- 1School of Pharmacy, Zhejiang Chinese Medical University, Hangzhou, China
- 2School of Pharmacy, Nanjing University of Chinese Medicine, Nanjing, China
- 3The Third Affiliated Hospital of Zhejiang Chinese Medical University, Hangzhou, China
Cornus officinalis, a kind of edible herbal medicine, has been widely used in the protection of liver and kidney due to its medicinal and nutritional effect. Its anti-inflammatory, anti-tumor, and anti-oxidant activities can be enhanced by wine-steamed (WS) processing. Based on the activations of hepatic stellate cells-T6 (HSC-T6) and HK-2, our study used single-factor plus orthogonal design to investigate the anti-fibrosis of C. officinalis processed with steamed (S), high-pressure steamed (HPS), WS, high-pressure wine-steamed (HPWS), wine-dipped (WD), and wine-fried (WF). The chemical constituents in processed C. officinalis with higher anti-fibrotic activities were detected by ultra-high performance liquid chromatography coupled with hybrid triple quadrupole time-of-flight mass spectrometry (UHPLC-Q-TOF-MS/MS). Results showed that C. officinalis with HPWS significantly inhibited the activations of HSC-T6 and HK-2. Moreover, compounds in C. officinalis with HPWS were obtained via UHPLC-Q-TOF-MS/MS, indicating that 27 components were changed compared with raw C. officinalis. These results demonstrated that HPWS is the optimal processing technology for anti-fibrosis of C. officinalis.
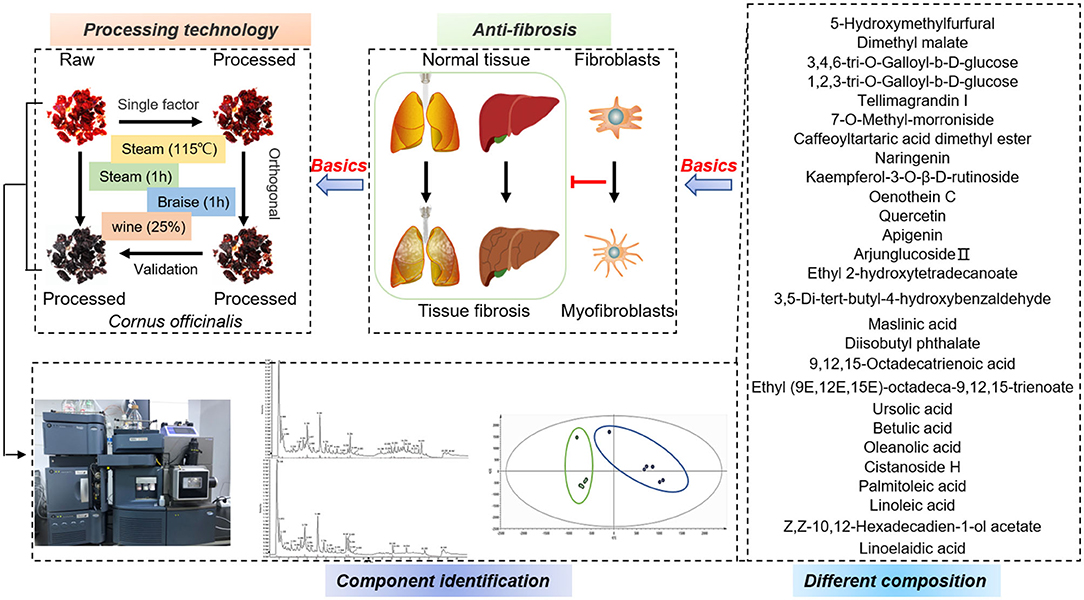
Graphical Abstract. The processing technology of C. officinalis was optimized in terms of its anti-fibrotic activity. Differences in its chemical composition after the preparation were detected by UHPLC-Q-TOF-MS/MS. These results provide material basis for further exploring the role of C. officinalis in liver and kidney protection.
Introduction
Cornus officinalis (C. officinalis), the dry mature fruit of C. officinalis Siebold & Zucc, is redefined as a class of herb and edible plant and has been commonly used in traditional Chinese medicine (TCM) (1). C. officinalis with mild warm nature, belongs to the meridians of the liver and kidney according to TCM theory; thus, it is commonly used in the prevention and treatments of liver and kidney diseases (2). Moreover, it can be found in foodstuff, such as medicinal dishes, healthcare products, and drinks due to its various pharmacological activities, including anti-inflammatory, antioxidant, and anti-apoptotic (3). To date, about 305 components have been isolated and identified from C. officinalis, including iridoids, alkaloids, polysaccharides, flavones, organic acid, essential oils, and terpenoids (1). Among these compounds, loganin and morroniside are active ingredients in C. officinalis and could alleviate osteoarthritis in mice by inhibiting pyroptosis and NF-kappaB activity (4, 5). Furthermore, morroniside could ameliorate neuropathic pain through the regulation of glucagon-like peptide-1 (GLP-1) receptors. 5-hydroxymethylfurfural (5-HMF), which are mainly isolated from processed C. officinalis, could prevent human umbilical vein endothelial cells (HUVECs) from oxidative stress induced by glucose (6). However, the compounds in C. officinalis may change with processing and in turn affect pharmacological activities of C. officinalis. In traditional crafts of China, C. officinalis were often processed with fried, steamed, wined, fated, salted, and others, to meet different clinical effects. For example, wined C. officinalis is most commonly used in clinical preparations, such as the Liuwei Dihuang pills in China, which has better effects in nourishing the liver and kidney compared to raw (R) C. officinalis. However, there is no unified requirement for steamed, braised times and wine amount, all of which could lead to the difference in the compositions and activities of C. officinalis. Therefore, the present study aimed to optimize the processing technology of C. officinalis through the single-factor method plus orthogonal experiment design based on the anti-fibrotic activities of C. officinalis.
Tissue fibrosis, characterized by excessive deposition of extracellular matrix (ECM), is the outcome of chronic tissue damage, leading to the formation of scar tissue, if without treatments, fibrosis will promote organ dysfunction and even failure (7). The ECM is mainly derived from proliferative and fibrogenic myofibroblasts, which are fibroblast-like cells with contractile properties (8, 9). Activated hepatic stellate cells (HSC-T6) are the main source of myofibroblasts and serve as a key driver of hepatic fibrosis in liver injury (9). In normal liver, HSC-T6 are in quiescent state and involved in the storage of vitamin A (10). During liver injury, quiescent HSC-T6 are activated, which indicated by the high expression of alpha smooth muscle actin (α-SMA) and excessive deposition of ECM (11). Similar to HSC-T6, the human proximal tubular epithelial cell (HK-2) cells play a key role in tubulointerstitial fibrosis (TIF), which is the final result of chronic kidney disease and closely related to the degeneration of renal function (12). Moreover, epithelial mesenchymal transition (EMT) is the main pathogenesis of the renal fibrosis and can transform differentiated epithelial cells into myofibroblasts, which are also characterized by the high expression of α-SMA (13, 14). During fibrogenesis, EMT may be driven by transforming growth factor β (TGF-β), which is the most important pro-fibrotic growth factors (15). Apart from these, TGF-β is regarded as a common vital switch for fibrosis in tissue or organs in response to chronic injuries (16).
