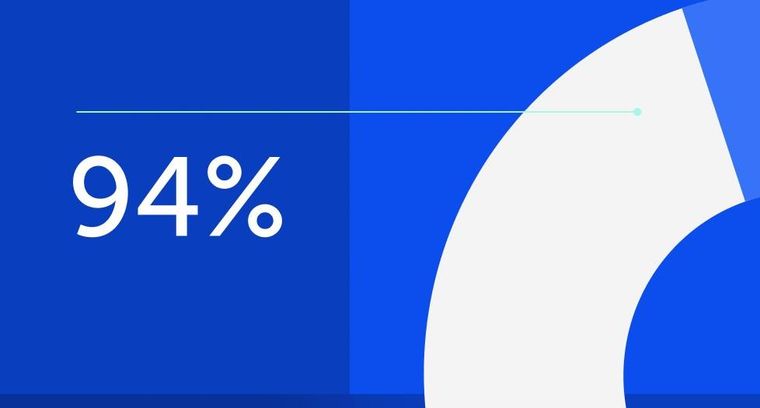
94% of researchers rate our articles as excellent or good
Learn more about the work of our research integrity team to safeguard the quality of each article we publish.
Find out more
ORIGINAL RESEARCH article
Front. Nutr., 11 January 2023
Sec. Nutrition and Food Science Technology
Volume 9 - 2022 | https://doi.org/10.3389/fnut.2022.1088128
This article is part of the Research TopicRising Stars in Nutrition and Food Science Technology: Innovative Research on Nutritional Marine FoodsView all 9 articles
In the present study, changes in volatile compounds during processing were analyzed using the headspace-gas chromatography-ion mobility spectrometry (HS-GC-IMS), to investigate the generation of aroma in hairtails (Trichiurus lepturus) during air-drying. Physicochemical indices, such as moisture content and thiobarbituric acid reactive substances (TBARS), were also detected. Flavor fingerprints were studied and developed to distinguish the samples of fresh hairtails (0 day) from air-dried hairtails (2 and 4 days). A total of 75 volatile organic compounds (VOCs) were identified in hairtails, in which alcohols, aldehydes, ketones, and esters were the principal contributors to the formation of the overall flavor of hairtails during air-drying. Seven flavor compounds (ethanol, 3-methyl-1-butanol, 1-pentanol, hexanal, octanal, benzaldehyde, and 3-methylbutanal), two flavor compounds (acetoin and dimethyl sulfide), and eight flavor compounds (1-hexanol, 1-octen-3-ol, nonanal, heptanal, 2-heptanone, ethyl acetate, trimethylamine, and ammonia) were identified in 0, 2, and 4 air-dried hairtails as biomarkers, respectively. The results showed that HS-GC-IMS could detect VOCs in different air-dried hairtails rapidly and comprehensively.
Hairtail (Trichiurus lepturus) is one of the most economic marine fish in China and is mainly distributed in the Eastern Pacific Ocean (1). Air-dried hairtails are one of the most popularly consumed dried seafoods in coastal regions and are of high nutritional value. Compared with the taste and texture of fish products, the volatile flavors of hairtails produce distinctive quality characteristics and have an enormous impact on the evaluation of the freshness and nutritional value of foods (2). Non-enzymatic reactions taking place in the formation of flavor compounds during food processing include Maillard reactions, caramelization reactions, oxidative and thermal degradation of lipids, and degradation of proteins and vitamins. The Maillard reaction, lipid degradation, and a combination of both these reactions are particularly important in flavor formation during processing (3). Most of the flavor components in dry-cured meat products are formed by fat oxidation (4, 5). In addition, the majority of these substances are unstable and form other stable substances. Moreover, chemical reactions mediated by enzymes and microorganisms continue to form unfavorable volatile compounds, in turn affecting product quality (6, 7). Recently, Ding et al. (8) described physicochemical indices and flavor profiles in traditional deep-fried and circulating air-fried hairtails based on headspace-gas chromatography-ion mobility spectrometry (HS-GC-IMS). Significant differences in flavor composition have been reported in traditional deep-fried hairtails compared with circulating air-fried hairtails. Silver carp (Hypophthalmichthys molitrix) is a highly nutritious fish from China, India, and Mexico. A recent study compared the lipid oxidation, flavor, and content of fat in silver carp using different drying methods (9). Leduc et al. (10) identified thiophene, hexanal, 1-octen-3-one, dimethyltrisulfide, and 1-nonen-3-ol as potential markers of quality in European seabass (Dicentrarchus labrax). However, studies on air-dried hairtails are limited, and changes in hairtail flavor during air-drying have not been investigated to date. Therefore, a simple method to recognize compounds and changes in the flavors of hairtails during the air-drying process is necessary.
The HS-GC-IMS is a gas-phase separation technology that relies on gas chromatography to preseparate samples, and then, the compounds are characterized subsequently based on gas-phase ion mobility (11). IMS is an easy-to-use technique that is inexpensive, shows ultrahigh sensitivity, and does not require time-consuming pretreatment of samples (12). IMS has been used in various areas such as environmental analysis (13), medical analysis (14), and food flavor analysis (12). Wang et al. (15) used GC-IMS to analyze volatile components and flavor fingerprints of samples from Jingyuan lambs of different ages, and they found that alcohols, ketones, aldehydes, esters, and thiazoles show high-intensity peaks in spectrometry. Jia et al. (6) identified volatile organic compounds (VOCs) in chill-stored silver carp by GC-IMS and evaluated changes in VOCs that are induced by three predominant bacteria in chill-stored silver carp after 14 days of storage. Yao et al. (16) investigated the flavor profile of five different regional Chinese smoked chickens through GC-IMS and identified 34 flavor compounds, with a majority of them consisting of heptanal, n-nonanal, furfurol, and hexanal. Nie et al. (17) reported that aldehydes are the principal contributors to the formation of the overall flavor of fermented sea bass based on GC-IMS and gas chromatography-mass spectrometry (GC-MS) analysis.
