- 1Division of Genetics, Indian Council of Agricultural Research (ICAR)-Indian Agricultural Research Institute (IARI), New Delhi, India
- 2Department of Botany, Deshbandhu College, University of Delhi, New Delhi, India
- 3Department of Botany, Maitreyi College, University of Delhi, New Delhi, India
- 4Faculty of Agricultural Sciences, Institute of Applied Sciences and Humanities, GLA University, Mathura, Uttar Pradesh, India
- 5Department of Botany, Hansraj College, University of Delhi, New Delhi, India
- 6School of Biotechnology, Institute of Science, Banaras Hindu University (BHU), Varanasi, Uttar Pradesh, India
- 7Indian Council of Agricultural Research (ICAR)-National Institute for Plant Biotechnology (NIPB), New Delhi, India
- 8Department of Botany, Institute of Science, Banaras Hindu University (BHU), Varanasi, Uttar Pradesh, India
- 9Centre for Plant Breeding and Genetics, Tamil Nadu Agricultural University, Coimbatore, Tamil Nadu, India
- 10Division of Genetics, Indian Council of Agricultural Research (ICAR)-Indian Agricultural Research Institute (IARI), Regional Research Centre, Dharwad, Karnataka, India
- 11Department of Plant Breeding and Genetics, Tamil Nadu Rice Research Institute, Tamil Nadu Agricultural University, Aduthurai, Tamil Nadu, India
- 12Division of Plant Physiology, Indian Council of Agricultural Research (ICAR)-Indian Agricultural Research Institute, New Delhi, India
Angiotensin-converting enzyme I (ACE I) is a zinc-containing metallopeptidase involved in the renin-angiotensin system (RAAS) that helps in the regulation of hypertension and maintains fluid balance otherwise, which results in cardiovascular diseases (CVDs). One of the leading reasons of global deaths is due to CVDs. RAAS also plays a central role in maintaining homeostasis of the CV system. The commercial drugs available to treat CVDs possess several fatal side effects. Hence, phytochemicals like peptides having plant-based origin should be explored and utilized as alternative therapies. Soybean is an important leguminous crop that simultaneously possesses medicinal properties. Soybean extracts are used in many drug formulations for treating diabetes and other disorders and ailments. Soy proteins and its edible products such as tofu have shown potential inhibitory activity against ACE. Thus, this review briefly describes various soy proteins and products that can be used to inhibit ACE thereby providing new scope for the identification of potential candidates that can help in the design of safer and natural treatments for CVDs.
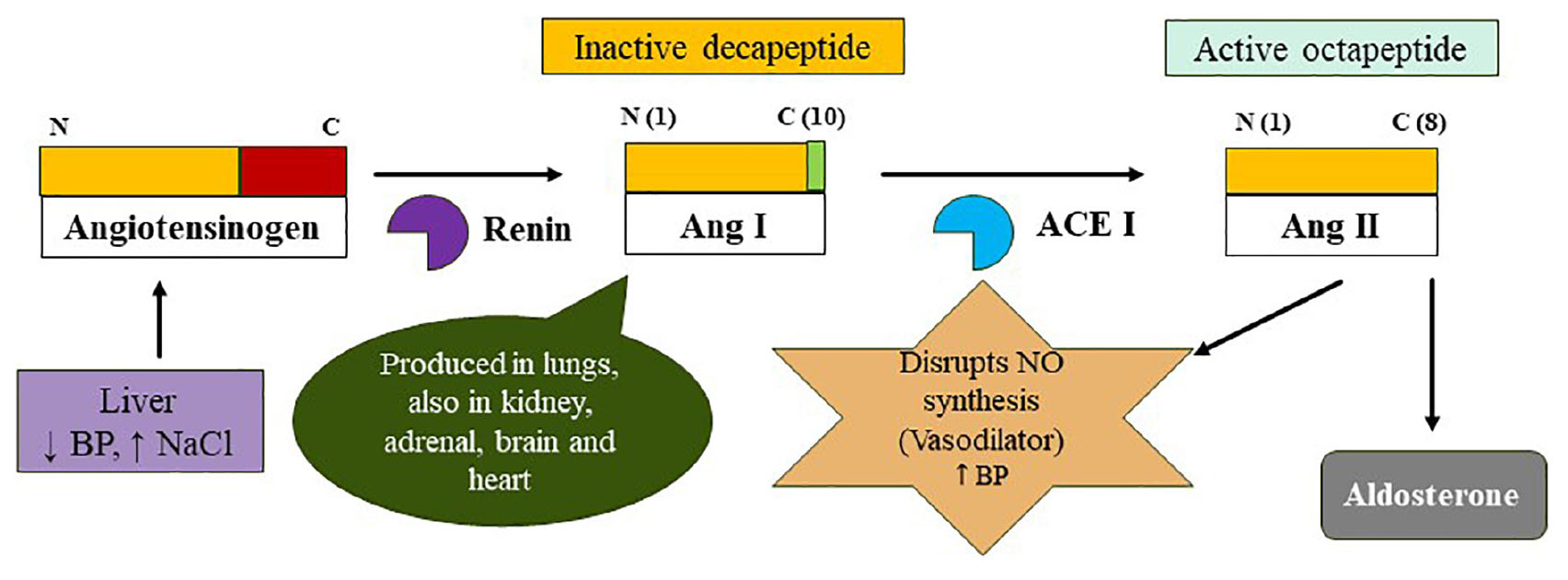
Graphical Abstract 1. The classical RAAS summarizes the process of formation of biologically active octapeptide angiotensin II. The degradation is a two-step process wherein the renin cleaves N-terminal of Angiotensinogen (AGT) to form Angiotensin I (Ang I; 10-amino acid long and biologically inactive) and in the second step, angiotensin-converting enzyme (ACE I) cleaves Ang I to yield an octapeptide angiotensin II (biologically active). The renin is released by the JG cells when the volume of circulating blood is reduced while the AGT is constitutively released by the liver. The Ang I is acted by the ACE I to form ACE II which disrupts the synthesis of nitric oxide (NO) and it also recruits aldosterone to elevate the blood pressure.
