- National Key Disciplines of Nutrition and Food Hygiene, Department of Nutrition and Food Hygiene, School of Public Health, Harbin Medical University, Harbin, China
Fatty acid amides (FAAs) are endogenous lipid molecules that exhibit various physiological activities. FAAs are usually present at nanomolar levels in biological samples. In this study, a method for the qualitative and quantitative determination of six FAAs (linoleamide, linoleoyl ethanolamide, oleoyl ethanolamide, palmitic amide, oleamide, and octadecanamide) in edible vegetable oils was established. All six FAAs were detected in sesame, peanut, soybean (decolorized and non-decolorized), and blended oils; five in sunflower oil; four in rice oil; three in linseed and olive oils; and two in corn and canola oils. The total contents of FAAs were highest in sesame oil (104.88 ± 3.01 μg/mL), followed by peanut oil (34.96 ± 3.87 μg/mL), soybean oil (16.75 ± 1.27 μg/mL), and blended oil (13.33 ± 0.77 μg/mL), and the contents in the other edible vegetable oils were all <1.03 μg/mL. The concentrations of linoleoyl ethanolamide and oleoyl ethanolamide were highest in non-decolorized soybean oil, while the other four FAAs (linoleamide, palmitic amide, oleamide, and octadecanamide) showed the highest concentrations in sesame oil. The total contents of these FAAs in eight different oils were higher than those in biological fluids and tissue. Our study confirmed that edible vegetable oils are rich in FAAs, and provides reliable data for evaluating the nutritive value of vegetable oils.
Introduction
Fatty acid amides (FAAs) are bioactive lipid signaling molecules that have a variety of physiological activities. They play key roles in biological functions, such as sleep induction, analgesic, anti-anxiety, anti-convulsion, and anti-epilepsy activities, neuroprotection, and they promote fat hydrolysis and weight loss (1–7). For example, linoleamide has been reported to exert sedative and hypnotic effects, and inhibits the migration of cancer cells in human subjects (8, 9). Linoleoyl ethanolamide can regulate pain, food intake, and blood sugar level (10, 11). Oleoyl ethanolamide can inhibit food intake, promote fat hydrolysis, and reduce body weight (12–14). Palmitic amide can induce sleep in animals and exhibit central inhibitory and anticonvulsant effects (15). Finally, oleamide has analgesic, anti-anxiety, and sleep induction activities, and can improve memory and reduce cognitive impairment, as well as preventing Alzheimer's disease, cardiovascular disease, and inflammation (5, 16–18). Owing to their broad range of bioactive functions, FAAs have generated a great deal of interest, especially in the fields of pharmacy and nutrition (19–23).
At present, FAAs are generally considered to be endogenous lipid molecules that are found mainly in mammals, such as cats, squirrels, and humans (19, 24–27). However, their concentrations in tissues and biological fluids of mammals are low, typically in the nanomolar range (26, 28). As a result, the extraction and purification of FAAs from tissues or organs is challenging.
Palmitic amide has been found in soybeans, peanut oil, and egg whites (29) and palmitic amide, oleamide, and N-acylethanolamines have been detected in different edible vegetable oils (30–33). In our previous study, we used liquid chromatography–mass spectrometry (LC–MS) to identify six FAAs in peanut oil: linoleoyl ethanolamide, linoleamide, oleoyl ethanolamide, palmitic amide, oleamide, and octadecanamide (34). This work showed that edible vegetable oils may be rich in FAAs. If enough FAAs are isolated or consumed from vegetable oils, they may play important roles in clinical treatment or nutritional intervention. Therefore, it is important to identify the types of FAAs present in different edible vegetable oils and to determine their contents. However, no qualitative and quantitative method of analysis for FAAs is available.
Therefore, this study aimed to establish a qualitative and quantitative method for the simultaneous determination of the six FAAs using ultra-performance liquid chromatography–tandem quadrupole time-of-flight mass spectrometry (UPLC/Q-TOF MSMS). The proposed method was used to identify FAAs and compare their concentrations in 11 edible vegetable oils, thus providing a basis for evaluating the nutritional value of different edible vegetable oils.
