- 1Department of Neurology, Xiangya Hospital, Central South University, Changsha, China
- 2National Clinical Research Center for Geriatric Disorders, Xiangya Hospital, Central South University, Changsha, China
- 3Health Management Center, Xiangya Hospital, Central South University, Changsha, China
- 4Department of Critical Care Medicine, The First People’s Hospital of Huaihua, Huaihua, China
- 5Department of Neurology, Hengyang Central Hospital, Hengyang, China
- 6Department of Human Anatomy and Neurobiology, School of Basic Medicine, Central South University, Changsha, China
- 7Department of Neurology, Xiangtan Central Hospital, Xiangtan, China
Background: Homocysteine (Hcy) has been indicated to be involved in pathophysiology of post stroke depression (PSD). There is a lack of research to study the relationship between Hcy metabolism genes and PSD. Our study aims to investigate the relationship among Hcy metabolism genes, Hcy, and early-onset PSD.
Materials and methods: We recruited 212 patients with stroke and collected their peripheral blood sample, clinical data, and laboratory test on admission. 12 single nucleotide polymorphisms (SNPs) in methylenetetrahydrofolate reductase (MTHFR), methionine synthase reductase (MTRR), and methionine synthase (MTR) genes were genotyped by high-resolution melt analysis. PSD was diagnosed by DSM-V at 2 weeks after stroke. Binary logistic regression and haplotype analysis were used to examine the association between Hcy metabolism genes and PSD. Mediation analysis was performed to clarify whether the SNPs exerted their effect on PSD by affecting the Hcy level.
Results: 81 patients were diagnosed with PSD, and the incidence rate was 38.2%. Hcy level in PSD group was significantly higher than it in non-PSD group (p = 0.019). MTHFR rs1801133 AA genotype an A allele were associated with an elevated risk of PSD after adjustment for some confounding factors (OR = 4.021, 95% CI: 1.459∼11.080, p = 0.007 for AA genotype; OR = 1.808, 95% CI: 1.172∼2.788, p = 0.007 for A allele). Furthermore, the effect of MTHFR rs1801133 AA genotype on PSD was mediated by Hcy (OR = 1.569, 95% CI: 0.013∼3.350, p < 0.05).
Conclusion: MTHFR rs1801133 and Hcy were associated with PSD, and MTHFR rs1801133 may exert an effect on PSD via mediating Hcy level. This offers a new perspective for treating PSD and understanding the mechanism of PSD.
Introduction
Post stroke depression (PSD) is a common neuropsychiatric sequela after stroke with an incidence rate of approximately 30% at any time after stroke (1, 2). PSD is associated with a poor prognosis of stroke in the form of worse functional and motor recovery, poorer cognitive impairment, lower quality of life, and higher mortality (1, 3, 4). It is of substantial clinical significance to achieve early identification, accurate diagnosis, and timely treatment of PSD. However, due to the paucity of reliable objective biomarkers of PSD, as well as the impediment of stroke-related neurological symptoms such as aphasia and abulia, many patients with PSD cannot be promptly diagnosed and treated (5). Therefore, investigating the mechanism of PSD is of great urgency for the early identification and treatment of PSD.
Homocysteine (Hcy), a sulfur-containing amino acid, is an essential intermediate product during the metabolism of methionine to cysteine. Previous researches have proved that elevated serum Hcy level was a risk factor for ischemic stroke and could predict mortality from ischemic stroke, especially in the large-artery atherosclerosis subtype (6, 7). Folic acid supplementation can effectively lower the risk and improve the outcome of stroke (8, 9). Meanwhile, a meta-analysis showed that the Hcy level was higher in depressed subjects compared with healthy controls, and elevated Hcy was associated with lifetime major depressive disorder and currently experiencing depressive symptoms (10–12). Lowering Hcy by promoting vitamin B12 and folate-rich food may help ameliorate depression and anxiety (13). Notably, a previous study reported elevated Hcy at admission was related to PSD at 3 months after stroke (14). High sensitivity C-reactive protein combined with Hcy could predict PSD at 3 months and 1 year after stroke more accurately, compared to any single factor (15, 16). Therefore, we speculate Hcy may be closely related to the occurrence and development of PSD. However, the mechanism behind the relationship between Hcy and PSD remains unknown.
Homocysteine (Hcy) concentration in serum can be influenced by diet, renal function, and genetic factors (17). Several genes, including methylenetetrahydrofolate reductase (MTHFR), methionine synthase (MTR), and methionine synthase reductase (MTRR), take part in the Hcy remethylation pathway via encoding related enzymes (18–20). MTHFR catalyzes the conversion of 5,10-methylene-tetrahydrofolate to 5-methyl-tetrahydrofolate, which serves as the substrate for Hcy into methionine catalyzed by MTR (21, 22). In this reaction, MTR require cobalamin(I) as a coenzyme, and cobalamin(I) is rapidly oxidized to cobalamin(II), resulting in the inactivation of MTR. MTRR maintains the activity of MTR by reductive methylation of cobalamin(II) (20). Little efforts on the role of Hcy metabolism genes in PSD have been made. Mei et al. only focus on the association between rs1801133 (MTHFR) and PSD and found that rs1801133 AG genotype and A allele increased the risk of PSD (23). However, whether other polymorphisms of Hcy metabolism genes are linked to PSD needs further investigation.
This study is aimed to examine the change in Hcy level in patients with PSD at 2 weeks after stroke (early-onset PSD). Furthermore, we explored the polymorphisms of Hcy metabolism genes to reveal the mechanism of altered Hcy levels in PSD.
