400,000 hectares, with an annual production of more than 1.5 million tons. In the future decade, the global demand for kudzu is estimated to increase sharply to 50 million tons per year.
Polyphenols derived from food and medicinal plants have been known to prevent many chronic diseases, such as diabetes, atherosclerosis, and obesity (3). The major constituents of kudzu (the root of P. lobata) were high levels of starch, but recent studies showed that the extracts of P. lobata contained rich polyphenols, such as puerarin, genistin, daidzin, and daidzein. The high levels of polyphenolic compounds in P. lobata have recently attracted much attention due to the functional properties of lowering cholesterol, inhibiting cancer cell proliferation and tumor growth, and modulating the immune system (4). Some studies focus on the protective effect of puerarin on alcoholic liver disease, for instance, reducing the production of free radicals, improving the activities of antioxidant enzymes, stimulating AMPK and Nrf2 signaling pathways, inhibiting the mTOR, regulating the activities of ethanol dehydrogenase and aldehyde dehydrogenase, as a result of keeping the balance of cellular redox state, and promoting liver energy metabolism dysfunction. In the process of oxidative stress on the diet-induced obesity model, daidzein in P. lobata is associated with reduction of apoptosis and stearoyl-CoA desaturase 1, which is a key enzyme relative to obesity (5). Furthermore, P. lobata has been demonstrated to possess the capability of regulating inflammatory factors and pathways, resulting in exhibiting potential anti-inflammatory activity. Some studies also found that the biological properties of antioxidant and antimicrobial activities displayed by the P. lobata extract were attributed to the existence of phenolic compounds (6, 7).
The gut microbiota is a microbial community in the intestinal with over 100 trillion microorganisms, which is the largest and most complex ecosystem in the human body, and has been shown the participation in the body’s nutrient metabolism, immune system, and overall health of the host (8). A healthy gut, as a result of stable gut microbiota and diverse ecosystem, played an active influence in regulating metabolic pathways and resisting pathogen invasion (9). In contrast, if the gut ecosystem was broken and suffered from an imbalance of gut microbiota, a great deal of host health problems would be induced ceaselessly, including obesity, metabolic syndrome, diabetes, and intestinal bowel disease (10–12). In recent studies, the gut microbiota has been widely considered as a key role in the modulation of transformation of nutrients and compounds in the dietary supplements. Likewise, bioactive components in functional foods give an effect on gut microbiota. In fact, a novel potential therapy, based on altering the gut microbiota, aroused worldwide concern, especially in the treatment of preventing metabolic disorders (13). The abundance and diversity of gut microbiota could be examined using next-generation sequencing analysis of 16S rRNA amplicons to get knowledge about the profile of microbial characterization influenced by bioactive components. Liu et al. found that the promotion of Bacteroides vulgatus, Parabacteroides distasonis, Prevotella, and Bifidobacterium is a master regulator of anti-obesity properties (14). Additionally, the abundance of the Prevotella and Akkermansia genus could be increased by the flavan-3-ols, which was reported to be reversely linked to body weight gain and adipose tissue inflammation. Therefore, it is notable that the presence of specific gut bacterial species would participate in the improvement of the healthy gut ecosystem and cellular antioxidant state.
Pervious study suggested that P. lobata extract was closely related to antioxidant, antiproliferative, and anti-inflammation effects via cell models (15). However, few studies paid attention to elucidate the relationship and mechanism of the effect of P. lobata extract on the antioxidant activities and the composition of gut microbiota. In addition, there is a need to characterize a functional P. lobata extract and demonstrate its bioactive properties in vitro and in vivo. Therefore, in this study, we had a polyphenol-rich P. lobata extract, investigated the antioxidant in vitro and in vivo, as well as profiled the specific gut microbiota modulated by the polyphenol-rich extract. The health benefits of P. lobata extract could be better understood according to the direct comparison of microbial composition and structure via treated by P. lobata extract.
Materials and Methods
Materials and Chemicals
Pueraria lobata was purchased from a local market (Hangzhou, China). Then, the fruiting bodies of P. lobata root were grounded into powder and stored at a low-temperature refrigerator before being used. The antioxidant indicator kits of superoxide dismutase activities (SOD), glutathione peroxidase peroxide (GSH-Px) activities, total antioxidant capacity (T-AOC) activities, and malondialdehyde (MDA) contents were obtained from the Nanjing Jiancheng Bioengineering Institute (Nanjing, China). The other chemicals and solvents were of liquid chromatography grade and purchased from Fisher Scientific or Beijing Solarbio Science & Technology Limited Company (Beijing, China).
Polyphenol-Rich Extract
The extraction method of P. lobata root was conducted according to a previous study with some modifications (16). In brief, P. lobata powder (10 g) was mixed with chilled acetone (200 ml) with ultra-sonicated for 30 min and then centrifuged for 20 min with the speed of 14,000 × g at 4°C. Then, the supernatant was removed, and the remaining pellet was re-extracted with another chilled acetone (200 ml). The pooled extracts were evaporated to dryness at 35°C. The macroporous resin column was used for the preliminary purification. The solution was then reconstituted in the solution of methanol, dried, and stored at −20°C before the test. The residue in this study referred to the P. lobata extract.
Determination of Total Phenolic Content
The total phenolic content of the P. lobata extract was measured based on the method described previously with some minor modifications (17). The P. lobata extract was dissolved in methanol to a concentration of 0.25 mg/ml. Gallic acid standards or the samples (20 μL) and diluted Folin–Ciocalteu reagent were incubated at room temperature for 1 min, followed by terminating reaction with sodium carbonate (75 mg/ml). Molecular Devices SpectraMax microplate spectrophotometer (San Jose, CA, United States) was used to record the absorbance at 760 nm. Total phenolic contents were expressed as μmol gallic acid equivalents/mg polyphenol-rich P. lobata extract.
High-Performance Liquid Chromatography Analysis
The chromatographic analysis of the P. lobata extract was performed using a high-performance liquid chromatography (HPLC) system (Waters, Milford, MA, United States) (18, 19). The mobile phases were prepared as follows: water with 0.1% formic acid and acetonitrile with 0.1% formic acid. The gradient procedure was as follows: 0–3 min, 5% B, 3–42 min, 5%–35% B, 42–46 min, 35%–90% B, 46–50 min, 90% B, 50–56 min, 90%–5% B. The column was maintained at 30°C. The flow rate was set at 0.8 ml/min. The P. lobata extract was separated using an Agilent ZORBAX Eclipse Plus C18 (250 mm × 4.6 mm, 5.0 μm particle size). The detection of the P. lobata extract was recorded using an ultraviolet detector at 280 nm wavelength.
Antioxidant Activities
2,2-Diphenyl-1-Picrylhydrazyl Free Radical Scavenging Activity
The 2,2-diphenyl-1-picrylhydrazyl (DPPH) assay solution was performed according to a previous study prepared by mixing samples or blank solution (20 μl) with 50% ethanol solution containing DPPH solution (180 μl) (20). The mixture was incubated at room temperature for 60 min in the dark. The absorbance was measured at 517 nm. The results were described using the DPPH scavenging ratio, which was calculated as follows:
Acontrol is the absorbance measured for blank, and Asample is the absorbance measured for the P. lobata extract. Ascorbic acid (vitamin C) was selected as the positive control.
2,2′-Azinobis-(3-Ethylbenzthiazoline-6-Sulphonate) Free Radical Scavenging Activity
The 2,2′-azinobis-(3-ethylbenzthiazoline-6-sulphonate) (ABTS) scavenging activity was measured based on the method studied described previously with minor modification (21). ABTS⋅ + was generated by mixing 7 mM of ABTS solution with a 2.45 mM of the aqueous solution of K2S2O8 and reacting for 12 h at room temperature in the dark. The ABTS⋅ + reaction solution (100 μl) with an absorbance of 0.70 cm–1 at 734 nm was used, and 100 μl of samples or blank solution was mixed and reacted for 60 min in the dark. The absorbance was recorded at 734 nm. The results were shown by the ABTS⋅ + scavenging ratio, which was calculated as follows:
Acontrol is the absorbance of water and Asample is the absorbance of samples. Ascorbic acid (vitamin C) was used as a positive control.
