- 1Jiangxi Province Key Laboratory of Animal Nutrition, Animal Nutrition and Feed Safety Innovation Team, College of Animal Science and Technology, Jiangxi Agricultural University, Nanchang, China
- 2Ganzhou Lvlinwan Agriculture and Animal Husbandry Co. Ltd., Ganzhou, China
This study evaluated the effect of glutamate supplementation on the physiological parameters of heat-stressed Hu sheep. Forty-eight male Hu sheep with an average initial body weight of 17.74 ± 0.17 kg were randomly divided into two groups: The control group (CON) was fed a basal diet and a treatment group (GLU) was fed a basal diet + 3 g/head/day of L-glutamate. There were six replications in each group with four sheep in each replication for a 90 days feeding test. Growth performance, serum biochemistry, and serum hormones were measured during phase 1 (1–30 days), phase 2 (31–60 days), and phase 3 (61–90 days) of the experiment; rumen fermentation characteristics, nutrient digestibility, and slaughter performance were measured at the end of the experimental periods. There were no differences in growth performance, serum biochemical indices, and immune indices between CON and GLU during phases 1 and 2. However, a higher average daily gain (ADG), a lower average daily feed intake (ADFI), and a lower F:G ratio (ADFI/ADG) were observed in GLU during phase 3 (p < 0.05). Serum levels of glutamate, globulin, immunoglobulin A, immunoglobulin G, immunoglobulin M, and growth hormone in GLU were higher than those in CON only on day 90 (p < 0.05). Serum levels of heat shock protein 70, adrenocorticotrophic hormone, corticosterone, triiodothyronine, and tetraiodothyronine in GLU were lower than those in CON on day 90 (p < 0.05). At the end of the experiment, ruminal pH, microbial crude protein, ammonia nitrogen, and isovalerate concentrations in GLU were higher than those in CON (p < 0.05). The apparent digestibility of dry matter, organic matter, and crude protein in GLU was higher than those in CON (p < 0.05). There were no differences in carcass traits and organ indices but spleen weight and spleen index tended to be higher in GLU. In conclusion, dietary glutamate supplementation improved rumen fermentation, increased nutrition digestibility and metabolism, enhanced immunity, and promoted growth performance of heat-stressed Hu sheep. This suggests that a longer period of glutamate supplementation (not less than 60 days) at a level of 3 g/head/day is beneficial to Hu sheep under heat stress.
Introduction
Hu sheep, commonly farmed in southern China, are renowned for their high fecundity and early sexual maturity (1). Heat stress decreases livestock productivity and fertility, and increases the disease susceptibility and mortality (2), making it one of the most difficult challenges in Hu sheep management in southern China. Heat stress inhibits protein synthesis and increases protein hydrolysis in animals (3). Degraded muscle protein supplies energy by providing amino acid (AA) substrates delivered to the liver in a process called gluconeogenesis (4). Heat stress decreases the plasma AA content (5) and increases the loss of endogenous intestinal proteins and AAs (6). Therefore, AA supplementation may be a beneficial approach to relieve heat stress in animals.
Glutamate is a functional AA. As the central hub of AA nitrogen exchange, it promotes the synthesis and degradation of proteins (7) and participates in cellular signal transduction, gene expression regulation, and metabolic cascades (8, 9). Glutamate enhances the intestinal and immune function of suckling and weaned piglets (10), and improves the productivity of pubertal rams and nulliparous ewes (11). However, the function of glutamate may be related to the physiological conditions and dosage of the supplement (10). A previous study has shown that glutamate is beneficial for enhancing the thermodynamic stability of protein ribonuclease A and α-lactalbumin for resisting temperature stress (12). Glutamate requirements are increased in heat-stressed broilers, therefore, supplementation of glutamate or its decarboxylated products (γ-GABA) can improve the intestinal morphology and survivability of broilers under heat stress (13, 14). Generally, AAs can be synthesized by rumen microorganisms and the supplementation of AAs to ruminants is unnecessary. However, under heat stress, the absorption and utilization of AAs are altered in ruminants, resulting in their reduced availability (15). In ruminants, glutamate may help to stimulate the rumen microbial growth in vitro, whereas deficiency of glutamate reduces the rumen microbial growth and efficiency (16, 17). Further, glutamate plays a vital role in generating α-ketoglutarate in the tricarboxylic acid cycle of rumen epithelial and duodenal mucosal cells in beef cattle (18). Glutamate may relieve heat-stress-induced intestinal epithelial cell damage in dairy cattle (19). However, little research has focused on the application effect of glutamate supplementation on ruminants, especially on sheep under heat stress.
We hypothesized that glutamate mitigates heat stress and improves the growth performance in Hu sheep under heat stress. The anti-heat stress effects of dietary glutamate supplementation on growth performance, nutrient digestibility, rumen fermentation characteristics, serum metabolites, and slaughter performance of heat-stressed Hu sheep were investigated, aiming to evaluate the application effect and mechanism of glutamate in the production of Hu sheep under heat stress.
Materials and Methods
Animals and Experimental Design
A total of forty-eight male Hu sheep with an average initial body weight of 17.74 ± 0.17 kg were randomly separated into a control group and a treatment group. The control group (CON) was fed a basal diet and the treatment group (GLU) was fed a basal diet + 3 g/head/d of L-glutamate. The L-glutamate was purchased from Shanghai Yuanye Biological Co. (Shanghai, China) and had a purity of 99%. The supplementation dosage of L-glutamate was estimated according to an in vitro pilot experiment (unpublished). The basal diet (Table 1) consisted of a complete mixed diet with a concentrate to forage ratio of 7:3 designed to meet the Chinese Feeding Standard of Sheep (NY/T816-2004) requirements for growing-sheep. Each treatment group contained twenty-four sheep divided into six replicates of four sheep.
The trial lasted for 90 days from 1 June to 29 August 2021. Animals were fed twice a day (8:00 and 17:00 h) with free access to water and feed. The feed intake of each replicate was recorded daily, and the body weight of sheep was recorded after fasting for 12 h on days 0, 30, 60, and 90. The feed efficiency was calculated using the F:G ratio based on the average daily feed intake (ADFI) and average daily gain (ADG).
Determination of Temperature and Humidity Index
Temperature and humidity were recorded by an Apresys® instrument (179-TH, Apresys International Inc., San Ramon, CA, United States), and the temperature and humidity index (THI) was calculated according to NRC (1971) (20) as follows:
where AT is air temperature (°C) and RH is relative humidity (%).
Serum Sampling and Analysis
Blood samples were collected with non-anticoagulation vacuum blood vessels before morning feeding after fasting for 12 h on days 30, 60, and 90. Serum was harvested following centrifugation at 3,000 × g for 10 min at 4°C and subsequently frozen at −80°C until analysis.
The concentrations of serum glucose, blood urea nitrogen (BUN), total protein (TP), albumin (ALB), glutamate, and globulin (GLB) were determined using corresponding commercial kits (Zhongsheng Beikong Bio-technology and Science Inc., Beijing, China) and an automatic biochemical analyzer (BS-420, Shenzhen Mindray Bio-medical Electronics Co., Shenzhen, China). The concentrations of glutamate, immunoglobulin A (IgA), immunoglobulin G (IgG), and immunoglobulin M (IgM) were measured using corresponding commercial kits (Beijing Sino-UK Institute of Biological Technology, Beijing, China) and a semi-automatic biochemical analyzer (A6, Beijing Songshang Technology Co., Beijing, China). The concentrations of growth hormone (GH), heat shock protein 70 (HSP70), adrenocorticotrophic hormone (ATCH), corticosterone (CORT), triiodothyronine (T3), and tetraiodothyronine (T4) were determined using corresponding commercial ELISA kits (Beijing Sino-UK Institute of Biological Technology, Beijing, China) and a microplate reader (DR-200BS, Wuxi Hua Wei De Lang Instrument Co., Wuxi, China).