Materials and methods
Study population
The patient population comprised 227 acute ischemic stroke patients consecutively admitted to Xiangya Hospital Central South University, Changsha from July 2019 to August 2021. The Ethics Committee of Xiangya Hospital, Central South University approved the protocol (No. 201910842). All patients provided written informed consent in accordance with the Declaration of Helsinki and its later amendments. The inclusion criteria were as follows: (1) diagnosed stroke patients by neurological examination, cerebral computed tomographic scan, and magnetic resonance imaging; (2) age between 18 and 75 years old; and (3) hospital admission within 2 weeks after stroke onset. The exclusive criteria were as follows: (1) unable to complete the evaluations and questionnaires because of disturbance of consciousness and comprehension, visual and hearing disturbance, and communication problems caused by aphasia and dysarthria; (2) comorbid with other concomitant neuropsychiatric diseases, for example, Parkinson’s disease, Alzheimer’s disease, and epilepsy; (3) brain dysfunction caused by other non-vascular causes, for instance, brain trauma, brain tumor, encephalitis and so on; (4) history of depression and other mental disorder.
Data collection and clinical assessment
We collected information on demographics, medical history, and clinical characteristics. The National Institutes of Health Stroke Scale (NIHSS) was used to evaluate stroke severity within 24 h after hospital admission by neurologists. Barthel Index (BI) scores and modified Rankin Scale (mRS) scores were also collected to assess the activity of daily living. Mini-mental State Examination (MMSE) was used to measure cognitive function. Diagnostic and Statistical Manual of Mental Disorders, 5th edition (DSM-V) was adopted to diagnose PSD by a psychiatrist at 2 weeks after stroke. The severity of depressive and anxious symptoms was evaluated with the Hamilton depression scale 17 items (HAMD-17) and Hamilton anxiety scale (HAMA) respectively. All researchers involved in conducting clinical assessments were blinded to genetic and serum biochemical data after uniform training.
Blood sample collection, storage, and measurements
We collected peripheral blood samples of patients on admission in vacutainer tubes containing the anticoagulant ethylenediaminetetraacetic acid and stored them at −80°C until use. The concentration of plasma Hcy was measured using the kit (Maccura Biotechnology Co., Ltd., Chengdu, China) according to the manufacturer’s instruction in the clinical laboratory of Xiangya Hospital.
Single nucleotide polymorphism selection and genotyping
According to information in the National Center for Biotechnology Information SNP database,1 12 SNPs of MTHFR, MTR, and MTRR were selected minor allele frequency of > 5% and previously reported (24–26). All SNPs were genotyped using a SNPscan™ multiplex SNP typing Kit (Cat#: G0104K, Genesky Biotechnologies Inc., Shanghai, China). The SNPscan assay technique is a rapid multiplex genetic screening system, and the basic principle of this technology is based on double ligation and multiplex fluorescent polymerase chain reaction (PCR). The procedure of this technique has been reported in the previous study (27). For quality control, 5% of duplicate DNA samples were analyzed with the same process.
Statistical analysis
We conducted statistical analysis by SPSS version 13.0 for windows (SPSS Inc., Chicago, IL, USA). Mann-Whitney test, Wilcoxon signed rank test, and Student’s t-test were used to determine whether the differences of median existed. Differences between proportions were assessed with the χ2-test or Fisher exact test. F test was assessed for equal variance, and normality was assessed by the Shapiro-Wilk normality test. Binary logistic regression analysis was adopted to examine the association between genotypes and PSD. The statistical significance threshold was set to a two-sided p-value < 0.05. The bootstrapping test was performed using the SPSS PROCESS version 4.1 to test the statistical significance of the mediating effect. The significance of the mediation effect was conducted using 5,000 bootstrapped iterations. Hardy-Weinberg equilibrium (HWE) test, linkage disequilibrium (LD), and haplotype association analysis were conducted by the SHEsis, an online program2 (28, 29). The results of categorical variables were expressed as percentages. Continuous variables were shown as the median and interquartile range (IQR) or the mean ± standard deviation (SD) depending on the normal or non-normal distribution of the data.
Results
Characteristics of the study population
Due to data missing and loss follow-up, 15 patients were excluded (Figure 1). We included 212 patients with ischemic stroke, and 81 patients were diagnosed with PSD, for a percentage of 38.2%. The demographic and clinical characteristics are shown in Table 1. Compared to the non-PSD group, the PSD group showed a higher NIHSS score [4 (2, 8.5) vs. 2 (1, 4), p < 0.001], mRS score [3 (1, 4) vs. 2 (1, 3); p < 0.001], HAMD score [14 (11, 20) vs. 5 (2, 8), p < 0.001], HAMA score [14 (9.5, 19) vs. 5.5 (3, 8.75), p < 0.001], and lower BI score [75 (35, 100) vs. 100 (70, 100); p < 0.001]. There were no significant differences between stroke patients with and without PSD in age, gender, marital status, educational level, body mass index, vascular risk factors (smoking, drinking, hypertension, and diabetes mellitus) location of lesion TOAST classification, MMSE, and serum biochemical index (creatinine, total cholesterol, triglycerides, low-density lipoprotein cholesterol, high-density lipoprotein cholesterol).
