- 1Department of Animal Science and Technology, Shandong Agricultural University, Taian, China
- 2College of Life Science, Qilu Normal University, Jinan, China
- 3Precision Livestock and Nutrition Unit, Gembloux Agro-Bio Tech, University of Liège, Gembloux, Belgium
This study was conducted to investigate the effects of Bacillus licheniformis DSM5749 on the production performance and intestinal health in laying hens. A total of 32-week-old laying hens (Hyline Brown) were randomly assigned to two dietary groups (10 replicates of 27 laying hens), namely, basal diet and basal diet complemented with 200 g/t B. licheniformis (3.2 × 109 CFU/kg). The trial lasted for 8 weeks, and samples were collected at the last week. Results revealed that B. licheniformis DSM5749 significantly improved laying performance, including an increase in egg production rate and average daily egg yield, and a decrease in the feed-to-egg ratio during the entire 8-week experimental period (P < 0.05). B. licheniformis DSM5749 increased the levels of superoxide dismutase and glutathione peroxidase in the liver and decreased the IL-1 level in the serum (P < 0.05). In addition, the integrity of intestinal morphology (villus height, crypt depth, and villus height/crypt depth), tight junctions (ZO-1, Claudin-1, and Occludin), and lipase vitality in the intestine were potentiated by B. licheniformis DSM5749 in laying hens (P < 0.05). B. licheniformis DSM5749 decreased the Firmicutes/Bacteroidetes ratio (P < 0.05) in the cecum. Furthermore, B. licheniformis DSM5749 modulated the microbiota in the cecum of the laying hens, increased the relative abundance of beneficial bacteria (e.g., Prevotella) at the genus level and decreased the relative abundance of potential pathogens (e.g., Desulfovibrio). In conclusion, B. licheniformis DSM5749 can improve laying performance, promote intestinal health, affect the composition of cecal microorganisms, and regulate the intestinal micro-ecological balance, making B. licheniformis a good probiotic candidate for application in the laying hens industry.
Introduction
Antibiotics are chemical substances produced by microorganisms that play a remarkable role in disease prevention and growth promotion in the poultry industry. However, residual and resistance problems caused by the long-term use of antibiotics lead to economic losses of livestock and the eventual ban of antibiotic growth promoters, which bring challenges and opportunities to the livestock industry and pressure the industry to find alternatives that are environmentally friendly, green, organic, and efficient for improving poultry growth performance (1). Under these conditions, there is a growing interest in supplementing and/or supporting a natural and beneficial microbiota, which appears to be a promising natural remedy (2, 3). Probiotics, beneficial bacteria that can positively affect the health of poultry (4), can improve growth performance and egg quality (5), ameliorate intestinal morphology and barrier function and enhance the immunoregulatory functionalities of poultry (6).
Recent research revealed that probiotics can promote feed conversion efficiency (7), improve egg production and shell quality (8), and regulate the colonization of symbiotic bacteria (9). Bacillus sp. has been reported to indicate promising applications as a probiotic for feeding because of its inherent ability to sporulate and survive under environmental stress. Spore formers manifest tremendous tolerance and survivability in extreme temperatures, pH, dehydration, or malnutrition (10). In addition, Bacillus probiotic biofilms incorporate biochemical effects such as antimicrobial and enzymatic activity, thus modulating immune system activity and helping to preserve poultry from the gastrointestinal tract (GIT) and other infections (11). Bacillus licheniformis K-508, an aerobic probiotic, possesses the capacity to improve the degradation, absorption, and utilization of nutrients (12), and B. licheniformis-fermented products can suppress the growth and multiplication of pathogens to promote intestinal health (13). Research on broilers demonstrated that supplementing diets with B. licheniformis HJDY01 can improve growth performance, increase short-chain fatty acid production, and regulate cecal microbiota in broilers (14). Meanwhile, some studies indicated that B. licheniformis H2 normalized the ileal microbiota of chickens infected with necrotic enteritis (15) and decreased the incidence of diarrhea in weaning piglets (16). Recent studies have shown that dietary B. licheniformis PY79 (17) and B. licheniformis CGMCC 1.3448 (18) can improve egg production performance and egg quality, ameliorate the adverse influence of heat on egg production (19) and regulate reproductive hormone secretions of laying hens (18).
To our knowledge, comprehensive studies on the intestinal health of B. licheniformis DSM5749 are scarce, and no relevant systematic study has been conducted on laying hens. Furthermore, B. licheniformis DSM5749 is a novel product, and no publication data are available. Therefore, the present experiment was conducted to investigate the effects of B. licheniformis DSM5749 on the laying performance, serum indicators, antioxidant enzyme capacity, and intestinal microecological balance of laying hens to furnish a reference basis for the implementation of B. licheniformis DSM5749 in laying hen production.
Materials and Methods
Experimental Design and Management
A total of 540 32-week-old laying hens (Hyline Brown) with good condition and similar weight were randomly divided into two groups: basal diet (T1 group) and basal diet supplemented with 200 g/t B. licheniformis powders (3.2 × 109 CFU/kg, T2 group). The additive dosage of B. licheniformis was optimized according to previous studies (18). Each group contained 10 replicates of 27 laying hens. The ingredient and nutrient levels in the basal diet are shown in Table 1. The laying hens were housed in a fully enclosed chicken house with a one-story ladder cage system and a relative humidity of approximately 65% during the trial period and received water and diet ad libitum. Fifteen hours (6:00 to 21:00 h) of artificial lighting with a light intensity of 20 lux was provided daily. The temperature was kept at 20–24°C, and eggs were picked up manually at 14:00 daily. Manure was cleaned regularly, and the hens were immunized according to normal immunization procedures. The experimental B. licheniformis (DSM5749) was provided by Chr. Hansen (Beijing) Trading Co., Ltd. (Beijing, China).
Laying Performance
Under the same management conditions, eggs were collected daily, and data on total eggs, egg weight, dirty eggs, broken eggs, soft eggs, malformed eggs, and feed consumption were recorded in duplicate. The egg production rate, qualified rate, average egg weight, average daily egg yield, and feed-egg ratio were subsequently calculated.
Sample Collection
One laying hen per replicate was randomly selected for sampling after laying performance was determined. Blood samples were collected from the wing vein using heparinized anticoagulant tubes. Then, the tubes were shaken gently and transferred to ice. Plasma was obtained after centrifugation at 4,000 rpm for 10 min at 4°C and stored at −20°C for further biochemical analysis. Laying hens were slaughtered by cervical dislocation after blood samples were obtained. Approximately 2 cm segments were excised from the jejunum (from the entry point of the bile duct to Meckel’s diverticulum), flushed repeatedly with cold saline solution, and immediately immersed in 4% paraformaldehyde solution for subsequent histological examination. Tissue samples were obtained from the liver and jejunum. Liver samples at the apical region of the liver were used for further analysis of lipid metabolism and antioxidation capacity. Jejunum samples were collected in the jejunal middle region and divided into two segments, one for gene expression analysis and the other for analysis of digestive enzyme activity and immunity parameters. The samples were washed with ice-cold sterilized saline solution, immediately frozen in liquid nitrogen, and stored at −80°C. The cecum was collected on ice, transported to the laboratory in a dry-ice bag, and then stored at −80°C for 16S rRNA high-throughput sequencing analysis.
Serum Analysis
Glucose (GLU), triglyceride (TG), total cholesterol (TC), low-density lipoprotein (LDL), and high-density lipoprotein (HDL) were analyzed using a fully automatic biochemical analyzer (7020; Hitachi, Co., Ltd., Tokyo, Japan). The immune response status in the serum was estimated by detecting the levels of interleukin 1 (IL-1) and immunoglobulin G (IgG) with enzyme-linked immunosorbent assay (ELISA) kits (MLBIO Co., Ltd., Shanghai, China). All operations were carried out in accordance with the kits’ instructions. The inter- and intra-assay coefficients of variation (CVs) were less than 10%.
Liver Analysis
Triglyceride (No. A110-1-1), total cholesterol (No. A111-1-1), and lipase viability (No. A054-1-1) contents in the liver were detected by biochemical methods following the instructions provided with the reagent kits purchased from Nanjing Jiancheng Bioengineering Institute of China. Superoxide dismutase (SOD, No. ml600830) and glutathione peroxidase (GSH-Px, No. ml036972) were estimated using ELISA kits from MLBIO Co., Ltd. (Shanghai, China).
Jejunal Morphology Analysis
The intestinal samples were trimmed and washed to remove the intestinal contents. The samples were dehydrated and embedded in paraffin following routine procedures. A tissue section was stained with hematoxylin and eosin for histological observation. Jejunal sections were scrutinized using ImageJ analysis software (version 1.47, Bethesda, MD, United States). Villus height (VH) and crypt depth (CD) were measured from 10 random villi in each section. Afterward, the VH-to-CR ratio (VH/CD) was calculated. The final result was expressed as the average of 10 measurements for statistical analysis.
Biochemical Assay of Jejunum
Jejunum tissue samples were homogenized, and then the homogenates were centrifuged at 4,000 rpm for 20 min at 4°C. Jejunal protease (No. A080-2), lipase (No: A054-1-1), and amylase (No. C016-1-1) activities were determined with diagnostic kits purchased from Nanjing Jiancheng Biotechnology Institute (Nanjing, China), and secreted immunoglobulin A (sIgA) levels were detected with ELISA kits (MLBIO Co., Ltd., Shanghai, China) according to the manufacturer’s instructions. The results were normalized to the protein concentration in each jejunal homogenate.
RNA Isolation and Quantitative Polymerase Chain Reaction
Gene expression in the jejunum was assessed using quantitative real-time polymerase chain reaction (PCR) with SYBR Green I labeling. Total RNA from the jejunum (n = 6) was extracted using TRIzol (Invitrogen Biotechnology Inc., CA, United States) according to the manufacturer’s protocols. Extracted RNA was dissolved in RNase-free water and quantified with a DeNovix spectrophotometer (DS-11, DeNovix Inc., Wilmington, DE, United States). The samples with A260/A280 ratios of 1.8–2.0 and A260/A230 ratios of 2.0–2.2 were chosen for subsequent PCR. RNA integrity was assessed through 1% agarose gel electrophoresis. Reverse transcription of total RNA was performed using the PrimeScript® RT reagent kit with gDNA Eraser (RR047A, Takara Bio Inc., Dalian, China). Real-time PCR was carried out using an Applied Biosystems 7500 Real-time PCR System (Applied Biosystems, Foster, CA, United States). The reaction procedure was as follows: predenaturation at 95°C for 10 s, denaturing by 40 cycles at 95°C for 5 s, annealing, and extension at 60°C for 34 s at the end. The primer sequences are described in Table 2. SYBR green fluorescence was measured at the end of each cycle to monitor the amount of PCR product, and a standard curve was plotted to calculate the efficiency of the real-time PCR primers. The relative expression of target genes was analyzed by the 2–ΔΔCt method (20) after the normalization of the geometric means of β-actin and glyceraldehyde-3-phosphate (GAPDH) expression. Each sample was assayed in triplicate.
