- 1School of Biological Sciences, The University of Hong Kong, Pokfulam, Hong Kong, SAR China
- 2Institute of Public Health and Clinical Nutrition, University of Eastern Finland, Kuopio, Finland
- 3Department of Environmental Health, Finnish Institute for Health and Welfare, Kuopio, Finland
Probiotic bacteria have potential use as immunomodulators but comparative data on their immunological effects are very limited. The aim of this study was to characterize the effect of oral administration of probiotic strains, alone or as mixtures, on systemic and organ-specific immune responses. For this purpose, healthy C57BL/6 mice were perorally administered probiotics for 3 weeks. A total of five common probiotic strains, Lactobacillus rhamnosus species GG (LGG) and LC705, Bifidobacterium breve 99 (Bb99), Propionibacterium freudenreichii Shermanii JS (PJS), and Escherichia coli Nissle 1917 (EcN), and two of their mixtures, were tested. Livers, spleens, and blood were collected for investigation. A number of five treatments increased the abundance of the natural killer (NK) cells. Bb99 had the most prominent effect on hepatic NK cells (20.0 ± 1.8%). LGG (liver: 5.8 ± 1.0%; spleen: 1.6 ± 0.4%), Bb99 (liver: 13.9 ± 4.3%; spleen: 10.3 ± 3.7%), and EcN (liver: 8.5 ± 3.2%; spleen: 1.0 ± 0.2%) increased the percentage of both the hepatic and splenic T-helper 17 cells. Moreover, LGG (85.5 ± 3.0%) and EcN (89.6 ± 1.2%) increased the percentage of splenic regulatory T-cells. The tested mixtures of the probiotics had different immunological effects from their individual components on cell-mediated responses and cytokine production. In conclusion, our results confirm that the immunomodulatory potential of the probiotics is strain- and organ/tissue-specific, and the effects of probiotic mixtures cannot be predicted based on their single constituents.
Introduction
Probiotic bacteria are living micro-organisms that confer health benefits on hosts upon consistent and sufficient administration (1–3). Probiotics have been shown to have moderate ameliorating or preventing effects in several diseases, including infectious- and antibiotic-associated diarrhea (4–6), childhood infections (7, 8), enterocolitis (9), inflammatory bowel diseases (10, 11), allergic diseases (12–15), and depression (16). The variety is mind-boggling but in line with the broad impact of gut-associated host-microbe interactions on the immunological, physiological, and anatomical phenotypes of the hosts (17, 18). Many of the observed clinical effects of the probiotics may be due to their immunological effects such as activating inflammation (19–22), antibody production (23, 24), or normalizing polarized helper T-cell responses (25, 26). Yet, the utilization of probiotics as immunomodulatory agents or adjunct immunotherapy is far from its potential (27–29).
One largely neglected area of the research is the immunotherapeutic potential and safety of the probiotics in hepatic conditions. The researchers may have overlooked the plausibility that the probiotics, their metabolites, or antigenic components may have direct access to the liver from the gut via the portal vein. Indeed, there is an indication that specific probiotics may affect natural killer (NK) and natural killer T (NKT)-cell populations in the liver (30) but less is known about their influence on the hepatic T-cell subpopulations.
The selection of probiotics for clinical trials is often arbitrary and the same strains are studied, almost as a custom, in multiple diseases even with opposing immunological etiologies. This shows underappreciation of the long-known strain specificity of the immunological effects of the probiotics (31, 32). There is also a shortage in comparative profiling of the immunological effects of different probiotic strains, which limits the possibility for evidence-based selection strains for clinical intervention trials.
A further complication to the selection process is brought by the increasing interest in using mixtures of probiotic strains over single-strain preparation. This interest is perhaps primarily driven by intuition based on the well-documented association of reduced gut microbial diversity with lower colonization resistance and a variety of disease states (17, 33, 34). However, regarding the efficacy of the probiotics which are allogenic microbes, this view is likely to be too simplistic, especially regarding the immunomodulatory properties. We hypothesize that the immunomodulatory effects of a mixture of probiotics are not directly derivable from the effects of its single-strain constituents and for specific immunostimulatory effects. The single strains might have a greater potential than the mixtures. This study aims to address these issues and to present a comparative analysis of the selected probiotics and their mixtures on systemic and liver-immune profiles in healthy C57BL/6 mice on a normal diet.
Materials and Methods
Animals
The male C57BL/6 mice aged 6 week old (n = 5–11) were assimilated for 1 week before the experiment. They were kept in plastic cages in an air-conditioned room and given free access to food and water. All procedures were performed according to the national legislation on animal experimentation and approved by the Committee of Animal Experiments in the County Administrative Board (the State Provincial Office of Finland, Hämeenlinna, Finland, permit #0703276).
Probiotic Strains and Feeding Procedures
Lactobacillus rhamnosus GG (LGG) (1.7 × 1012 colony-forming units (CFU)/g), Propionibacterium freudenreichii ssp. shermanii JS (PJS) (2.2 × 1012 CFU/g), Lactobacillus rhamnosus LC705 (LC705) (2 × 1011 CFU/g), and Bifidobacterium breve 99 (Bb99) (4.2 × 1011 CFU/g) were provided by Valio Ltd (Helsinki, Finland) while Escherichia coli Nissle 1917 (EcN) (1 × 1011 CFU/g) was provided by Ardeypharm GmbH, Germany. These strains were selected as (1) they represent a heterogeneous array of different bacterial genera including the representatives of the most common genera, Lactobacillus and Bifidobacterium; (2) the gram-negative cell wall of an Escherichia strain would be expected to act via different receptors than the gram-positive strains; and (3) a Propionibacterium strain could have a special immunomodulatory potential due to its very high genomic GC content (>67%) (35, 36). To address the strain specificity, two closely related strains, namely LGG and LC705 were included in the study. A known difference between these two strains is the better mucosal adherence capacity of LGG. We also studied two combinations of these probiotics: a previously studied mixture (37) of LGG, PJS, LC705, and Bb99 (referred to here on as GGmix), and a novel mixture of PJS and EcN (referred to here on as ECPJSmix) comprising the representatives of the less typical probiotic genera.
The suspensions with probiotic bacteria were freshly prepared each day by weighing a defined amount of freeze-dried powders with a known concentration (as confirmed by serial dilution plate culturing) and suspending that in sterile saline. The probiotic mixtures were prepared by mixing equal amounts of the freshly prepared single-probiotic suspensions. The mice were administered with a total dose of 107 CFU of probiotic bacteria in 150–200 μl of saline (6–8 ml/kg) per day by a syringe fitted with an oral gavage needle (75-7202, Scanbur A/S, Karlslunde, Denmark) for 3 consecutive weeks by experienced animal laboratory personnel. Based on a body surface area normalization method, the dose corresponds to approximately 2–3 × 109 CFU of probiotic bacteria per day for an adult human (38). The control group mice were only administered sterile saline.
A total of 71 mice were used in the experiment, of which 11 mice were excluded (ncontrol: 11; nLGG: 7; nLC705: 10; nPJS: 5; nBb99: 5; nEcN: 6; nGGmix: 11; nECPJSmix: 5) due to visibly poor compliance to the gavaging procedure resulting in visible stress and difficult or impossible dosing, declining health including signs of dehydration and weight loss, and in some cases, esophageal damage (confirmed by autopsy). These dosing-procedure-associated side effects did not differ significantly between the treatment groups and the control groups.
