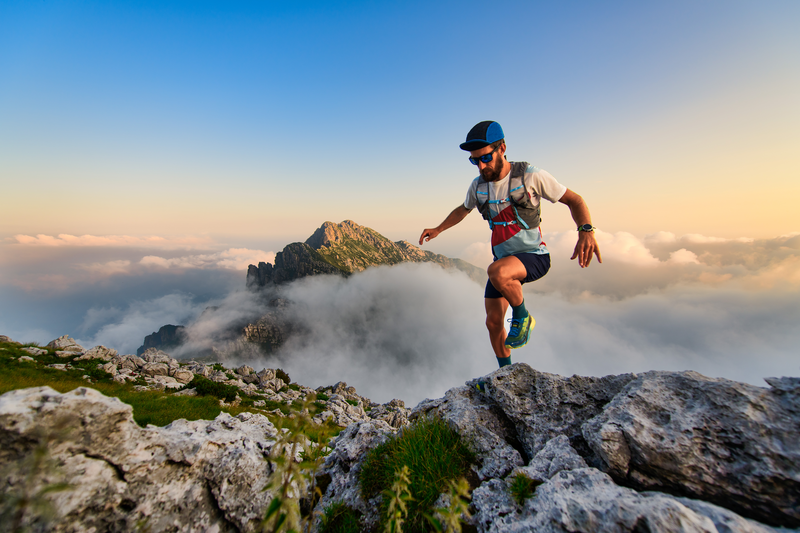
94% of researchers rate our articles as excellent or good
Learn more about the work of our research integrity team to safeguard the quality of each article we publish.
Find out more
ORIGINAL RESEARCH article
Front. Nutr. , 22 March 2022
Sec. Nutritional Immunology
Volume 9 - 2022 | https://doi.org/10.3389/fnut.2022.846974
This article is part of the Research Topic Nutrients, Gut Microbiome, and Intestinal Inflammation View all 35 articles
Based on the characteristic of low diarrhea in native Chinese breeds, we introduce the enterotype model for piglets, which is a new perspective to decipher the colonization and the transition of the gut microbiota among various pig breeds. After eliminating environmental influences represented by diet, the microbiota, mainly shaped by host genetics, is focused. Three representative enterotype clusters were identified, which were represented by Bacteroides, Streptococcus, and Lactobacillus. Chinese native breeds were distributed in enterotype 1 (E1) and E3, which collectively drove the diversification and functionality of the microbial community of various Chinese pig breeds. Next, the Lactobacillus reuteri (L. reuteri), which is the representative strain of E3, was specifically isolated in all three enterotypes. The excellent stress-resistance of L. reuteri-E3 not only highlighted the stronger disease resistance of Chinese breeds but also had a great potential to intervene in weaned piglet diseases. Enterotype classification based on host genetics is much more deterministic and predictable, clarifying the driver of the host-microbiome dynamics and constructing the picture of the micro-coevolution of human host genetics with the gut microbiome.
The diarrhea of weaned piglets is the biggest challenge to the development of intensive pig farming. Fortunately, some native Chinese breeds show a stronger disease resistance in practical production (1, 2), accompanying the development of gastrointestinal mucosal structure and discrepant gut microbiota. Gut microbial communities are involved in a series of regulations, from nutrition to disease defense (3, 4). Understanding the essential drivers of host-microbiome dynamics, and ultimately clarifying biomarkers that efficiently regulate intestinal health, could provide excellent opportunities to construct associations between stable microbiota and diseases, and improve the weaker anti-stress capacity of commercial hybrid pigs to reduce the use of antibiotics (5, 6).
An especially diverse bacteria ecosystem plays an important role in the gut, impacting a series of pathologies in diverse ways (7). Taxonomic and functional differentiation also occurs in both population and individual levels of microbiota (8, 9), and ultimately shapes some core species that are generally shared among different individuals and contribute most to gut microenvironment homeostasis. Nonetheless, questions related to the nature and origin of the intestinal flora community remain to be clarified. In order to find core clusters with stable, deterministic and predictable characteristics, enterotype was identified. Enterotype is distinct clusters characterized by the abundance of signature bacterial genera, which are characterized as “densely populated areas in a multidimensional space of community composition” (10). The occurrence of enterotype (E1, Bacteroides; E2, Prevotella; and E3, Ruminococcus) was first proposed in diverse human populations (10, 11), then, mirrored in chimpanzees (12), and have been introduced to bumblebees (3), wild mice (13), and african buffaloes (14). However, the classification of enterotypes of diverse pig breeds is still lacking.
Although the gut microbiota of commercial hybrid pigs have been extensively explored, the connection between native pig breeds and their flora are less understood. Abundant Chinese native pig breeds, along with differences in disease resistance, will be an important resource for mapping pig functional enterotypes. Characterizing the gut microbiota diversity in various Chinese pig breeds, not only contributes to the exploration of microbiota variation on a larger geographic scale, but also has the potential to identify drivers of host-microbiota dynamics to maximize agricultural productivity.