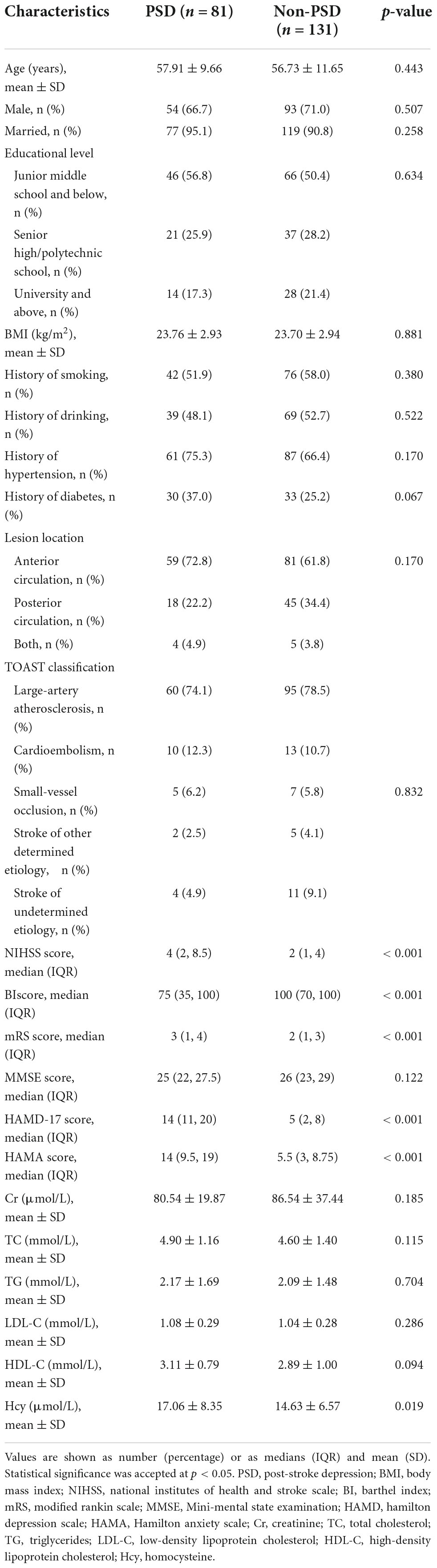
Table 1. Clinical and demographic characteristics of post stroke depression (PSD) and non-PSD patients.
Association between genotypes of MTHFR, MTRR, MTR, Hcy, and early-onset PSD
As shown in Table 1, the PSD group had a higher Hcy level than non-PSD group (17.06 ± 8.35 vs. 14.63 ± 6.57, p = 0.019). All SNPs distribution of MTHFR, MTRR, and MTR were consistent with Hardy-Weinberg equilibrium (p > 0.05). The rs1801133 (MTHFR) was significantly associated with PSD. AA genotype (OR = 3.531, 95% CI: 1.351∼9.227, p = 0.010) and A allele (OR = 1.792, 95% CI: 1.186∼2.706, p = 0.006) were risk factors of PSD, and AG genotype was marginally associated with PSD (OR = 1.790, 95% CI: 0.980∼3.269, p = 0.058). The rs1801131 T allele was related to an elevated risk of PSD [0.573 (0.341∼0.964), p = 0.036]. When adjusting for confounding factors (age, gender, body mass index, NIHSS score, and MMSE score), rs1801133 AA genotype (OR = 4.021, 95% CI: 1.459∼11.080, p = 0.007) and A allele (OR = 1.808, 95% CI: 1.172∼2.788, p = 0.007) were still strongly related to PSD (Table 2), but not rs1801131 T allele. Other SNPs did not show statistically significant differences between PSD group and non-PSD group (Supplementary Table 1). In addition, the mean Hcy level concentrations according to corresponding genotypes of rs1801133 were shown in Figure 2A. The Hcy level was significantly correlated with rs1801133 (p < 0.001) but no other SNPs.
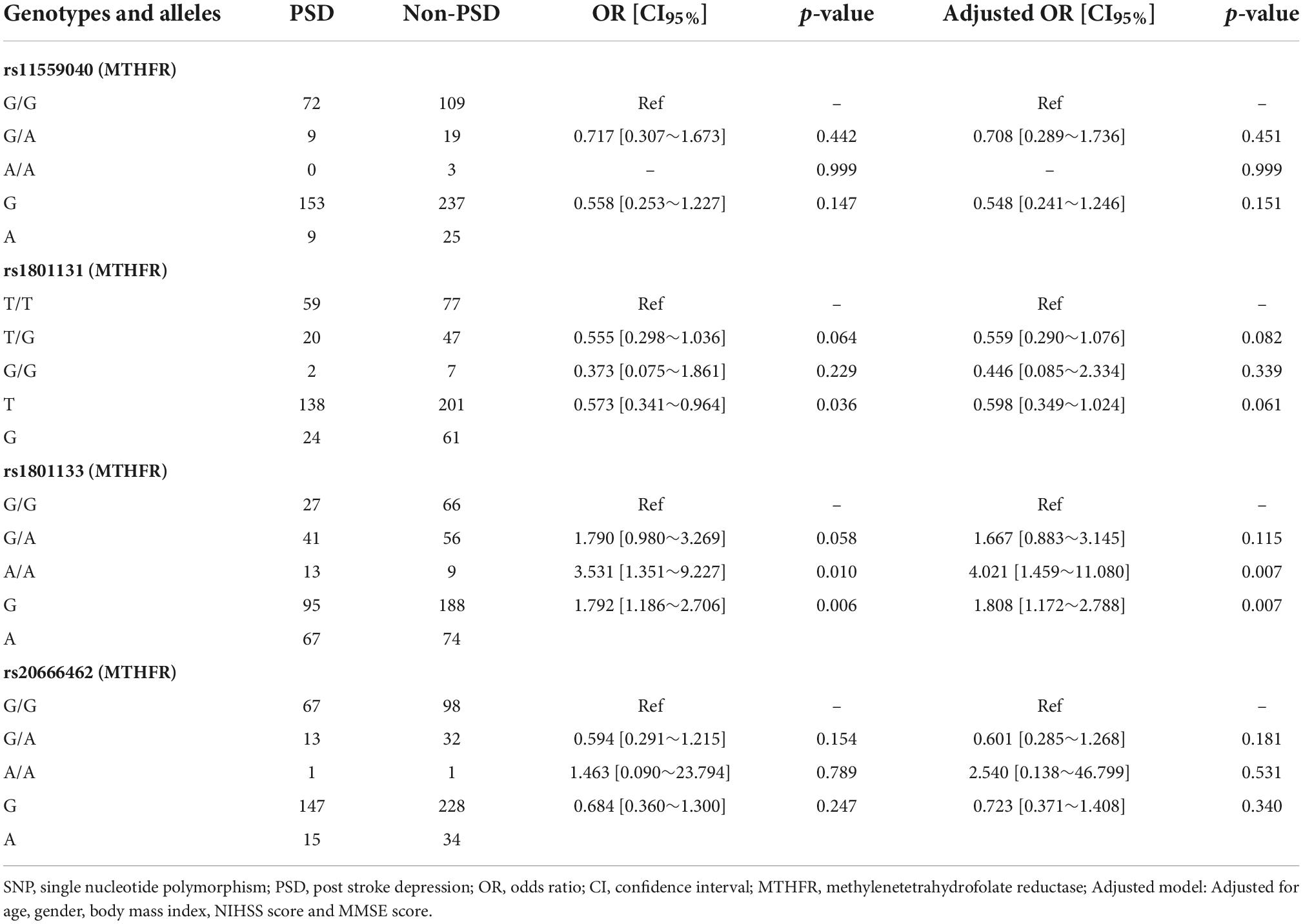
Table 2. Association of genotypes and alleles of methylenetetrahydrofolate reductase (MTHFR) and post stroke depression (PSD).