Determination of Rumen Fermentation Characteristics
Rumen fluid pH was measured immediately after slaughter using a portable pH meter [Testo 206-pH1, Testo Instruments International (shanghai) CO., Shanghai, China]. Then samples were collected for the determination of microbial crude protein (MCP), ammonia nitrogen (NH3-N), and volatile fatty acids (VFAs). The VFA concentration was analyzed by gas chromatography (GC-2014, Shimadzu, Tokyo, Japan) equipped with a capillary column (Stabilwax, Restek, Bellefonte, PA, United States). NH3-N concentration was determined according to the method of Broderica and Kang (21). MCP concentration was analyzed according to the method of Makkar et al. (22).
Determination of Apparent Nutrient Digestibility
Feces were collected for five consecutive days at the end of the trial. In the laboratory, all feces of each replicate were evenly mixed and 10% sulfuric acid was added to fix nitrogen. Fecal samples were stored at −20°C for subsequent determination.
Dry matter (DM), crude protein (CP), ash, and ether extract (EE) of the feed samples and fecal samples were determined according to the Association of Analytical Communities (AOAC) (23) using the methods 967.03, 984.13, 924.05, and 920.39, respectively. Neutral detergent fiber (NDF) and acid detergent fiber (ADF) were determined using an Ankom A200i Fiber analyzer (ANKOM Technology Co., New York, NY, United States) according to Van Soest et al. (24). The content of hydrochloric acid insoluble ash (AIA) was determined according to Keulen and Young (25) described, and AIA was used as a digestibility marker. The formula was:
where AD represents the apparent digestibility of the nutrient content, a represents the nutrient content of feedstuffs, b represents the nutrient content of feces, c represents the AIA content of feedstuffs, and d represents the AIA content of feces.
Determination of Slaughter Performance
One sheep was randomly selected from each replicate for slaughter, after fasting for 12 h. After the removal of head, feet, tail, and internal organs, the carcass, heart, spleen, liver, lungs, and kidneys were weighed. The dressing percentage was calculated as: carcass weight (kg)/live weight (kg) before slaughter × 100%. The organ index was calculated as organ index = organ weight (g)/live weight (kg) before slaughter. Backfat thickness, eye muscle area (EMA), and grade-rule (GR) tissue depth values were measured on the left side of the carcass according to Song et al. (26). Backfat thickness is the thickness between the 12th and 13th ribs. EMA is the cross-sectional area of the 12th and 13th ribs. GR is the total tissue thickness between the 12th and 13th rib and 11 cm from the dorsal midline of the carcass, which can represent the carcass fat content.
Statistical Analysis
All data were analyzed using SPSS 17.0 software (SPSS Inc., Chicago, IL, United States). Data were analyzed using double-tailed t-tests after checking normal distribution. The data were considered significantly different if p ≤ 0.05, and a tendency was suggested if 0.05 < p < 0.10.
Results
Temperature and Humidity Index Values
The Hu sheep was suffering from heat stress throughout the whole trial period, as shown in Figure 1. The days that the Hu sheep spent at different THI thresholds were: 72 ≤ THI < 78 for 2 days, 78 ≤ THI < 90 for 48 days, and THIs ≥ 90 for 40 days.
Growth Performance
The growth performance of the Hu sheep is shown in Table 2. No differences were observed in body weight on days 30, 60, and 90 between CON and GLU (p > 0.05). No differences were observed in ADFI, ADG, and F:G ratio between GLU and CON during phase 1 (1–30 days) and phase 2 (31–60 days) (p > 0.05). However, during phase 3 (61–90 days), the ADG in GLU was higher than in CON (p < 0.05), and the ADFI and F:G ratio in GLU was lower, compared with those in CON (p < 0.05).
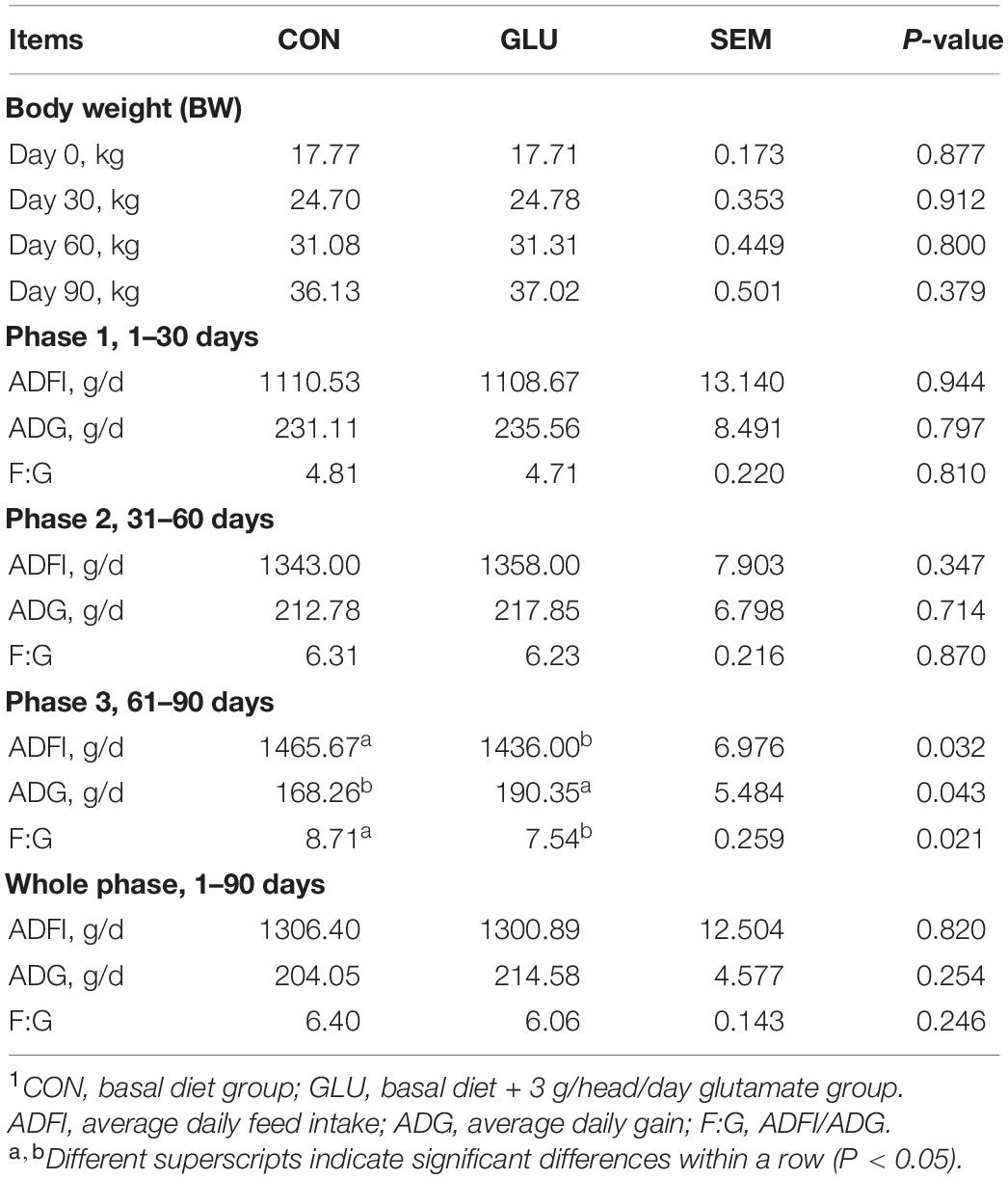
Table 2. Effects of glutamate supplementation on growth performance of heat-stressed Hu sheep (n = 6)1.