Hepatocytes and Splenocytes
The mice were sacrificed by cervical dislocation. The liver and splenic lymphocytes were isolated as done previously with minor modifications (31, 32). In brief, livers and spleens were homogenized and washed two times with the RPMI-1640 medium (300 × g for 10 min at 4°C). The OptiPrepTM working solution (OPWS)-40% iodixanol (Sigma Chemical, St. Louis, Mo., USA) was added to the liver pellets and then layered with Hank's balanced salt solution (Sigma) while the red blood cell lysing buffer (Sigma R7757) was added to the spleen pellets. The pellets were then washed at least two times with the RMPI-1640 medium (300 × g for 7 min at 4°C) and stained with anti-mouse antibodies, including CD3-Alexa 700, CD4-APC/Cy7 or CD4-FITC, CD8-PE/Cy7, NK1.1-PerCP/Cy5.5, CD25-PE, IFNγ-Alexa Fluor 488, IL4-PE, IL17-Alexa Fluor 647, and FOXP3-APC (all from eBioscience). The staining procedures and analyses were performed according to the manufacturer's protocol. The samples were analyzed by the FACSCalibur flow cytometer (BD Biosciences) as previously described (39).
Blood Cytokines
The blood samples for the serum analyses were collected from the submandibular vein by the Microvette CB 300 capillary tubes according to the manufacturer's instructions (Sartsedt AG & Co, Nümbrecht, Germany) before the mice were sacrificed. The serum levels of 22 cytokines and chemokines were measured using the Mouse Cytokine/Chemokine Premixed Lincoplex kit (Cat.# MCYTO-70K-PMX; Millipore Billerica, MA) with standard protocol as previously described (40).
Statistical Analyses
Data were presented as mean ± SEM. Kruskal–Wallis test and Mann–Whitney test were used to evaluate the significant differences between the probiotic treatment groups in reference to the control groups. Statistical significance of the observed differences was evaluated at 10% (*) and 5% (**) significance levels without adjustment for multiple comparisons and at a 5% significance level with Bonferroni adjustment (***). Statistical calculations were performed using SPSS 20 for Windows (Chicago, IL, United States) and GraphPad Software Prism 5.04 (San Diego, CA, United States).
Results
Effects on the Body, Spleen, and Liver Weights
None of the treatments showed significant effects on weight gain during the treatment or the spleen and liver weights after the treatment as compared to the control group (Figure 1).
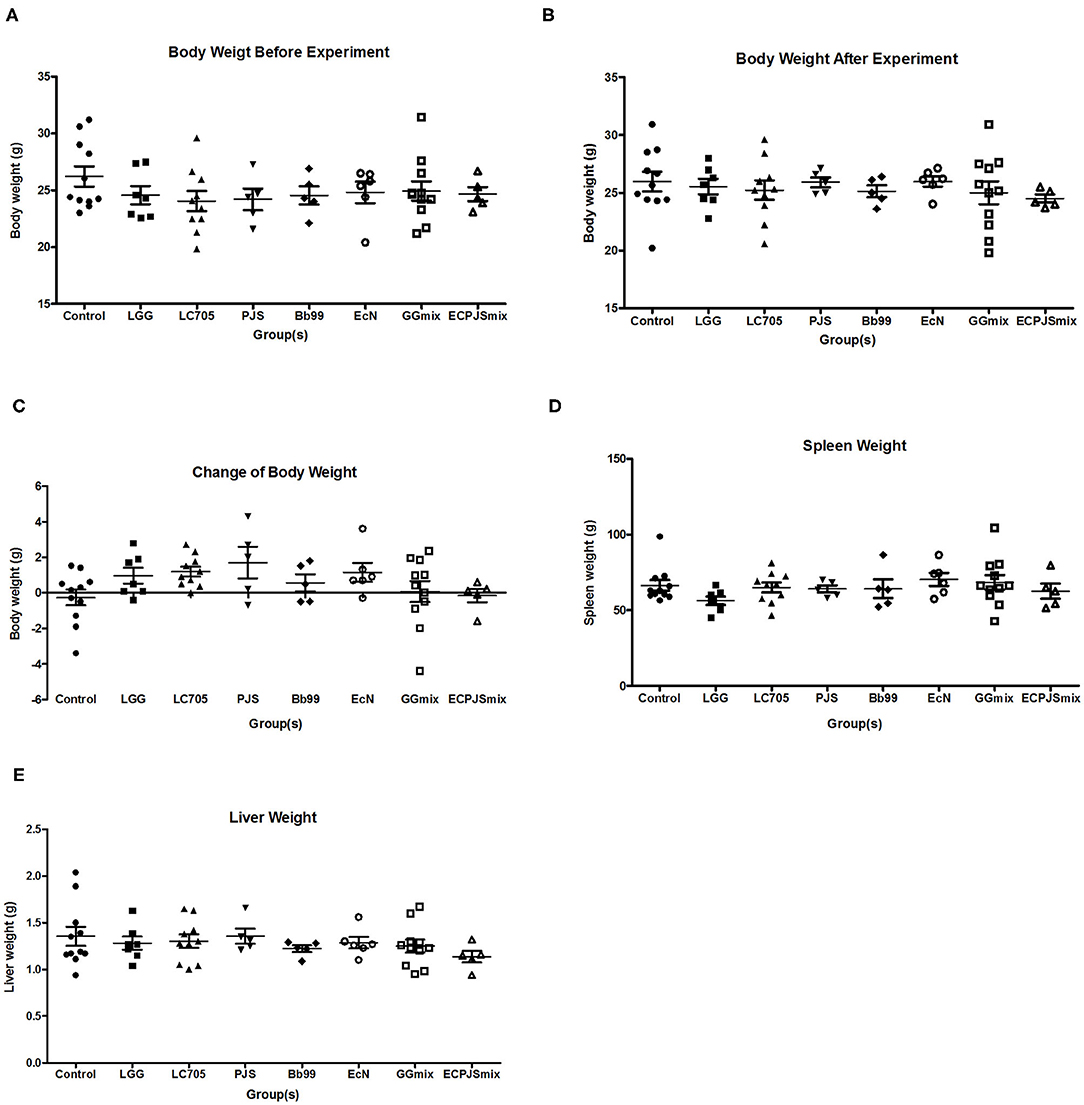
Figure 1. Weight analysis of the body, spleen, and liver of the mice. (A) Body weight of the mice before the experiment, (B) body weight of the mice after the experiment, (C) change of body weight of the mice, (D) spleen weight of the mice, and (E) liver weight of the mice were shown (mean ± SEM, ncontrol: 11; nLGG: 7; nLC705: 10; nPJS: 5; nBb99: 5; nEcN: 6; nGGmix: 11; nECPJSmix: 5). The statistical significance of the observed differences was evaluated and *** indicates the Bonferroni corrected p-value (α = 0.05).