Hydroxyl Radical Scavenging Activity
The hydroxyl radical scavenging activity was measured based on the method reported in a previous study (21). In brief, the P. lobata extract solution (100 μl) was mixed with 100 μl of 6 mM ferrous sulfate solution and 6 mM salicylic acid ethanol solution. The mixtures were mixed with 1 ml of 0.1% hydrogen peroxide and reacted for 30 min in the dark. The absorbance was recorded at 510 nm, and the results were displayed by the hydroxyl radical scavenging ratio.
Mice Animal Design
The animal experimental protocols were approved and authorized by the Institutional Animal Care and Use Committee of China Pharmaceutical University (SYXK-2020-0023). The experimental test was designed according to the study with minor modifications (22). Twelve specific pathogen-free male C57BL/6 mice (6 weeks of age, around 18 g) were purchased from the Model Animal Research Center of Nanjing University. The mice were divided randomly into two groups after acclimatization for 1 week. In brief, except for daily gavage with water, the two-group mice were separately fed by the normal diet with or without P. lobata extract (200 mg/kg/day). The body weight of each mouse was measured daily. Also, the feces were collected at the end of the feeding duration and the experimental period. The feces of two experimental groups were immediately frozen in liquid nitrogen and stored at −80°C before the sequence analysis. All the animals were sacrificed using isoflurane as an anesthetic. Livers from each mouse were excised and collected for biochemical assays.
The Antioxidant Status of Mice Liver
The biochemical parameters of liver samples from the mice, including SOD, GSH-Px activity, T-AOC, and MDA activity, were prepared according to the study and determined by using commercial kits following the manufacturer’s instructions (23).
The Sequencing of Gut Microbiota
Total genome DNA was extracted from the fecal samples of the control and the P. lobata extract groups using a QIAamp DNA stool kit, and the DNA concentration and purity were monitored on 1% agarose gels, and then diluted to 1 ng/μl for amplicon generation. The 16S V3-V4 rDNA genes were amplified using the specific primer with the barcode. All PCR reactions were carried out with the high-fidelity PCR master mix, forward and reverse primers, and template DNA. Thermal cycles were conducted as the following: initial denaturation at 98°C for 1 min, 30 cycles of denaturation at 98°C for 10 s, annealing at 50°C for 30 s, and elongation at 72°C for 5 min. Then, the PCR products were mixed and purified using the Axygen AxyPrep DNA Gel Extraction Kit. Finally, the sequencing libraries were generated using the NEBNext® Ultra™ DNA Library Prep Kit following the manufacturer’s instructions and then sequenced on an Illumina MiSeq platform (San Diego, CA, United States).
Gut Microbiota Data Analysis
The paired-end reads from the original DNA fragments from the control and the P. lobata extract groups were merged using the Flash software. The aired-end reads were assigned by the different barcodes. Then, the Upare software package was used to perform the sequences. The alpha and beta diversities were also analyzed by the In-house Perl scripts. The sequences with ≥97% similarity were assigned to the same operational taxonomic units (OTUs). The observed species were used to estimate the amount of unique OTUs, as well as the rarefaction curves were generated based on these three metrics. The cluster analysis was illustrated using the principal component analysis and the principal coordinate analysis (PCoA). The Stamp software was utilized to study the differences in the abundances of individual taxonomy. The Linear discriminant analysis Effect Size (LEfSe) was used for the quantitative analysis of biomarkers among different groups. The 16S rRNA raw sequence data generated were deposited into the National Center for Biotechnology Information Sequence Read Archive Sprout and available under the accession number of PRJNA8050701.
Statistical Analysis
The SPSS software (version 24.0) and excel (version 2020) were used for statistical analysis. The Student’s t-test was used to assess the mean difference between the P. lobata extract and the control groups. A p-value of <0.05 or <0.01 was considered statistically significant. The results were expressed as the mean ± standard deviation.
Results and Discussion
Identification of Polyphenolic Compounds in the P. lobata Extract
Pueraria lobata has been in the Chinese List of Food and Medicine Homologous Substance since 2002 and is currently regarded as a functional food in the food industry due to possessing a great number of bioactive compounds such as isoflavones, polysaccharides, terpenoids, and amino acids. It has been reported that the antioxidant activity of the P. lobata extract was well correlated with the content of phenolic compounds (24). Although other antioxidants were probably present in the P. lobata extract, phenolic compounds made a significant contribution to the antioxidant activity. The polyphenol-rich P. lobata extract (EPM) was eluted by gradient elution HPLC (Figure 1). The yield content of this P. lobata extract is 1.2%. The total phenolic content of this extract was 67.3 mg gallic acid equivalents/g dry weight.
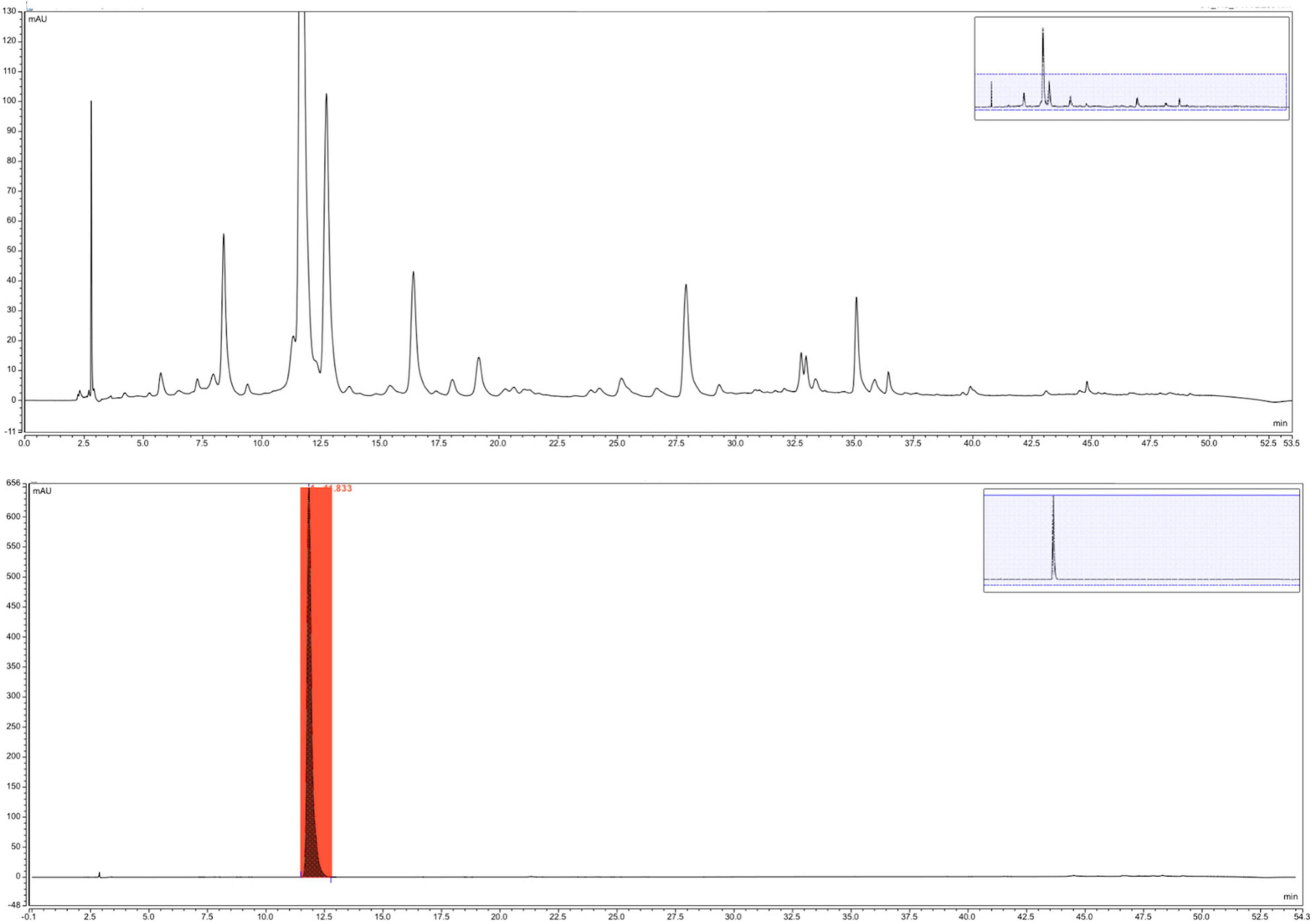
Figure 1. Phenolic high-performance liquid chromatography (HPLC) spectra of the Pueraria lobata extract and compound puerarin.