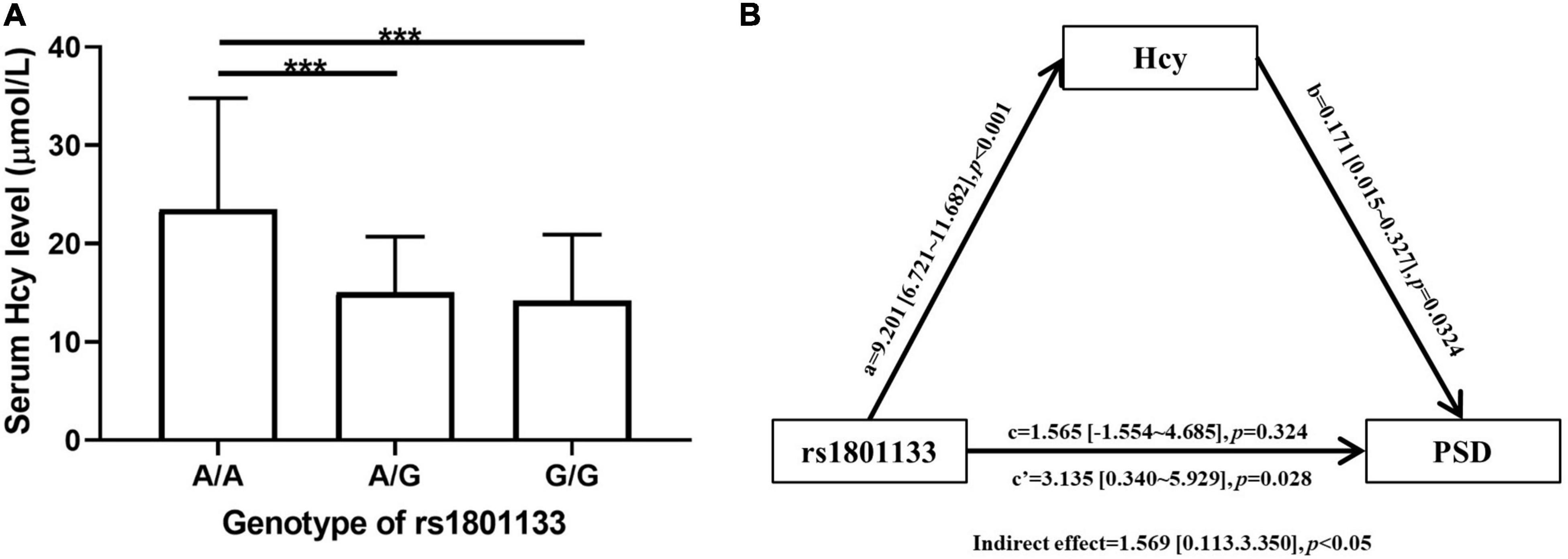
Figure 2. (A) Comparison of Homocysteine (Hcy) level among AA, AG, and GG genotype of rs1801133. Statistical significance was accepted at p < 0.05. ***p < 0.001. (B) Mediation analysis of association between rs1801133 [methylenetetrahydrofolate reductase (MTHFR)] and post stroke depression (PSD). The genotypes of rs1801133 were divided into AA and GA + GG group. The severity of PSD was represented as HAMD-17 score. (a) Direct effect of rs1801133 on Hcy; (b) Direct effect of Hcy on PSD; (c’) Total effect of rs1801133 on PSD; (c) Direct effect of rs1801133 on PSD.
Association between haplotype of MTHFR, MTRR, and MTR and early-onset PSD
Haplotype analysis is a powerful strategy to examine whether SNPs have a greater effect when analyzed together. As shown in Table 3, rs11559040, rs1801131, rs1801133, and rs2066462 exhibited strong LD (D’ > 0.95) (30). They formed four main haplotypes (AGGG, GGGA, GTAG GTGG), and those with frequency < 0.03 were excluded from the analysis (Table 4). We found that the GTAG haplotype was associated with an increased risk of PSD (OR = 1.852, 95% CI: 1.223∼2.805, p = 0.003), while haplotypes of MTRR and MTR were not.
Mediation analysis
To clarify the relationship among rs1801133, Hcy, and PSD, we adopted mediation analysis to test whether rs1801133 AA genotype would affect the incidence of PSD via mediating the Hcy level with age, gender, body mass index, NIHSS score, and MMSE score as covariate (Figure 2B). We found that rs1801133 AA genotype was significantly associated with PSD (total effect, OR = 3.135, 95% CI: 0.340∼5.929, p = 0.028). However, rs1801133 AA genotype was not linked to increased risk of PSD when removing the effect of Hcy (direct effect, OR = 1.565, 95% CI: −1.554∼4.685, p = 0.324), meanwhile, the effect of rs1801133 on PSD was mediated via Hcy (indirect effect, OR = 1.569, 95% CI: 0.113∼3.350, p < 0.05).
