- 1Centro de Investigaciones Biomédicas, Universidad Veracruzana, Xalapa, Mexico
- 2LADISER Inmunología y Biología Molecular, Facultad de Ciencias Químicas, Universidad Veracruzana, Orizaba, Mexico
- 3Unidad de Neurometabolismo, Centro de Investigación y Desarrollo en Ciencias de la Salud, Universidad Autónoma de Nuevo León, San Nicolás de los Garza, Mexico
- 4Unidad de Investigación en Ambiente y Salud, Universidad Autónoma de Occidente, Los Mochis, Mexico
During pregnancy the human fetus receives timed cues from the circadian rhythms of temperature, metabolites, and hormones from the mother. This influence is interrupted after parturition, the infant does not secrete melatonin and their circadian rhythms are still immature. However, evolution provided the solution to this problem. The newborn can continue receiving the mother's timed cues through breastmilk. Colostrum, transitional, and mature human milk are extraordinary complex biofluids that besides nutrients, contain an array of other non-nutritive components. Upon birth the first milk, colostrum, is rich in bioactive, immunological factors, and in complex oligosaccharides which help the proper establishment of the microbiome in the gut, which is crucial for the infants' health. Hormones, such as glucocorticoids and melatonin, transfer from the mother's plasma to milk, and then the infant is exposed to circadian cues from their mother. Also, milk components of fat, proteins, amino acids, and endogenous cannabinoids, among others, have a markedly different concentration between day and night. In the present review, we give an overview of nutritive and non-nutritive components and their daily rhythms in human milk and explore their physiological importance for the infant. Finally, we highlight some interventions with a circadian approach that emphasize the importance of circadian rhythms in the newborn for their survival, proper growth, and development. It is estimated that ~600,000 deaths/year are due to suboptimal breastfeeding. It is advisable to increase the rate of exclusive breastfeeding, during the day and night, as was established by the evolution of our species.
Introduction
Being active and eating during typical times of rest, as with night-shift work, disrupts circadian clocks and is related to a higher risk of several metabolic disorders (1). Recent studies have also found that restricting eating to certain times of day can be beneficial to health (2), and from these observations the field of chrononutrition has developed (3). The goal of chrononutrition is to adjust nutrition quality and intake to coordinate with an individual's biological clock, so that one consumes the optimal type and quantity of food at the correspondingly optimal time of day (3). Most of the current understanding of chrononutrition arose from work in adults and thus does not encompass the lifespan of developing humans. Rest and activity patterns and nutritional needs change as humans develop. Unlike adults, newborns ingest milk during the day and night, and this has an important biological significance: the concentration of milk components changes according to the circadian rhythms of the mother. Moreover, the infant is sensitive to milk and environmental circadian cues. In the present contribution, we review the current evidence about these two topics related to rhythms in breastmilk and environmental conditions and discuss their relevance for the proper establishment of the infant's circadian rhythms. Some studies label the temporal changes of milk components as circadian but, in most cases, they describe only different concentrations between specific hours of the day and night; in the present review, we will refer to them as daily rhythms. Our main emphasis will be on human studies.
In the womb, the fetus is exposed for approximately 9 months to the circadian, physiological, metabolic, and behavioral rhythms of the mother. This circadian milieu is abruptly interrupted upon birth, but nature developed the perfect substitution: maternal milk whose composition changes according to the circadian rhythms of the mother (4–7). Not surprisingly, in humans the newborn ingests milk during the day and the night, and the nutritive and non-nutritive components change accordingly. The world health organization recommends exclusive breastfeeding for at least the first 6 months of age to improve child survival, healthy growth, and development but unfortunately, breastfeeding rates in the world are low (8).
Nutrients in Human Milk Through Lactation
Human milk is an extremely complex biofluid with dynamic composition, beginning with the first milk, colostrum, through transitional and mature milk; it changes significantly depending on the maternal diet, environmental factors, and whether milk is produced for preterm or term infants (9–11). Mature human milk composition contains 88% water, 7% carbohydrates, 4% fat, and 1% protein (9). Protein and total amino acid concentration is highest in colostrum 14–16 g/L, then steadily decreases to 7–8 g/L in mature milk (12). Caseins and whey proteins are the main proteins in milk, but proteomic analysis has identified ~1,700 different proteins (13). Fat concentration has an increasing trend from 26 g/L in colostrum to 37 g/L in mature milk (11). Carbohydrates are the most abundant macronutrient, in which lactose concentration is very stable during lactation with a mean concentration of 61.4 g/L (4), with a lower concentration in colostrum (56 g/L) than in mature milk in which it reaches its highest concentration (68 g/L)to support the increasing growth of the infant [Figure 1; (16)]. Moreover, milk also has vitamins, enzymes and coenzymatic factors, hormones, and immunological factors [(14); Figure 1].
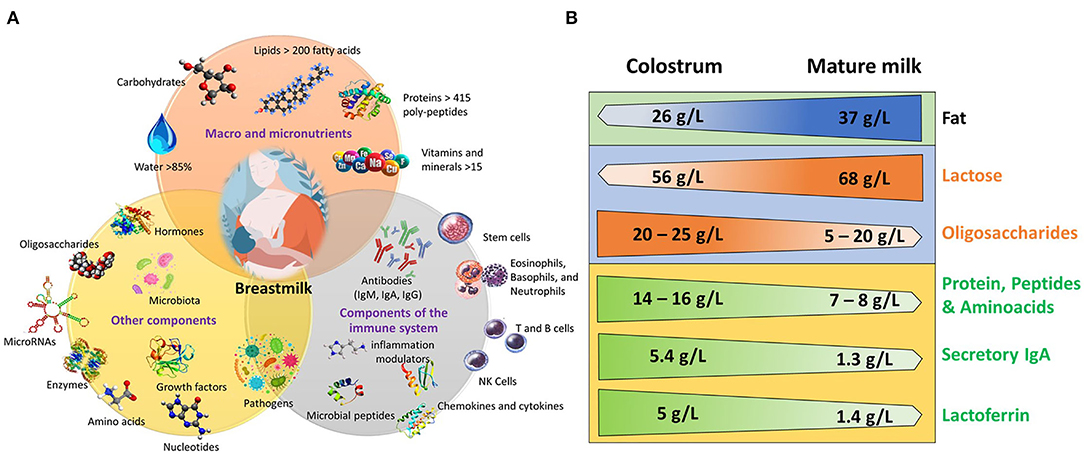
Figure 1. Components of human milk and differences between colostrum and mature milk. (A) Macro and micronutrients, components of the immune system, and other components. Modified from (7, 9, 10, 14, 15). (B) Concentration of some components in colostrum and in mature milk. Modified from (4, 11–14, 16).