Introduction
Cardiovascular diseases (CVDs) are leading cause of mass mortality around 17.9 million deaths each year (1). The primary reason for CVDs is hypertension which affects vital organs like the brain and kidneys. Several other pathophysiological processes also occur simultaneously which include stiffening of large ducts (aorta, carotid artery) and elastic artery, smooth muscle cell proliferation, vasoconstriction, and dysfunction of the endothelium (2). The renin-angiotensin-aldosterone system (RAAS) helps in the regulation of fluid balance and plays a crucial part in maintaining homeostasis of the cardiovascular system and normalizing blood pressure (BP) (3). One of the components of the RAAS is the angiotensin-converting enzyme (ACE). ACE was first isolated in 1956 from rat kidneys (4). It is essentially required for the regulation of the formation of angiotensin II (Ang II) from its precursor molecule angiotensin I (Ang I) and in turn, increases BP. The inhibitors aid in the regulation of blood pressure levels by inhibiting the formation of Ang II and thereby prevent CVDs (4–11). Renin is the primary enzyme involved in the RAAS and is required for the formation of Ang I and is released into the bloodstream. This enzyme cleaves a stretch of 10 amino acid residues from the N-terminal region of angiotensinogen leading to the production of Ang I. This peptide is acted upon by ACE which then forms Ang II and stimulates the release of aldosterone, which eventually elevates BP (3, 5, 9, 12). Simultaneously, the degradation of a potent vasodilator, bradykinin is also catalyzed (2). BP is a complex process involving a series of steps with the involvement of multiple organs, and the autonomic nervous system (ANS), vasopressor and vasodepressor hormones, the total volume of body fluid, renal function, and the vasculature. The endothelium is directly involved in controlling BP by producing multiple vasodilators and vasoconstrictors such as nitric oxide (NO) (2, 13, 14).
The ACE inhibitors (ACEIs) bind to ACE competitively thereby restricting the Ang I to Ang II conversion thus limiting the circulation of Ang II. There are various commercially available synthetic ACEIs are classified as carboxyl containing such as Lisinopril, Benazepril, Perindopril, Cilazapril, Quinapril, Ramipril, and trandolapril contain greater lipophilicity over other ACEIs, other group that include phosphoryl containing such as Fosinopril. These ACEIs groups have replaced the sulphydryl group containing first ACEI (Captopril) that cause skin rashes, proteinuria and disturbed taste along with several other effects including headache, hyperkalemia, nausea, fatigue, dizziness, swelling of the lower portion of the skin, cough, and angioneurotic edema (3, 15). Plant compounds have been reported to have ACE inhibitory activities as reviewed by Patten et al. (16) that do not cause such severe side effects and are safe for consumption. The plant-based bioactives are better alternatives to synthetic drugs due to their non-toxic, ease of availability, and no side effects (13, 14, 16, 17). Therefore, there is a need to switch and find alternative natural or plant-based sources, especially from food proteins (protein-derived bioactive peptides) having promising health-promoting benefits with less or no side effects (17–20). Previously, many ACEIs have been identified from legumes. For instance, Akıllıoğlu and Karakaya (21), inhibitory activity of ACE was observed using common and pinto beans and green lentil, similarly, Roy et al. (22) analyzed pea, chickpea and lentil. Boschin et al. (23) analyzed the enzymatic protein hydrolysate activity for ACE using Lupinus albus L., pea, lentil, chickpea, common bean, and soybean. Lentil, black soybean and black turtle bean were tested for the presence of ACE inhibitory effects by Zhang and Chang (24) using in vitro gastrointestinal proteolytic digestion (24) and Bollati et al. (25) used pea. Among legumes, one such wonder and multipurpose crop is the soybean.
Glycine max (L.) Merr. (Soybean) is one of the economically important and versatile legumes. It contains a plethora of nutritional compounds including proteins (40–42%), and lipids (15–20%) including polyunsaturated fatty acids (PUFA), alpha-linolenic acids, vitamins, and minerals (26–30). Soybean is native to East Asia, probably North and Central parts of Asia and it has been cultivated in China for 4,000 years (31). Soybean has been widely associated with reducing BP and obesity. It shows anti-cholesterol activity by lowering both genic and non-genic origin-based hypercholesterolemic and triglycerides, thus reducing the risk of CVD along with it is also reducing postmenopausal symptoms, and risk of osteoporosis and antimutagenic effects (32). It also possesses hypotensive activities like inhibition of ACE I, and anti-microbial and anti-thrombotic activities (33). Soybean extracts serve as a primary ingredient in many drug formulations curing various deadly diseases. For instance, cyanidin-3-glucoside (an anthocyanin) found in black soybean helps in treating diabetes and obesity. It has also been shown to act as an antineoplastic agent and helps in scavenging free radicals (32, 34). It possesses anti-inflammatory and anti-proliferative effects on human HT29 colon cancer cells and human leukemia Molt 4Bcells (32, 35, 36). The Bowman–Birk protease inhibitor (BBI) and its concentrate (BBIC) when administered orally lowers inflammation and demyelination of the spinal cord (37, 38) and lunasin is used as anti-inflammatory and anti-cancer peptide (39, 40). Soybean is a high proteinaceous legume, it acts as an ideal source for the identification of bioactive peptides against hypertension with other effects (10). Recently, Ramlal et al. (28) showed that soybean isoflavonoids especially Genistein can also be used against ACE (28).
The current article aims to emphasize various ACE inhibitors identified in soy proteins and fermented foods that would eventually be helpful in the identification and development of novel functional food additives and useful in the design of safer drugs for ACE inhibition.
Angiotensin-converting enzyme I: The key player
Angiotensin-converting enzyme I (ACE I) with EC 3.4.15.1 is a zinc-containing chloride-dependent peptidyl-dipeptidase. A enzyme (15, 41, 42) and helps in maintaining the homeostasis of the cardiovascular system (Figure 1) (43). Two isoforms of ACE exist; the somatic ACE (sACE) and testicular ACE (tACE) which contain one and two catalytically active domains, respectively, referred to as N and C termini in the sACE (called cACE and nACE). Although, the two domains exhibit high sequence and structural similarity, show distinct substrate specificity and inhibitor binding mechanisms (5, 44). The structure of ACE consists of 27 helices which include 20 alpha−helices and seven 310 helices, and six short beta strands (42). The ACE is an important component of the RAAS system (45). RAAS is an endocrine system that balances systemic BP and maintains the balance of fluid-electrolytes (46, 47). The pathway begins in the juxtaglomerular cells (JG) as it helps in the biosynthesis of renin in the renal glomerulus. Initially, renin is an immature preprohormone (prorenin) following this bioactive is formed through the proteolytic cleavage of 43 amino acids from the N-terminal (3). The first rate-limiting step in the pathway is the N-terminal cleavage of AGT by the renin forming an inactive decapeptide Ang I or Ang (1–10). AGT is stored primarily in the liver (3, 48), it is constitutively secreted (thus plasma levels remain normal), however, its expression is also observed in other tissues like the adrenal gland, heart, brain, kidney, vascular, adipose tissue, placenta and ovary, and also in vascular endothelial cells (3, 49–51). This biologically inert decapeptide Ang I is then hydrolyzed to form octapeptide Ang II [Ang (1–8)] by the enzyme called ACE I. This octapeptide is a potent vasoconstrictor and biologically very active (3), as depicted in Figure 1. Ang II acts directly on vessels and thereby stimulates vasoconstriction leading to an increase in BP. Similarly, it also acts on adrenal glands to stimulate the release of aldosterone. The released aldosterone further acts on the kidneys to stimulate the reabsorption of water and NaCl, thereby increasing blood volume and pressure due to renal and systemic arterioles constrictions (3, 49).