However, the enterotype paradigm has not been thoroughly explored. Its fragile plasticity is disturbed by environmental factors such as geographical location and dietary factors (14). To relatively maximize the control of environmental effects, four types of native Chinese breeds, which include Tibet pigs (Tibet), Beijing Black pigs (BeiJH), Bama pigs (BaM), and Ningxiang pigs (NingX), are removed to the same place with commercial hybrid Duroc-landrace-Yorkshire piglets (Con), while feeding the same diet. Therefore, in this cohort, a non-genetic heterogeneity is relatively well-controlled, while the genetic distinction is dominant, to achieve a deterministic and predictable enterotype division.
The detailed experimental procedures were described in the method section in Supplementary Material.
Fecal samples were collected following a standardized procedure (Supplementary Method). To relatively maximize the control of environmental effects, four types of native Chinese breeds, which include Tibet, BeiJH, BaM, and NingX, were removed from the same place with a commercial hybrid Con, while feeding with the same diet. At the same time, to ensure the stability of the microbial structure, piglets after weaning were selected in this experiment, and the age of the experimental subjects remained the same.
An appropriate amount of fresh pig feces was added to the modified Man Rogosa Sharpe Medium (MRS) liquid medium, and after 24 h of enrichment culture, the medium was streaked on the modified MRS agar plate with an inoculation loop, and the anaerobic culture was carried out at 37°C for 24–48 h. Colonies of different colors were streaked and inoculated, followed by pure culture. Microscopic observation and sequencing were carried out for identification. The strains Lactobacillus reuteri-E1 (L. reuteri-E1), L. reuteri-E2, and L. reuteri-E3 were directly isolated from the feces of pigs that belonged to enterotype 1, 2, and 3, specifically. The L. reuteri was cultured using the MRS in the micro-anaerobic incubation system (LongYue, Shanghai).
The 16S rDNA sequencing procedure was illustrated in detail in the Supplementary Method portion. And we briefly described the workflow here. The bacterial DNA was extracted by using a Stool DNA Kit (D4015-01, Omega Bio-tek, GA, America), then amplified with the V3–V4 region primers. The amplicons were purified, and then, sequenced via the Illumina MiSeq platform. The α diversity (Chao and Shannon index), β diversity (PCoA), microbial composition, differences between groups, and enterotype were analyzed after the assembly of the sequence. All raw sequencing data have been deposited in the National Center for Biotechnology Information (NCBI) Sequence Read Archive under the BioProject PRJNA793337.
In pigs, various breeds have significantly variant anti-stress capacities, accompanied by discrepant gut microbiota. However, exploring the essential elements that shaped the differentiated microbe structure of some Chinese breeds is still challenging as are have widely different environmental factors from each other. To concentrate on the impact of genetic discrepancy in pig breeds and gut microbiota, exclusion of factors such as age, geographical location, and diet should be considered. So, in this study, some Chinese breeds, such as Tibet, BeiJH, BaM, and NingX, and the commercial hybrid Con, have been controlled to feed with the same diet (Supplementary Table 1), and in the same geographic location from their mother's generation (Figure 1A). In addition, the selected piglets among five breeds were of similar age after the weaning. Considering that the flora of piglets after weaning tends to be stable, this specific period can best reflect the true gut microbial composition among the variant pig breeds.
Figure 1. Native Chinese breeds showed stronger diarrhea resistance than commercial hybrid piglets. (A) The geographic location of the original population of four Chinese native pig breeds on the map, and the schematic diagram of the project process. (B) Chinese native pig breeds exhibited a significantly reduced diarrhea rate than commercial hybrid piglets. (C) Chinese native pig breeds showed reduced water content in feces. ****P < 0.05.
Fecal samples were collected on-site following a standardized procedure (see Supplementary Method). It can be found that the piglets of commercial hybrid are prone to diarrhea with a generally high diarrhea rate at about 27% (Figure 1B), which is accompanied by increased water content in feces (Figure 1C). In contrast, Chinese breeds all showed a visibly mild to even no diarrhea symptoms, which is consistent with a lower water content (Figures 1B,C).
This suggests that the intestinal microbiota co-evaluated with host genome. Genome is still the main mediator that affects the diarrhea rate and the intestinal health of the weaned piglets, even if external environmental factors are controlled.
To profile the micro-coevolution of the intestinal microbiota among the Con and various Chinese breeds, we collected the bacteria of 40 piglets from 5 breeds using Illumina MiSeq sequencing of the V3–V4 region of bacterial 16S rRNA. After the size-filtering, quality control, and chimera removal, the total number of 3,905,171 sequences was obtained. Sequences were clustered into operational taxonomic units (OTUs) with 97% minimum identity. Additionally, the estimated sample coverage was more than 99.8% without exception, suggesting the reliable accuracy and the reproducibility of sequencing.
The β-diversity was calculated at genus level. Results in principal coordinates analysis (PCoA), presented in the histogram, confirmed a significantly separate clustering between Con and Chinese breeds, with main principal component (PC) scores: PC1 = 30.3%, PC2 = 13%, demonstrating a different clustering (Figure 2A), which is consistent with Principal Component Analysis (PCA) analysis (Figure 2B) and with weighted unifrac-based PCoA (Supplementary Figure 1A). Meanwhile, the Tibet microbiota was similar to BeiJH microbiota, while the microbiota in BaM was similar to NingX. However, these two groups revealed a distinct clustering pattern, and between them, the Con group was more distributed (Figure 2A).
