- 1Applied Biomedical Research Center, Mashhad University of Medical Sciences, Mashhad, Iran
- 2Department of Physiology, School of Medicine, Zahedan University of Medical Sciences, Zahedan, Iran
- 3Cardiovascular Diseases Research Center, Birjand University of Medical Sciences, Birjand, Iran
- 4Physiology-Pharmacology Research Center, Research Institute of Basic Medical Sciences, Rafsanjan University of Medical Sciences, Rafsanjan, Iran
- 5Department of Physiology and Pharmacology, School of Medicine, Rafsanjan University of Medical Sciences, Rafsanjan, Iran
- 6Department of Physiology, Faculty of Medicine, Mashhad University of Medical Sciences, Mashhad, Iran
The harmful effects of various noxious agents (NA) are well-known and there are reports regarding the induction of various lung disorders due to exposure to these agents both in animal and human studies. In addition, various studies have shown the effects of natural products (NP) on NA-induced lung disorders. The effects of various NP, including medicinal plants and their derivatives, on lung injury induced by NA, were reviewed in this study. The improving effects of various NP including medicinal plants, such as Aloe vera, Anemarrhena asphodeloides, Avena sativa, Crocus sativus, Curcuma longa, Dioscorea batatas, Glycyrrhiza glabra, Gentiana veitchiorum, Gentiopicroside, Houttuynia cordata, Hibiscus sabdariffa, Hochu-ekki-to, Hippophae rhamnoides, Juglans regia, Melanocarpa fruit juice, Mikania glomerata, Mikania laevigata, Moringa oleifera, Myrtus communis L., Lamiaceae, Myrtle, Mosla scabra leaves, Nectandra leucantha, Nigella sativa, Origanum vulgare L, Pulicaria petiolaris, Paulownia tomentosa, Pomegranate seed oil, Raphanus sativus L. var niger, Rosa canina, Schizonepeta tenuifolia, Thymus vulgaris, Taraxacum mongolicum, Tribulus Terrestris, Telfairia occidentalis, Taraxacum officinale, TADIOS, Xuebijing, Viola yedoensis, Zataria multiflora, Zingiber officinale, Yin-Chiao-San, and their derivatives, on lung injury induced by NA were shown by their effects on lung inflammatory cells and mediators, oxidative stress markers, immune responses, and pathological changes in the experimental studies. Some clinical studies also showed the therapeutic effects of NP on respiratory symptoms, pulmonary function tests (PFT), and inflammatory markers. Therefore, the results of this study showed the possible therapeutic effects of various NP on NA-induced lung disorders by the amelioration of various features of lung injury. However, further clinical studies are needed to support the therapeutic effects of NP on NA-induced lung disorders for clinical practice purposes.
Introduction
The respiratory function of the lung is critical and important for survival because oxygen is a vital molecule for the production of energy that is essential for the life of organisms (1). Studies have shown the effects of exposure to air pollutants on respiratory disorders such as COPD, asthma, and lung cancer (2). Specific alterations of passive and active non-respiratory functions generate functional or anatomical disorders that compromise breathing later. The basic scientific and clinical research of various diseases generated by alterations of these functions can produce knowledge on the pathophysiology, biochemistry, genetics and immunology (3).
Respiratory disorders such as COPD and asthma are related to immune and inflammatory reactions and the status of oxidants which were remarkably enhanced in respiratory disorders (4). Allergic disorders have increased in recent decades due to increased allergens and air pollutants in the environment and workplace (5). In allergic disorders such as skin and respiratory allergies, the reaction of the immune system to exposure and re-exposure to allergens releases allergy-related mediators (6).
Bleomycin (BLM) is a type of antibiotic used for cancer chemotherapy. This drug reduces or stops the growth of cancer cells in the body. It inhibits DNA metabolism and is used as an antineoplastic (anticancer) agent, especially for solid tumors. At high concentrations of the drug, protein production and cellular RNA are also inhibited. It has the least toxic effect on blood-forming tissues and the immune system. Unfortunately, due to the complication of pulmonary fibrosis (PF), the use of this drug is clinically limited (7).
Cadmium (Cd) is a naturally occurring toxic element. Several studies have shown that exposure to Cd from cigarette smoking and occupational resources causes lung disorders. There are reports regarding the involvement of pro-inflammatory chemokines and cytokines, such as interleukins, growth factors, and nuclear factor kappa B (NF-κB), a transcription factor that regulates the expression of genes of cytokines which play an important role in pulmonary fibrosis due to Cd exposure (8, 9).
Studies have shown that dust particles may penetrate deep into the lungs, throat, and airways and cause respiratory disorders. The entry of dust into the lung parenchymal macrophage cells leads to the chemical secretion of chemotaxis and inflammatory mediators, leukotrienes and thromboxane, causing the invasion of inflammatory cells from the vessels to the lung damage area. This process, in turn, stimulates the synthesis of the fibroblasts and causes fibrotic pulmonary parenchyma (10). It was also reported that lipopolysaccharide (LPS) causes lung damage (11) through several inflammatory mechanisms (12).
Paraquat (PQ) causes human or animal toxicity and the lungs are the primitive target organ due to being the main exposed organ to this toxin (13). The effects of PQ on the lung result in lung edema, hypoxia, and lung fibrosis (14, 15). Also, the effects of PQ on interleukin 6 (IL 6) and tumor necrosis factor-α (TNF α) in the macrophages have been reported (16, 17). It was also shown that the mechanisms of pulmonary injuries caused by the PQ are mainly related to the inflammatory and oxidative stress processes (18). Chemical agents such as sulfur mustard (SM) might cause acute and chronic injuries in the lung tissue (19) due to enhanced inflammatory oxidant stress mechanisms (20, 21).
Medicinal herbs are applied for the medical treatment of various disorders (22). People use different products from plant resources traditionally for the treatment of respiratory disorders including asthma and bronchitis (22). Several natural ingredients such as polyphenols, flavonoids, and alkaloids derived from medicinal plants showed potent anticancer activity (23).
Natural products (NP) can be considered as the alternative therapeutic potential for respiratory diseases caused by toxic agents since different inflammatory mediators are involved in these disorders and several NP showed anti-inflammatory effects. Most of the studies are pointing out the effects of NP on the inhibition of NF-κB and MAPK pathways, besides the antioxidant effects associated with these products. However, clinical trials using these compounds are scarce in the literature and the safety and efficacy should be confirmed for further studies.
Since no study has been done on the effect of NP on the noxious agents-induced lung disorders so far, this review article is to present available basic and clinical evidence about the efficacy of the mentioned NP and the herbal constituents in the prevention or treatment of lung disorders induced by the noxious agents-induced similar inflammatory and pathological changes in the lung as induced by BLM, Cd, dust, and LPS in experimental and SM in clinical models. Therefore, the effects of NP and their constituents on noxious agents-induced lung changes were also suggested in the present review to support their effect on lung changes induced by noxious agents in clinical studies.
Method
In this review, the keywords including “chemical agent” and “medicinal plants” or “natural products” and “lung injury” or “respiratory system” were searched on different databases such as Web of Science, PubMed, and Scopus from 1989 to the end of September 2021.
In total, 224 articles were retrieved including 115 duplicates articles. Therefore, 109 articles (14 reviews, 4 book chapters, and 91 original articles) related to the described topic were included in this review article (Figure 1).
Results
Bleomycin-Induced Lung Disorders
Experimental Studies
The prophylactic effect of common walnut (Juglans regia) (150 mg/kg) on rats exposed to BLM was shown by increased glutathione reductase (GR) and catalase (CAT) levels and decreased lung inflammation and apoptosis through regulation of NF-κB activity. The treatment with Juglans regia also causes modulated lung injury through markers of cellular injury including lactate dehydrogenase (LDH), alkaline phosphatase, and reduced glutathione (GSH) (24).
Gentiana veitchiorum treatment decreased collagen VI and improved the lung injury induced by BLM. The treatment with Gentiana veitchiorum also decreased the malondialdehyde (MDA) level and increased the superoxide dismutase (SOD) and GSH activities, which correlated with oxidation resistance and scavenging of free radicals. Finally, Gentiana veitchiorum deceased the inflammatory lung damages through the alleviation of tumor necrosis factor-alpha (TNF-α) expression (25).
In the BLM-induced lung inflammation and pulmonary fibrosis (PF) mouse model, treatment with Juglanin (80 mg/kg), that was mainly divided from the green walnut husks of Juglans mandshuric, improved the survival rate in the treated mice. Also, the PF induced by the BLM was markedly attenuated by Juglanin with the decreasing of the expression of the transforming growth factor-β1 (TGF-β1), metallo-proteinase-9 (MMP-9), α-smooth muscle actin (α-SMA), and collagen I (26).
The treatment with a Japanese herbal medicine, Hochu-ekki-to (TJ-41), in a mouse model of BLM-induced PF, for 2 months before and 1 month after receiving BLM, prevented PF through the modification of the Th1/Th2 imbalance toward the Th2 balance (27). Feitai is a Chinese herb used for the treatment of systemic inflammatory response syndrome (SIRS) and multiple organ dysfunction syndrome (MODS). In the BLM-induced pulmonary fibrosis in rats, feitai blocked lung p38 MAPK, NF-κB65, HIF-1α, p-IκB-α, and TGF-β1 expression, and enhanced the Nrf2 and IkB expression (28).
The administration of Rosmarinus officinalis L. extract (75 mg/kg) protected against the BLM-induced acute lung injury in the animal model via declines in lung edema, septal thickening, alveolar subsidens, hemorrhage, and oxidative stress (29). The findings of the other study showed that apigenin (4, 5, 7-trihydroxyflavone) with doses 10, 15, and 20 mg/kg is a potent anti-inflammatory and antifibrotic agent against the BLM-induced PF (30).
The effects of indirubin, a compound derived from mollusks of the family Muricidae, on the BLM-induced PF were examined by pathological staining, western blot, RT-PCR, and immunofluorescent staining. The treatment with indirubin protected the mice against the BLM-induced PF by alleviated fibroblast differentiation indicating its possible therapeutic effect on PF (31). In a model of PF induced by a single endotracheal injection of BLM, the extract of Nigella sativa (500 mg/kg) was effective for early and late prevention of PF and inflammation (32).
The administration of Raphanus sativus L. var niger (RSN), from the black radish plant, ameliorated the BLM-induced acute lung injury. The post-treatment of rats with intravenously administered RSN (75, 150, 300 mg/kg) protected the lung against the BLM-induced oxidative stress and reduced the number of neutrophils and lymphocytes as well as the IL-6, TNF-α, IL-1β, and TGF-β1 levels (33). Houttuynia cordata (HC) has shown antioxidant activity, free radical scavenging capacity as well as anti-inflammatory and anticancer activities. These results suggested that Houttuynia cordata has a protective effect against BLM-induced PF (34).
The Myrtus communis L. extract (50 mg/kg) effectively inhibited the inflammation and fibrosis of lung parenchyma in a rat model of BLM-induced pulmonary injuries. This impact might be due to the decrement of tissue inflammation and inhibition of oxidative stress (35). The treatment with resveratrol (10 mg/kg), a phenolic compound, prevented the BLM-induced PF in the rats by the suppression of oxidative stress and endothelin-1 (ET-1) expression. The results demonstrated that resveratrol with its potent free radical scavenging and antioxidant properties seems to be a highly promising agent in protecting lung tissue against oxidative damage and in preventing PF due to BLM treatment (36).