Discussion
This is the first study to determine the association among early-onset PSD, Hcy, and polymorphism of Hcy metabolism genes with a prospective cohort. We demonstrated that rs1801133 AA genotype, A allele, and haplotype GTAG (rs11559040-rs1801131-rs1801133-rs2066462) were associated with PSD, meanwhile, PSD group showed a higher Hcy level than non-PSD group. Furthermore, rs1801133 influenced PSD through mediating the Hcy levels.
In our study, 38.2% of the patients were diagnosed with PSD at 2 weeks after stroke onset. Similar to our results, a meta-analysis reported that approximately 31% of stroke survivors were found to have depression at any time-point up to 5 years after stroke (2). We discovered that PSD group showed higher NIHSS score and mRS score and lower BI score, compared to non-PSD group, which indicated the higher severity of disability and stroke in PSD group. Adverse physical conditions may be stressors of developing psychological problems like depression (31). This can explain the cause of PSD to some extent.
Methylenetetrahydrofolate reductase (MTHFR) is an essential enzyme in metabolizing folate. The rs1801133 (also named C677T) is a non-synonymous variant, leading to an amino acid change from alanine to valine. This substitution could reduce the activity of MTHFR and folate levels and elevate serum Hcy levels (19, 32). MTHFR rs1801133 mutations and folate deficiency could increase the risk of coronary heart disease and ischemic stroke in later life (33–36). Folic acid supplementation could prevent birth defects, including neural tube, abdominal wall, and congenital heart defects (37–39). The MTHFR activity of heterozygous and homozygous mutant individuals are respectively 67 and 25% of the wild-type ones (40). Consistently, we found that the Hcy level of stroke patients with rs1801133 AA genotype was higher than those with AG or GG genotype. Our study demonstrated that MTHFR rs1801133 AA genotype and A allele were closely related to early-onset PSD. Mei et al. discovered that mutations of MTHFR rs1801133 AG genotype and A allele were significantly related to the elevated risk of PSD (23), which was partially consistent with our results. The discrepancy may be attributed to the difference in assessment time of PSD and study population like age, gender composition, and so on.
We found that Hcy was significantly related to PSD. Michaela et al. discovered Hcy was linked to depressive symptomatology in elderly Swedish 1 year after stroke (41). Chatterjee et al. found that stroke patients with higher Hcy and lower folate levels may be more susceptible to PSD (42). A meta-analysis reported that the high Hcy level in the acute phase of ischemic stroke may be a risk factor for PSD (43). These are in accordance with our results, and we further revealed that Hcy was regulated by MTHFR rs1801133 to affect PSD.
The association between Hcy and PSD might be explained by several potential pathophysiological pathways. Firstly, Hcy may influence depressive symptoms via alterations of neurotransmitters. Previous research showed that depressed patients with raised Hcy level had decreased serum, red cell, and cerebrospinal fluid folate and cerebrospinal fluid S-adenosylmethionine (44). S-adenosylmethionine not only has antidepressant properties but also acts as the methyl donor involved in methylation reactions of monoamines and neurotransmitters (45–47). Hcy treatment significantly decreased central monoamines levels (norepinephrine, dopamine, and 5-hydroxytryptamine) in post-stroke rats (48). Secondly, Hcy is a toxic substance and can cause damage to the central nervous system. Hcy treatment aggravated depressive-like disorders and contributed to the decrease in the number of synapses and postsynaptic density in post-stroke rats, compared to the control group (48). Thirdly, a strong association between Hcy level and inflammation has been reported in various studies (49–51), and inflammatory processes have been implicated in the pathophysiology of depression (52).
Some limitations of this study should be noted. Firstly, it was an observational study with a relatively small sample size, and patients were only recruited in one clinical institution. The conclusion could not be over-interpreted. Secondly, we excluded those patients with a severe condition and severe aphasia, which would result in biases for the prevalence of PSD. Thirdly, the Hcy level of patients was measured at admission, and the intervals between measurement and stroke onset were different. Fourthly, we did not record the history of taking vitamins B or folic acid. Future researchers need to consider the effect of vitamins B and folate on Hcy level. Fifthly, we only focused on Hcy at admission and PSD at 2 weeks after stroke. Further studies with long-term follow-up samples are required to explore how Hcy levels change during the course of PSD.
In conclusion, our study suggested that Hcy and rs1801133 AA genotype, A allele, and haplotype GTAG (rs11559040-rs1801131-rs1801133-rs2066462) may be associated with the increased risk of early-onset PSD. The rs1801133 may affect early-onset PSD via mediating Hcy level. These results can shed new light on the mechanism of PSD. Clinicians should pay attention to the Hcy level of ischemic stroke patients and evaluate depressive symptoms timely. Hcy-lowering treatment may be a potential therapeutic target for the prevention and intervention of PSD.
Data availability statement
The original contributions presented in this study are included in the article/Supplementary material, further inquiries can be directed to the corresponding authors.
Ethics statement
The study involving human participants were reviewed and approved by the Ethics Committee of Xiangya Hospital of Central South University (No. 201910842). The patients/participants provided their written informed consent to participate in this study.
Author contributions
FT and MS conceived and designed the protocol. JZ, HC, FL, QL, DS, YX, WX, and DZ recruited and evaluated patients. CZ and XH analyzed the data. JZ interpreted the results and wrote the manuscript. All authors contributed to the article and approved the submitted version.
Funding
This work was supported by National Key R&D Program of China (2017YFC1310003) for FT, Natural Science Foundation of Hunan Province (2021JJ41018), and China International Medical Foundation (Z-2016-20-2101-03) for MS.