Non-Nutritive Components and Immunological Factors
The immune system of the newborn is immature and human milk is rich in immunological factors. It contains immunoglobulins, immune cells, complement proteins, and immunomodulatory and antimicrobial factors (17–19). Immunoglobulin A (IgA), Immunoglobulin G (IgG) and Immunoglobulin M (IgM) are present in colostrum, but IgA is different from that found in plasma. It is a dimer with a joining J chain protein and a secretory component named secretory IgA (SIgA), which increases its resistance to proteolytic degradation in the intestine (20). Consistent with this finding, significant quantities of SigA are found in the infant stool, which decreases in parallel with that in milk (21). Levels of sIgA are much larger in colostrum, in comparison with IgG and IgM. Their levels decline from 5.4 g/L in colostrum to 1.3 g/L in mature milk [(17); Figure 1]. Lactoferrin, an iron-binding glycoprotein with bacteriostatic and bactericidal activity against Escherichia coli, Candida albicans, and other pathogens, such as viruses (22), is higher in colostrum (5 g/L) than in mature milk [1.4g/L; (17); Figure 1]. In addition, human breast milk contains pluripotent stem cells and leucocytes (23–26), plus inflammatory and anti-inflammatory cytokines (27). Polyunsaturated fatty acids, such as docosahexaenoic (DHA) and arachidonic (ARA) acids, have immunomodulatory properties and participate in several developmental and cognitive processes (28). Contrary to the common belief that human milk is sterile, it has been established that in mature milk, the infant consumes ~8 X 104-106 commensal bacteria, fungi, and viruses that colonize the infant gut to form a microbiome that allows proper development of the immune system (9, 29). Also, breastmilk contains ~1,400 species of mature microRNAs that are absorbed by the intestinal epithelial cells and are involved in immunomodulatory and epigenetic regulation (30, 31). Milk also contains a complex array of approximately 200 oligosaccharides, which have important immunological properties and are essential to the development of a “healthy” microbiome. Their concentration is highest in colostrum (20–25 g/L) and decrease through lactation to 5–20 g/L (14, 32). Cannabinoids are among the several neurochemicals found in milk; these include anandamide (AEA) and 2-arachidonoyl glycerol (2-AG), the latter in much larger concentration [(33); Figure 1]. Thus, milk is a complex biofluid that contains myriad nutritive and non-nutritive components. Human milk has an addition allevel of complexity: it reflects circadian rhythms of the mother.
Diurnal Changes in Milk Components
In human milk, there are circadian hormonal variations, including glucocorticoids (GLUC), melatonin, (MEL), Leptin, Ghrelin, and others. Plasma GLUC increases during the last phase of the night, reaches a peak during the morning, and then decreases (34). In contrast, MEL increases during the night and it is almost negligible in plasma during the day (35). GLUC and MEL may communicate “time of day and night” to the body; they are associated with alertness and sleep phases. GLUC and MEL are transferred to breast milk, and their concentration mirrors that in plasma. GLUC concentration in human milk is higher in the morning. MEL levels are low in the evening and the first part of the night, then increase again (5, 36). At 3–4 days after parturition MEL is below the limit of detection between 1,400 and 1,700 h, but during the night between 0200 and 0400 it reaches a concentration of 99 ± 26 pmol/L (6, 35). Concentration in human milk of another hormone, Leptin, is significantly higher during 10 pm and 4 am compared to 10 am−10 pm (37). Lipids increase in the morning, reach a peak from midday to evening, and reach lower values at night (38–40). Ghrelinisalso present in human milk as well as insulin, adiponectin, obestatin, and other metabolic hormones (15, 41). No diurnal differences have been found among these latter hormones or in total proteins, carbohydrates, and lactose, or there are controversies about their diurnal differences (42, 43). However, of 17 amino acids explored, only a clear rhythm of tryptophan was observed in colostrum, transitional, and mature human milk with an acrophase at approximately 0400 h, and then its levels decrease and reach a nadir during the afternoon (7). In mature milk, clear rhythms were detected also in methionine, aspartic acid, histidine, phenylalanine, and tyrosine, with an acrophase at different times of the day (7). In mature milk, there are also rhythms of nucleotides, adenosine 5'monophosphate (5'AMP), guanosine 5'monophosphate (5'GMP), uridine 5'monophosphate (5'UMP),cytidine5'monophosphate (5'CMP) and inosine 5'mono‘hosphate (5'IMP), the first three with higher levels during the night and the latter two during the day (44). In mature milk, 2-AG showed significantly higher concentration during the day than during the night, which mirrored plasma levels in the mother [(33); Figure 2A].