Materials and Methods
Materials and Reagents
Decolorized and non-decolorized soybean oils (Jiusan Oils and Grains Industries Group Co., Ltd., Heilongjiang, China), sesame oil (Liaoning Qingyan Temple Food Co., Ltd., Liaoning, China), peanut oil (Shandong Luhua Group Co., Ltd., Shandong, China), canola oil (Hulun Buir Heshijia Food Co., Ltd., Inner Mongolia, China), corn oil (Xiwang Foodstuffs Co., Ltd., Shandong, China), linseed oil (Ngol League Hong Jing Yuan Grease Co., Ltd., Inner Mongolia, China), olive oil (Mueloliva, Cordoba, Spain), rice oil (China Resources Ng Fung, Shenzhen, China), sunflower oil (Shanghai Standard Foods Co., Ltd., Shanghai, China), and blended oil (sunflower oil 25%, canola oil 24%, corn oil 18.9%, soybean oil 18%, peanut oil 6%, rice oil 5%, linseed oil 2.5%, sesame oil 0.6%; Yihai Kerry Arawana Holdings Co., Ltd, Shanghai, China) were purchased in local supermarkets in Harbin, China.
Acetonitrile and methanol (Fisher Scientific, Suwannee, GA, USA), and formic acid (Tianjin Institute of Fine Chemicals, Tianjin, China) were of chromatography grade. Ultra-pure water was obtained from a water purification system (Milli-Q, Milford, MA, USA). Standard compounds, linoleoyl ethanolamide, linoleamide, oleoyl ethanolamide, palmitic amide, oleamide, and octadecanamide (purity ≥98%) were obtained from Shanghai ZZBio (Shanghai, China). Stock solutions of the six FAAs (100 μg/mL) were prepared with methanol and stored in amber glass vials at −20°C until analysis.
Experimental Methods
Sample Pretreatment
A sample of vegetable oil (0.8 mL) was put into a 15-mL Eppendorf tube with 1.6 mL of methanol solution, mixed by vortexing for 3 min (Scientific Industries Inc., Bohemia, NY, USA), and then centrifuged at 58.3 ×g for 10 min in a refrigerated high-speed centrifuge (Eppendorf AG, Hamburg, Germany). The supernatant (400 μL) was removed, added to a sample bottle, and then analyzed by ultra-performance liquid chromatography (ACQUITY UPLC System, Waters, Milford, MA, USA) coupled with mass spectrometric detection (Micromass Q-Tof Micro; Waters, Wilmslow, UK).
UPLC Conditions
Analysis of the composition of peanut oil in our previous study showed that the six FAAs could be well separated using an ACQUITY UPLC BEH C18 column (100 × 2.1 mm; 1.7 μm, Waters) (34). Therefore, the same column and chromatographic conditions were used to separate the six FAAs in the 11 edible vegetable oils investigated in the present study. For the UPLC chromatographic separation, the column temperature was 35°C, the sample chamber temperature was 4°C, the mobile phase flow rate was 350 μL/min, and the injection volume was 4 μL. Mobile phase A was ultrapure water containing 0.1% formic acid and mobile phase B was acetonitrile. A linear gradient elution procedure was performed as follows: 0–0.5 min, 98% A; 0.5–3 min, 98–30% A; 3–10.5 min, 30–2% A; 10.5–12 min, 2% A; 12–14 min, 2–98% A; 14–16 min, 98% A.
Mass Spectrometry Conditions
The mass spectrometer (Micromass Q-Tof Micro, Waters) interfaced with the ESI source was operated in the positive ion mode (ESI+). The system analytical parameters were as follows: capillary voltage, 3.0 kV; cone hole voltage, 35 V; source temperature, 125°C; cone gas flow (nitrogen), 50 L/h; desolvation temperature, 320°C; desolvation gas (nitrogen) flow, 720 L/h; collision gas, argon; microchannel plate detector voltage, 2400 V. Leucine-enkephalin (Waters) was used as the lock mass (m/z 556.2771) in ESI+ at a concentration of 200 pg/mL. The data were collected in centroid mode using the full scan mode at a mass ratio of m/z 50–1,000, and the data were collected from 0 to 16 min. The Q-TOF mass acquisition rate was set at 0.4 with an interscan delay of 0.1 s.
Qualitative and Quantitative Method
The retention times and the parent ion peaks of the different FAAs were obtained by first-order mass spectrometry of the six FAA standard solutions. The optimal secondary mass spectra (MSMS) of the different FAAs were obtained by optimizing the collision energy. The characteristic fragment ions of the six FAAs were selected as the qualitative ions. The types of potential FAAs in different edible vegetable oils were qualitatively analyzed by comparing their retention times, the mass charge ratios (m/z) of the parentions, and the characteristic fragment ions of the standard substances.
Standard solutions of the six FAAs were prepared by diluting the standard solutions with methanol. The linear measuring ranges were evaluated by constructing standard curves for each standard.