Ganoderic acid A has been shown to mitigate the increment in NF-κB p65, TNF-α, and IL-1β and IL-6 mRNA expression, and improved the expression of the anti-inflammatory cytokine IL-10 following the BLM injection. The treatment with ganoderic acid A (25 and 50 mg/kg, for 3 weeks) significantly improved the MPO activity and lung histopathology in the mice. Also, the protective effect of ganoderic acid A may be related to a decrease in TNF-α, IL-1β, IL-6, and MDA and an increment of SOD (37). The gallic acid (75, 150, and 300 mg/kg, for 3 weeks) in an animal model of BLM-induced PF reduced the inflammation process to some extent and could exert its effects through TGF β1/Smad2-signaling pathway and balancing NOX4/factor erythroid-2-related factor 2 (Nrf2) (38). Yin-Chiao-San (YCS), a Kampo medicine, is widely applied for pulmonary diseases. The treatment of rats with YCS (1,000 mg/kg/day, i.v.) protected the lung against the BLM-induced and reduced the lung index, MDA, HP, and TNF-α as well as significantly enhanced the CAT activity (39).
Several reports have evaluated the effects of NP on the BLB-induced lung disorders in experimental models and it is suggested that the herbs and their active ingredients are a promising source of compounds that can play pivotal roles in the alternative adjuvant chemotherapy in reducing the pulmonary fibrosis of BLM. However, clinical trials in this field are not found and should be performed in the future. The therapeutic effects of NP in the BLM-induced lung injury are summarized in Table 1 and Figure 2.
Cadmium-Induced Lung Disorders
Experimental Studies
The administration of Nigella sativa oil (1 ml/kg, i.p.) ameliorated the Cd-induced lung damage with the reduction of histopathological changes in the lung architecture (41). The treatment effects of Tribulus Terrestris against the Cd-induced toxicity in the mice showed that the alcoholic extract of Tribulus Terrestris fruit (200 mg/kg, for 10 days) eliminated the free radicals and increased the antioxidant enzymes expression as well as the down-regulation of pro-inflammatory markers in cellular injuries (42). The anti-inflammatory effects of the phenolic compounds from grape seeds were associated with their regulatory effect on the expression of the pro-inflammatory genes, such as cyclooxygenase and lipoxygenase and also by acting on the NF-κB signaling and MAPK. The findings showed that the phenolic compounds of the grape seeds ameliorate the toxic impacts of Cd in the lung tissue via its free radical scavenging property, antioxidant activity, and antiapoptotic potential (43). In the rabbits challenged with Cd (6 mg/kg, i.p.) and treated with pomegranate seed oil (0.8 ml/kg), a significant decrease in the blood volume and hemoglobin was seen (44). Some herbs and NP exhibited significant protection on Cd-induced respiratory insults in experimental animal models and pre-clinical studies but the clinical studies were not found in this regard. The findings from these studies may lead to new therapeutic development for a new drug for the treatment of Cd-induced respiratory injuries. These studies may also guide other investigators to develop quality NP clinical trials in the future. The effects of NP on Cd-induced lung injury are summarized in Table 2 and Figure 3.
Environmental Dust-Induced Lung Disorders
Experimental Studies
Pneumoconiosis is a lung disease caused by certain kinds of dust particles in the workplace. In rats exposed to an inoculation inside the trachea, coal dust (3 mg/0.3 ml of saline), treatment with Mikania glomerata and Mikania laevigata extracts (100 mg/kg, s.c., for 2 weeks) prevented the increase in the total cell count and LDH activity in the bronchoalveolar lavage fluid (BALF), and diminished the lung inflammatory infiltration induced by the coal dust, as assessed by the histopathologic analyses. These findings suggested that both extracts showed a protective effect on the oxidation of thiol groups (45).
In rats exposed to uranium ore dust inhalation (period of 3, 7, 30, and 60 days), the administration of licorice (Glycyrrhiza glabra) aqueous extract normalized the pyruvic acid contents in the lung tissue during the study periods and decreased the lactic/pyruvic acid ratio (46).
In albino rats exposed to cement dust, the antioxidant activities of roselle (Hibiscus sabdariffa), moringa (Moringa oleifera), ginger (Zingiber officinale), and ‘ugwu' (Telfairia occidentalis), fed with herbal extracts (400 mg/kg) for 6 months were evaluated. The lungs of non-treated rats showed severe interstitial fibrosis and cellular debris. Moderate fibrosis was seen in the lung tissues of the rats treated with Hibiscus sabdariffa and Moringa oleifera extracts. The rats that were fed with the mixture of the extracts had mild septal fibrosis (47).
Treatment with Hibiscus sabdariffa, Moringa oleifera, Zingiber officinale, and Telfairia occidentalis in the rats exposed to cement dust showed moderate to normal biochemical parameters when compared with the non-treated rats. Higher hematological parameters were observed in the treated rats than in the non-treated rats. Overall, the mixture of extracts decreased the adverse effects of cement-dust exposure more than any individual extract alone. Individually, Telfairia occidentalis performed the best, followed by Zingiber officinale, Moringa oleifera, and Hibiscus sabdariffa (48). The elemental analysis of the cement dust shows that it contains 57% calcium, 23% silicon, 10.5% aluminum, 8.5 % chromium, and 8.0 % lead (47). The data of these studies indicated the antioxidant properties of the food plants which modulated the effects of cement dust. Hence, the plants could be used as supportive care in polluted environments to lower the health problems associated with cement-dust exposures. These experimental studies indicated that Hibiscus sabdariffa, Moringa oleifera, Zingiber officinale, Telfairia occidentalis, Mikania glomerata, Mikania laevigata, and Glycyrrhiza glabra might be candidates for the prevention of lung injury caused by swine barn, coal, uranium ore, and cement-dust exposure. These results suggested a potential role for oxidative stress pathways in mediating occupational lung diseases and antioxidants effect of the plants in reducing dust-mediated oxidative stress in lung disorders of exposed workers.
This review study indicates the therapeutic effect of NP on dust-induced lung disorders but more clinical studies are required to establish the clinical efficacy of these plants and their constituents on lung and allergic disorders. The therapeutic effects of NP including anti-inflammatory effects and reduction of airway responsiveness in the animal models of asthma and COPD were also illustrated. Different NP and their components were identified as anti-asthmatic components. We suggest the possible therapeutic effect of NP on lung disorders of dust-exposed patients via the decrement of inflammatory and enhanced anti-inflammatory mediators, and improved pulmonary function tests. The effects of NP on dust-induced lung disorders are summarized in Table 3.
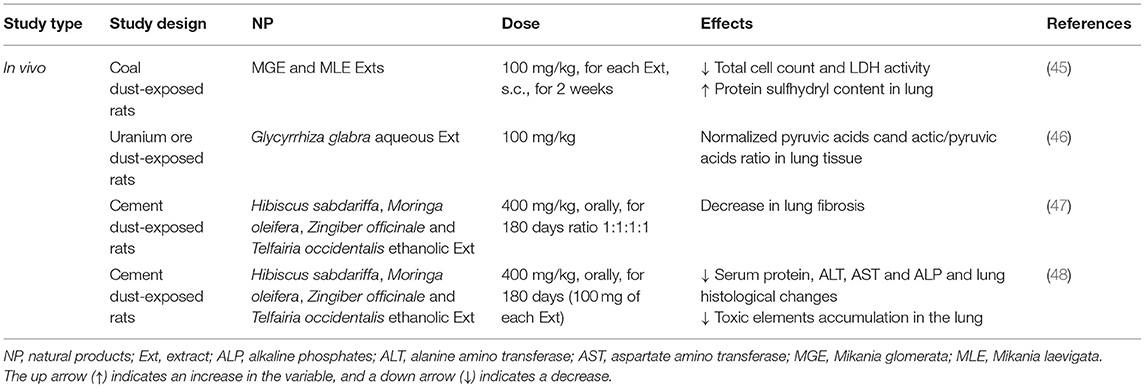
Table 3. The possible therapeutic effects of medicinal herbs in the dust-induced lung disorders in experimental studies.
Lipopolysaccharide-Induced Lung Disorders
Experimental Studies
The administration of the extract of Paulownia tomentosa stem bark (2.5, 5, 10, 20, and 40 μg/ml, for 24 h), represses the release of IL-6 and TNF-α in the RAW 264.7 macrophages stimulated by LPS (49). In this cell line, dehydrodieugenol B from Nectandra leucantha (10, 20, 30, and 60 μM) did not influence the cell viability but hindered the enhancement in IL-1β and IL-6 gene expression and NO release (50).
In vitro, Barbaloin (a major anthraquinone compound) (25, 50, or 100 μM), decreased the expression of TNF-α, IL-1β, and IL-6 as well as the activation of ROS-mediated PI3K/AKT/NF-κB pathway dose-dependently (51). The ethanolic extract of the aerial parts of Houttuynia cordata (30, 50, 100, and 300 μg/mL) also inhibited the iNOS-mediated NO release from the LPS-stimulated MH-S cells (a mouse alveolar macrophage cell line) concentration-dependently (52).
Incubating the RAW264.7 cells with LPS and alpinumisoflavone (1, 5, and 10 μg/mL, for 24 h), a plant-derived pyranoisoflavone remarkably inhibited the release of NO, cytokines, and ICAM-1 protein expression. Treatment with alpinumisoflavone blocked the IκBα phosphorylation and degradation and decreased the phosphorylation of IKK and NF-κB. In addition, it effectively decreased the phosphorylation of ERK, Jc-Jun-NH2 terminal kinase (JNK), and p38. In LPS activation of the NLRP3 inflammasome, caspase-1, and IL-1β proteins were inhibited by alpinumisoflavone, especially at its high dose. The alpinumisoflavone treatment also remarkably decreased the IL-17A and iNOS protein expression but it did not block the LPS-induced cyclooxygenase-2 (COX-2) induction. Furthermore, alpinumisoflavone significantly enhanced the expression of antioxidant enzymes dose-dependently. The LPS induction of intracellular ROS production was also significantly inhibited by the treatment with alpinumisoflavone (53).
The treatment of LPS-stimulated and non-stimulated splenocytes with the aqueous extract of Curcuma longa (0.8–500 μg/mL, for 48 h), remarkably enhanced NO, pro-inflammatory cytokines, tumor necrosis factor, interferon-gamma, and monocyte chemoattractant protein-1(MCP-1). The levels of IL-12 and PGE2 in the LPS-stimulated cells were also inhibited by the plant extract (54). In the LPS-activated epithelial cell line, the levels of NF-κB p52, NF-κB p65 transcription factors protein, IL-1ß, interleukin-8 (IL-8), and mucus secretion were significantly reduced by the hydroalcoholic extract of Thymus vulgaris (0.04–0.60%) (55).
Daidzein (100 μM,), a diphenolic isoflavone 15 min after LPS stimulation, obviously inhibited the expressions of myeloid differentiation factor 88 (MyD88), toll-like receptor 4 (TLR4), and the activation of NF-κB in the A549 alveolar epithelial cells stimulated by 10 μg/ml LPS (56). In the RAW264.7 cells, treatment with a mixture of Taraxacum officinale (a herbal formulation) (0.5, 1, and 2 mg/mL), repressed the LPS (100 ng/mL)-induced inflammatory responses (57). Also, the expression of pro-inflammatory cytokines activated the Nrf2-HO-1 axis and oxidative stress was inhibited in the treated LPS-stimulated cells (57).