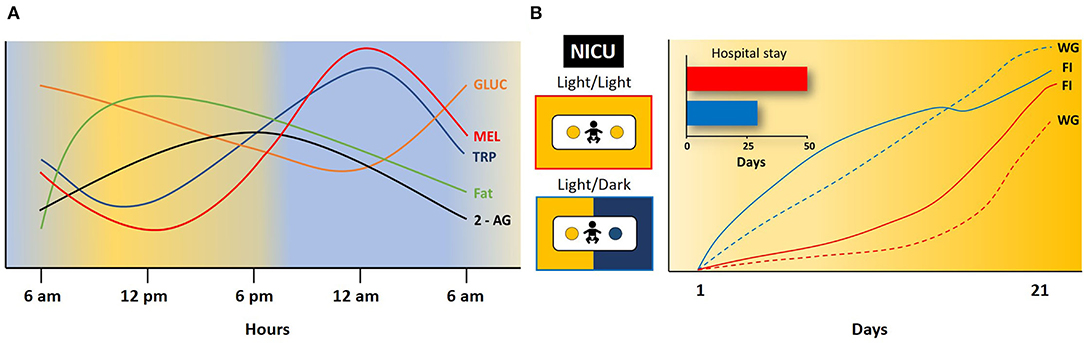
Figure 2. Daily rhythm in some milk components and effect of light/dark conditions on infant development. (A) Changes in concentration of glucocorticoids (GLUC), melatonin (MEL), tryptophan (TRP), fat and 2-arachidonoyl glycerol (2-AG) in 24 h. Modified from (5, 6, 33, 35, 36). (B) Effect of constant light (Light/Light) or 12 h light and 12 h dark (Light/Dark) on food intake (FI), weight gain (WG), and length of hospital stay in infants at the neonatal intensive care unit (NICU). Blue line and dashed lines infants in Light/Dark; Red line and dashed lines infants in Light/Dark. Note the shorter hospital stay and the increase in food intake and weight gain in infants in Light/Dark cycle in comparison to infants in Light/Light conditions. Modified from (45).
Diurnal Changes in Immunological Factors
Earlier studies reported no differences in concentration of IgA, IgM, and IgG between day and night (46, 47). Further, in a study of 36 mothers, colostrum, transitional and mature human milk, sIgA was higher during the day at 12:00 h than during the night at 24:00 h. IgG and IgM were also higher during the day than during the night in transitional and mature milk [(48); but see (19)]. C3 and C4 complement proteins, which opsonize pathogens and participate in the innate and adaptive response, increased significantly in colostrum during the diurnal period in humans (48). There are also reports about significant differences between day and night concentrations of cytokines IFNγ, IL4, IL5, and IL10 (49, 50). No diurnal differences have been found in Lactoferrin concentrations (46).
Physiological Importance of Diurnal Changes in Milk Components
During late pregnancy the fetus expresses rhythms of total activity, heart rate, and general and breathing movements (51–53). This is not only a response to maternal rhythms, as the fetal brain is necessary for the integration of the mother's cues (54). Disruption of circadian rhythms during pregnancy leads to an array of negative pregnancy outcomes, such as increased frequency of miscarriage, preterm infant delivery, and low birth weight (55). Upon birth the newborn is exposed to a variety of manipulations and environmental changes and time of the establishment of circadian rhythms of CORT, MEL and day/night rest and sleep rhythm vary widely at 3–6 months of age (56). In a study in which the infant was breastfed on demand during the day and night and exposed to light only during day time, the circadian rhythms of temperature, sleep/wake, and MEL were detected during the first week and at 30 and 45 days of life, respectively (57), significantly earlier than most reports. Accumulating evidence reveals the benefits of changes in milk components and environmental conditions for the infant.
The higher concentration of tryptophan and nucleotides 5'AMP, 5'GMP, and 5'UMP may play an important role as sleep promoters. It has been established that adenosine decreases cellular activity and is a somnogen (58). In agreement, there is an increase of 5'GMP in adult human plasma during sleep in the night in comparison to the period of wakefulness (59). In human milk, the concentration of 5'AMP, 5'GMP, and 5'UMP is higher during the night than during the day (44). The effect of the differential concentration of tryptophan and the nucleotides was tested in children. Sleep-wake cycle in newborns is not established and they nurse during the day and the night (60). They ingest tryptophan and nucleotides during the night, when the concentrations are highest. Tryptophan is a precursor of the hormone melatonin, and its ingestion corresponds with the variable levels of 6-sulfatoxymelatonin in urine, a metabolite of melatonin (61). To test the effect of this amino acid on sleep, tryptophan was added to formula milk, along with 5'AMP and 5'UMP to children 4–20 weeks of age. Children that received formula with this amino acid and nucleotides from 18:00 to 06:00 h showed a reduction in their latency to sleep as well as an increase in their hours of sleep (62). In another study of the same group, 8–16 month old children were fed a cereal enriched with tryptophan, 5'AMP, and 5'UMP during the night. Actigraphic recording revealed an improvement in sleep parameters (63). These experiments support the assumption that tryptophan, 5'AMP, 5'UMP, and MEL in human milk during the night improve infant sleep and help to consolidate their sleep/wake cycle. Vitamin B12 also may improve sleep in children. In humans, it has been associated with the modulation of sleep and circadian rhythms (64–66), and its deficiency during pregnancy has been associated with a subsequent increase in the infants' crying (67). Together the evidence indicates that there are chronobiotic substances in milk that contribute to the establishment of the sleep-wake cycle of the infant. Indeed, a recent study demonstrates that breastfed infants achieved a circadian rest-activity rhythm at 6th week age in contrast to 12 weeks in mixed, formula and breastmilk-fed babies (68). Exclusively breastfed infants had better sleep parameters in comparison to formula-fed infants (69). Infants at 2 months of age who were breastfed, in contrast to formula-fed infants, had a significantly lower frequency of colic attacks and severity of irritability attacks, which was associated with the night-time consumption of MEL through milk (70).