The present study aimed to identify VOCs in hairtails during the air-drying process using HS-GC-IMS. Principal component analysis (PCA) was performed to assess the efficiency of HS-GC-IMS in the detection and identification of hairtail samples during the air-drying process. The present study also intended to analyze the flavor characteristics of hairtails during the air-drying process to provide theoretical support to processing and quality control in the production of air-dried hairtails.
Nine fresh individual fish samples belonging to hairtail (T. lepturus) species weighing 300–400 g and having a length of 50–60 cm were purchased from a local fish market in Zhoushan, Zhejiang Province, China. Hairtail samples were immersed in flaked ice in a foam box and transported to the laboratory within 30 min. Upon arrival, the fish samples were taken out from the foam box and prepared manually by removing the gills, the viscera, the tail, and the black membrane in the abdominal cavity but they were not sectioned nor the intramuscular bone removed. Another six hairtail individuals were salted with 2% (w/w) sodium chloride (NaCl) and then air-dried under outdoor natural conditions (temperature: 4–7°C, relative humidity: 40–60%) and wind speed: 2–6 m/s). The muscle tissue located below the abdominal region (6.0 × 5.0 × 1.0-cm) from each hairtail was collected for analysis.
The moisture content of hairtails during the air-drying process was measured according to the method of Ma et al. (18) with minor modifications. Briefly, 2 g of minced samples was placed in a dry container and dried at 105°C. The moisture content of the samples was calculated using the following formula: Moisture content (%) = (M1 – M2)/M1*100%, where M1 and M2 signify sample weights before and after drying, respectively. The TBARS content of the samples was measured, as described by Wang et al. (19) with minor modifications. Five grams of muscle and 50 mL of 7.5% (v/v) trichloroacetic acid (TCA) solution were added into a centrifuge tube for homogenization in ice for 30 s, followed by centrifugation for 5 min (10,000 × g, 4°C). The supernatant solution (5 mL) and thiobarbituric acid (TBA) solution (0.02 mol/L, 5 mL) were mixed and boiled for 30 min. Finally, the solution was cooled in ice water for 20 min, and the absorbance was measured at a wavelength of 532 nm. The results were expressed as milligrams (mg) of malondialdehyde (MDA) per kilogram (kg) (mg MDA/kg) of muscle.
Changes in the volatile compounds of hairtails were investigated using HS-GC-IMS (FlavourSpec®, G.A.S., Dortmund, Germany) with a wax capillary column (30 m × 0.53 mm × 1 μm) (Restek, USA). Two grams of fish muscle was transferred to a 20-mL headspace vial and incubated at 60°C for 20 min. Then, a headspace-gas sample of 500 μL was automatically drawn by a syringe needle (85°C) and injected into the GC injector (column temperature, 60°C; drift tube temperature, 45°C). High-purity nitrogen was employed as sample gas at a flow rate of 150 mL/min. The carrier gas flow rate was set to 2 mL/min for the first 2 min, at which point the carrier gas flow rate was increased to 10 mL/min for 10 min, to 100 mL/min for 20 min, to 100 mL/min for a 30 min, and then the flow was stopped. The retention index (RI) was calculated with reference to n-ketone C4–C9 (Sinopharm Chemical Reagent Beijing Co., Ltd., China). The VOCs were identified by comparing the RI and the drift time of the standard in the GC-IMS library.
All tests were repeated three times. The HS-GC-IMS chromatograms were analyzed by HS-GC-IMS Library Search, Reporter Gallery plot, Dynamic PCA, and Laboratory Analytical Viewer (LAV) processing software (FlavourSpec®, G.A.S., Dortmund, Germany). The data were processed further by one-way analysis of variance (ANOVA) using SPSS 21.0 software (SPSS Inc., Chicago, IL, USA), with the significance level set as a P-value of < 0.05. Correlation analysis among volatile compounds was performed using Origin 2021 software (Origin-Lab Corporation, Northampton, MA, USA).
The moisture content decreased significantly from 76.69% in raw fish to 61.92% in the final product (P < 0.05) with prolongation of air-drying duration (Figure 1A). The results indicated that the air-drying time and salt-drying stage were the major factors that led to water loss in hairtails. Some studies indicated that the generation of ester and 1-octen-3-ol is correlated to a decrease in moisture content (20, 21), which is similar to the present results. Lipid oxidation is recognized as a biochemical reaction that is strongly associated with the production of odor (22). The TBARS values represent the content of aldehydes and ketones that stem from lipid degradation (23). Moreover, the TBARS values increased significantly (P < 0.05) with prolongation of air-dried time (Figure 1B), which showed that lipid oxidation occurred rapidly in the hairtail muscle. This feature could be ascribed to the generation of several unstable peroxides by the oxidation and decomposition of unsaturated fatty acids. The peroxide compounds were then converted further into aldehydes, ketones, alcohols, and other shorter-chain hydrocarbons (24, 25). However, the moisture content and TBARS values of hairtails may also be affected by the penetration of salt.
Figure 1. The moisture content (A) and thiobarbituric acid reactive substance (TBARS) values (B) in hairtails during the air-drying process. Different letters mean each substrate's significant difference (P < 0.05).
The samples of fresh hairtails (0 d), hairtails air-dried for 2 days (2 d), and hairtails air-dried for four days (4 d) were analyzed by GC-IMS to obtain information on volatile compounds (Figure 2). The x-axis, y-axis, and z-axis, respectively, represent the drift time, the gas chromatography retention time, and the signal intensity of ionic compounds. In Figure 2, each point represents a volatile compound, and one compound may have two or three spots representing dimers or multimers (26). These results indicated that the signal peak intensities differ, and the content of volatile compounds also varied among samples.