Effects on Hepatic and Splenic NK, NKT, and CD8+ Cells
As shown in Figure 2A, the percentage of NK cells in both the hepatic and splenic lymphocyte populations was higher in the GGmix-treated group than those in the control group. In the ECPJSmix group, the percentage of NK cells was increased in the splenic lymphocyte population only. For the individual strains, the percentages of NK cells in LC705-, Bb99-, and EcN-treated groups were higher than those in the control group in the hepatic lymphocyte population, with the Bb99-treated group showing the most prominent effect. The percentage of NK cells in the LGG-treated group was comparable to the control group. The percentage of NK cells in the PJS-treated group was increased in the hepatic lymphocyte population but decreased in the splenic lymphocyte population. IFN-gamma (IFNg) production, as indicated by mean fluorescence intensity (MFI), of the hepatic NK cells in the ECPJSmix-treated group decreased whereas that of the splenic NK cells in the PJS-treated group increased (Figure 2B).
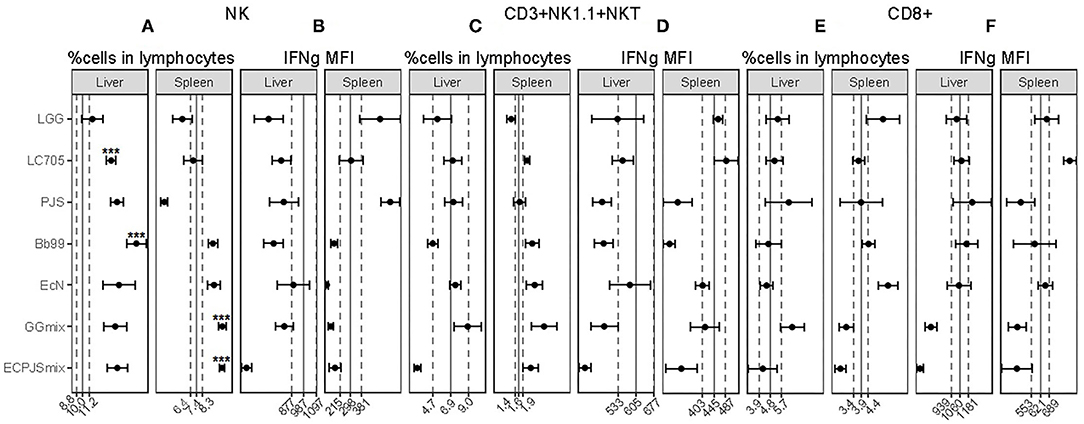
Figure 2. The effects of the probiotics on the NK, CD3+NK1.1+NKT, and CD8+ cell populations. The percentages of (A) NK, (C) CD3+NK1.1+NKT, and (E) CD8+ cells in the lymphocyte population and IFNg mean fluorescence intensity (MFI) within (B) NK, (D) CD3+NK1.1+NKT, and (F) CD8+ cells in the liver and spleen were shown (mean ± SEM, ncontrol: 11; nLGG: 7; nLC705: 10; nPJS: 5; nBb99: 5; nEcN: 6; nGGmix: 11; nECPJSmix: 5). The vertical solid lines indicate the mean value of the respective control groups. The vertical dotted lines indicate the SEM of the respective control groups. Statistical significance of the observed differences was evaluated and *** indicates the Bonferroni corrected p-value (α = 0.05).
The percentage of CD3+NK1.1+NKT cells in the ECPJSmix-treated group was significantly lower than that in the control group in the hepatic lymphocyte population whereas in the LC705- and GGmix-treated groups, the percentage of these cells was increased in the splenic lymphocyte population (Figure 2C). IFNg MFIs of the splenic CD3+NK1.1+NKT cells in the PJS-, Bb99-, and ECPJSmix-treated groups were depressed in comparison with the control group (Figure 2D).
No significant differences were observed in the percentage of the CD8+ cells or the IFNg MFIs of the CD8+ cells in the hepatic lymphocyte population between any of the probiotic-treated groups and the control group. Among the splenic lymphocytes, the percentage of the CD8+ cells was increased in the EcN-treated group (Figure 2E). The IFNg MFI of the splenic CD8+ cells was increased in the LC705-treated group and decreased in the GGmix-treated group in comparison with the control group (Figure 2F).
Effects on the Helper T (TH)-Cells
It seemed that none of the probiotic treatments influenced the TH1/TH2 balance in the liver or spleen (Figure 3A). In contrast, all the treatment groups, except LC705, showed an increase in the percentage of TH17 cells in either or both tested organs when compared with the control group (Figure 3B). Moreover, the percentages of the splenic regulatory T (Treg)-cells in the LGG-treated and EcN-treated groups were higher than those in the control group (Figure 3C). Nonetheless, the splenic Treg/TH17 ratio of the LGG-, Bb99-, and ECPJSmix-treated groups was lower than that in the control group (Figure 3D).
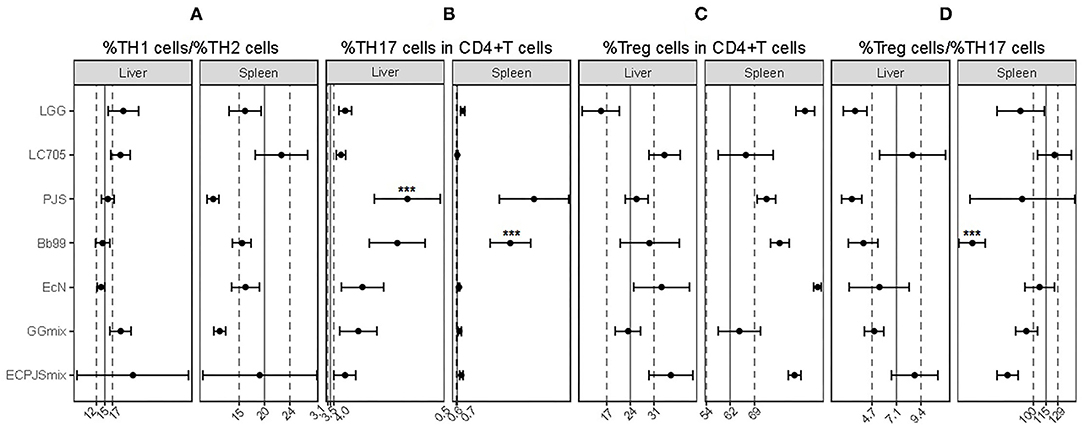
Figure 3. The effects of probiotic treatments on helper T-cell populations. (A) The ratio of %TH1 cells to %TH2 cells; percentages of (B) TH17, and (C) regulatory T (Treg)- cells in all CD4+ T-cells; and (D) the ratio of %Treg cells to %TH17 cells in the liver and spleen were shown (mean ± SEM, ncontrol: 11; nLGG: 7; nLC705: 10; nPJS: 5; nBb99: 5; nEcN: 6; nGGmix: 11; nECPJSmix: 5). The vertical solid lines indicate the mean value of the respective control groups. The vertical dotted lines indicate the SEM of the respective control groups. Statistical significance of the observed differences was evaluated and *** indicates the Bonferroni corrected p-value (α = 0.05).