Slaughter Performance
Table 3 shows no differences in carcass weight, dressing percentage, backfat thickness, eye muscle area, GR value, organ weight, and organ index between GLU and CON (p > 0.05). However, spleen weight (p = 0.078) and spleen index (p = 0.079) in GLU tended to increase (by 54.11 and 53.71%), respectively, as compared with CON (Table 4).
Apparent Nutrient Digestibility
As shown in Table 5, the digestibility of DM, OM, and CP in GLU was higher than those in CON (p < 0.05), whereas no significant differences were observed in the digestibility of EE, NDF, ADF, and soluble carbohydrates (SCHO) between GLU and CON (p > 0.05).
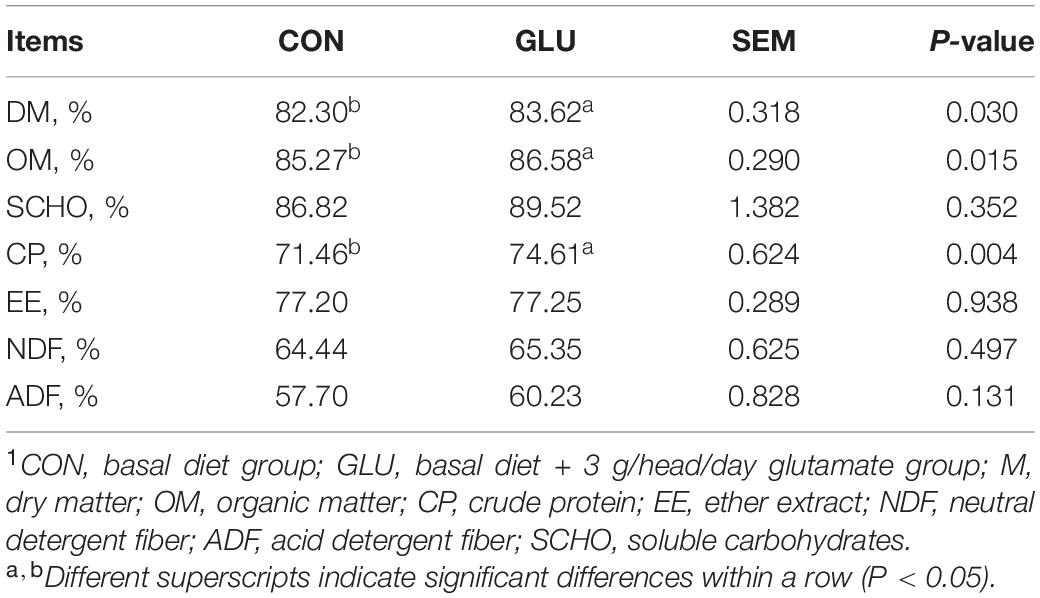
Table 5. Effects of glutamate supplementation on apparent nutrient digestibility of heat-stressed Hu sheep (n = 6)1.
Rumen Fermentation Characteristics
Rumen fermentation characteristics are shown in Table 6. Ruminal pH, MCP, NH3-N, and isovalerate concentration in GLU were all higher than those in CON (p < 0.05). The concentrations of TVFAs and VFAs in GLU, except for isovalerate, were similar to those in CON (p > 0.05).
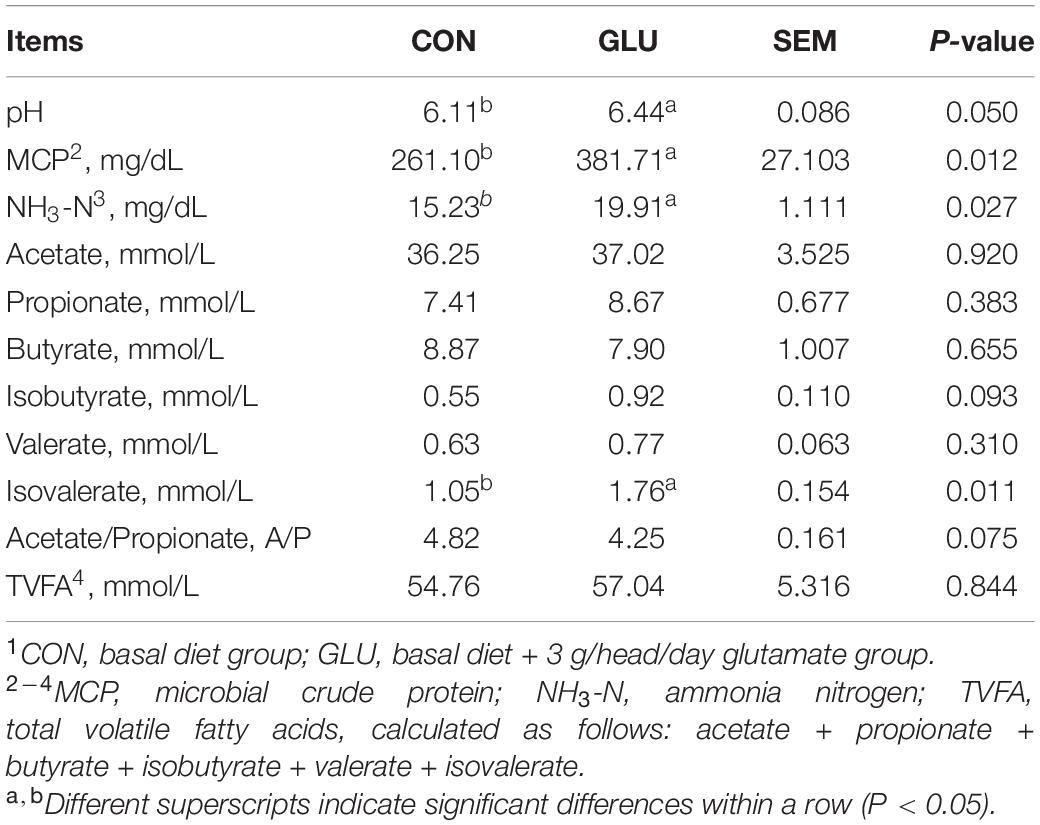
Table 6. Effects of glutamate supplementation on rumen fermentation characteristics of heat-stressed Hu sheep (n = 6)1.
Serum Biochemical Indices and Serum Hormones
The levels of serum glutamate, GLB, IgA, IgG, and IgM in GLU were higher than in CON on day 90 (p < 0.05), and the ALB/GLB in GLU was lower than that in CON on day 90 (p < 0.05) (Table 7). However, all the serum biochemical parameters-listed above were similar between GLU and CON on days 30 and 60 (p > 0.05).