Effect of the P. lobata Extract on Antioxidant Activity Analysis
Effect of the P. lobata Extract on 2,2-Diphenyl-1-Picrylhydrazyl Radical Scavenging Activity
The scavenging activity of DPPH free radical is widely used to evaluate the free radical scavenging capability of natural bioactive compounds (20). All the P. lobata extract fractions tested exhibited strong antioxidant activities in this assay in a dose-dependent manner (Figure 2A). For instance, the DPPH free radical scavenging ratio of the P. lobata extract was 62.3, 78.8, 85.2, and 88.0% at the concentrations of 100, 200, 300, and 400 μg/ml, respectively. Additionally, the IC50 values of DPPH radical scavenging activity by the P. lobata extract fraction were 50.8 μg/ml. As well, there were differences in the scavenging ratios exhibited by the different concentrations of the P. lobata extract fractions. The antioxidant activity of the P. lobata extract was still considerably lower than that of vitamin C at the same concentration. Cherdshewasart et al. found that the same concentration of puerarin (IC50 value 93.26 μg/ml) exhibited insignificant antioxidant activity evaluated by DPPH activity as compared with vitamin C (IC50 value 72.33 μg/ml) (24). The mechanism of the P. lobata extract scavenging DPPH radical could be through providing protons to reduce oxidative free radicals, besides preventing chain reactions of free radicals (25). These findings suggested that the P. lobata extract owned a robust antioxidative capacity of scavenging free radicals.
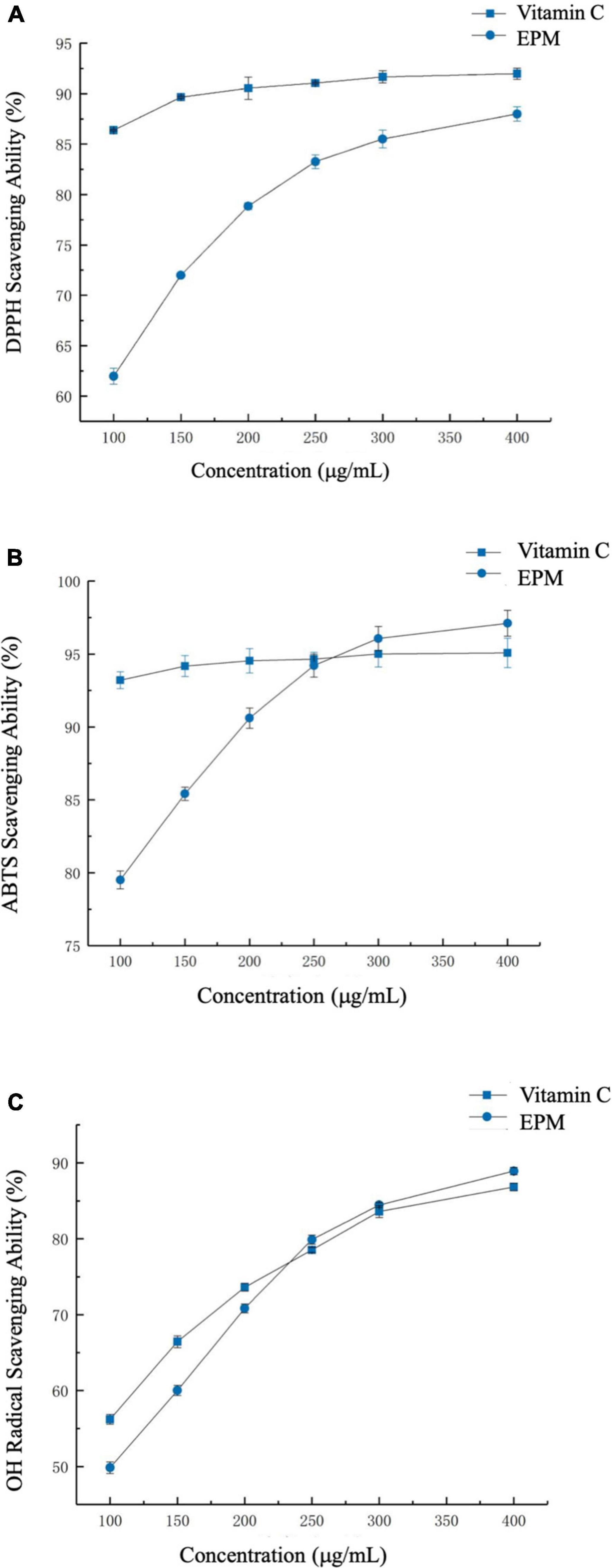
Figure 2. The antioxidant activities of the Pueraria lobata extract. (A) 2,2-Diphenyl-1-picrylhydrazyl (DPPH) scavenging activity, (B) 2,2′-azinobis-(3-ethylbenzthiazoline-6-sulphonate) (ABTS) scavenging activity, and (C) hydroxyl radical scavenging activity. CTL, normal control group; EPM, P. lobata extract group.
Effect of the P. lobata Extract on 2,2′-Azinobis-(3-Ethylbenzthiazoline-6-Sulphonate) Radical Scavenging Activity
ABTS free radicals can be inhibited by diverse antioxidant compounds sourced from foods, resulting in the reduced formation of nitrogen-centered ABTS⋅ + cation (26). The P. lobata extract scavenged ABTS free radicals in a dose-dependent manner (Figure 2B). For the P. lobata extract fraction, the scavenging capabilities were 79.6, 90.7, 96.0, and 97.2% at the concentrations of 100, 200, 300, and 400 μg/ml, respectively. Additionally, the IC50 values of ABTS radical scavenging activity by the P. lobata extract fraction were 13.9 μg/ml. In addition, the scavenging ability of the P. lobata extract was slightly higher than that of ascorbic acid when the concentration of the P. lobata extract was over 250 μg/ml. Wang et al. showed that polysaccharides extracted from P. lobata exerted a comparative activity to scavenge ABTS⋅ + (27). This capacity of the P. lobata extract fraction to scavenge ABTS⋅ + was similar to that result obtained from DPPH radical scavenging assay. All these findings suggested that the P. lobata extract, rich in polyphenols, had excellent abilities of scavenging free radicals.
Effect of the P. lobata Extract on Hydroxyl Radical Scavenging Activity
The antioxidant activities of the P. lobata extract were further tested and confirmed by measuring the activity to cleanse hydroxyl radicals. Hydroxyl radicals could attack the biological molecules, interference with normal cellular functions, leading to cause human adverse physical reactions (28). Similar to the results shown above, the P. lobata extract (100–400 μg/ml) dose-dependently cleansed hydroxyl radical, which suggested that the P. lobata extract could inhibit the production of hydroxyl radical and effectively diminish the oxidative damage in human bodies (Figure 2C). For the P. lobata extract fraction, the scavenging activities were 49.7, 70.6, 84.5, and 88.8% at the concentrations of 100, 200, 300, and 400 μg/ml, respectively. In addition, the IC50 values of hydroxyl radical scavenging activity by the P. lobata extract fraction were 100.4 μg/ml. For comparison, the scavenging capacity of the P. lobata extract was higher than that of positive control when the concentration was higher than 225 μg/ml. Consistently, Jin et al. reported that the polyphenols in P. lobata riched in daidzein, genistein, B-2-O-glucopyranoside, (+)-puerarol, and puerarin could exhibit strong scavenging hydroxyl radical ratios (29). These results denoted that the P. lobata extract did excellently in scavenging oxidants through the series of analyses in vitro.