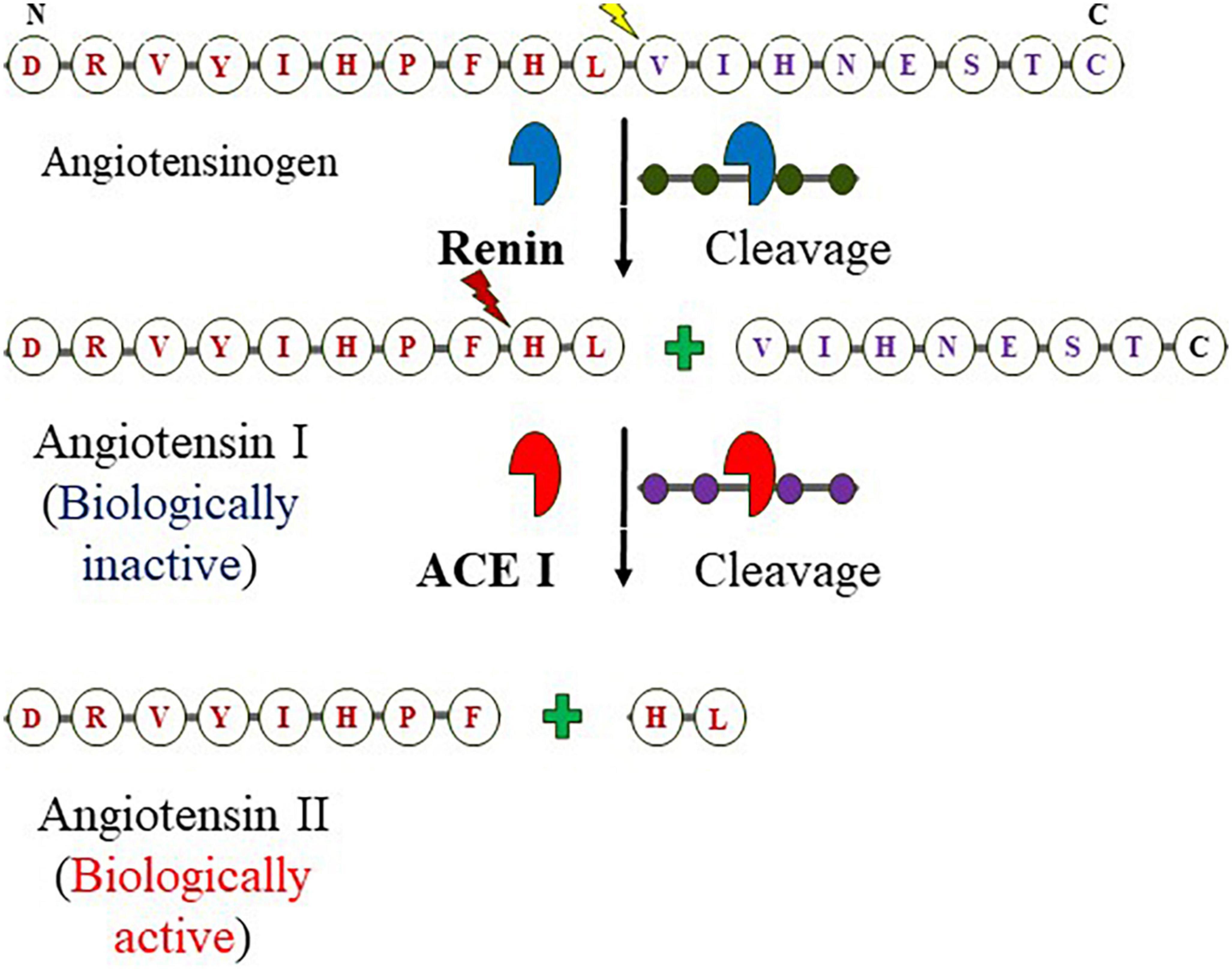
Figure 1. Components of RAAS and formation of angiotensin II from angiotensinogen: The enzymatic reaction cleaves off N-terminal of angiotensinogen by the kidney-derived enzyme renin at Leucine–Valine to form angiotensin I (decapeptide) which is in turn digested by the endothelium-bound angiotensin-converting enzyme (ACE I) at Phenylalanine-Histidine yields the octapeptide.
Angiotensin-converting enzyme inhibitors derived from soybean and its various products
There are many conventional and modern methods used for the prediction, identification, and discovery of biologically active peptides. The conventional approaches involve the selection of enzymes and proteins for proteolysis, fractionation, and identification followed by analysis. However, the modern or in silico based-approach includes virtual screening, and structure-function analysis through various computational methods followed by molecular docking studies (52). Food-derived peptides derived from functional food products are nowadays being used for the identification of novel inhibitors of ACE (53). Using the conventional and in silico approaches, various groups have discovered and identified many peptides showing inhibitory action against the ACE which are being discussed below.
There are many in vitro assays which are being used to investigate the activity of ACEIs based on the substrate used which include Cushman and Cheung Method using a substrate hippuryl-histidyl-leucine (HHL) (54), Carmel and Yaron method used substrate o-aminobenzoylglycyl-p-nitrophenylalanilproline (55), Holmquist method using a substrate furanacryloyl-tripeptide (56), Elbl and Wagner method considered benzoil-[l-14C] glicyl-L-histidine-L-leucine as a substrate (57), and Lam method using 3-hydroxybutyrylglycyl-glycyl-glycine as substrate (58). Several different methods to measure the results of enzymatic reactions or separating substrate with products, including spectrophotometric, fluorometric, high-performance liquid chromatography (HPLC), electrophoresis, and radiochemistry (59). Table 1 describes and summarizes the ACEIs so far from soybean which are mostly identified using the enzymatic processes and also through in silico molecular docking approaches.