MEL has a strong interaction with the immune system. Human lymphocytes contain membrane MEL receptors which suggest that they can detect physiological changes in this hormone (71). In vitro studies have demonstrated an effect of MEL on the phagocytic activity of mononuclear and polymorphonuclear lymphocytes from colostrum. When exposed to Escherichia coli, in the presence of MEL, these cells increase their phagocytic, and bactericidal activity by stimulating cellular oxidative metabolism (6). In another example, TNF-α, a regulator of inflammatory processes, which is present in human milk, inhibits the in vitro synthesis of MEL in the ratpineal gland (72). This information is relevant, as cesarean section delivery in humans increased the production of TNF-α in colostrum, in parallel with a suppression of the nocturnal MEL increase (50). This can lead to an inflammatory process and to a disruption of the beneficial actions of MEL in the newborn. There are reports that the addition of nucleotides to formula milk significantly increased weight gain (73) and the rate of the occipitofrontal head circumference gain in infants 2 months of age (74). Infants fed with milk supplements enriched with DHA had a lower incidence of bronchiolitis and bronchitis during the first year of age (75).
The high level of 2-AG cannabinoids in human milk may modulate the infant's food intake, and this also can be influenced by the weight of the mother. Milk of overweight and obese mothers has a larger concentration of 2-AG and this may have an impact on the body mass index of the infant because of the effect of endocannabinoids on food intake and the hedonic properties of food (33, 76, 77). Overweight mothers give birth to overweight babies (78). Cannabinoids are involved in development, as the administration of an antagonist of the CB1 receptor within the first day after birth in mice; inhibited milk ingestion due to an impairment of the pup's suckling response (79). This evidence and that cannabinoids are one of the few compounds in human milk with diurnal variation; suggest that they play an important role for the infant. It is noteworthy that breastfeeding also has important benefits for the mother, as it reduces the risk of ovarian cancer, mammary cancer, and postpartum depression (80).
Effects of Environmental Light/Dark Cycle on Neonates
The importance of circadian rhythms for the wellbeing of infants has been reported in preterm infants in a neonatal intensive care unit. After parturition, premature infants were separated from their mothers and usually did not ingest their mother's milk. The infant's pineal in humans does not secrete MEL, and those infants were maintained in constant light conditions (81). This strategy severely affected their survival. Infants in this facility maintained in constant light had a lower weight gain, spent more days on the ventilator and on phototherapy, displayed lower motor coordination, and showed a delayed response to be fed orally, in contrast to babies maintained in a cycled light-dark condition (81). In another study, a personalized helmet over the head of the infant that covered their eyes but permitted airflow was used to maintain 12:12 h, light/dark condition, and infants were fed with mother's milk. Infants under this procedure showed faster weight gain, improved oxygen saturation, more rapidly developed a melatonin rhythm, and attained a shorter discharge stay in the hospital [(45); Figure 2B]. These studies are examples of the importance of circadian rhythms after birth in infant. Fortunately, the compelling evidence of the benefits of exposing premature infants to a light/dark cycle is leading neonatal care societies to recommend this practice for clinical applications (82).
Conclusion
The complexity of milk stands in sharp contrast to the concept of simple food for the infant. The analysis of milk components and their diurnal changes has led to the enrichment of milk substitutes. Unfortunately, there is only limited research on the role of breastfeeding and control of light-dark conditions in the establishment of infant circadian rhythms and wellbeing. Based on the evidence reviewed herein, we consider this issue to deserve attention, as regarding infant nutrition, there is increasing use of milk supplements, which are devoid of myriad nutritive and non-nutritive components, and lack the diurnal rhythms inherent in breastmilk. Long-term studies demonstrate that breastfeeding, in contrast to infant formula, has multiple benefits: decreased risk of developing obesity and type 2 diabetes, gastrointestinal, ear, and respiratory tract infections, and improved cognitive neurodevelopment and mental and behavioral health (83–85). Many of these benefits are epigenetic, via the components of breast milk, including the infant's microbiome (86). In a recent survey of 130 countries, it was concluded that suboptimal breastfeeding causes ~600,000 child deaths/year primarily from pneumonia and diarrhea (87). Moreover, breastfeeding since birth is effective in preventing death in premature babies, in contrast to conventional care practices (88). A milk substitute cannot replace the complex composition and diurnal dynamic changes of breastmilk, so major approaches must be developed to promote the benefits of breastfeeding over commercial formula to optimize infant nutrition and subsequent health. Thus, the control of the light/dark conditions (89) in addition to the circadian variations in milk components through exclusive breastfeeding every 2–5 h (57), is strongly recommended as an important strategy to improve health and proper infant development.
Author Contributions
MC-F, AR-L, AC-M, CM-V, RV-C, and MC contributed to the conception, writing, and figures design. All authors approved the submitted version of this article.
Funding
This study was funded by the COVEICYDET (151746) to MC.
Conflict of Interest
The authors declare that the research was conducted in the absence of any commercial or financial relationships that could be construed as a potential conflict of interest.
Publisher's Note
All claims expressed in this article are solely those of the authors and do not necessarily represent those of their affiliated organizations, or those of the publisher, the editors and the reviewers. Any product that may be evaluated in this article, or claim that may be made by its manufacturer, is not guaranteed or endorsed by the publisher.
Acknowledgments
We thank Dr. Colin Saldanha, Dr. Antonio Nunez, and Dr. Barry R. Komisaruk for corrections and helpful comments.