Eugenol and dehydrodieugenol B from Nectandra leucantha (30 mg/kg) in the mice with the LPS-induced ALI, decreased lung edema, inflammatory cells, and the IL-6 and IL-1 β levels in the BALF as well as decreased inflammatory cell infiltration and those positive to iNOS, MMP-9, and TIMP-1, and decreased the collagen content and the 8-isoprostane expression in the lung tissue (50).
In LPS-challenged mice, treatment with thymol (30 and 100 mg/kg, i.p.), one of the primary active constituents derived from Thymus vulgaris, before or after the LPS challenge, significantly improved the pathological changes in the lung tissues. Thymol also inhibited the LPS-induced inflammatory cells influx and protein concentration in the BALF. Additionally, thymol markedly inhibited the LPS-induced elevation of MDA and MPO levels as well as reduction of the SOD activity. Thymol also effectively inhibited the NF-κB activation in the lung (58). In the LPS-induced ALI, treatment with methanolic extract of Pulicaria petiolaris (50 and 100 mg/kg, p.o., for 5 days) reduced pulmonary edema, ameliorated the LDH level in the BALF, improved the histopathological lesions in the lung tissue, and showed antioxidant capacity (59).
The administration of a single dose of cannabidiol (0.3, 1.0, 10, 20, 30, 40, and 80 mg/kg, i.p.), extracted from Cannabis sativa, before the LPS-induced ALI, decreased the migration of leukocytes into the lungs, albumin concentration in the BALF, production of pro-inflammatory cytokines and chemokines, and MPO activity in the lung tissue. In addition, in the LPS-induced ALI, ZM241385 (a selective adenosine A2A receptor antagonist), inhibited all anti-inflammatory effects of cannabidiol which indicate the contribution of adenosine A2A receptor in the anti-inflammatory effects of cannabidiol (60).
The linalool (25 mg/kg, i.p.) treatment attenuated the production of LPS—decreased the changes in TNF-α and IL-6 as well as lung histopathologic changes in the ALI mouse model (61). In the mice with the LPS-induced ALI, Barbaloin extracted from Aloe vera ameliorated lung pathological changes such as infiltration of inflammatory cells, alveolar hyperemia, necrosis, and lung epithelial cell detachment (51).
The treatment with the aqueous extract of Taraxacum mongolicum Hand.-Mazz (5 and 10 g/kg, p.o.) inhibited the LPS-induced lung injury in female BALB/c mice by reducing the inflammatory cell infiltration in the BALF, lung protein levels and PI3K/Akt/mTOR signaling. It also improved the activity of SOD and inhibited the MPO activity (62).
The Portulaca oleracea extract (50, 100, and 200 mg/kg, p.o., 1 h before LPS injection) suppressed the LPS-induced rat ALI by decreasing IL-6, IL-β, TNF-α, TGF-β, and, PGE2 but increasing IL-10 levels. Portulaca oleracea improved the levels of the white blood cells (WBC), MDA, MPO, and thiol as well as SOD and CAT activities. The lung wet/dry ratio (an index of interstitial edema) was also significantly reduced. Therefore, the Portulaca oleracea extract displayed antioxidant and anti-inflammatory activity dose-dependently on the LPS-induced ALI model in the rat model (63).
In the LPS-induced ALI, xanthohumol (10 or 50 mg/kg, i.p.), a prenylflavonoid extracted from the hop plants (Humulus lupulus) (0, 18, 35, and 70 μmol/kg, i.p., for 30 min), showed a protective effect against oxidative stress and inflammatory damage by regulation of the Nrf2 pathway through AMPK/GSK3β activation, and suppression of LPS-activated Txnip / NLRP3 inflammation and the NF-κB signaling pathway (64). In LPS-induced lung inflammation in mice, pre-treatment with luteolin (0, 18, 35, and 70 μmol/kg, i.p., for 30 min) decreased IL-6 and TNF-α levels and expression of COX-2 and iNOS. In addition, luteolin represses activation of NFκB and its upstream molecular factor, Akt (65).
The treatment of ALI mice with the ethanolic extract of Glycyrrhiza glabra (200 and 400 mg/kg, p.o., for 4 days) significantly reduced the exudation of protein and the total cell count into the BALF but increased the BALF SOD and CAT activities (66). The alcoholic extract of Anemarrhena asphodeloides decreased the inflammatory cells in the BALF and inhibited lung inflammation by its saponin-enriched fraction. The inflammatory markers in the LPS-induced ALI in mice were significantly inhibited by oral administration of timosaponin A-III (67).
The pre-treatment of mice exposed to LPS with crude extract of Eleusine indica (400 mg/kg) inhibited the lung neutrophil recruitment 98% dose-dependently. Vitexin (8-C-β-glucopyranosylapigenin) and schaftoside (6-C-β-glucopyranosyl-8-C-α-arabinopyranosylapigenin) isolated from aerial parts (400 μg/kg), inhibited lung neutrophil influx, by 62 and 80%, respectively (68). The pre-treatment with astragalin (25, 50, and 75 mg/kg, p.o., 1 h before LPS challenge), a flavonoid from several medicinal plants, decreased inflammatory responses and improved survival in lethal endotoxemia of a murine model of the LPS-induced ALI. The anti-inflammatory effect of astragalin was correlated with the reduction of IL-1, IL-6, and TNF-α levels produced through the inactivation of NF-κB (69).
The Jojoba oil dry (400 mg, i.t., the air flow rate of 60 L/min for the duration of 7 s) nanoemulsion powders indicated more anti-inflammatory effects on the LPS-induced ALI than dexamethasone with a detrimental effect on the total protein content and down-regulation of TNF-α, IL-1β, IL-6, and NF-κB p65 (116).
Among carvone isomers, pre-treatment with D-carvone (25 and 50 mg/kg, i.g., 1 h before LPS challenge), significantly alleviated the LPS-induced lung injury by diminishing the lung wet/dry ratio and the number of inflammatory cells in the BALF. The serum pro-inflammatory cytokines were remarkably decreased in D-carvone-treated mice. The lung histopathological changes in the LPS-induced lung injury were improved by D-carvone. In addition, the comparable effects of D-carvone with those of dexamethasone were seen (70). Myricetin (10, 20, and 40 mg/kg, 30 min after the LPS challenge), a member of the flavonoid class of polyphenolic compounds, significantly decreased lung inflammation by reduction of the lungs' wet/dry weight ratio, protein levels in the BALF, cytokine levels, and migration of the inflammatory cells. The TLR4, MyD88, and NF-κB expressions were also decreased and the activities of MPO, SOD, GPx, and CAT were increased in the mice exposed to LPS (71).
The administration of petroleum ether fraction of Viola yedoensis (2, 4, and 8 mg/kg, p.o.) in the LPS-induced ALI in mice significantly reduced the wet/dry weight ratio of the lung, total inflammatory cells, the activity of MPO, and protein levels in the BALF. The lung morphology improved, the complement deposition was markedly reduced, and the expression of pro-inflammatory cytokines was suppressed in the treated group (72) and pre-treated with rhamnazin (5, 10, and 20 mg/kg, i.p., 2 days before LPS) significantly reduced the inflammatory parameters, improved lung histopathology changes, activated Nrf2 pathway, and attenuated ROS as well as H2O2, MDA, and hydroxyl ion in the LPS-exposed rats (73). In the LPS-induced lung inflammation and oxidative stress model, the administration of Nigella sativa extract (100–400 mg/kg, i.p.) decreased the total and differential WBC counts, oxidative stress, and inflammatory (TGF-β1, IFN-γ, PGE2, and IL-4) markers in the BALF and serum as well as the pathological changes of the lung tissue in the rats (74).
Therefore, the experimental studies showed that pre-treatment with various NP remarkably reduced the inflammatory markers and improved the lung histopathology in the LPS-induced ALI animal models, indicating the therapeutic effect of NP on ALI in the animal models due to their antioxidant and anti-inflammatory properties. The underlying mechanisms of the anti-inflammatory action of NP are inhibition of the Nrf2-mediated antioxidative pathway. Among the biological activities of NP derived from plants anti-inflammatory, antiviral, antitumor, antiallergic, and antioxidant activities can be pointed out. Although many reports have evaluated the effects of these compounds in the experimental models, studies evaluating clinical trials are scarce in the literature. In this section, the effects of different NP on the LPS-induced lung disorders in the experimental models and some possible mechanisms of action were shown. Some experimental data suggest that supplementation with NP may be an effective treatment for patients with the LPS-induced respiratory disorders. The effects of NP on the LPS-induced lung disorders are summarized in Table 4.
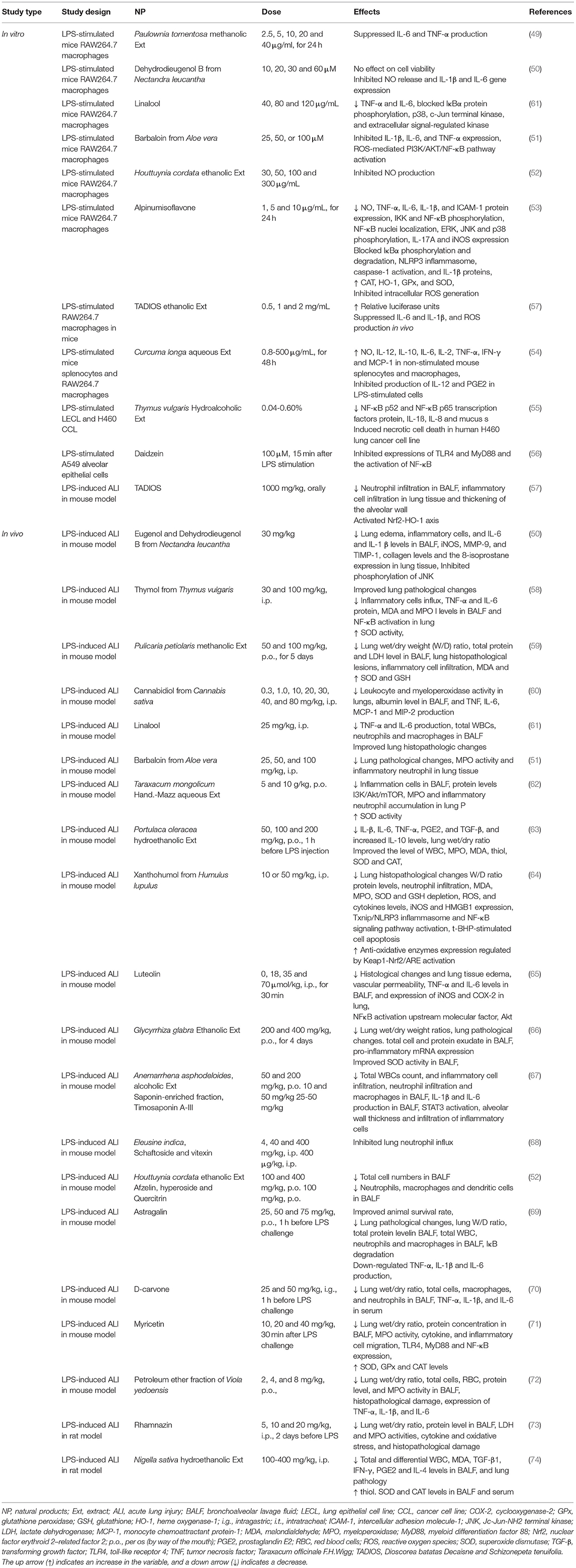
Table 4. The possible therapeutic effects of medicinal herbs in the LPS-induced lung disorders in experimental studies.