Evaluationof Methodology
Recovery Rate of Method
The recoveries of the added standards were assessed by comparing the concentrations of the six FAAs in peanut oil before and after the addition of specific amounts of FAAs. The mixed standard solutions of the six FAAs at low, medium, and high concentrations (0.1, 0.5, and 1 μg/mL) were added to the peanut oil. Six parallel samples were made for each concentration. The samples were prepared as described in Sample Pretreatment, and the concentrations were calculated by quantitative analysis as described below. Peanut oil with no added standard solution was also analyzed six times in parallel, and the concentrations of the six FAAs were calculated. The recoveries of the added standards were calculated according to the formula:
where Cadded sample is the concentration of sample with the added standards; Cunadded sample is the concentration of the sample without the added standards.
Precision of Method
Replicate analyses (n = 6) of three QC samples were conducted to assess the intra-assay and inter-day precision and accuracy. The precision was evaluated by the coefficient of variation (CV%) and the accuracy was calculated as the bias or percentage deviation between the nominal and measured concentrations.
Limits of Detection and Quantitation
The limits of detection (LOD) and quantitation (LOQ) of the six different FAAs were calculated as the concentration that provided signals three and ten times higher than the background noise, respectively, measured at a time close to each chromatographic signal.
Qualitative and Quantitative Analysis of FAAs in Edible Vegetable Oils
Using the established method, six FAAs in 11 common edible vegetable oils were qualitatively and quantitatively analyzed. Each sample was measured three times in parallel. The contents of the different FAAs in each sample were calculated from the standard working curve.
Results
Chromatography and Mass Spectrometry
As shown in Figure 1, the six types of FAA were well separated under the chromatographic conditions, confirming that the method can be used to separate and detect FAAs in vegetable oil.
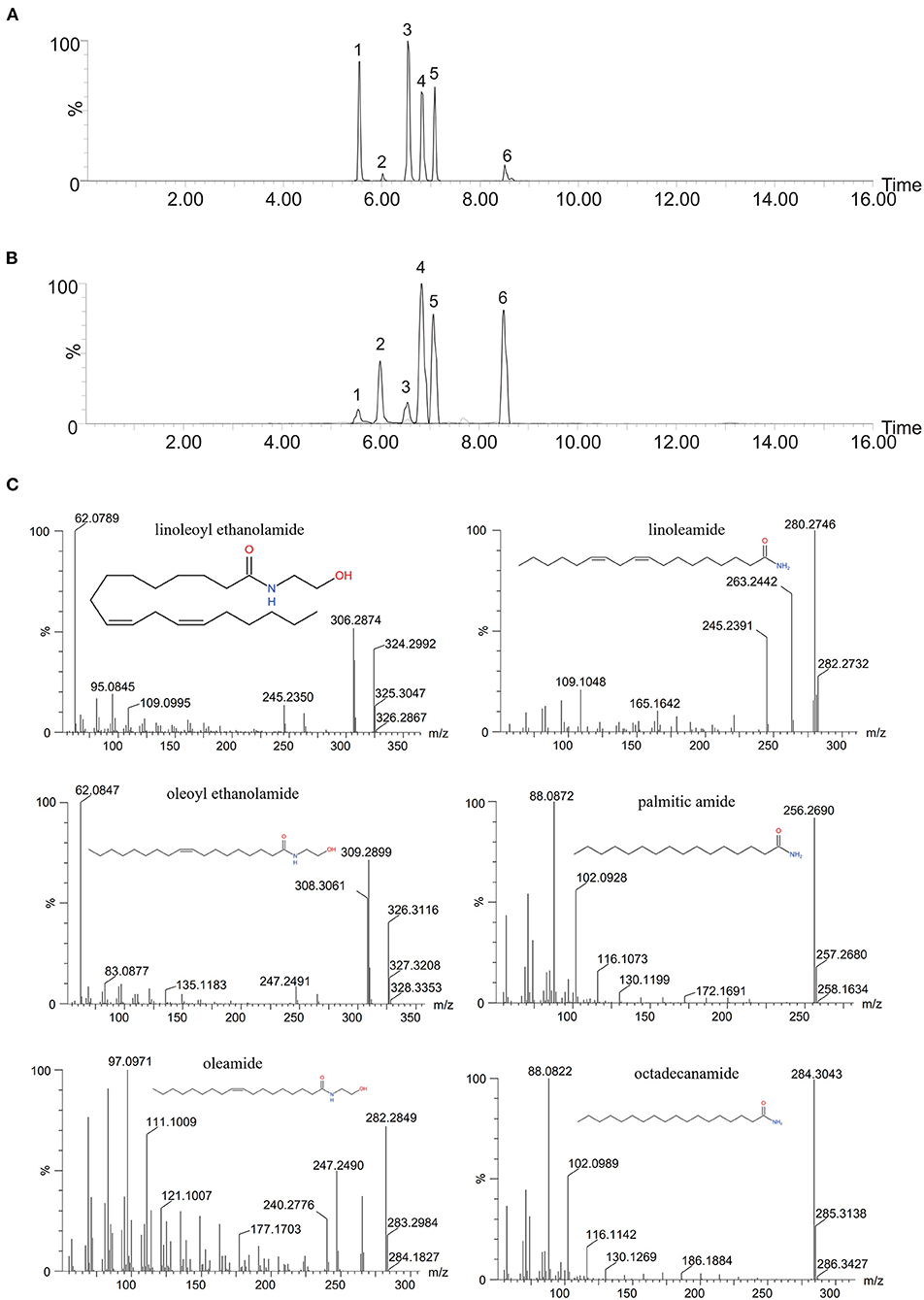
Figure 1. Base peak intensity ion chromatograms and MSMS spectra of six FAAs in standard solution and vegetable oil. (A) Six FAAs in standard solutions; (B) six FAAs in sesame oil. 1: Linoleoyl ethanolamide, 2: linoleamide, 3: oleoyl ethanolamide, 4: palmitic amide, 5: oleamide, 6: octadecanamide. (C) MSMS spectra.