Effect of the P. lobata Extract on the Antioxidant Status of Mice Liver in vivo
As the mechanisms of antioxidant evaluation methods in vitro and in vivo were different, in order to determine the potential antioxidative activities comprehensively, mice model was used, and the SOD, GSH-Px activity, T-AOC, and MDA contents in the mice liver were measured, which could be mirrored the cellular antioxidant status as key indicators (Table 1) (30). The main endogenous antioxidant enzymes including SOD, GSH-PX, T-AOC, and MDA in the body system were a protective mechanism, and these compounds could prevent oxidative stress and cell damage (31). Puerarin was reported to possess the potential antioxidative activities both in vitro and in vivo. The antioxidant effect in vivo was partly responded via increasing the activities of antioxidant enzymes, for instance, increasing GSH and the ratio of GSH/GSSG in rat liver, as well as reducing the related activity of caspase-3 relative to cell apoptosis (23).
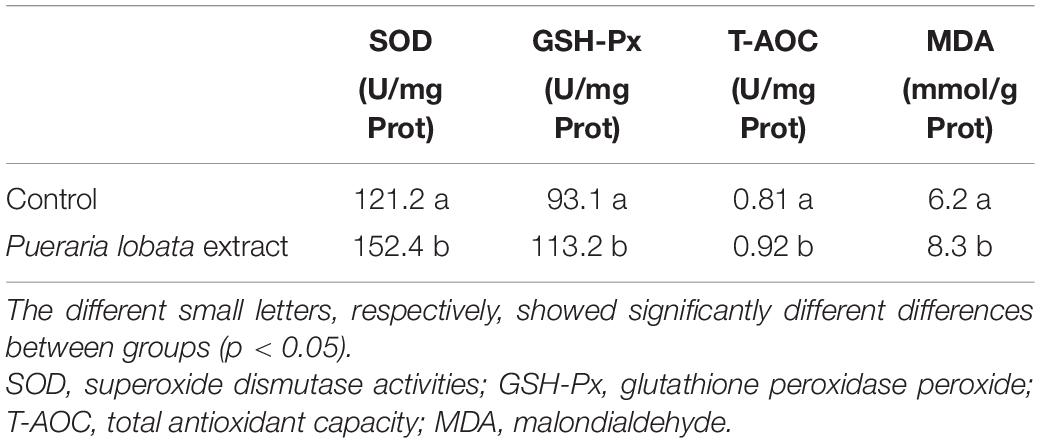
Table 1. Effects of the Pueraria lobata extract on the activities of SOD, GSH-Px, T-AOC, and MDA in the liver of mice, respectively.
There was an insignificant difference in the body weight between the control and the P. lobata extract groups during the feeding period. The GSH-Px activity in the liver in the P. lobata extract group was significantly increased by 21.6% compared with that in the control group (p < 0.05). As for the SOD activity, T-AOC and MDA parameters in the mice liver of the P. lobata extract group were significantly increased by 25.7, 13.6, and 33.9%, respectively (p < 0.05), compared with control. As a result, our study noted that the P. lobata extract could enhance the activities of antioxidant enzymes in the liver and inhibit the oxidative damage inside the body, which was evidenced for potentially affecting positively the protection of the liver. Similar findings have been reported by Senevirathne et al. (32). Polyphenols extracted from foods or medical herbs have been also demonstrated a strong ability to scavenge free radicals and terminate oxidative reactions, thereby in consideration of their antioxidant capacities (33, 34). Previously, dietary polyphenol-rich supplementation of barley bran and cranberry (Vaccinium macrocarpon) peel could help to boost the antioxidant status of serum and liver based on the mice model evaluation (35, 36). Notably, the results indicated that the enhanced antioxidant capacities of polyphenol-rich extract of P. lobata in vitro could be further verified in this mice liver model via increasing the activities of SOD, GSH-Px, and MDA, as well as did better in T-AOC than those in the control.
Effect of the P. lobata Extract on the Gut Microbiota
The P. lobata extract was proved to possess the antioxidant bioactivities using in vitro and in vivo methodologies, and to further investigate the potential effect on the gut microbes, the in vivo evaluation of the P. lobata extract on the composition and structure of the gut microbiota was performed (Figure 3A). Gut microbiota is responsible for the production of human functional compounds, vitamins, and metabolization of the dietary compound and has a potential beneficial protection against the unhealthy pathogens. Numerous studies showed that any imbalanced status about the composition and structure of gut microbiota would potentially cause the development of colonic dysbiosis, which is powerfully associated with several pathologies and human chronic diseases, including intestinal bowel disease, colon cancer, and obesity (37–39). Polyphenols derived from foods and medicine plants have the capability to reduce bacterial metabolizing enzymes, thereby reducing the colonic tumor incidence and improving cell metabolism; as well, the growth of harmful microbiome could be inhibited, and the abundance of the health-benefit microbiome was enhanced, such as Lactobacillus and Bifidobacterium species (40). The link of the antioxidant effect of polyphenols in vivo and hinder the bacterial proliferation, and the growth of enterpathogenic bacteria was also discussed in several studies (41, 42).
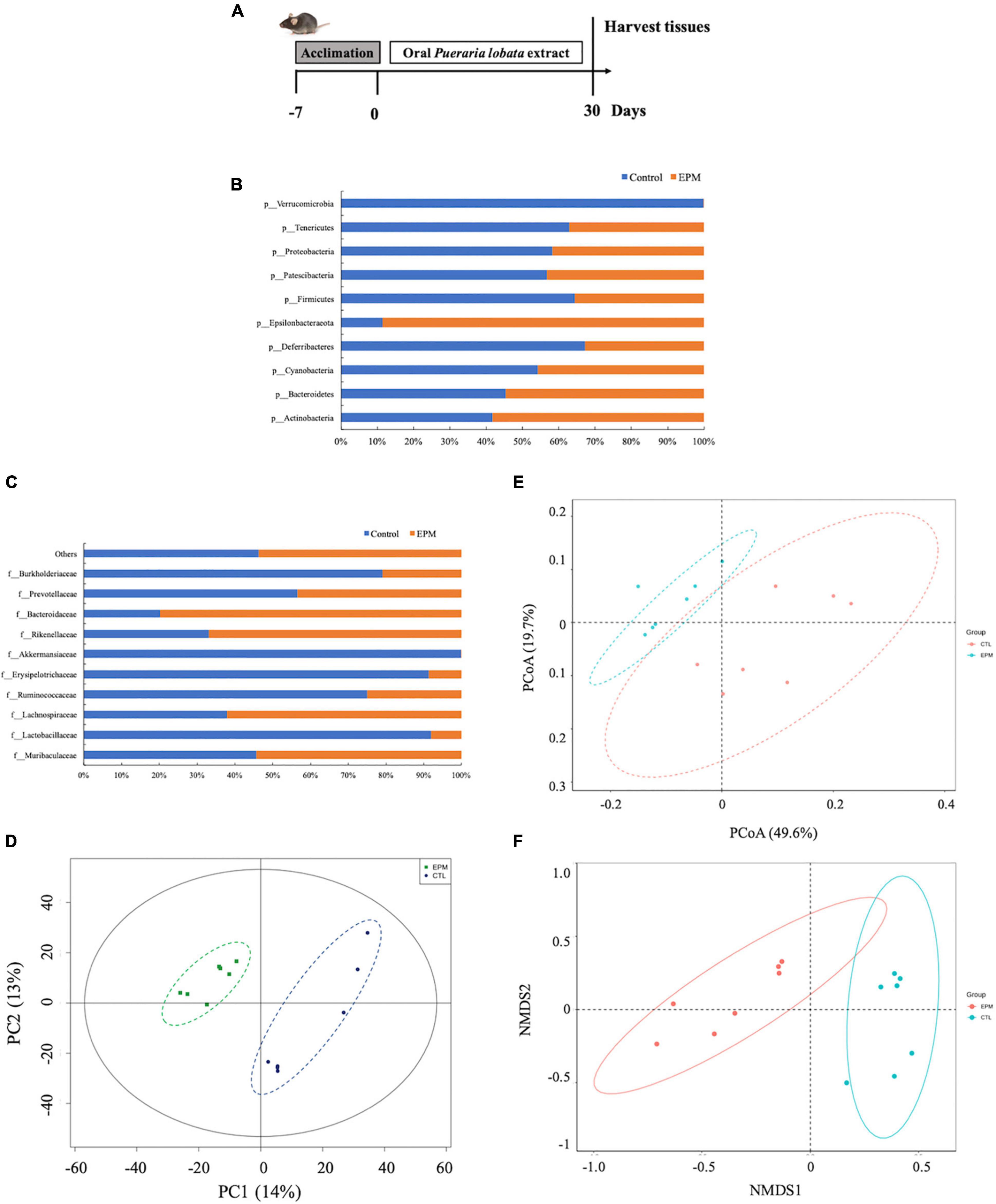
Figure 3. (A) Animal design and the antioxidant of the Pueraria lobata extract in vivo. (B) Gut bacterial taxonomic profiling of the control and the P. lobata extract groups at the phylum level. (C) Gut bacterial taxonomic profiling of the control and the P. lobata extract groups at the class level. (D) PCA, (E) PCoA, and (F) NMDS plots of weighted UniFrac and Unweighted UniFrac distance analyses of beta-diversity among samples showing a higher similarity among the bacterial community between the control group and the P. lobata extract group.
