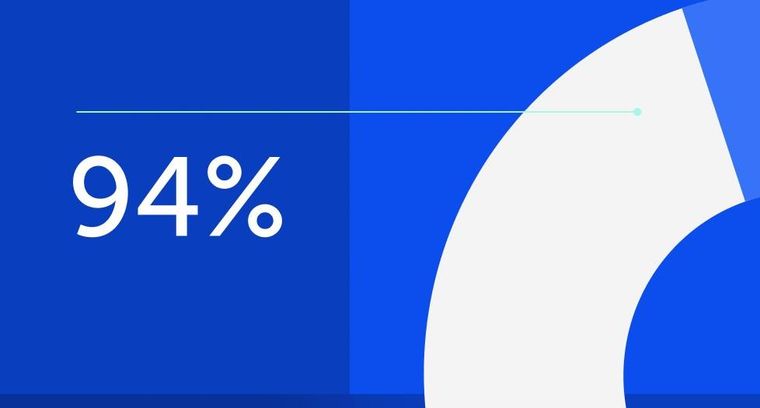
94% of researchers rate our articles as excellent or good
Learn more about the work of our research integrity team to safeguard the quality of each article we publish.
Find out more
ORIGINAL RESEARCH article
Front. Nutr., 24 March 2022
Sec. Nutrition and Food Science Technology
Volume 9 - 2022 | https://doi.org/10.3389/fnut.2022.846409
Studies have shown that inhibiting inflammation and regulating intestinal microflora imbalance is a significant factor in controlling the development of type 2 diabetes mellitus (T2DM). This experiment studied the protective effect of polyphenol extract from germinated mung beans on diabetic C57BL/6 mice.
Results: Fasting blood glucose (FBG) was decreased, glucose tolerance was increased, insulin resistance was decreased, serum lipid indexes in T2DM mice were improved, and the enzyme activities of alanine aminotransferase (ALT) and aspartate transaminase (AST) in serum were reduced. Meanwhile, the levels of interleukin 6 (IL-6), tumor necrosis factor-α (TNF-α), and C-reactive protein (CRP) in serum were decreased, the concentration of interleukin 10 (IL-10) in serum was increased, inhibiting the inflammatory reaction induced by diabetes and repairing the morphology of mice liver tissue. At the same time, germinated mung bean polyphenol (GMP) can regulate the main intestinal flora, Firmicutes, Bacteroidetes, and Proteobacteria in diabetic mice and can also regulate species diversity and improve intestinal flora imbalance. Taken together, the experimental conclusion is a certain dose of polyphenol extract from germinated mung beans that can improve mouse T2DM by inhibiting inflammatory reaction and regulating intestinal microflora.
Type 2 diabetes mellitus (T2DM) is a chronic disease accompanied by an inflammatory response, commonly referred to as non-insulin-dependent diabetes mellitus (1). The main features are increased blood glucose and insulin resistance (2, 3). According to WHO data, T2DM accounts for 90–95% of the cases, and its rapid growth has always been an international issue (4). T2DM has different degrees of the chronic low-level inflammatory response (5). The release of inflammatory factors may lead to islets β-cell structure and function is damaged, and even insulin secretion and transport function are impaired (6). Most treatments for T2DM are to stimulate insulin secretion, reduce glucose production, and enhance the effect of insulin on target tissues to reduce blood glucose levels (7, 8). In recent years, many studies have found that intestinal flora is also a critical factor involved in the treatment of diabetes, and it is found that it is connected to inflammation, insulin resistance, and islet function. Because of its sensitive reaction to factors, it is considered to be an important valuable tool for developing and promoting human health.
Legumes are considered to be the second most important food crops. Mung bean is one of the beans that people often eat in China (9) and is recommended for diabetics because of its high fiber content and low sugar content. At present, there is evidence that mung beans can ameliorate blood glucose, blood lipid, and blood pressure, protect the liver, and regulate immune activity. The beneficial effects mainly come from the active compounds in mung beans. It is reported that the active components of mung beans, especially polyphenols, have health benefits (10–12). As one of the food-processing methods, germination can significantly improve the nutritional value and the content of bioactive components of mung beans. Mung bean has more functional substances after germination (13), and its internal macromolecular substances will degrade into small molecules, which is more conducive to human absorption. The process of Mung Bean Germination is essentially the activation process of mung beans. Phenols are the products of secondary metabolism. They have health benefits (14), can reduce some risk factors of T2DM, and also show some anti-inflammatory effects (15, 16). In vitro and clinical studies have shown that polyphenols can regulate intestinal microbiota, conducive to intestinal health changes, reduce inflammatory process, and improve physical health indicators. It is considered as a new treatment strategy to treat various metabolic diseases, such as T2DM, by regulating intestinal flora and improving inflammatory response (17).
Therefore, mung bean was used as raw material in this experiment. After germination, polyphenol extract was obtained after extraction and purification. A high-fat diet (HFD) combined with an intraperitoneal injection of Streptozotocin (STZ) was used to establish a mouse model of T2DM. T2DM mice were fed with different concentrations of germinated mung bean polyphenols (GMPs) extract. The levels of fasting blood glucose (FBG), glucose tolerance, insulin level, blood lipid level, inflammatory factors, and liver tissue sections were analyzed. The aim of the study was to explore the effect of GMP extract on improving glucose and lipid metabolism and inhibiting inflammation in T2DM mice. The intestinal flora of mice was analyzed by Illumina NovaSeq and explored the relationship between diabetes and intestinal flora and inflammatory factors. This experiment provides experimental evidence for improving T2DM and also meets the demand for natural, safe, and effective hypoglycemic functional foods in the market at present.