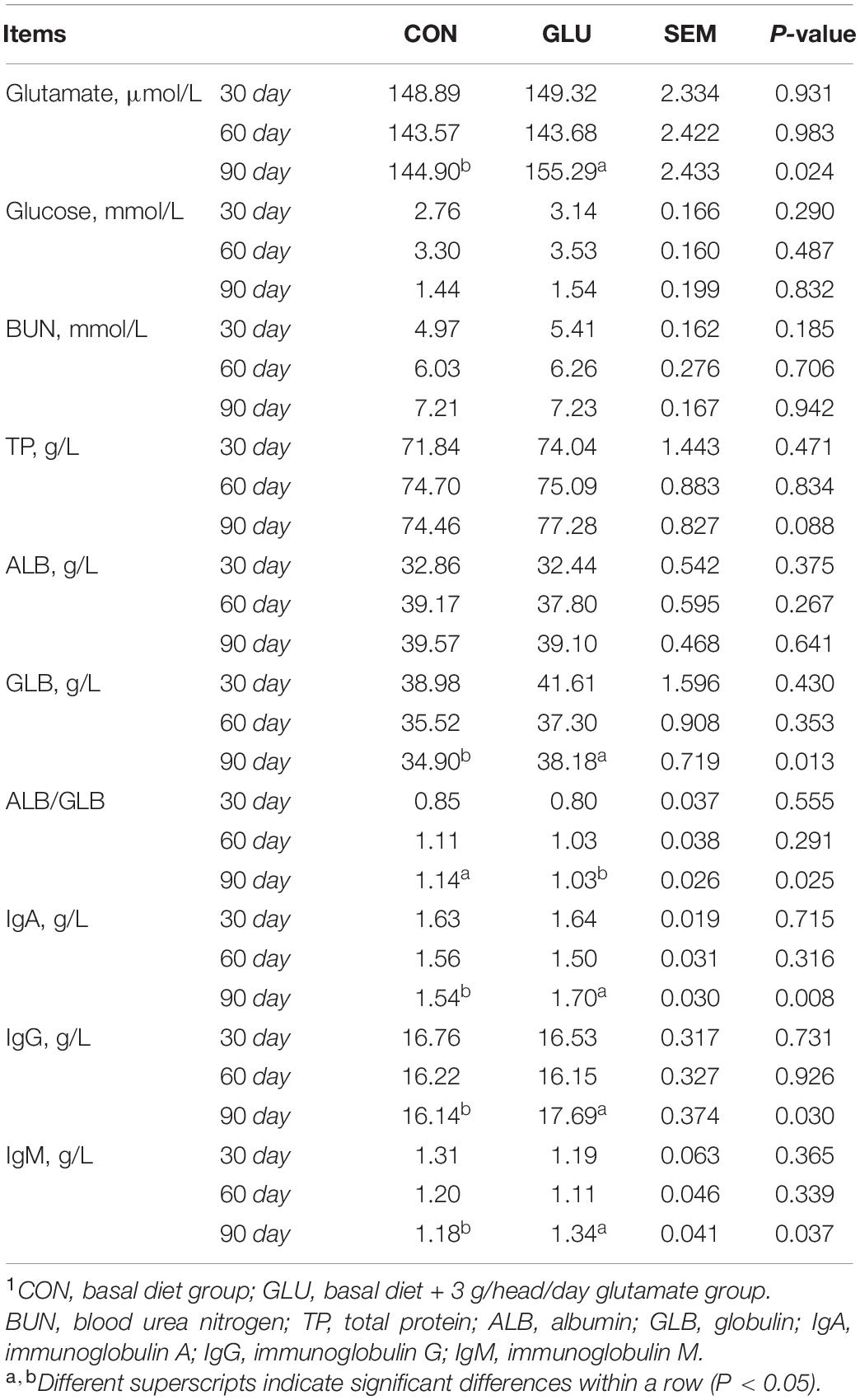
Table 7. Effects of glutamate supplementation on serum biochemistry of heat-stressed Hu sheep (n = 6)1.
The levels of serum hormones and HSP70 are shown in Table 8. The level of GH in GLU was higher than those in CON on day 90 (p = 0.001), and the levels of HSP70, ACTH, CORT, T3, and T4 in GLU were lower than those in CON on day 90 (p < 0.001). However, no differences were found between GLU and CON on days 30 and 60 (p > 0.05), expect that level of T3 was lower in GLU on day 30 (p < 0.05).
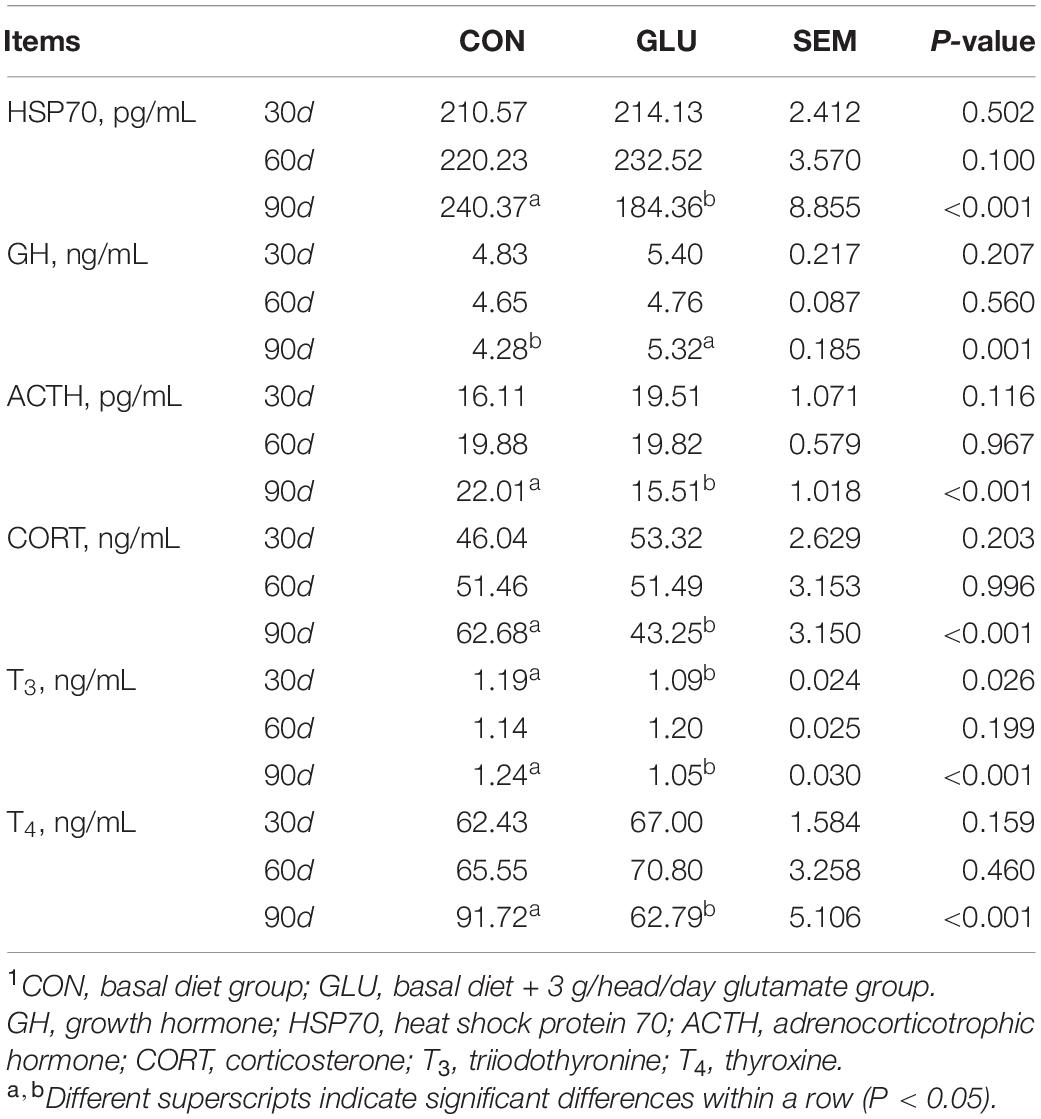
Table 8. Effects of glutamate supplementation on serum hormone and HSP70 of heat-stressed Hu sheep (n = 6)1.