In summary, we will evaluate the effects of C. officinalis on anti-fibrosis via the expressions of α-SMA in TGF-β induced-HSC-T6 and HK-2 cells, with the goal of exploring the protective effect of C. officinalis on the liver and kidney, and finally select the processing technology with higher anti-fibrotic activity. Moreover, the changes of chemical constituents in processed C. officinalis will be detected by ultra-high performance liquid chromatography coupled with hybrid triple quadrupole time-of-flight mass spectrometry (UHPLC-Q-TOF-MS/MS).
Materials and Methods
Plant Materials and Processing Technology
The samples of C. officinalis were selected from the PanAn City of Zhejiang Province and identified by Professor Jianwei Chen of the Department of Chinese Medicine Identification, School of Pharmacy, Nanjing University of Chinese Medicine. According to single-factor experiments, C. officinalis samples were steamed at different times (1, 2, 4, 6, and 8 h), different temperatures (100, 105, 110, 115, 120, and 125°C) and then dried at 60°C, respectively. Next, the samples were braised at different times (0.5, 1, 2, and 4 h) with different dosages of rice wine (w/w) (15, 20, 25, 30, and 40%), respectively. These samples were then steamed again for 1 h at 105°C and then finally dried at 60°C. The orthogonal experimental design was based on the results of single-factor experiments as discussed in the following: The C. officinalis samples were processed with three factors (steamed times, steamed temperatures, and braised times) at different dosages of rice wine in line with L9 (34) design orthogonal table shown in S1, S3, and S5, respectively. Moreover, the optimal parameters of C. officinalis with high-pressure wine steamed (HPWS) were identified with steamed for 1 h at 115°C, braised time of 1 h, and rice wine dosage (w/w) at 25%. The HPS was obtained with processed for 1 h at 125°C. Additionally, C. officinalis could be processed with wine-dipped (WD) and wine fried (WF). Cornus officinalis that steamed for 1 h was named as S.
Extraction Preparation
The processed C. officinalis (both R and processed) was crushed and sifted through 16-mesh screen. The powder (5 g) was refluxed with 90% ethanol (50 ml) twice and filtered with four layers of gauze. The filtrates were collected and combined. Next, the filtrates were transformed into freeze-dried powder through vacuum concentration and lyophilization.
UHPLC-Q-TOF-MS/MS Analysis
The LC–MS/MS analysis were performed using an UHPLC (Shimadzu LC-30AD, Japan) coupled with a Triple TOF 5600 Plus System (AB Sciex, USA) (17, 18). The parameters that were followed were as follows: Column temperature was 30°C; mobile phase was acetonitrile (B) and 0.1% formic acid in water (A); flow rate was 0.3 ml/min; and the injection volume was 3 μl. The gradient elution procedure was as follows: 0–3.0 min, 5–20% B; 3.0–7.0 min, 20–80% B; 7.0–30 min, 80–90% B; 30–32 min, 90–5% B; 30–32 min, B was maintained at 5%. For UHPLC separation, the samples were analyzed with a 2.1 mm × 100 mm ZORBAX Extend-C18 1.8 μm column (Agilent, USA). The conditions in both electrospray ionization (ESI), positive and negative modes were set as follows: The ion source temperature was 550°C, IonSpray voltage was 5,500–5,500 V, auxiliary spray gas was nitrogen, Ion Source Gas1 (Gas1) was 55 psi, Ion Source Gas2 (Gas2) was 55 psi, curtain gas (CUR) was 35 psi, declustering potential (DP) was 60 V, and collision energy was 30 V. The scanned ranges of TOF–MS and TOF–MS/MS were 100–2,000 and 50–1,000 Da, respectively.
Cell Culture and Treatment
The HSC-T6 and HK-2 cells were cultured in Dulbecco's modified Eagle medium (DMEM) (Abcam, UK) and Dulbecco's Modified Eagle Media DMEM/F12 (Abcam, UK), respectively, supplemented with 100 mg/ml streptomycin, 100 U/ml penicillin, and 10% fetal bovine serum (FBS) (Abcam, UK) under 5% CO2 at 37°C. When establishing liver fibrosis in vitro, HSC-T6 and HK-2 cells were cultured in six-well plates and treated with TGF-β (PeproTech, USA), respectively. To detect the effects of C. officinalis on anti-fibrosis, cells were treated with extracts derived from C. officinalis (both R and processed).
Western Blot Analysis
The cells were lysed using radio-immunoprecipitation assay (RIPA) buffer supplemented with 1% phenylmethanesulfonyl fluoride (PMSF) (Solarbio, Beijing, China) and then quantified by BCA protein assay kit (Beyotime, Shanghai, China). The equivalent protein samples were separated by sodium dodecyl sulfate–polyacrylamid gel electrophoresis (SDS–PAGE) and then transferred to polyvinylidene fluoride (PVDF) membranes (GE, Freiburg, Germany). The membranes were blocked with 5% skimmed milk for 1 h at room temperature and incubated with anti-α-SMA antibody (Abcam, UK) overnight at 4°C. The membranes were incubated with horse radish peroxidase (HRP)-conjugated secondary antibodies for 1 h at room temperature and visualized with ECL Reagent (Beyotime, Shanghai, China). Finally, the membranes were stripped and probed with GAPDH (loading control). The intensities of bands were quantified by Quantity One software (Bio-Rad, Hercules, CA, USA).
Immunocytochemistry
The HSC-T6 cells were cultured in six-well plates and fixed with 4% paraformaldehyde, permeabilized in 0.1% Triton X-100, and then incubated with rabbit polyclonal anti-α-SMA (1:100 dilution) (Abcam, UK) at 4°C overnight. The cells were then incubated with goat anti-rabbit IgG H&L (Alexa Fluor® 488, 1:200 dilution), after which the cell nuclei were stained with DAPI (Beyotime, Shanghai, China). Finally, the images were observed and analyzed with a Nikon TI-E fluorescence microscope.