To distinguish differences among volatile compounds in hairtails during the air-drying process, the three-dimensional (3D) topographic plots in Figure 2 were converted into two-dimensional (2D) spectra plots (Figure 3). Differences in air-dried hairtail samples were compared using the difference comparison model. The 2D spectra plot of the 0-day hairtail was used as the reference, and the topographic plots of the other two hairtail samples were obtained from the reference. If the volatile components in different samples were consistent with each other, then the background after subtracting was white, the blue corresponds to the low peak intensity of volatile compounds, and the red to the higher peak intensity of volatile compounds. The results showed that numerous signal peaks appeared in the retention time of 200–800 s and the drift time of 1.0–1.5 s. Compared with 0-day hairtail samples, the 2-day hairtail samples have more blue spots within the range of 200–500 s retention time, indicating that most signal peaks of volatile compounds decreased during early air-drying, and the 4-day hairtail samples have more red spots within the range of 400–1,000 s retention time, indicating that most signal peaks of volatile compounds increased during the later stage of air-drying.
The VOCs of hairtail samples were confirmed, according to the drift time, retention time, and retention index of VOCs, and these parameters were then compared with similar parameters cited in the IMS database. Each number represents one VOC, and the results of the identification of each VOC are shown in Table 1. The concentrations of some single VOCs were different and thus produced multiple signals. Ninety signal peaks and 75 VOCs were identified in three hairtail samples compared with the GC-IMS library, including 22 species of alcohols, 21 species of aldehydes, 11 species of ketones, 7 species of esters, 4 species of hydrocarbons, 3 species of nitrogen-bearing, 2 species of pyrazine, 2 species of acid, 1 species of furan, 1 species of sulfur-bearing, and 1 species of pyridine. The intensities of (Z)-2-penten-1-ol, cis-4-heptenal, 2-butanol, 2-propanol, ethyl acetate, isobutyl acetate, butyl 2-methylbutanoate, ammonia-M, ammonia-D, and (E)-2-heptenal increased with air-drying time. The intensities of 3-methyl-1-butanol-M, 1-penten-3-ol-D, 2-methyl-1-propanol-M, 1-propanol-M, 3-pentanone-M, 3-pentanone-D, ethanol-M, ethanol-D, 3-methylbutanal, diethyl acetate, and acetone decreased with air-drying time. The compounds, such as acetoin, 1-pentanol, 3-methyl-1-butanol, heptanal, 1-penten-3-ol, 1-butanol, (E)-2-pentenal, 2-methyl-1-propanol, hexanal, 1-propanol, 1-penten-3-one, 3-pentanone, ethanol, and ammonia, have double peaks, which may be attributable to the presence of monomers and their dimers.
Table 1. Results of the qualitative analysis of volatile flavor compounds in hairtails during air-drying.
To visualize changes in VOCs during the air-drying processes of hairtails, the VOCs were analyzed qualitatively (Figure 4). Each row represents all signal peaks of a sample, and each column represents a single volatile compound of different samples. Its color shows the strength of signal peaks in the hairtail samples, and the brighter the color, the greater the strength. Figure 4 shows that the VOCs were similar among various air-dried hairtail samples, but the brightness of each spot showed obvious changes, and the peak intensity was relatively different, indicating that the content of VOCs during the air-drying process was distinct among hairtail samples. The content of acetic acid, benzaldehyde, (E)-2-hexenal, octanal, hexanal, 3-methylbutanal, butanal, 2-methylpropanal, acrolein, diethyl acetate, ethyl heptanoate, isoamyl acetate, 2-ethyl-3,5-dimethylpyrazine, styrene, 3-methyl-1-butanol-M, 1-penten-3-ol-D, 2-methyl-1-propanol-M, hexanal-D, 1-propanol-M, 3-pentanone, ethanol, acetone, toluene, and 28 other VOCs in the 0-day hairtail samples was higher than those of the other two samples, indicating that the mentioned compounds could turn out to be important VOCs in fresh hairtails. The content of most of the VOCs was lowest in the 2-day hairtail samples, with only acetoin and dimethyl sulfide markedly higher than the other two groups, indicating that these compounds play a crucial role in the flavor of the 2-day hairtail samples. The content of nonanal, (E)-2-octenal, (E,E)-2,4-heptadienal, cis-4-heptenal, (E)-2-heptenal, linalool, 1-octen-3-ol, 1-hexanol, (Z)-2-penten-1-ol, 1-butanol-D, 2-butanol, 2-propanol, ethyl acetate, ethyl butanoate, isobutyl acetate, butyl 2-methylbutanoate, trimethylamine, ammonia, and 30 other VOCs in the 4-day hairtail samples increased with air-drying time.
According to the results of GC-IMS, alcohols are the first largest VOCs' component in hairtails. Alcohols are mainly formed by lipid oxidation, Maillard reaction, and reduction of the carbonyl compound. Moreover, the threshold of alcohols is very high and contributes less to the formation of overall flavor; these compounds include ethanol, 1-butanol, 1-propanol, and other short straight-chain alcohols. When the concentration of ethanol is higher, it generates herb, xylon, and fat odor (7). The content of ethanol decreased during the air-drying process, as ethanol is a highly volatile compound and is a part of the ethanol participate esterification reaction to form flavor. A previous study suggested that ethanol could be used as a substrate for ester synthesis in fish products (27). Some long straight-chain alcohols have relatively low threshold values and significantly impact hairtail flavors such as 1-pentanol, 1-hexanol, 1-octen-3-ol, and 3-methyl-1-butanol (7). The Strecker degradation of leucine or isoleucine leads to the formation of 3-methyl-1-butanol, which imparts a malty aroma sensation that contributes to the overall aroma of hairtail (28). The oxidation of oleic acid produces 1-pentanol, which also contributes to the green and woody aroma (29). Both compounds accumulated mainly in the 0-day hairtail. Alcohols, such as 1-hexanol, has a rosin and flower aroma, and 1-octen-3-ol has a mushroom aroma, and all these aromas are mainly present in the 4-day hairtail samples. Compounds 1-hexanol and 1-octen-3-ol are formed from the oxidation of oleic acid (C18:1n9c) or palmitoleic acid (C16:1) (30) and C18:3n3 or C18:2n6 (31). A previous study showed that the content of 1-octen-3-ol is highly correlated to TBARS values and thus could reflect the oxidation levels of fat in fish (32). The signal peak of 1-octen-3-ol was enhanced with air-drying time, indicating that the level of lipid oxidation in hairtail gradually increased during the air-drying process, which confirmed our earlier results.