16S rRNA Sequencing and Analysis
Samples of the cecal contents were prepared (n = 6). Total DNA was extracted from cecal contents with an E.Z.N.A.® Soil DNA Kit (Omega Bio-Tek, Norcross, GA, United States) according to the manufacturer’s instructions. The quantity of extracted DNA was evaluated with a NanoDrop2000 spectrophotometer (Thermo Fisher Scientific, Wilmington, NC, United States), and integrity was examined by 1% agarose gel electrophoresis. Specific barcodes were synthesized based on the V3–V4 regions of the bacterial 16S rRNA gene. The extracted DNA was used as the template for the PCR amplification of the 16S rRNA V3–V4 variable regions.
The universal primers 338F (5′-ACTCCTACGGGAGGCAGCAG-3′) and 806R (5′-GGACTACHVGGGTWTCTAAT-3′) (21) were incorporated into the PCR system. A 20 μL mixture of 10ng template DNA, 0.4 μL of FastPfu polymerase, 0.8 μL of each primer (5 mM), 2 μL of 2.5 mM dNTPs, and 4 μL of 5 × FastPfu buffer was prepared for PCRs with three replicates per sample. The amplification procedure was established with the Applied Biosystems GeneAmp 9700 system (ABI, United States) as follows: 95°C for 3 min, 95°C for 30 s for 27 cycles, 55°C for 30 s, 72°C for 45 s, and 72°C for 10 min. The PCR products were examined by 2% agarose gel electrophoresis, purified using the AxyPrep DNA gel recovery kit (Axygen Biosciences, Union City, CA, United States), and quantified with a QuantiFluor™-ST blue fluorescence quantitative system (Promega, United States). Libraries were constructed, and paired-end reads were sequenced on the Illumina MiSeq PE300 platform (Illumina, San Diego, CA, United States) from Majorbio Biomedical Technologies Ltd. (Shanghai, China).
The raw sequencing data were quality filtered, trimmed, concatenated, and merged according to FLASH (version 1.2.11)1 overlap relationships to produce the final quality sequence for subsequent analysis. High-quality sequences with ≥97% similarity were classified by UPARSE (version 7.1)2 into identical operational taxonomic units (OTUs). The OTU representative sequence was annotated to species, and the species composition of each sample at different taxonomic levels was counted with the RDP Classifier (version 2.2)3 against the Silva 16S rRNA database (release 119)4 at a confidence threshold of 70%.
Data Statistical Analysis
All data were subjected to a T test in SPSS software (SPSS 26.0, SPSS, Chicago, IL, United States). Data were presented as the mean ± standard error (SE). Differences were considered significantly different at P < 0.05. A tendency was defined at “0.05 < P < 0.1”.
Results
Laying Performance
The egg production rate, qualified rate, average daily egg yield, average egg weight, and feed-to-egg ratio were calculated to evaluate the effects of B. licheniformis DSM5749 on the laying performance of laying hens (Table 3). After 8 weeks of treatment, the egg production rate and average daily egg yield were increased, whereas the feed-to-egg ratio were decreased in the B. licheniformis DSM5749 group compared with those in the control group (P < 0.05). Dietary B. licheniformis DSM5749 did not affect the qualified rate or average egg weight of laying hens (P > 0.05).
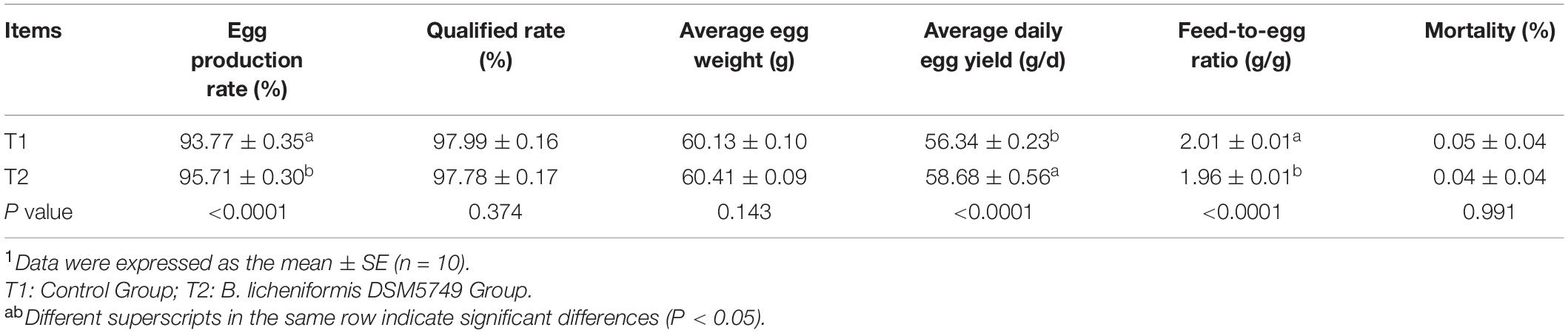
Table 3. Effects of dietary B. licheniformis DSM5749 on the production performance of laying hens at 8 weeks.1
Serum Biochemical Indexes and Immune Parameters
We tested the biochemistry profile and immune parameters in the serum of laying hens, including the contents of GLU, TG, TC, HDL, LDL, IL-1, and IgG, to determine the effects of B. licheniformis DSM5749 on the health status of laying hens (Table 4). On week 8, B. licheniformis DSM5749 reduced the GLU levels (P < 0.05) but had no significant influence on the other indicators in the serum (P > 0.05). Dietary B. licheniformis DSM5749 showed a downregulated level of IL-1 (P < 0.05) and a tendency to upregulate IgG levels (P = 0.094) in the serum of laying hens (P > 0.05).
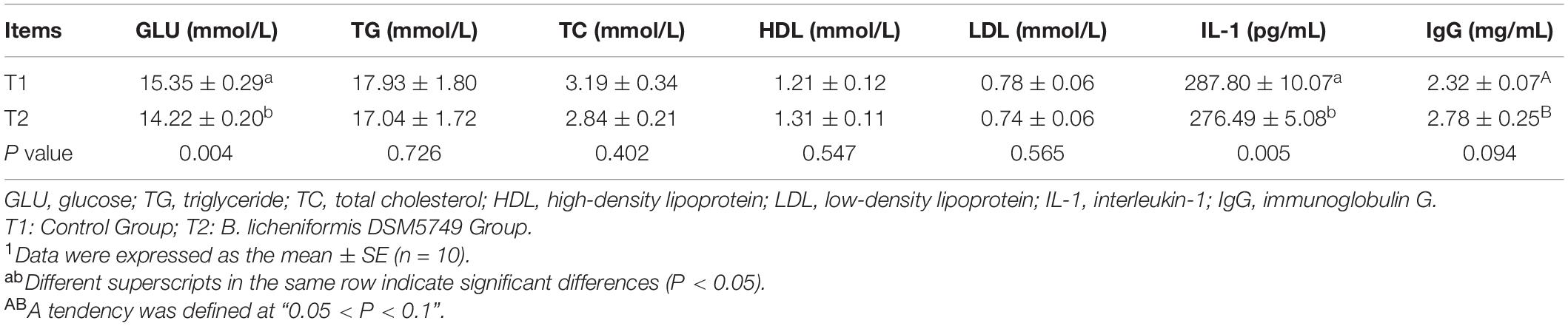
Table 4. Effects of dietary B. licheniformis DSM5749 on the serum biochemical and immunological indicators of laying hens at 8 weeks.1
Hepatic Lipid Metabolism and Antioxidant Indicators
The effects of dietary B. licheniformis DSM5749 on liver antioxidant statuses were shown in Table 5. Liver GSH-Px and SOD concentrations were higher in the B. licheniformis DSM5749-treated group (P < 0.05), whereas TC, TG, and lipase viability in the liver were not affected by dietary B. licheniformis DSM5749 (P > 0.05).

Table 5. Effects of dietary B. licheniformis DSM5749 on the liver lipid metabolism and antioxidant indicators of laying hens at 8 weeks.1
Biochemical Assay of the Jejunum
Digestive enzyme activity, including trypsin activity, lipase viability, amylase activity, and sIgA, were tested to evaluate the digestive enzyme and immunity role of B. licheniformis DSM5749 in laying hens (Table 6). B. licheniformis DSM5749 improved the viability of jejunum lipase in laying hens (P < 0.05) but had no significant influence on the other indicators (P > 0.05).
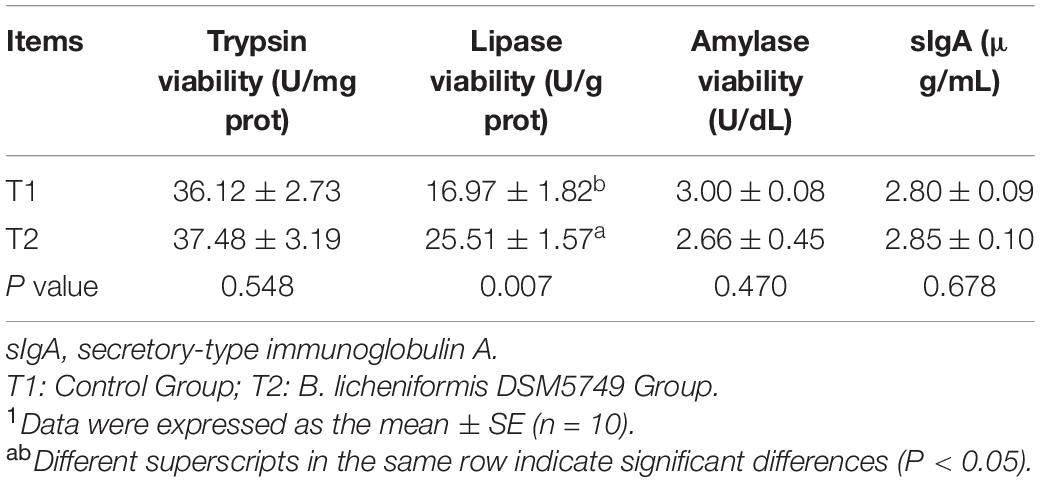
Table 6. Effects of dietary B. licheniformis DSM5749 on intestinal digestive enzyme viability and sIgA of laying hens at 8 weeks.1
Jejunal Intestinal Morphology
Crypt depth, VH, and VH/CD were presented in Table 7 to evaluate the effects of B. licheniformis DSM5749 on jejunal morphological traits. Intestinal morphology analysis demonstrated that VH exhibited positive responses to B. licheniformis DSM5749 (P < 0.05). Moreover, CD and VH/CD showed an increasing tendency in laying hens (P = 0.090, P = 0.056).