Statistical Analysis
The data in the experiments were present as mean ± SD. The comparison of the results was evaluated by GraphPad Prism program (Graphpad Software, Inc., San Diego, CA, USA) with one-way analysis of variance (ANOVA) and Tukey's multiple comparison tests. Statistical significance between groups was considered with a p < 0.05. The difference analyses were detected by MarkerView™ and t-test. The original data were imported into MarkerView™ and statistical analysis via t-test. The t-test was employed to identify significant differences among processed products. Here, p < 0.05 was considered a significant difference, and a t > 0 indicated an increase in ingredient contents.
Results and Discussion
Evaluation of C. officinalis (R) on Anti-fibrosis
The cytotoxicity of C. officinalis (R) on HSC-T6 and HK-2 cells were evaluation by CCK8, which is a widely method in the detection of cytotoxicity and drug sensitivity. In this study, HSC-T6 and HK-2 cells were treated with different doses of C. officinalis (0–10 mg/ml) for 24, 48, and 72 h. Results showed that C. officinalis (R) at a dose range of 0–1 mg/ml had little cytotoxicity on HSC-T6, while the sample at a dose of 10 mg/ml significantly inhibited the survival of cells compared with the normal sample at 24, 48, and 72 h. For HK-2 cells, the minimal cytotoxicity of C. officinalis at a dose range of 0–1 mg/ml was observed only at 48 h and was not observed at 24 and 72 h (Figures 1A–C, respectively). Therefore, we chose 48 h for the follow-up experiments.
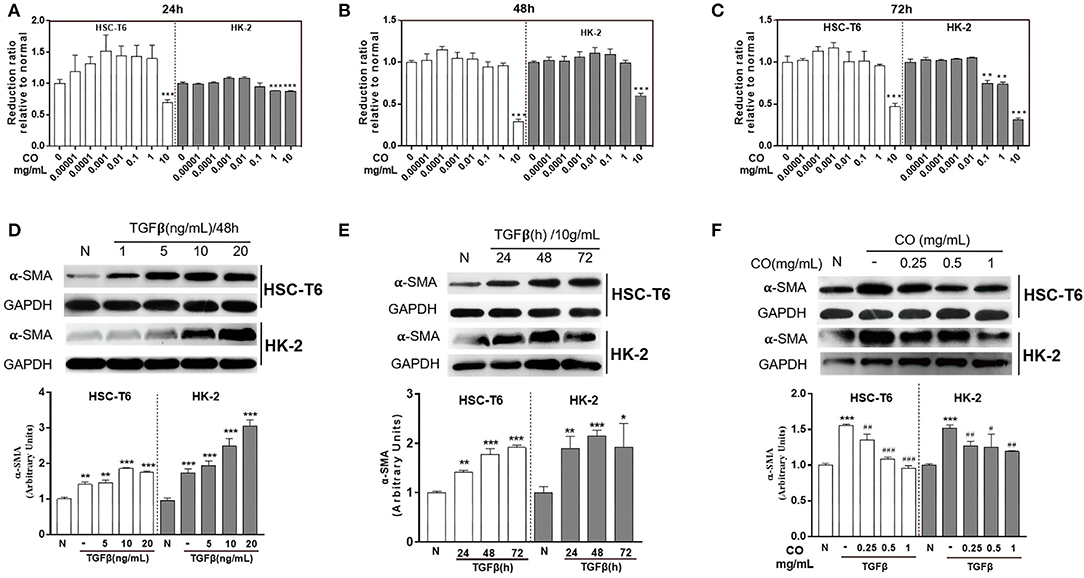
Figure 1. Pharmacodynamic evaluation of C. officinalis. The cytotoxicity of C. officinalis on HSC-T6 and HK2 cells for 24 (A), 48 (B), and 72 h (C). HSC-T6 and HK2 cells were activated by TGF-β with different concentrations (D) and for different times (E). (F) The effects of C. officinalis on activated HSC-T6and HK2 cells. Data are represented as means ± SEM. *p ≤ 0.05, **p ≤ 0.01, and ***p ≤ 0.001 vs. the control group; #p ≤ 0.05, #p ≤ 0.01, and ###p ≤ 0.001 vs. the TGF-β group.
To establish a preferable fibrosis model in vitro, HSC-T6 and HK-2 cells were treated with different concentrations of TGF-β at different times. The results showed that TGF-β at 1, 5, 10, and 20 ng/ml activated HSC-T6 and HK-2 cells, which manifested by the higher expressions of α-SMA compared to the normal group (Figure 1D), moreover, TGF-β at different times 24, 48, and 72 h also up-regulated the expressions of α-SMA in HSC-T6 and HK-2 cells (Figure 1E). On comparing the results, TGF-β (10 ng/ml) at 48 h was used to activate the cells. Moreover, Figure 1D showed that C. officinalis (R) at different doses (0.25, 0.5, and 1 mg/ml) inhibited the expressions of α-SMA increased by TGF-β in HSC-T6 and HK-2 cells, with the best effect identified at a dose of 1 mg/ml (Figure 1F).
In TCM, C. officinalis was often used in replenishing the liver and kidney due to its tonic effect (1). Furthermore, modern pharmacology indicated that C. officinalis showed low toxicity on cells only at high concentrations (19), as confirmed by the results of CCK8. The present study also provided a theoretical basis for further research on liver- and kidney-related diseases. In liver and kidney fibrosis, persistent or dysregulated fibrogenic reactions may hamper regeneration and promote dysfunction (20), which could ultimately raise susceptibility to organ failure and death (21). During these processes, TGF-β contributes to a fibrogenic phenotype by activating fibroblasts cells (20), including HSC-T6 and HK-2 cells. Taking all these elements into account, the present study aimed to develop a therapeutic implementation of anti-TGF-β approaches. The results showed that C. officinalis down-regulated the TGF-β-induced expression of α-SMA and provided a direction for us to optimize the processing technology of C. officinalis with higher anti-fibrotic activity.