Paraquat-Induced Lung Disorders
Experimental Studies
The treatment with Zataria multiflora (200 and 800 mg/kg) and carvacrol (20 and 80 mg/kg) improved the inhaled PQ-induced systemic oxidative stress and inflammation (75). Also, treatment with carvacrol reduced the WBC (total and differential) count, oxidant biomarkers, and inflammatory cytokines, but increased the antioxidants including CAT and SOD, and anti-inflammatory cytokines in the inhaled PQ-exposed rats similar to the effects of pioglitazone and dexamethasone (76).
The administration of the bark extract of Bathysa cuspidata (200 and 400 mg) was shown to protect against PQ-induced acute lung injury and mortality in the rats exposed to PQ as substantiated by the significant decreases in lung edema, septal thickening, alveolar collapse, hemorrhage, cell migration, malondialdehyde, and proteins carbonyl levels (77). In another similar study, salidroside (10 mg/kg), derived from Rhodiola rosea, alleviated the PQ-induced lung injury in the rats via down-regulation of the TGF-β1 expression (78). In an experimental study, the rats were exposed to a single dose of PQ and treated with pioglitazone (5 mg/kg), pioglitazone plus Zataria multiflora extract (200 mg/kg), pioglitazone plus carvacrol (20 mg/kg), and dexamethasone (0.03 mg/kg). The results indicated that the treatment of lung and systemic oxidative stress and inflammation induced by the inhaled PQ in the rats with a combination of pioglitazone plus Zataria multiflora or carvacrol showed more effect than the effect of pioglitazone or the plant and carvacrol alone (79).
In the rats exposed to PQ and treated with Zataria multiflora, the levels of IL-10, IL-4, TNF-α, and IFN-γ were significantly increased and IL-6, IL-8, and IL-2 levels were decreased (80). The treatment with Zataria multiflora (200 and 800 mg/kg) markedly reduced the WBC (total and differential) counts, serum levels of NO2, MDA, IL-17, and TNF-α as well as improved the PQ-induced acute lung injuries (81). Ligustrazine (30 mg/kg, i.g.), an active substance extracted from the Umbelliferae plant Ligusticum chuanxiong (30 mg/kg, i.g.), improved the lipid peroxidation damage, improved the lung injury, and induced the concentrations of NK-κB and iNOS caused by acute poisoning with PQ (82). In the lung injury induced by sub-acute exposure with PQ, treatment with curcumin (30 mg/kg, i.g.) and nano-curcumin-attenuated lung fibrosis may be associated with their antioxidant properties (83).
Diosmin (50 and 100 mg/kg), in a mouse model of PQ-lung injury, showed antioxidant, anti-inflammatory, and antifibrotic effects (84). In a similar study, the PQ-exposed mice treated with the extract of Rosa canina fruits (200 and 400 mg/kg) improved the oxidant-antioxidant balance in the lung tissue (85). The treatment of PQ-exposed mice with apigenin (25, 50, and 100 mg/kg) significantly reduced lung injury by inhibition of oxidative stress and inflammation (86).
The studies have shown that NP have a variety of medicinal activities including anti-inflammatory, antioxidant, and anticancer. Due to their low water solubility, NP are significantly limited in clinical application. Many potential strategies are expected to be developed to improve their pharmacokinetic values and bioavailability. The experimental evidence supports the causal relationship between oxidative stress and various chronic diseases. Thus, numerous studies are focused on ameliorating the PQ-induced lung injury by decreasing oxidative stress. The experimental studies suggest that NP can combat oxidative stress and reduce the morbidity and mortality associated with PQ-induced lung injury. The effects of NP on PQ-induced lung injury are summarized in Table 5 and Figure 4.
Sulfur Mustard-Induced Lung Disorders
Experimental Studies
The aqueous extract of Crocus sativus (225, 450, and 900 μg) decreased DNA damage and MDA but increased the GSH level in the SM-exposed macrophage cells (87).
The effects of antioxidants caffeic acid (CA) (250 μM) and quercetin (100 μM) on normal human epithelial keratinocytes (NHEKs) treated with SM (200 μM) showed their protective effects on the cytotoxicity induced by SM. Also, CA improved cell viability at concentrations > 250 μM dose-dependently. In addition, the treatment with CA and quercetin decreased the phosphorylation of p38 and p53 but increased the phosphorylation of JNK 1/2 induced by SM. Furthermore, CA and quercetin reduced the expression levels of cyclooxygenase 2 (COX-2), inducible NO synthase (iNOS), and the induction of oxidative stress irrespective of the p38 and lipoxygenase pathway (88).
In female mice exposed to SM, ethanolic extract of Hippophae rhamnoides L. leaf (HL-EOH), water and ethanolic extract of Hippophae rhamnoides fruit (HF-W and HF-EOH), and H. rhamnoides flavone from fruit (HR-flavone) significantly protected the lethal effect of SM. Treatment with HL-EOH and HR-flavone markedly protected the bodyweight loss, levels of GSH, oxidized glutathione (GSSG), and MDA. The LD50 of all extracts was more than 5 g/kg indicating their non-toxic property (89).
The Nigella sativa extract (0.08 g/day) in drinking water markedly decreased airway responsiveness to methacholine and WBC count in the guinea pigs exposed to SM (90). Similarly, the Nigella sativa extract at the above dose for 2 weeks also remarkably reduced airway responsiveness, neutrophil, eosinophil, lymphocyte, and monocyte percentage in the SM-exposed guinea pigs and the effects of Nigella sativa were similar to the onset of dexamethasone (5 mg/kg, i.p.) (91). The therapeutic effects of Salvia miltiorrhiza and Anemarrhena asphodeloides mixture (MSTF) (30, 60, 120 mg/kg) after exposure of the rats to SM (3.5 mg/kg, s.c.) significantly enhanced the survival and diminished the SM-induced morphological changes in the liver, small intestine, and testis tissues. The administration of MSTF (60 and 120 mg/kg) markedly increased the GSH level and prevented the differential expression of genes in the SM-exposed rats (92).
Clinical Studies
In a case-control study, the treatment of patients with lung disorder due to SM exposure (n=20) with boiled extract of Nigella sativa (0.375 ml/kg) significantly improved the PFT values, and chest wheeze 30 and 60 days after treatment compared to the placebo-treated group (n=20) and also compared to the beginning of the study (93). The treatment effects of the Avena sativa plant (0.1% cream twice a day for 4 weeks) on chronic pruritus in the SM-exposed patients in a double-blind clinical trial were studied. A total of seventy-five patients were divided into 3 groups including Avena sativa ointment, placebo, and betamethasone groups. At the end of the study period, the pruritus severity was significantly reduced in groups A and B compared to group C. The Avena sativa ointment treatment also improved the quality of life and quality of sleep in the patients (94).
In a randomized clinical study, the treatment of patients with lung disorder due to SM exposure with a syrup made from Zataria multiflora (5 and 10 mg/kg) for 2 months) reduced the WBCs (total and different) and oxidant biomarker but increased thiol, SOD, and CAT activities, and increased the PFT values (20). In a similar study, the serum levels of inflammatory mediators were reduced but the PFT values were increased due to a 2-month treatment with Zataria multiflora in the patients with lung disorders due to SM exposure (95). The treatment with Zataria multiflora extract in these patients also diminished cytokines, and respiratory symptoms, but increased some PFT values (96).
The 2-month treatment of the patients with lung disorder for a long time (27–30 years) exposing to SM with carvacrol (1.2 mg/kg) significantly enhanced the CAT and SOD activities, thiol level, and PEF values, but, declined the MDA level, total WBC and neutrophil count (21). The 2-month treatment with carvacrol in similar patients also remarkably reduced the respiratory symptoms and serum levels of IL-2, IL-4, IL-6, IL-8, EGF, and VEGF, but incremented the IFN-γ and IL-10 levels in the serum. In addition, the carvacrol treatment increased MEF25, 50, and 75 (maximum expiratory flow at 25, 50, and 75% of vital capacity) and MMEF (maximum mid-expiratory flow) values after 2 months of treatment (97, 98).
In the patients exposed to SM with chronic pruritic skin lesions (n = 96) treated by curcumin (1 g/d) or placebo for 4 weeks, the serum levels of high-sensitivity C-reactive protein (hs-CRP) and IL-8 were reduced in both groups. However, a higher effect of curcumin was observed compared to the placebo group and only treatment with curcumin reduced the calcitonin gene-related peptide (CGRP) level. In addition, in the curcumin group, IL-8 was correlated with the dermatology life quality index (DLQI) change (99). The treatment with curcumin in SM-induced chronic pruritus also improved the antioxidant status, quality of life (QoL), and pruritus (100).
The treatment of the patients with pulmonary complications induced by SM, with curcuminoids (500 mg) for 4 weeks, increased FEV1/FVC compared to placebo-treated patients. The inflammatory mediators (IL-6, IL-8, TGFβ, TNFα, hs-CRP, substance P, CGRP, and MCP-1 also improved remarkably greater than the placebo group. Therefore, in patients with SM-induced chronic pulmonary disorders, short-term curcuminoids treatment reduced lung and systemic inflammation (101).
In the other study, the SM-exposed patients were treated with standard drugs plus curcuminoids and piperine (1,500 and 15 mg/day, respectively) or placebo for 4 weeks. The serum level of GSH was increased but that of MDA decreased and the health-related quality of life (HRQoL) was significantly improved at the end of the study in both groups. However, GSH, MDA, and the HRQoL changes in the curcuminoids-piperine-treated group were markedly greater than the placebo group (102).
The above studies showed the protective effect of NP on the cell viability, inflammation, and pathological changes in the experimental studies that were exposed to SM. The clinical studies also indicated that NP improve the quality of life, PFT values, respiratory symptoms inflammatory mediators, and oxidative stress markers of SM-induced lung disorder. The therapeutic effects of NP in SM-induced lung injury are summarized in Table 6 and Figure 5. The molecular mechanism of SM-induced toxicity is shown in Figure 5.