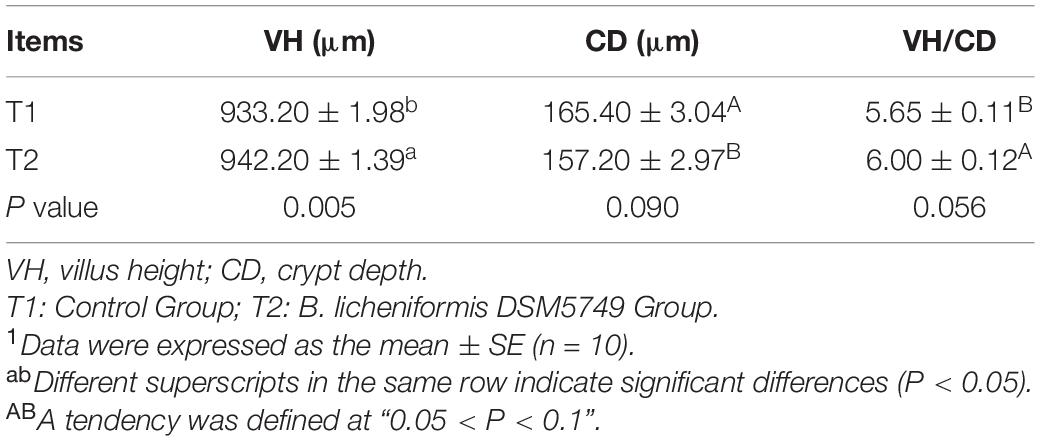
Table 7. Effects of dietary B. licheniformis DSM5749 on the intestinal morphology of laying hens at 8 weeks.1
Intestinal Gene Expression
The expression of genes related to tight junctions was determined in the jejunum by q-PCR to further investigate the molecular basis of the effect of B. licheniformis DSM5749 on the intestinal barrier (Figure 1). As shown in Figure 1, the mRNA levels of ZO-1, Occludin and Claudin-1 were significantly increased in the jejunum (P < 0.05).
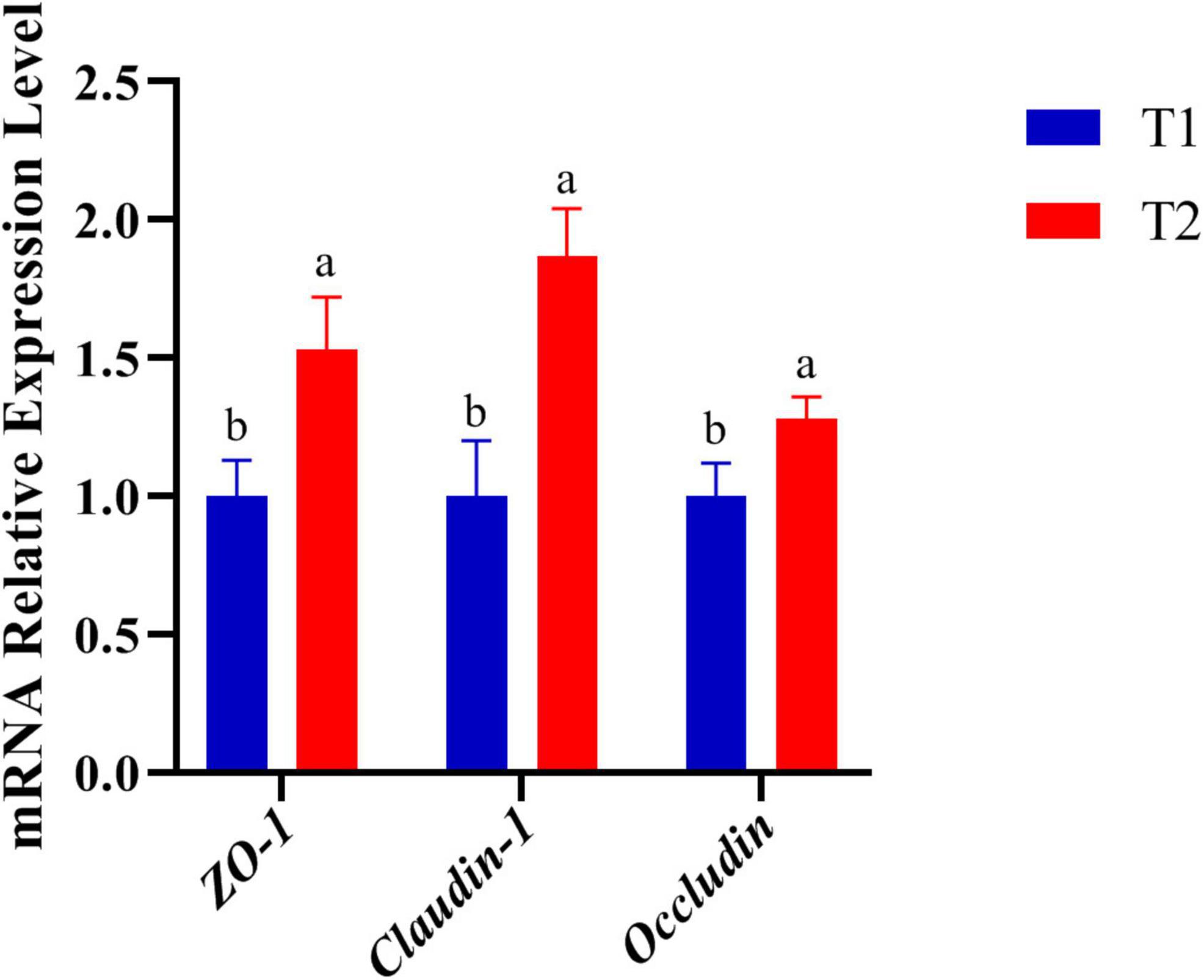
Figure 1. Effects of B. licheniformis DSM5749 supplementation on the physical barrier in the jejunum of laying hens. Total RNA was extracted, and the mRNA levels of ZO-1, Occludin, and Claudin-1 were measured by real-time PCR. GAPDH, glyceraldehyde-3-phosphate; ZO-1, zonula occludens-1. Different letters indicated values significantly different (P < 0.05) among the groups. T1: Control Group; T2: B. licheniformis DSM5749 Group.
Cecal Microbiota Analysis
16S rRNA gene high-throughput sequencing was performed to determine the impact of B. licheniformis DSM5749 on the cecal microbiota of laying hens. The Venn diagram generated after the OTU clustering of effective tags from all samples with 97% consistency showed that the control group contained 2767 OTUs, and the B. licheniformis DSM5749 group contained 2780 OTUs, with 1221 OTUs shared. The control and B. licheniformis DSM5749 groups had 1546 and 1559 unique OTUs, respectively (Figure 2A). A principal coordinate analysis (PCoA) was performed to assess similarities and differences among samples and groups (Figure 2B). The B. licheniformis DSM5749 group revealed reduced similarity in community structure compared to the control group. The results demonstrated that the B. licheniformis DSM5749 diet altered the β diversity index compared to the control diet. B. licheniformis DSM5749 had no significant effect on the cecal microbial alpha diversity of laying hens (P > 0.05, Figure 2C). Figure 3A showed the changes in the relative abundance of species at the phylum level in the cecum of laying hens. The results indicated that the relative abundances of Firmicutes, Bacteroidetes, and Firmicutes/Bacteroidetes (the ratio of Firmicutes to Bacteroidetes was 1:1) were obviously altered among the control and B. licheniformis DSM5749 groups. A reduced proportion of Proteobacteria was observed in the B. licheniformis DSM5749 group (P < 0.05) (Figure 3B). In addition, the Firmicutes/Bacteroidetes ratio decreased in the ceca of 40-week-old laying hens by B. licheniformis DSM5749 (P < 0.05) (Figure 3C). Furthermore, the relative abundances of the 20 predominant genera in each group were analyzed to illustrate the specific changes in microbial taxa (Figure 4A). The results showed that B. licheniformis DSM5749 enriched the abundance of Prevotella (P < 0.05) and decreased those of Faecalibacterium and Desulfovibrio (P < 0.05) in week 40 (Figure 4B).