Aldehydes are important VOCs in meat and meat products that present with a smell of fat, green, paint, faint scent, and fruit and are the second largest VOC component in hairtails (33, 34). Aldehydes are generated from the oxidative degradation of unsaturated fatty acids and the Strecker degradation reaction of amino acids (35). Due to the low odor threshold, these aldehydes contribute largely to the flavor of food (11). Previous studies detected nonanal, hexanal, octanal, and heptanal in salted dry fish products and were verified to be important VOCs (36, 37), which agreed with the results of the current study. Nonanal and octanal were produced by the oxidation of oleic acid. Nonanal has a gassy and fatty odor, while octanal generates a fruity and fatty taste (15). The oxidation of linoleic acid and arachidonic acid produces hexanal (fragrance of apple, leaf, and delicate) and heptanal (flavor of nutty and fruity green) (38, 39), respectively. The signal peaks of hexanal and octanal were higher in the 0-day hairtail samples, and the signal peaks of nonanal and heptanal were higher in the 4-day hairtail samples, giving the hairtails their distinctive flavor. Benzaldehyde (flavor of almond bitterness) is formed from the Strecker degradation of phenylalanine and highly accumulated in 0 d hairtails (40). Another compound with a low odor threshold, 3-methylbutanal, gives the hairtail a fruity and cheese aroma (41), which is formed by the Strecker degradation of isoleucine or leucine and which largely contributes to fish odor.
Ketones are mainly produced from lipid oxidation, Maillard reaction, and amino acid degradation (42). Furthermore, ketones are also generated by the oxidation of alcohols and the decomposition of esters (43). These ketones are usually related to creamy, spicy, fatty, and fruity flavors (11, 44), which are also important for the generation of aroma in hairtails. The levels of 2-heptanone significantly increased with air-drying time. 2-Heptanone has a blue cheese odor, which is obtained through the decomposition of linoleic acid (11). Acetoin has a buttery aroma and the strongest signal peak on day 2 of air-drying (45). Acetoin is a precursor of diacetyl and the by-product of the metabolism of certain microbial carbohydrates, which play crucial roles in the synthesis of diols, esters, and glycosides and which have a significant impact on the overall aroma of food (46). Acetone, 2-butanone, 3-pentanone, and 1-penten-3-one are the most abundant VOCs in the 0-day hairtail samples. Acetone imparts a buttery smell to hairtails (47). 2-Butanone is related to the taste of milk (48) and is a spoilage marker for freshness in fish (49). Due to the influence of the smell of freshwater microalga, 3-pentanone has a wispy and fruity flavor (50). 3-Pentanone is highly enriched in the 0-day hairtail samples, which agreed with the findings on hairtails by Ding et al. (8). 1-Penten-3-one has a spicy and garlic odor. The level of ketones significantly differed among the three samples and contributed to a major effect on hairtail aroma.
Esters are important VOCs that impart a pleasant odor (fruit smell) to food (51). Ester compounds are synthesized by the esterification of alcohols and carboxylic acids and are also derived from the alcoholysis of acylglycerols and fatty alcohols (52). Moreover, short-chain esters may possess a sweet or fruity odor, while long-chain esters produce fatty aromas (53). Ethyl acetate has a pleasant, fruity, and brandy-like aroma (40, 54) and was observed at its highest level on day 4. Ethyl heptanoate, a compound with a fruity and winy odor (40), reached its highest level on day 0. The formation of esters in dry-cured meat is correlated to specific microbial enzymes (55). The levels of several esters increased with air-drying time and may be caused by the Maillard reaction in hairtails during the air-drying process, providing a unique flavor in hairtails. Subsequently, compounds formed from ester degradation consistently react with the intermediate product of amino acids, contributing to all aromatic odors in meat. These studies indicate that air-drying effectively increases the concentration of esters in processed hairtails compared with raw hairtails.
Pyrazines are special flavor substances of the Maillard reaction and have a peculiar baking and nutty taste (56, 57). With its high threshold value, acids are mainly formed from the hydrolysis of fats and do not contribute to the flavor of meat (58). 2-Ethylfuran is the degradation product of thiamine and has a stronger burnt flavor, which is mainly enriched on day 4. Dimethyl sulfide generates synergistic effects with other flavors, and its content is high on day 2 and may have impacted fruity odor expression (59). Trimethylamine is the product of facultative anaerobe reduction of trimethylamine oxide, which is the main component of fishy odor. The level of trimethylamine gradually increased during the air-drying process, possibly because trimethylamine oxide is extremely unstable and can be reduced easily to form trimethylamine by the action of the enzyme. The signal peak of ammonia was enhanced during the air-drying process, possibly due to the decomposition of proteins by microorganisms, transformation to peptides and amino acids, and the formation of ammonia. Trimethylamine and ammonia emit a bad smell and are considered putrefaction markers of flavor in hairtails.
Principal component analysis is a multivariate statistical analysis tool that uses a multivariate linear transformation to extract information on significant variables (60). The PCA model is selected as the separation model when the cumulative contribution rate reaches 60%. In this study, PCA was used to analyze changes in identified VOCs in air-dried hairtail samples (Figure 5), in which the cumulative variance contribution rate of the first part PC1 (first principal component) (73%) and the second part PC2 (second principal component) (24%) was 97%. The 0-day, 2-day, and 4-day groups were located in different places and far away from each other; the hairtail samples were clearly distinguished, indicating the relationship of flavor compounds among different air-drying times.