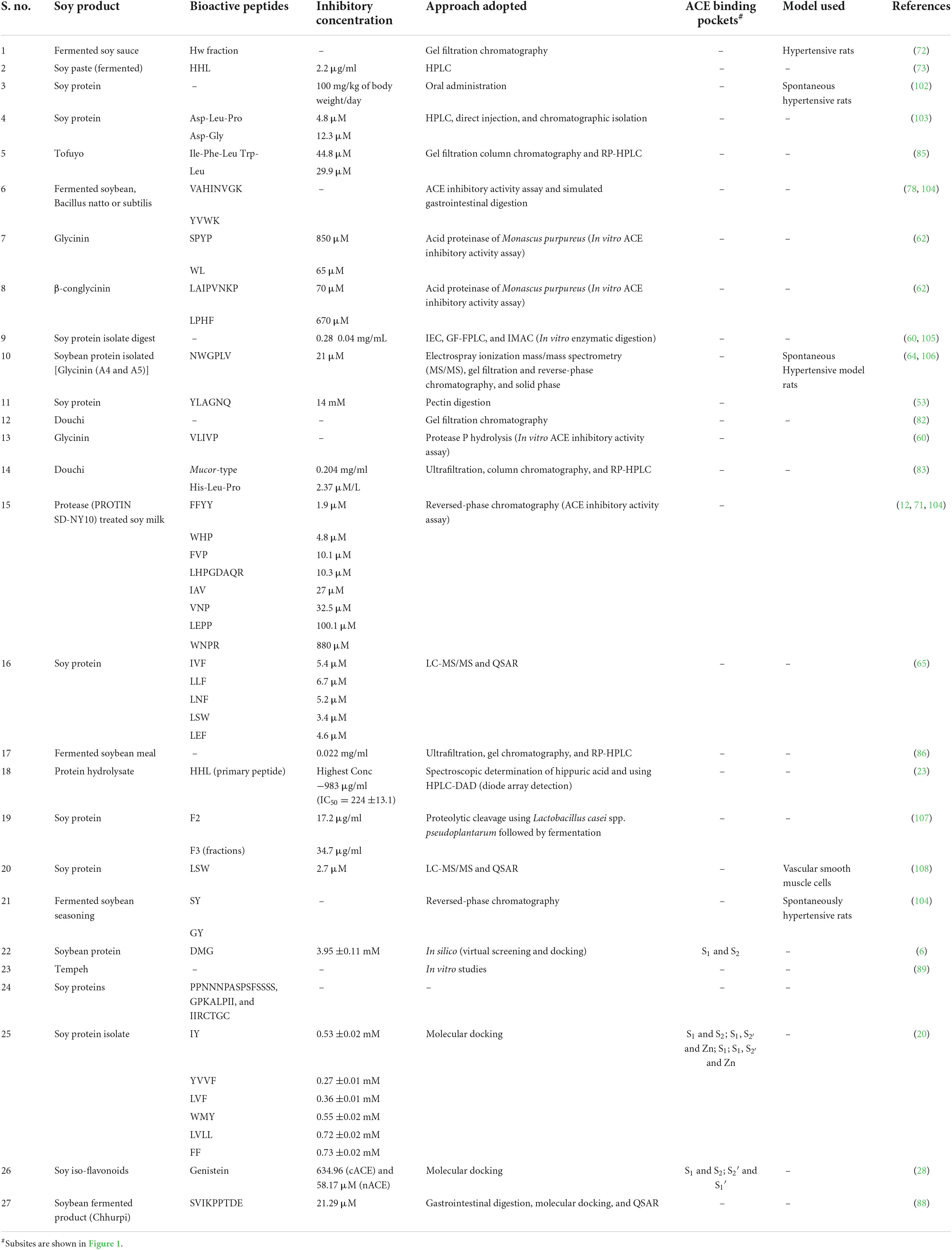
Table 1. Summary of inhibitory soybean bioactive peptides with their IC50 values and interacting ACE subsites.
The various soy proteins such as (proteins, protein isolates, and hydrolysates), soy foods (milk, sauce, paste, and other products), fermented products (fermented seasoning, tempeh, douchi, tofuyo, meal, extract, and chhurpi), and similarly, soy isoflavonoids showing the ACE inhibitory activities have been summarized below.
Soybean proteins
Zhao et al. (6) identified around 161 novel tripeptides using soybean hypothetical protein sequences (NCBI: KRH47534.1). Based on the toxicity and solubility studies, only 12 potential peptides (DTW, EGW, RPR, CIR, DMG, AGR, MDL, HDW, MDY, DVF, and LPR) were selected. ACE inhibitory activity analysis carried out using reversed-phase (RP)-HPLC showed that DMG, out of the 12 tripeptides selected was the most effective peptide with IC50 value of 3.95 ±0.11 mM. The docking revealed that the DMG peptide interaction with the active amino acids of the S1 and S2 subsites of the ACE (6).
Glycinin is obtained through enzymatic hydrolysis using protease P from the soybean and is considered a potential and potent ACE inhibitor. One such potent inhibitor includes protease P glycinin hydrolysate with sequence VLIVP (primary peptide). The inhibitor uses the peptic digestion of the soy protein the IC50 was found to be 14 μM for the peptide YLAGNQ (53, 60, 61). The peptides formed from the hydrolysis of β-conglycinin and glycinin through the acid proteinase of Monascus purpureus include SPYP (IC50 = 850 μM) and WL (IC50 = 65 μM) and LAIPVNKP (IC50 = 70 μM) and LPHF (IC50 = 670 μM), respectively (61, 62). Furthermore, using the Edman’s process and peptic digestion of the soybean protein hydrolysates, many ACE inhibitors were identified which include IA, YLAGNQ, FFL, IYLL, and VMDKPQG having IC50 values 153, 14, 37, 42, and 39 μM, respectively (61, 63).
Soybean protein isolate (SPI) is an abundant low-cost protein source and was known to possess many inhibitory peptides from SPI hydrolysates namely DLP, LSW, DG, and NWGPLV (IC50 = 21 μM) (20). Around eight novel inhibitory peptides were identified and among them, NWGPLV was found to be the most potent peptide which was treated with D3 protease obtained from soybean (64). Using the approach of LC-MS/MS along with the QSAR model, soybean protein hydrolysate treated with pepsin and thermolysin yielded IC50 values 51.8 and 53.6 μg/mL, respectively, and also identified five novel tripeptides having potential inhibitory activities (shown in Table 1) (65). Many di and tripeptides were enzymatically isolated using trypsin, pepsin, and thermolysin from soybean protein and calculated IC50 values of 33 such peptides (65). Xu et al. (20) have identified many novel peptides with inhibitory activities against ACE. Peptides with good results include LVF, WMY, IY, FF, YVVF, WMY, and LVLL with significant hydrophobic and predicted activity scores. IY binding with the active sites and occurring in subsites S1 and S2 and WMY (S1, and interacting with Zn) were found to be potent inhibitors for the enzyme. YVVF and LVLL occur in the subsites S1 and and form hydrogen bonds with the catalytic site zinc ion. whereas LVF occurs in S1 (20). Although, Rudolph et al. (66) identified many ACE inhibitors including IY with IC50 = 5.2 ±1.4 μM/L which was higher than that shown by Xu et al. (20). The soy peptides also show anticancerous activities (67) and may reduce many severe physiological (age-dependent) diseases (68).