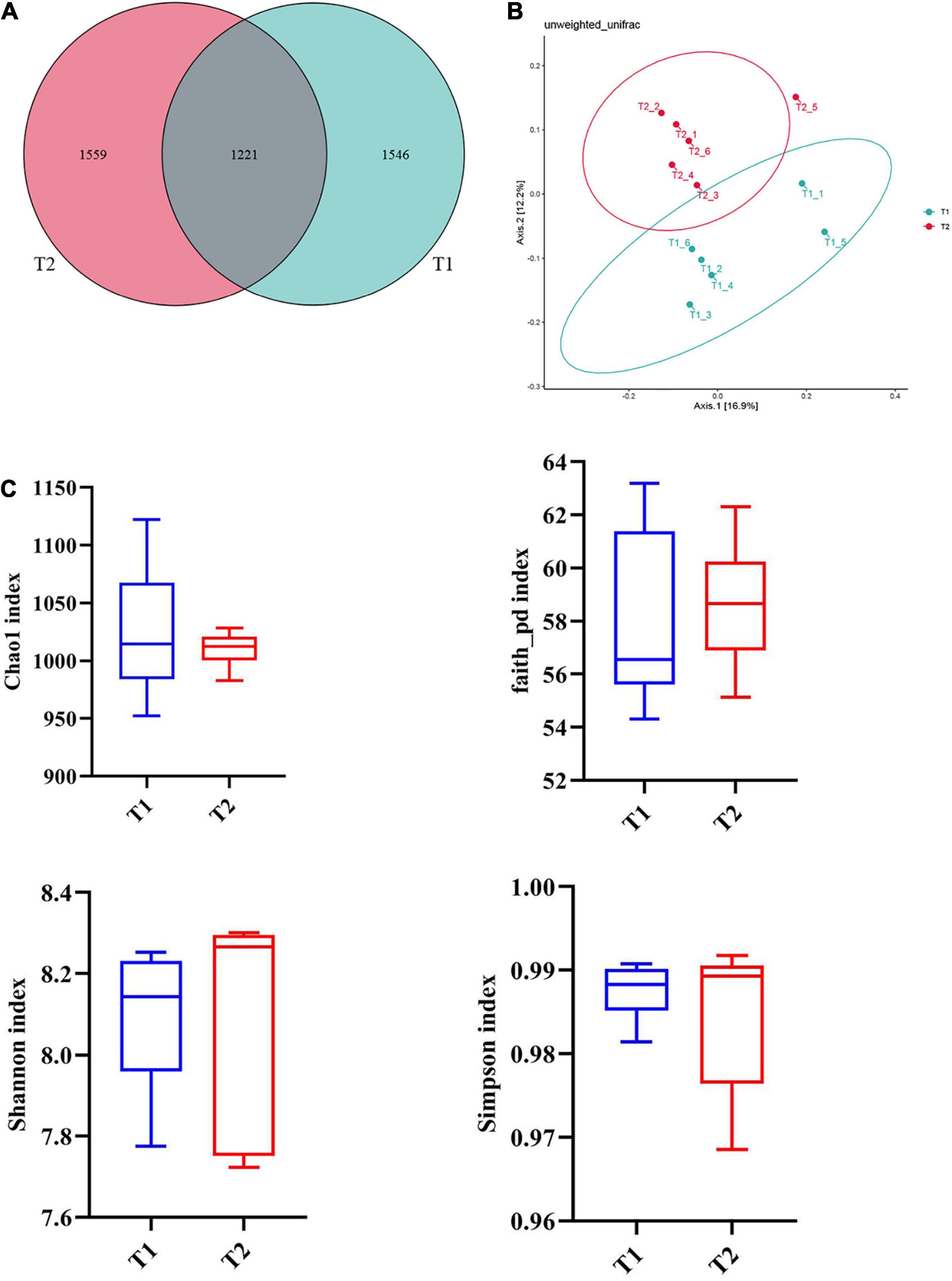
Figure 2. (A) Venn diagram between treatments at the OTU level. (B) PCoA based on unweighted UniFrac distances. (C) An alpha diversity index box graph was established based on the Chao 1, Shannon, Faith_pb, and Simpson indexes. The x-axis represented the group name, and the y-axis represented the alpha diversity index. OTUs, operational taxonomic units. The absence of a superscript on the same row indicated a non-significant difference (P > 0.05). T1: Control Group; T2: B. licheniformis DSM5749 Group.
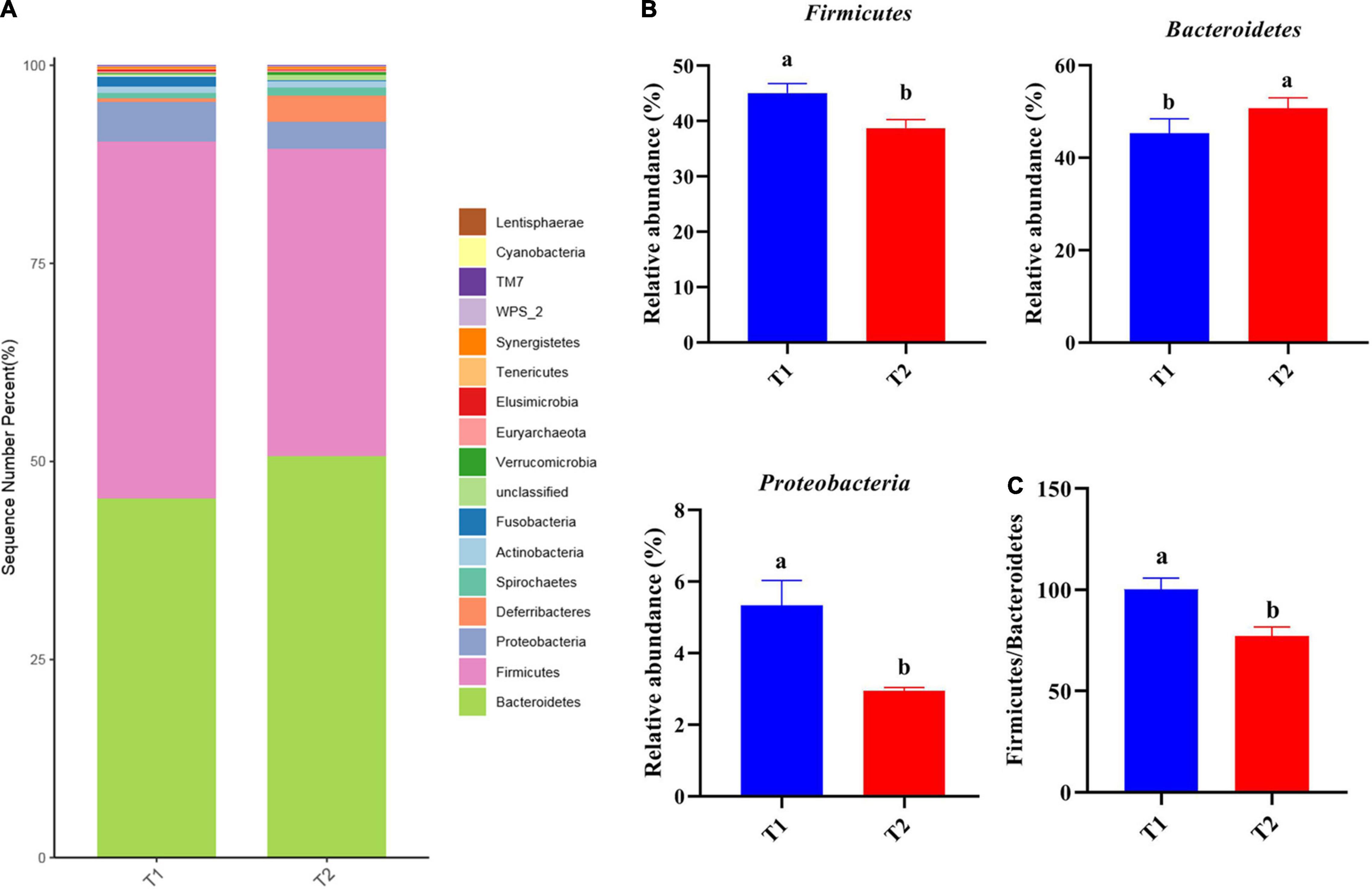
Figure 3. (A) The top 20 phyla in the relative abundance of each group. (B) Relative abundance of differentially abundant bacteria at the phylum level. (C) The ratio of Firmicutes/Bacteroidetes. (A) LEfSe analysis of cecal microbiota. Different superscripts indicated significant differences (P < 0.05). T1: Control Group; T2: B. licheniformis DSM5749 Group.
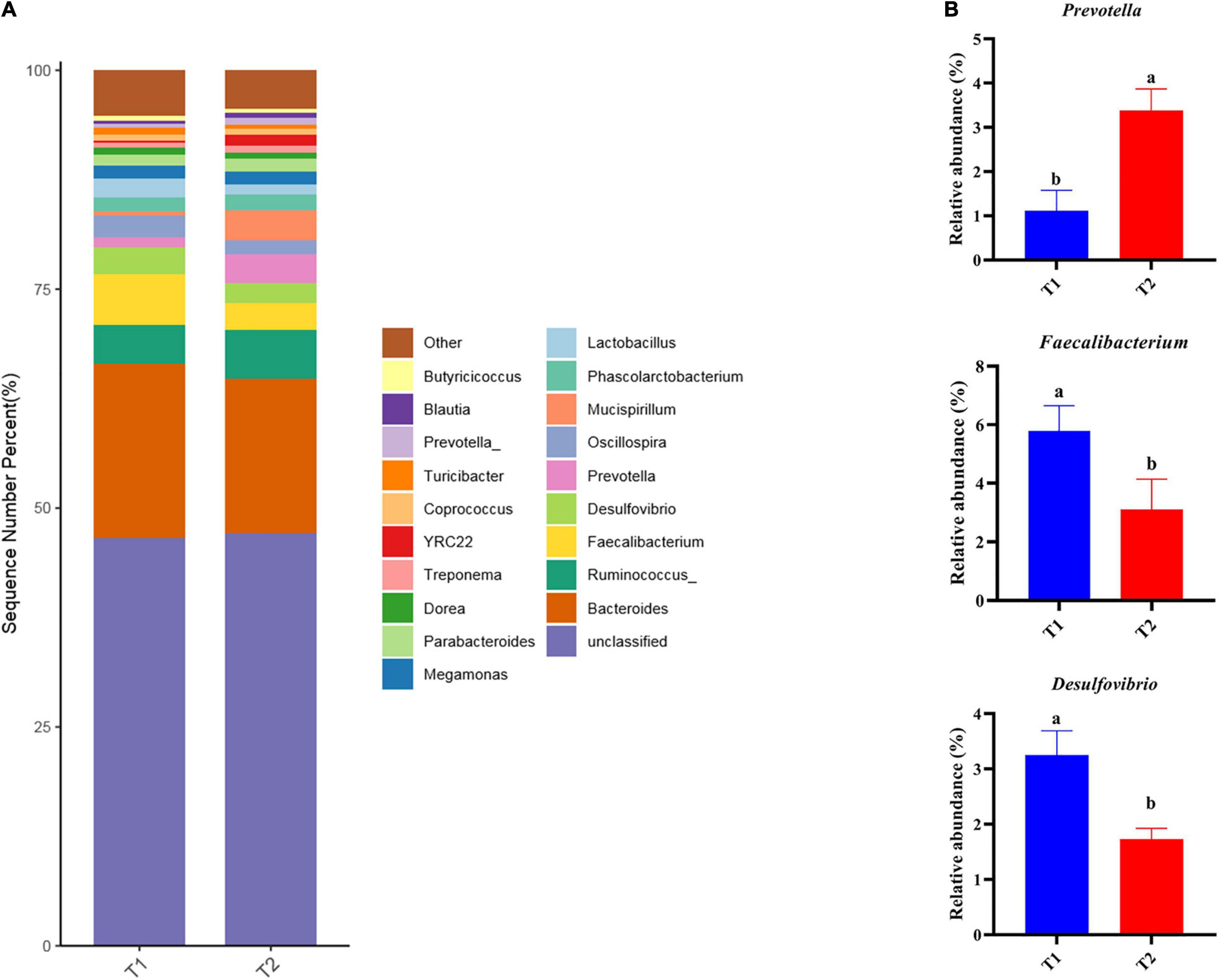
Figure 4. (A) Relative abundance of the top 20 bacterial genera were presented in each group, and the relative abundance differed significantly among groups at the genus level. (B) The relative abundance differed significantly among groups at the genus level. Different superscripts indicated significant differences (P < 0.05). T1: Control Group; T2: B. licheniformis DSM5749 Group.