Figure 2. Species diversity analysis of microbial community. (A) Scatterplot from principal coordinates analysis (PCoA) in bacterial communities of fecal microbiota on genus level based on the bray_curtis distance. (B) 3D scatterplot from PCA in bacterial communities of fecal microbiota on genus level. The bacterial α diversity of fecal microbiota of piglets based on (C) Chao1 and (D) Shannon indexes. Data were shown as mean ± SD (n ≤ 8). Student's T-test was conducted. *P < 0.05; **P < 0.01.
Chao1 and Shannon, two indicators describing the α diversity, were observed at the genus level. Tibet and BeiJH groups showed an obviously risen Chao1 and Shannon index compared to Con, while the α diversity of BaM and NingX significantly decreased compared with BeiJH, respectively (Figures 2C,D).
To evaluate the similarity in groups and to examine differences between treatments, a similarity analysis (ANOSIM/Adonis) was performed. Notably, differences between groups were greater than intragroup differences with the P-value of 0.001 (Supplementary Figure 1B). In addition, results of Partial Least Squares Discriminant Analysis (PLS-DA) also highlighted the similar microbiome composition in ethnically similar individuals (Supplementary Figure 1C), which collectively indicated the differential microbiota between various breeds.
Sequences were clustered into 3275 OTUs, which were then binned into 393 genera, based on BLAST searches against the SILVA SSU database. Each breed had its own unique OTU category and has 814 OTUs in common (Figure 3A). A community bar-plot analysis at the phylum level exhibited a relative alteration of the microbial community. Firmicutes and Bacteroidetes were dominant phyla of feces. The Tibet and BeiJH possessed similar phylum structures with a decreased ratio of Firmicutes to Bacteroidetes; meanwhile, they also had a considerable proportion of Verrucomicrobia. Conversely, the consistent structure presented in BaM and NingX, in which, the Firmicutes proportion increased significantly, while the Bacteroidetes decreased significantly compared to the other three groups (Figure 3B). Relative abundances of microbes on family (Figure 3C) and genus (Figure 3D) levels were also presented in each breed. The internal microbiome taxonomic compositions between Tibet and BeiJH were relatively uniform, while the microbiome composition between BaM and NingX was also similar. However, their respective proportion and relative priority were all varied with Con, indicating that the differentiation between the microbiota and the host genetic admixture is consistent.
Figure 3. Differential composition of microbial community among various pig breeds. (A) Venn diagram analysis of five pig breeds. (B) Histogram of species abundance at the phylum level. (C) The heat map of bacteria composition at the family level. (D) Histogram of the microbial community at the genus level.
In the comparison of the fecal microbiome by 16S rRNA gene amplicon sequencing analysis at genus level, we found that the fecal microbiota of Con was dominated by Streptococcus, unspecified_Muribaculaceae, Prevotellaceae_NK3B31_group, and Terrisporobacter (Figure 3D). Besides, Lachnospiraceae_AC2044_group, Prevotella_9, and Clostridium_sensu_stricto_1 were also identified as the unique microbiota in Con (Figures 4A,B). The proportion of these bacteria was all significantly increased in the Con group (P < 0.05; Figure 4C).
Figure 4. Various breeds had significantly variant intestinal micro-environment. Histogram (A) and cladogram (B) of Linear Discriminant Analysis (LDA) value distribution by setting score 4 at genus level in LEfSe analysis. (C) Kruskal–Wallis H-test bar plot of bacteria that were abundant in Con group. (D) Kruskal–Wallis H-test bar plot of bacteria that significantly increased in both Tibet and BeiJH groups. (E) Bacteria that gained increased proportion in BaM and NingX groups. *P < 0.05; **P < 0.01; ***P < 0.001.
Tibet and BeiJH were characterized by a similar microbiota profile, in which Bacteroides_p_251_o5 possessed a considerable proportion (Figure 3D). According to LefSe analysis, BeiJH was also dominated by Prevotellaceae_UCG_003, while Tibet was also more abundant in Rikenellaceae_RC9_gut_group, Ruminococcaceae_UCG_005, Alloprevotella, and Akkermansia (Figures 4A,B). The Kruskal–Wallis H-test consistently showed higher levels of these mentioned bacteria (P < 0.05) in contrast with the other three pig breeds (Con, BaM, and NingX) (Figure 4D).
Relative to Con, indigenous pig breeds no matter in the south (represented by BaM and NingX) or living in the north (such as Tibet and BeiJH), both had a considerable proportion of Lactobacillus (Figure 3D). Lactobacillus, considered as a traditional prebiotic, was not only identified as the shared unique bacteria between BaM and NingX (Figures 4A,B) but has also displayed a significant increase compared to other three groups (Figure 4E). Other bacteria, associated with the generation of short-chain fatty acids (SCFAs), also had a higher ratio, including Ruminococcus, Blautia, Faecalibacterium, Agathobacter, and Roseburia (Figure 4E).