Acknowledgments
We thank Jun Yang of Second Xiangya Hospital of Department of Psychiatry for assistance with proofreading. We also thank all participants involved in this study and all authors.
Conflict of interest
The authors declare that the research was conducted in the absence of any commercial or financial relationships that could be construed as a potential conflict of interest.
Publisher’s note
All claims expressed in this article are solely those of the authors and do not necessarily represent those of their affiliated organizations, or those of the publisher, the editors and the reviewers. Any product that may be evaluated in this article, or claim that may be made by its manufacturer, is not guaranteed or endorsed by the publisher.
Supplementary material
The Supplementary Material for this article can be found online at: https://www.frontiersin.org/articles/10.3389/fnut.2022.1078281/full#supplementary-material
Footnotes
References
1. Ayerbe L, Ayis S, Wolfe CD, Rudd AG. Natural history, predictors and outcomes of depression after stroke: systematic review and meta-analysis. Br J Psychiatry. (2013). 202:14–21. doi: 10.1192/bjp.bp.111.107664
2. Hackett ML, Pickles K. Part I: frequency of depression after stroke: an updated systematic review and meta-analysis of observational studies. Int J Stroke. (2014) 9:1017–25. doi: 10.1111/ijs.12357
3. Ayerbe L, Ayis S, Crichton S, Wolfe CD, Rudd AG. The long-term outcomes of depression up to 10 years after stroke; the south london stroke register. J Neurol Neurosurg Psychiatry. (2014) 85:514–21. doi: 10.1136/jnnp-2013-306448
4. Paolucci S, Grasso MG, Antonucci G, Troisi E, Morelli D, Coiro P, et al. One-year follow-up in stroke patients discharged from rehabilitation hospital. Cerebrovasc Dis. (2000) 10:25–32. doi: 10.1159/000016021
5. Bates B, Choi JY, Duncan PW, Glasberg JJ, Graham GD, Katz RC, et al. Veterans affairs/department of defense clinical practice guideline for the management of adult stroke rehabilitation care: executive summary. Stroke. (2005) 36:2049–56. doi: 10.1161/01.Str.0000180432.73724.Ad
6. Kelly PJ, Rosand J, Kistler JP, Shih VE, Silveira S, Plomaritoglou A, et al. Homocysteine, Mthfr 677c–>T polymorphism, and risk of ischemic stroke: results of a meta-analysis. Neurology. (2002) 59:529–36. doi: 10.1212/wnl.59.4.529
7. Shi Z, Guan Y, Huo YR, Liu S, Zhang M, Lu H, et al. Elevated total homocysteine levels in acute ischemic stroke are associated with long-term mortality. Stroke. (2015) 46:2419–25. doi: 10.1161/strokeaha.115.009136
8. Wang X, Qin X, Demirtas H, Li J, Mao G, Huo Y, et al. Efficacy of folic acid supplementation in stroke prevention: a meta-analysis. Lancet. (2007) 369:1876–82. doi: 10.1016/s0140-6736(07)60854-x
9. Yahn GB, Leoncio J, Jadavji NM. The role of dietary supplements that modulate one-carbon metabolism on stroke outcome. Curr Opin Clin Nutr Metab Care. (2021) 24:303–7. doi: 10.1097/mco.0000000000000743
10. Gu P, DeFina LF, Leonard D, John S, Weiner MF, Brown ES. Relationship between serum homocysteine levels and depressive symptoms: the cooper center longitudinal study. J Clin Psychiatry. (2012) 73:691–5. doi: 10.4088/JCP.11m07223
11. Moradi F, Lotfi K, Armin M, Clark CCT, Askari G, Rouhani MH. The association between serum homocysteine and depression: a systematic review and meta-analysis of observational studies. Eur J Clin Investig. (2021) 51:e13486. doi: 10.1111/eci.13486
12. Nabi H, Bochud M, Glaus J, Lasserre AM, Waeber G, Vollenweider P, et al. Association of serum homocysteine with major depressive disorder: results from a large population-based study. Psychoneuroendocrinology. (2013) 38:2309–18. doi: 10.1016/j.psyneuen.2013.04.018
13. Saraswathy KN, Ansari SN, Kaur G, Joshi PC, Chandel S. Association of vitamin B12 mediated hyperhomocysteinemia with depression and anxiety disorder: a cross-sectional study among bhil indigenous population of India. Clin Nutr ESPEN. (2019) 30:199–203. doi: 10.1016/j.clnesp.2019.01.009
14. Li Y, Cao LL, Liu L, Qi QD. Serum levels of homocysteine at admission are associated with post-stroke depression in acute ischemic stroke. Neurological. (2017) 38:811–7. doi: 10.1007/s10072-017-2848-2
15. Cheng LS, Tu WJ, Shen Y, Zhang LJ, Ji K. Combination of high-sensitivity c-reactive protein and homocysteine predicts the post-stroke depression in patients with ischemic stroke. Mol Neurobiol. (2018) 55:2952–8. doi: 10.1007/s12035-017-0549-8
16. Yin J, Zhong C, Zhu Z, Bu X, Xu T, Guo L, et al. Elevated circulating homocysteine and high-sensitivity C-reactive protein jointly predicts post-stroke depression among Chinese patients with acute ischemic stroke. Clin Chim Acta. (2018) 479:132–7. doi: 10.1016/j.cca.2018.01.011
17. Alvares Delfino VD, de Andrade Vianna AC, Mocelin AJ, Barbosa DS, Mise RA, Matsuo T. Folic acid therapy reduces plasma homocysteine levels and improves plasma antioxidant capacity in hemodialysis patients. Nutrition. (2007) 23:242–7. doi: 10.1016/j.nut.2007.01.002