Effects on Serum Cytokine and Chemokine Levels
The effects of the probiotics on the serum cytokine and chemokine levels are shown in Figure 4. In comparison with the control, lower serum levels of IL-2 were seen in the LGG-, ECPJSmix-, Bb99-, and PJS-treated groups; KC in the LGG-, Bb99-, EcN-, and ECPJSmix-treated groups; IFNγ and IL-17 in the LGG- and ECPJSmix-treated groups; IL-10 in the Bb99- and ECPJSmix-treated groups; IL-6 in the LGG-treated group; and TNFα and IL-13 in the ECPJSmix-treated group. Only the level of IL-6 in the PJS- and GGmix-treated groups was higher than that in the control group.
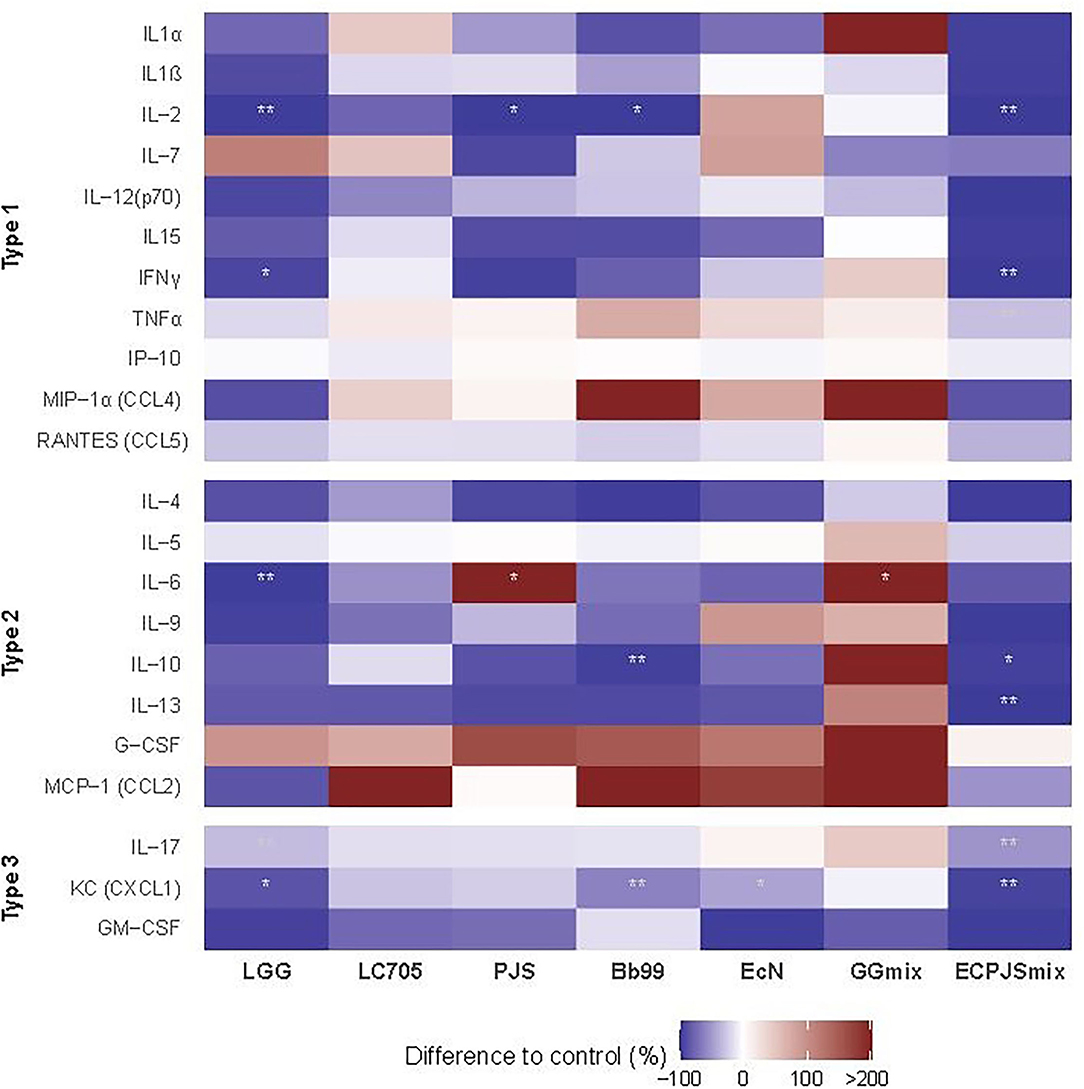
Figure 4. The effects of the probiotics on the serum cytokine and chemokine levels. The cytokine levels presented as percent difference in the geometric mean of the cytokine levels compared to controls (control = 0) in a heat map (ncontrol: 11; nLGG: 7; nLC705: 10; nPJS: 5; nBb99: 5; nEcN: 6; nGGmix: 11; nECPJSmix: 5) with red indicating higher and blue indicating lower mean levels compared to the control. One observation of IL-10 in the GGmix group with mean > + 2SD was excluded. For a rough indication of the nature of the studied cytokines, they are divided into type 1 cytokines, referring to primary involvement in the innate and cell-mediated and TH1-associated immune responses, type 2 cytokines referring to involvement in the humoral and TH2-associated immune responses, and type 3 immunity referring to TH17-mediated immune responses or more than one type of immune response. Statistical significance of the observed differences was evaluated at 10% (*) and 5% (**) significance levels without adjustment for multiple comparisons and at s 5% significance level with Bonferroni adjustment (***). CCL, chemokine C-C motif ligand; CXCL, C-X-C motif chemokine ligand; G-CSF, granulocyte colony-stimulating factor; GM-CSF, granulocyte-macrophage colony-stimulating factor; IFN, interferon; IL, interleukin; IP, interferon-inducible protein; KC, keratinocyte-derived chemokine; MCP, monocyte chemoattractant protein; MIP, macrophage inflammatory protein; RANTES, regulated upon activation, normal T-cell expressed and secreted; TNF, tumor necrosis factor.
Discussion
This study provided several key observations. First, the study confirms that probiotics have the potential to affect the relative abundance and activity of lymphocyte populations in the liver and spleen. Second, this potential varies between different probiotic species and even between closely related strains (LGG vs. LC705). Third, probiotic supplementation may concomitantly increase the pool of a particular lymphocyte population in one organ while decreasing it in another organ. Fourth, the probiotics appeared to increase the relative abundance of the hepatic NK cells and TH17 cells in the liver and spleen in a nearly universal manner. Fifth, LGG and EcN increased the relative abundance of the Treg cells in the spleen. Sixth, the studied probiotics had little influence on the TH1/TH2 balance in this animal model. Seventh, the effects of the two mixtures studied were different from their individual components but tended to be in the same direction with each other.
In the study, LC705, PJS, Bb99, EcN, and GGmix increased the percentage of the NK cells in the liver with Bb99 showing the most prominent effect. The potential of specific probiotics to stimulate the NK cells that are known central anti-viral and anti-tumor cells of the innate immune system is in line with some previous studies. For example, B. adolescentis BBMN23 and B. longum BBMN68 have been shown to increase the NK cell activity in the BALB/c mice (41) and B. longum SP 07/3 has been shown to enhance the NK cell activation and activity in the ex vivo models with human peripheral blood mononuclear cells (PBMCs) (42). Lactobacillus casei Shirota (LcS) have been shown to increase the NK cell activity in the peripheral blood of smokers and patients with biliary cancer surgery (43–45) and to increase the cytotoxicity of the NK cells in the mouse spleen (46). However, in healthy adults, LcS have been shown to depress the lytic activity of the NK cells (44). LGG had little influence on the splenic or hepatic NK cells in our study, while it has been shown to increase the activity of the NK cells in the in vitro model with human PBMCs (47).