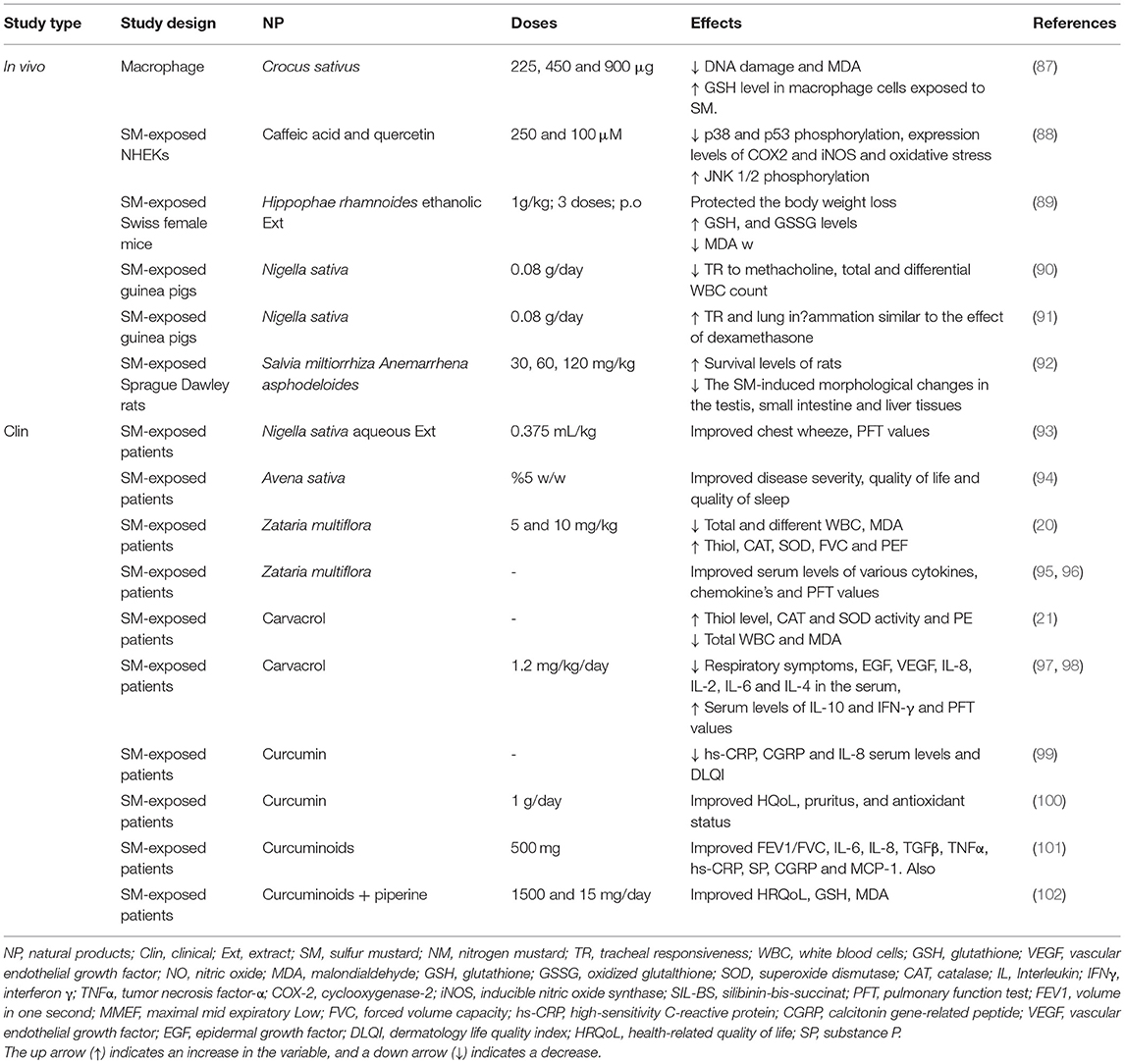
Table 6. The possible therapeutic effects of medicinal plants and their derivatives in the SM-induced lung injuries.
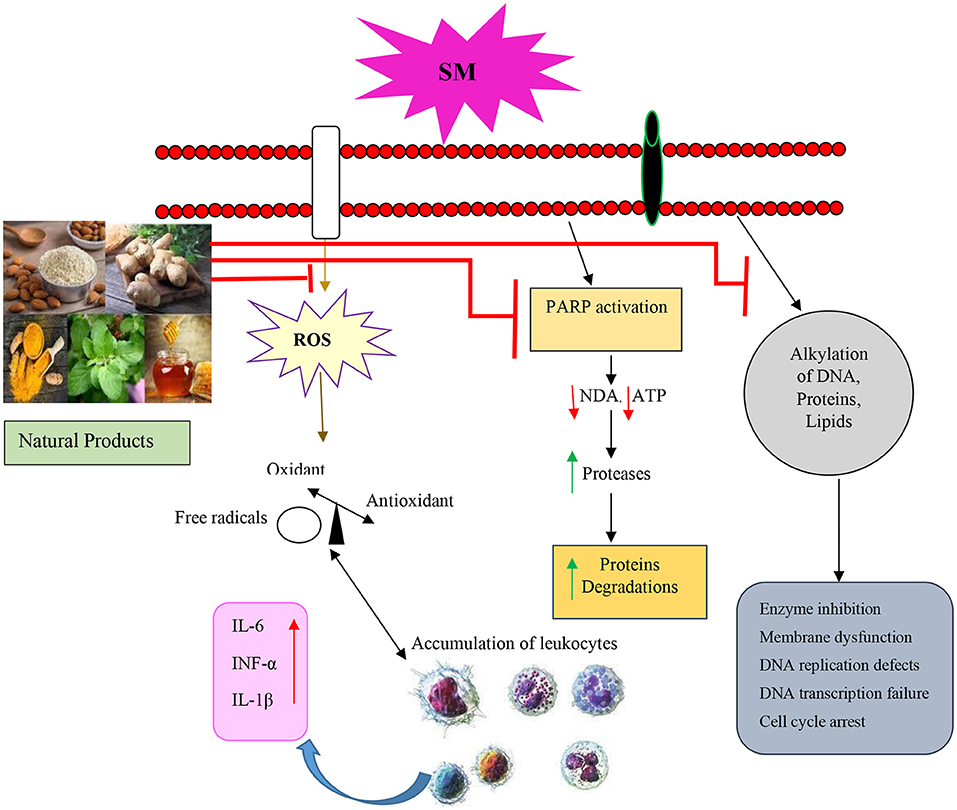
Figure 5. Molecular mechanism of SM-induced toxicity of the relevant studies. SM, Sulfur mustard; ROS, Reactive oxygen species; PARP, Poly (ADP-ribose) polymerase; IL, Interleukin; TNFα, Tumor necrosis factor-α.
Other Noxious Agents-Induced Lung Disorders
Experimental Studies
The pre-treatment with Origanum vulgare extract (50, 100, 200, and 400 mg/kg) protected the lung tissues from cyclophosphamide (CP)-induced pulmonary damage and suggested a role for oxidative stress in the pathogenesis of the lung disease induced by CP (103). In the rats challenged with methotrexate (MTX) (20 mg/kg) and treated with alpha-lipoic acid (ALA) after MTX administration, the levels of IL-1β, MDA, GSH, TNF-α, MPO, and sodium potassium-adenosine triphosphatase (Na+/K+ATPase) were improved due to the ALA treatment (104).
In a rat model of amiodarone-induced lung insult, the serum levels of TGF-β1 and TNF-α markedly increased. The treatment with grape seed extract (150 mg/kg) ameliorated oxidative and fibrotic damages in the lung of the amiodarone-treated rats (105). The aqueous extract of caffeic acid phenethyl ester (5 and 10 μmol /day) significantly attenuated the acute lung injury induced by amiodarone (7.5 UI/kg). The activities of myeloperoxidase and SOD enzymes were significantly decreased in the group which was treated with caffeic acid phenethyl ester (106). In the amiodarone-induced lung toxicity, two phenolic acids, ferulic acid and gallic acid, showed a protective effect on the inflammatory biomarkers and oxidative stress (107).
A protective effect of A. melanocarpa fruit juice against amiodarone-induced pulmonary toxicity was shown by the reduction of amiodarone-induced direct toxic damage signs, oxidative stress, and fibrosis (108). Treatment with grape seed and Ginkgo biloba (100 mg/kg) ameliorated the histopathological structure, increased the contents of glycogen, and improved the ultrastructure alternations of the lung tissue in the rats exposed to a single dose of amiodarone (40 mg/kg). Grape seed was markedly more effective than Ginkgo biloba in protecting the rats against amiodarone (109). The therapeutic effects of NP in the other noxious agents-induced lung injury are summarized in Table 7 and Figure 3.
Discussion
The induction of various lung disorders due to exposure to NA of the general environment or in the workplace was shown, both in animal and human studies. Among the most important NA, exposure to BLM, Cd, environmental dust, LPS, PQ, SM, and amiodarone can cause lung diseases. Exposure to these NA usually leads to PF and COPD but it also can induce various other lung disorders such as emphysema. The induction of lung disorders due to exposure to NA is accomplished with lung pathological changes, wet/dry lung weight disturbance, oxidative stress in the lung, lung inflammation indicated by increased inflammatory mediators, and immune dysregulation indicated by the changes in the cytokine levels and other immune markers in the BALF or lung tissues.
The results of this review study showed the pharmacological and therapeutic effects of different NP including medicinal plants and their derivatives on lung disorders both in the experimental and clinical studies. The experimental studies indicated the effects of different medicinal plants including Aloe vera, Anemarrhena asphodeloides, Avena sativa, Crocus sativus, Curcuma longa, Dioscorea batatas, Glycyrrhiza glabra, Gentiana veitchiorum, Gentiopicroside, Houttuynia cordata, Hibiscus sabdariffa, Hochu-ekki-to, Hippophae rhamnoides, Juglans regia, Melanocarpa fruit juice, Mikania glomerata, Mikania laevigata, Moringa oleifera, Myrtus communis L., Lamiaceae, Myrtle, Mosla scabra leaves, Nectandra leucantha, Nigella sativa, Origanum vulgare L, Pulicaria petiolaris, Paulownia tomentosa, Pomegranate seed oil, Raphanus sativus L. var niger, Rosa canina, Schizonepeta tenuifolia,Thymus vulgaris, Taraxacum mongolicum, Tribulus Terrestris, Telfairia occidentalis, Taraxacum officinale, TADIOS, Xuebijing, Viola yedoensis, Zataria multiflora, Zingiber officinale, Yin-Chiao-San, and their derivatives on the lung injury induced by NA. The treatment with NP in NA-induced lung disorders ameliorated all lung changes induced by NA such as lung pathological changes, lung oxidative stress, lung inflammation, and immune dysregulation. In clinical studies, the effects of medicinal plants and their derivatives such as Avena sativa, Curcuma longa, Nigella sativa, and Zataria multiflora on SM-induced lung disorders were shown by reducing respiratory symptoms, oxidative stress markers, inflammatory mediators, and cytokine levels as well as increasing PFT.
The results of this review study showed the possible therapeutic effects of various NP on NA-induced lung disorders by amelioration of various features of lung injury. However, further clinical studies, especially on the effect of NP on lung diseases induced by BLM, Cd, environmental dust, LPS, PQ, and other noxious agents are needed to support the therapeutic effect on NP on NA-induced lung disorders for clinical practice purposes.
Author Contributions
SS, SB, and MK prepared the first draft of the manuscript and helped in the revision of the final version of the manuscript. MB designed the study, critically edited, and revised the manuscript. All authors contributed to the article and approved the submitted version.
Conflict of Interest
The authors declare that the research was conducted in the absence of any commercial or financial relationships that could be construed as a potential conflict of interest.
Publisher's Note
All claims expressed in this article are solely those of the authors and do not necessarily represent those of their affiliated organizations, or those of the publisher, the editors and the reviewers. Any product that may be evaluated in this article, or claim that may be made by its manufacturer, is not guaranteed or endorsed by the publisher.
References
1. Semenza GL. Oxygen sensing, homeostasis, and disease. N Engl J Med. (2011) 365:537–47. doi: 10.1056/NEJMra1011165
2. Kim D, Chen Z, Zhou LF, Huang SX. Air pollutants and early origins of respiratory diseases. Chronic Dis Transl Med. (2018) 4:75–94. doi: 10.1016/j.cdtm.2018.03.003
3. Alvarado A, Arce I. Metabolic functions of the lung, disorders and associated pathologies. J Clin Med Res. (2016) 8:689. doi: 10.14740/jocmr2668w
4. Sethi GS, Dharwal V, Naura AS. Immunological Basis of Oxidative Stress-Induced Lung Inflammation in Asthma and COPD. Oxidative Stress in Lung Diseases. Springer: Springer (2019). p. 195–223.