The optimal secondary mass spectra of each FAA were obtained by optimizing the collision energy, with the two characteristic ions with the highest abundance selected as the qualitative ions. The parent ions, qualitative ions, and mass spectrum parameters of the six FAAs are shown in Table 1. These data can be used for qualitative analysis of the target compounds in different edible vegetable oils.
Method Evaluation
Standard Curves, Limits of Detection, and Limits of Quantitation
Table 2 shows that the linear relationships of the six FAAs were good over the stated linear ranges, with all R2 values >0.99. The LODs were 0.001–0.01 μg/mL and the LOQs were 0.005–0.02 μg/mL.
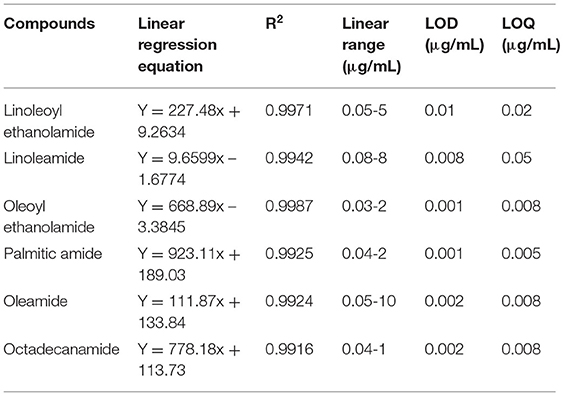
Table 2. Linear regression equations, linear ranges, detection limits and quantitation limits of standard curves for six fatty acid amides.
Recovery of Added Standards and Method Precision
Supplementary Table 1 shows that the recoveries of the added standards of the six FAAs were in the range of 86.2–96.0%, and the relative standard deviations (RSD) from six measurements were in the range of 3.6–11.3%. Supplementary Table 2 shows that the RSD for intra-day precision ranged from 3.5 to 7.7%, and the RSD of inter-day precision ranged from 6.2 to 11.3%. These results indicated that the established method has good accuracy and precision, and can be used for the quantitative analysis of FAAs in edible vegetable oils.
Qualitative and Quantitative Analysis of FAAs in Edible Vegetable Oils
Using the established method, qualitative and quantitative analyses of the six FAAs in 11 edible vegetable oils were conducted. Table 3 shows that all six FAAs were detected in sesame, peanut, soybean (decolorized and non-decolorized), and blended oils; five were detected in the rice and sunflower oils, three in the olive and linseed oils, and two in the corn and canola oils.
The quantitative results indicated that the contents of FAAs varied among the different vegetable oils. Of the 11 edible vegetable oils, the total concentration of FAAs was the highest in sesame oil (84.89 μg/mL), followed by peanut oil (34.96 μg/mL) and soybean oil (non-decolorized) (16.76 μg/mL). The total concentrations of FAAs in the sunflower, rice, linseed, olive, corn, and canola oils were all <1.03 μg/mL, with that in canola oil being the lowest.
The concentrations of linoleamide, palmitic amide, oleamide, and octadecanamide were highest in sesame oil, and were all more than twice those in the other edible vegetable oils. The concentrations of linoleoyl ethanolamide and oleoyl ethanolamide were highest in soybean oil (non-decolorized).