LGG, Bb99, and EcN were all shown to increase the percentage of the TH17 cells in both the liver and spleen but the PJS increased the percentage of the TH17 cells in the liver only. The cytokine measurements indicated, however, that in the peripheral blood, the TH17 cell-signature cytokine was decreased by LGG treatment, possibly indicating a lower peripheral activity or drainage of the peripheral pool of the TH17 cell to the other tissues such as the liver and spleen as indicated by the cellular data. LGG has been previously shown to increase the percentage of the TH17 cells when co-cultured with human PBMCs (48). Also, a higher percentage of the TH17 cells was observed in the spleens of LGG groups when compared to that of the control groups in Shi et al.'s study (49). In contrast, the probiotic strains, Enterococcus faecalis FK-23 and Bifidobacterium infantis, have been associated with a reduced TH17-immune-response in different animal models (50, 51). The TH17 cells play an important role in defense against extracellular pathogens (52), and in this regard, their activation in response to probiotic administration is logical. While an increase in the abundance of the TH17 cells could improve host defense against bacterial infection, the chronic effects of long-term consumption of the probiotics should be further investigated in clinical trials as an inappropriate or uncontrolled activation of the TH17 response is linked to proinflammatory and autoimmune diseases (53–56).
Notably, LGG and EcN increased the percentage of the Treg cells in the spleen. Tregs are known as the key immunoregulatory cells in suppressing immune responses and maintaining immunological homeostasis. This is in line with some previous studies. For example, the LGG administration has been associated with increased Treg activity in the murine model with asthma (57) and ovalbumin-immunized rats (58) and EcN treatment with an increase in the intradermal Treg cells in the mouse model of atopic dermatitis (59). Also, in the study of Shi et al., the mice in the low-dose LGG group had a higher percentage of Treg cells in the spleens than those in the control group and high-dose LGG group (49). Previously another Bifidobacterium breve strain has been shown to prevent the decrease in the splenic and intestinal Tregs associated with food allergen sensitization in the mouse model of food allergy without a significant effect in the naïve mice (60). In our study, the Bb99 treatment was associated with a non-significant increase in the percentage of the Treg cells and significantly reduced Treg/TH17 cell ratio in the spleen, indicative of an anti-inflammatory potential for the studied strain.
Only a few associations were observed in our study between the different probiotic treatments and other studied effector cells, namely CD8+ cells and CD3+NK1.1+NKT cells. As cytotoxic cells, CD8+ cells act especially against intracellular pathogens. Thus, it would seem rational that exposure to non-invasive probiotic bacteria would primarily not activate them. NKT cells are important players in the first line of innate defense against bacterial and viral infections and bridge the innate and adaptive immune system. Their activation by probiotics has been suggested to improve high-fat diet-induced hepatic steatosis and insulin resistance (30).
We also investigated the effects of probiotics in cytokine profiles. Interestingly, the closely related Lactobacillus rhamnosus strains, that is, LGG and LC705, had distinctly different effects. The more adherent LGG strain was associated with nearly universal suppression of all cytokine levels while LC705 had less effect. It was also interesting that some of the components of the GGmix particularly, LGG and Bb99, affected some of the cytokine levels, for example, both LGG and Bb99 reduced the level of neutrophil chemoattractant KC, but the mixture did not seem to affect any cytokine levels. This could be partly because of the lower dose of the individual strains in the mixture. However, it could also indicate that exposure to a single bacterial strain elicits a different response than exposure to the bacterial mixture and thus a concomitant encounter of a wider array of different immunogenic signals. Previously, a combination of LGG and LC705 has been shown to be more immunogenic to human monocyte-derived macrophages than the individual strains (61). ECPJSmix was associated with a prominent universal decrease in the cytokine levels compared to the control and as with GGmix, the effect of the mixture was distinct from that of its two individual components. Further studies are called for to investigate the differences in the immunological effects following exposure to individual bacterial strains or their mixture. Overall, there is a shortage of comparable studies on the effects of the probiotics studied here on the peripheral cytokine profile in healthy mice. However, the tendency of the probiotics, LGG, and the ECPJSmix, in particular, to reduce the proinflammatory cytokine levels is in agreement with the potential anti-inflammatory effects associated with LGG and many other probiotics in clinical studies (62–64). In vitro probiotics tend to stimulate the secretion of a wide range of cytokines from the cultured PBMCs, including the proinflammatory cytokines (25). Interestingly in one comparative study of different probiotics, including EcN and LGG, the LGG-derived extracts and cell debris were shown as the weakest inducers of proinflammatory cytokines (65), which is in line with our results.
All the studied individual strains and GGmix have a long history of safe human use and the ECPJSmix combination created for experimental purposes is the only exception. For this reason, a safety assessment of these strains was out of the scope of this study. None of the treatments were seen to influence weight gain or the weight of the studied organs: spleen and liver. However, gavaging per se was associated with visible stress in some of the mice and the effect of this on the results is unknown. The stress would be expected to be equal between the treatment groups and all mice that responded poorly to the procedure were excluded. The number of excluded mice did not significantly differ between the treatment groups and the control group. Nonetheless, while oral gavaging was chosen here to guarantee comparable dosing of the probiotics in each mouse, we strongly recommend against using it in similar experiments in the future where the effects of daily administration of food supplements are addressed.
In conclusion, this study confirms that the immunomodulatory potential of probiotic bacteria is strain- and organ/tissue-specific. Therefore, the immunomodulatory properties of the probiotic candidates should not be extrapolated from the observations obtained with the other strains or even with the same strain in different target organ/tissue. Based on the animal model and the probiotic strains and their combinations in our study, probiotic supplementation would seem to have the most potential to influence NK, TH17 cell-, and Treg cell-mediated immune responses both systemically and in the liver, but little influence on the TH1/TH2 balance. The influence on the phagocytosing cells was not studied here and it should be addressed in future studies. Our study indicated that the immunological effects of the probiotic mixtures can be dramatically different from that of their individual components; and thus, the effects of a probiotic mixture cannot be extrapolated from those of its individual strains. The specific immunological effects may be different with human exposure and different dosages than what was observed here in the mice with a single dose. Therefore, the presented specific immunological effects should be only utilized as supporting evidence and for hypothesis generation. However, for general guidance, our results highlight that the immunomodulation by the probiotic bacteria is strain-specific as well as organ/tissue-specific and that the effects of the probiotic mixtures cannot be predicted based on the effects of their single constituents.
Data Availability Statement
The raw data supporting the conclusions of this article will be made available by the authors, without undue reservation.
Ethics Statement
The animal study was reviewed and approved by Committee of Animal Experiments in the County Administrative Board (The State Provincial Office of Finland, Hämeenlinna, Finland).