Mung bean was purchased from Chifeng, Inner Mongolia, GMP extract is made by the laboratory of Food College of Heilongjiang Bayi Agricultural Reclamation University; STZ was purchased from Sigma of the United States; metformin hydrochloride was purchased from Shanghai Yuanye Biotechnology Co., Ltd. (>99.9% purity), and basic feed and high-fat feed were purchased from TROPHIC Animal Feed High-Tech Co. LTD, China. Feed code: TP23300.
Mung bean with good fresh quality was selected for germination. Using the response surface optimization method, the optimum germination process of mung bean was determined as follows: soaking temperature 25°C, soaking time 11 h, germination temperature 28°C, germination time 3 d, and CaCl2 concentration of soaking solution 2 mmol/L. Under these conditions, the polyphenol content was 487.81 mg/100 g. It is three times the content of mung beans. Mung beans are germinated, taken out and washed drying (40°C, blast drying oven), and crushing (80 mesh screening). Weigh the screened germinated mung bean powder into a beaker, add 70% ethanol solution according to the material-liquid ratio parameter of 1:26 (g/ml), take the supernatant through ultrasound, centrifugation, rotate, and evaporate to dryness at 45°C to obtain the crude polyphenol extract of germinated mung bean (18–21). Purify the crude polyphenol extract (AB-8 macroporous resin), collect the purified solution, and freeze-drying to obtain the GMP.
These experiments were carried out on 36 male C57BL/6 mice (4 weeks old), with an average weight of (18–20) g, provided by Liaoning Changsheng Biotechnology Co., Ltd. (Liaoning, China). The animal use license number is scxk (Liaoning) 2020-0001. The mice were placed at room temperature (23 ± 2°C), relative humidity (45 ± 5%), and light (12 h/12 h light/dark), cage feeding was carried out in a well-ventilated environment, and free eat food and drink water was adopted throughout the whole experiment.
A high-fat diet combined with an intraperitoneal injection of STZ was used in the experiment (30 mg/kg BW) to establish type 2 diabetic mice. Specific pathogen free (SPF) grade male mice were fed with the basal diet for 1 week. According to body weight, 30 mice were randomly selected as a diabetic model group. After 4 weeks, all mice were fasted 12 h later and injected with STZ. Normal mice were intraperitoneally injected with the same volume of citric acid buffer (pH = 4), continuously injected two times, and FBG was measured after 3 days. Blood was collected by caudal vein. After 1 week, the FBG in mice was more than 11.1 mmol/L for 7 days, and the type 2 diabetes mouse model was successfully established.
Six mice were randomly selected and fed normally as the control group (N). Diabetic mice were randomly divided into T2DM model group (M), low dose intervention group (GMPL), medium dose intervention group (GMPM), and high dose intervention group (GMPH). The low (50 mg/kg), medium (100 mg/kg), and high (150 mg/kg) dose intervention groups were given GMP every day. N group and M group received saline by gastric perfusion, for 5 weeks. Throughout the experiment, the diet, mental state, hair color, urine volume, body weight, and blood glucose of mice were observed. After intragastric administration for 5 weeks, fresh feces of mice in each group were collected in sealed sterile plastic tubes. Feces are frozen at −80°C for standby. After the last intervention, the mice fasted for 6 h, intragastric administration of 2 g/kg glucose (concentration 25%), blood glucose levels were measured at 0, 30, 60, 90, and 120 min after gavage. Before the end of the experiment, all mice fasted for 12 h, measured FBG and body weight, and then were anesthetized with ether. The eyeballs were taken out for blood. The blood was centrifuged at 3,500 r/min for 10 min, and the separated serum was stored at −80°C for index detection. Under anesthesia, the mice were dissected, taken out, and weighed to measure liver tissue. The left liver was fixed with 4% paraformaldehyde for histopathological analysis. The right liver was immediately frozen at −80°C for the determination of other indexes.
The concentrations of total cholesterol (TC), triglycerides (TG), high-density lipoprotein (HDL-C), high-density lipoprotein (LDL-C), alanine aminotransferase (ALT), and aspartate transaminase (AST) in serum were measured by kit, and the levels of inflammatory factors interleukin (IL)-6, IL-10, tumor necrosis factor-α (TNF-α), and C-reactive protein (CRP) were measured by ELISA kit. Start the operation according to the instructions of the kit. The kit was purchased from Nanjing Jiancheng Biotechnology Company (Nanjing, China). Homeostasis model assessment insulin resistance index (HOMA-IR) is often used to observe the level of insulin resistance. Calculation formula: HOMA-IR = FBG (mmol/L) × fasting insulin (FINS) (μU/ml)/22.5 (22). Homeostasis model assessment-β calculation formula: HOMA-β = (20 × FINS)/(FBG–3.5) (23).
After dissection, some liver tissues were fixed with 4% paraformaldehyde solution. The fixed liver tissues were embedded in paraffin, sectioned, and stained with hematoxylin-eosin (H&E). The liver injury and inflammatory cell infiltration were observed under a light microscope.
The feces of 4 mice in each group were randomly selected for intestinal flora analysis. Mouse fecal samples were tested by Beijing Baimaike Co., Ltd. for amplification, purify, quantify, and homogenize the products, form a sequencing database, and sequence them with Illumina NovaSeq 6,000.