Effects of Processed C. officinalis on Anti-fibrosis Changes With Univariate Elements
The processing methods of C. officinalis found in the Pharmacopeia of the People's Republic of China (ChP) including wine-steamed (WS) and wine-braised processing. To identify and optimize the processing technology with the best anti-fibrosis activity, single-factor experiment was used in this study. The results showed that C. officinalis steamed at different times (1, 2, 4, 6, and 8 h) or temperatures (100, 105, 110, 115, 120, and 125°C) inhibited the expressions of α-SMA induced by TGF-β both in HSC-T6 and HK-2 cells. Among these, for HSCs cells, the anti-liver fibrosis activity of C. officinalis steamed for 4 h at 100°C was the best, while steamed at a temperature of 105°C for 1 h had the best anti-fibrosis effects on HK-2 cells (Figures 2A,B, respectively). Furthermore, braised C. officinalis for 0.5, 1, 2, and 4 h inhibited TGF-β-induced over-expressions of α-SMA in HSC-T6 and HK-2 cells, especially at 1 h in HSC-T6 and 4 h in HK2 cells (Figure 2C). C. officinalis processed with different dosages of rice wine (w/w) also showed different degrees of anti-fibrosis activities, especially 15% in HSC-T6 and 25% in HK-2 cells (Figure 2D).
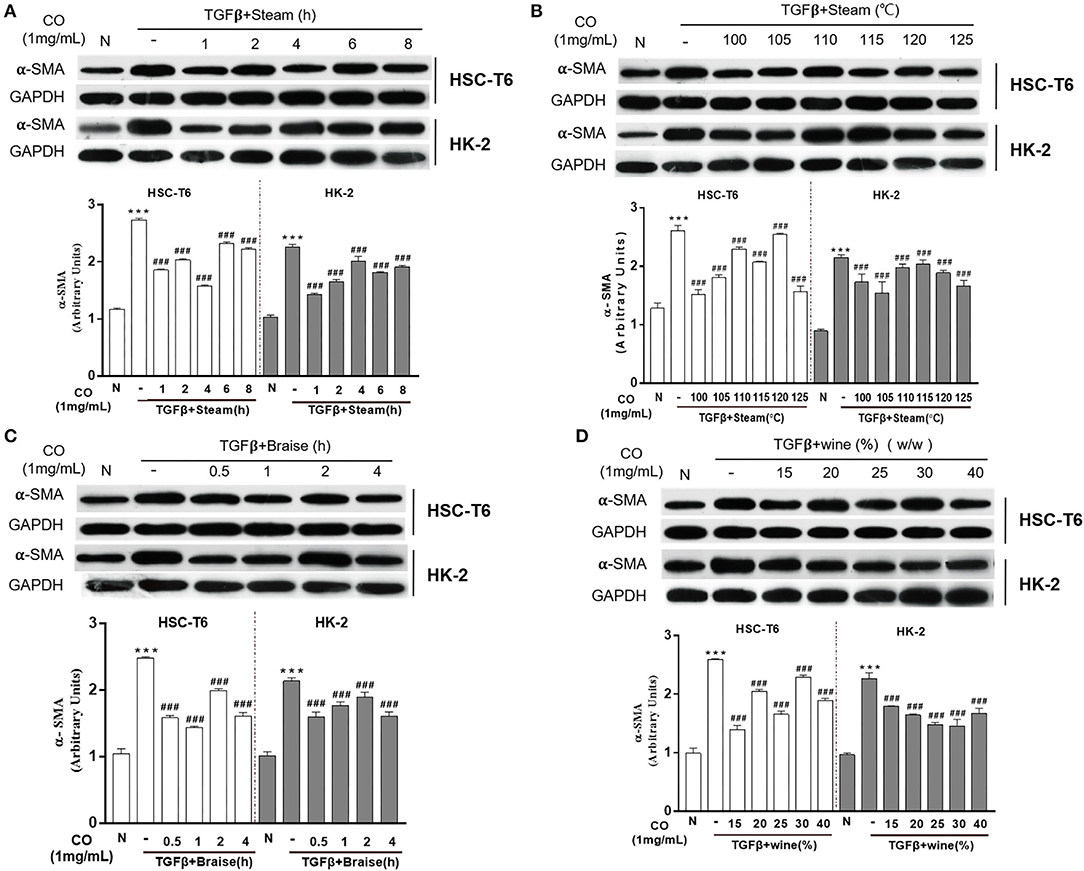
Figure 2. Effects of processed C. officinalis on anti-fibrosis changes with univariate elements. Anti-fibrosis activities of the C. officinalis samples steamed at different times (A), different temperatures (B), braised at different times (C), and with different rice wine dosages (w/w) (D). Data are represented as means ± SEM. ***p ≤ 0.001 vs. the control group. ###p < 0.01 vs. the TGF-β group.
Wined C. officinalis is a traditional and common processing method that applied until now. However, different indexes of evaluation are accompanied by varying wine processing techniques. Thus, this research aimed to explore the suitable wined technology of C. officinalis based on the anti-fibrosis synergistic effects produced by each technique. The results showed that the anti-fibrosis effect of C. officinalis was negatively correlated with the expression of α-SMA. Thus, the data were non-negatively analyzed, which are discussed as follows: Xij = max (X1j, X2j, …, Xnj) – Xij/max (X1j, X2j, …, Xnj) – min (X1j, X2j, …, Xnj) + 1; i = 1, 2, …, n, j = 1, 2, …, n). Moreover, the expressions of α-SMA in HSC-T6 and HK-2 cells were used as indicators for comprehensive scoring, each with a weight of 0.5. The composite score was calculated using the following formula: Xij (HK-2) × 0.5 + Xij (HSC-T6) × 0.5. The results showed that C. officinalis processed with the following parameters: steamed time of 1 h, steamed temperatures of 105 and 125°C, braised times of 0.5 and 4 h, and rice wine dosage (w/w) at 25%, respectively, had the best inhibitory effects on activated HSC-T6 and HK-2; thus, laying the foundation for the subsequent orthogonal experiments. However, considering the time benefit, the term of orthogonal experiment was finally determined as follows: The steamed times were 1, 2, and 3 h; steamed temperatures were 105, 115, and 125°C; braised times were 0.5, 1, and 1.5 h; and rice wine dosages (w/w) were 25, 30, and 35%.