Author Contributions
PK designed the study with contributions from HE-N. PK supervised and OM contributed to the laboratory work. Result analyses were primarily carried out by FF with contributions from OM, HE-N, and PK. The manuscript was written by FF and PK with important contributions to the intellectual content from OM and HE-N. All authors contributed to the article and approved the submitted version.
Funding
This study was supported by grants from the Academy of Finland (Number 122015), the Finnish Cultural Foundation (Yrjö Similä Fund), Emil Aaltonen Foundation, and the General Research Fund (GRF) from the Research Grant Council (GRC) of Hong Kong (Number 765810).
Conflict of Interest
The authors declare that the research was conducted in the absence of any commercial or financial relationships that could be construed as a potential conflict of interest.
Publisher's Note
All claims expressed in this article are solely those of the authors and do not necessarily represent those of their affiliated organizations, or those of the publisher, the editors and the reviewers. Any product that may be evaluated in this article, or claim that may be made by its manufacturer, is not guaranteed or endorsed by the publisher.
Acknowledgments
We would like to thank the UEF animal unit personnel for their expert help in animal maintenance, dosing, and sampling and Jaana Hannula (née Isola) for her invaluable help in the laboratory work. We would also like to thank Pauli Tuoresmäki for his invaluable help in drawing the figures.
References
1. Guarner F, Schaafsma GJ. Probiotics. Int J Food Microbiol. (1998) 39:237–8. doi: 10.1016/S0168-1605(97)00136-0
2. FAO/WHO. Joint FAO/WHO Expert Consultation on Evaluation of Health and Nutritional Properties of Probiotics in Food Including Powder Milk with Live Lactic Acid Bacteria. (2001). Cordoba, Argentina
3. Reid G, Sanders ME, Gaskins HR, Gibson GR, Mercenier A, Rastall R, et al. New scientific paradigms for probiotics and prebiotics. J Clin Gastroenterol. (2003) 37:105–18. doi: 10.1097/00004836-200308000-00004
4. Szymański H, Pejcz J, Jawień M, Chmielarczyk A, Strus M, Heczko PB. Treatment of acute infectious diarrhoea in infants and children with a mixture of three Lactobacillus rhamnosus strains—a randomized, double-blind, placebo-controlled trial. Aliment Pharmacol Ther. (2006) 23:247–53. doi: 10.1111/j.1365-2036.2006.02740.x
5. Vanderhoof JA, Mitmesser SH. Probiotics in the management of children with allergy and other disorders of intestinal inflammation. Benef Microbes. (2010) 1:351–6. doi: 10.3920/BM2010.0034
6. Mantegazza C, Molinari P, D'Auria E, Sonnino M, Morelli L, Zuccotti GV. Probiotics and antibiotic-associated diarrhea in children: a review and new evidence on Lactobacillus rhamnosus GG during and after antibiotic treatment. Pharmacol Res. (2018) 128:63–72. doi: 10.1016/j.phrs.2017.08.001
7. Shimizu T, Haruna H, Hisada K, Yamashiro Y. Effects of Lactobacillus gasseri OLL 2716 (LG21) on Helicobacter pylori infection in children. (2002) J Antimicrob Chemother 50:617–8. doi: 10.1093/jac/dkf157
8. Hojsak I, Snovak N, Abdovic S, Szajewska H, Misak Z, Kolacek S. Lactobacillus GG in the prevention of gastrointestinal and respiratory tract infections in children who attend day care centers: a randomized, double-blind, placebo-controlled trial. Clin Nutr. (2010) 29:312–6. doi: 10.1016/j.clnu.2009.09.008
9. El-Sawaf M, Siddiqui S, Mahmoud M, Drongowski R, Teitelbaum DH. Probiotic prophylaxis after pullthrough for Hirschsprung disease to reduce incidence of enterocolitis: a prospective, randomized, double-blind, placebo-controlled, multicenter trial. J Pediatr Surg. (2013) 48:111–7. doi: 10.1016/j.jpedsurg.2012.10.028
10. Van Gossum A, Dewit O, Louis E, de Hertogh G, Baert F, Fontaine F, et al. Multicenter randomized-controlled clinical trial of probiotics (Lactobacillus johnsonii, LA1) on early endoscopic recurrence of Crohn's disease after lleo-caecal resection. Inflamm Bowel Dis. (2007) 13:135–42. doi: 10.1002/ibd.20063
11. Curro D., Ianiro G, Pecere S, Bibbo S, Cammarota G. Probiotics, fibre and herbal medicinal products for functional and inflammatory bowel disorders. Br J Pharmacol. (2017) 174:1426–49. doi: 10.1111/bph.13632
12. Kalliomaki M, Salminen S, Arvilommi H, Kero P, Koskinen P, Isolauri E. Probiotics in primary prevention of atopic disease: a randomised placebo-controlled trial. Lancet. (2001) 357:1076–9. doi: 10.1016/S0140-6736(00)04259-8
13. Pochard P, Gosset P, Grangette C, Andre C, Tonnel AB, Pestel J, et al. Lactic acid bacteria inhibit TH2 cytokine production by mononuclear cells from allergic patients. J Allergy Clin Immunol. (2002) 110:617–23. doi: 10.1067/mai.2002.128528
14. Abrahamsson TR, Jakobsson T, Bottcher MF, Fredrikson M, Jenmalm MC, Bjorksten B, Oldaeus G. Probiotics in prevention of IgE-associated eczema: a double-blind, randomized, placebo-controlled trial. J Allergy Clin Immunol. (2007) 119:1174–80. doi: 10.1016/j.jaci.2007.01.007
15. Wickens K, Barthow C, Mitchell EA, Kang J, van Zyl N, et al. Effects of Lactobacillus rhamnosus HN001 in early life on the cumulative prevalence of allergic disease to 11 years. Pediatr Allergy Immunol. (2018) 29:808–14. doi: 10.1111/pai.12982
16. Rao AV, Bested AC, Beaulne TM, Katzman MA, Iorio C, Berardi JM, Logan AC. A randomized, double-blind, placebo-controlled pilot study of a probiotic in emotional symptoms of chronic fatigue syndrome. Gut Pathog. (2009) 1:6. doi: 10.1186/1757-4749-1-6
17. Round JL, Mazmanian SK. The gut microbiota shapes intestinal immune responses during health and disease. Nat Rev Immunol. (2009) 9:313–23. doi: 10.1038/nri2515
18. Cerf-Bensussan N, Gaboriau-Routhiau V. The immune system and the gut microbiota: friends or foes? Nat Rev Immunol. (2010) 10:735–44. doi: 10.1038/nri2850
19. Dotan I, Rachmilewitz D. Probiotics in inflammatory bowel disease: possible mechanisms of action. Curr Opin Gastroenterol. (2005) 21:426–30. doi: 10.1097/01.mog.0000163168.06081.7d
20. Viljanen M, Pohjavuori E, Haahtela T, Korpela R, Kuitunen M, Sarnesto A, Vaarala O, Savilahti E. Induction of inflammation as a possible mechanism of probiotic effect in atopic eczema-dermatitis syndrome. J Allergy Clin Immunol. (2005) 115:1254–9. doi: 10.1016/j.jaci.2005.03.047