References
1. Guerrero-Vargas NN, Espitia-Bautista E, Buijs RM, Escobar C. Shift-work: is time of eating determining metabolic health? evidence from animal models. Proc Nutr Soc. (2018) 77:199–215. doi: 10.1017/S0029665117004128
2. Flanagan A, Bechtold DA, Pot GK, Johnston JD. Chrono-nutrition: from molecular and neuronal mechanisms to human epidemiology and timed feeding patterns. J Neurochem. (2021) 157:53–72. doi: 10.1111/jnc.15246
3. Tahara Y, Shibata S. Chrono-biology, chrono-pharmacology, and chrono-nutrition. J Pharmacol Sci. (2014) 124:320–35. doi: 10.1254/jphs.13R06CR
4. Mitoulas LR, Kent JC, Cox DB, Owens RA, Sherriff JL, Hartmann PE. Variation in fat, lactose and protein in human milk over 24 h and throughout the first year of lactation. Br J Nutr. (2002) 88:29–37. doi: 10.1079/BJN2002579
5. van der Voorn B, de Waard M, van Goudoever JB, Rotteveel J, Heijboer AC, Finken MJ. Breast-milk cortisol and cortisone concentrations follow the diurnal rhythm of maternal hypothalamus-pituitary-adrenal axis activity. J Nutr. (2016) 146:2174–9. doi: 10.3945/jn.116.236349
6. Honorio-França AC, Hara CCP, Ormonde JVS, Nunes GT, França EL. Human colostrum melatonin exhibits a day-night variation and modulates the activity of colostral phagocytes. J Appl Biomed. (2013) 11:153–62. doi: 10.2478/v10136-012-0039-2
7. Sánchez CL, Cubero J, Sánchez J, Franco L, Rodríguez AB, Rivero M, et al. Evolution of the circadian profile of human milk amino acids during breastfeeding. J Appl Biomed. (2013) 11:59–70. doi: 10.2478/v10136-012-0020-0
8. Infant Young Child Feeding. (2021). Available online at: https://www.who.int/news-room/fact-sheets/detail/infant-and-young-child-feeding (accessed March 26, 2022).
9. Kim SY, Yi DY. Components of human breast milk: from macronutrient to microbiome and microRNA. Clin Exp Pediatr. (2020) 63:301–9. doi: 10.3345/cep.2020.00059
10. Perrella S, Gridneva Z, Lai CT, Stinson L, George A, Bilston-John S, et al. Human milk composition promotes optimal infant growth, development and health. Semin Perinatol. (2021) 45:151380. doi: 10.1016/j.semperi.2020.151380
11. Gidrewicz DA, Fenton TR. A systematic review and meta-analysis of the nutrient content of preterm and term breast milk. BMC Pediatr. (2014) 14:216. doi: 10.1186/1471-2431-14-216
12. Lönnerdal B. Nutritional and physiologic significance of human milk proteins. Am J Clin Nutr. (2003) 77:1537S−3S. doi: 10.1093/ajcn/77.6.1537S
13. Zhu J, Dingess KA. The functional power of the human milk proteome. Nutrients. (2019) 11:E1834. doi: 10.3390/nu11081834
14. Dror DK, Allen LH. Overview of nutrients in human milk. Adv Nutr. (2018) 9:278S−94. doi: 10.1093/advances/nmy022
15. Badillo-Suárez PA, Rodríguez-Cruz M, Nieves-Morales X. Impact of metabolic hormones secreted in human breast milk on nutritional programming in childhood obesity. J Mammary Gland Biol Neoplasia. (2017) 22:171–91. doi: 10.1007/s10911-017-9382-y
16. Coppa GV, Gabrielli O, Pierani P, Catassi C, Carlucci A, Giorgi PL. Changes in carbohydrate composition in human milk over 4 months of lactation. Pediatrics. (1993) 91:637–41. doi: 10.1542/peds.91.3.637
17. Lönnerdal B, Erdmann P, Thakkar SK, Sauser J, Destaillats F. Longitudinal evolution of true protein, amino acids and bioactive proteins in breast milk: a developmental perspective. J NutrBiochem. (2017) 41:1–11. doi: 10.1016/j.jnutbio.2016.06.001
18. Camacho-Morales A, Caba M, García-Juárez M, Caba-Flores MD, Viveros-Contreras R, Martínez-Valenzuela C. Breastfeeding contributes to physiological immune programming in the newborn. Front Pediatr. (2021) 9:1120. doi: 10.3389/fped.2021.744104
19. Massmann PF, França EL, Souza EG de, Souza MS, Brune MFSS, Honorio-França AC. Maternal hypertension induces alterations in immunological factors of colostrum and human milk. Front Life Sci. (2013) 7:155–63. doi: 10.1080/21553769.2013.876451
20. Lindh E. Increased resistance of immunoglobulin A dimers to proteolytic degradation after binding of secretory component. J Immunol. (1975) 114:284–6.