Optimization of the Processing Technology With Orthogonal Test
In accordance with the terms of the single-factor experiments, the processing parameters were optimized with an orthogonal L9 (34) test design. Our results showed that C. officinalis processed with three levels of two factors (steamed times and steamed temperatures), as shown in Supplementary Table S1, inhibited the expressions of α-SMA in HSC-T6 and HK-2 induced by TGF-β (Figure 3A). The composite score of the anti-fibrosis effects was calculated with non-negative analysis, and the results indicated that the maximum was 1.92 (Supplementary Table S1). Furthermore, in selecting the better processing term, the values of K and R are shown in Supplementary Table S1, and the variance analysis results are shown in Supplementary Table S2. These results showed that C. officinalis steamed (HPS) showed optimum anti-fibrosis effect. The craft conditions of C. officinalis with WS were also optimized with an orthogonal design (Supplementary Table S3), and its anti-fibrosis effects on both HSC-T6 and HK-2 were evaluated via the expressions of α-SMA induced by TGF-β (Figure 3B). The results indicated that C. officinalis with WS inhibited the activation of myoblasts, which was mainly manifested by the decrease in levels of α-SMA (Figure 3B). Combined with the results of variance analysis (Supplementary Table S4) and the values of K and R in Supplementary Table S3, the optimal processing term with anti-fibrosis effects were as follows: steamed time of 2 h, braised time of 0.5 h, and rice wine dosage (w/w) of 30%. Similarly, steamed HWPS were optimized with orthogonal design (Supplementary Table S5), variance analysis (Supplementary Table S6), and the expression of α-SMA (Figure 3C).
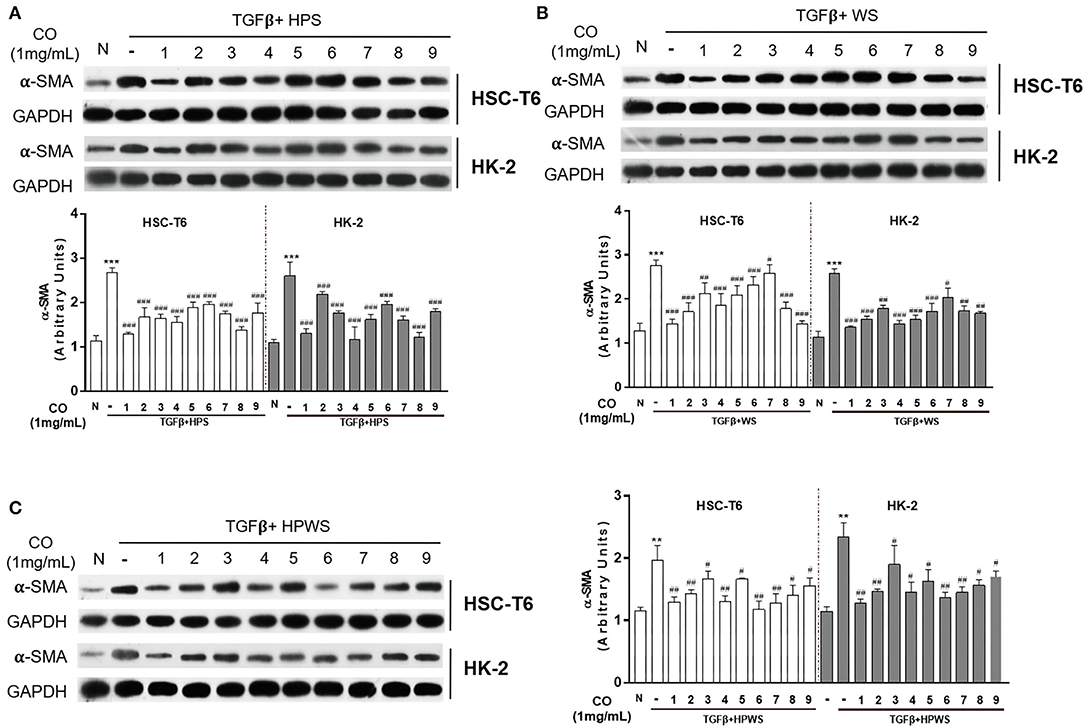
Figure 3. Optimization of the processing technology with orthogonal test. Anti-fibrosis activities of the C. officinalis samples processed with HPS (A), WS (B), and HPWS (C) technologies. Data are represented as means ± SEM. **p ≤ 0.01 and ***p ≤ 0.001 vs. the control group. #p < 0.05, ##p <0.01, and ###p < 0.01 vs. the TGF-β group.
Due to the fact that steamed time, steamed temperature, braised time, and rice wine dosage are vital criteria in the processing of C. officinalis (22), processed C. officinalis using different technologies have varying antidiabetic effects (23). Besides, the results of our study showed that the C. officinalis had different inhibitory effects on activated fibrosis cells. Therefore, optimization of processing criteria is the essential step in screening the technology with the optimal anti-fibrosis effect. Studies have shown that the orthogonal test design is a common method for the optimization of experimental conditions (24, 25). Based on these, the current study used the orthogonal L9 (34) test design to detect the anti-fibrosis of C. officinalis processed with different factors for the single-factor experiments and obtained several artifacts of C. officinalis via S, WS, and HPWS technologies.
Validation of Processed C. officinalis in Terms of Anti-fibrosis Effects
The optimal processing technologies of processed C. officinalis were determined by single-factor plus orthogonal experiments. The processed products of C. officinalis were prepared according to the terms of HPWS and the stipulation in Chp, including WD and WF technology. The expressions of α-SMA were detected by western blot to verify the anti-fibrosis activity of all processed products of C. officinalis. The results showed that C. officinalis (both R and processed products) inhibited the expressions of α-SMA induced by TGF-β in both HSC-T6 and HK-2, especially C. officinalis processed with HPWS (Figure 4A). Then, immunofluorescence assay for α-SMA in HSC-T6 was conducted to confirm the anti-fibrosis effect enhanced by C. officinalis processed with HPWS. The results also showed that C. officinalis inhibited the positive expression of α-SMA (in green) induced by TGF-β, especially the C. officinalis processed with HPWS (Figure 4B).
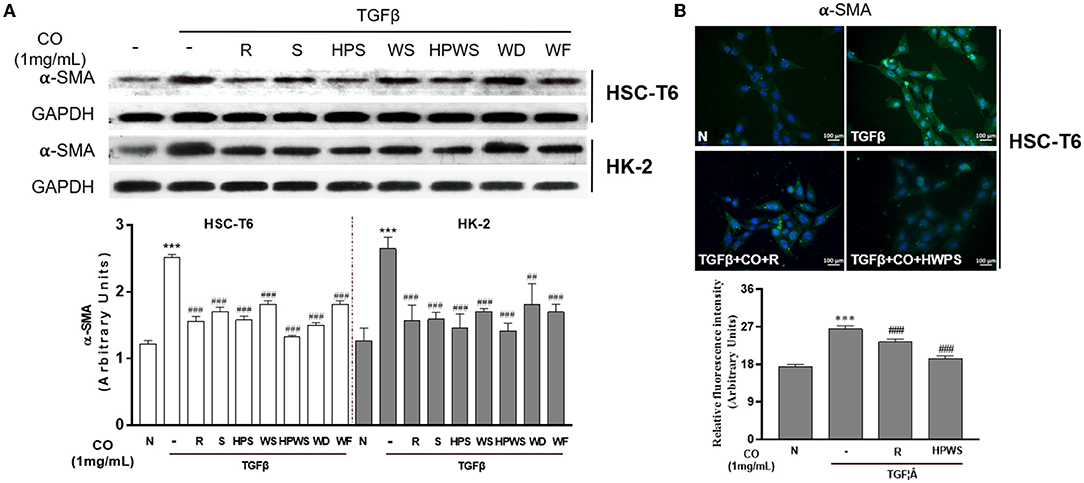
Figure 4. Validation of processed C. officinalis in terms of anti-fibrosis activity. The anti-fibrosis activities of all processed C. officinalis samples were detected by Western blot analysis (A); the effects of immunofluorescent staining of α-SMA in HSC-T6 treated with C. officinalis processed with HPWS present in 400 × magnification (B). Data are represented as means ± SEM. *p ≤ 0.05, **p ≤ 0.01, and ***p ≤ 0.001 vs. the control group. #p < 0.05, ##p < 0.01, and ###p < 0.01 vs. the TGF-β group.