The data were expressed as mean ± SD by origin (2018) software and statistically analyzed by SPSS (26.0). p < 0.05 was statistically significant.
The body weight and liver index of mice in the normal group and experimental group are shown in Figure 1. In the last week of GMP intervention, compared with group N, the weight of mice in the T2DM model group was declined significantly (Figure 1A). The weight of GMPM and GMPH intervention groups was increased significantly compared with group M.
Figure 1. Changes of body weight and liver index during GMP intervention. (A) Body weight. (B) Liver index. The data in the figure are expressed as mean ± SD (n = 6). Significance of difference: *means p < 0.05, **means p < 0.001, compared with group M. ##means p < 0.001, compared with group N. GMP, germinated mung bean polyphenol.
The liver index shows the relationship between liver weight and body weight of experimental animals and reflects the pathological phenomenon of swelling or atrophy of liver tissue in mice. Compared with group N, the liver index of mice in group M was increased significantly by 49.26%; compared with group M, the liver index of the GMPM group and GMPH group was decreased significantly (Figure 1B). The results show that T2DM mice will have obvious liver swelling, and GMP intervention can reduce the liver swelling of T2DM mice.
The effect of GMP on blood glucose in diabetic mice is shown in Figure 2A. The blood glucose level in group N was stable. Blood glucose level of group M was increased gradually after the establishment of the diabetes model. Compared with group M, FBG in GMPM and GMPH groups was decreased significantly after 4 weeks of GMP intervention. At the end of the whole experiment, the FBG concentration in GMPL, GMPM, and GMPH groups was significantly less than that in group M. The measurement results of FBG showed that GMP could improve the FBG level of T2DM mice during the intervention. In addition, the intervention effect of the GMPH group was better than that of other intervention groups.
Figure 2. Effects of different doses of GMP on (A) fasting blood glucose levels. (B) Oral glucose tolerance. (C) Blood glucose area under curve. (D) Fasting serum insulin levels. (E) HOMA-IR. (F) HOMA-β. The data in the figure are expressed as mean ± SD (n = 6). Significance of difference: *means p < 0.05, **means p < 0.001, compared with group M. ##means p < 0.001, compared with group N. GMP, germinated mung bean polyphenol; HOMA-IR, Homeostasis model assessment insulin resistance index.
Glucose tolerance refers to the body's ability to tolerate glucose according to the degree of glucose tolerance, judge the ability of islet β cells to regulate blood glucose (24). Blood glucose area under curve (AUC) can indicate the degree of glucose utilization and clearance by the human body. If the number increases, it indicates that the glucose tolerance of the body decreases, on the contrary, it indicates that the glucose tolerance of the body increases. The FBG concentration of mice in group N tended to change gently with time (Figure 2B). The blood glucose in group M was increased rapidly after 30 min of intragastric glucose, reached the peak, and then was decreased, but the FBG level was relatively high. The blood glucose level of each intervention group was decreased slowly after 60 min, but did not return to the initial blood glucose. AUC in group M was higher than that in group N; compared with group M, the performance of each intervention group was significantly lower (Figure 2C). The results showed that GMP could improve glucose tolerance of T2DM mice to a certain extent, and the recovery effect of the GMPH group was better in terms of recovery degree.
Insulin is the only hormone secreted by islet β cells that can reduce blood glucose and has an important effect on regulating blood glucose. As shown in Figures 2D,E, compared with group N, FINS level and HOMA-IR index in group M have increased significantly; compared with group M, different doses of GMP intervention group significantly reduced HOMA-IR index level. Although the insulin level decreased, there was no significant difference. HOMA-β is an important indicator (25) that can evaluate islets β cell function. When diabetes becomes serious, it decreased islet β cell function (26). As shown in Figure 2F, compared with group N, the HOMA-β index of group M has decreased significantly. Compared with group M, the HOMA-β index of each dose of the GMP intervention group can be significantly improved. According to the results of Figure 2, GMP can increase insulin sensitivity, reduce insulin resistance, and repair damaged islets β cells function in T2DM mice.
Diabetes is associated with blood lipid levels, and the lowering of blood lipids helps to improve diabetes. Compared with group N, the concentrations of TC, TG, and LDL-C in group M mice were significantly increased, and the concentration of HDL-C was decreased (Figure 3). The results showed that T2DM mice had lipid metabolism disorder. Compared with group M, the TC concentration in GMPL, GMPM, and GMPH groups was decreased by 16.86, 24.31, and 27.84%, respectively (p < 0.01), the LDL-C concentration was also decreased significantly, and the TG concentration in the GMPM group was also decreased by 20.49%. The results showed that GMP could regulate the disorder of lipid metabolism in T2DM mice.
Figure 3. Effects of different doses of GMP on TC, TG, HDL-C, and LDL-C levels. The data in the figure are expressed as mean ± SD (n = 6). Significance of difference: *means p < 0.05, **means p < 0.001, compared with group M. ##means p < 0.001, compared with group N. GMP, germinated mung bean polyphenol; TC, total cholesterol; TG, triglycerides; HDL-C, high-density lipoprotein; HDL-C, low-density lipoprotein (LDL-C), ALT, alanine aminotransferase; AST, aspartate transaminase.
Alanine aminotransferase and AST enzyme activities are necessary indexes to reflect liver function. However, liver pathology can more intuitively judge the degree of tissue damage (27). Compared with group N, the ALT and AST activities in mice M were increased, and compared with group M, the ALT and AST activities in the GMP intervention group were decreased significantly (Figure 4). The results showed that GMP could reduce the activity of serum ALT and AST in mice.