Figure 5. Principal component analysis (PCA) of flavor compounds in hairtails during the air-drying process.
Moreover, a correlation analysis (Figure 6) was performed in the present study to determine the relationship between 15 VOCs using Spearman's correlation tests. The moisture content and TBARS values were significantly correlated (P < 0.05). This result was consistent with the experimental conclusions of this study. Ethanol showed a significantly negative correlation with ammonia, trimethylamine, 1-hexanol, and ethyl acetate (P < 0.05). The alcohols and esters were significantly negatively correlated with acetoin (P < 0.05) but were significantly positively correlated with aldehydes. This may be the reason why lipid oxidation, the degradation of proteins, and the growth of microorganisms lead to changes in VOCs during the air-drying process, with these VOCs mutually transformed and emitting a distinctive flavor in air-dried hairtails.
In this study, volatile compounds of hairtails during air-drying were analyzed using HS-GC-IMS. The physicochemical results revealed a decrease in moisture content, while the TBARS values increased with air-drying time. A total of 75 VOCs, including 22 alcohols, 21 aldehydes, 11 ketones, 7 esters, 4 hydrocarbons, 3 nitrogen-bearing, 2 pyrazines, 2 acids, 1 furan, 1 sulfur-bearing, and 1 pyridine, were identified in hairtails. Among these, ethanol, 3-methyl-1-butanol, 1-pentanol, hexanal, octanal, benzaldehyde, and 3-methylbutanal were the main volatile components in the 0-day hairtails. Acetoin and dimethyl sulfide were the characteristic VOCs in 2 d hairtails. Compounds, such as 1-hexanol, 1-octen-3-ol, nonanal, heptanal, 2-heptanone, ethyl acetate, trimethylamine, and ammonia, were identified in the 4-day hairtail samples and thus considered biomarkers. In addition, our results showed that different samples were relatively independent and distinguishable in the PCA chart. Correlation analysis of flavor compounds indicated that these compounds were significantly correlated and promoted the flavor of hairtails via a synergistic effect. As an emerging technology, HS-GC-IMS could be a rapid and reliable method for analyzing flavor characteristics, which could readily distinguish different samples of hairtails during the air-drying process.
The original contributions presented in the study are included in the article/supplementary material, further inquiries can be directed to the corresponding authors.
No animals or humans were used as subjects in this study.
YL, YD, YW, QD, JX, and JJ contributed to the conception and design of the opinion-study and drafted the manuscript first. SB, YH, HL, and BZ edited, revised, and approved the final version of the submitted manuscript. All authors contributed to the article and approved the submitted version.
This study was funded by the Zhejiang Leading Training Program (Grant No. 2020R52027) and the Fundamental Research Funds of Zhejiang Province (2021JD005).
We thank LetPub (www.letpub.com) for their linguistic assistance during the preparation of this manuscript.
The authors declare that the research was conducted in the absence of any commercial or financial relationships that could be construed as a potential conflict of interest.
All claims expressed in this article are solely those of the authors and do not necessarily represent those of their affiliated organizations, or those of the publisher, the editors and the reviewers. Any product that may be evaluated in this article, or claim that may be made by its manufacturer, is not guaranteed or endorsed by the publisher.
1. Liu JL, Shao Y, Yuan CH, Takaki KC, Li YJ, Ying YB, et al. Eugenol-chitosan nanoemulsion as an edible coating: its impact on physicochemical, microbiological and sensorial properties of hairtail (trichiurus haumela) during storage at 4°C. Int J Biol Macromol. (2021) 183:2199–204. doi: 10.1016/j.ijbiomac.2021.05.183
2. Arshad MS, Sohaib M, Ahmad RS, Nadeem MT, Imran A, Arshad MU, et al. Ruminant meat flavor influenced by different factors with special reference to fatty acids. Lipids Health Dis. (2018) 17:1–13. doi: 10.1186/s12944-018-0860-z
3. Diez-Simon C, Mumm R, Hall RD. Mass spectrometry-based metabolomics of volatiles as a new tool for understanding aroma and flavour chemistry in processed food products. Metabolomics. (2019) 15:1–20. doi: 10.1007/s11306-019-1493-6
4. Chung HY, Yeung CW, Kim JS, Chen F. Static headspace analysis-olfactometry (SHA-O) of odor impact components in salted-dried white herring (Ilisha elongata). Food Chem. (2007) 104:842–51. doi: 10.1016/j.foodchem.2006.08.036
5. Czerner M, Tomás MC, Yeannes MI. Ripening of salted anchovy (Engraulis anchoita): development of lipid oxidation, colour and other sensorial characteristics. J Sci Food Agr. (2011) 91:609–15. doi: 10.1002/jsfa.4221
6. Jia SL Li Y, Zhuang S, Sun XH, Zhang LT, Shi J, et al. Biochemical changes induced by dominant bacteria in chill-stored silver carp (Hypophthalmichthys molitrix) and GC-IMS identification of volatile organic compounds. Food Microbiol. (2019) 84:103248. doi: 10.1016/j.fm.2019.103248