Microbial community structure seemed to reveal more subtle changes that were shaped by the host genetics (Figure 5A). BaM and NingX were hosts to the related Lactobacillus, while Tibet and BeiJH possessed specific clusters of Bacteroides_p_251_o5, and Con was dominated by Streptococcus. Accordingly, the enterotype clusters were subsequently classified based on broader-scale patterns across the host genetics and the geographical regions.
Figure 5. Compositional analysis of enterotype-like clusters. (A) Hierarchical clustering tree on OTU level. (B) The classification of enterotype among five pig breeds. (C) Ternary analysis for determinants in three enterotypes. Dominant bacteria in (D) Enterotype 1, (E) Enterotype 2, and (F) Enterotype 3. The abundance of Bacteroidales-p-251-o5 (G), Streptococcus (H), and Lactobacillus (I), in three enterotypes, specifically. (J–L) Enterotype characteristic bacteria were located in the center of each enterotype network interaction map. **P < 0.01; ***P < 0.001.
Microbiota communities were stratified into three robust enterotypes according to the previously described clustering method by Arumugam et al. (10) (Figure 5B, Supplementary Figure 2A). The driving genera were identified, which obtained the Bacteroides enterotype (E1), Streptococcus enterotype (E2), and Lactobacillus enterotype (E3) (Figures 5B,C). Among microbiome belonging to E2, Prevotellaceae still dominates in second place (Figure 5E). Meanwhie, in E3, Ruminococcus was also dominated (Figure 5F). Notably, Prevotellaceae and Ruminococcus are both enterobacteriaceae that identified in human enterotype previously (11). Consistent with the classification of human enterotypes, the enterotype of piglets had also obtained three significant clusters based on their common and their characteristic bacteria (Supplementary Figure 2B). Moreover, the enterotype-associated host genetics was also reflected in the differentiation of microbiome genetics (Supplementary Figure 2C), collectively illustrating the micro-coevolution of host and microbes.
It can be found that the gradient distribution of the genera Bacteroides-p251-o5 (Figures 5D,G,J), Streptococcus (Figures 5E,H), and Lactobacillus (Figures 5F,I) was highly correlated with enterotype clustering. Additionally, the best driver of each enterotype was identified by the network of the co-occurring genera, which centered around its specific driving genus. It can be consistently found that since E1 was driven by Bacteroides (Figure 5J), Streptococcus was the most dominant contributor causing differences between groups and the similarity within E2 (Figure 5K), while the best driver for E3 was Lactobacillus (Figure 5L).
Distinct enterotypes corresponded to different functional annotations of gut microbiota. Among them, the SCFA and lactic acid-production-related enterotype (E3) was expected to be healthier. L. reuteri was the characteristic bacteria of E3, which was significantly different from the other two enterotypes (Figure 6A). Based on enrichment culture and screening of acids production, we have successfully isolated and identified three desired strains of L. reuteri, which were isolated from E1, E2, and E3, and, were named was L. reuteri-E1, E2, and E3, accordingly (Figure 6C, Supplementary Figure 3). The isolated strain is white, opaque, with neat edges, and smooth and round convex surfaces. It can be found that the colony of L. reuteri-E3 is thicker, while the L. reuteri-E2's is the smallest and thinnest (Figure 6B). Scanning electron microscopy (SEM) results also show that filamentous protrusions are formed on the surface of L. reuteri-E3, which is related to the secretion of polysaccharides (Figure 6D). The L. reuteri-E3 has a superior growth rate than the L. reuteri-E1 and E2, in which it started the logarithmic growth phase at 4 h and the plateau stage at 8 h (Figure 6E). The L. reuteri-E3 has also a desirable capacity of producing acid, which pH value dropped to 4.39 after 12 h of cultivation (Figure 6F), concurrent with the inhibition against Escherichia coli (Figure 6G), Salmonella (Figure 6H), and Staphylococcus aureus (Figure 6I). In addition, the L. Reuteri-E3 has the strongest ability to withstand low pH values, which is prominently manifested in the integrity of the bacterial membrane (Figure 6M) and the maintenance of a higher survival rate (Figure 6J, Supplementary Figure 4). However, although L. reuteri-E2 shows better performance than L. reuteri-E1 (Figure 6J), there are still pores in the bacterial membrane under an extremely acidic condition (Figures 6K,L).
Figure 6. Screening and function exploration of E3 representative strain Lactobacillus reuteri (L. reuteri) among three enterotypes. (A) The abundance of L. reuteri in three Enterotypes. (B) Photograph of the plate, (C) Gram stain, and (D) SEM pictures of L. reuteri isolated from the three enterotypes. The magnification is 10.0k. (E) Growth curve analysis of three strains of L. reuteri. (F) Comparison of the acid production capacity of three strains of L. reuteri. The inhibition against (G) Escherichia coli, (H) Salmonella, and (I) Staphylococcus aureus. (J) Comparison of the survival rates of the three types of L. reuteri under the condition of pH = 2. Scanning electron microscope (SEM) picture of the destruction of the outer membrane of (K) L. reuteri-E1, (L) L. reuteri-E1, and (M) L. reuteri-E3. *P < 0.05; **P < 0.01; ***P < 0.001; ****P < 0.0001.