To further investigate the differences in intestinal flora structure between the different treatment groups, LEfSe analysis was carried out on the cecal contents of the hens, and the results were shown in Figure 5. As seen from the graph, g_Prevotella differed significantly between groups and was significantly enriched in the B. licheniformis DSM5749 group.
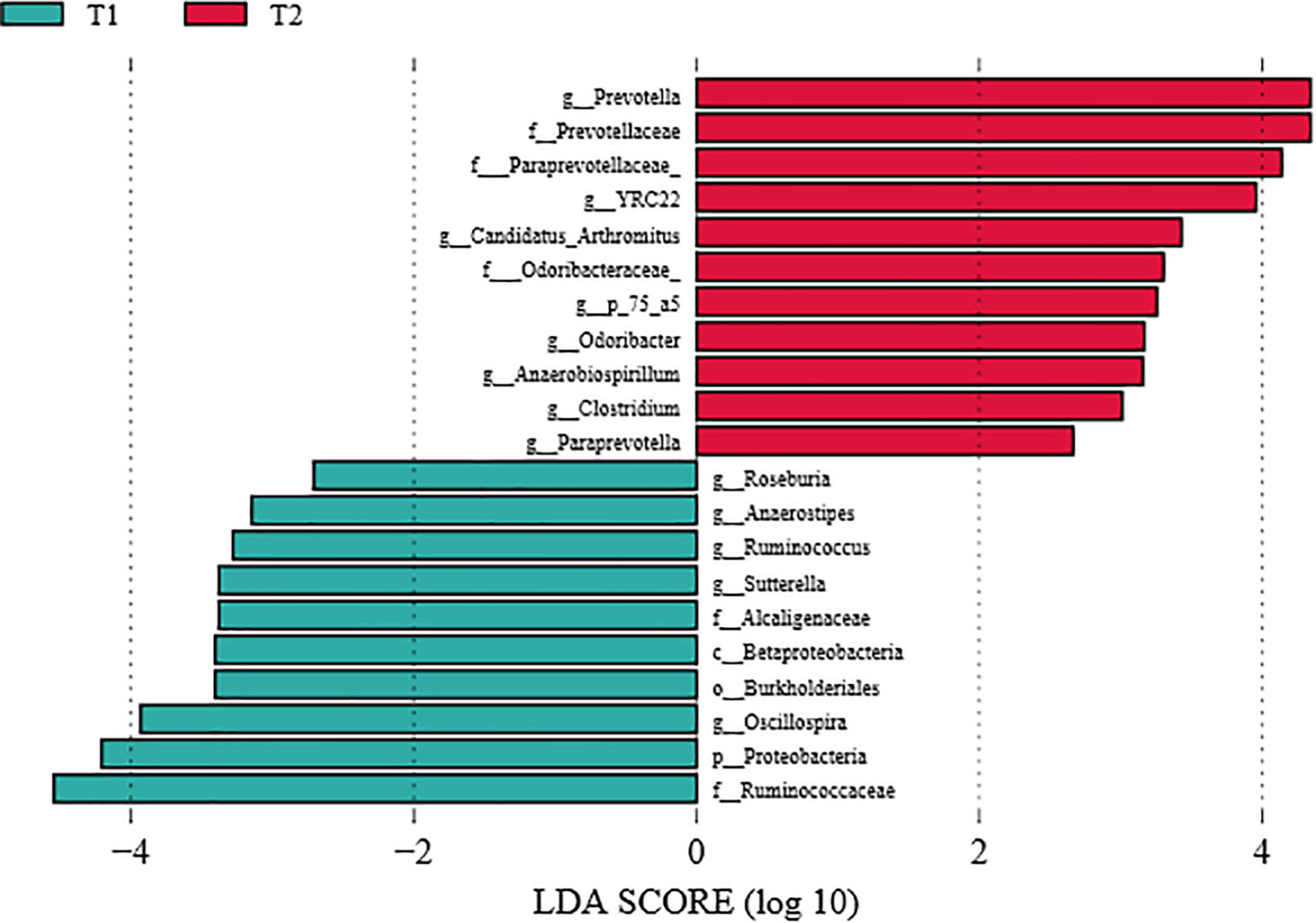
Figure 5. LEfSe analysis of the cecal microbiota of laying hens (LDA score > 4). T1: Control Group; T2: B. licheniformis DSM5749 Group.
Discussion
Production performance is closely related to the economic benefits of laying hens. Probiotics, as safe and green microecological agents, has played an important role in maintaining animal health and performance (22). The use of 0.01% 2 × 1013 CFU/kg B. licheniformis in laying hens was previously found to increase egg production, whereas no significant differences for feed-conversion efficiency (23). In addition, Deng et al. found that 1.0 × 1010 CFU/kg B. licheniformis could attenuate the negative effects of heat stress on the performance of laying hens (19). Recent studies have shown that the laying rates of hens in 0.01% 1.0 × 106CFU/kg B. licheniformis yb-214245 groups were significantly higher than in the control group (24). In the current study, 200g/t 3.2 × 109 CFU/kg B. licheniformis DSM5749 increased the egg production rate and average daily egg yield, which was consistent with previous studies (19, 23–25). In contrast, adding 2.0 g/t of a probiotic consisting of Bacillus subtilis DSM 32324, Bacillus subtilis DSM 32325, and Bacillus amyloliquefaciens DSM 25840 had no remarkable effect on egg production, egg weight, or egg yield (26). Feed to egg ratio are important indicators of egg production (27). In the current study, dietary treatment with 200g/t 3.2 × 109 CFU/kg B. licheniformis DSM5749 effectively decreased feed to egg ratio of laying hens. Conversely, in the broilers, no significant differences were observed in feed: gain (F:G) ratio with dietary 1.5 × 109 CFU/kg B. licheniformis HJDY01 (14). Notably, most research on laying performance has focused on egg production rate and egg quality (19, 23–25). There are scarce reports on the feed-egg ratio we observed in 32-week-old laying hens, which made B. licheniformis DSM5749 distinguishable from previous strains. One hypothesis for enhanced laying performance is related to the production of beneficial metabolites by B. licheniformis, such as extracellular digestive enzymes, lysozyme, antifungal proteins and various antibiotics (14). Increased levels of lipase viability after B. licheniformis DSM5749 treatment may be one of the reasons for the increased laying performance in the present study. In addition, one promising approach is microbial modulation, in which intestinal flora are exploited to enhance laying hens’ immune response to improve production performance (26).
Blood sugar is the main energy supply material of the body and determines the energy supply state of the body and the normal metabolic level of the body. Probiotic preparations can decompose starch and polysaccharides and convert them into monosaccharides, which play a certain role in blood glucose regulation. 1.0 × 106CFU/mL Lactobacillus rhamnosus IMC 501® modulates the expression of genes involved in glucose metabolism and reduces whole organism glucose levels in zebrafish larvae (28). The level of serum glucose were lower in hens fed with 1.0 × 1012CFU/kg probiotic product called PrimaLac® diet (29) and 2.5 × 107 CFU/kg Clostridium butyricum diet (30) compared to those in hens fed with the control diet. In the current study, reduced blood glucose levels were observed in the sera after dietary treatment with 200g/t 3.2 × 109 CFU/kg B. licheniformis DSM5749, which is consistent with previous studies (28–31). One potential mechanism may be due to the decomposition of glycogen or gluconeogenesis in laying hens promoted by probiotics (32). Furthermore, another possible reason for this result is that certain probiotics facilitate the production of short-chain fatty acids (SCFAs), leading to the secretion of incretin hormones, which may influence glucose levels (33). The mechanisms of probiotic regulation of blood glucose are currently unclear, and more human interventions and dynamic measurements of insulin sensitivity are needed. It is also necessary to investigate the treatment effects of specific strain(s) at different durations on insulin resistance.
Immunoglobulin G, a major serum glycoprotein acting as an antibody in the immune system, is a principal component of serum immunoglobulin with the longest residence time in vivo. This multifunctional sugar interacts with various binding proteins through antigen recognition (34). IL-1, as the predominant inflammatory cytokine, mediates many local and systemic features of inflammation and activates IL-1β, which plays a critical role in the occurrence and proliferation of the host response to invasion and is an essential component in triggering an inflammatory response and provoking immune function (35). In the present study, we found that 200g/t 3.2 × 109 CFU/kg dietary B. licheniformis DSM5749 reduced serum IL-1 and showed a gradual increase in IgG levels. Proinflammatory cytokine IL-1 levels decreased in the sera after 5.0 × 106 CFU/kg probiotic treatment with Lactobacillus acidophilus LA5 and Bifidobacterium animalis subsp. lactis BB12 supplementation, which alleviates the systemic inflammatory response in postinfectious (Pi)-irritable bowel syndrome (IBS)-induced mice (36). Moreover, 0.03% B. licheniformis CGMCC 1.3448 administration significantly elevates IgG concentrations but drops the secretion of IL-1β (18). A similar result was observed in this study. The results indicated that 200g/t 3.2 × 109 CFU/kg B. licheniformis DSM5749 was capable of modulating immune function in laying hens. The cell barrier of Bacillus is composed of dextran, which serves as an immune stimulant (37). In addition, previous studies on probiotics have shown that serum IgG, IgM, and IgA showed increased and diversified status in broilers and mice by 1.0 × 109CFU/day Bacillus subtilis BS1 or 4.0 × 109CFU/m2 Bacillus amyloliquefaciens, altering the immune response (38, 39). All these results demonstrated that Bacillus has a positive impact on improving the immune capacity. The immunomodulatory effect of probiotics is attributed to the release of cytokines, including ILs, transforming growth factor (TGF), and interferons (IFNs) (40). Furthermore, it has been established that probiotics increase gut barrier functions by influencing cytokine production (41). However, the effects of probiotics on the immunomodulation of cytokines are strain-specific (41). Therefore, in this study, the immunomodulatory effect of B. licheniformis DSM5749 on laying hens was reflected in the up-regulation of IgG levels and down-regulation of IL-1.