Discussion
Temperature and humidity index is widely used as a practical indicator of the degree of heat stress. Thornton et al. (27) summarized that the THI threshold for heat stress in sheep was as follows: absence of heat stress (THI < 72), moderate heat stress (72 ≤ THI < 78), high heat stress (78 ≤ THI < 90), and extreme heat stress (THI ≥ 90). Li et al. (28) indicated that Hu sheep suffered from heat stress when they were exposed to THIM ≥ 75 conditions. In the present study, the Hu sheep spent the whole period of 90 days in THI ≥ 75 conditions. It suggested that the Hu sheep suffered from either high or extreme heat stress throughout the whole trial period, especially during phases 2 and 3.
Glutamate Improves Growth Performance of Heat-Stressed Hu Sheep
It is known that the heat stress leads to decreases in feed intake, nutrient digestibility, and absorption capacity, resulting in reduced animal growth performance of livestock (29). The research results of glutamate on the growth performance of animals are inconsistent. Wang et al. (30) found that the glutamate was a key neurotransmitter in the hypothalamus, which could inhibit the feed intake in animals. Other researchers indicated that it could also increase the feed intake by activating taste receptors in the digestive system (31). Hu et al. (32) and Rezaei et al. (33) reported lower ADFI after glutamate supplementation in pigs. In growing-finishing pigs, glutamate supplementation of 1% had no effect on ADG and F:G for the 60 days feeding regimen (32). By contrast, it linearly increased ADG, and decreased ADFI and F:G with monosodium glutamate addition of 0.5–4% in post-weaning pigs (33). In dairy calves, a lower dosage of glutamate (0.1 or 0.3%) has no obvious effect on ADFI, ADG, and F:G within a 70 or 56 days feeding period (34, 35). In this study, similar results of glutamate supplementation were shown in Hu sheep during phase 1 (1–30 days) and phase 2 (31–61 days). However, glutamate resulted in a marked increase of ADG, and a decrease of ADFI and F:G during phase 3 (61–90 days), which might be because of the long experimental period of glutamate supplementation. The levels of serum glutamate, serum biochemical indices and serum hormones were similar during phases 1 and 2, whereas a higher level of serum glutamate, GLB, IgA, IgG, IgM, and GH, and a lower level of HSP70, ACTH, CORT, T3, and T4 in GLU were observed during phase 3 in this study. It suggested that a longer period of glutamate supplementation increased the serum glutamate to promote the growth performance, improve the metabolism, and enhance the immunity of Hu sheep.
Glutamate Improves Rumen Fermentation
Accounting for the highest proportion of AA composition in rumen microorganisms, glutamate plays an essential role in MCP synthesis and ruminant growth (36). In this study, a higher concentration of MCP was observed owing to the dietary supplementation of glutamate, which promoted rumen microbial growth (16, 17). The NH3-N is the main source of nitrogen for rumen microbial protein synthesis, and its utilization rate reflects the balance between substrate nitrogen degradation and microbial synthesis utilization (37). In the present study, the NH3-N concentration was notably increased by adding glutamate, which was consistent with a previous report demonstrating that dietary glutamate addition increased the NH3-N concentration in dairy cows (38). In this study, higher levels of NH3-N via the supplementation with glutamate provided a nitrogen source promoting microbial growth and reproduction, which supported the higher MCP in GLU. The higher NH3-N concentration in GLU indicated a higher rumen fermentation rate; however, the NH3-N concentration in this study did not reach the 235 mg/L required for the maximal fermentation rate (39), suggesting that the specific dosage of glutamate addition did not inhibit the rumen fermentation in Hu sheep. The concentrations of NH3-N and VFAs are considered to be the main factors affecting ruminal pH. In the present study, dietary glutamate supplementation increased rumen pH, which might be attributed to the higher NH3-N production in GLU. The results were consistent with the study of Dann et al. (38) who reported that glutamate supplementation could increase the rumen pH. VFAs are absorbed by the ruminal epithelial cells and provide the main energy substances for the ruminant production. These organic acids can improve the morphology of rumen mucosa, and reduce the infection of intestinal diseases by improving the intestinal microbial structure and morphology (40, 41, 42). Isovalerate is also an energy substance for the ruminal epithelial cells, and linear increases in isovalerate are positively correlated with the promotion of rumen development (40). In this study, the higher isovalerate in GLU might be associated with an improved feed degradation rate.
Glutamate Improves Nutrient Digestibility of Heat-Stressed Hu Sheep
Oral intake of glutamate stimulates the salivation essential for mastication, swallowing, and digestion (43). A previous study demonstrated that free glutamate in digestive juices obtained from dietary digestion, absorption, or glutamine synthesis, could improve nutrient digestibility by stimulating the release of digestive enzymes and pancreatic hormones (44). Glutamate also could improve intestinal morphology and promote nutrient utilization in heat-stressed broilers (13). In the present study, the higher digestibility of DM, OM, and CP in GLU was probably due to higher serum glutamate, as mentioned above. The results of this study were similar to the research of Dann et al. (38) who reported that supplementation of 80 g glutamate per cow/day tended to increase the digestibility of DM and OM.
Glutamate Improves Immunity of Heat-Stressed Hu Sheep
Heat stress reduces animal immune function, such as decreased immune antibody secretions and spleen developmental retardation (45, 46). As the largest secondary lymphoid organ, the spleen is rich in various immune cells, such as B cells and T cells, which play vital roles in immune function and are responsible for IgA, IgG, and IgM production (47, 48). In the present study, glutamate supplementation tended to increase the spleen weight and the spleen index by 54.11 and 53.71%, respectively, probably because glutamate was beneficial to spleen development through the activation of T cell function and protection against T cell apoptosis (49). It is critical to emphasize that glutamate is an important immunomodulator (50). A previous study reported that the glutamate receptors (GluRs) were highly expressed in T cells and B cells (51), suggesting that glutamate had a positive effect on targeting them. However, if serum glutamate concentration exceeds the pathological 10–3 moles level, then T cell function and survival are inhibited (49). Serum glutamate in this study was within normal physiological concentrations. As mentioned above, higher levels of IgA, IgG, and IgM in GLU might result from the higher serum glutamate in GLU stimulating spleen development and activating T cell function. Furthermore, the decreased level of ALB/GLB in GLU might be because of the increased GLB concentration.