We performed the high-throughput sequencing of the 16S rDNA region to determine the gut microbial community treated by the P. lobata extract. The sequencing depth reads of the control and the P. lobata extract groups were in the range of 272,642 base pairs (bp) and 295,776 bp, respectively. The data indicated that most of the species in the gut microbiota were captured, and the output of these data was valid. Considering the bacterial change at the phylum level, the microbiome of the control and the P. lobata extract groups mainly consisted of Actinobacteria, Bacteroidetes, Cyanobacteria, Deferribacteres, Epsilonbacteraeota, Firmicutes, Patescibacteria, Proteobacteria, Tenericutes, and Verrucomicrobia (Figure 3B). The ratios of Actinobacteria and Bacteroidetes in the P. lobata extract group were 1.39-fold and 1.20-fold higher than those in the control group, respectively. However, the ratios of Cyanobacteria, Firmicutes, and Proteobacteria decreased by 15.5, 44.7, and 28.1% compared with the control group, respectively. The ratio of Bacteroidetes and Firmicutes phyla consisted of more than 90% population in the gut microbiota, and they would play an important role in human health (43). The Bacteroidetes was considered to have an effect to modulate the immune responses and activate the immune lymphocyte T cells (44). In summary, the supplementation of the P. lobata extract contributed to the functional differences of mice gut microbiota.
All the 10 most abundant or representative families were Muribaculaceae, Lachnospiraceae, Lactobacillaceae, Ruminococcaceae, Akkermansiaceae, Erysipelotrichaceae, Rikenellaceae, Bacteroidaceae, Prevotellaceae, and Burkholderiaceae (Figure 3C). The ratios of Lactobacillaceae, Rikenellaceae, and Bacteroidetes in the P. lobata extract group were 1.64-fold, 2.02-fold, and 3.96-fold higher than those in the control group, respectively. However, the ratio of Ruminococcaceae, Prevotellaceae, and Burkholderiaceae decreased by 66.8, 23.3, and 73.6% than those in the control group. Interestingly, the P. lobata extract almost decreased over 90% of Erysipelotrichaceae and Akkermansiaceae at the family level, which could be useful when these two families of microbiome were overgrown and overproduced in the gut. These results noted that the P. lobata extract would be a useful ingredient to restore the balance of the gut ecosystem.
When searching how the P. lobata extract and control differ in terms of the composition and structure of gut microbiota profiles, a distance square matrix was calculated to reflect the dissimilarity between the two groups (weighted UniFrac and unweighted UniFrac distances) (Figure 3D). The QIIME software could calculate both weighted and unweighted UniFrac distance, which are phylogenetic measures of beta diversity. We used unweighted UniFrac distance for the PCoA, and the PCoA could help to get principal coordinates and visualize them from complex, multidimensional data (Figure 3E). The results demonstrated that the bacterial communities of the P. lobata extract were significantly different from those of the control group. A long-distance between these groups was observed, pointing out that the P. lobata extract modulated the bacterial gut microbiome. This distance difference was also significantly evident in the non-metric multidimensional scaling, which displayed the differences among gut microbiota of the P. lobata extract and control groups (Figure 3F).
Altogether, the metagenomics showed the modulation of gut microbiota by the consumption of the P. lobata extract group via the changing of the abundance of Firmicutes and Bacteroidetes phyla and the decrease of Ruminococcaceae, Verrucomicrobiae, and Prevotellaceae phyla in mice gut (Figures 4A,B). Chen et al. administered 12.5 mg/kg polysaccharides extracted in P. lobata to both normal mice and antibiotic-associated diarrhea mice for 2 weeks (45). They showed that the P. lobata extract fractions significantly increased the abundance of beneficial bacteria, involving Oscillospira and Anaerotruncus, whereas relieving colonic pathological changes and gut microbiota dysbiosis additionally. Thus, P. lobata polyphenol extract may be a promising source of the phenolic compound that exerts prebiotic effects in vivo for human health. In addition to taxonomic composition, the functional profiles were predicted by PICRUSt, and the functional differences are shown in Figure 5. The LEfSe result could illustrate biological explanations to establish the biological consistency and estimation of predicted biomarkers (46). In the treatment of P. lobata, the phenolic-rich extracts induced functional differences of microbial communities consisted of cellular process and signaling, circulatory system, and neurodegenerative diseases. This was in consistent with the previous studies that the Puerarin, the main bioactive compound found in P. lobata, was found to be beneficial to the neurodegenerative diseases in vitro and in vivo (47, 48). A similar study found that the bioactive compound puerarin had an obvious anti-inflammatory effect using mice model, and it repaired intestinal mucosal integrity via regulating the abundance and kinds of the disorder gut microbiota, increasing the express of tight binding proteins ZO-1 and occludin, and these bacterial Ruminococcaceae, Lachnospiraceae, Eubacterium_xylanophilum, and Ruminococcus_1 were the key species related (49). This study provides new insight into the potential application of P. lobata to be developed as a functional ingredient, which would have a beneficial effect on gut health. The physiological effects of fermentation of P. lobata on the potential colon health will be further investigated.