5. Kawai M, Hirano T, Higa S, Arimitsu J, Maruta M, Kuwahara Y, et al. Flavonoids and related compounds as anti-allergic substances. Allergol Int. (2007) 56:113–23. doi: 10.2332/allergolint.R-06-135
6. Singh A, Holvoet S, Mercenier A. Dietary polyphenols in the prevention and treatment of allergic diseases. Clin Exp Allergy. (2011) 41:1346–59. doi: 10.1111/j.1365-2222.2011.03773.x
7. Phan SH, Thrall RS, Williams C. Bleomycin-induced pulmonary fibrosis: effects of steroid on lung collagen metabolism. Am Rev Respir Dis. (1981) 124:428–34. doi: 10.1164/arrd.1981.124.4.428
8. Wormser U, Nir I. Effect of age on cadmium-induced metallothionein synthesis in the rat. Arch Toxicol. (1988) 62:392–4. doi: 10.1007/BF00293629
9. Go Y-M, Orr M, Jones DP. Actin cytoskeleton redox proteome oxidation by cadmium. Am J Physiol Lung Cell Mol Physiol. (2013) 305:L831–L43. doi: 10.1152/ajplung.00203.2013
10. Jindal SK, Aggarwal AN, Gupta D. Dust-induced interstitial lung disease in the tropics. Curr Opin Pulm Med. (2001) 7:272–7. doi: 10.1097/00063198-200109000-00004
11. Ingenito EP, Mora R, Cullivan M, Marzan Y, Haley K, Mark L, et al. Decreased surfactant protein-B expression and surfactant dysfunction in a murine model of acute lung injury. Am J Respir Cell Mol Biol. (2001) 25:35–44. doi: 10.1165/ajrcmb.25.1.4021
12. Orfanos S, Mavrommati I, Korovesi I, Roussos C. Pulmonary endothelium in acute lung injury: from basic science to the critically ill. Appl Physiol Intens Care Med. (2006) 30:171–83. doi: 10.1007/3-540-37363-2_30
13. Bismuth C, Hall A, Wong A. Paraquat Poisoning: Mechanisms-Prevention-Treatment. New York, NY: Marcel Dekker (1995). 10:195–210.
14. Sato S. Human Paraquat Toxicology: Prognostic Factors. Paraquat Poisoning: Mechanisms-Prevention-Treatment. New York, NY: Marcel Dekker (1995). p. 267–74.
15. Suzuki K, Takasu N, Arita S, Maenosono A, Ishimatsu S, Nishina M, et al. A new method for predicting the outcome and survival period in paraquat poisoning. Hum Toxicol. (1989) 8:33–8. doi: 10.1177/096032718900800106
16. Bianchi M, Fantuzzi G, Bertini R, Perin L, Salmona M, Ghezzi P. The pneumotoxicant paraquat induces IL-8 mRNA in human mononuclear cells and pulmonary epithelial cells. Cytokine. (1993) 5:525–30. doi: 10.1016/1043-4666(93)90045-7
17. Liu MW, Su MX, Zhang W, Wang YQ, Chen M, Wang L, et al. Protective effect of Xuebijing injection on paraquat-induced pulmonary injury viadown-regulating the expression of p38 MAPK in rats. BMC Complement Altern Med. (2014) 14:1–14. doi: 10.1186/1472-6882-14-498
18. Reshetnikov V, Hahn J, Maueröder C, Czegley C, Munoz LE, Herrmann M, et al. Chemical tools for targeted amplification of reactive oxygen species in neutrophils. Front Immunol. (2018) 9:1827. doi: 10.3389/fimmu.2018.01827
19. Emad A, Rezaian G, Hosseini K, Ghayyoomi S. Chronic pulmonary sequelae of sulfur mustard gas exposure in man: a report of 36 Cases. Irn J Med Sci. (1995) 20:1–4.
20. Khazdair MR, Rajabi O, Balali-Mood M, Beheshti F, Boskabady MH. The effect of Zataria multiflora on pulmonary function tests, hematological and oxidant/antioxidant parameters in sulfur mustard exposed veterans, a randomized doubled-blind clinical trial. Environ Toxicol Pharmacol. (2018) 58:180–8. doi: 10.1016/j.etap.2018.01.006
21. Khazdair M, Alavinezhad A, Boskabady M. Carvacrol ameliorates haematological parameters, oxidant/antioxidant biomarkers and pulmonary function tests in patients with sulphur mustard-induced lung disorders: a randomized double-blind clinical trial. J Clin Pharm Ther. (2018) 43:664–74. doi: 10.1111/jcpt.12684
22. Kayani S, Ahmad M, Zafar M, Sultana S, Khan MPZ, Ashraf MA, et al. Ethnobotanical uses of medicinal plants for respiratory disorders among the inhabitants of Gallies–Abbottabad, Northern Pakistan. J Ethnopharmacol. (2014) 156:47–60. doi: 10.1016/j.jep.2014.08.005
23. Singh V, Kumar K, Purohit D, Verma R, Pandey P, Bhatia S, et al. Exploration of therapeutic applicability and different signaling mechanism of various phytopharmacological agents for treatment of breast cancer. Biomed Pharmacother. (2021) 139:111584. doi: 10.1016/j.biopha.2021.111584
24. Beigh S, Rashid H, Sharma S, Parvez S, Raisuddin S. Bleomycin-induced pulmonary toxicopathological changes in rats and its prevention by walnut extract. Biomed Pharmacother. (2017) 94:418–29. doi: 10.1016/j.biopha.2017.07.124
25. Liang X., Tian Q, Wei Z, Liu Fe, Chen J, Zhao Y, et al. Effect of Feining on bleomycin-induced pulmonary injuries in rats. J Ethnopharmacol. (2011) 134:971–6. doi: 10.1016/j.jep.2011.02.008
26. Sun SC, Han R, Hou SS, Yi HQ, Chi SJ, Zhang AH. Juglanin alleviates bleomycin-induced lung injury by suppressing inflammation and fibrosis viatargeting sting signaling. Biomed Pharmacother. (2020) 127:110119. doi: 10.1016/j.biopha.2020.110119
27. Tajima S, Bando M, Yamasawa H, Ohno S, Moriyama H, Terada M, et al. Preventive effect of hochu-ekki-to, a Japanese herbal medicine, on bleomycin-induced lung injury in mice. Respirology. (2007) 12:814–22. doi: 10.1111/j.1440-1843.2007.01146.x
28. Gong LK, Li XH, Wang H, Zhang L, Cai Y, Qi XM, et al. Feitai attenuates bleomycin-induced pulmonary fibrosis in rats. Biol Pharmac Bull. (2004) 27:634–40. doi: 10.1248/bpb.27.634
29. Bahri S, Ben Ali R, Gasmi K, Mlika M, Fazaa S, Ksouri R, et al. Prophylactic and curative effect of rosemary leaves extract in a bleomycin model of pulmonary fibrosis. Pharm Biol. (2017) 55:462–71. doi: 10.1080/13880209.2016.1247881
30. Chen L, Zhao W. Apigenin protects against bleomycin-induced lung fibrosis in rats. Exp Ther Med. (2016) 11:230–4. doi: 10.3892/etm.2015.2885
31. Wang Q, Yu J, Hu Y, Chen X, Zhang L, Pan T, et al. Indirubin alleviates bleomycin-induced pulmonary fibrosis in mice by suppressing fibroblast to myofibroblast differentiation. Biomed Pharmacother. (2020) 131:110715. doi: 10.1016/j.biopha.2020.110715
32. Poursalehi HR, Fekri MS, Far FS, Mandegari A, Izadi A, Mahmoodi R, et al. Early and late preventive effect of Nigella sativa on the bleomycin-induced pulmonary fibrosis in rats: an experimental study. Avicenna J Phytomed. (2018) 8:263.
33. Asghari MH, Hobbenaghi R, Nazarizadeh A, Mikaili P. Hydro-alcoholic extract of Raphanus sativus L. var niger attenuates bleomycin-induced pulmonary fibrosis via decreasing transforming growth factor β1 level. Res Pharm Sci. (2015) 10:429.
34. Ng LT, Yen FL, Liao CW, Lin CC. Protective effect of Houttuynia cordata extract on bleomycin-induced pulmonary fibrosis in rats. Am J Chin Med. (2007) 35:465–75. doi: 10.1142/S0192415X07004989
35. Samareh Fekri M, Mandegary A, Sharififar F, Poursalehi HR, Nematollahi MH, Izadi A, et al. Protective effect of standardized extract of Myrtus communis L. (myrtle) on experimentally bleomycin-induced pulmonary fibrosis: biochemical and histopathological study. Drug Chem Toxicol. (2018) 41:408–14. doi: 10.1080/01480545.2018.1459670
36. Sener G, Topaloglu N, Sehirli AÖ, Ercan F, Gedik N. Resveratrol alleviates bleomycin-induced lung injury in rats. Pulm Pharmacol Ther. (2007) 20:642–9. doi: 10.1016/j.pupt.2006.07.003
37. Wen G, Li T, He H, Zhou X, Zhu J. Ganoderic acid A inhibits bleomycin-induced lung fibrosis in mice. Pharmacology. (2020) 105:566–73. doi: 10.1159/000505297
38. Rong Y, Cao B, Liu B, Li W, Chen Y, Chen H, et al. A novel Gallic acid derivative attenuates BLM-induced pulmonary fibrosis in mice. Int Immunopharmacol. (2018) 64:183–91. doi: 10.1016/j.intimp.2018.08.024
39. Yen FL, Wu TH, Liao CW, Lin CC. A kampo medicine, Yin-Chiao-san, Prevents bleomycin-induced pulmonary injury in rats. Phytother Res. (2007) 21:251–8. doi: 10.1002/ptr.2056
40. Nikbakht J, Hemmati AA, Arzi A, Mansouri MT, Rezaie A, Ghafourian M. Protective effect of gallic acid against bleomycin-induced pulmonary fibrosis in rats. Pharmacol Rep. (2015) 67:1061–7. doi: 10.1016/j.pharep.2015.03.012
41. El-Ebiary AA, El-Ghaiesh S, Hantash E, Alomar S. Mitigation of cadmium-induced lung injury by Nigella sativa oil. Environ Sci Pollut Res. (2016) 23:25356–63. doi: 10.1007/s11356-016-7603-3
42. Alsammak M. Effect of Cadmium Chloride on the Histological Structure of Lung in Adult Male Mice with and without Parsley Oil. Open Access Macedonian J Med Sci. (2021) 9:676–9. doi: 10.3889/oamjms.2021.6207
43. Baiomy A. Protective role of grape seeds extract against cadmium toxicity in the lung of male wistar rats. J Cytol Histol S. (2016) S5:004. doi: 10.4172/2157-7099.S5-004
44. AL-Kraie NIH, Dalas IS, Razooqi QA. The Toxic Effect of Cadmium Chloride on Lung Function and Tissue and the Protective Role of Pomegranate Seed Oil in Female Rabbits. Indian J Forensic Med Toxicol. (2020) 14:275.
45. Freitas TP, Silveira PC, Rocha LG, Rezin GT, Rocha J, Citadini-Zanette V, et al. Effects of Mikania glomerata Spreng. and Mikania laevigata Schultz Bip ex Baker (Asteraceae) extracts on pulmonary inflammation and oxidative stress caused by acute coal dust exposure. J Med Food. (2008) 11:761–6. doi: 10.1089/jmf.2008.0051
46. Kalibekova A, Mustafina RK, Kazymbet P editors. The impact of prolonged uranium dust inhalation in small doses to the ratio of the end product of glycolysis in the lung tissues of rats, corrective effects of licorice roots extracts. In: Materials of 4 International Theoretical and Practical Conference 'Medical-biological and Radio-Ecological Problems on Uranium-and Oil-producing Regions' (2010).