18. Chen J, Stampfer MJ, Ma J, Selhub J, Malinow MR, Hennekens CH, et al. Influence of a methionine synthase (D919g) polymorphism on plasma homocysteine and folate levels and relation to risk of myocardial infarction. Atherosclerosis. (2001) 154:667–72. doi: 10.1016/s0021-9150(00)00469-x
19. Frosst P, Blom HJ, Milos R, Goyette P, Sheppard CA, Matthews RG, et al. A candidate genetic risk factor for vascular disease: a common mutation in methylenetetrahydrofolate reductase. Nat Genet. (1995) 10:111–3. doi: 10.1038/ng0595-111
20. Leclerc D, Wilson A, Dumas R, Gafuik C, Song D, Watkins D, et al. Cloning and mapping of a cdna for methionine synthase reductase, a flavoprotein defective in patients with homocystinuria. Proc Natl Acad Sci USA. (1998) 95:3059–64. doi: 10.1073/pnas.95.6.3059
21. Biselli JM, Goloni-Bertollo EM, Haddad R, Eberlin MN, Pavarino-Bertelli EC. The Mtr A2756g polymorphism is associated with an increase of plasma homocysteine concentration in brazilian individuals with down syndrome. Braz J Med Biol Res. (2008) 41:34–40. doi: 10.1590/s0100-879x2006005000195
22. Martínez-Frías ML, Pérez B, Desviat LR, Castro M, Leal F, Rodríguez L, et al. Maternal polymorphisms 677c-T and 1298a-C of Mthfr, and 66a-G Mtrr Genes: is there any relationship between polymorphisms of the folate pathway, maternal homocysteine levels, and the risk for having a child with down syndrome? Am J Med Genet Part A. (2006) 140:987–97. doi: 10.1002/ajmg.a.31203
23. Mei F, Wu Y, Ding G, Pan F, Chen L, Wu J. Association of methylenetetrahydrofolate reductase gene 677c>T polymorphism with post-stroke depression risk and antidepressant treatment response in han Chinese. J Pak Med Assoc. (2018) 68:888–92.
24. Li WX, Dai SX, Zheng JJ, Liu JQ, Huang JF. Homocysteine metabolism gene polymorphisms (Mthfr C677t, Mthfr A1298c, Mtr A2756g and Mtrr A66g) jointly elevate the risk of folate deficiency. Nutrients. (2015) 7:6670–87. doi: 10.3390/nu7085303
25. Wei L, Niu F, Wu J, Chen F, Yang H, Li J, et al. Association study between genetic polymorphisms in folate metabolism and gastric cancer susceptibility in Chinese han population: a case-control study. Mol Genet Genomic Med. (2019) 7:e633. doi: 10.1002/mgg3.633
26. Kumar A, Palfrey HA, Pathak R, Kadowitz PJ, Gettys TW, Murthy SN. The metabolism and significance of homocysteine in nutrition and health. Nutr Metab. (2017) 14:78. doi: 10.1186/s12986-017-0233-z
27. Du W, Cheng J, Ding H, Jiang Z, Guo Y, Yuan HA. Rapid method for simultaneous multi-gene mutation screening in children with nonsyndromic hearing loss. Genomics. (2014) 104:264–70. doi: 10.1016/j.ygeno.2014.07.009
28. Li Z, Zhang Z, He Z, Tang W, Li T, Zeng Z, et al. A partition-ligation-combination-subdivision em algorithm for haplotype inference with multiallelic markers: update of the shesis (Http://Analysis.Bio-X.Cn). Cell Res. (2009) 19:519–23. doi: 10.1038/cr.2009.33
29. Shi YY, He L. Shesis, a powerful software platform for analyses of linkage disequilibrium, haplotype construction, and genetic association at polymorphism loci. Cell Res. (2005) 15:97–8. doi: 10.1038/sj.cr.7290272
30. Chen J, Wang M, Waheed Khan RA, He K, Wang Q, Li Z, et al. The Gsk3b gene confers risk for both major depressive disorder and schizophrenia in the han chinese population. J Affect Disord. (2015) 185:149–55. doi: 10.1016/j.jad.2015.06.040
31. Thabrew H, Stasiak K, Hetrick SE, Donkin L, Huss JH, Highlander A, et al. Psychological therapies for anxiety and depression in children and adolescents with long-term physical conditions. Cochrane Database Syst Rev. (2018) 12:Cd012488. doi: 10.1002/14651858.CD012488.pub2
32. Tsang BL, Devine OJ, Cordero AM, Marchetta CM, Mulinare J, Mersereau P, et al. Assessing the Association between the Methylenetetrahydrofolate Reductase (Mthfr) 677c>T polymorphism and blood folate concentrations: a systematic review and meta-analysis of trials and observational studies. Am J Clin Nutr. (2015) 101:1286–94. doi: 10.3945/ajcn.114.099994
33. Wang ZM, Zhou B, Nie ZL, Gao W, Wang YS, Zhao H, et al. Folate and risk of coronary heart disease: a meta-analysis of prospective studies. Nutr Metab Cardiovasc Dis. (2012) 22:890–9. doi: 10.1016/j.numecd.2011.04.011
34. Klerk M, Verhoef P, Clarke R, Blom HJ, Kok FJ, Schouten EG. Mthfr 677c–>T polymorphism and risk of coronary heart disease: a meta-analysis. Jama. (2002) 288:2023–31. doi: 10.1001/jama.288.16.2023
35. Qin X, Spence JD, Li J, Zhang Y, Li Y, Sun N, et al. Interaction of serum vitamin B(12) and folate with mthfr genotypes on risk of ischemic stroke. Neurology. (2020) 94:e1126–36. doi: 10.1212/wnl.0000000000008932
36. Zhu XY, Hou RY, Pan XD, Wang YC, Zhang ZS, Guo RY. Association between the methylenetetrahydrofolate reductase (Mthfr) Gene C677t polymorphism and ischemic stroke in the Chinese population: a meta-analysis. Int J Neurosci. (2015) 125:885–94. doi: 10.3109/00207454.2014.984295