Soy protein hydrolysates (SPHs)—The SPHs are obtained through a sequential processing of soy proteins with different methods (hydrolysis, thermal treatment, gastrointestinal digestion, and microbial fermentation) yielding a mixture of peptides (69). The soy proteins have shown good inhibitory activities and are widely being used as potential functional foods which can be commercialized to use for ACE inhibition. Studies done by Daliri et al. (70) have reported ACE inhibitory activity using soybean protein hydrolysates (70) and similarly, Bollati et al. (25) reported ACE activity with an IC50 = 0.33 0.01 mg/ml (25).
Soyfoods
Processed soy milk—The PSM was used for the identification of ACE inhibitory peptides. It was digested by the bacterial proteases and obtained eight novel peptides showing activity against the enzyme ACE. Among them, two peptides namely, FFYY and WHP found to be more suitable than others, and therefore, PSM could act as a good source for the development of antihypertensive drugs and it is, in turn, a suitable candidate food (71). A similar study carried out by Shimakage et al. (12) who identified eight novel peptides including FFYY, WHP, FVP, LHPGDAER, IAV, VNP, LGPP, and WNPR with IC50 1.9, 4.8, 10.1, 10.3, 27.0, 32.5, 100.1, and 880.1 μM possess inhibitory activity against the ACE. It was observed that FFYY and WHP were more potent inhibitors than others (12).
Soy sauce—Fermented soy sauce using the Japanese method was used to extract antihypertensive peptides. Two fractions were obtained as high (Hw) and low (Lw) molecular weight using gel filtration chromatography. It was observed that the Hw fraction showed inhibitory properties when orally given to rats (72).
Soy paste—Fermented soybean paste was used for the identification of ACE inhibitors. The in vitro analysis showed that Korean soy paste can inhibit ACE. A novel peptide was isolated and identified with IC50 2.2 μg/ml for HHL (73). Li et al. showed the similar reports (74).
Other soy products (milk, yogurt, and natto)—Other works carried out using raw, steamed, and soaked soybean and different natto samples revealed they can be potential functional foods and showed ACE inhibitory activity (75). Soy food products including natto, soy yogurt, soymilk, tempeh, and tofu showed inhibitory activity against ACE after in vitro gastrointestinal digestion while traditional soymilk both raw and cooked showed the highest antihypertensive inhibitory activity. Among fermented soy foods, tempeh showed the least inhibitory activity than natto and soy yogurt which depicted higher inhibition. It also reported that two major proteins namely 7S and 11S of soybean also showed effective ACE inhibition (7). A Japanese traditional fermented food, natto is prepared by fermenting it with boiled soybeans and Bacillus natto and found to be effective against hypercholesterolemia, arteriosclerosis, and hypertension. Previous studies showed that spontaneous hypertension rats (SHRs) were fed with not boiled natto showed decreased blood pressure while Okamoto et al. (76) showed that the ACE inhibitory potential is gained through boiling and fermentation processes. Fermented milk is also known to possess inhibitory activities against the ACE, the studies carried out by Fan et al. (77), Hernández-Ledesma et al. (78), and (77–79).
Fermented soy products
Fermented soybean seasoning (FSS) or Soy sauce-like seasoning—The FSS was modified from the soy sauce’s normal production. It was more concentrated than that of normal sauce and contain 2.7 folds more peptides and in terms of inhibition IC50 for FSS found to be 454 μM and regular soy sauce IC50 was 1,620 μM. The peptides isolated were AW IC50 = 10 μM, GW IC50 = 30 μM, AY IC50 48 μM, SY IC50 67 μM, GY IC50 = 97 μM, AF IC50 = 190 μM, VP IC50 = 480 μM, AI IC50 = 690 μM, VG IC50 = 1,100 μM, and nicotianamine IC50 = 0.26 μM (80). The potent peptides among these were found to be GY and SY in the rat models used (81).
Douchi—Douchi is a Chinese recipe made of fermented soybean and is in various traditional medicines. Soy paste and sauce have also been prepared from douchi. Douchi was fermented along with Aspergillus egypticus and the peptides were analyzed thereon. It showed better results against ACE inhibition (82). Another study showed that Mucor-type douchi (Yongchuan douchi), one of the three types of douchi prepared in China with IC50 value 0.204 mg/ml. The peptide which was isolated was found to be HLP (His-Leu-Pro) with a 50% inhibitory concentration of 2.37 μmol/L (83). Similar results were reported by Li et al. (84).
Tofuyo—It is a traditional Chinese fermented prepared from tofu similar to that of cream cheese. IFL and YL were isolated from the tofuyo using gel filtration and RP HPLC methods with IC50 44.8 and 29.9 μM, respectively (85).
Fermented soybean meal—Using the Bacillus subtilis natto, fermentation and proteolysis were carried out. The inhibitory activity was found to be 84.1% with IC50 value of 0.022 mg/ml (86).
Fermented soybean extract—The composition of the inhibitory peptide is LVQGS, isolated using the Edman degradation method with IC50 value 22 μg/mL while the inhibitory activity of the fermented extract was obtained as 1.46 mg/ml (87).
Chhurpi—Soy chhurpi is a product prepared using the fermented soymilk and proteolytic Lactobacillus delbrueckii WS4. With the help of gastrointestinal digestion, molecular docking, and QSAR, a glycinin-derived peptide was identified, SVIKPPTDE with an IC50 value of 21.29 μM and reported the first production of chhurpi soy cheese (88).
Tempeh—It is an Indonesian dish made from fermented soybean alongside Rhizopus sp. It is shown to possess health-promoting benefits for humans. Tempeh inhibits angiogenesis during cancer, improves bone’s health, and acts as an antioxidant and anti-bacterial agent. It is also useful in treating Alzheimer’s disease and dementia. Chalid et al. through in vitro analysis showed that tempeh has inhibitory activity (89). Tempeh derived-isoflavonoid, genistein showed anti-angiogenesis properties (89, 90).