47. Yahaya T, Okpuzor J, Ajayi T. Antioxidant activity of Roselle (Hibiscus sabdariffa), Moringa (Moringa oleifera), Ginger (Zingiber officinale) and ‘Ugwu'(Telfairia occidentalis) in the lungs of Albino rats (Rattus norvegicus) exposed to cement dust. Ann Res Rev Biol. (2014) 4:736–46. doi: 10.9734/ARRB/2014/5440
49. Lee JW, Seo KH, Ryu HW, Yuk HJ, Park HA, Lim Y, et al. Anti-inflammatory effect of stem bark of Paulownia tomentosa Steud. in lipopolysaccharide (LPS)-stimulated RAW264 7 macrophages and LPS-induced murine model of acute lung injury. J Ethnopharmacol. (2018) 210:23–30. doi: 10.1016/j.jep.2017.08.028
50. Bittencourt-Mernak MI, Pinheiro NM, da Silva RC, Ponci V, Banzato R, Pinheiro AJ, et al. Effects of Eugenol and Dehydrodieugenol B from Nectandra leucantha against Lipopolysaccharide (LPS)-Induced Experimental Acute Lung Inflammation. J Nat Prod. (2021) 84:2282–94. doi: 10.1021/acs.jnatprod.1c00386
51. Jiang K, Guo S, Yang C, Yang J, Chen Y, Shaukat A, et al. Barbaloin protects against lipopolysaccharide (LPS)-induced acute lung injury by inhibiting the ROS-mediated PI3K/AKT/NF-κB pathway. Int Immunopharmacol. (2018) 64:140–50. doi: 10.1016/j.intimp.2018.08.023
52. Lee JH, Ahn J, Kim JW, Lee SG, Kim HP. Flavonoids from the aerial parts of Houttuynia cordata attenuate lung inflammation in mice. Arch Pharm Res. (2015) 38:1304–11. doi: 10.1007/s12272-015-0585-8
53. Li PY, Liang YC, Sheu MJ, Huang SS, Chao CY, Kuo YH, et al. Alpinumisoflavone attenuates lipopolysaccharide-induced acute lung injury by regulating the effects of anti-oxidation and anti-inflammation both in vitro and in vivo. RSC Adv. (2018) 8:31515–28. doi: 10.1039/C8RA04098B
54. Chandrasekaran CV, Kannan Sundarajan JRE, Gururaja GM, Mundkinajeddu D, Agarwal A. Immune-stimulatory and anti-inflammatory activities of Curcuma longa extract and its polysaccharide fraction. Pharmacognosy Res. (2013) 5:71. doi: 10.4103/0974-8490.110527
55. Oliviero M, Romilde I, Beatrice MM, Matteo V, Giovanna N, Consuelo A, et al. Evaluations of thyme extract effects in human normal bronchial and tracheal epithelial cell lines and in human lung cancer cell line. Chem Biol Interact. (2016) 256:125–33. doi: 10.1016/j.cbi.2016.06.024
56. Feng G, Sun B. Li TZ. Daidzein attenuates lipopolysaccharide-induced acute lung injury via toll-like receptor 4/NF-kappaB pathway. Int Immunopharmacol. (2015) 26:392–400. doi: 10.1016/j.intimp.2015.04.002
57. Lee W, Lee CH, Lee J, Jeong Y, Park JH, Nam IJ, et al. Botanical formulation, TADIOS, alleviates lipopolysaccharide (LPS)-Induced acute lung injury in mice via modulation of the Nrf2-HO-1 signaling pathway. J Ethnopharmacol. (2021) 270:113795. doi: 10.1016/j.jep.2021.113795
58. Wan L, Meng D, Wang H, Wan S, Jiang S, Huang S, et al. Preventive and therapeutic effects of thymol in a lipopolysaccharide-induced acute lung injury mice model. Inflammation. (2018) 41:183–92. doi: 10.1007/s10753-017-0676-4
59. Ahmed N, Aljuhani N, Salamah S, Surrati H, El-Agamy DS, Elkablawy MA, et al. Pulicaria petiolaris effectively attenuates lipopolysaccharide (LPS)-induced acute lung injury in mice. Arch Biol Sci. (2018) 70:699–706. doi: 10.2298/ABS180510033A
60. Ribeiro A., Ferraz-de-Paula V, Pinheiro ML, Vitoretti LB, Mariano-Souza DP, Quinteiro-Filho WM, et al. Cannabidiol, a non-psychotropic plant-derived cannabinoid, decreases inflammation in a murine model of acute lung injury: role for the adenosine A2A receptor. Eur J Pharmacol. (2012) 678:78–85. doi: 10.1016/j.ejphar.2011.12.043
61. Huo M, Cui X, Xue J, Chi G, Gao R, Deng X, et al. Anti-inflammatory effects of linalool in RAW 264. 7 macrophages and lipopolysaccharide-induced lung injury model. J Surg Res. (2013) 180:e47–54. doi: 10.1016/j.jss.2012.10.050
62. Ma C, Zhu L, Wang J, He H, Chang X, Gao J, et al. Anti-inflammatory effects of water extract of Taraxacum mongolicum hand. -Mazz on lipopolysaccharide-induced inflammation in acute lung injury by suppressing PI3K/Akt/mTOR signaling pathway. J Ethnopharmacol. (2015) 168:349–55. doi: 10.1016/j.jep.2015.03.068
63. Baradaran Rahimi V, Rakhshandeh H, Raucci F, Buono B, Shirazinia R, Samzadeh Kermani A, et al. Anti-inflammatory and anti-oxidant activity of Portulaca oleracea extract on LPS-induced rat lung injury. Molecules. (2019) 24:139. doi: 10.3390/molecules24010139
64. Lv H, Liu Q, Wen Z, Feng H, Deng X, Ci X. Xanthohumol ameliorates lipopolysaccharide (LPS)-induced acute lung injury via induction of AMPK/GSK3β-Nrf2 signal axis. Redox Biol. (2017) 12:311–24. doi: 10.1016/j.redox.2017.03.001
65. Li YC, Yeh CH, Yang ML, Kuan YH. Luteolin suppresses inflammatory mediator expression by blocking the Akt/NFκB pathway in acute lung injury induced by lipopolysaccharide in mice. Evid Based Complement Altern Med. (2012) 2012:383608. doi: 10.1155/2012/383608
66. Shen Y, Han N, Chen H, Zhang M, Cai W. Evaluation of lipopolysaccharide-induced acute lung injury attenuation in mice by Glycyrrhiza glabra. Pharmacogn Mag. (2020) 16:92. doi: 10.4103/pm.pm_189_19
67. Park BK, So KS, Ko HJ, Kim HJ, Kwon KS, Kwon YS, et al. Therapeutic potential of the rhizomes of Anemarrhena asphodeloides and timosaponin A-III in an animal model of lipopolysaccharide-induced lung inflammation. Biomol Ther. (2018) 26:553. doi: 10.4062/biomolther.2017.249
68. De Melo GO, Muzitano MF, Legora-Machado A, Almeida TA, De Oliveira DB, Kaiser CR, et al. C-glycosylflavones from the aerial parts of Eleusine indica inhibit LPS-induced mouse lung inflammation. Planta Med. (2005) 71:362–3. doi: 10.1055/s-2005-864104
69. Soromou LW, Chen N, Jiang L, Huo M, Wei M, Chu X, et al. Astragalin attenuates lipopolysaccharide-induced inflammatory responses by down-regulating NF-κB signaling pathway. Biochem Biophys Res Commun. (2012) 419:256–61. doi: 10.1016/j.bbrc.2012.02.005
70. Zhao M, Du J. Anti-inflammatory and protective effects of D-carvone on lipopolysaccharide (LPS)-induced acute lung injury in mice. J King Saud Univ Sci. (2020) 32:1592–6. doi: 10.1016/j.jksus.2019.12.016
71. Mao M, Huang M. Myricetin attenuates lung inflammation and provides protection against lipopolysaccharide-induced acute lung injury by inhibition of NF-κB pathway in rats. Trop J Pharm Res. (2017) 16:2585–93. doi: 10.4314/tjpr.v16i11.3
72. Li W, Xie JY, Li H, Zhang YY, Cao J, Cheng ZH, et al. Viola yedoensis liposoluble fraction ameliorates lipopolysaccharide-induced acute lung injury in mice. Am J Chin Med. (2012) 40:1007–18. doi: 10.1142/S0192415X12500747
73. Wu G, Dai X, Li X, Jiang H. Antioxidant and anti-inflammatory effects of Rhamnazin on lipopolysaccharide-induced acute lung injury and inflammation in rats. Afr J Trad Complement Altern Med. (2017) 14:201–12. doi: 10.21010/ajtcam.v14i4.23
74. Mokhtari-Zaer A, Norouzi F, Askari VR, Khazdair MR, Roshan NM, Boskabady M, et al. The protective effect of Nigella sativa extract on lung inflammation and oxidative stress induced by lipopolysaccharide in rats. J Ethnopharmacol. (2020) 253:112653. doi: 10.1016/j.jep.2020.112653
75. Amin F, Roohbakhsh A, Memarzia A, Kazerani HR, Boskabady MH. Paraquat-induced systemic inflammation and increased oxidative markers in rats improved by Zataria multiflora extract and carvacrol. Avicenna J Phytomed. (2020) 10:513.
76. Amin F, Memarzia A, Kazemi Rad H, Shakeri F, Boskabady MH. Systemic inflammation and oxidative stress induced by inhaled paraquat in rat improved by carvacrol, possible role of PPARγ receptors. BioFactors. (2021) 2021. doi: 10.1002/biof.1761
77. Novaes RD, Gonçalves RV, Cupertino MC, Marques DC, Rosa DD, Peluzio MdCG, et al. Bark extract of Bathysa cuspidata attenuates extra-pulmonary acute lung injury induced by paraquat and reduces mortality in rats. Int J Exp Pathol. (2012) 93:225–33. doi: 10.1111/j.1365-2613.2012.00808.x
78. Zhang Z, Ding L, Wu L, Xu L, Zheng L, Huang X. Salidroside alleviates paraquat-induced rat acute lung injury by repressing TGF-β1 expression. Int J Clin Exp Pathol. (2014) 7:8841.
79. Amin F, Memarzia A, Kazerani HR, Boskabady MH. Carvacrol and Zataria multiflora influenced the PPARγ agonist effects on systemic inflammation and oxidative stress induced by inhaled paraquat in rat. Iran J Basic Med Sci. (2020) 23:930. doi: 10.22038/ijbms.2020.45962.10648
80. Heydari M, Mokhtari-Zaer A, Amin F, Memarzia A, Saadat S, Hosseini M, et al. The effect of Zataria multiflora hydroalcoholic extract on memory and lung changes induced by rats that inhaled paraquat. Nutr Neurosci. (2021) 24:674–87. doi: 10.1080/1028415X.2019.1668173
81. Amin F, Memarzia A, Roohbakhsh A, Shakeri F, Boskabady MH. Zataria multiflora and Pioglitazone Affect Systemic Inflammation and Oxidative Stress Induced by Inhaled Paraquat in Rats. Mediators Inflamm. (2021) 2021:5575059. doi: 10.1155/2021/5575059
82. Liu H, Zhao Y, Cui Y, Chen C. Therapeutical effect of ligustrazine to acute lung injury induced by paraquat in rats. Shandong Med J. (2009) 49:32–4.