Figure 4. Effects of different doses of GMP on ALT and AST. The data in the figure are expressed as mean ± SD (n = 6). Significance of difference: *means p < 0.05, **means p < 0.001, compared with group M. ##means p < 0.001, compared with group N. GMP, germinated mung bean polyphenol; ALT, alanine aminotransferase; AST, aspartate transaminase.
The effect of GMP on the inflammatory response of T2DM mice is shown in Figure 5. Compared with group N, IL-6 in serum of group M mice was increased from 227.37 to 309.25 ng/L, TNF-α from 44.22 to 81.64 ng/L, CRP was increased from 27.84 to 47.68 μg/ml, and IL-10 was decreased significantly. CRP is a non-specific inflammatory marker. When the CRP is 10–50 mg/L, it indicates mild inflammation. Compared with group N, group M had an inflammatory reaction.
Figure 5. Effects of different doses of GMP on serum inflammatory factors in mice. (A) Serum TNF-α level. (B) Serum IL-6 level. (C) Serum IL-10 level. (D) Serum CRP level. The data in the figure are expressed as mean ± SD (n = 6). Significance of difference: *means p < 0.05, **means p < 0.001, compared with group M. ##means p < 0.001, compared with group N. GMP, germinated mung bean polyphenol; IL, interleukin, TNF-α, tumor necrosis factor-α.
Compared with group M, the concentrations of IL-6 and CRP in GMPM and GMPH groups were decreased significantly. Although they decreased in GMPL, the difference was not significant. The concentration of TNF-α was decreased significantly and the concentration of IL-10 was increased significantly in low, medium, and high dose intervention groups. The results showed that the GMP intervention group could further reduce the inflammatory reaction induced by diabetes by reducing the levels of IL-6, TNF-α, and CRP and increasing IL-10.
Compared with group M, there was no serious injury to the liver tissue of animals, indicating that the gavage dose of GMP is safe for animals. As shown in Figure 3, the liver tissue structure of group N is basically normal, no inflammatory cell infiltration is found, the liver cell structure is complete, the central vein of the tissue is clearly visible, the hepatic sinus is radial along the central vein, and the red arrow shows the macrophages of the hepatic sinus. Group M had abnormal liver tissue structure, loose hepatocyte structure, and more inflammatory cell infiltration (as shown by the black arrow in Figure 6). In the GMPL group, some hepatocytes are slightly edematous, and obvious inflammatory cell infiltration is seen in the tissue, as shown by the black arrow in Figure 6. GMPM liver tissue structure is slightly abnormal, no obvious inflammatory cell infiltration is found, and some hepatocytes are slightly edematous, as shown by the yellow arrow in Figure 6. In the GMPH group, the liver tissue structure was basically normal, the hepatocyte structure was full, the central vein of the tissue was clearly visible, and there was no obvious inflammatory cell infiltration.
Figure 6. Effects of different doses of GMP on liver tissue of mice. (A) Normal group. (B) T2DM model group. (C) GMPL group. (D) GMPM group. (E) GMPH group. Magnification: (A–E) ×100. GMP, germinated mung bean polyphenol; T2DM, type 2 diabetes mellitus; GMPL, low dose intervention group; GMPM, medium dose intervention group; GMPH, high dose intervention group.
Alpha diversity shows species abundance and diversity of samples. As shown in Figure 7, the indexes of ACE, Chao, Shannon, and Simpson of intestinal flora in the M group are lower than in the N group, indicating that the species diversity of T2DM mice is reduced. Compared with group M, GMP could improve the intestinal flora α diversity index that can inhibit the decline of intestinal flora richness and increase the species diversity of flora. This experiment also counts the coverage rate, indicating that the sequencing results of the experiment represent the real situation of microorganisms in the sample. The higher the coverage rate, the greater the probability of species being detected. It shows that GMP intervention can balance the imbalance of intestinal flora to a certain extent.
Figure 7. Changes of intestinal flora in mice α diversity index. (A) Chao index. (B) Ace index. (C) Shannon index. (D) Simpson index. (E) Cover rate. (F) Rarefaction Curve. (G) Shannon index. (H) Rank Abundance Curve. Significance of difference: *means p < 0.05, **means p < 0.001, compared with group M. ##means p < 0.001, compared with group N.
A rarefaction curve can be used to judge whether the sample sequencing quantity is sufficient. As shown in Figure 7F, within the scope of the experiment, with the increase of sequencing times, the curve rises rapidly, indicating that many new species have been found; if the curve is flat, it indicates that the species in the environment will not increase with the increase of sequencing times. Shannon index curve shows the microbial diversity in samples with different sequencing quantities. The greater the Shannon index, the richer the species. As shown in Figure 7G, when the curve is flat, it indicates that the amount of data sequenced are sufficient, and the species will not increase. Increased sequencing times, Rank Abundance Curve can describe the abundance and consistency of species in the sample. As shown in Figure 7H, the wider the curve, the more the species composition, and the flat curve indicates that the uniformity of species composition is higher.
As shown in Figure 8, the comparative analysis of each group at the gate level shows that the intestinal flora mainly includes Firmicutes, Bacteroidetes, Proteobacteria, Actinobacteria, Verrucomicrobia, etc. The number of Firmicutes and Bacteroides in group M was significantly higher than that N group (p < 0.01). After GMP intervention, the number of Firmicutes were decreased significantly compared with group M, the abundance of Bacteroidetes was increased significantly, and the abundance of Proteus was decreased significantly. In short, the intestinal flora can balance in diabetic mice, and GMP intervention can regulate the abundance and diversity of T2DM mice.