Glutamate Improves Serum Hormone and HSP70 Levels in Heat-Stressed Hu Sheep
The hypothalamic–pituitary–adrenal (HPA) axis and hypothalamic–pituitary–thyroid (HPT) axis can be activated by heat stress, which in turn regulate the corresponding hormones secreted by endocrine glands (52). Higher secretion of serum ACTH and CORT has been reported in animals exposed to heat stress (53). Generally, CORT is an appropriate end-product of the HPA axis, and higher ACTH leads to increase CORT (54). However, excessive CORT would accelerate the heat stress-induced protein degradation (55), which partially explained the lower body weight in CON in this experiment. The lower levels of ACTH and CORT in GLU on day 90 might result from the higher level of serum glutamate, which reduced the secretion of ACTH and CORT and the protein degradation of muscle.
The T3 and T4 (the main thyroid hormones), are key regulators of energy metabolism in the body (56). It is essential for animals to maintain the thermal balance by lowering the secretion of T3 and T4 (56). Several studies have reported that monosodium glutamate has a cumulative effect on reducing thyroid function (57, 58). Similarly, in this study, a decrease in T3 and T4 after dietary glutamate supplementation was observed on day 90, suggesting that dietary glutamate supplementation might reduce heat stress responses by lowering heat production. A higher GH level often indicates a better anti-heat stress capability in goats (59). As an excitatory AA, glutamate contributes to the stimulation of GH secretion by regulating the hypothalamus (60). Serum GH concentrations are linearly correlated with serum glutamate (60), which can explain the higher level of GH in GLU in this study.
The HSP70, the most widely distributed and expressed HSP in the body, is involved in regulating the stability of cellular proteins, increasing the tolerance of cells to stressors, and reducing cell apoptosis (61). Higher HSP70 is usually positively related to the degree of heat stress (62). A previous study reported that glutamate was conducive to enhancing the thermodynamic stability of proteins against temperature stress (12). Another study showed that glutamine normalized metabolism by reducing the serum levels of HSP70 in atrial fibrillation patients (63). In the present study, HSP70 content in GLU decreased on day 90, suggesting that glutamate supplementation might reduce the heat stress response and normalize metabolism by reducing HSP70 levels.
Conclusion
In the present study, we found that a longer period of dietary glutamate supplementation (not less than 60 days) at a dosage of 3 g/head/day was beneficial to heat-stressed Hu sheep. It might improve the growth performance of the animals by increasing the level of serum glutamate to promote growth hormone level, decrease heat stress-related hormones and HSP70 levels, and enhance immune function. These findings suggest that a strategy of glutamate supplementation has important advantages in the sheep industry under heat stress in summer.
Data Availability Statement
The original contributions presented in the study are included in the article/supplementary material, further inquiries can be directed to the corresponding authors.
Ethics Statement
The animal care and experimental procedures in this study were approved by the Animal Ethics Committee of the Jiangxi Agricultural University (JXAULL-2021-036).
Author Contributions
CL, KO, and QQ designed the experiments. CL and JZ carried out the experiments and performed the analysis. CL drafted the manuscript. YL, XZ, HL, KO, and QQ revised the manuscript. KL provided the feeding grounds for the experiment. All authors contributed to the article preparation and approved the submitted version.
Funding
This work was supported by the National Natural Science Foundation of China (32160807 and 31560648), the Jiangxi Agriculture Research System (JXARS-13), and the Science and Technology Project of Education Department of Jiangxi Province (GJJ200451 and GJJ210405).
Conflict of Interest
KL was employed by Ganzhou Lvlinwan Agriculture and Animal Husbandry Co. Ltd.
The remaining authors declare that the research was conducted in the absence of any commercial or financial relationships that could be construed as a potential conflict of interest.
Publisher’s Note
All claims expressed in this article are solely those of the authors and do not necessarily represent those of their affiliated organizations, or those of the publisher, the editors and the reviewers. Any product that may be evaluated in this article, or claim that may be made by its manufacturer, is not guaranteed or endorsed by the publisher.
Acknowledgments
We appreciate assistance from our colleagues and collaborators.
References
1. Yue GH. Reproductive characteristics of Chinese Hu sheep. Anim Reprod Sci. (1996) 44:223–30. doi: 10.1016/0378-4320(96)01562-X
2. Godde CM, Mason-D’Croz D, Mayberry DE, Thornton PK, Herrero M. Impacts of climate change on the livestock food supply chain; a review of the evidence. Glob Food Security. (2021) 28:100488. doi: 10.1016/j.gfs.2020.100488
3. Gonzalez-Esquerra R, Leeson S. Effects of acute versus chronic heat stress on broiler response to dietary protein. Poultry Sci. (2005) 84:1562–9. doi: 10.1093/ps/84.10.1562
4. Ma BB, Zhang L, Li JL, Xing T, Jiang Y, Gao F. Heat stress alters muscle protein and amino acid metabolism and accelerates liver gluconeogenesis for energy supply in broilers. Poultry Sci. (2021) 100:215–23. doi: 10.1016/j.psj.2020.09.090
5. Chowdhury VS, Han GF, Eltahan HM, Haraguchi S, Gilbert ER, Cline MA, et al. Potential role of amino acids in the adaptation of chicks and market-age broilers to heat stress. Front Vet Sci. (2021) 7:610541. doi: 10.3389/fvets.2020.610541
6. Morales A, Hernández L, Buenabad L, Avelar E, Bernal H, Baumgard LH, et al. Effect of heat stress on the endogenous intestinal loss of amino acids in growing pigs. J Anim Sci. (2016) 94:165–72. doi: 10.2527/jas.2015-9393
7. Wang LX, Yan SL, Li JZ, Li YL, Ding XQ, Yin J, et al. Rapid communication: the relationship of enterocyte proliferation with intestinal morphology and nutrient digestibility in weaning piglets. J Anim Sci. (2019) 97:353–8. doi: 10.1093/jas/sky388
8. Paudel S, Wu GY, Wang XQ. Amino acids in cell signaling: regulation and function. In: Wu G editor. Amino Acids in Nutrition and Health. (Vol. 1332), Cham: Springer (2021). p. 17–33. doi: 10.1007/978-3-030-74180-8_2
9. Wu GY, Bazer FW, Dai ZL, Li DF, Wang JJ, Wu ZL. Amino acid nutrition in animals: protein synthesis and beyond. Annu Rev Anim Biosci. (2014) 2:387–417. doi: 10.1146/annurev-animal-022513-114113
10. Watford M. Glutamine and glutamate: nonessential or essential amino acids. Anim Nutr. (2015) 1:119–22. doi: 10.1016/j.aninu.2015.08.008
11. Guadalupe CL, Cesar AMH, Rafael RM, Oscar AG, Raymundo RM, Juan VDB, et al. Effect of glutamate and/or testosterone administration on appetitive and consummatory sexual behaviors in pubertal rams and their influence on the reproductive performance of nulliparous anovulatory ewes. J Vet Behav. (2019) 30:96–102. doi: 10.1016/j.jveb.2018.12.008
12. Anumalla B, Prabhu NP. Glutamate induced thermal equilibrium intermediate and counteracting effect on chemical denaturation of proteins. J Phys Chem B. (2018) 122:1132–44. doi: 10.1021/acs.jpcb.7b10561
13. Porto ML, Givisiez PEN, Saraiva EP, Costa FGP, Moreira Filho ALB, Andrade MFS, et al. Glutamic acid improves body weight gain and intestinal morphology of broiler chickens submitted to heat stress. Braz J Poultry Sci. (2015) 17:355–62. doi: 10.1590/1516-635x1703355-362
14. Zhong G, Shao D, Wang Q, Tong HB, Shi SR. Effects of dietary supplemented of γ-amino butyric acid on growth performance, blood biochemical indices and intestinal morphology of yellow-feathered broilers exposed to a high temperature environment. Italian J Anim Sci. (2020) 19:431–8. doi: 10.1080/1828051X.2020.1747953
15. Ríus AG. Invited review: adaptations of protein and amino acid metabolism to heat stress in dairy cows and other livestock species. Appl Anim Sci. (2019) 35:39–48. doi: 10.15232/aas.2018-01805
16. Kajikawa H, Mitsumori M, Ohmomo S. Stimulatory and inhibitory effects of protein amino acids on growth rate and efficiency of mixed ruminal bacteria. J Dairy Sci. (2002) 85:2015–22. doi: 10.3168/jds.S0022-0302(02)74278-1
17. Kajikawa H, Mitsumori M, Tajima K, Kurihara M. Short communication: amino acid antagonistic to the amino acids inhibitory for growth rate of mixed ruminal bacteria. J Dairy Sci. (2005) 88:2601–3. doi: 10.3168/jds.S0022-0302(05)72936-2
18. El-Kadi SW, Baldwin RL VI, McLeod KR, Sunny NE, Bequette BJ. Glutamate is the major anaplerotic substrate in the tricarboxylic acid cycle of isolated rumen epithelial and duodenal mucosal cells from beef cattle. J Nutr. (2009) 139:869–75. doi: 10.3945/jn.108.103226
19. Chen X. Repairment of Glutamic Acid on the Damage by Heat Stress in Dairy Cattle Intestinal Epithelial Cells. Master’s thesis. Luoyang: Henan University of Science and Technology (2019).