Discussion
Because of its advantages of rapid analysis, high sensitivity, and qualitative and quantitative accuracy, LC–MS has been used in a variety of fields to determine the contents of various nutrients in edible vegetable oils (35–37) and in heated vegetable oils used for frying (38, 39). In the present study, we have established for the first time a qualitative and quantitative method for determining six types of FAA in edible vegetable oils. The retention time and characteristic ions were used to identify the amides in the qualitative results by eliminating interference caused by chromatographic time drift. Therefore, our established method will be of great use for the accurate quantitative analysis of different FAAs in edible vegetable oils.
Our quantitative results showed that of the six FAAs analyzed in this study, two were detected in all 11 edible vegetable oils. The total contents of FAAs in eight different oils were higher than their concentrations in tissue and biological fluids (>1 nM). Therefore, our results indicated that edible vegetable oils, especially sesame, soybean, and peanut oils, are rich in FAAs, although the concentrations of the six FAAs varied in different vegetable oils. Sesame oil, peanut oil, the two types of soybean oil, and blended oil not only contained all six types of FAA, but also contained high total concentrations (Figure 2). Notably, the concentration of FAAs in decolorized soybean oil (5.46 μg/mL) was significantly lower than that in non-decolorized soybean oil (16.76 μg/mL), but the proportions of the six types of FAA were similarin both soybean oils. Nutrients, such as squalene, tocopherol, sterol, and carotenoid, have been reported to be lost or to decrease during the decolorization and deodorization processes used for edible vegetable oil (40, 41). Our results suggest that the refining process for edible oil reduced the contents of FAAs in decolorized soybean oil. Therefore, the refining process must be improved to retain nutrients to the greatest extent while still removing any harmful substances.
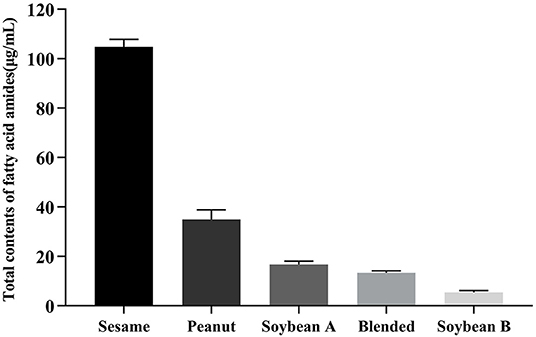
Figure 2. Total contents of six FFA in five edible vegetable oils. Soybean A: non-decolorized; soybean B: decolorized.
The blended oil used in the present study included soybean, peanut, sesame, and other edible vegetable oils in given proportions, and thus the six FAAs were also detected in the blended oil. Our results demonstrated that mixing different vegetable oils improves their nutritional value. The present study has also provided valuable information on the concentrations of FAAs in different vegetable oils that can aid in the preparation of blended oils.
The present study found that sesame oil contained six types of FAA, with higher concentrations of linoleamide, palmitic amide, oleamide, and octadecanamide than in the other vegetable oils. There sults suggested that these high concentrations of FAAs were responsible for the high nutritional value and good physiological functions of sesame oil. However, sesame oil is used mainly as a seasoning oil for culinary purposes, and therefore, total dietary intake tends to be relatively low. This means that its contribution in terms of dietary intake of FAAs is low. In contrast, peanut and soybean oils are used much more often and in larger amounts in daily life, and therefore their contributions in terms of dietary intake of FAAs are relatively high. Therefore, to ensure adequate dietary intake of FAAs, it is recommended that the availability of FAA-rich oils be promoted by increasing production of peanut and non-decolorized soybean oil, as well as blended oils rich in sesame and peanut oils.
Conclusion
Our established analytical method can be used for qualitative and quantitative analysis of the FAAs in a wide range of edible vegetable oils. Our study confirmed that edible vegetable oils, especially sesame, soybean, and peanut oils, are rich in FAAs. This work provides a reference for the detection of FAAs in edible vegetable oils and provides a scientific basis for further evaluation of the nutritional value, production technology, improved blending, and consumption patterns of edible vegetable oils.
Data Availability Statement
The original contributions presented in the study are included in the article/Supplementary Material, further inquiries can be directed to the corresponding authors.
Author Contributions
MW and CS contributed to the study's conception and design. ZL and FD drafted and revised the manuscript and performed experimental work. FD, YS, ZS, and XS performed data collection and data analysis. YD, XH, JZ, and RZ performed data verification and data presentation. The final draft was read and approved by all authors.
Funding
This work was supported by a grant from National Nature Science Foundation of China [81973036].
Conflict of Interest
The authors declare that the research was conducted in the absence of any commercial or financial relationships that could be construed as a potential conflict of interest.
Publisher's Note
All claims expressed in this article are solely those of the authors and do not necessarily represent those of their affiliated organizations, or those of the publisher, the editors and the reviewers. Any product that may be evaluated in this article, or claim that may be made by its manufacturer, is not guaranteed or endorsed by the publisher.