83. Hosseini A, Rasaie D, Soleymani Asl S, Nili Ahmadabadi A, Ranjbar A. Evaluation of the protective effects of curcumin and nanocurcumin against lung injury induced by sub-acute exposure to paraquat in rats. Toxin Rev. (2021). 40:1233–41. doi: 10.1080/15569543.2019.1675707
84. Mirzaee S, Mansouri E, Shirani M, Zeinvand-Lorestani M, Khodayar MJ. Diosmin ameliorative effects on oxidative stress and fibrosis in paraquat-induced lung injury in mice. Environ Sci Pollut Res. (2019) 26:36468–77. doi: 10.1007/s11356-019-06572-2
85. Amirshahrokhi K. The Effect of Rosa canina Extract Against Paraquat-induced Lung Injury. J Ardabil Univ Med Sci. (2020) 19:400–9. doi: 10.29252/jarums.19.4.400
86. Luan RL, Meng XX, Jiang W. Protective effects of apigenin against paraquat-induced acute lung injury in mice. Inflammation. (2016) 39:752–8. doi: 10.1007/s10753-015-0302-2
87. Zarei Mahmoudabadi A, Sharif F, Jafari M. Effect of Crocus sativus L. marine extract on genotoxicity of Syrian mouse macrophages. Kowsar Med J. (2011) 16:79–86.
88. Kim S, Jeong KJ, Cho SK, Park JW, Park WJ. Caffeic acid, morin hydrate and quercetin partially attenuate sulfur mustard-induced cell death by inhibiting the lipoxygenase pathway. Mol Med Rep. (2016) 14:4454–60. doi: 10.3892/mmr.2016.5766
89. Vijayaraghavan R, Gautam A, Kumar O, Pant S, Sharma M, Singh S, et al. Protective Effect of Ethanolic and Water Extracts of Sea Buckthorn (Hippophae rhamnoides L.) Against the Toxic Effects of Mustard Gas. Indian J Exp Biol. (2006) 44:821–31.
90. Hossein BM, Nasim V, Sediqa A. The protective effect of Nigella sativa on lung injury of sulfur mustard-exposed Guinea pigs. Exp Lung Res. (2008) 34:183–94. doi: 10.1080/01902140801935082
91. Boskabady MH, Vahedi N, Amery S, Khakzad MR. The effect of Nigella sativa alone, and in combination with dexamethasone, on tracheal muscle responsiveness and lung inflammation in sulfur mustard exposed guinea pigs. J Ethnopharmacol. (2011) 137:1028–34. doi: 10.1016/j.jep.2011.07.030
92. Li J, Chen L, Wu H, Lu Y, Hu Z, Lu B, et al. The mixture of salvianolic Acids from Salvia miltiorrhiza and total flavonoids from Anemarrhena asphodeloides attenuate sulfur mustard-induced injury. Int J Mol Sci. (2015) 16:24555–73. doi: 10.3390/ijms161024555
93. Boskabady MH, Farhadi J. The possible prophylactic effect of Nigella sativa seed aqueous extract on respiratory symptoms and pulmonary function tests on chemical war victims: a randomized, double-blind, placebo-controlled trial. J Altern Complement Med. (2008) 14:1137–44. doi: 10.1089/acm.2008.0049
94. Shohrati M, Davoud M, Rezazadeh S, Najafian B. Clinical efficacy of topical Avena sativa versus Betamethasone in chronic pruritus due to sulfur mustard exposure. J Med Plants. (2017) 16:68–77.
95. Khazdair MR, Ghorani V, Alavinezhad A, Boskabady MH. The effect of Zataria multiflora on the levels of serum cytokine and pulmonary function tests in sulfur mustard induced lung disorders, a randomized doubled-blind clinical trial. J Ethnopharmacol. (2020) 248:112325. doi: 10.1016/j.jep.2019.112325
96. Khazdair MR, Rezaeetalab F, Rafatpanah H, Boskabady MH. The effect of Zataria multiflora on inflammatory cytokine and respiratory symptoms in veterans exposed to sulfur mustard. Environ Sci Pollut Res. (2020) 27:22451–60. doi: 10.1007/s11356-020-08855-5
97. Khazdair MR, Boskabady MH. A double-blind, randomized, placebo-controlled clinical trial on the effect of carvacrol on serum cytokine levels and pulmonary function tests in sulfur mustard induced lung injury. Cytokine. (2019) 113:311–8. doi: 10.1016/j.cyto.2018.07.031
98. Khazdair MR, Boskabady MH. The effect of carvacrol on inflammatory mediators and respiratory symptoms in veterans exposed to sulfur mustard, a randomized, placebo-controlled trial. Respir Med. (2019) 150:21–9. doi: 10.1016/j.rmed.2019.01.020
99. Panahi Y, Sahebkar A, Parvin S, Saadat A. A randomized controlled trial on the anti-inflammatory effects of curcumin in patients with chronic sulphur mustard-induced cutaneous complications. Ann Clin Biochem. (2012) 49:580–8. doi: 10.1258/acb.2012.012040
100. Panahi Y, Sahebkar A, Amiri M, Davoudi SM, Beiraghdar F, Hoseininejad SL, et al. Improvement of sulphur mustard-induced chronic pruritus, quality of life and antioxidant status by curcumin: results of a randomised, double-blind, placebo-controlled trial. Br J Nutr. (2012) 108:1272–9. doi: 10.1017/S0007114511006544
101. Panahi Y, Ghanei M, Bashiri S, Hajihashemi A, Sahebkar A. Short-term Curcuminoid Supplementation for Chronic Pulmonary Complications due to Sulfur Mustard Intoxication: Positive Results of a Randomized Double-blind Placebo-controlled Trial. Drug Res. (2014) 65:567–73. doi: 10.1055/s-0034-1389986
102. Panahi Y, Ghanei M, Hajhashemi A, Sahebkar A. Effects of curcuminoids-piperine combination on systemic oxidative stress, clinical symptoms and quality of life in subjects with chronic pulmonary complications due to sulfur mustard: a randomized controlled trial. J Diet Suppl. (2014) 13:93–105 doi: 10.3109/19390211.2014.952865
103. Shokrzadeh M, Ahmadi A, Chabra A, Naghshvar F, Salehi F, Habibi E, et al. An ethanol extract of Origanum vulgare attenuates cyclophosphamide-induced pulmonary injury and oxidative lung damage in mice. Pharm Biol. (2014) 52:1229–36. doi: 10.3109/13880209.2013.879908
104. Arpag H, Gül M, Aydemir Y, Atilla N, Yigitcan B, Cakir T, et al. Protective effects of alpha-lipoic acid on methotrexate-induced oxidative lung injury in rats. J Investig Surg. (2018) 31:107–13. doi: 10.1080/08941939.2017.1296513
105. Madkour NK, Ahmed M. Amelioration of amiodarone-induced lung fibrosis in rats by grape seed extract. J Appl Sci Res. (2013) 9:3698–707.
106. Zaeemzadeh N, Hemmati A, Arzi A, Jalali M, Rashidi I. Protective effect of caffeic acid phenethyl ester (CAPE) on amiodarone-induced pulmonary fibrosisin rat. Iran J Pharm Res. (2011) 10:321.
107. Alazragi R. Protective Role of Ferulic acid and/or Gallic acid Against Pulmonary Toxicity Induced by Amiodarone in Rats. Arch Pharm Pract. (2020) 11:83–91.
108. Valcheva-Kuzmanova S, Stavreva G, Dancheva V, Terziev L, Atanasova M, Stoyanova A, et al. Effect of Aronia melanocarpa fruit juice on amiodarone-induced pneumotoxicity in rats. Pharmacogn Mag. (2014) 10:132. doi: 10.4103/0973-1296.131024
109. Galaly SR, Abdul-Hamid M, Mahmoud H, Mostafa F. Ameliorative effect of grape seed and ginkgo biloba against pulmonary damage induced by amiodarone in male albino rats. J Adv Pharm Edu Res. (2018) 8:33–42.
Glossary
Ach: acetylcholineAHR: hyperresponsivenessBALF: Broncho-alveolar lavage fluidCAT: catalaseCGRP: calcitonin gene-related peptideCOPD: Chronic obstructive pulmonary diseaseCW: Chemical warfareDLQI: Dermatology Life Quality IndexDOX: DoxycyclineEPO: peroxidaseFDA: Food and Drug AdministrationFEV1: Forced expiratory volume in the first secondFVC: Forced vital capacityGSH: glutathioneHDAC: Histone deacetylase complexhPBMCs: human peripheral blood mononuclear cellsHRQoL: health-related quality of lifehs-CRP: high-sensitivity C-reactive proteinIFNγ: Interferon-gammaIg: immunoglobulinIL: InterleukiniNOS: induced nitric oxide synthaseIPF: idiopathic pulmonary fibrosisLPS: LipopolysaccharideLTC4: leukotriene C4MCHC: mean corpuscular hemoglobin concentrationMCP-1: monocyte chemotactic protein-1MCV: mean corpuscular volume
MDA: malondialdehydemMCP-1: mouse mast cell protease-1MMEF: maximum mid-expiratory flowMMPs: matrix metalloproteinaseNAC: N-AcetylcysteineNFkB: nuclear transcription factorNK cells: natural killer cellsNO: oxide productionOVA: OvalbuminPaCO2: arterial blood carbon dioxidePE: pulmonary embolismPEF: peak expiratory flowPF: pulmonary fibrosisPFT: Pulmonary function testsPQ: paraquatQoL: quality of lifeROS: Reactive oxygen speciesSaO2: arterial blood oxygenation saturationSIL-BS: silibinin-bis-succinatSM: Sulfur mustardSOD: superoxide dismutaseTAT: Thrombin anti-thrombinTFPI: Tissue factor pathway inhibitorTGF-β1: transforming growth factor beta1TNFα: Tumor necrosis factor-αtPA: Tissue plasminogen activatorTR: tracheal responsivenessTSM: tracheal smooth muscleUV: ultravioletWBC: White blood cells.
Keywords: natural product, medicinal plants, bleomycin, cadmium, dust, lipopolysaccharide, sulfur mustard, lung injury
Citation: Saadat S, Beigoli S, Khazdair MR, Amin F and Boskabady MH (2022) Experimental and Clinical Studies on the Effects of Natural Products on Noxious Agents-Induced Lung Disorders, a Review. Front. Nutr. 9:867914. doi: 10.3389/fnut.2022.867914
Received: 01 February 2022; Accepted: 16 March 2022;
Published: 18 May 2022.
Edited by:
Surasak Saokaew, University of Phayao, ThailandReviewed by:
Qiyun WU, Hong Kong University of Science and Technology, Hong Kong SAR, ChinaSontaya Sookying, University of Phayao, Thailand
Copyright © 2022 Saadat, Beigoli, Khazdair, Amin and Boskabady. This is an open-access article distributed under the terms of the Creative Commons Attribution License (CC BY). The use, distribution or reproduction in other forums is permitted, provided the original author(s) and the copyright owner(s) are credited and that the original publication in this journal is cited, in accordance with accepted academic practice. No use, distribution or reproduction is permitted which does not comply with these terms.
*Correspondence: Mohammad Hossein Boskabady, Ym9za2FiYWR5bWgmI3gwMDA0MDttdW1zLmFjLmly; Ym9za2FiYWR5MiYjeDAwMDQwO2dtYWlsLmNvbQ==
†These authors share first authorship