21. Lorea Baroja M, Kirjavainen PV, Hekmat S, Reid G. Anti-inflammatory effects of probiotic yogurt in inflammatory bowel disease patients. Clin Exp Immunol. (2007) 149:470–9. doi: 10.1111/j.1365-2249.2007.03434.x
22. Prescott SL, Bjorksten B. Pobiotics for the prevention or treatment of allergic diseases. J Allergy Clin Immunol. (2007) 120:255–62. doi: 10.1016/j.jaci.2007.04.027
23. Isolauri E, Joensuu J, Suomalainen H, Luomala M, Vesikari T. Improved immunogenicity of oral D x RRV reassortant rotavirus vaccine by Lactobacillus casei GG. Vaccine. (1995) 13:310–2. doi: 10.1016/0264-410X(95)93319-5
24. Kukkonen K, Nieminen T, Poussa T, Savilahti E, Kuitunen M. Effect of probiotics on vaccine antibody responses in infancy—a randomized placebo-controlled double-blind trial. Pediatr Allergy Immunol. (2006) 17:416–21. doi: 10.1111/j.1399-3038.2006.00420.x
25. Pohjavuori E, Viljanen M, Korpela R, Kuitunen M, Tiittanen M, Vaarala O, Savilahti E. Lactobacillus GG effect in increasing IFN-gamma production in infants with cow's milk allergy. J Allergy Clin Immunol. (2004) 114:131–6. doi: 10.1016/j.jaci.2004.03.036
26. Tan M, Zhu JC, Du J, Zhang LM, Yin HH. Effects of probiotics on serum levels of Th1/Th2 cytokine and clinical outcomes in severe traumatic brain-injured patients: a prospective randomized pilot study. Crit Care. (2011) 15:R290. doi: 10.1186/cc10579
27. Jia W, Li H, Zhao L, Nicholson JK. Gut microbiota: a potential new territory for drug targeting. Nat Rev Drug Discov. (2008) 7:123–9. doi: 10.1038/nrd2505
28. Petrovsky N. Immunomodulation with microbial vaccines to prevent type 1 diabetes mellitus. Nat Rev Endocrinol. (2010) 6:131–8. doi: 10.1038/nrendo.2009.273
29. Hancock RE, Nijnik A, Philpott DJ. Modulating immunity as a therapy for bacterial infections. Nat Rev Microbiol. (2012) 10:243–54. doi: 10.1038/nrmicro2745
30. Ma X, Hua J, Li Z. (2008). Probiotics improve high fat diet-induced hepatic steatosis and insulin resistance by increasing hepatic NKT cells. Journal of Hepatology 49:821–830. doi: 10.1016/j.jhep.2008.05.025
31. Kirjavainen PV, ElNezami HS, Salminen SJ, Ahokas JT, Wright PF. Effects of orally administered viable Lactobacillus rhamnosus GG and Propionibacterium freudenreichii subsp shermanii JS on mouse lymphocyte proliferation. Clin Diagn Lab Immunol. (1999) 6:799–802. doi: 10.1128/CDLI.6.6.799-802.1999
32. Kirjavainen PV, El-Nezami HS, Salminen SJ, Ahokas JT, Wright PF. The effect of orally administered viable probiotic and dairy lactobacilli on mouse lymphocyte proliferation. FEMS Immunol Med Microbiol. (1999) 26:131–5. doi: 10.1111/j.1574-695X.1999.tb01380.x
33. Kleerebezem M, Vaughan EE. Probiotic and gut lactobacilli and bifidobacteria: molecular approaches to study diversity and activity. Annu Rev Microbiol. (2009) 63:269–90. doi: 10.1146/annurev.micro.091208.073341
34. Uronis JM, Arthur JC, Keku T, Fodor A, Carroll IM, Cruz ML, et al. Gut microbial diversity is reduced by the probiotic VSL#3 and correlates with decreased TNBS-induced colitis. Inflamm Bowel Dis. (2011). 17:289-297. doi: 10.1002/ibd.21366
35. Ojala T, Laine PKS, Ahlroos T, Tanskanen J, Pitkanen S, Salusjarvi T, Kankainen M, Tynkkynen S, Paulin L, Auvinen P. Functional genomics provides insights into the role of Propionibacterium freudenreichii ssp. shermanii JS in cheese ripening. Int J Food Microbiol. (2017) 241:39–48. doi: 10.1016/j.ijfoodmicro.2016.09.022
36. Kant R, de Vos WM, Palva A Satokari R. Immunostimulatory CpG motifs in the genomes of gut bacteria and their role in human health and disease. J Med Microbiol. (2014) 63:293–308. doi: 10.1099/jmm.0.064220-0
37. Kekkonen RA., Holma R, Hatakka K, Suomalainen T, Poussa T, Adlercreutz H, Korpela R. A probiotic mixture including galactooligosaccharides decreases fecal beta-glucosidase activity but does not affect serum enterolactone concentration in men during a two-week intervention. J Nutr. (2011) 141:870–6. doi: 10.3945/jn.110.137703
38. Reagan-Shaw S, Nihal M, Ahmad N. Dose translation from animal to human studies revisited. FASEB J. (2008) 22:659–61. doi: 10.1096/fj.07-9574LSF
39. Kirjavainen PV, Pautler S, Baroja ML, Anukam K, Crowley K, Carter K, Reid G. Abnormal immunological profile and vaginal microbiota in women prone to urinary tract infections. Clin Vaccine Immunol. (2009) 16:29–36. doi: 10.1128/CVI.00323-08
40. Mykkanen OT, Huotari A, Herzig KH, Dunlop TW, Mykkanen H, Kirjavainen PV. Wild blueberries (Vaccinium myrtillus) alleviate inflammation and hypertension associated with developing obesity in mice fed with a high-fat diet. PLoS ONE. (2014) 9:e114790. doi: 10.1371/journal.pone.0114790
41. Yang HY, Liu SL, Ibrahim SA, Zhao L, Jiang JL, Sun WF, Ren FZ. Oral administration of live Bifidobacterium substrains isolated from healthy centenarians enhanced immune function in BALB/c mice. Nutr Res. (2009) 29:281–9. doi: 10.1016/j.nutres.2009.03.010
42. Genel F, Atlihan F, Gulez N, Kazanci E, Vergin C, Terek DT, Yurdun OC. Evaluation of adhesion molecules CD64, CD11b and CD62L in neutrophils and monocytes of peripheral blood for early diagnosis of neonatal infection. World J Pediatr. (2012) 8:72–5. doi: 10.1007/s12519-011-0304-6
43. Sugawara G, Nagino M, Nishio H, Ebata T, Takagi K, Asahara T, Nomoto K, Nimura Y. Perioperative synbiotic treatment to prevent postoperative infectious complications in biliary cancer surgery: a randomized controlled trial. Ann Surg. 244:706–14. doi: 10.1097/01.sla.0000219039.20924.88
44. Seifert S, Bub A, Franz CM, Watzl B. Probiotic Lactobacillus casei Shirota supplementation does not modulate immunity in healthy men with reduced natural killer cell activity. J Nutr. (2011) 141:978–84. doi: 10.3945/jn.110.136440
45. Reale M, Boscolo P, Bellante V, Tarantelli C, Di Nicola M, Forcella L, Li Q, Morimoto K, Muraro R. Daily intake of Lactobacillus casei Shirota increases natural killer cell activity in smokers. Br J Nutr. (2012) 108:308–14. doi: 10.1017/S0007114511005630
46. Soltan Dallal MM, Yazdi MH, Holakuyee M, Hassan ZM, Abolhassani M, Mahdavi M. Lactobacillus casei ssp.casei induced Th1 cytokine profile and natural killer cells activity in invasive ductal carcinoma bearing mice. Iran J Allergy Asthma Immunol. (2012) 11:183–9.