Soy isoflavonoids
Flavonoids are polyphenolic secondary metabolites primarily found in plants and some bacteria. They play a wide variety of functions in plants from signaling molecules, phytoalexins, detoxifying agents, stimulating germination of spores and seeds and acting as attractants of pollinators, and many others. One of the three categories of flavonoids include isoflavonoids (91). Recently, to identify natural compounds which could act as inhibitors of ACE plant-derived polyphenolics, peptides, and terpenes are being explored owing to their pharmacological properties (92) including isoflavonoids. Isoflavones are abundant in soybean and act as health enhancers. Daidzein, Glycitein, and Genistein are the primary isoflavones in soybean. They help in the prevention of cancers, reduce the level of cholesterol, and lower hypertension (93). Previous studies carried out on soybean isoflavonoids (94–97) showed that these isoflavonoids have inhibitory action against ACE and aid in protection from renal diseases (98). The soy isoflavones resemble mammalian estrogen (phytoestrogen). Ramlal et al. (28) have shown the molecular basis of the selectivity of isoflavones from soybean namely Genistein, Glycitein, and Daidzein as ACE inhibitors through in silico molecular docking approaches. According to the study, Genistein was found to be more potent as compared to the others having more hydrogen bonds and hydrophobic interactions with the catalytic subsites ensuring a tight binding which is further correlated with the observed inhibition constants. It was observed that Genistein is a moderate cACE but selective nACE inhibitor (inhibition constants 634.96 and 58.17 μM, respectively) while the other two isoflavones Daidzein and Glycitein exhibited selective inhibition profiles for the N domain of ACE (inhibition constants 47.37 and 228.5 μM, respectively) (28).
Discussion
Although, the common medication for the treatment of CVDs and renal diseases including heart failures and hypertension the commercial drugs are preferred. However, despite the lower rates of success due to prolonged treatment procedures, persistent side effects (angioedema, cough), and no one-time remedy of the commercially available drugs (Enalapril, ramipril, and similarly captopril, perindopril, and lisinopril) are recommended for the initial therapy (15). Furthermore, with the outbreak of coronavirus, it has been shown that the virus uses ACE II as its receptor to invade the cells (99). Moreover, since the outbreak of the pandemic and even before the occurrence, herbal medicines were the preferred choice over synthetic drugs due to their side effects (100, 101). Therefore, phytocompounds are being searched for their inhibitory activity against ACE as an alternative therapy. This article describes and highlights the various ACE inhibitory obtained from proteins and isoflavonoids from soybean signifying the importance in the treatment of hypertension and heart-related problems for making future drugs.
Conclusion and future prospects
Angiotensin-converting enzyme is a key enzyme in the RAAS which helps in the regulation of hypertension. The overproduction of angiotensin by the activity of ACE leads to a medical condition known as hypertension and also due to the consumption of synthetic drugs which lead to cause side effects and sometimes even death. Therefore, it becomes very important to control or inhibit the ACE to control/treat hypertension using the phytocompounds like saponins, terpenes, and isoflavonoids. The clinical and therapeutic importance of ACE inhibitors is well understood. The identification of phytocompounds with potential ACE inhibitor activity can be good alternative for chemical drugs because of no or minimum side effects than the latter ones. The article provides a clue to researchers like plant breeders who can breed and develop specialty soybean varieties meant to provide ACE inhibiting compounds. It also provides a hint to the pharmacy sector to capitalize the soybean phytocompounds as ACE inhibitors in place of synthetic drugs. Therefore, natural compounds, and other phytoconstituents should be searched for their inhibitory activity against ACE for a safer alternative and future drug design.
Author contributions
AyR and AmR contributed to the conception and design of the study. AyR wrote the first draft and curated the data. All authors equally contributed to the manuscript revision, editing, APC, read, and approved the published version.
Acknowledgments
AyR and AmR thank Urvashi Sharma, Ramalingaswami Fellow, Institute of Bioinformatics and Applied Biotechnology (IBAB), Bengaluru, India for the preparation of the manuscript and figure.
Conflict of interest
The authors declare that the research was conducted in the absence of any commercial or financial relationships that could be construed as a potential conflict of interest.
Publisher’s note
All claims expressed in this article are solely those of the authors and do not necessarily represent those of their affiliated organizations, or those of the publisher, the editors and the reviewers. Any product that may be evaluated in this article, or claim that may be made by its manufacturer, is not guaranteed or endorsed by the publisher.
References
1. Roth GA, Mensah GA, Johnson CO, Addolorato G, Ammirati E, Baddour LM, et al. Global burden of cardiovascular diseases and risk factors, 1990–2019: update from the GBD 2019 study. J Am Coll Cardiol. (2020) 76:2982–3021.
2. Margalef M, Bravo F I, Arola-Arnal A, Muguerza B. Natural Angiotensin Converting Enzyme (ACE) inhibitors with antihypertensive properties. In: Andrade P, Valentao P, Pereira DM editors. Natural Products Targeting Clinically Relevant Enzymes. Weinheim: Wiley-VCH Verlag GmbH & Co (2017). p. 45–67. doi: 10.1002/9783527805921.ch3
3. Atlas SA. The renin-angiotensin aldosterone system: pathophysiological role and pharmacologic inhibition. J Manag Care Pharm. (2007) 13:9–20. doi: 10.18553/jmcp.2007.13.s8-b.9
4. Skeggs LT Jr., Kahn JR, Shumway NP. The preparation and function of the hypertensin-converting enzyme. J Exp Med. (1956) 103:295. doi: 10.1084/jem.103.3.295
5. Guang C, Phillips RD, Jiang B, Milani F. Three key proteases–angiotensin-I-converting enzyme (ACE), ACE2 and renin–within and beyond the renin-angiotensin system. Arch Cardiovasc Dis. (2012) 105:373–85. doi: 10.1016/j.acvd.2012.02.010
6. Zhao W, Xue S, Yu Z, Ding L, Li J, Liu J. Novel ACE inhibitors derived from soybean proteins using in silico and in vitro studies. J Food Biochem. (2019) 43:e12975. doi: 10.1111/jfbc.12975
7. Handa CL, Zhang Y, Kumari S, Xu J, Ida EI, Chang SK. Comparative study of angiotensin I-converting enzyme (ACE) inhibition of soy foods as affected by processing methods and protein isolation. Processes. (2020) 8:978. doi: 10.3390/pr8080978
8. Tahir RA, Bashir A, Yousaf MN, Ahmed A, Dali Y, Khan S, et al. In Silico identification of angiotensin-converting enzyme inhibitory peptides from MRJP1. PLoS One. (2020) 15:e0228265. doi: 10.1371/journal.pone.0228265
9. Kircheva N, Dobrev S, Yakimova B, Stoineva I, Angelova S. Molecular insights into the interaction of angiotensin I-converting enzyme (ACE) inhibitors and HEXXH motif. Biophys Chem. (2021) 276:106626. doi: 10.1016/j.bpc.2021.106626
10. Li T, Zhang X, Ren Y, Zeng Y, Huang Q, Wang C. Antihypertensive effect of soybean bioactive peptides: a review. Curr Opin Pharmacol. (2022) 62:74–81. doi: 10.1016/j.coph.2021.11.005
11. Agrawal V, Gupta JK, Qureshi SS, Vishwakarma VK. (2016). Role of cardiac renin angiotensin system in ischemia reperfusion injury and preconditioning of heart. Indian Heart J. 68:856–61.