37. Liu J, Li Z, Ye R, Liu J, Ren A. Periconceptional folic acid supplementation and sex difference in prevention of neural tube defects and their subtypes in china: results from a large prospective cohort study. Nutr J. (2018) 17:115. doi: 10.1186/s12937-018-0421-3
38. Liu J, Li Z, Ye R, Ren A, Liu J. Folic acid supplementation and risk for fetal abdominal wall defects in china: results from a large population-based intervention cohort study. Br J Nutr. (2021) 126:1558–63. doi: 10.1017/s0007114521000337
39. Mao B, Qiu J, Zhao N, Shao Y, Dai W, He X, et al. Maternal folic acid supplementation and dietary folate intake and congenital heart defects. PLoS One. (2017) 12:e0187996. doi: 10.1371/journal.pone.0187996
40. Wan L, Li Y, Zhang Z, Sun Z, He Y, Li R. Methylenetetrahydrofolate reductase and psychiatric diseases. Transl Psychiatry. (2018) 8:242. doi: 10.1038/s41398-018-0276-6
41. Pascoe MC, Crewther SG, Carey LM, Noonan K, Crewther DP, Linden T. Homocysteine as a potential biochemical marker for depression in elderly stroke survivors. Food Nutr Res. (2012) 56:14973. doi: 10.3402/fnr.v56i0.14973
42. Chatterjee K, Fall S, Barer D. Mood after stroke: a case control study of biochemical, neuro-imaging and socio-economic risk factors for major depression in stroke survivors. BMC Neurol. (2010) 10:125. doi: 10.1186/1471-2377-10-125
43. Chen Y, Zou H, Peng M, Chen Y. Association between homocysteine levels in acute stroke and poststroke depression: a systematic review and meta-analysis. Brain Behav. (2022) 12:e2626. doi: 10.1002/brb3.2626
44. Bottiglieri T, Laundy M, Crellin R, Toone BK, Carney MW, Reynolds EH. Homocysteine, folate, methylation, and monoamine metabolism in depression. J Neurol Neurosurg Psychiatry. (2000) 69:228–32. doi: 10.1136/jnnp.69.2.228
45. Aishwarya S, Rajendiren S, Kattimani S, Dhiman P, Haritha S, Ananthanarayanan PH. Homocysteine and serotonin: association with postpartum depression. Asian J Psychiatry. (2013) 6:473–7. doi: 10.1016/j.ajp.2013.05.007
46. Bottiglieri T. Homocysteine and folate metabolism in depression. Prog Neuropsychopharmacol Biol Psychiatry. (2005) 29:1103–12. doi: 10.1016/j.pnpbp.2005.06.021
47. Reynolds E. Vitamin B12, folic acid, and the nervous system. Lancet Neurol. (2006) 5:949–60. doi: 10.1016/s1474-4422(06)70598-1
48. Wang M, Liang X, Zhang Q, Luo S, Liu H, Wang X, et al. Homocysteine can aggravate depressive like behaviors in a middle cerebral artery occlusion/reperfusion rat model: a possible role for nmdars-mediated synaptic alterations. Nutr Neurosci. (2022) [Online ahead of print]. doi: 10.1080/1028415x.2022.2060642.
49. Álvarez-Sánchez N, Álvarez-Ríos AI, Guerrero JM, García-García FJ, Rodríguez-Mañas L, Cruz-Chamorro I, et al. Homocysteine and C-reactive protein levels are associated with frailty in older spaniards: the toledo study for healthy aging. J Gerontol A Biol Sci Med Sci. (2020) 75:1488–94. doi: 10.1093/gerona/glz168
50. Fang P, Li X, Shan H, Saredy JJ, Cueto R, Xia J, et al. Ly6c(+) inflammatory monocyte differentiation partially mediates hyperhomocysteinemia-induced vascular dysfunction in type 2 Diabetic Db/Db Mice. Arteriosc Thromb Vasc Biol. (2019) 39:2097–119. doi: 10.1161/atvbaha.119.313138
51. Liu Z, Luo H, Zhang L, Huang Y, Liu B, Ma K, et al. Hyperhomocysteinemia exaggerates adventitial inflammation and angiotensin ii-induced abdominal aortic aneurysm in mice. Circ Res. (2012) 111:1261–73. doi: 10.1161/circresaha.112.270520
Keywords: post stroke depression, homocysteine, single nucleotide polymorphisms, MTHFR, MTRR, MTR
Citation: Zhang J, Zeng C, Huang X, Liao Q, Chen H, Liu F, Sun D, Luo S, Xiao Y, Xu W, Zeng D, Song M and Tian F (2022) Association of homocysteine and polymorphism of methylenetetrahydrofolate reductase with early-onset post stroke depression. Front. Nutr. 9:1078281. doi: 10.3389/fnut.2022.1078281
Received: 24 October 2022; Accepted: 21 November 2022;
Published: 06 December 2022.
Edited by:
Jufen Liu, Peking University, ChinaReviewed by:
Jolanta Dorszewska, Poznań University of Medical Sciences, PolandIsis Trujillo-Gonzalez, University of North Carolina at Chapel Hill, United States
Copyright © 2022 Zhang, Zeng, Huang, Liao, Chen, Liu, Sun, Luo, Xiao, Xu, Zeng, Song and Tian. This is an open-access article distributed under the terms of the Creative Commons Attribution License (CC BY). The use, distribution or reproduction in other forums is permitted, provided the original author(s) and the copyright owner(s) are credited and that the original publication in this journal is cited, in accordance with accepted academic practice. No use, distribution or reproduction is permitted which does not comply with these terms.
*Correspondence: Fafa Tian, dGlhbmZmNDB3QDE2My5jb20=; Mingyu Song, c29uZ21pbmd5dTE5ODZAMTI2LmNvbQ==