Based on the single-factor, orthogonal tests, variance analysis, and the evaluation of anti-fibrosis activity, C. officinalis processed with HPWS showed better anti-fibrosis activity than other processed products of C. officinalis. The differences in the anti-fibrosis activities of C. officinalis are mainly due to various active components in the C. officinalis (26), which are possible to transform with qualitative and quantitative changes during processing. Therefore, exploring changes of composition in C. officinalis processed with HPWS can provide material basis for further clarifying the mechanism of C. officinalis with HPWS-enhanced anti-hepatic fibrosis.
Ingredient Identification of C. officinalis Processed With HPWS
The analysis of the compounds in C. officinalis (both R and HPWS) were identified by ESI positive and negative modes (Figure 5). The chemical name, molecular mass, molecular formula, and molecular structure of components in C. officinalis were retrieved and download from the database, after which the accurate mass-to-charge ratio of plasma morphology were calculated in both ESI positive and negative modes. The raw data were imported into the PeakView™ software. All the chemical components were encoded and a new session was established under the XIC Manager template. Then, the first-level data matching was conducted with reference standards, standard mass spectrometric database, and literature according to m/z. The chromatographic peak with the retention time error within 0.2 min and the m/z error within 10 ppm was identified as a unified compound. Further, the identification validation and chromatographic peak attribution were based on molecular structures and secondary fragments of compounds.
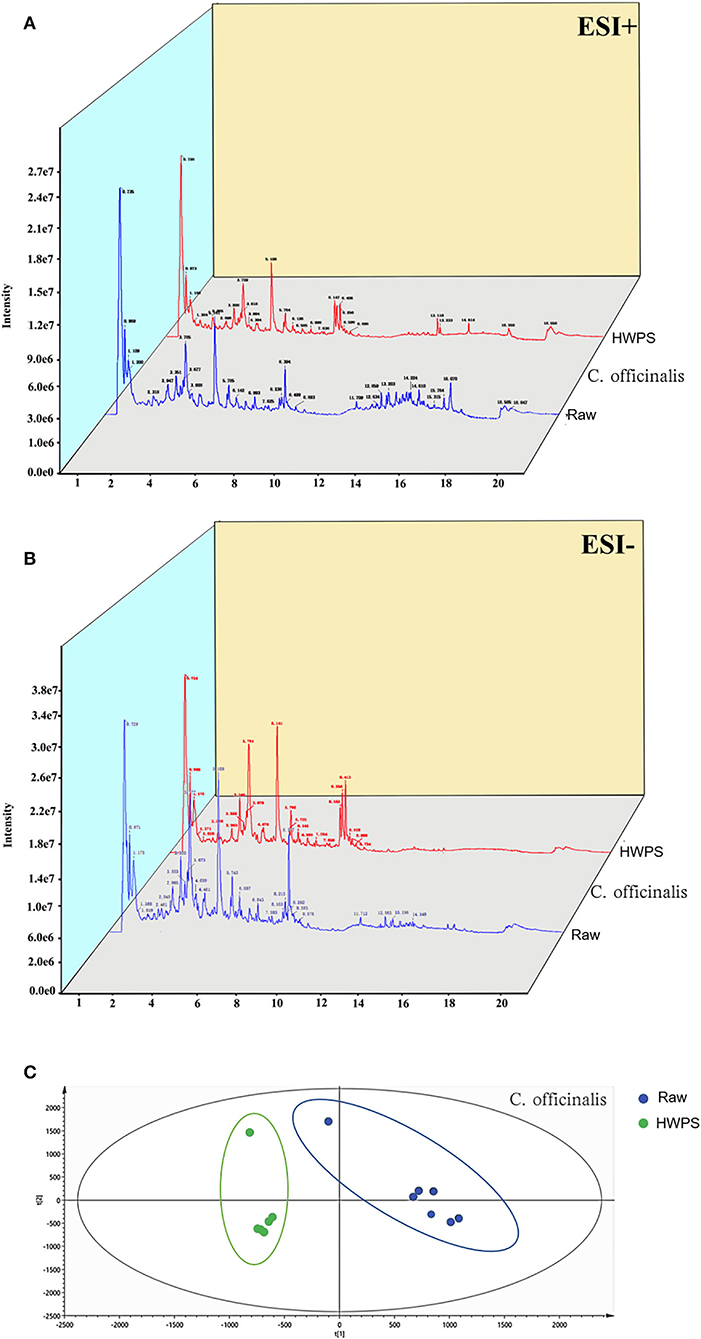
Figure 5. Ingredient identification of C. officinalis processed with HPWS. (A) Raw sample positive and negative ion pattern diagram. (B) The product's positive and negative ion pattern is illustrated. (C) PLS-DA score plot.
As shown in Table 1, 27 components in C. officinalis were changed with HPWS, including flavonoids, iridoid glycosides, and organic acids. Moreover, 5-HMF was a typical emerging compound in C. officinalis with HPWS. We take quercetin as an example to illustrate the identification procedure: [M – H]− of peak 11 was 301.0354, formula was calculated as C15H10O7 with mass (Da) 302.0427, the main secondary fragment was 121.0305, and neutral loss was 180.0019. After consulting the literature (27) and comparing the results with standards, we confirmed that the compound is quercetin.
To further excavate the differences in C. officinalis between R and HPWS products, the data were standardized with SIMCA 14.1 (version, country) and analyzed via PLS-DA under supervised recognition mode. The results in Figure 5 show the PLS-DA score plot of C. officinalis before and after processing. As can be seen, C. officinalis processed with R and HPWS s were obviously clustered into two categories, indicating that the processing has changed the chemical composition in C. officinalis.