12. Shimakage A, Shinbo M, Yamada S. ACE inhibitory substances derived from soy foods. J Biol Macromol. (2012) 12:72–80. doi: 10.14533/jbm.12.72
13. Khan MY, Kumar V. Mechanism & inhibition kinetics of bioassay-guided fractions of Indian medicinal plants and foods as ACE inhibitors. J Tradit Comp Med. (2019) 9:73–84. doi: 10.1016/j.jtcme.2018.02.001
14. Chamata Y, Watson KA, Jauregi P. Whey-derived peptides interactions with ACE by molecular docking as a potential predictive tool of natural ACE inhibitors. Int J Mol Sci. (2020) 21:864. doi: 10.3390/ijms21030864
15. Sharma RK, Espinoza-Moraga M, Poblete H, Douglas RG, Sturrock ED, Caballero J, et al. The dynamic nonprime binding of sampatrilat to the C-domain of angiotensin-converting enzyme. J Chem Inf Model. (2016) 56:2486–94. doi: 10.1021/acs.jcim.6b00524
16. Patten GS, Abeywardena MY, Bennett LE. Inhibition of angiotensin converting enzyme, angiotensin II receptor blocking, and blood pressure lowering bioactivity across plant families. Crit Rev Food Sci Nutr. (2016) 56:181–214. doi: 10.1080/10408398.2011.651176
17. Zhang B, Liu J, Wen H, Jiang F, Wang E, Zhang T. Structural requirements and interaction mechanisms of ACE inhibitory peptides: molecular simulation and thermodynamics studies on LAPYK and its modified peptides. Food Sci Hum Well. (2022) 11:1623–30. doi: 10.1016/j.fshw.2022.06.021
18. Hermida RC, Ayala DE, Fernández JR, Portaluppi F, Fabbian F, Smolensky MH. Circadian rhythms in blood pressure regulation and optimization of hypertension treatment with ACE inhibitor and ARB medications. Am J Hypertens. (2011) 24:383–91. doi: 10.1038/ajh.2010.217
19. Sitanggang AB, Putri JE, Palupi NS, Hatzakis E, Syamsir E, Budijanto S. Enzymatic preparation of bioactive peptides exhibiting ACE inhibitory activity from soybean and velvet bean: a systematic review. Molecules. (2021) 26:3822. doi: 10.3390/molecules26133822
20. Xu Z, Wu C, Sun-Waterhouse D, Zhao T, Waterhouse GI, Zhao M, et al. Identification of post-digestion angiotensin-I converting enzyme (ACE) inhibitory peptides from soybean protein Isolate: their production conditions and in silico molecular docking with ACE. Food Chem. (2021) 345:128855. doi: 10.1016/j.foodchem.2020.128855
21. Akıllıoğlu HG, Karakaya S. Effects of heat treatment and in vitro digestion on the angiotensin converting enzyme inhibitory activity of some legume species. Eur Food Res Technol. (2009) 229:915–21. doi: 10.1007/s00217-009-1133-x
22. Roy F, Boye JI, Simpson BK. Bioactive proteins and peptides in pulse crops: pea, chickpea and lentil. Food Res Int. (2010) 43:432–42. doi: 10.1016/j.foodres.2009.09.002
23. Boschin G, Scigliuolo GM, Resta D, Arnoldi A. ACE-inhibitory activity of enzymatic protein hydrolysates from lupin and other legumes. Food Chem. (2014) 145:34–40. doi: 10.1016/j.foodchem.2013.07.076
24. Zhang Y, Chang SK. Comparative studies on ACE inhibition, degree of hydrolysis, antioxidant property and phenolic acid composition of hydrolysates derived from simulated in vitro gastrointestinal proteolysis of three thermally treated legumes. Food Chem. (2019) 281:154–62. doi: 10.1016/j.foodchem.2018.12.090
25. Bollati C, Xu R, Boschin G, Bartolomei M, Rivardo F, Li J, et al. Integrated evaluation of the multifunctional DPP-IV and ACE inhibitory effect of soybean and pea protein hydrolysates. Nutrients. (2022) 14:2379. doi: 10.3390/nu14122379
26. Rajendran A, Lal SK. Assessing the need of pre-germination anaerobic tress tolerant varieties in Indian soybean (Glycine max (L.) Merrill). Natl Acad Sci Lett. (2020) 43:593–7. doi: 10.1007/s40009-020-00937-9
27. Rajendran A, Lal SK, Raju D, Ramlal A. Associations of direct and indirect selection for pregermination anaerobic stress tolerance in soybean (Glycine max). Plant Breed. (2022) 141:634–43. doi: 10.1111/pbr.13048
28. Ramlal A, Dey N, Sharma U, Rajendran A. In-silico studies to reveal the potential inhibitory capacity of soy isoflavonoids against angiotensin-converting enzyme. In: Chopra H, Ghuriani V, Arora PM, Babbar S, Baweja P editors. Proceedings of the Conference: Sustainable Future for Humanity: The New Learning Curve. Mumbai: Imperial Publications (2022). p. 155–67.
29. Ramlal A, Nautiyal A, Baweja P, Mahto RK, Mehta S, Mallikarjun BP, et al. Harnessing heterosis and male sterility in soybean (Glycine max (L.) Merr.): a critical revisit. Front Plant Sci. (2022) 13:981768. doi: 10.3389/fpls.2022.981768
30. Mandal A, Cho H, Chauhan BS. (2022). Experimental investigations of multiple fry waste soya bean oil in an agricultural Cl engine. Energies. 15:3209.
31. Hymowitz T. On the domestication of the soybean. Econ Bot. (1970) 24:408–21. doi: 10.1007/BF02860745
32. Chen KI, Erh MH, Su NW, Liu WH, Chou CC, Cheng KC. Soyfoods and soybean products: from traditional use to modern applications. Appl Microbiol Biotechnol. (2012) 96:9–22. doi: 10.1007/s00253-012-4330-7
34. Tsoyi K, Park HB, Kim YM, Chung J I, Shin SC, Shim HJ, et al. Protective effect of anthocyanins from black soybean seed coats on UVB-induced apoptotic cell death in vitro and in vivo. J Agric Food Chem. (2008) 56:10600–5. doi: 10.1021/jf802112c
35. Katsuzaki H, Hibasami H, Ohwaki S, Ishikawa K, Imai K, Kimura Y, et al. Cyanidin 3-O-β-D-glucoside isolated from skin of black Glycine max and other anthocyanins isolated from skin of red grape induce apoptosis in human lymphoid leukemia Molt 4B cells. Oncol Rep. (2003) 10:297–300.