Figure 8. Analysis of phylum level of intestinal flora in mice. (A) Phylum level species distribution. (B) Heat map of species distribution at the gate level. (C) Firmicutes. (D) Bacteroidetes. (E) Proteobacteria. Significance of difference: *means p < 0.05, **means p < 0.001, compared with group M. ##means p < 0.001, compared with group N.
The correlation between serum inflammatory factors, intestinal flora, and metabolic indexes was analyzed by Spearman. There is a positive correlation between blood glucose index (FBG, AUC, FINS, and HOMA-IR) and blood lipid index (TC, TG, and LDL-C), indicating that blood glucose index increases and blood lipid level also increases (Figure 9). IL-10 and Bacteroidetes were negatively correlated with blood glucose and lipid index, ALT, AST, IL-6 and TNF-α, CRP, Firmicutes, and Proteobacteria were positively correlated with blood glucose and lipid index. Firmicutes, Proteobacteria and IL-6, TNF-α, CRP were positively correlated and negatively correlated with IL-10. Bacteroidetes and IL-6, TNF-α, CRP were negatively correlated and positively correlated with IL-10. It indicates that inflammatory factors and intestinal flora are closely related to diabetes mellitus.
Figure 9. Heat map of correlation analysis between serum inflammatory factors, intestinal flora, and metabolic indexes Purple indicates negative correlation and red indicates positive correlation.*p < 0.05 and **p < 0.001, respectively.
This experiment discusses the molecular mechanism of GMP improving T2DM from the perspective of intestinal microbiota and inflammatory factors. The results showed that GMP could improve glucose and lipid metabolism in T2DM mice induced by HFD and STZ, reduce hyperglycemia and insulin resistance, enhance glucose tolerance, repair liver tissue damage, and inhibit the inflammatory response. Further studies show that the mechanism of GMP regulating blood glucose may be related to the regulation of intestinal microbiota. 16SrRNA high-throughput sequencing showed that GMP could significantly decrease the abundance of Firmicutes and Proteobacteria and promote the proliferation of Bacteroidetes.
Type 2 diabetes mellitus is a metabolic disease based on the diagnosis of continuous hyperglycemia (28). The most obvious feature is glucose and lipid metabolism disorder, which can lead to pathological changes in tissues, organs, and digestive system (29), which is mainly caused by genetic and environmental factors, and the morbidity rate has continued to rise in recent years. Although the commonly used drugs for diabetes treatment in the market have good effects, most of them have some side effects. Therefore, the study of a natural and harmless good hypoglycemic functional food will soon become the focus of the current study of diabetes demand. Studies have shown that polyphenols extracted from beans (30), corn (31), black rice (32), and black tea (33) can inhibit glucose transport. Intake of polyphenol-rich diet can significantly reduce the risk of diabetes (34), which is due to polyphenols beneficial to glucose metabolism, can significantly improve glucose tolerance (35). This experiment found that GMPs can also reduce blood sugar, improve insulin resistance, and enhance glucose tolerance, which is consistent with the research conclusions mentioned above. Diabetes is closely related to blood lipid levels. Lowering blood lipid levels will help improve diabetes. It has been reported that HDL-C decreases and TC, TG, and LDL-C increase has adverse effects on mice (36). The results showed that the germination of mung bean polyphenol extract could reduce the levels of TC, TG, and LDL-C in serum of diabetic mice, increase HDL-C level, have a good regulatory effect on lipid metabolism disorder in T2DM mice, and it has positive effects on regulating the development of diabetes.
Diabetic patients will produce chronic inflammation in the body. TNF-α, IL-6, and IL-10 are inflammatory factors secreted by inflammatory cells (37). TNF-α, as the earliest inflammatory factor in the process of body inflammatory, plays an important role in glycoprotein signal transduction and synthesis. The level of TNF-α in patients with abnormal glucose tolerance increases significantly; IL-6 can control the signal transduction of insulin receptor and reduce insulin sensitivity. CRP is a non-specific inflammatory marker, which is synthesized by hepatocytes. The most important regulatory factors of CRP synthesis are IL-6 and TNF-α. The degree of its increase reflects the size and activity of inflammatory tissue. The liver is the key organ of metabolism and participates in the storage and emission of metabolites (38). Experiments show that GMP can reduce the levels of IL-6, TNF- α, and CRP, increase the level of IL-10, and then alleviate the inflammatory reaction induced by diabetes and repair the phenomenon of hepatocyte lysis and inflammatory cell infiltration in mice, so as to improve the liver injury. Studies have proved that germinated mung bean is an effective liver protective agent, which can reduce liver enzyme activity and repair damaged liver tissue within the experimental dose range (39).