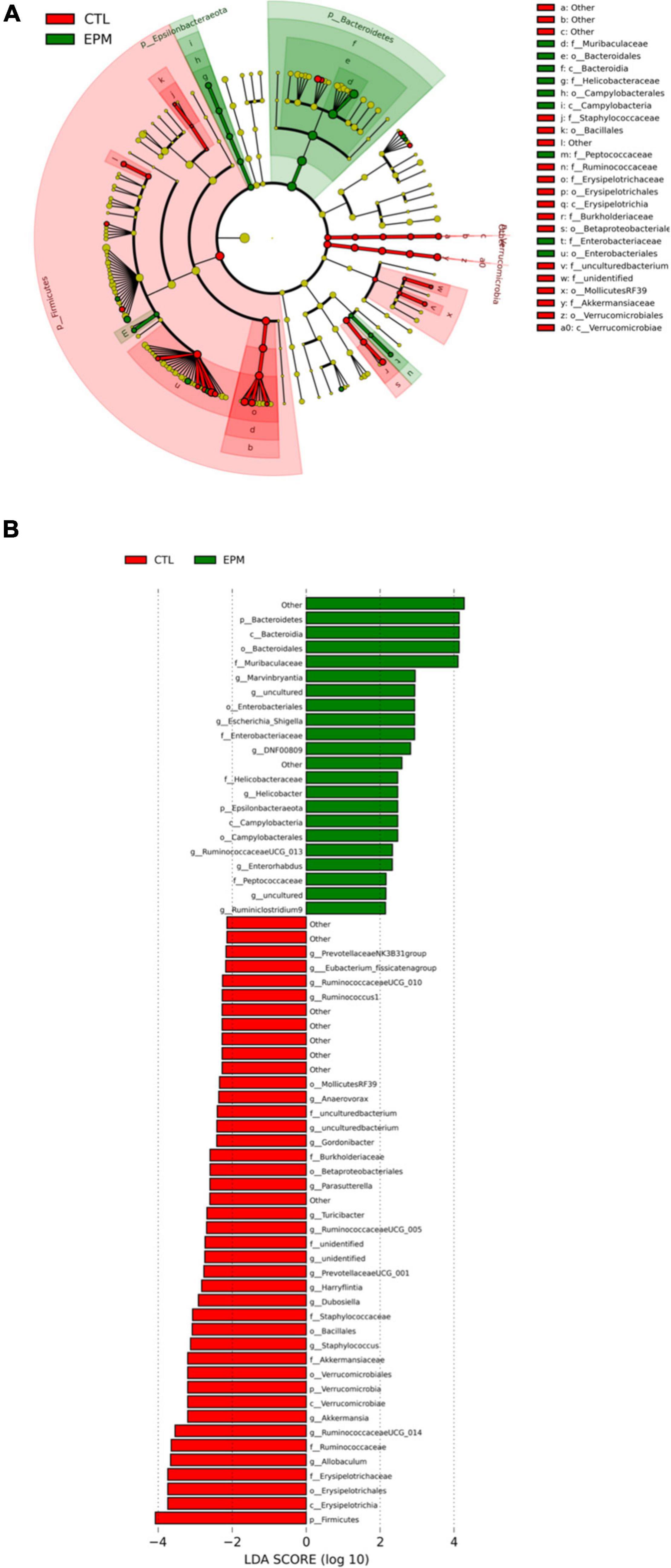
Figure 4. Linear discriminant analysis Effect Size (LEfSe) plot of microbial communities affected by the Pueraria lobata extract diet. (Red) CTL; (Green) EPM. The brightness of each dot was proportional to its effect size (A). The taxa had a significant LDA threshold value of > 2 were shown (B). CTL, normal control group; EPM: P. lobata extract group.
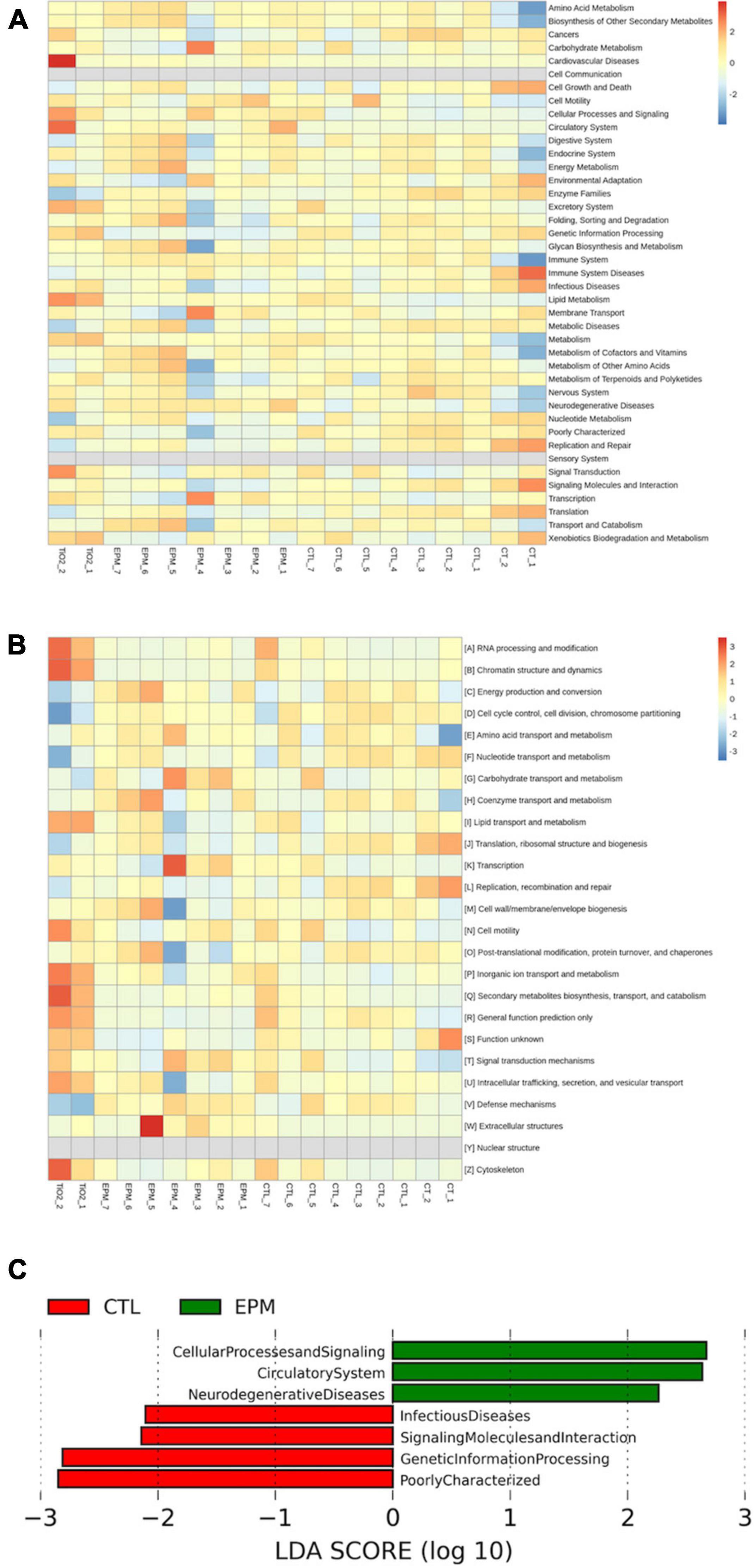
Figure 5. Effect of the normal control group and the Pueraria lobata extract on differential operational taxonomic units (OTUs) and predicted microbial community functions by PICRUSt. (A) Kyoto Encyclopedia of Genes and Genomes (KEGG) heat map analysis. (B) Cluster of orthologous groups of proteins (COG) heat map analysis. (C) PICRUSt function prediction. CTL, normal control group; EPM, P. lobata extract group.
Conclusion
In this study, we purified the polyphenol-rich extract from Pueraria lobata and investigated systematically its antioxidant activities and its effect on gut microbiota. The P. lobata extract consisted of a large amount of puerarin that exhibited strong antioxidative capabilities in vitro and in vivo. Moreover, it had a positive effect on the structure and composition of gut microbiota. In summary, the phenolic-rich extract of P. lobata has considerable potential for utilization as a natural antioxidative food additive, as well as a type of gut microbiota-modulating health-promoting food ingredient. Therefore, we suggested that the P. lobata extract could be utilized as a promising supplement for functional foods that contributed to the prevention of various human chronic diseases.
Data Availability Statement
The datasets presented in this study can be found in online repositories. The names of the repository/repositories and accession number(s) can be found in the article/supplementary material.
Ethics Statement
The animal study was reviewed and approved by the Institutional Animal Care and Use Committee of China Pharmaceutical University.
Author Contributions
XX conceived the project idea and obtained the funding. XX and CS assigned the project, performed the research, and wrote the manuscript. YG, SC, WM, and XLX finished all the research. JM, JS, and CS revised the manuscript. XX, SH, LJ, and JM participated in the method and review. All authors approved the final version of the manuscript.