21. Davidson LA, Lönnerdal B. Persistence of human milk proteins in the breast-fed infant. Acta PaediatrScand. (1987) 76:733–40. doi: 10.1111/j.1651-2227.1987.tb10557.x
22. Wheeler TT, Hodgkinson AJ, Prosser CG, Davis SR. Immune components of colostrum and milk–a historical perspective. J Mammary Gland Biol Neoplasia. (2007) 12:237–47. doi: 10.1007/s10911-007-9051-7
23. Briere CE, McGrath JM, Jensen T, Matson A, Finck C. Breast milk stem cells: current science and implications for preterm infants. Adv Neonatal Care. (2016) 16:410–9. doi: 10.1097/ANC.0000000000000338
24. Li S, Zhang L, Zhou Q, Jiang S, Yang Y, Cao Y. Characterization of stem cells and immune cells in preterm and term mother's milk. J Hum Lact. (2019) 35:528–34. doi: 10.1177/0890334419838986
25. Witkowska-Zimny M, Kaminska-El-Hassan E. Cells of human breast milk. Cell Mol Biol Lett. (2017) 22:11. doi: 10.1186/s11658-017-0042-4
26. Trend S, de Jong E, Lloyd ML, Kok CH, Richmond P, Doherty DA, et al. Leukocyte populations in human preterm and term breast milk identified by multicolour flow cytometry. PLoS ONE. (2015) 10:e0135580. doi: 10.1371/journal.pone.0135580
27. Kiełbasa A, Gadzała-Kopciuch R, Buszewski B. Cytokines-biogenesis and their role in human breast milk and determination. Int J Mol Sci. (2021) 22:6238. doi: 10.3390/ijms22126238
28. Carlson SE, Colombo J. Docosahexaenoic acid and arachidonic acid nutrition in early development. Adv Pediatr. (2016) 63:453–71. doi: 10.1016/j.yapd.2016.04.011
29. Selma-Royo M, Calvo Lerma J, Cortés-Macías E, Collado MC. Human milk microbiome: from actual knowledge to future perspective. Semin Perinatol. (2021) 45:151450. doi: 10.1016/j.semperi.2021.151450
30. Melnik BC, Schmitz G. MicroRNAs: milk's epigenetic regulators. Best Pract Res Clin Endocrinol Metab. (2017) 31:427–42. doi: 10.1016/j.beem.2017.10.003
31. Benmoussa A, Provost P. Milk microRNAs in health and disease. Compr Rev Food Sci Food Saf. (2019) 18:703–22. doi: 10.1111/1541-4337.12424
32. Bode L. Human milk oligosaccharides: every baby needs a sugar mama. Glycobiology. (2012) 22:1147–62. doi: 10.1093/glycob/cws074
33. Datta P, Melkus MW, Rewers-Felkins K, Patel D, Bateman T, Baker T, et al. Human milk endocannabinoid levels as a function of obesity and diurnal rhythm. Nutrients. (2021) 13:2297. doi: 10.3390/nu13072297
34. Dickmeis T. Glucocorticoids and the circadian clock. J Endocrinol. (2009) 200:3–22. doi: 10.1677/JOE-08-0415
35. Illnerová H, Buresová M, Presl J. Melatonin rhythm in human milk. J Clin Endocrinol Metab. (1993) 77:838–41. doi: 10.1210/jcem.77.3.8370707
36. Pundir S, Wall CR, Mitchell CJ, Thorstensen EB, Lai CT, Geddes DT, et al. Variation of human milk glucocorticoids over 24 hour period. J Mammary Gland Biol Neoplasia. (2017) 22:85–92. doi: 10.1007/s10911-017-9375-x
37. Cannon AM, Kakulas F, Hepworth AR, Lai CT, Hartmann PE, Geddes DT. The effects of leptin on breastfeeding behaviour. Int J Environ Res Public Health. (2015) 12:12340–55. doi: 10.3390/ijerph121012340
39. Lubetzky R, Mimouni FB, Dollberg S, Salomon M, Mandel D. Consistent circadian variations in creamatocrit over the first 7 weeks of lactation: a longitudinal study. Breastfeed Med. (2007) 2:15–8. doi: 10.1089/bfm.2006.0013
40. Paulavičiene IJ. Changes in human milk macronutrient content of non-breastfeeding mothers after delivery and factors or procedures affecting its composition. [doctoral dissertation]. Vilnius University, Vilnius (2021).
41. Aydin S, Ozercan HI, Aydin S, Ozkan Y, Daglin F, Oguzoncul F, et al. Biological rhythm of saliva ghrelin in humans. Biol Rhythm Res. (2006) 37:169–77. doi: 10.1080/09291010600576860
42. Italianer MF, Naninck EFG, Roelants JA, van der Horst GTJ, Reiss IKM, Goudoever JB, van, et al. Circadian variation in human milk composition, a systematic review. Nutrients. (2020) 12:E2328. doi: 10.3390/nu12082328
43. Paulaviciene IJ, Liubsys A, Molyte A, Eidukaite A, Usonis V. Circadian changes in the composition of human milk macronutrients depending on pregnancy duration: a cross-sectional study. Int Breastfeed J. (2020) 15:49. doi: 10.1186/s13006-020-00291-y
44. Sánchez CL, Cubero J, Sánchez J, Chanclón B, Rivero M, Rodríguez AB, et al. The possible role of human milk nucleotides as sleep inducers. NutrNeurosci. (2009) 12:2–8. doi: 10.1179/147683009X388922
45. Vásquez-Ruiz S, Maya-Barrios JA, Torres-Narváez P, Vega-Martínez BR, Rojas-Granados A, Escobar C, et al. A light/dark cycle in the NICU accelerates body weight gain and shortens time to discharge in preterm infants. Early Hum Dev. (2014) 90:535–40. doi: 10.1016/j.earlhumdev.2014.04.015
46. Goldsmith SJ, Dickson JS, Barnhart HM, Toledo RT, Eiten-Miller RR. IgA, IgG, IgM and lactoferrin contents of human milk during early lactation and the effect of processing and storage. J Food Prot. (1983) 46:4–7. doi: 10.4315/0362-028X-46.1.4
47. Peitersen B, Bohn L, Andersen H. Quantitative determination of immunoglobulins, lysozyme, and certain electrolytes in breast milk during the entire period of lactation, during a 24-hour period, and in milk from the individual mammary gland. Acta Paediatr Scand. (1975) 64:709–17. doi: 10.1111/j.1651-2227.1975.tb03909.x