In recent years, many animal and clinical experiments have shown that polyphenols can regulate the intestinal flora and the proportion of Firmicutes to Bacteroides (F/B) (40–42). The first organ contacted after dietary intake is the gastrointestinal tract, so the intestinal tract may be the main place where polyphenols play a metabolic role (43). The improvement of blood glucose and the regulation of blood lipid in this study may be closely related to the inhibition of the level of inflammatory factors in serum and the regulation of intestinal flora richness. One of the reasons why GMP can have an anti-inflammatory effect may be the regulation of intestinal flora. It was found that the higher the abundance of Firmicutes and Proteobacteria, the higher the levels of blood glucose and inflammatory indexes. The abundance of Bacteroidetes increased and the levels of blood glucose and inflammatory indexes decreased. It is reported that Bacteroidetes have anti-inflammatory and immune regulating effects, and the number of Bacteroidetes is reduced in the intestinal flora of the inflammatory mouse model (44, 45). The species diversity and abundance of intestinal flora in T2DM mice are closely related to the levels of blood glucose and inflammatory factors. Improving T2DM metabolic diseases by regulating intestinal flora and improving inflammatory response is considered to be a new treatment strategy (17).
In summary, the results show that GMP can increase the intestinal microbial diversity, balance the dysfunctional intestinal flora, reduce inflammation and repair the liver injury, regulate the disorder of glucose and lipid metabolism, and finally reduce the blood glucose and blood lipid levels of T2DM mice. The results showed that the polyphenol extract from germinated mung bean is a natural hypoglycemic substance with potential and research value and has potential application value in the treatment of diabetes.
The original contributions presented in the study are publicly available. This data can be found here: https://www.ncbi.nlm.nih.gov/search/all/?term=PRJNA796743.
The animal study was reviewed and approved by the Committee on Animal Care of the College of Animal Science and Veterinary Medicine of Heilongjiang Bayi Agricultural University.
DZ and AZ provided experimental plans and ideas. XS conducted experiments, sorted out data, and drew drawings. XJ, LQ, and FZ helped analyze data and participated in paper writing. All authors have read and agreed to submit the manuscript.
Natural Science Fund Research Team Project of Heilongjiang Provincial Department of Science and Technology (TD2020C003); National Key R&D Plan, National Key R&D Plan of the Ministry of Science and Technology (2018YFE0206300).
The authors declare that the research was conducted in the absence of any commercial or financial relationships that could be construed as a potential conflict of interest.
All claims expressed in this article are solely those of the authors and do not necessarily represent those of their affiliated organizations, or those of the publisher, the editors and the reviewers. Any product that may be evaluated in this article, or claim that may be made by its manufacturer, is not guaranteed or endorsed by the publisher.
1. Wang YM, Dilidaxi D, Wu YC, Sailike J, Sun X, Nabi XH. Composite probiotics alleviate type 2 diabetes by regulating intestinal microbiota and inducing GLP-1 secretion in db/db mice. Biomed Pharmacother. (2020) 125:109914. doi: 10.1016/j.biopha.2020.109914
2. Kahn SE, Hull RL, Utzschneider KM. Mechanisms linking obesity to insulin resistance and type 2 diabetes. Nature. (2006) 444:840–6. doi: 10.1038/nature05482
3. Kubota T, Kubota N, Kadowaki T. Imbalanced insulin actions in obesity and type 2 diabetes: key mouse models of insulin signaling pathway. Cell Metab. (2017) 25:797–810. doi: 10.1016/j.cmet.2017.03.004
4. DeFronzo RA, Ferrannini E, Groop L, Henry RR, Herman WH, Holst JJ, et al. Type 2 diabetes mellitus. Nat Rev Dis Primers. (2015) 1:15019. doi: 10.1038/nrdp.2015.19
5. Huang JZ, Guan BB, Lin LJ, Wang YP. Improvement of intestinal barrier function, gut microbiota, and metabolic endotoxemia in type 2 diabetes rats by Curcumin. Bioengineered. (2021) 12:11947–58. doi: 10.1080/21655979.2021.2009322
6. Yang K, Lauritzen KH, Olsen MB, Dahl TB, Ranheim T, Ahmed MS, et al. Low cellular NAD compromises lipopolysaccharide-induced inflammatory responses via inhibiting TLR4 signal transduction in human monocytes. J Immunol. (2019) 203:1598–608. doi: 10.4049/jimmunol.1801382
7. Kahn SE. The relative contributions of insulin resistance and beta-cell dysfunction to the pathophysiology of type 2 diabetes. Diabetologia. (2003) 46:3–19. doi: 10.1007/s00125-002-1009-0
8. Stumvoll M, Goldstein BJ, van Haeften TW. Type 2 diabetes: principles of pathogenesis and therapy. Lancet. (2005) 365:1333–46. doi: 10.1016/S0140-6736(05)61032-X