20. NRC. A Guide to Environmental Research on Animals. Washington, DC: National Academy Press (1971).
21. Broderica GA, Kang JH. Automated simultaneous determination of ammonia and total amino acids in ruminal fluid and in vitro media. J Dairy Sci. (1980) 63:64–75. doi: 10.3168/jds.S0022-0302(80)82888-8
22. Makkar HPS, Sharma OP, Dawra RK, Negi SS. Simple determination of microbial protein in rumen liquor. J Dairy Sci. (1982) 65:2170–3.
23. AOAC. Official Methods of Analysis of the Association of Official Analytical Chemists. 16th ed. Arlington: Association of Analytical Communities (1995).
24. Van Soest PJ, Robertson JB, Lewis BA. Methods for dietary fiber, neutral detergent fiber, and nonstarch polysaccharides in relation to animal nutrition. J Dairy Sci. (1991) 74:3583–97. doi: 10.3168/jds.S0022-0302(91)78551-2
25. Van Keulen J, Young BA. Evaluation of acid-insoluble ash as a natural marker in ruminant digestibility studies. J Anim Sci. (1977) 44:282–7. doi: 10.2527/jas1977.442282x
26. Song SZ, Wu JP, Zhao SG, Casper DP, Zhang LP, He B, et al. The effect of periodic energy restriction on growth performance, serum biochemical indices, and meat quality in sheep. J Anim Sci. (2018) 96:4251–63. doi: 10.1093/jas/sky299
27. Thornton P, Nelson G, Mayberry D, Herrero M. Increases in extreme heat stress in domesticated livestock species during the twenty-first century. Glob Change Biol. (2021) 27:5762–72. doi: 10.1111/gcb.15825
28. Li YK, Kong LX, Deng M, Lian ZQ, Liu GB, Liu DW, et al. Heat stress-responsive transcriptome analysis in the liver tissue of Hu sheep. Genes. (2019) 10:395. doi: 10.3390/genes10050395
29. Alhidary IA, Shini S, Al Jassim RAM, Abudabos AM, Gaughan JB. Effects of selenium and vitamin E on performance, physiological response, and selenium balance in heat-stressed sheep. J Anim Sci. (2015) 93:576–88. doi: 10.2527/jas.2014-8419
30. Wang S, Khondowe P, Chen SF, Yu JJ, Shu G, Zhu XT. Effects of “bioactive” amino acids leucine, glutamate, arginine and tryptophan on feed intake and mRNA expression of relative neuropeptides in broiler chicks. J Anim Sci Biotechnol. (2012) 3:27. doi: 10.1186/2049-1891-3-27
31. Gabriel AS, Uneyama H. Amino acid sensing in the gastrointestinal tract. Amino Acids. (2013) 45:451–61. doi: 10.1007/s00726-012-1371-2
32. Hu CJ, Jiang QY, Zhang T, Yin YL, Li FN, Deng JP, et al. Dietary supplementation with arginine and glutamic acid modifies growth performance, carcass traits, and meat quality in growing-finishing pigs. J Anim Sci. (2017) 95:2680–9. doi: 10.2527/jas.2017.1388
33. Rezaei R, Knabe DA, Tekwe CD, Dahanayaka S, Ficken MD, Fielder SE, et al. Dietary supplementation with monosodium glutamate is safe and improves growth performance in postweaning pigs. Amino Acids. (2013) 44:911–23. doi: 10.1007/s00726-012-1420-x
34. Thais da Silva J, Manzoni T, Rocha NB, Santos G, Miqueo E, Slanzon G, et al. Evaluation of milk replacer supplemented with lysine and methionine in combination with glutamate and glutamine in dairy calves. J Appl Anim Res. (2018) 46:960–6. doi: 10.1080/09712119.2018.1436549
35. Ahangarani MA, Bach A, Bassols A, Vidal M, Valent D, Ruiz-Herrera S, et al. Short communication: performance, intestinal permeability, and metabolic profile of calves fed a milk replacer supplemented with glutamic acid. J Dairy Sci. (2020) 103:433–8. doi: 10.3168/jds.2019-17077
36. Sok M, Ouellet DR, Firkins JL, Pellerin D, Lapierre H. Amino acid composition of rumen bacteria and protozoa in cattle. J Anim Sci. (2017) 100:5241–9. doi: 10.3168/jds.2016-12447
37. Qiu QH, Wei X, Zhang L, Li YJ, Qu MR, Ouyang KH. Effect of dietary inclusion of tea residue and tea leaves on ruminal fermentation characteristics and methane production. Anim Biotechnol. (2021). doi: 10.1080/10495398.2021.1998092 [online ahead of print].