Based on the characteristic of low diarrhea in the native Chinese breeds, we introduced an enterotype model for piglets, which is a new perspective to decipher the colonization and the transition of the gut microbiota among various pig breeds (15). After removing environmental effects on the gut microbiome, including geographical location and diet, the function of different genetic backgrounds of pig breeds on enterotype clusters was mainly focused, since the microbiota shaped by host genetics is much more deterministic and predictable. Three representative enterotype clusters were identified, which were represented by Bacteroides, Streptococcus, and Lactobacillus. Native Chinese breeds were distributed in E1 and E3, which collectively drove the diversification and functionality of the microbial community of various Chinese pig breeds. Furthermore, the L. reuteri, which is the representative strain of E3, was specifically isolated among three enterotypes. The excellent stress-resistance of L. reuteri-E3 not only highlights the stronger disease resistance of Chinese breeds but also constructs the picture of the micro-coevolution of the human host genetics with the gut microbiome.
China possesses abundant native pig resources, some of which always show stronger disease resistance in practical production, such as the Tibet pigs, Bama pigs, and so on. Various breeds have significantly variant anti-stress capacities and accompany a discrepant gut microbiota (1, 2). Gut microbiota contributes to the disease resistance, which is partially regulated by their genetic background.
As one of the Chinese native breeds, Tibet pigs are strong in anti-stress capacity, characterized by a lower diarrhea rate (16). The BeiJH pigs are derived from the local North China pigs in Beijing, with a characteristic tolerance to rough-feeding and anti-stress. Bama pigs serve as ideal models for biomedical research, which are exhibited by a high intramuscular fat content (17). In addition, NingX pigs, a well-known Chinese indigenous fatty-type breed, have gained more diverse bacterial communities (18). Although the performance, appearance, and original geographic location of these breeds are variant, the microbiota does contribute to shaping their anti-stress ability compared to commercial hybrid piglets.
It is well-acknowledged that diet and geography can alter gut microbial composition and metabolism (19). To eliminate the impact of environmental factors (geographical location and diet) that are likely to cause microorganism turbulence as much as possible, four types of native Chinese breeds are removed from the same place with commercial hybrid Duroc-landrace-Yorkshire piglets, while feeding with the same diet. Here, the composition of the microbiome differed in the feces among the five breeds. Tibet and BeiJH pigs possess a similar microbial structure; the microbial evolution of BaM and NingX is closer. However, they are all very different from commercial hybrid pigs.
Following the designation of enterotypes in humans (10) and chimps (20), the driver bacteria of E1 in our study is Bacteroides, which is coincident in humans and chimps. Contributors to “E2 and E3” in piglets follow a similar pattern with humans, however, their arrangement within the enterotype has changed. Specifically, the order of Prevotella (a significant contributor to human E2) drops after the Streptococcus in E2 in pigs, resulting from an overall reduction of Prevotella abundance in pigs than in humans (13). In addition, the Ruminococcus-dominated enterotype in humans is replaced by Lactobacillus, which is the major contributing genus to E3 in pigs. Interestingly, major contributors in human enterotypes are all present in the enterotype core clusters in pigs, suggesting the homologic similarity between pigs and humans. However, Streptococcus and Lactobacillus in pigs are both absent in the human enterotype (21), suggesting that pig communities may lack some vital biological functions.
The Bacteroides enterotype is dominant in Tibet and BeiJH groups, suggesting a stronger degradation ability for carbohydrates than for E2 and E3 (22). The close relation between Bacteroides/Prevotella indicates that the preferred utilization of fiber or protein has been widely shown (11). Other remarkable genus in E1 also include Rikenellaceae_RC9_gut_groups, Lachnospiraceae, Ruminococcaceae UCG-005, and Anaerovorax (17), which are known for the ability to ferment dietary polysaccharides or plant fibers. Akkermansia also showed a remarkable predominance in E1. Akkermansia is an intestinal symbiont colonizing in the mucosal layer, which is considered to be a promising candidate as a probiotic. Akkermansia is known to have an important value in improving the host's metabolic functions and immune responses, also improving host defense in mild inflammatory conditions, and increasing mucus production by promoting the differentiation of secretory intestinal epithelial cells lineages (23). Moreover, members of Lachnospiraceae, Rikenellaceae, and Bacteroidaceae families are also identified to compete with pathogens for mucin-derived sugars and, therefore, serve as ecological gatekeepers in healthy guts (24). The structure of E1 is consistent with the original harsh natural conditions for Tibet and BeiJH to survive, and their instinct to digest a high-fiber diet. The main representative strain of E2 is Streptococcus, while the bacteria, such as Succinivibrio, Terrisporobacter, and Muribaculaceae, were also significantly increased in E2. The Streptococcus includes groups A and B: group A Streptococcus (GAS) species are responsible for a wide variety of human diseases that range from noninvasive, mild infections, to life-threatening, and invasive conditions (25); and Group B Streptococcus (GBS) remains a leading cause of serious neonatal infection (26). Moreover, the functional coverage of Terrisporobacter is controversial. Sometimes, it is considered as pathogenic bacteria (27) that is linked to a polymicrobial infection in clinical cases (28). However, the genus Terrisporobacter is also closely related to SCFA contents and oxidative indicators (29) and plays a key role in degrading organic matter (28). The Succinivibrio was also enriched in E2, which is regarded as a double-edged sword, not only involved in the accumulation of anti-inflammatory cytokines and viral inhibitors but also induced the inflammatory responses in the gut (30). Besides being converted into propionate by the cross-feeding effect among the microbiota, Succinivibrio is also metabolized to directly succinate, which could activate immune cells and aggravate inflammation (31). Muribaculaceae was also obviously increased in E2, which is functionally distinct from the neighboring families and is versatile concerning the complex carbohydrate degradation (32), especially for mucin degradation (33). Thus, it is considered a major mucin monosaccharide forager (24). Additionally, the abundant microbes that generate postbiotics are gathered in the E3, including lactic acid-producing bacteria, Lactobacillus, butyric acid-generating bacteria, Blautia (34), Roseburia (35), and Holdemanella (36).