Superoxide dismutase and GSH-Px, as two important antioxidant enzymes in animal organisms, promote antioxidative stress. GSH-Px, a ubiquitous present in vivo, has the capabilities to regulate cellular redox, whereas SOD efficiently converts superoxide anions into hydrogen peroxide, which are degraded to water by GSH-Px (42). A study (23) demonstrated that supplementation with B. licheniformis has no impact on improving the antioxidant enzyme activities in laying hens except for glutathione S-transferase (GST). Notably, the present experiment indicated that dietary treatment with B. licheniformis DSM5749 effectively improved the GSH-Px and SOD capacity in the liver, suggesting that B. licheniformis DSM5749 can decrease lipid peroxidation and enhance the antioxidant capacities of laying hens. These features are beneficial to growth performance (43). Similarly, recent research of broiler chickens found that B. licheniformis HJDY01 increased SOD and GSH-Px activity in the serum (14). Previous studies have summarized that probiotics may regulate the redox status of the host through their metal ion chelating ability, antioxidant enzymes, regulatory signaling pathways, and gut microbiota (44, 45). Among these pathways, antioxidant enzymes (SOD, MnSOD, CAT, GSH, and GSH-Px) seem to be the first line of defense during oxidative stress and have a beneficial impact on preventing oxidative damage in poultry (46). This study confirmed the antioxidant effect of B. licheniformis DSM5749 on laying hens, suggesting that B. licheniformis DSM5749 has potential as a probiotic antioxidant.
The digestive enzymes of poultry consist of protease, amylase, and lipase. Research in broilers found that the activity of digestive enzymes was increased in broilers by supplementation with probiotic B. coagulans NJ0516 (47), which was similarly observed in the present study. In addition, Yang et al. (48) reported that compound-supplemented B. subtilis yb-114,246 and B. licheniformis yb-214,245 improved the activities of chymotrypsin, lipase, and amylase in the digestion of chicken small intestines. The multienzyme digestive system of the intestine provides an environment that promotes nutrient absorption after the administration of B. licheniformis. Such a view explains the results of the present study. The data suggested that B. licheniformis DSM5749 has a lipase production role, making nutrients more digestible and easily absorbed by the intestine (49).
Small intestinal VH and CD are the key factors for determining nutrient digestion and absorption. One study observed an increase in the VH/CD of laying hens fed a diet supplemented with probiotics, which improved nutrient absorption efficiency and laying production (37). The data indicated that B. licheniformis DSM5749 progressively decreased CD while increasing VH and VH/CD of laying hens. The acceleration of VH/CD in the jejunum in the present study supported the idea that B. licheniformis mediates laying production through a competitive space mechanism associated with the attenuation of intestinal pathogenic bacteria (19). B. licheniformis H2 with anti-inflammatory effects restores the damaged intestinal morphology attacked by subclinical necrotic enteritis (SNE) (45, 50). Therefore, the improvement in intestinal integrity can be partially explained by the decreased expression of the proinflammatory cytokine IL-1 in this study. The intestine maintains relatively high integrity of the tight junction barrier during the normal physiological state of the animal organism, and the transport of toxic intraluminal substances and molecules through tight junctions is well regulated (51). Recent reports have demonstrated that B. licheniformis CGMCC 1.3448 enhances epithelial barrier integrity in laying hens (18) and B. licheniformis B26 in broiler chickens (52), characterized by the increased expression of ZO-1, Occludin and Claudin-1 at the mRNA level in the gut. A study suggested that B. licheniformis preadministration alleviated intestinal injury and improved gut barrier function during and after heat stroke (HS) onset (53). This research indicated that B. licheniformis maintained the mucosal barrier and intestinal health. Similarly, in this study, dietary B. licheniformis DSM5749 effectively upregulated ZO-1, Occludin, and Claudin-1 expression, demonstrating the beneficial effect of B. licheniformis DSM5749 on the intestinal barrier of laying hens.
The balance of microflora in the chicken gut has physiological importance to host health and performance (54). The cecum is the predominant location for microbial fermentation and is the most sought-after part of the chicken gut for research. In this study, the impact of B. licheniformis DSM5749 on the intestinal health of laying hens was investigated by analyzing changes in the microbial composition of the cecum at the phylum and genus levels based on species annotation. At the phylum level, Firmicutes and Bacteroidetes accounted for the largest proportion (>80%) of the total microbial community in the ceca of laying hens, which was consistent with previous findings (55). Proteobacteria are often overrepresented in metabolic disorders and inflammatory bowel disease, mostly with an inflammatory phenotype (55). A common trait of Proteobacteria is Gram-negative staining and, thus, the presence of lipopolysaccharide in the outer membrane (56). Carvalho et al. found that mice progressing to colitis showed a definite microbiota signature characterized by increased levels of Proteobacteria, especially of the Escherichia genus (57). The present study demonstrated that the cecal microbiota in B. licheniformis DSM5749-treated laying hens had a reduced relative abundance of Proteobacteria, suggesting that B. licheniformis DSM5749 may have the potential to reduce the incidence of inflammatory diseases in laying hens. This result supports a reduction in serum IL-1 levels in laying hens. An increase in the Firmicutes/Bacteroidetes ratio is the pattern of an impaired intestinal barrier. This leads to the stimulation of regulatory T cells, transport of carbohydrates, and bacterial chemotaxis (58). In the experiment, B. licheniformis DSM5749 showed a decreased Firmicutes/Bacteroidetes ratio in laying hens, which may be the potential reason for the enhanced intestinal barrier. To our knowledge, the decreased level of Firmicutes/Bacteroidetes ratio regulated by B licheniformis in laying hens’ cecum has been the first-time reported. In addition, the Firmicutes/Bacteroidetes ratio is positively associated with fat storage and is remarkably higher in obese individuals than in adults with normal weight (59). This result may be due to the strong fermentation capacity of Firmicutes, which is more suitable as an energy source than Bacteroides and can produce more short-chain fatty acids and metabolic lipids that contribute to efficient heat absorption and subsequent weight gain (60). In terms of the relative abundance of species at the genus level, B. licheniformis DSM5749 increased the amount of Prevotella, which contributes to the fermentation of indigestible carbohydrates to butyrate, protein decomposition, and carbon hydration and is capable of promoting the repertoire of liver glycogen in mice (6). This finding indicates that B. licheniformis DSM5749 can elevate the decomposition of macromolecular substances, such as protein, in laying hens and accelerate nutrient absorption. There is increasing interest in Faecalibacterium, one of the most abundant bacterial species found in the gut, given its potentially important role in promoting gut health. The most notable species in the Faecalibacterium genus is Faecalibacterium prausnitzii (F. prau). There is evidence that the A2-165 strain of F. prau has been found to induce a distinct cytokine response, with high IL-10 secretion compared to other F. prau strains tested, which is partially explained by higher butyrate production (61). Moreover, experiments on Caco-2 cells showed protective effects at 2 mM butyrate and detrimental effects at 8 mM butyrate (62). Therefore, F. prau, a highly abundant butyrate-producing bacterium, has been proposed both as a biomarker for the development of different gut pathologies and as a potential treatment due to its production of anti-inflammatory metabolites; however, studies on forms of administration, and mechanisms of action are still necessary to improve our understanding of the most appropriate use of this bacterium (63). In the present study, the relative abundance of Faecalibacterium was lower in the B. licheniformis DSM5749 group, which was consistent with previous findings (16). It has been reported that the abundance of F. prausnitzii is positively correlated with fasting glucose levels (64), which may be the potential reason for decreased blood glucose levels. A prominent peculiarity of Desulfovibrio has been reported is the production of hydrogen sulfide (H2S), which is anomerized for sulfate reduction using sulfate as the electron acceptor for respiration (65). H2S affects cell signaling in neuronal cells at low concentrations but causes severe toxicities at high concentrations. In addition, Desulfovibrio is positively correlated with the amount of H2S, which promotes intestinal health by inhibiting the oxidation of short-chain fatty acids, such as butyrate, and disrupting the H2S detoxification pathway in intestinal epithelial cells (66). In a recent study of B. licheniformis HJDY01 in broiler, no significant differences in the relative abundance of Desulfovibrio were demonstrated (14). Interestingly, in this study, the cecal microbiota in the B. licheniformis DSM5749-treated laying hens presented a decreased level of Desulfovibrio, which means that dietary B. licheniformis DSM5749 can maintain intestinal health by reducing the colonization of harmful bacteria.
Conclusion
In summary, under the conditions of this experiment, dietary B. licheniformis DSM5749 has growth-promoting, antioxidant, and anti-inflammatory properties, strengthens the physical barrier function of the intestine by exhibiting a higher expression of the tight junction protein, and induces a healthier microbiota composition characterized by a higher ratio of beneficial bacteria and a lower ratio of harmful bacteria. Therefore, our study demonstrated that B. licheniformis DSM5749 could be a potential alternative to antibiotics to enhance the growth performance and maintain the intestinal microecological balance of laying hens, further expanding the resources of strains of laying hen probiotics.
Data Availability Statement
The datasets presented in this study can be found in online repositories. The names of the repository/repositories and accession number(s) can be found here: https://www.ncbi.nlm.nih.gov/bioproject/797943.
Ethics Statement
The animal study was reviewed and approved by Ethics Committee of the Shandong Agricultural University.
Author Contributions
XP designed the experiments and wrote the manuscript. LK performed the in vivo experiments. XP, LK, and QZ collected the sample and data. YC analyzed the data. ZS reviewed and modified the final manuscript. All authors read and approved the final manuscript.
Funding
This work was supported by the Shandong Province Agricultural Industry Technology (SDAIT-11-08) and the Natural Science Foundation of Shandong Province (ZR2020MC170).