Acknowledgments
The authors thank Liwen Bianji (www.liwenbianji.cn/) for English language editing and review services.
Supplementary Material
The Supplementary Material for this article can be found online at: https://www.frontiersin.org/articles/10.3389/fnut.2022.857858/full#supplementary-material
References
1. Hermes DJ, Xu C, Poklis JL, Niphakis MJ, Cravatt BF, Mackie K, et al. Neuroprotective effects of fatty acid amide hydrolase catabolic enzyme inhibition in a HIV-1 Tat model of neuroAIDS. Neuropharmacology. (2018) 141:55–65. doi: 10.1016/j.neuropharm.2018.08.013
2. Murkar A, De Koninck J, Merali Z. Cannabinoids: revealing their complexity and role in central networks of fear and anxiety. Neurosci Biobehav Rev. (2021) 131:30–46. doi: 10.1016/j.neubiorev.2021.09.002
3. Ishida T, Nishiumi S, Tanahashi T, Yamasaki A, Yamazaki A, Akashi T, et al. Linoleoyl ethanolamide reduces lipopolysaccharide-induced inflammation in macrophages and ameliorates 2,4-dinitrofluorobenzene-induced contact dermatitis in mice. Eur J Pharmacol. (2013) 699:6–13. doi: 10.1016/j.ejphar.2012.11.030
4. Xu X, Guo H, Jing Z, Yang L, Chen C, Peng L, et al. N-Oleoylethanolamine Reduces Inflammatory Cytokines and Adhesion Molecules in TNF-alpha-induced Human Umbilical Vein Endothelial Cells by Activating CB2 and PPAR-alpha. J Cardiovasc Pharmacol. (2016) 68:280–91. doi: 10.1097/FJC.0000000000000413
5. Nam HY, Na EJ, Lee E, Kwon Y, Kim HJ. Antiepileptic and Neuroprotective Effects of Oleamide in Rat Striatum on Kainate-Induced Behavioral Seizure and Excitotoxic Damage via Calpain Inhibition. Front Pharmacol. (2017) 8:817. doi: 10.3389/fphar.2017.00817
6. Post JM, Loch S, Lerner R, Remmers F, Lomazzo E, Lutz B, et al. Antiepileptogenic Effect of Subchronic Palmitoylethanolamide Treatment in a Mouse Model of Acute Epilepsy. Front Mol Neurosci. (2018) 11:67. doi: 10.3389/fnmol.2018.00067
7. Kobayashi Y, Watanabe N, Kitakaze T, Sugimoto K, Izawa T, Kai K, et al. Oleamide rescues tibialis anterior muscle atrophy of mice housed in small cages. Br J Nutr. (2021) 126:481–91. doi: 10.1017/S0007114520004304
8. Huang JK, Jan CR. Linoleamide, a brain lipid that induces sleep, increases cytosolic Ca2+ levels in MDCK renal tubular cells. Life Sci. (2001) 68:997–1004. doi: 10.1016/S0024-3205(00)01002-X
9. Yerlikaya S, Baloglu MC, Diuzheva A, Jeko J, Cziaky Z, Zengin G. Investigation of chemical profile, biological properties of Lotus corniculatus L. extracts and their apoptotic-autophagic effects on breast cancer cells. J Pharm Biomed Anal. (2019) 174:286–99. doi: 10.1016/j.jpba.2019.05.068
10. Hansen HS. Role of anorectic N-acylethanolamines in intestinal physiology and satiety control with respect to dietary fat. Pharmacol Res. (2014) 86:18–25. doi: 10.1016/j.phrs.2014.03.006
11. Ho M, Anderson GH, Lin L, Bazinet RP, Kubant R. Ethanolamides of essential alpha-linolenic and linoleic fatty acids suppress short-term food intake in rats. Food Funct. (2020) 11:3066–72. doi: 10.1039/C9FO02884F
12. Fu J, Gaetani S, Oveisi F, Lo Verme J, Serrano A, Rodriguez De Fonseca F, et al. Oleylethanolamide regulates feeding and body weight through activation of the nuclear receptor PPAR-alpha. Nature. (2003) 425:90–3. doi: 10.1038/nature01921
13. Laleh P, Yaser K, Abolfazl B, Shahriar A, Mohammad AJ, Nazila F, et al. Oleoylethanolamide increases the expression of PPAR-Alpha and reduces appetite and body weight in obese people: a clinical trial. Appetite. (2018) 128:44–9. doi: 10.1016/j.appet.2018.05.129
14. Brown JD, Karimian Azari E, Ayala JE. Oleoylethanolamide: a fat ally in the fight against obesity. Physiol Behav. (2017) 176:50–8. doi: 10.1016/j.physbeh.2017.02.034
15. Peng F, Hong YU, Feng XU, Zhou B, Liu D, Zhao Q. Central inhibition effect of the egg oil of Rana temporaria chensinensis David egg oil. J Shenyang Pharmac Univ. (2002) 19:204–7. doi: 10.3969/j.issn.1006-2858.2002.03.015