48. França EL, Nicomedes T. dos R, Calderon I de MP, França ACH. Time-dependent alterations of soluble and cellular components in human milk. Biol Rhythm Res. (2010) 41:333–47. doi: 10.1080/09291010903407441
49. Morais TC, Honorio-França AC, Silva RR, Fujimori M, Fagundes DLG, França EL. Temporal fluctuations of cytokine concentrations in human milk. Biol Rhythm Res. (2015) 46:811–21. doi: 10.1080/09291016.2015.1056434
50. Pontes GN, Cardoso EC, Carneiro-Sampaio MMS, Markus RP. Pineal melatonin and the innate immune response: the TNF-alpha increase after cesarean section suppresses nocturnal melatonin production. J Pineal Res. (2007) 43:365–71. doi: 10.1111/j.1600-079X.2007.00487.x
51. Arduini D, Rizzo G, Parlati E, Dell'Acqua S, Romanini C, Mancuso S. Loss of circadian rhythms of fetal behaviour in a totally adrenalectomized pregnant woman. Gynecol Obstet Invest. (1987) 23:226–9. doi: 10.1159/000298865
52. Patrick J, Challis J, Campbell K, Carmichael L, Richardson B, Tevaarwerk G. Effects of synthetic glucocorticoid administration on human fetal breathing movements at 34 to 35 weeks' gestational age. Am J Obstet Gynecol. (1981) 139:324–8. doi: 10.1016/0002-9378(81)90019-3
53. McKenna H, Reiss IKM. The case for a chronobiological approach to neonatal care. Early Hum Dev. (2018) 126:1–5. doi: 10.1016/j.earlhumdev.2018.08.012
54. Mirmiran M, Maas YGH, Ariagno RL. Development of fetal and neonatal sleep and circadian rhythms. Sleep Med Rev. (2003) 7:321–34. doi: 10.1053/smrv.2002.0243
55. Caba M, González-Mariscal G, Meza E. Circadian rhythms and clock genes in reproduction: insights from behavior and the female rabbit's brain. Front Endocrinol. (2018) 9:106. doi: 10.3389/fendo.2018.00106
56. Rivkees SA. The development of circadian rhythms: from animals to humans. Sleep Med Clin. (2007) 2:331–41. doi: 10.1016/j.jsmc.2007.05.010
57. McGraw K, Hoffmann R, Harker C, Herman JH. The development of circadian rhythms in a human infant. Sleep. (1999) 22:303–10. doi: 10.1093/sleep/22.3.303
58. Porkka-Heiskanen T, Alanko L, Kalinchuk A, Stenberg D. Adenosine and sleep. Sleep Med Rev. (2002) 6:321–32. doi: 10.1053/smrv.2001.0201
59. Zhdanova IV, Simmons M, Marcus JN, Busza AC, Leclair OU, Taylor JA. Nocturnal increase in plasma cGMP levels in humans. J Biol Rhythms. (1999) 14:307–13. doi: 10.1177/074873099129000722
60. Kent JC, Mitoulas LR, Cregan MD, Ramsay DT, Doherty DA, Hartmann PE. Volume and frequency of breastfeedings and fat content of breast milk throughout the day. Pediatrics. (2006) 117:e387–395. doi: 10.1542/peds.2005-1417
61. Cubero J, Valero V, Sánchez J, Rivero M, Parvez H, Rodríguez AB, et al. The circadian rhythm of tryptophan in breast milk affects the rhythms of 6-sulfatoxymelatonin and sleep in newborn. Neuro Endocrinol Lett. (2005) 26:657–61.
62. Cubero J, Narciso D, Terrón P, Rial R, Esteban S, Rivero M, et al. Chrononutrition applied to formula milks to consolidate infants' sleep/wake cycle. Neuro Endocrinol Lett. (2007) 28:360–6.
63. Cubero J, Chanclón B, Sánchez S, Rivero M, Rodríguez AB, Barriga C. Improving the quality of infant sleep through the inclusion at supper of cereals enriched with tryptophan, adenosine-5'-phosphate, and uridine-5'-phosphate. NutrNeurosci. (2009) 12:272–80. doi: 10.1179/147683009X423490
64. Hashimoto S, Kohsaka M, Morita N, Fukuda N, Honma S, Honma K. Vitamin B12 enhances the phase-response of circadian melatonin rhythm to a single bright light exposure in humans. Neurosci Lett. (1996) 220:129–32. doi: 10.1016/S0304-3940(96)13247-X
65. Ohta T, Ando K, Iwata T, Ozaki N, Kayukawa Y, Terashima M, et al. Treatment of persistent sleep-wake schedule disorders in adolescents with methylcobalamin (vitamin B12). Sleep. (1991) 14:414–8.
66. Uchiyama M, Mayer G, Okawa M, Meier-Ewert K. Effects of vitamin B12 on human circadian body temperature rhythm. Neurosci Lett. (1995) 192:1–4. doi: 10.1016/0304-3940(95)11591-J
67. Goedhart G, van der Wal MF, van Eijsden M, Bonsel GJ. Maternal vitamin B-12 and folate status during pregnancy and excessive infant crying. Early Hum Dev. (2011) 87:309–14. doi: 10.1016/j.earlhumdev.2011.01.037
68. Kikuchi S, Nishihara K, Horiuchi S, Eto H. The influence of feeding method on a mother's circadian rhythm and on the development of her infant's circadian rest-activity rhythm. Early Hum Dev. (2020) 145:105046. doi: 10.1016/j.earlhumdev.2020.105046
69. Abdul Jafar NK, Tham EKH, Pang WW, Fok D, Chua MC, Teoh OH, et al. Association between breastfeeding and sleep patterns in infants and preschool children. Am J Clin Nutr. (2021) 114:1986–96. doi: 10.1093/ajcn/nqab297
70. Cohen Engler A, Hadash A, Shehadeh N, Pillar G. Breastfeeding may improve nocturnal sleep and reduce infantile colic: potential role of breast milk melatonin. Eur J Pediatr. (2012) 171:729–32. doi: 10.1007/s00431-011-1659-3
71. García-Pergañeda A, Pozo D, Guerrero JM, Calvo JR. Signal transduction for melatonin in human lymphocytes: involvement of a pertussis toxin-sensitive G protein. J Immunol. (1997) 159:3774–81.