Funding
This study was financially supported by the Natural Science Foundation of Zhejiang Province, China (LQ21C200003), Natural Science Foundation of Shaoxing University (2019LG1012), and Scientific Research Start-Up Fund of Shaoxing University (20195015).
Conflict of Interest
The authors declare that the research was conducted in the absence of any commercial or financial relationships that could be construed as a potential conflict of interest.
Publisher’s Note
All claims expressed in this article are solely those of the authors and do not necessarily represent those of their affiliated organizations, or those of the publisher, the editors and the reviewers. Any product that may be evaluated in this article, or claim that may be made by its manufacturer, is not guaranteed or endorsed by the publisher.
Acknowledgments
We acknowledge the Institutional Animal Care and Use Committee of China Pharmaceutical University for the support of animal experiments in our study.
Footnotes
References
1. Fang Q. Some current study and research approaches relating to the use of plants in the traditional Chinese medicine. J Ethnopharmacol. (1980) 2:57–63. doi: 10.1016/0378-8741(80)90031-8
2. Wong KH, Li GQ, Li KM, Razmovski-Naumovski V, Chan K. Kudzu root: traditional uses and potential medicinal benefits in diabetes and cardiovascular diseases. J Ethnopharmacol. (2011) 134:584–607. doi: 10.1016/j.jep.2011.02.001
3. Tome-Carneiro J, Visioli F. Polyphenol-based nutraceuticals for the prevention and treatment of cardiovascular disease: review of human evidence. Phytomedicine. (2016) 23:1145–74. doi: 10.1016/j.phymed.2015.10.018
4. Xu L, Zheng N, He Q, Li R, Zhang K, Liang T. Puerarin, isolated from Pueraria lobata (Willd.), protects against hepatotoxicity via specific inhibition of the TGF-β1/Smad signaling pathway, thereby leading to anti-fibrotic effect. Phytomedicine. (2013) 20:1172–9. doi: 10.1016/j.phymed.2013.06.008
5. Park HJ, Jeon YK, You DH, Nam MJ. Daidzein causes cytochrome c-mediated apoptosis via the Bcl-2 family in human hepatic cancer cells. Food Chem Toxicol. (2013) 60:542–9. doi: 10.1016/j.fct.2013.08.022
6. Son E, Yoon J-M, An B-J, Lee YM, Cha J, Chi G-Y, et al. Comparison among activities and isoflavonoids from Pueraria thunbergiana aerial parts and root. Molecules. (2019) 24:912. doi: 10.3390/molecules24050912
7. Lim DW, Lee C, Kim I-H, Kim YT. Anti-inflammatory effects of total isoflavones from Pueraria lobata on cerebral ischemia in rats. Molecules. (2013) 18:10404–12. doi: 10.3390/molecules180910404
8. Gagniere J, Raisc JH, Veziant J, Barnich N, Bonnet R, Buc E, et al. Gut microbiota imbalance and colorectal cancer. World J Gastroenterol. (2016) 24:501–18. doi: 10.3748/wjg.v22.i2.501
9. Flint HJ, Scott KP, Louis P, Duncan SH. The role of the gut microbiota in nutrition and health. Nat Rev Gastroenterol Hepatol. (2012) 9:577–89. doi: 10.1038/nrgastro.2012.156
10. Morgan XC, Tickle TL, Sokol H, Gevers D, Devaney KL, Ward DV, et al. Dysfunction of the intestinal microbiome in inflammatory bowel disease and treatment. Genome Biol. (2012) 13:R79. doi: 10.1186/gb-2012-13-9-r79
11. Kostic AD, Xavier RJ, Gevers D. The microbiome in inflammatory bowel disease: current status and the future ahead. Gastroenterology. (2014) 146:1489–99. doi: 10.1053/j.gastro.2014.02.009
12. Everard A, Belzer C, Geurts L, Ouwerkerk JP, Druart C, Bindels LB, et al. Cross-talk between Akkermansia muciniphila and intestinal epithelium controls diet-induced obesity. Proc Natl Acad Sci USA. (2013) 110:9066–71. doi: 10.1073/pnas.1219451110
13. Laparra JM, Sanz Y. Interactions of gut microbiota with functional food components and nutraceuticals. Pharmacol Res. (2010) 61:219–25. doi: 10.1016/j.phrs.2009.11.001
14. Liu Z, Chen Q, Zhang C, Ni L. Comparative study of the anti-obesity and gut microbiota modulation effects of green tea phenolics and their oxidation products in high-fat-induced obese mice. Food Chem. (2022) 367:130735. doi: 10.1016/j.foodchem.2021.130735
15. Choi Y-H, Hong SS, Shin YS, Hwan BYG, Park S-Y, Lee D. Phenolic compounds from Pueraria lobata protect PC12 cells against a beta-induced toxicity. Arch Pharm Res. (2010) 33:1651–4. doi: 10.1007/s12272-010-1014-7
16. Okarter N, Liu C-S, Sorrells ME, Liu RH. Phytochemical content and antioxidant activity of six diverse varieties of whole wheat. Food Chem. (2010) 33:249–57. doi: 10.1016/j.foodchem.2009.06.021
17. Alvarez-Parrilla E, de la Rosa LA, Amarowicz R, Shahidi F. Antioxidant activity of fresh and processed jalapeño and serrano peppers. J Agric Food Chem. (2011) 59:163–73. doi: 10.1021/jf103434u
18. Wagle A, Seong SH, Jung HA, Choi JS. Identifying an isoflavone from the root of Pueraria lobata as a potent tyrosinase inhibitor. Food Chem. (2019) 276:383–9. doi: 10.1016/j.foodchem.2018.10.008
19. Bing J, Xiao X, McClements DJ, Biao Y, Chongjiang C. Protein corona formation around inorganic nanoparticles: food plant proteins-TiO2 nanoparticle interactions. Food Hydrocol. (2021) 115:106594. doi: 10.1016/j.foodhyd.2021.106594
20. Biao Y, Jiannan H, Yaolan C, Shujie C, Dechun H, Julian Mcclements D, et al. Identification and characterization of antioxidant and immune-stimulatory polysaccharides in flaxseed hull. Food Chem. (2020) 315:126266. doi: 10.1016/j.foodchem.2020.126266
21. Xu X, Hu W, Zhou S, Tu C, Xia X, Zhang J, et al. Increased phenolic content and enhanced antioxidant activity in fermented glutinous rice supplemented with fu brick tea. Molecules. (2019) 24:671. doi: 10.3390/molecules2404067
22. Hu Q, Yuan B, Wu X, Du H, Gu M, Han Y, et al. Dietary intake of Pleurotus eryngii ameliorated dextran-sodium-sulfate-induced colitis in mice. Mol Nutr Food Res. (2019) 63:e1801265. doi: 10.1002/mnfr.201801265
23. Liu C-M, Ma J-Q, Sun Y-Z. Protective role of puerarin on lead-induced alterations of the hepatic glutathione antioxidant system and hyperlipidemia in rats. Food Chem Toxicol. (2011) 49:3119–27. doi: 10.1016/j.fct.2011.09.007
24. Cherdshewasart W, Sutjit W. Correlation of antioxidant activity and major isoflavonoid contents of the phytoestrogen-rich Pueraria mirifica and Pueraria lobata tubers. Phytomedicine. (2008) 15:38–43. doi: 10.1016/j.phymed.2007.07.058
25. Huang DJ, Ou BX, Prior RL. The chemistry behind antioxidant capacity assays. J Agric Food Chem. (2005) 53:1841–56. doi: 10.1021/jf030723c
26. Re R, Pellegrini N, Proteggente A, Pannala A, Yang M, Rice-Evans C. Antioxidant activity applying an improved ABTS radical cation decolorization assay. Free Radic Biol Med. (1999) 26:1231–7. doi: 10.1016/s0891-5849(98)00315-3