9. Hou DZ, Yousaf L, Xue Y, Hu JR, Wu JH, Hu XS, et al. Vigna radiate mung bean (L.): bioactive polyphenols, polysaccharides, peptides, and health benets. Nutrients. (2019) 11:1238. doi: 10.3390/nu11061238
10. Giusti F, Caprioli G, Ricciutelli M, Torregiani E, Vittori S, Sagratini G. Analysis of 17 polyphenolic compounds in organic and conventional legumes by high-performance liquid chromatography-diode array detection (HPLC-DAD) and evaluation of their antioxidant activity. Int J Food Sci Nutr. (2018) 69:557–65. doi: 10.1080/09637486.2017.1399258
11. Ketha K, Gudipati M. Immunomodulatory activity of non starch polysaccharides isolated from green gram (Vigna radiata). Food Res Int. (2018) 113:269–76. doi: 10.1016/j.foodres.2018.07.010
12. Sonklin C, Laohakunjit N, Kerdchoechuen O. Assessment of antioxidant properties of membrane ultrafiltration peptides from mungbean meal protein hydrolysates. Peer J. (2018) 6:e5337. doi: 10.7717/peerj.5337
13. Huang XY, Cai WX, Xu BJ. Kinetic changes of nutrients and antioxidant capacities of germinated soybean (Glycine max L.) and mung bean (Vigna radiata L.) with germination time. Food Chem. (2014) 143:268–76. doi: 10.1016/j.foodchem.2013.07.080
14. Guo XB, Li T, Tang KX, Liu RH. Effect of germination on phytochemical profiles and antioxidant activity of mung bean sprouts (Vigna radiata). J Agric Food Chem. (2012) 60:11050–5. doi: 10.1021/jf304443u
15. Kalt W, Cassidy A, Howard LR, Krikorian R, Stull AJ, Tremblay F, et al. Recent research on the health benefits of blueberries and their anthocyanins. Adv Nutr. (2020) 11:224–36. doi: 10.1093/advances/nmz065
16. Williamson G. The role of polyphenols in modern nutrition. Nutr Bull. (2017) 42:226–35. doi: 10.1111/nbu.12278
17. Zhang LY, Chen XH, Wang HL, Huang HP, Li MY, Yao L, et al. Adjusting internal organs and dredging channel electroacupuncture ameliorates insulin resistance in type 2 diabetes mellitus by regulating the intestinal flora and inhibiting inflammation. Diabetes Metab Syndrom Obesity Targets Therapy. (2021) 14:2595–607. doi: 10.2147/DMSO.S306861
18. López-Fernández O, Bohrer BM, Munekata PES, Domínguez R, Pateiro M, Lorenzo JM. Improving oxidative stability of foods with apple-derived polyphenols. Compr Rev Food Sci Food Saf . (2021) 21:296–320. doi: 10.1111/1541-4337.12869
19. Atiya A, Majrashi T, Begum YM, Abdul QSF, Alqahtani AS, Ali AAS, et al. Opuntia cus-indicaInuence of solvent selection and extraction methods on the determination of polyphenols, antioxidant, lipoxygenase and tyrosinase inhibition activities of fruits peel and pulp collected from the Kingdom of Saudi Arabia (KSA). Nat Prod Res. (2021) 10:1–8. doi: 10.1080/14786419.2021.1983571
20. Skenderidis P, Leontopoulos S, Petrotos K, Giavasis I. Optimization of vacuum microwave-assisted extraction of pomegranate fruits peels by the evaluation of extracts' phenolic content and antioxidant activity. Foods. (2020) 9:1655. doi: 10.3390/foods9111655
21. Oroian M, Ursachi F, Dranca F. Ultrasound-assisted extraction of polyphenols from crude pollen. Antioxidants. (2020) 9:322. doi: 10.3390/antiox9040322
22. Wallace TM, Levy JC, Matthews DR. Use and abuse of HOMA modeling. Diabetes Care. (2004) 27:1487–95. doi: 10.2337/diacare.27.6.1487
23. Haffner SM, Greenberg AS, Weston WM, Chen HZ, Williams K, Freed MI. Effect of rosiglitazone treatment on nontraditional markers of cardiovascular disease in patients with type 2 diabetes mellitus. Circulation. (2002) 106:679–84. doi: 10.1161/01.cir.0000025403.20953.23
24. Hossain MS, Barua A, Tanim MAH, Hasan MS, Islam MJ, Hossain MR, et al. Ganoderma applanatum mushroom provides new insights into the management of diabetes mellitus, hyperlipidemia, and hepatic degeneration: a comprehensive analysis. Food Sci Nutr. (2021) 9:4364–74. doi: 10.1002/fsn3.2407
25. Tian SH, Li XF, Wang YF, Lu YJ. The protective effect of sulforaphane on type II diabetes induced by high-fat diet and low-dosage streptozotocin. Food Sci Nutr. (2021) 9:747–56. doi: 10.1002/fsn3.2040
26. Daems C, Welsch S, Boughaleb H, Vanderroost J, Robert A, Sokal E, et al. βEarly treatment with empagliflozin and GABA improves -cell mass and glucose tolerance in streptozotocin-treated mice. J Diabetes Res. (2019) 2019:2813489. doi: 10.1155/2019/2813489
27. Khattab H, Fouad A, Hamza M, Mohey MA, El-Akel W, Ghoneim H, et al. Relation of ALT and AST levels to the histopathological changes in liver biopsies of patients with chronic hepatitis C genotype 4. Arab J Gastroenterol. (2015) 16:50–3. doi: 10.1016/j.ajg.2015.06.004
28. Gerstein HC, Miller ME, Byington RP, Goff DC, Bigger JT, Buse JB, et al. Effects of intensive glucose lowering in type 2 diabetes. N Engl J Med. (2008) 358:2545–59. doi: 10.1056/NEJMoa0802743
29. Subramani S, Leelavinothan P. Ameliorative effect of diosmin, a citrus flavonoid against streptozotocin-nicotinamide generated oxidative stress induced diabetic rats. Chem Biol Interact. (2012) 195:43–51. doi: 10.1016/j.cbi.2011.10.003