16. Hernandez-Diaz C, Juarez-Oropeza MA, Mascher D, Pavon N, Regla I, Paredes-Carbajal MC. Effects of Oleamide on the Vasomotor Responses in the Rat. Cannabis Cannabinoid Res. (2020) 5:42–50. doi: 10.1089/can.2019.0014
17. Moon SM, Lee SA, Hong JH, Kim JS, Kim DK, Kim CS. Oleamide suppresses inflammatory responses in LPS-induced RAW264. 7 murine macrophages and alleviates paw edema in a carrageenan-induced inflammatory rat model. Int Immunopharmacol. (2018) 56:179–85. doi: 10.1016/j.intimp.2018.01.032
18. Tao R, Huang S, Zhou J, Ye L, Shen X, Wu J, et al. Neonatal supplementation of oleamide during suckling promotes learning ability and memory in adolescent mice. J Nutr. (2021) 152:889–98. doi: 10.1093/jn/nxab442
19. Farrell EK, Merkler DJ. Biosynthesis, degradation and pharmacological importance of the fatty acid amides. Drug Discov Today. (2008) 13:558–68. doi: 10.1016/j.drudis.2008.02.006
20. Dongdem JT, Helegbe GK, Opare-Asamoah K, Wezena CA, Ocloo A. Assessment of NSAIDs as potential inhibitors of the fatty acid amide hydrolase I (FAAH-1) using three different primary fatty acid amide substrates in vitro. BMC Pharmacol Toxicol. (2022) 23:1. doi: 10.1186/s40360-021-00539-1
21. Rodrigues de Oliveira F, Eleuterio Rodrigues K, Hamoy M, Sarquis Í R, Otake Hamoy A, Luiz Martins do Nascimento J, et al. Fatty Acid Amides Synthesized from Andiroba Oil (Carapa guianensis Aublet). Exhibit Anticonvulsant Action with Modulation on GABA-A Receptor in Mice: A Putative Therapeutic Option. Pharmaceuticals (Basel). (2020) 13:43. doi: 10.3390/ph13030043
22. Ni R, Bhandari S, Mitchell PR Jr, Suarez G, Patel NB, Lamb K, et al. Synthesis, Quantification, and Characterization of Fatty Acid Amides from In Vitro and In Vivo Sources. Molecules. (2021) 26:2543. doi: 10.3390/molecules26092543
23. Dovrtelova G, Zendulka O, Noskova K, Jurica J, Pes O, Dusek J, et al. Effect of Endocannabinoid Oleamide on Rat and Human Liver Cytochrome P450 Enzymes in In Vitro and In Vivo Models. Drug Metab Dispos. (2018) 46:913–23. doi: 10.1124/dmd.117.079582
24. Arafat ES, Trimble JW, Andersen RN, Dass C, Desiderio DM. Identification of fatty acid amides in human plasma. Life Sci. (1989) 45:1679–87. doi: 10.1016/0024-3205(89)90278-6
25. Cravatt BF, Prospero-Garcia O, Siuzdak G, Gilula NB, Henriksen SJ, Boger DL, et al. Chemical characterization of a family of brain lipids that induce sleep. Science. (1995) 268:1506–9. doi: 10.1126/science.7770779
26. Divito EB, Cascio M. Metabolism, physiology, and analyses of primary fatty acid amides. Chem Rev. (2013) 113:7343–53. doi: 10.1021/cr300363b
27. Wakamatsu K, Masaki T, Itoh F, Kondo K, Sudo K. Isolation of fatty acid amide as an angiogenic principle from bovine mesentery. Biochem Biophys Res Commun. (1990) 168:423–9. doi: 10.1016/0006-291X(90)92338-Z
28. Castillo-Peinado LS, López-Bascón MA, Mena-Bravo A, Luque de Castro MD, Priego-Capote F. Determination of primary fatty acid amides in different biological fluids by LC-MS/MS in MRM mode with synthetic deuterated standards: Influence of biofluid matrix on sample preparation. Talanta. (2019) 193:29–36. doi: 10.1016/j.talanta.2018.09.088
29. Yang JY, Chunfu WU. Progress in the study of endogenous fatty acid amides. Chin Pharmacol Bull. (2000) 16:1–3. doi: 10.3321/j.issn:1001-1978.2000.01.002
30. Bradshaw HB, Leishman E. Levels of bioactive lipids in cooking oils: olive oil is the richest source of oleoyl serine. J Basic Clin Physiol Pharmacol. (2016) 27:247–52. doi: 10.1515/jbcpp-2015-0104
31. De Luca L, Ferracane R, Vitaglione P. Food database of N-acyl-phosphatidylethanolamines, N-acylethanolamines and endocannabinoids and daily intake from a Western, a Mediterranean and a vegetarian diet. Food Chem. (2019) 300:125218. doi: 10.1016/j.foodchem.2019.125218
32. Long Z. Chemical Constituents of Olive Oil and from Camellia Oleifera Seed Oil. J Chin Cereals Oils Assoc. (2008) 23:121–3.