7. Shi Y, Li X, Huang AX, A. metabolomics-based approach investigates volatile flavor formation and characteristic compounds of the Dahe black pig dry-cured ham. Meat Sci. (2019) 158:107904. doi: 10.1016/j.meatsci.2019.107904
8. Ding YX, Zhou T, Liao YQ, Lin HM, Deng SG, Zhang, B. Comparative studies on the physicochemical and volatile flavour properties of traditional deep fried and circulating-air fried hairtail (Trichiurus lepturus). Foods. (2022) 11:2710. doi: 10.3390/foods11172710
9. Fu XJ, Lin QL, Xu SY, Wang Z. Effect of drying methods and antioxidants on the flavor and lipid oxidation of silver carp slices. LWT-Food Sci Technol. (2015) 61:251–7. doi: 10.1016/j.lwt.2014.10.035
10. Leduc F, Tournayre P, Kondjoyan N, Mercier F, Malle P, Kol O, et al. Evolution of volatile odorous compounds during the storage of European seabass (Dicentrarchus labrax). Food Chem (2012) 131(4). (2012) 1304–11. doi: 10.1016/j.foodchem.2011.09.123
11. Liu DY, Bai L, Feng X, Chen YP, Zhang D, Yao WS, et al. Characterization of Jinhua ham aroma profiles in specific to aging time by gas chromatography-ion mobility spectrometry (GC-IMS). Meat Sci. (2020) 168:108178. doi: 10.1016/j.meatsci.2020.108178
12. Hernández-Mesa M, Escourrou A, Monteau F, Le Bizec B, Dervilly-Pinel G. Current applications and perspectives of ion mobility spectrometry to answer chemical food safety issues. TRAC-Trend Anal Chem. (2017) 94:39–53. doi: 10.1016/j.trac.2017.07.006
13. Zheng XY, Wojcik R, Zhang X, Ibrahim YM, Burnum-Johnson KE, Orton DJ, et al. Coupling front-end separations, ion mobility spectrometry, and mass spectrometry for enhanced multidimensional biological and environmental analyses. Annu Rev Anal Chem. (2017) 10:71–92. doi: 10.1146/annurev-anchem-061516-045212
14. Van Gaal N, Lakenman R, Covington J, Savage R, De Groot E, Bomers M, et al. Faecal volatile organic compounds analysis using field asymmetric ion mobility spectrometry: non-invasive diagnostics in paediatric inflammatory bowel disease. J Breath Res. (2017) 12:016006. doi: 10.1088/1752-7163/aa6f1d
15. Wang F, Gao YQ, Wang HB Xi B, He XN, Yang XL, et al. Analysis of volatile compounds and flavor fingerprint in Jingyuan lamb of different ages using gas chromatography-ion mobility spectrometry (GC-IMS). Meat Sci. (2021) 175:108449. doi: 10.1016/j.meatsci.2021.108449
16. Yao WS, Cai YX, Liu DY, Zhao ZN, Zhang ZH, Ma SY, et al. Comparative analysis of characteristic volatile compounds in Chinese traditional smoked chicken (specialty poultry products) from different regions by headspace-gas chromatography-ion mobility spectrometry. Poultry Sci. (2020) 99:7192–201. doi: 10.1016/j.psj.2020.09.011
17. Nie S, Li LH, Wang YQ, Wu YY, Li CS, Chen SJ, et al. Discrimination and characterization of volatile organic compound fingerprints during sea bass (Lateolabrax japonicas) fermentation by combining GC-IMS and GC-MS. Food Biosci. (2022) 3:102048. doi: 10.1016/j.fbio.2022.102048
18. Ma YB, Zhang JX, Zhou XQ, Jiang WD, Wu P, Liu Y, et al. Effect of tea polyphenols on flavour, healthcare components, physicochemical properties, and mechanisms of collagen synthesis in growing grass carp (Ctenopharyngodon idella) muscle. Aquaculture. (2021) 534:736237. doi: 10.1016/j.aquaculture.2020.736237
19. Wang YQ, Wang HF, Wu YY, Xiang H, Zhao YQ, Chen SJ, et al. Insights into lipid oxidation and free fatty acid profiles to the development of volatile organic compounds in traditional fermented golden pomfret based on multivariate analysis. LWT - Food Sci Technol. (2022) 171:114112. doi: 10.1016/j.lwt.2022.114112
20. Lou XW, Zhang YB, Sun YY, Wang Y, Pan DD, Cao JX. The change of volatile compounds of two kinds of Vinasse-cured ducks during processing. Poultry Sci. (2018) 97:2607–17. doi: 10.3382/ps/pey109
21. Zhang JH, Cao J, Pei ZS, Wei PY, Xiang D, Cao XY, et al. Volatile flavour components and the mechanisms underlying their production in golden pompano (Trachinotus blochii) fillets subjected to different drying methods: a comparative study using an electronic nose, an electronic tongue and SDE-GC-MS. Food Res Int. (2019) 123:217–25. doi: 10.1016/j.foodres.2019.04.069
22. Zhang JH, Zhen ZY, Zhang WG, Zeng T, Zhou GH. Effect of intensifying hightemperature ripening on proteolysis, lipolysis and flavour of Jinhua ham. J Sci Food Agr. (2009) 89:834–42. doi: 10.1002/jsfa.3521
23. Ren S, Li PP, Geng ZM, Sun C, Song H, Wang DY, et al. Lipolysis and lipid oxidation during processing of Chinese traditional dry-cured white amur bream (Parabramis pekinensis). J Aquat Food Prod T. (2017) 26:719–30. doi: 10.1080/10498850.2016.1276112
24. Huang YC Li HJ, Huang T, Li F, Sun J. Lipolysis and lipid oxidation during processing of Chinese traditional smoke-cured bacon. Food Chem. (2014) 149:31–9. doi: 10.1016/j.foodchem.2013.10.081
25. Zhao YQ, Yang SL, Yang XQ Li LH, Hao SX, Cen JW, et al. Effects of ozonated water treatment on physico-chemical, microbiological and sensory characteristics changes of Nile Tilapia (Oreochromis niloticus) fillets during storage in ice. Ozone-Sci Eng. (2020) 42:408–19. doi: 10.1080/01919512.2019.1688133