We further isolated the dominant strain L. reuteri from E3 and separated its equivalents, L. reuteri-E1 and L. reuteri-E2, to explore their different structure and function associated with host genetics. We found that L. reuteri from E3 has stronger resistance to stress, and the growth rate, acid production capacity, and antibacterial properties of L. reuteri-E3 are also more advantageous. Thus, unitizing the micro-coevolution of intestinal microbiome and native Chinese breeds is warranted to improve anti-diarrhea treatment strategies.
Our study provides several points of enterotype-like community clusters in pigs. However, larger populations, more breeds, and comprehensive physiological indices are still required to divide a more deterministic and predictable enterotype in pigs. In addition, the effect of covariates and a wider survey of nutritional sources on the gut microbiota is warranted to accurately assess the composition of enterotypes and possible transformation factors.
In conclusion, we put forward three functionally enterotype-like clusters present in Chinese native breeds and commercial hybrid pigs. Remarkably, enterotypes in pigs display some common characteristics with humans and chimpanzees as reported, indicating the existence of ancient shared traits in mammalian hosts. However, some unique contributors in pig enterotype emphasize the micro-coevolution of intestinal microbiota and pig genetics. Although controlling environmental effects relatively well, the host genetic still essentially contributes to enterotype status, which is essential for the deterministic and predictable regulation of microbiota. Moreover, some remarkable genus, as well as their generating post-biotics within specific enterotypes, may also have great potential as biomarkers for intervening the weaned piglet diseases, thus, relying on diversified Chinese native pig resources with strong stress resistance.
The datasets supporting the conclusions of this article are available in the NCBI Sequence Read Archive (SRA) repository under accession number PRJNA793337.
The animal study was reviewed and approved by the Animal Care and Use Ethics Committee of China Agricultural University.
XM conceived and designed the study, gave a critical reading and editing, and resourced the project. NM conducted most of the in vitro bacteria experiments, performed the statistical analysis for all the data, and wrote the original draft. JC contributed to collecting fecal samples. YS, ZQ, and CL performed Gram staining, inhibition zone determination, and acid resistance-related experiments. All authors read and approved the final manuscript.
This work was supported by the National Natural Science Foundation of China (31930106 and 31829004), the National Ten-thousand Talents Program of China (23070201), the Henan Province Public Benefit Research Foundation (201300111200-05), the 2115 Talent Development Program of China Agricultural University (1041-00109019), and the 111 Project (B16044).
The authors declare that the research was conducted in the absence of any commercial or financial relationships that could be construed as a potential conflict of interest.
All claims expressed in this article are solely those of the authors and do not necessarily represent those of their affiliated organizations, or those of the publisher, the editors and the reviewers. Any product that may be evaluated in this article, or claim that may be made by its manufacturer, is not guaranteed or endorsed by the publisher.