Conflict of Interest
The authors declare that the research was conducted in the absence of any commercial or financial relationships that could be construed as a potential conflict of interest.
Publisher’s Note
All claims expressed in this article are solely those of the authors and do not necessarily represent those of their affiliated organizations, or those of the publisher, the editors and the reviewers. Any product that may be evaluated in this article, or claim that may be made by its manufacturer, is not guaranteed or endorsed by the publisher.
Footnotes
- ^ https://ccb.jhu.edu/software/FLASH/index.shtml
- ^ http://www.drive5.com/uparse/
- ^ http://sourceforge.net/projects/rdp-classifier/
- ^ http://www.arb-silva.de
References
1. Mehdi Y, Létourneau-Montminy MP, Gaucher ML, Chorfi Y, Suresh G, Rouissi T, et al. Use of antibiotics in broiler production: global impacts and alternatives. Anim Nutr. (2018) 4:170–8. doi: 10.1016/j.aninu.2018.03.002
2. Quigley EM. Prebiotics and probiotics; modifying and mining the microbiota. Pharmacol Res. (2010) 61:213–8. doi: 10.1016/j.phrs.2010.01.004
3. Butel MJ. Probiotics, gut microbiota and health. Med Mal Infect. (2014) 44:1–8. doi: 10.1016/j.medmal.2013.10.002
4. Rajput IR, Li LY, Xin X, Wu BB, Juan ZL, Cui ZW, et al. Effect of Saccharomyces boulardii and Bacillus subtilis B10 on intestinal ultrastructure modulation and mucosal immunity development mechanism in broiler chickens. Poult Sci. (2013) 92:956–65. doi: 10.3382/ps.2012-02845
5. Nahashon SN, Nakaue HS, Mirosh LW. Production variables and nutrient retention in single comb White Leghorn laying pullets fed diets supplemented with direct-fed microbials. Poult Sci. (1994) 73:1699. doi: 10.3382/ps.0731699
6. Patel R, DuPont HL. New approaches for bacteriotherapy: prebiotics, new-generation probiotics, and synbiotics. Clin Infect Dis. (2015) 60:S108–21. doi: 10.1093/cid/civ177
7. Xiang Q, Wang C, Zhang H, Lai W, Wei H, Peng J. Effects of different probiotics on laying performance, egg quality, oxidative status, and gut health in laying hens. Animals. (2019) 9:1110. doi: 10.3390/ani9121110
8. Mikulski D, Jankowski J, Naczmanski J, Mikulska M, Demey V. Effects of dietary probiotic (Pediococcus acidilactici) supplementation on performance, nutrient digestibility, egg traits, egg yolk cholesterol, and fatty acid profile in laying hens. Poult Sci. (2012) 91:2691–700. doi: 10.3382/ps.2012-02370
9. Bai SP, Wu AM, Ding XM, Lei Y, Bai J, Zhang KY, et al. Effects of probiotic-supplemented diets on growth performance and intestinal immune characteristics of broiler chickens. Poult Sci. (2013) 92:663–70. doi: 10.3382/ps.2012-02813
10. Jeżewska-Fra̧ckowiak J, Seroczynska K, Banaszczyk J, Wozniak D, Skowron M, Ozog A, et al. Detection of endospore producing Bacillus species from commercial probiotics and their preliminary microbiological characterization. J Environ Biol. (2017) 38:1435–40. doi: 10.22438/jeb/38/6/MRN-478
11. Jeżewska-Fra̧ckowiak J, Seroczyńska K, Banaszczyk J, Jedrzejczak G, Żylicz-Stachula A, Skowron PM. The promises and risks of probiotic Bacillus species. Acta Biochim Pol. (2018) 65:509–19. doi: 10.18388/abp.2018_2652
12. Rozs M, Manczinger L, Vágvölgyi C, Kevei F. Secretion of a trypsin-like thiol protease by a new keratinolytic strain of Bacillus licheniformis. FEMS Microbiol Lett. (2001) 205:221–4. doi: 10.1111/j.1574-6968
13. Chen YC, Yu YH. Bacillus licheniformis-fermented products improve growth performance and the fecal microbiota community in broilers. Poult Sci. (2020) 99:1432–43. doi: 10.1016/j.psj.2019.10.061
14. Xu Y, Yu Y, Shen Y, Li Q, Lan J, Wu Y, et al. Effects of Bacillus subtilis and Bacillus licheniformis on growth performance, immunity, short-chain fatty acid production, antioxidant capacity, and cecal microflora in broilers. Poult Sci. (2021) 100:101358. doi: 10.1016/j.psj.2021.101358
15. Xu S, Lin Y, Zeng D, Zhou M, Zeng Y, Wang H, et al. Bacillus licheniformis normalize the ileum microbiota of chickens infected with necrotic enteritis. Sci Rep. (2018) 8:1744. doi: 10.1038/s41598-018-20059-z
16. Lin KH, Yu YH. Evaluation of Bacillus licheniformis-fermented feed additive as an antibiotic substitute: effect on the growth performance, diarrhea incidence, and cecal microbiota in weaning piglets. Animals (Basel). (2020) 10:1649. doi: 10.3390/ani10091649
17. Duc le H, Hong HA, Barbosa TM, Henriques AO, Cutting SM. Characterization of Bacillus probiotics available for human use. Appl Environ Microbiol. (2004) 70:2161–71. doi: 10.1128/AEM.70.4.2161-2171.2004
18. Wang Y, Du W, Lei K, Wang B, Wang Y, Zhou Y, et al. Effects of dietary Bacillus licheniformis on gut physical barrier, immunity, and reproductive hormones of laying hens. Probiotics Antimicrob Proteins. (2017) 9:292–9. doi: 10.1007/s12602-017-9252-3
19. Deng W, Dong XF, Tong JM, Zhang Q. The probiotic Bacillus licheniformis ameliorates heat stress-induced impairment of egg production, gut morphology, and intestinal mucosal immunity in laying hens. Poult Sci. (2012) 91:575–82. doi: 10.3382/ps.2010-01293
20. Livak KJ, Schmittgen TD. Analysis of relative gene expression data using real-time quantitative PCR and the 2 (-Delta Delta C(T)) Method. Methods. (2001) 25:402–8. doi: 10.1006/meth.2001.1262
21. Fadrosh DW, Ma B, Gajer P, Sengamalay N, Ott S, Brotman RM, et al. An improved dual-indexing approach for multiplexed 16S rRNA gene sequencing on the Illumina MiSeq platform. Microbiome. (2014) 2:6. doi: 10.1186/2049-2618-2-6
22. Abd El-Hack ME, El-Saadony MT, Shafi ME, Qattan SYA, Batiha GE, Khafaga AF, et al. Probiotics in poultry feed: a comprehensive review. J Anim Physiol Anim Nutr (Berl). (2020) 104:1835–50. doi: 10.1111/jpn.13454
23. Lei K, Li YL, Yu DY, Rajput IR, Li WF. Influence of dietary inclusion of Bacillus licheniformis on laying performance, egg quality, antioxidant enzyme activities, and intestinal barrier function of laying hens. Poult Sci. (2013) 92:2389–95. doi: 10.3382/ps.2012-02686
24. Yang J, Zhan K, Zhang M. Effects of the use of a combination of two Bacillus species on performance, egg quality, small intestinal mucosal morphology, and cecal microbiota profile in aging laying hens. Probiotics Antimicrob Proteins. (2020) 12:204–13. doi: 10.1007/s12602-019-09532-x
25. Upadhaya SD, Rudeaux F, Kim IH. Efficacy of dietary Bacillus subtilis and Bacillus licheniformis supplementation continuously in pullet and lay period on egg production, excreta microflora, and egg quality of Hyline-Brown birds. Poult Sci. (2019) 98:4722–8. doi: 10.3382/ps/pez184
26. Khan S, Chousalkar KK. Functional enrichment of gut microbiome by early supplementation of Bacillus based probiotic in cage free hens: a field study. Anim Microbiome. (2021) 3:50. doi: 10.1186/s42523-021-00112-5
27. Geng AL, Zhang Y, Zhang J, Wang HH, Chu Q, Liu HG. Effects of lighting pattern and photoperiod on egg production and egg quality of a native chicken under free-range condition. Poult Sci. (2018) 97:2378–84. doi: 10.3382/ps/pey104
28. Falcinelli S, Rodiles A, Unniappan S, Picchietti S, Gioacchini G, Merrifield DL, et al. Probiotic treatment reduces appetite and glucose level in the zebrafish model. Sci Rep. (2016) 6:18061. doi: 10.1038/srep18061
29. Tang SGH, Sieo CC, Ramasamy K, Saad WZ, Wong HK, Ho YW. Performance, biochemical and haematological responses, and relative organ weights of laying hens fed diets supplemented with prebiotic, probiotic and synbiotic. BMC Vet Res. (2017) 13:248. doi: 10.1186/s12917-017-1160-y
30. Zhan HQ, Dong XY, Li LL, Zheng YX, Gong YJ, Zou XT. Effects of dietary supplementation with Clostridium butyricum on laying performance, egg quality, serum parameters, and cecal microflora of laying hens in the late phase of production. Poult Sci. (2019) 98:896–903. doi: 10.3382/ps/pey436
31. Liu M, Tang L, Hu C, Huang Z, Sun B, Lam JCW, et al. Antagonistic interaction between perfluorobutane sulfonate and probiotic on lipid and glucose metabolisms in the liver of zebrafish. Aquat Toxicol. (2021) 237:105897. doi: 10.1016/j.aquatox.2021.105897
32. Falcinelli S, Rodiles A, Hatef A, Picchietti S, Cossignani L, Merrifield DL, et al. Influence of probiotics administration on gut microbiota core: a review on the effects on appetite control, glucose, and lipid metabolism. J Clin Gastroenterol. (2018) 52:S50–6. doi: 10.1097/MCG.0000000000001064
33. Kim YA, Keogh JB, Clifton PM. Probiotics, prebiotics, synbiotics and insulin sensitivity. Nutr Res Rev. (2018) 31:35–51. doi: 10.1017/S095442241700018X
34. Yagi H, Yanaka S, Kato K. Structure and dynamics of immunoglobulin G glycoproteins. Adv Exp Med Biol. (2018) 1104:219–35. doi: 10.1007/978-981-13-2158-0_11