30. Ademiluyi AO, Oboh G. Phenolic-rich extracts from selected tropical underutilized legumes inhibit α-amylase, α-glucosidase, and angiotensin I converting enzyme in vitro. J Basic Clin Physiol Pharmacol. (2012) 23:17–25. doi: 10.1515/jbcpp-2011-0005
31. Kwon YI, Apostolidis E, Shetty K. In vitro studies of eggplant (Solanum melongena) phenolics as inhibitors of key enzymes relevant for type 2 diabetes and hypertension. Bioresour Technol. (2008) 99:2981–8. doi: 10.1016/j.biortech.2007.06.035
32. Yao Y, Sang W, Zhou MJ, Ren GX. Antioxidant and alpha-glucosidase inhibitory activity of colored grains in China. J Agric Food Chem. (2010) 58:770–4. doi: 10.1021/jf903234c
33. Koh LW, Wong LL, Loo YY, Kasapis S, Huang DJ. Evaluation of different teas against starch digestibility by mammalian glycosidases. J Agric Food Chem. (2010) 58:148–54. doi: 10.1021/jf903011g
34. Grosso G, Stepaniak U, Micek A, Kozela M, Stefler D, Bobak M, et al. Dietary polyphenol intake and risk of type 2 diabetes in the polish arm of the health, alcohol and psychosocial factors in eastern Europe (HAPIEE) study. Br J Nutr. (2017) 118:60–8. doi: 10.1017/S0007114517001805
35. Shoji T, Yamada M, Miura T, Nagashima K, Ogura K, Inagaki N, et al. Chronic administration of apple polyphenols ameliorates hyperglycaemia in high-normal and borderline subjects: a randomised, placebo-controlled trial. Diabetes Res Clin Pract. (2017) 129:43–51. doi: 10.1016/j.diabres.2017.03.028
36. Stein O, Stein Y. Atheroprotective mechanisms of HDL. Atherosclerosis. (1999) 144:285–301. doi: 10.1016/s0021-9150(99)00065-9
37. Jang SY, Choi SH, Kikkawa D, Lee EJ, Yoon JS. Association of fibroblast growth factor 10 with the fibrotic and inflammatory pathogenesis of Graves' orbitopathy. PLoS ONE. (2021) 16:e0255344. doi: 10.1371/journal.pone.0255344
38. Dey A, Cederbaum AI. Alcohol and oxidative liver injury. Hepatology. (2006) 43:S63–74. doi: 10.1002/hep.20957
39. Lays ARL, Maria do Carmo de CM, Luciana MF, Karoline de Macêdo GF. Cholesterol-lowering and liver-protective eects of cooked and germinated mung beans (Vigna radiata L.). Nutrients. (2018) 10:821. doi: 10.3390/nu10070821
40. Etxeberria U, Arias N, Boqué N, Macarulla MT, Portillo MP, Martínez JA, et al. Reshaping faecal gut microbiota composition by the intake of trans-resveratrol and quercetin in high-fat sucrose diet-fed rats. J Nutr Biochem. (2015) 26:651–60. doi: 10.1016/j.jnutbio.2015.01.002
41. Taira T, Yamaguchi S, Takahashi A, Okazaki Y, Yamaguchi A, Sakaguchi H, et al. Dietary polyphenols increase fecal mucin and immunoglobulin A and ameliorate the disturbance in gut microbiota caused by a high fat diet. J Clin Biochem Nutr. (2015) 57:212–6. doi: 10.3164/jcbn.15-15
42. Seo DB, Jeong HW, Cho DY, Lee BJ, Lee JH, Choi JY, et al. Fermented green tea extract alleviates obesity and related complications and alters gut microbiota composition in diet-induced obese mice. J Med Food. (2015) 18:549–56. doi: 10.1089/jmf.2014.3265
43. Barnes S, Prasain J, D'Alessandro T, Weaver CM. The metabolism and analysis of isoavones and other dietary polyphenols in foods and biological systems. Food Funct. (2011) 2:235–44. doi: 10.1039/c1fo10025d
44. Su LL, Mao CQ, Wang XC, Li L, Tong HJ, Mao J, et al. Schisandra chinensis the anti-colitis effect of polysaccharide is associated with the regulation of the composition and metabolism of gut microbiota. Front Cell Infect Microbiol. (2020) 10:519479. doi: 10.3389/fcimb.2020.519479
Keywords: T2DM, inflammation, intestinal flora, hypoglycemic, germinated mung bean polyphenols
Citation: Shen X, Jiang X, Qian L, Zhang A, Zuo F and Zhang D (2022) Polyphenol Extracts From Germinated Mung Beans Can Improve Type 2 Diabetes in Mice by Regulating Intestinal Microflora and Inhibiting Inflammation. Front. Nutr. 9:846409. doi: 10.3389/fnut.2022.846409
Received: 31 December 2021; Accepted: 17 January 2022;
Published: 24 March 2022.
Edited by:
Zhaojun Wei, Hefei University of Technology, ChinaReviewed by:
Bailiang Li, Northeast Agricultural University, ChinaCopyright © 2022 Shen, Jiang, Qian, Zhang, Zuo and Zhang. This is an open-access article distributed under the terms of the Creative Commons Attribution License (CC BY). The use, distribution or reproduction in other forums is permitted, provided the original author(s) and the copyright owner(s) are credited and that the original publication in this journal is cited, in accordance with accepted academic practice. No use, distribution or reproduction is permitted which does not comply with these terms.
*Correspondence: Dongjie Zhang, YnluZHpkakAxMjYuY29t
Disclaimer: All claims expressed in this article are solely those of the authors and do not necessarily represent those of their affiliated organizations, or those of the publisher, the editors and the reviewers. Any product that may be evaluated in this article or claim that may be made by its manufacturer is not guaranteed or endorsed by the publisher.
Research integrity at Frontiers
Learn more about the work of our research integrity team to safeguard the quality of each article we publish.