The Supplementary Material for this article can be found online at: https://www.frontiersin.org/articles/10.3389/fnut.2022.846974/full#supplementary-material
1. Yan SJ, Zhu C, Yu T, Huang WJ, Huang JF, Kong Q, et al. Studying the Differences of bacterial metabolome and microbiome in the colon between landrace and meihua piglets. Front Microbiol. (2017) 8:1812. doi: 10.3389/fmicb.2017.01812
2. Hu J, Ma LB, Nie YF, Chen JW, Zheng WY, Wang XK, et al. A microbiota-derived bacteriocin targets the host to confer diarrhea resistance in early-weaned piglets. Cell Host Microbe. (2018) 24:817–32.e8. doi: 10.1016/j.chom.2018.11.006
3. Li JL, Powell JE, Guo J, Evans JD, Wu J, Williams P, et al. Two gut community enterotypes recur in diverse bumblebee species. Curr Biol. (2015) 25:R652–3. doi: 10.1016/j.cub.2015.06.031
4. Ma N, Ma X. Dietary amino acids and the gut-microbiome-immune axis: physiological metabolism and therapeutic prospects. Compr Rev Food Sci Food Saf. (2019) 18:221–42. doi: 10.1111/1541-4337.12401
5. Ma N, He T, Johnston LJ, Ma X. Host–microbiome interactions: the aryl hydrocarbon receptor as a critical node in tryptophan metabolites to brain signaling. Gut Microbes. (2020) 11:1203–19. doi: 10.1080/19490976.2020.1758008
6. Ma N, Zhang J, Reiter RJ, Ma X. Melatonin mediates mucosal immune cells, microbial metabolism, and rhythm crosstalk: a therapeutic target to reduce intestinal inflammation. Med Res Rev. (2020) 40:606–32. doi: 10.1002/med.21628
7. Ma N, Guo P, Zhang J, He T, Kim SW, Zhang G, et al. Nutrients mediate intestinal bacteria–mucosal immune crosstalk. Front Immunol. (2018) 9:5. doi: 10.3389/fimmu.2018.00005
8. Lachance J, Vernot B, Elbers CC, Ferwerda B, Froment A, Bodo JM, et al. Evolutionary history and adaptation from high-coverage whole-genome sequences of diverse african hunter-gatherers. Cell. (2012) 150:457–69. doi: 10.1016/j.cell.2012.07.009
9. Tett A, Huang KD, Asnicar F, Fehlner-Peach H, Pasolli E, Karcher N, et al. The prevotella copri complex comprises four distinct clades underrepresented in westernized populations. Cell Host Microbe. (2019) 26:666–79.e7. doi: 10.1016/j.chom.2019.08.018
10. Arumugam M, Raes J, Pelletier E, Le Paslier D, Yamada T, Mende DR, et al. Enterotypes of the human gut microbiome. Nature. (2011) 473:174–80. doi: 10.1038/nature09944
11. Costea PI, Hildebrand F, Manimozhiyan A, Backhed F, Blaser MJ, Bushman FD, et al. Enterotypes in the landscape of gut microbial community composition. Nat Microbiol. (2018) 3:388. doi: 10.1038/s41564-018-0114-x
12. Moeller AH, Peeters M, Ndjango JB, Li YY, Hahn BH, Ochman H. Sympatric chimpanzees and gorillas harbor convergent gut microbial communities. Genome Res. (2013) 23:1715–20. doi: 10.1101/gr.154773.113
13. Wang J, Linnenbrink M, Kunzel S, Fernandes R, Nadeau MJ, Rosenstiel P, et al. Dietary history contributes to enterotype-like clustering and functional metagenomic content in the intestinal microbiome of wild mice. Proc Natl Acad Sci USA. (2014) 111:E2703–10. doi: 10.1073/pnas.1402342111
14. Couch CE, Stagaman K, Spaan RS, Combrink HJ, Sharpton TJ, Beechler BR, et al. Diet and gut microbiome enterotype are associated at the population level in African buffalo. Nat Commun. (2021) 12:2267. doi: 10.1038/s41467-021-22510-8
15. Xiao LW, Wang JF, Zheng JY, Li XQ, Zhao FQ. Deterministic transition of enterotypes shapes the infant gut microbiome at an early age. Genome Biol. (2021) 22:243. doi: 10.1186/s13059-021-02463-3
16. Yang WP, Xin HY, Cao FJ, Hou JX, Ma L, Bao LJ, et al. The significance of the diversity and composition of the cecal microbiota of the Tibetan swine. Ann Microbiol. (2018) 68:185–94. doi: 10.1007/s13213-018-1329-z
17. Huan Y, Shen YJ, Zou H, Jiang QY. Analysis of long non-coding RNAs in skeletal muscle of Bama Xiang pigs in response to heat stress. Trop Anim Health Prod. (2021) 53:259. doi: 10.1007/s11250-021-02701-7
18. Lei LF, Wang ZB, Li JZ, Yang HS, Yin YL, Tan B, et al. Comparative microbial profiles of colonic digesta between ningxiang pig and large white pig. Animals. (2021) 11:1862. doi: 10.3390/ani11071862
19. Lu J, Zhang L, Zhai QX, Zhao JX, Zhang H, Lee YK, et al. Chinese gut microbiota and its associations with staple food type, ethnicity, and urbanization. NPJ Biofilms Microbiomes. (2021) 7:71. doi: 10.1038/s41522-021-00245-0
20. Moeller AH, Degnan PH, Pusey AE, Wilson ML, Hahn BH, Ochman H. Chimpanzees and humans harbour compositionally similar gut enterotypes. Nat Commun. (2012) 3:1179. doi: 10.1038/ncomms2159
21. Hildebrand F, Nguyen TLA, Brinkman B, Yunta RG, Cauwe B, Vandenabeele P, et al. Inflammation-associated enterotypes, host genotype, cage and inter-individual effects drive gut microbiota variation in common laboratory mice. Genome Biol. (2013) 14:R4. doi: 10.1186/gb-2013-14-1-r4
22. Cheng MY, Ge XL, Zhong CF, Fu RQ, Ning K, Xu SH. Micro-coevolution of host genetics with gut microbiome in three Chinese ethnic groups. J Genet Genomics. (2021) 48:972–83. doi: 10.1016/j.jgg.2021.09.002
23. Kim S, Shin YC, Kim TY, Kim Y, Lee YS, Lee SH, et al. Mucin degrader akkermansia muciniphila accelerates intestinal stem cell-mediated epithelial development. Gut Microbes. (2021) 13:1892441. doi: 10.1080/19490976.2021.1892441
24. Pereira FC, Wasmund K, Cobankovic I, Jehmlich N, Herbold CW, Lee KS, et al. Rational design of a microbial consortium of mucosal sugar utilizers reduces clostridiodes difficile colonization. Nat Commun. (2020) 11:5104. doi: 10.1038/s41467-020-18928-1
25. Cohen-Poradosu R, Kasper DL. Group A streptococcus epidemiology and vaccine implications. Clin Infect Dis. (2007) 45:863–5. doi: 10.1086/521263
26. Fultz-Butts K, Gorwitz RJ, Schuchat A, Schrag S. (2002). Prevention of perinatal group B Streptococcal disease. Atlanta: Revised Guidelines From CDC.