35. Boraschi D, Bossu P, Macchia G, Ruggiero P, Tagliabue A. Structure-function relationship in the IL-1 family. Front Biosci. (1996) 1:d270–308. doi: 10.2741/a132
36. Hong KB, Seo H, Lee JS, Park Y. Effects of probiotic supplementation on post-infectious irritable bowel syndrome in rodent model. BMC Complement Altern Med. (2019) 19:195. doi: 10.1186/s12906-019-2610-9
37. Abdelqader A, Irshaid R, Al-Fataftah AR. Effects of dietary probiotic inclusion on performance, eggshell quality, cecal microflora composition, and tibia traits of laying hens in the late phase of production. Trop Anim Health Prod. (2013) 45:1017–24. doi: 10.1007/s11250-012-0326-7
38. Luan SJ, Sun YB, Wang Y, Sa RN, Zhang HF. Bacillus amyloliquefaciens spray improves the growth performance, immune status, and respiratory mucosal barrier in broiler chickens. Poult Sci. (2019) 98:1403–9. doi: 10.3382/ps/pey478
39. Li A, Wang Y, Li Z, Qamar H, Mehmood K, Zhang L, et al. Probiotics isolated from yaks improves the growth performance, antioxidant activity, and cytokines related to immunity and inflammation in mice. Microb Cell Fact. (2019) 18:112. doi: 10.1186/s12934-019-1161-6
40. Savan R, Sakai M. Genomics of fish cytokines. Comp Biochem Physiol Part D Genomics Proteomics. (2006) 1:89–101. doi: 10.1016/j.cbd.2005.08.005
41. Azad MAK, Sarker M, Wan D. Immunomodulatory effects of probiotics on cytokine profiles. Biomed Res Int. (2018) 9:1–10. doi: 10.1155/2018/8063647
42. Gökçe Çokal B, Yurtdaş M, Keskin Güler S, Güneş HN, Ataç Uçar C, Aytaç B, et al. Serum glutathione peroxidase, xanthine oxidase, and superoxide dismutase activities and malondialdehyde levels in patients with Parkinson’s disease. Neurol Sci. (2017) 38:425–31. doi: 10.1007/s10072-016-2782-8
43. Liu T, Li C, Zhong H, Feng F. Dietary medium-chain α-monoglycerides increase BW, feed intake, and carcass yield in broilers with muscle composition alteration. Poult Sci. (2021) 100:186–95. doi: 10.1016/j.psj.2020.09.056
44. Liao X, Wu R, Ma G, Zhao L, Zheng Z, Zhang R. Effects of Clostridium butyricum on antioxidant properties, meat quality and fatty acid composition of broiler birds. Lipids Health Dis. (2015) 14:36. doi: 10.1186/s12944-015-0035-0
45. Zhao Y, Zeng D, Wang H, Qing X, Sun N, Xin J, et al. Dietary probiotic Bacillus licheniformis H2 enhanced growth performance, morphology of small intestine and liver, and antioxidant capacity of broiler chickens against Clostridium perfringens-induced subclinical necrotic enteritis. Probiotics Antimicrob Proteins. (2020) 12:883–95. doi: 10.1007/s12602-019-09597-8
46. Zhang L, Bai K, Zhang J, Xu W, Huang Q, Wang T. Dietary effects of Bacillus subtilis fmbj on the antioxidant capacity of broilers at an early age. Poult Sci. (2017) 96:3564–73. doi: 10.3382/ps/pex172
47. Wang Y, Gu Q. Effect of probiotic on growth performance and digestive enzyme activity of Arbor Acres broilers. Res Vet Sci. (2010) 89:163–7. doi: 10.1016/j.rvsc.2010.03.009
48. Yang J, Huang K, Wang J, Wu D, Liu Z, Yu P, et al. Combined use of Bacillus subtilis yb-114,246 and Bacillus licheniformis yb-214,245 improves body growth performance of Chinese Huainan partridge shank chickens by enhancing intestinal digestive profiles. Probiotics Antimicrob Proteins. (2021) 13:327–42. doi: 10.1007/s12602-020-09691-2
49. Patel N, Rai D, Shivam, Shahane S, Mishra U. Lipases: sources, production, purification, and applications. Recent Pat Biotechnol. (2019) 13:45–56. doi: 10.2174/1872208312666181029093333
50. Zhao Y, Zeng D, Wang H, Sun N, Xin J, Yang H, et al. Analysis of miRNA expression in the ileum of broiler chickens during Bacillus licheniformis H2 supplementation against subclinical necrotic enteritis. Probiotics Antimicrob Proteins. (2021) 13:356–66. doi: 10.1007/s12602-020-09709-9
51. Awad WA, Dublecz F, Hess C, Dublecz K, Khayal B, Aschenbach JR, et al. Campylobacter jejuni colonization promotes the translocation of Escherichia coli to extra-intestinal organs and disturbs the short-chain fatty acids profiles in the chicken gut. Poult Sci. (2016) 95:2259–65. doi: 10.3382/ps/pew151
52. Musa BB, Duan Y, Khawar H, Sun Q, Ren Z, Elsiddig Mohamed MA, et al. Bacillus subtilis B21 and Bacillus licheniformis B26 improve intestinal health and performance of broiler chickens with Clostridium perfringens-induced necrotic enteritis. J Anim Physiol Anim Nutr (Berl). (2019) 103:1039–49. doi: 10.1111/jpn.13082
53. Li L, Wang M, Chen J, Xu Z, Wang S, Xia X, et al. Preventive effects of Bacillus licheniformis on heat stroke in rats by sustaining intestinal barrier function and modulating gut microbiota. Front Microbiol. (2021) 12:630841. doi: 10.3389/fmicb.2021.630841
54. Oakley BB, Lillehoj HS, Kogut MH, Kim WK, Maurer JJ, Pedroso A, et al. The chicken gastrointestinal microbiome. FEMS Microbiol Lett. (2014) 360:100–12. doi: 10.1111/1574-6968.12608
55. Zhang G, Wang H, Zhang J, Tang X, Raheem A, Wang M, et al. Modulatory effects of Bacillus subtilis on the performance, morphology, cecal microbiota and gut barrier function of laying hens. Animals (Basel). (2021) 11:1523. doi: 10.3390/ani11061523
56. Rizzatti G, Lopetuso LR, Gibiino G, Binda C, Gasbarrini A. Proteobacteria: a common factor in human diseases. Biomed Res Int. (2017) 8:1–7. doi: 10.1155/2017/9351507
57. Carvalho FA, Koren O, Goodrich JK, Johansson ME, Nalbantoglu I, Aitken JD, et al. Transient inability to manage proteobacteria promotes chronic gut inflammation in TLR5-deficient mice. Cell Host Microbe. (2012) 12:139–52. doi: 10.1016/j.chom.2012.07.004
58. Olejniczak-Staruch I, Cia̧żyńska M, Sobolewska-Sztychny D, Narbutt J, Skibińska M, Lesiak A. Alterations of the skin and gut microbiome in psoriasis and psoriatic arthritis. Int J Mol Sci. (2021) 22:3998. doi: 10.3390/ijms22083998
59. Koliada A, Syzenko G, Moseiko V, Budovska L, Puchkov K, Perederiy V, et al. Association between body mass index and Firmicutes/Bacteroidetes ratio in an adult Ukrainian population. BMC Microbiol. (2017) 17:120. doi: 10.1186/s12866-017-1027-1
60. Krajmalnik-Brown R, Ilhan ZE, Kang DW, DiBaise JK. Effects of gut microbes on nutrient absorption and energy regulation. Nutr Clin Pract. (2012) 27:201–14. doi: 10.1177/0884533611436116
61. Rossi O, van Berkel LA, Chain F, Tanweer Khan M, Taverne N, Sokol H, et al. Faecalibacterium prausnitzii A2-165 has a high capacity to induce IL-10 in human and murine dendritic cells and modulates T-cell responses. Sci Rep. (2016) 6:18507. doi: 10.1038/srep18507
62. Peng L, He Z, Chen W, Holzman IR, Lin J. Effects of butyrate on intestinal barrier function in a Caco-2 cell monolayer model of intestinal barrier. Pediatr Res. (2007) 61:37–41. doi: 10.1203/01.pdr.0000250014.92242.f3
63. Maioli TU, Borras-Nogues E, Torres L, Barbosa SC, Martins VD, Langella P, et al. Possible benefits of Faecalibacterium prausnitzii for obesity-associated gut disorders. Front Pharmacol. (2021) 12:740636. doi: 10.3389/fphar.2021.740636
64. Aguirre de Cárcer D, Cuív PO, Wang T, Kang S, Worthley D, Whitehall V, et al. Numerical ecology validates a biogeographical distribution and gender-based effect on mucosa-associated bacteria along the human colon. ISME J. (2011) 5:801–9. doi: 10.1038/ismej.2010.177
65. Carbonero F, Benefiel AC, Alizadeh-Ghamsari AH, Gaskins HR. Microbial pathways in colonic sulfur metabolism and links with health and disease. Front Physiol. (2012) 3:448. doi: 10.3389/fphys.2012.00448
Keywords: Bacillus licheniformis, production performance, laying hens, intestinal health, microbiota
Citation: Pan X, Cai Y, Kong L, Xiao C, Zhu Q and Song Z (2022) Probiotic Effects of Bacillus licheniformis DSM5749 on Growth Performance and Intestinal Microecological Balance of Laying Hens. Front. Nutr. 9:868093. doi: 10.3389/fnut.2022.868093
Received: 02 February 2022; Accepted: 18 March 2022;
Published: 27 April 2022.
Edited by:
Yeshi Yin, Hunan University of Science and Engineering, ChinaReviewed by:
Neeta Agarwal, Indian Veterinary Research Institute (IVRI), IndiaHengjia Ni, Institute of Subtropical Agriculture (CAS), China
Copyright © 2022 Pan, Cai, Kong, Xiao, Zhu and Song. This is an open-access article distributed under the terms of the Creative Commons Attribution License (CC BY). The use, distribution or reproduction in other forums is permitted, provided the original author(s) and the copyright owner(s) are credited and that the original publication in this journal is cited, in accordance with accepted academic practice. No use, distribution or reproduction is permitted which does not comply with these terms.
*Correspondence: Zhigang Song, naposong@qq.com; zhigangs@sdau.edu.cn