27. Wang Z-B, Chen B-B, Luo L, Yan J-K. Fractionation, physicochemical characteristics and biological activities of polysaccharides from Pueraria lobata roots. J Taiwan Inst Chem Eng. (2016) 67:54–60. doi: 10.1016/j.jtice.2016.07.029
28. Treml J, Smejkal K. Flavonoids as potent scavengers of hydroxyl radicals. Compr Rev Food Sci Food Saf. (2016) 15:720–38. doi: 10.1111/1541-4337.12204
29. Jin SE, Son YK, Min B-S, Jung HA, Choi JS. Anti-inflammatory and antioxidant activities of constituents isolated from Pueraria lobata roots. Arch Pharm Res. (2012) 35:823–37. doi: 10.1007/s12272-012-0508-x
30. Olawole TD, Okundigie MI, Rotimi SO, Okwumabua O, Afolabi IS. Preadministration of fermented sorghum diet provides protection against hyperglycemia-induced oxidative stress and suppressed glucose utilization in alloxan-induced diabetic rats. Front Nutr. (2018) 5:16. doi: 10.3389/fnut.2018.00016
31. Duangjan C, Rangsinth P, Zhang S, Gu X, Wink M, Tencomnao T. Vitis vinifera leaf extract protects against glutamate-induced oxidative toxicity in HT22 hippocampal neuronal cells and increases stress resistance properties in Caenorhabditis elegans. Front Nutr. (2021) 8:634100. doi: 10.3389/fnut.2021.634100
32. Senevirathne M, Ahn C-B, Je J-Y. Hepatoprotective effect of chitooligosaccharides against tert-butylhydroperoxide-induced damage in chang liver cells. Carbohydr Polym. (2011) 83:995–1000. doi: 10.1016/j.carbpol.2010.09.016
33. RiceEvans CA, Miller NJ, Paganga G. Structure-antioxidant activity relationships of flavonoids and phenolic acids. Free Radic Biol Med. (1996) 20:933–56. doi: 10.1016/0891-5849(95)02227-9
34. RiceEvans CA, Miller J, Paganga G. Antioxidant properties of phenolic compounds. Trends Plant Sci. (1997) 2:152–9. doi: 10.1016/S1360-1385(97)01018-
35. Zavodnik I, Buko V, Lukivskaya O, Lapshina E, Ilyich T, Belonovskaya E, et al. Cranberry (Vaccinium macrocarpon) peel polyphenol-rich extract attenuates rat liver mitochondria impairments in alcoholic steatohepatitis in vivo and after oxidative treatment in vitro. J Funct Foods. (2019) 57:83–94. doi: 10.1016/j.jff.2019.04.001
36. Zhang Y, Yin L, Huang L, Tekliye M, Xia X, Li J, et al. Composition, antioxidant activity, and neuroprotective effects of anthocyanin-rich extract from purple highland barley bran and its promotion on autophagy. Food Chem. (2021) 339:127849. doi: 10.1016/j.foodchem.2020.127849
37. Round JL, Mazmanian SK. The gut microbiota shapes intestinal immune responses during health and disease. Nat Rev Immunol. (2009) 9:313–23. doi: 10.1038/nri2515
38. Louis P, Hold GL, Flint HJ. The gut microbiota, bacterial metabolites and colorectal cancer. Nat Rev Microbiol. (2014) 12:661–72.
39. Tremaroli V, Backhed F. Functional interactions between the gut microbiota and host metabolism. Nature. (2012) 12:242–9. doi: 10.1038/nature11552
40. Loo YT, Howell K, Chan M, Zhang P, Ng K. Modulation of the human gut microbiota by phenolics and phenolic fiber-rich foods. Compre Rev Food Sci Food Saf. (2020) 19:1268–98. doi: 10.1111/1541-4337.12563
41. Yin J, Ren W, Wei B, Huang H, Li M, Wu X, et al. Characterization of chemical composition and prebiotic effect of a dietary medicinal plant Penthorum chinense Pursh. Food Chem. (2020) 319:126568. doi: 10.1016/j.foodchem.2020.126568
42. Gowd V, Bao T, Wang L, Huang Y, Chen S, Zheng X, et al. Antioxidant and antidiabetic activity of blackberry after gastrointestinal digestion and human gut microbiota fermentation. Food Chem. (2018) 269:618–27. doi: 10.1016/j.foodchem.2018.07.020
43. Turnbaugh PJ, Ley RE, Mahowald MA, Magrini V, Mardis ER, Gordon JI. An obesity-associated gut microbiome with increased capacity for energy harvest. Nature. (2006) 444:1027–31. doi: 10.1038/nature05414
44. Picard C, Fioramonti J, Francois A, Robinson T, Neant F, Matuchansky C. Review article: bifidobacteria as probiotic agents – physiological effects and clinical benefits. Aliment Pharm Ther. (2005) 22:495–512. doi: 10.1111/j.1365-2036.2005.02615.x
45. Chen R, Liu B, Wang X, Chen K, Zhang K, Zhang L, et al. Effects of polysaccharide from Pueraria lobata on gut microbiota in mice. Int J Biol Macromol. (2020) 158:740–9. doi: 10.1016/j.ijbiomac.2020.04.201
46. Liu J, Liu L, Zhang G, Peng X. Poria cocos polysaccharides attenuate chronic nonbacterial prostatitis by targeting the gut microbiota: comparative study of Poria cocos polysaccharides and finasteride in treating chronic prostatitis. Int J Biol Macromol. (2021) 189:346–55. doi: 10.1016/j.ijbiomac.2021.08.139
47. Zou Y, Hong B, Fan L, Zhou L, Liu Y, Wu Q, et al. Protective effect of puerarin against beta-amyloid-induced oxidative stress in neuronal cultures from rat hippocampus: involvement of the GSK-3 beta/Nrf2 signaling pathway. Free Radic Res. (2013) 47:55–63. doi: 10.3109/10715762.2012.742518
48. Mahdy HM, Mohamed MR, Emam MA, Karim AM, Abdel-Naim A, Khalifa AE. The anti-apoptotic and anti-inflammatory properties of puerarin attenuate 3-nitropropionic-acid induced neurotoxicity in rats. Can J Physiol Pharmacol. (2014) 92:252–8. doi: 10.1139/cjpp-2013-0398
49. Li B, Liu M, Wang Y, Gong S, Yao W, Li W, et al. Puerarin improves the bone micro-environment to inhibit OVX-induced osteoporosis via modulating SCFAs released by the gut microbiota and repairing intestinal mucosal integrity. Biomed Pharmacother. (2020) 132:110923. doi: 10.1016/j.biopha.2020.110923
Keywords: Pueraria lobata, antioxidant, polyphenols, gut microbiota, mice model
Citation: Xu X, Guo Y, Chen S, Ma W, Xu X, Hu S, Jin L, Sun J, Mao J and Shen C (2022) The Positive Influence of Polyphenols Extracted From Pueraria lobata Root on the Gut Microbiota and Its Antioxidant Capability. Front. Nutr. 9:868188. doi: 10.3389/fnut.2022.868188
Received: 02 February 2022; Accepted: 28 February 2022;
Published: 29 March 2022.
Edited by:
Fuguo Liu, Northwest A&F University, ChinaReviewed by:
Jiarun Han, Zhejiang Gongshang University, ChinaDaoyuan Ren, Shaanxi Normal University, China
Copyright © 2022 Xu, Guo, Chen, Ma, Xu, Hu, Jin, Sun, Mao and Shen. This is an open-access article distributed under the terms of the Creative Commons Attribution License (CC BY). The use, distribution or reproduction in other forums is permitted, provided the original author(s) and the copyright owner(s) are credited and that the original publication in this journal is cited, in accordance with accepted academic practice. No use, distribution or reproduction is permitted which does not comply with these terms.
*Correspondence: Jian Mao, bWFvamlhbkBqaWFuZ25hbi5lZHUuY24=; Chi Shen, YWNoYUAxMzkuY29t