The current results also showed that along with HPWS processing the chemical composition in C. officinalis changed qualitatively, including 5-HMF, linoelaidic acid, and quercetin; 5-HMF prevents L02 hepatocytes from injury induced by GalN/TNF-α (28) and attenuates liver fibrosis by inhibiting oxidative stress in mice (29). Furthermore, quercetin can protect the liver and kidney, as reported in another study (30). Thus, this research could reveal a chemical basis for the enhanced anti-fibrosis activity of C. officinalis processed with HPWS.
Conclusions
As a kind of medicine and edible herbal, C. officinalis has been shown to suppress liver and kidney fibrosis by inhibiting the activation of HSC-T6 and HK-2 cells. Studies have also shown that its anti-fibrosis activity can change with processing. Based on this information, our experiment used enhanced anti-fibrosis effects as the indicator to optimize the processing technology of C. officinalis through single-factor and orthogonal tests. Finally, we identified the processing that can produce optimal anti-fibrosis activity, namely, C. officinalis with HPWS, whose chemical composition was identified by UHPLC-Q-TOF-MS/MS analysis. The experimental result is a further step. The results of this study also provided material basis for further exploring the role of C. officinalis in liver and kidney protection.
Data Availability Statement
The original contributions presented in the study are included in the article/Supplementary Material, further inquiries can be directed to the corresponding author/s.
Author Contributions
GC and PX contributed to the design of the study, acquisition of data, and analysis and interpretation of the data. XH, CD, and YN contributed to the acquisition of data and analysis and interpretation of data. All authors participated in drafting or revising the manuscript and approved the final version of the manuscript for submission.
Funding
This work was financially supported by the National Natural Science Foundation of China (Nos. 81973481, 81922073, and 8210142131), the Traditional Chinese Medicine Key Scientific Research Fund Project of Zhejiang Province (No. 2018ZY004), and Zhejiang Province Traditional Chinese Medicine Science and Technology Program (2022ZQ033).
Conflict of Interest
The authors declare that the research was conducted in the absence of any commercial or financial relationships that could be construed as a potential conflict of interest.
Publisher's Note
All claims expressed in this article are solely those of the authors and do not necessarily represent those of their affiliated organizations, or those of the publisher, the editors and the reviewers. Any product that may be evaluated in this article, or claim that may be made by its manufacturer, is not guaranteed or endorsed by the publisher.
Supplementary Material
The Supplementary Material for this article can be found online at: https://www.frontiersin.org/articles/10.3389/fnut.2022.807071/full#supplementary-material
Supplementary Figure 1. Anti-fibrotic activity of main ingredients in C. officinalis processed on HSC-T6.
Supplementary Table S1. C. officinalis with HPS.
Supplementary Table S2. Variance analysis of C. officinalis with HPS.
Supplementary Table S3. C. officinalis with WS.
Supplementary Table S4. Variance analysis of C. officinalis with WS.
Supplementary Table S5. C. officinalis with HPWS.
Supplementary Table S6. Variance analysis of C. officinalis with HPWS.
Abbreviations
TCM, traditional Chinese medicine; 5-HMF, 5-hydroxymethylfurfural; HUVECs, human umbilical vein endothelial cells; ECM, extracellular matrix; α-SMA, alpha smooth muscle actin; HK-2, human proximal tubular epithelial cell; TIF, tubulointerstitial fibrosis; HSC-T6, hepatic stellate cells; EMT, epithelial mesenchymal transition; TGF-β, transforming growth factor β; UHPLC-Q-TOF-MS/MS, ultra-high performance liquid chromatography coupled with hybrid triple quadrupole time-of-flight mass spectrometry; ChP, Pharmacopeia of the People's Republic of China; HPS, high-pressure steamed; HPWS, high-pressure wine steamed.
References
1. Huang J, Zhang Y, Dong L, Gao Q, Yin L, Quan H, et al. Ethnopharmacology, phytochemistry, and pharmacology of Cornus officinalis Sieb. et Zucc. J Ethnopharmacol. (2018) 213:280–301. doi: 10.1016/j.jep.2017.11.010
2. Gao X, Liu Y, An Z, Ni J. Active components and pharmacological effects of Cornus officinalis: literature review. Front Pharmacol. (2021) 12:633447. doi: 10.3389/fphar.2021.633447
3. Quah Y, Lee SJ, Lee EB, Birhanu BT, Ali MS, Abbas MA, et al. Cornus officinalis ethanolic extract with potential anti-allergic, anti-inflammatory, and antioxidant activities. Nutrients. (2020) 12:3317. doi: 10.3390/nu12113317
4. Hu J, Zhou J, Wu J, Chen Q, Du W, Fu F, et al. Loganin ameliorates cartilage degeneration and osteoarthritis development in an osteoarthritis mouse model through inhibition of NF-κB activity and pyroptosis in chondrocytes. J Ethnopharmacol. (2020) 247:112261. doi: 10.1016/j.jep.2019.112261
5. Yu H, Yao S, Zhou C, Fu F, Luo H, Du W, et al. Morroniside attenuates apoptosis and pyroptosis of chondrocytes and ameliorates osteoarthritic development by inhibiting NF-kappaB signaling. J Ethnopharmacol. (2021) 266:113447. doi: 10.1016/j.jep.2020.113447
6. Cao G, Cai H, Cai B, Tu S. Effect of 5-hydroxymethylfurfural derived from processed Cornus officinalis on the prevention of high glucose-induced oxidative stress in human umbilical vein endothelial cells and its mechanism. Food Chem. (2013) 140:273–9. doi: 10.1016/j.foodchem.2012.11.143
7. Parola M, Pinzani M. Pathophysiology of organ and tissue fibrosis. Mol Aspects Med. (2019) 65:1. doi: 10.1016/j.mam.2019.02.001