33. Yuan X. GS-MS Determination of Chemical Components in Pure Sesame Oil. chin Cereals Oils Assoc. (2005) 20:131–3. doi: 10.3321/j.issn:1003-0174.2005.06.031
34. Li Z, Deng M, Wang C. Component analysis of peanut oil based on UPLC/Q-TOF MS/MS. China Oils and Fats. (2021) 46:122–7. doi: 10.19902/j.cnki.zgyz.1003-7969.2021.03.024
35. Chernova A, Gubaev R, Mazin P, Goryunova S, Demurin Y, Gorlova L, et al. UPLC(-)MS Triglyceride Profiling in Sunflower and Rapeseed Seeds. Biomolecules. (2018) 9:9. doi: 10.3390/biom9010009
36. Peng Z, Zhu L, Deng M, Zhang X, Zheng Y. Simultaneous determination of 14 nutrients in vegetable oils by ultra high performance liquid chromatographytandem mass spectrometry. Chin J chromatograp. (2018) 36:1140–6. doi: 10.3724/SP.J.1123.2018.07013
37. Zhang L, Wang S, Yang R, Mao J, Jiang J, Wang X, et al. Simultaneous determination of tocopherols, carotenoids and phytosterols in edible vegetable oil by ultrasound-assisted saponification, LLE and LC-MS/MS. Food Chem. (2019) 289:313–9. doi: 10.1016/j.foodchem.2019.03.067
38. Aniołowska M, Kita A. The effect of frying on glycidyl esters content in palm oil. Food Chem. (2016) 203:95–103. doi: 10.1016/j.foodchem.2016.02.028
39. Li Z, Sun Y, Dong F, Yu Q, Fan L, Zhao J, et al. Discrimination of heating and frying vegetable oils based on UPLC/Q-TOF MSMS and chemometrics. Food Res Int. (2021) 140:109874. doi: 10.1016/j.foodres.2020.109874
40. Chen L, Liu Y, Aipeng HU, Tao LI. Effect of soybean oil deodorization and distillate trapping process on the quality of products. J Henan Univ Technol. (2018) 39:43–50. doi: 10.16433/j.cnki.issn1673-2383.2018.05.008
Keywords: edible vegetable oil, fatty acid amides, LC/MS, qualitative analysis, quantitative analysis, content
Citation: Li Z, Dong F, Sun Y, Sun Z, Song X, Dong Y, Huang X, Zhong J, Zhang R, Wang M and Sun C (2022) Qualitative and Quantitative Analysis of Six Fatty Acid Amides in 11 Edible Vegetable Oils Using Liquid Chromatography–Mass Spectrometry. Front. Nutr. 9:857858. doi: 10.3389/fnut.2022.857858
Received: 19 January 2022; Accepted: 02 March 2022;
Published: 28 March 2022.
Edited by:
Qing Guo, China Agricultural University, ChinaReviewed by:
Mohamed Fawzy Ramadan Hassanien, Umm Al-Qura University, Saudi ArabiaLeqi Cui, Florida State University, United States
Copyright © 2022 Li, Dong, Sun, Sun, Song, Dong, Huang, Zhong, Zhang, Wang and Sun. This is an open-access article distributed under the terms of the Creative Commons Attribution License (CC BY). The use, distribution or reproduction in other forums is permitted, provided the original author(s) and the copyright owner(s) are credited and that the original publication in this journal is cited, in accordance with accepted academic practice. No use, distribution or reproduction is permitted which does not comply with these terms.
*Correspondence: Maoqing Wang, d2FuZ19tYW9xaW5nQDEyNi5jb20=; Changhao Sun, Y2hhbmdoYW9zdW4yMDAyQDE2My5jb20=
†These authors have contributed equally to this work