38. Dann HM, Ballard CS, Grant RJ, Cotanch KW, Carter MP, Suekawa M. Effects of glutamate on microbial efficiency and metabolism in continuous culture of ruminal contents and on performance of mid-lactation dairy cows. Anim Feed Sci Technol. (2006) 130:204–24. doi: 10.1016/j.anifeedsci.2006.01.009
39. Mehrez AZ, Ørskov ER, McDonald I. Rates of rumen fermentation in relation to ammonia concentration. Br J Nutr. (1977) 38:437–47. doi: 10.1079/BJN19770108
40. Liu Q, Wang C, Guo G, Huo WJ, Zhang YL, Pei CX, et al. Effects of isovalerate supplements on morphology and functional gene expression of rumen mucosa in pre- and post-weaning dairy calves. Animal. (2018) 12:491–500. doi: 10.1017/S175173111700194X
41. Zhang S, Shen YR, Wu S, Xiao YQ, He Q, Shi SR. The dietary combination of essential oils and organic acids reduces Salmonella enteritidis in challenged chicks. Poultry Sci. (2019) 98:6349–55. doi: 10.3382/ps/pez457
42. Hu Y, Wang LD, Shao D, Wang Q, Wu YY, Shi SR, et al. Selectived and reshaped early dominant microbial community in the cecum with similar proportions and better homogenization and species diversity due to organic acids as AGP alternatives mediate their effects on broilers growth. Front Microbiol. (2020) 10:2948. doi: 10.3389/fmicb.2019.02948
43. Uneyama H, Gabriel AS, Kawai M, Tomoe M, Torii K. Physiological role of dietary free glutamate in the food digestion. Asia Pacific J Clin Nutr. (2008) 17:372–5. doi: 10.6133/apjcn.2008.17.s1.93
44. Torii K, Uneyama H, Nakamura E. Physiological roles of dietary glutamate signaling via gut–brain axis due to efficient digestion and absorption. J Gastroenterol. (2013) 48:442–51. doi: 10.1007/s00535-013-0778-1
45. Dahl GE, Tao S, Monteiro APA. Effects of late-gestation heat stress on immunity and performance of calves. J Dairy Sci. (2016) 99:3193–8. doi: 10.3168/jds.2015-9990
46. Zhang SS, Zhen L, Feng JH, Hu CH, Peng QQ, Chang Y, et al. Effects of continuous partial heat treatment on immune organ indexes, small intestinal morphology and mucosal immunity indexes of broiler chickens. Chin J Anim Nutr. (2015) 27:3887–94.
47. Kashimura M. The human spleen as the center of the blood defense system. Int J Hematol. (2020) 112:147–58. doi: 10.1007/s12185-020-02912-y
48. Lewis SM, Williams A, Eisenbarth SC. Structure and function of the immune system in the spleen. Sci Immunol. (2019) 4:eaau6085. doi: 10.1126/sciimmunol.aau6085
49. Ganor Y, Levite M. The neurotransmitter glutamate and human t cells: glutamate receptors and glutamate-induced direct and potent effects on normal human t cells, cancerous human leukemia and lymphoma t cells, and autoimmune human t cells. J Neural Transmission. (2014) 121:983–1006. doi: 10.1007/s00702-014-1167-5
50. Pacheco R, Gallart T, Lluis C, Franco R. Role of glutamate on T-cell mediated immunity. J Neuroimmunol. (2007) 185:9–19. doi: 10.1016/j.jneuroim.2007.01.003
51. Ganor Y, Levite M. Glutamate in the immune system: glutamate receptors in immune cells, potent effects, endogenous production and involvement in disease. In: Levite M editor. Nerve-Driven Immunity. Vienna: Springer (2012). p. 121–61. doi: 10.1007/978-3-7091-0888-8_4
52. Afsal A, Sejian V, Madiajagan B, Krishnan G, Vandana GD, Bhatta R. Heat stress and livestock adaptation: neuro-endocrine regulation. Int J Vet Anim Med. (2018) 1:108. doi: 10.31021/ijvam.20181108
53. He XF, Lu Z, Ma BB, Zhang L, Li JL, Jiang Y, et al. Chronic heat stress alters hypothalamus integrity, the serum indexes and attenuates expressions of hypothalamic appetite genes in broilers. J Ther Biol. (2019) 81:110–7. doi: 10.1016/j.jtherbio.2019.02.025
54. Titto CG, Negrão JA, Canaes TDS, Titto RM, Leme-dos Santos TMDC, Henrique FL, et al. Heat stress and ACTH administration on cortisol and insulin-like growth factor 1 (IGF-1) levels in lactating Holstein cows. J Appl Anim Res. (2017) 45:1–7. doi: 10.1080/09712119.2015.1091326
55. Furukawa K, Kikusato M, Kamizono T, Toyomizu M. Time-course changes in muscle protein degradation in heat-stressed chickens: possible involvement of corticosterone and mitochondrial reactive oxygen species generation in induction of the ubiquitin-proteasome system. General Comp Endocrinol. (2016) 228:105–10. doi: 10.1016/j.ygcen.2016.02.007
56. Sejian V, Maurya VP, Naqvi SM. Adaptive capability as indicated by endocrine and biochemical responses of Malpura ewes subjected to combined stresses (thermal and nutritional) in a semi-arid tropical environment. Int J Biometeorol. (2010) 54:653–61. doi: 10.1007/s00484-010-0341-1
57. Dhindsa KS, Omran RG, Bhup R. Histological changes in the thyroid gland induced by monosodium glutamate in mice. Acta Anatom. (1981) 109:97–102. doi: 10.1159/000145371
58. Snežana C, Miloš F, Jović ZK, Nešic M, Gordana P, Mirjana R. Histopathologic changes at the hypothalamic nucleus arcuatus and thyroid level in rats treated with monosodium glutamate. Acta Facultatis Med Naissensis. (2004) 21: 89–94.
59. Pragna P, Sejian V, Bagath M, Krishnan G, Archana PR, Soren NM, et al. Comparative assessment of growth performance of three different indigenous goat breeds exposed to summer heat stress. J Anim Physiol Anim Nutr. (2018) 102:825–36. doi: 10.1111/jpn.12892
60. Barb CR, Campbell RM, Armstrong JD, Cox NM. Aspartate and glutamate modulation of growth hormone secretion in the pig: possible site of action. Domestic Anim Endocrinol. (1996) 13:81–90. doi: 10.1016/0739-7240(95)00047-X
61. Qiang S, Ma FT, Wei JY, Li HY, Ma H, Sun P. Physiological functions of heat shock proteins. Curr Protein Peptide Sci. (2020) 21:751–60. doi: 10.2174/1389203720666191111113726
62. Bharati J, Dangi SS, Chouhan VS, Mishra SR, Bharti MK, Verma V, et al. Expression dynamics of HSP70 during chronic heat stress in Tharparkar cattle. Int J Biometeorol. (2017) 61:1017–27. doi: 10.1007/s00484-016-1281-1
Keywords: glutamate, growth performance, immunity, rumen fermentation, serum metabolites, heat stress, Hu sheep
Citation: Li C, Zhang J, Li Y, Zhao X, Liang H, Li K, Qu M, Qiu Q and Ouyang K (2022) Glutamate Supplementation Improves Growth Performance, Rumen Fermentation, and Serum Metabolites in Heat-Stressed Hu Sheep. Front. Nutr. 9:851386. doi: 10.3389/fnut.2022.851386
Received: 09 January 2022; Accepted: 01 March 2022;
Published: 06 April 2022.
Edited by:
Shourong Shi, Poultry Institute (CAAS), ChinaReviewed by:
Min Wang, Institute of Subtropical Agriculture (CAS), ChinaMarcin Barszcz, Department of Animal Nutrition, The Kielanowski Institute of Animal Physiology and Nutrition (PAN), Poland
Mengzhi Wang, Yangzhou University, China
Copyright © 2022 Li, Zhang, Li, Zhao, Liang, Li, Qu, Qiu and Ouyang. This is an open-access article distributed under the terms of the Creative Commons Attribution License (CC BY). The use, distribution or reproduction in other forums is permitted, provided the original author(s) and the copyright owner(s) are credited and that the original publication in this journal is cited, in accordance with accepted academic practice. No use, distribution or reproduction is permitted which does not comply with these terms.
*Correspondence: Qinghua Qiu, cmNhdXFxaEBjYXUuZWR1LmNu; Kehui Ouyang, b3V5YW5na2VodWlAc2luYS5jb20=