8. Friedman SL. Hepatic stellate cells: protean, multifunctional, and enigmatic cells of the liver. Physiol Rev. (2008) 88:125–72. doi: 10.1152/physrev.00013.2007
9. Higashi T, Friedman SL, Hoshida Y. Hepatic stellate cells as key target in liver fibrosis. Adv Drug Deliv Rev. (2017) 121:27–42. doi: 10.1016/j.addr.2017.05.007
10. Puche JE, Saiman Y, Friedman SL. Hepatic stellate cells and liver fibrosis. Compr Physiol. (2013) 3:1473–92. doi: 10.1002/cphy.c120035
11. Bataller R, Brenner DA. Liver fibrosis. J Clin Invest. (2005) 115:209–18. doi: 10.5152/tjg.2018.17330
12. Zhang Y, Li K, Li Y, Zhao W, Wang L, Chen Z, et al. Profibrotic mechanisms of DPP8 and DPP9 highly expressed in the proximal renal tubule epithelial cells. Pharmacol Res. (2021) 169:105630. doi: 10.1016/j.phrs.2021.105630
13. Iwano M, Plieth D, Danoff TM, Xue C, Okada H, Neilson EG. Evidence that fibroblasts derive from epithelium during tissue fibrosis. J Clin Invest. (2002) 110:341–50. doi: 10.1172/JCI1551
14. Loeffler I, Wolf G. Epithelial-to-mesenchymal transition in diabetic nephropathy: fact or fiction? Cells. (2015) 4:631–52. doi: 10.3390/cells4040631
15. Meng XM, Tang PM, Li J, Lan HY. TGF-β/Smad signaling in renal fibrosis. Front Physiol. (2015) 6:82. doi: 10.3389/fphys.2015.00082
16. Weiskirchen R, Weiskirchen S, Tacke F. Organ and tissue fibrosis: molecular signals, cellular mechanisms and translational implications. Mol Aspects Med. (2019) 65:2–15. doi: 10.1016/j.mam.2018.06.003
17. Duan H, Wang GC, Khan GJ, Su XH, Guo SL, Niu YM, et al. Identification and characterization of potential antioxidant components in Isodon amethystoides (Benth). Hara tea leaves by UPLC-LTQ-Orbitrap-MS. Food Chem Toxicol. (2021) 148:111961. doi: 10.1016/j.fct.2020.111961
18. Duan H, Wang W, Li Y, Jilany Khan G, Chen Y, Shen T, et al. Identification of phytochemicals and antioxidant activity of Premna microphylla Turcz. stem through UPLC-LTQ-Orbitrap-MS. Food Chem. (2022) 373(Pt B):131482. https://doi.org/10.1016/j.foodchem.2021.131482
19. Kao ST, Wang SD, Lin CC, Lin LJ. Jin Gui Shen Qi Wan, a traditional Chinese medicine, alleviated allergic airway hypersensitivity and inflammatory cell infiltration in a chronic asthma mouse model. J Ethnopharmacol. (2018) 227:181–90. doi: 10.1016/j.jep.2018.08.028
20. Frangogiannis N. Transforming growth factor-β in tissue fibrosis. J Exp Med. (2020) 217:e20190103. doi: 10.1084/jem.20190103
21. Eming SA, Wynn TA, Martin P. Inflammation and metabolism in tissue repair and regeneration. Science. (2017) 56:1026–30. doi: 10.1126/science.aam7928
22. Cao G, Cai H, Zhang Y, Cong X, Zhang C, Cai B. Identification of metabolites of crude and processed Fructus Corni in rats by microdialysis sampling coupled with electrospray ionization linear quadrupole ion trap mass spectrometry. J Pharm Biomed Anal. (2011) 56:118–25. doi: 10.1016/j.jpba.2011.04.013
23. Ma W, Wang KJ, Cheng CS, Yan GQ, Lu WL, Ge JF, et al. Bioactive compounds from Cornus officinalis fruits and their effects on diabetic nephropathy. J Ethnopharmacol. (2014) 153:840–5. doi: 10.1016/j.jep.2014.03.051
24. Cai DJ, Shu Q, Xu BQ, Peng LM, He Y. Orthogonal test design for optimization of the extraction of flavonid from the Fructus Gardeniae. Biomed Environ Sci. (2011) 24:688–93. doi: 10.3967/0895–3988.2011.06.015
25. Wei L, Huang X, Huang Z, Zhou Z. Orthogonal test design for optimization of lipid accumulation and lipid property in Nannochloropsis oculata for biodiesel production. Bioresour Technol. (2013) 147:534–8. doi: 10.1016/j.biortech.2013.08.079
26. Dong Y, Feng ZL, Chen HB, Wang FS, Lu JH. Corni Fructus: a review of chemical constituents and pharmacological activities. Chin Med. (2018) 13:34. doi: 10.1186/s13020–018-0191-z
27. Cai H, Cao G, Cai B. Rapid simultaneous identification and determination of the multiple compounds in crude Fructus Corni and its processed products by HPLC-MS/MS with multiple reaction monitoring mode. Pharm Biol. (2013) 51:273–8. doi: 10.3109/13880209.2012.720689
28. Jiang ZQ, Ma YX, Li MH, Zhan XQ, Zhang X, Wang MY. 5-Hydroxymethylfurfural protects against ER stress-induced apoptosis in GalN/TNF-α-injured L02 hepatocytes through regulating the PERK-eIF2α signaling pathway. Chin J Nat Med. (2015) 13:896–905. doi: 10.1016/S1875–5364(15)30095–9
29. Han XY, Hu JN, Wang Z, Wei SN, Zheng SW, Wang YP, et al. 5-HMF attenuates liver fibrosis in CCl4-plus-alcohol-induced mice by suppression of oxidative stress. J Nutr Sci Vitaminol. (2017) 63:35–43. doi: 10.3177/jnsv.63.35
Keywords: Cornus officinalis, fibrosis, wine steamed, processing technology, composition difference
Citation: Han X, Ding C, Ning Y, Shan Q, Niu M, Cai H, Xu P and Cao G (2022) Optimizing Processing Technology of Cornus officinalis: Based on Anti-Fibrotic Activity. Front. Nutr. 9:807071. doi: 10.3389/fnut.2022.807071
Received: 01 November 2021; Accepted: 29 March 2022;
Published: 03 May 2022.
Edited by:
Yasmina Sultanbawa, The University of Queensland, AustraliaReviewed by:
Kefeng Zhai, Suzhou University, ChinaTao Su, Guangzhou University of Chinese Medicine, China
Yanling Wu, Yanbian University, China
Copyright © 2022 Han, Ding, Ning, Shan, Niu, Cai, Xu and Cao. This is an open-access article distributed under the terms of the Creative Commons Attribution License (CC BY). The use, distribution or reproduction in other forums is permitted, provided the original author(s) and the copyright owner(s) are credited and that the original publication in this journal is cited, in accordance with accepted academic practice. No use, distribution or reproduction is permitted which does not comply with these terms.
*Correspondence: Gang Cao, Y2FvZ2FuZzMzQDE2My5jb20=; Peng Xu, NjAweHVwQDE2My5jb20=
†These authors have contributed equally to this work