26. Yao WS, Cai YX, Liu DY, Chen Y, Li JR, Zhang MC, et al. Analysis of flavor formation during production of Dezhou braised chicken using headspace-gas chromatography-ion mobility spec-trometry (HS-GC-IMS). Food Chem. (2022) 370:130989. doi: 10.1016/j.foodchem.2021.130989
27. Nordvi B, Langsrud Ø, Egelandsdal B, Slinde E, Vogt G, Gutierrez M, et al. Characterization of volatile compounds in a fermented and dried fish product during cold storage. J Food Sci. (2007) 72:S373–80. doi: 10.1111/j.1750-3841.2007.00421.x
28. Luo XY, Xiao ST, Ruan QF, Gao Q, An YQ, Hu Y, et al. Differences in flavor characteristics of frozen surimi products reheated by microwave, water boiling, steaming, and frying. Food Chem. (2022) 372:131260. doi: 10.1016/j.foodchem.2021.131260
29. Timón ML, Ventanas J, Carrapiso AI, Jurado A, Garcia C. Subcutaneous and intermuscular fat characterisation of dry-cured Iberian hams. Meat Sci. (2001) 58:85–91. doi: 10.1016/S0309-1740(00)00136-4
31. Mezgebo GB, Monahan FJ, McGee M, O'Riordan EG, Richardson IR, Brunton NP, et al. Fatty acid, volatile and sensory characteristics of beef as affected by grass silage or pasture in the bovine diet. Food Chem. (2017) 235:86–97. doi: 10.1016/j.foodchem.2017.05.025
32. Iglesias J, Medina I. Solid-phase microextraction method for the determination of volatile compounds associated to oxidation of fish muscle. J Chromatography A. (2008) 1192:9–16. doi: 10.1016/j.chroma.2008.03.028
33. Chang C, Wu GC, Zhang H, Jin QZ, Wang XG. Deep-fried flavor: characteristics, formation mechanisms, and influencing factors. Crit Rev Food Sci. (2020) 60:1496–514. doi: 10.1080/10408398.2019.1575792
34. Wu N, Wang XC. Identification of important odorants derived from phosphatidylethanolamine species in steamed male Eriocheir sinensis hepatopancreas in model systems. Food Chem. (2019) 286:491–9. doi: 10.1016/j.foodchem.2019.01.201
35. Yang Y, Zhang X, Wang Y, Pan DD, Sun YY, Cao JX. Study on the volatile compounds generated from lipid oxidation of Chinese bacon (unsmoked) during processing. Eur J Lipid Sci Tech. (2017) 119:1600512. doi: 10.1002/ejlt.201600512
36. Moretti VM, Vasconi M, Caprino F, Bellagamba F. Fatty acid profiles and volatile compounds formation during processing and ripening of a traditional salted dry fish product. J Food Process Pres. (2017) 41:e13133. doi: 10.1111/jfpp.13133
37. Yin XY, Lv YC, Wen RX, Wang Y, Chen Q, Kong BH. Characterization of selected Harbin red sausages on the basis of their flavour profiles using HS-SPME-GC/MS combined with electronic nose and electronic tongue. Meat Sci. (2021) 172:108345. doi: 10.1016/j.meatsci.2020.108345
38. Tanimoto S, Kitabayashi K, Fukusima C, Sugiyama S, Hashimoto T. Effect of storage period before reheating on the volatile compound composition and lipid oxidation of steamed meat of yellowtail Seriola quinqueradiata. Fisheries Sci. (2015) 81:1145–55. doi: 10.1007/s12562-015-0921-4
39. Wang D, Zhang J, Zhu ZS, Lei Y. Huang, SH, Huang M. Effect of ageing time on the flavour compounds in Nanjing water-boiled salted duck detected by HS-GC-IMS. LWT-Food Sci Technol. (2022) 155:112870. doi: 10.1016/j.lwt.2021.112870
40. Deng WY, Tian GF, Wang ZX, Mao KM, Liu XH, Ding QY, et al. Analysis of volatile components changes of Ruditapes philippinarum during boiling by HS-GC-IMS coupled with multivariate analyses. Aquacult Rep. (2022) 25:101193. doi: 10.1016/j.aqrep.2022.101193
41. De Palencia PF, de la Plaza M, Mohedano ML, Martinez-Cuesta MC, Requena T, López P, et al. Enhancement of 2-methylbutanal formation in cheese by using a fluorescently tagged Lacticin 3,147 producing Lactococcus lactis strain. Int J Food Microbiol. (2004) 93:335-347. doi: 10.1016/j.ijfoodmicro.2003.11.018
42. Zhang J, Zhang WG, Zhou L, Zhang RY. Study on the influences of ultrasound on the flavor profile of unsmoked bacon and its underlying metabolic mechanism by using HS-GC-IMS. Ultrasoni Sonochem. (2021) 80:105807. doi: 10.1016/j.ultsonch.2021.105807
43. Elmore JS, Mottram DS. Flavour development in meat. Improving the sensory and nutritional quality of fresh meat. (2009) 4:111–46. doi: 10.1533/9781845695439.1.111
44. Chen Y, Li P, Liao LY, Qin YY, Jiang LW, Liu Y. Characteristic fingerprints and volatile flavor compound variations in Liuyang Douchi during fermentation via HS-GC-IMS and HS-SPME-GC-MS. Food Chem. (2021) 361:130055. doi: 10.1016/j.foodchem.2021.130055
45. Toldra F. Proteolysis and lipolysis in flavour development of dry-cured meat products. Meat Sci. (1998) 49:S101–10. doi: 10.1016/S0309-1740(98)00077-1
46. Cai CC, Zhang M, Chen HM, Chen WX, Chen WJ, Zhong QP. Enhancement of norisoprenoid and acetoin production for improving the aroma of fermented mango juice by Bacillus subtilis-HNU-B3. Process Biochem. (2022) 113:177–84. doi: 10.1016/j.procbio.2022.01.002
47. Barbieri G, Bolzoni L, Parolari G, Virgili R, Buttini R, Careri M, et al. Flavor compounds of dry-cured ham. J Agr Food Chem. (1992) 40:2389–94. doi: 10.1021/jf00024a013