72. Fernandes PACM, Cecon E, Markus RP, Ferreira ZS. Effect of TNF-alpha on the melatonin synthetic pathway in the rat pineal gland: basis for a “feedback” of the immune response on circadian timing. J Pineal Res. (2006) 41:344–50. doi: 10.1111/j.1600-079X.2006.00373.x
73. Singhal A, Kennedy K, Lanigan J, Clough H, Jenkins W, Elias-Jones A, et al. Dietary nucleotides and early growth in formula-fed infants: a randomized controlled trial. Pediatrics. (2010) 126:e946–953. doi: 10.1542/peds.2009-2609
74. Wang L, Mu S, Xu X, Shi Z, Shen L. Effects of dietary nucleotide supplementation on growth in infants: a meta-analysis of randomized controlled trials. Eur J Nutr. (2019) 58:1213–21. doi: 10.1007/s00394-018-1640-2
75. Pastor N, Soler B, Mitmesser SH, Ferguson P, Lifschitz C. Infants fed docosahexaenoic acid- and arachidonic acid-supplemented formula have decreased incidence of bronchiolitis/bronchitis the first year of life. Clin Pediatr. (2006) 45:850–5. doi: 10.1177/1073858406289801
76. Hanlon EC, Tasali E, Leproult R, Stuhr KL, Doncheck E, de Wit H, et al. Sleep restriction enhances the daily rhythm of circulating levels of endocannabinoid 2-arachidonoylglycerol. Sleep. (2016) 39:653–64. doi: 10.5665/sleep.5546
77. Monteleone AM, Di Marzo V, Monteleone P, Dalle Grave R, Aveta T, Ghoch ME, et al. Responses of peripheral endocannabinoids and endocannabinoid-related compounds to hedonic eating in obesity. Eur J Nutr. (2016) 55:1799–805. doi: 10.1007/s00394-016-1153-9
78. Johnsson IW, Lindberger E, Ahlsson F, Gustafsson J, Lundgren ME. Relation of maternal birthweight with early pregnancy obesity, gestational diabetes, and offspring macrosomia. J Dev Orig Health Dis. (2022). doi: 10.1017/S2040174421000751
79. Fride E. The endocannabinoid-CB(1) receptor system in pre- and postnatal life. Eur J Pharmacol. (2004) 500:289–97. doi: 10.1016/j.ejphar.2004.07.033
80. Franco-Antonio C, Santano-Mogena E, Chimento-Díaz S, Sánchez-García P, Cordovilla-Guardia S. A randomised controlled trial evaluating the effect of a brief motivational intervention to promote breastfeeding in postpartum depression. Sci Rep. (2022) 12:373. doi: 10.1038/s41598-021-04338-w
81. Miller CL, White R, Whitman TL, O'Callaghan MF, Maxwell SE. The effects of cycled versus noncycled lighting on growth and development in preterm infants. Infant Behav Dev. (1995) 18:87–95. doi: 10.1016/0163-6383(95)90010-1
82. Zores-Koenig C, Kuhn P, Caeymaex L, the Group of Reflection and Evaluation of the Environment of Newborns study group of the French Neonatology Society. Recommendations on neonatal light environment from the French Neonatal Society. Acta Paediatr. (2020) 109:1292–301. doi: 10.1111/apa.15173
83. Bar S, Milanaik R, Adesman A. Long-term neurodevelopmental benefits of breastfeeding. CurrOpinPediatr. (2016) 28:559–66. doi: 10.1097/MOP.0000000000000389
84. Wu Y, Lye S, Dennis CL, Briollais L. Exclusive breastfeeding can attenuate body-mass-index increase among genetically susceptible children: a longitudinal study from the ALSPAC cohort. PLoS Genet. (2020) 16:e1008790. doi: 10.1371/journal.pgen.1008790
85. Pérez-Escamilla R, Martinez JL, Segura-Pérez S. Impact of the baby-friendly hospital initiative on breastfeeding and child health outcomes: a systematic review. Matern Child Nutr. (2016) 12:402–17. doi: 10.1111/mcn.12294
86. Indrio F, Martini S, Francavilla R, Corvaglia L, Cristofori F, Mastrolia SA, et al. Epigenetic matters: the link between early nutrition, microbiome, and long-term health development. Front Pediatr. (2017) 5:178. doi: 10.3389/fped.2017.00178
87. Walters DD, Phan LTH, Mathisen R. The cost of not breastfeeding: global results from a new tool. Health Policy Plan. (2019) 34:407–17. doi: 10.1093/heapol/czz050
88. WHO Immediate KMC Study Group, Arya S, Naburi H, Kawaza K, Newton S, Anyabolu CH, et al. Immediate “Kangaroo Mother Care” and survival of infants with low birth weight. N Engl J Med. (2021) 384:2028–38. doi: 10.1056/NEJMoa2026486
Keywords: chrononutrition, melatonin, glucocorticoids, circadian feeding, tryptophan, cannabinoids, oligosaccharides, secretory IgA
Citation: Caba-Flores MD, Ramos-Ligonio A, Camacho-Morales A, Martínez-Valenzuela C, Viveros-Contreras R and Caba M (2022) Breast Milk and the Importance of Chrononutrition. Front. Nutr. 9:867507. doi: 10.3389/fnut.2022.867507
Received: 01 February 2022; Accepted: 11 April 2022;
Published: 12 May 2022.
Edited by:
Carolina Escobar, National Autonomous University of Mexico, MexicoReviewed by:
Theresa M. Casey, Purdue University, United StatesCopyright © 2022 Caba-Flores, Ramos-Ligonio, Camacho-Morales, Martínez-Valenzuela, Viveros-Contreras and Caba. This is an open-access article distributed under the terms of the Creative Commons Attribution License (CC BY). The use, distribution or reproduction in other forums is permitted, provided the original author(s) and the copyright owner(s) are credited and that the original publication in this journal is cited, in accordance with accepted academic practice. No use, distribution or reproduction is permitted which does not comply with these terms.
*Correspondence: Mario Caba, mcaba@uv.mx