27. Tang S, Zhang S, Zhong R, Su D, Xia B, Liu L, et al. Time-course alterations of gut microbiota and short-chain fatty acids after short-term lincomycin exposure in young swine. Appl Microbiol Biotechnol. (2021) 105:8441–56. doi: 10.1007/s00253-021-11627-x
28. Wang Y, Jin T, Zhang N, Li J, Wang Y, Kulyar MF-e-A, et al. Effect of stocking density and age on physiological performance and dynamic gut bacterial and fungal communities in Langya hens. Microb Cell Fact. (2021) 20:218. doi: 10.1186/s12934-021-01707-y
29. Li H, Shang Z, Liu X, Qiao Y, Wang K, Qiao J. Clostridium butyricum alleviates enterotoxigenic escherichia coli K88-induced oxidative damage through regulating the p62-Keap1-Nrf2 signaling pathway and remodeling the cecal microbial community. Front Immunol. (2021) 12:771826. doi: 10.3389/fimmu.2021.771826
30. Serrano-Villar S, Rojo D, Martínez-Martínez M, Deusch S, Vázquez-Castellanos JF, Bargiela R, et al. Gut bacteria metabolism impacts immune recovery in HIV-infected individuals. EBioMedicine. (2016) 8:203–16. doi: 10.1016/j.ebiom.2016.04.033
31. Connors J, Dawe N, Van Limbergen J. The role of succinate in the regulation of intestinal inflammation. Nutrients. (2019) 11:25doi: 10.3390/nu11010025
32. Lagkouvardos I, Lesker TR, Hitch TCA, Gálvez EJC, Smit N, Neuhaus K, et al. Sequence and cultivation study of muribaculaceae reveals novel species, host preference, and functional potential of this yet undescribed family. Microbiome. (2019) 7:28. doi: 10.1186/s40168-019-0637-2
33. Lee KS, Palatinszky M, Pereira FC, Nguyen J, Fernandez VI, Mueller AJ, et al. An automated Raman-based platform for the sorting of live cells by functional properties. Nat Microbiol. (2019) 4:1035–48. doi: 10.1038/s41564-019-0394-9
34. Liu XM, Mao BY, Gu JY, Wu JY, Cui SM, Wang G, et al. Blautia-a new functional genus with potential probiotic properties? Gut Microbes. (2021) 13:1–21. doi: 10.1080/19490976.2021.1875796
35. La Rosa SL, Leth ML, Michalak L, Hansen ME, Pudlo NA, Glowacki R, et al. The human gut firmicute roseburia intestinalis is a primary degrader of dietary beta-mannans. Nat Commun. (2019) 10:905. doi: 10.1038/s41467-019-08812-y
Keywords: enterotype, pig, diet, genetic, microbe, micro-coevolution
Citation: Ma N, Sun Y, Chen J, Qi Z, Liu C and Ma X (2022) Micro-Coevolution of Genetics Rather Than Diet With Enterotype in Pigs. Front. Nutr. 9:846974. doi: 10.3389/fnut.2022.846974
Received: 31 December 2021; Accepted: 26 January 2022;
Published: 22 March 2022.
Edited by:
Hongkui Wei, Huazhong Agricultural University, ChinaReviewed by:
Tiejun Li, Institute for Nutritional Sciences, ChinaCopyright © 2022 Ma, Sun, Chen, Qi, Liu and Ma. This is an open-access article distributed under the terms of the Creative Commons Attribution License (CC BY). The use, distribution or reproduction in other forums is permitted, provided the original author(s) and the copyright owner(s) are credited and that the original publication in this journal is cited, in accordance with accepted academic practice. No use, distribution or reproduction is permitted which does not comply with these terms.
*Correspondence: Xi Ma, bWF4aUBjYXUuZWR1LmNu
Disclaimer: All claims expressed in this article are solely those of the authors and do not necessarily represent those of their affiliated organizations, or those of the publisher, the editors and the reviewers. Any product that may be evaluated in this article or claim that may be made by its manufacturer is not guaranteed or endorsed by the publisher.
Research integrity at Frontiers
Learn more about the work of our research integrity team to safeguard the quality of each article we publish.