48. Pham AJ, Schilling MW, Mikel WB, Williams JB, Martin JM, Coggins PC. Relationships between sensory descriptors, consumer acceptability and volatile flavor compounds of American dry-cured ham. Meat Sci. (2008) 80:728–37. doi: 10.1016/j.meatsci.2008.03.015
49. Wang SQ, Chen HT, Sun BG. Recent progress in food flavor analysis using gas chromatography-ion mobility spectrometry (GC-IMS). Food Chem. (2020) 315:126158. doi: 10.1016/j.foodchem.2019.126158
50. Hosoglu MI, Karagul-Yuceer Y, Guneser O. Aroma characterization of heterotrophic microalgae Crypthecodinium cohnii using solid-phase microextraction and gas chromatography-mass spectrometry/olfactometry during different growth phases. Algal Res. (2020) 49:101928. doi: 10.1016/j.algal.2020.101928
51. García-Béjar B, Sánchez-Carabias D, Alarcon M, Arévalo-Villena M, Briones A. Autochthonous yeast from pork and game meat fermented sausages for application in meat protection and aroma developing. Animals. (2020) 10:2340. doi: 10.3390/ani10122340
52. Liu SQ, Holland R, Crow VL. Esters and their biosynthesis in fermented dairy products: a review. Int Dairy J. (2004) 14:923–45. doi: 10.1016/j.idairyj.2004.02.010
53. Martin D, Antequera T, Muriel E, Andres AI, Ruiz J. Oxidative changes of fresh loin from pig, caused by dietary conjugated linoleic acid and monounsaturated fatty acids, during refrigerated storage. Food Chem. (2008) 111:730–7. doi: 10.1016/j.foodchem.2008.04.048
54. Watanabe A, Kamada G, Imanari M, Shiba N, Yonai M, Muramoto T. Effect of aging on volatile compounds in cooked beef. Meat Sci. (2015) 107:12–9. doi: 10.1016/j.meatsci.2015.04.004
55. Ordóñez JA, Hierro EM, Bruna JM, Hoz LDL. Changes in the components of dry-fermented sausages during ripening. Crit Rev Food Sci. (1999) 39:329–67. doi: 10.1080/10408699991279204
56. Hou H, Liu C, Lu XS, Fang DL, Hu QH, Zhang YY, et al. Characterization of flavor frame in shiitake mushrooms (Lentinula edodes) detected by HS-GC-IMS coupled with electronic tongue and sensory analysis: Influence of drying techniques. LWT-Food Sci Technol. (2021) 146:111402. doi: 10.1016/j.lwt.2021.111402
57. Yang LZ, Liu J, Wang XY, Wang RR, Ren F, Zhang Q, et al. Characterization of volatile component changes in jujube fruits during cold storage by using headspace-gas chromatography-ion mobility spectrometry. Molecules. (2019) 24:3904. doi: 10.3390/molecules24213904
58. Wang Y, Song HL, Zhang Y, Tang JN Yu DH. Determination of aroma compounds in pork broth produced by different processing methods. Flavour Frag J. (2016) 31:319–28. doi: 10.1002/ffj.3320
59. Lytra G, Tempere S, Marchand S, de Revel G, Barbe JC. How do esters and dimethyl sulphide concentrations affect fruity aroma perception of red wine? Demonstration by dynamic sensory profile evaluation. Food Chem. (2016) 194:196–200. doi: 10.1016/j.foodchem.2015.07.143
60. Dong WJ, Hu RS, Long YZ Li HH, Zhang YJ, Zhu KX, et al. Comparative evaluation of the volatile profiles and taste properties of roasted coffee beans as affected by drying method and detected by electronic nose, electronic tongue, and HS-SPME-GC-MS. Food Chem. (2019) 272:723–31. doi: 10.1016/j.foodchem.2018.08.068
Keywords: air-drying, hairtail, HS-GC-IMS, volatile flavors, fingerprint
Citation: Liao Y, Ding Y, Wu Y, Du Q, Xia J, Jia J, Lin H, Benjakul S, Zhang B and Hu Y (2023) Analysis of volatile compounds and flavor fingerprint in hairtail (Trichiurus lepturus) during air-drying using headspace-gas chromatography-ion mobility spectrometry (HS-GC-IMS). Front. Nutr. 9:1088128. doi: 10.3389/fnut.2022.1088128
Received: 03 November 2022; Accepted: 13 December 2022;
Published: 11 January 2023.
Edited by:
Yueqi Wang, South China Sea Fisheries Research Institute, CAFS, ChinaReviewed by:
Liyan Zhao, Nanjing Agricultural University, ChinaCopyright © 2023 Liao, Ding, Wu, Du, Xia, Jia, Lin, Benjakul, Zhang and Hu. This is an open-access article distributed under the terms of the Creative Commons Attribution License (CC BY). The use, distribution or reproduction in other forums is permitted, provided the original author(s) and the copyright owner(s) are credited and that the original publication in this journal is cited, in accordance with accepted academic practice. No use, distribution or reproduction is permitted which does not comply with these terms.
*Correspondence: Huimin Lin, linhuixiaomin@126.com;
lin.huimin@zjou.edu.cn; Bin Zhang,
zhangbin@zjou.edu.cn;
zhangbin_ouc@163.com
†These authors have contributed equally to this work and share first authorship
‡ORCID: Huimin Lin orcid.org/0000-0003-2098-0352
Bin Zhang orcid.org/0000-0003-4696-9098
Disclaimer: All claims expressed in this article are solely those of the authors and do not necessarily represent those of their affiliated organizations, or those of the publisher, the editors and the reviewers. Any product that may be evaluated in this article or claim that may be made by its manufacturer is not guaranteed or endorsed by the publisher.
Research integrity at Frontiers
Learn more about the work of our research integrity team to safeguard the quality of each article we publish.