47. Dong H, Rowland I, Yaqoob P. Comparative effects of six probiotic strains on immune function in vitro. Br J Nutr. (2012) 108:459–70. doi: 10.1017/S0007114511005824
48. Donkor ON, Ravikumar M, Proudfoot O, Day SL, Apostolopoulos V, Paukovics G, et al. Cytokine profile and induction of T helper type 17 and regulatory T cells by human peripheral mononuclear cells after microbial exposure. Clin Exp Immunol. (2012) 167:282–95. doi: 10.1111/j.1365-2249.2011.04496.x
49. Shi CW, Cheng MY, Yang X, Lu YY, Yin HD, Zeng Y, et al. Probiotic Lactobacillus rhamnosus GG promotes mouse gut microbiota diversity and T cell differentiation. Front Microbiol. (2020) 11:607735. doi: 10.3389/fmicb.2020.607735
50. Tanabe S, Kinuta Y, Saito Y. Bifidobacterium infantis suppresses proinflammatory interleukin-17 production in murine splenocytes and dextran sodium sulfate-induced intestinal inflammation. Int J Mol Med. (2008) 22:181–5. Available online at: https://doi.org/10.3892/ijmm_00000006
51. Zhang B, An J, Shimada T, Liu S, Maeyama K. Oral administration of Enterococcus faecalis FK-23 suppresses Th17 cell development and attenuates allergic airway responses in mice. Int J Mol Med. (2012) 30:248–54. doi: 10.3892/ijmm.2012.1010
52. Ishigame H, Kakuta S, Nagai T, Kadoki M, Nambu A, Komiyama Y, et al. Differential roles of interleukin-17A and−17F in host defense against mucoepithelial bacterial infection and allergic responses. Immunity. (2009) 30:108–19. doi: 10.1016/j.immuni.2008.11.009
53. Veldhoen M, Hocking RJ, Flavell RA, Stockinger B. Signals mediated by transforming growth factor-beta initiate autoimmune encephalomyelitis, but chronic inflammation is needed to sustain disease. Nat Immunol. (2006) 7:1151–6. doi: 10.1038/ni1391
54. Ghoreschi K, Laurence A, Yang XP, Tato CM, McGeachy MJ, Konkel JE, et al. Generation of pathogenic T(H)17 cells in the absence of TGF-beta signalling. Nature. (2010) 467:967–71. doi: 10.1038/nature09447
55. Waite JC, Skokos D. Th17 response and inflammatory autoimmune diseases. Int J Inflam. (2012) 2012:819467. doi: 10.1155/2012/819467
56. Raveney BJ, Oki S, Yamamura T. Nuclear Receptor NR4A2 Orchestrates Th17 Cell-Mediated Autoimmune Inflammation via IL-21 Signalling. PLoS ONE. (2013) 8:e56595. doi: 10.1371/journal.pone.0056595
57. Feleszko W, Jaworska J, Rha RD, Steinhausen S, Avagyan A, et al. Probiotic-induced suppression of allergic sensitization and airway inflammation is associated with an increase of T regulatory-dependent mechanisms in a murine model of asthma. Clin Exp Allergy. (2007) 37:498–505. doi: 10.1111/j.1365-2222.2006.02629.x
58. Finamore A, Roselli M, Britti MS, Merendino N, Mengheri E. Lactobacillus rhamnosus GG and Bifidobacterium animalis MB5 induce intestinal but not systemic antigen-specific hyporesponsiveness in ovalbumin-immunized rats. J Nutr. (2012) 142:375–81. doi: 10.3945/jn.111.148924
59. Weise C, Zhu Y, Ernst D, Kuhl AA, Worm M. Oral administration of Escherichia coli Nissle 1917 prevents allergen-induced dermatitis in mice. Exp Dermatol. (2011) 20:805–9. doi: 10.1111/j.1600-0625.2011.01326.x
60. Zhang LL, Chen X, Zheng PY, Luo Y, Lu GF, Liu ZQ, et al. Oral Bifidobacterium modulates intestinal immune inflammation in mice with food allergy. J Gastroenterol Hepato. (2010) 25:928–34. doi: 10.1111/j.1440-1746.2009.06193.x
61. Lehtoranta L, Pitkaranta A, Korpela R. The effects of probiotic combination Lactobacillus rhamnosus GG and Lactobacillus rhamnosus LC705 in cytokine and chemokine response in human macrophages. Int J Probiotics Prebiotics. (2011) 7:17–22.
62. Plaza-Diaz J, Ruiz-Ojeda FJ, Vilchez-Padial LM, Gil A. Evidence of the anti-inflammatory effects of probiotics and synbiotics in intestinal chronic diseases. Nutrients. (2017) 9:555. doi: 10.3390/nu9060555
63. Pagnini C, Corleto VD, Martorelli M, Lanini C, D'Ambra G, Di Giulio E, et al. Mucosal adhesion and anti-inflammatory effects of Lactobacillus rhamnosus GG in the human colonic mucosa: a proof-of-concept study. World J Gastroenterol. (2018) 24:4652–62. doi: 10.3748/wjg.v24.i41.4652
64. Zhao W, Peng C, Sakandar HA, Kwok LY, Zhang W. Meta-analysis: randomized trials of Lactobacillus plantarum on immune regulation over the last decades. Front Immunol. (2021) 12:643420. doi: 10.3389/fimmu.2021.643420
Keywords: probiotic bacteria, immunomodulation, functional foods, Lactobacillus rhamnosus, Bifidobacterium breve, Propionibacterium freudenreichii ssp. shermanii JS, Escherichia coli Nissle 1917
Citation: Fong FLY, El-Nezami H, Mykkänen O and Kirjavainen PV (2022) The Effects of Single Strains and Mixtures of Probiotic Bacteria on Immune Profile in Liver, Spleen, and Peripheral Blood. Front. Nutr. 9:773298. doi: 10.3389/fnut.2022.773298
Received: 09 September 2021; Accepted: 04 March 2022;
Published: 12 April 2022.
Edited by:
Periyar Selvam Sellamuthu, SRM Institute of Science and Technology, IndiaReviewed by:
Xianbin Zhang, Shenzhen University, ChinaReshma B. Nambiar, Zhejiang University, China
Copyright © 2022 Fong, El-Nezami, Mykkänen and Kirjavainen. This is an open-access article distributed under the terms of the Creative Commons Attribution License (CC BY). The use, distribution or reproduction in other forums is permitted, provided the original author(s) and the copyright owner(s) are credited and that the original publication in this journal is cited, in accordance with accepted academic practice. No use, distribution or reproduction is permitted which does not comply with these terms.
*Correspondence: Hani El-Nezami, elnezami@hku.hk