36. Kim HA, Jeong KS, Kim YK. Soy extract is more potent than genistein on tumor growth inhibition. Anticancer Res. (2008) 28:2837–41.
37. Hsieh CC, Fernández-Tomé S, Hernández-Ledesma B. Functionality of soybean compounds in the oxidative stress-related disorders. In: Gracia-Sancho J, Salvado J editors. Gastrointestinal Tissue: Oxidative stress and antioxidants. Cambridge, MA: Academic Press (2017). p. 339–53. doi: 10.1016/B978-0-12-805377-5.00027-8
38. Harlen WC, Jati IRA. Antioxidant activity of anthocyanins in common legume grains. In: Watson R, Preedy V, Zibadi S editors. Polyphenols: Mechanisms of Action in Human Health and Disease. Cambridge, MA: Academic Press (2018). p. 81–92. doi: 10.1016/B978-0-12-813006-3.00008-8
39. de Mejia EG, Vásconez M, de Lumen BO, Nelson R. Lunasin concentration in different soybean genotypes, commercial soy protein, and isoflavone products. J Agric Food Chem. (2004) 52:5882–7. doi: 10.1021/jf0496752
40. Hao Y, Fan X, Guo H, Yao Y, Ren G, Lv X, et al. Overexpression of the bioactive lunasin peptide in soybean and evaluation of its anti-inflammatory and anti-cancer activities in vitro. J Biosci Bioeng. (2020) 129:395–404. doi: 10.1016/j.jbiosc.2019.11.001
41. Bünning P, Riordan JF. The role of zinc in angiotensin converting enzyme. Israel J Chem. (1981) 21:43–7. doi: 10.1002/ijch.198100011
42. Natesh R, Schwager SL, Sturrock ED, Acharya KR. Crystal structure of the human angiotensin-converting enzyme–lisinopril complex. Nature. (2003) 421:551–4. doi: 10.1038/nature01370
43. Acharya KR, Sturrock ED, Riordan JF, Ehlers MR. Ace revisited: a new target for structure-based drug design. Nat Rev Drug Discov. (2003) 2:891–902. doi: 10.1038/nrd1227
44. Cozier GE, Schwager SL, Sharma RK, Chibale K, Sturrock ED, Acharya KR. Crystal structures of sampatrilat and sampatrilat-Asp in complex with human ACE–a molecular basis for domain selectivity. FEBS J. (2018) 285:1477–90. doi: 10.1111/febs.14421
45. Fagyas M, Uri K, Siket IM, Fülöp GÁ, Csato V, Darago A, et al. New perspectives in the renin-angiotensin-aldosterone system (RAAS) II: albumin suppresses angiotensin converting enzyme (ACE) activity in human. PLoS One. (2014) 9:e87844. doi: 10.1371/journal.pone.0087844
46. Leung PS, Chappell MC. A local pancreatic renin-angiotensin system: endocrine and exocrine roles. Int J Biochem Cell Biol. (2003) 35:838–46. doi: 10.1016/S1357-2725(02)00179-6
47. Gelen V, Kükürt A, Şengül E. Role of the Renin-Angiotensin-Aldosterone System in Various Disease Processes: An Overview. London: IntechOpen (2021). doi: 10.5772/intechopen.97354
48. Naftilan AJ, Zuo WM, Inglefinger J, Ryan T, Pratt RE, Dzau VJ. Localization and differential regulation of angiotensinogen mRNA expression in the vessel wall. J Clin Invest. (1991) 87:1300–11. doi: 10.1172/JCI115133
49. Goodfriend TL, Elliott ME, Catt KJ. Angiotensin receptors and their antagonists. N Engl J Med. (1996) 334:1649–55. doi: 10.1056/NEJM199606203342507
50. Csikos T, Chung O, Unger T. Receptors and their classification: focus on angiotensin II and the AT 2 receptor. J Hum Hypertens. (1998) 12:311–8. doi: 10.1038/sj.jhh.1000639
51. Grote K, Drexler H, Schieffer B. Renin–angiotensin system and atherosclerosis. Nephrol Dial Transplant. (2004) 19:770–3.
52. Nongonierma AB, FitzGerald RJ. Strategies for the discovery and identification of food protein-derived biologically active peptides. Trends Food Sci Technol. (2017) 69:289–305. doi: 10.1016/j.tifs.2017.03.003
53. Gouda KGM, Gowda LR, Rao AA, Prakash V. Angiotensin I-converting enzyme inhibitory peptide derived from glycinin, the 11S globulin of soybean (Glycine max). J Agric Food Chem. (2006) 54:4568–73. doi: 10.1021/jf060264q
54. Cushman DW, Cheung HS. Spectrophotometric assay and properties of the angiotensin-converting enzyme of rabbit lung. Biochem Pharmacol. (1971) 20:1637–48. doi: 10.1016/0006-2952(71)90292-9
55. Carmel A, Yaron A. An intramolecularly quenched fluorescent tripeptide as a fluorogenic substrate of angiotensin-i-converting enzyme and of bacterial dipeptidyl carboxypeptidase. Eur J Biochem. (1978) 87:265–73. doi: 10.1111/j.1432-1033.1978.tb12375.x
56. Holmquist B, Bünning P, Riordan JF. A continuous spectrophotometric assay for angiotensin converting enzyme. Anal Biochem. (1979) 95:540–8. doi: 10.1016/0003-2697(79)90769-3
57. Elbl G, Wagner H. A new method for the in vitro screening of inhibitors of angiotensin-converting enzyme (ACE), using the chromophore-and fluorophore-labelled substrate, dansyltriglycine. Planta Med. (1991) 57:137–41. doi: 10.1055/s-2006-960050
58. Lam LH, Shimamura T, Manabe S, Ishiyama M, Ukeda H. Assay of angiotensin I-converting enzyme-inhibiting activity based on the detection of 3-hydroxybutyrate with water-soluble tetrazolium salt. Anal Sci. (2008) 24:1057–60. doi: 10.2116/analsci.24.1057
59. Ahmad I, Yanuar A, Mulia K, Mun’Im A. Review of angiotensin-converting enzyme inhibitory assay: rapid method in drug discovery of herbal plants. Pharmacogn Rev. (2017) 11:1–7. doi: 10.4103/phrev.phrev_45_16
60. Wang W, Dia VP, Vasconez M, De Mejia EG, Nelson RL. Analysis of soybean protein-derived peptides and the effect of cultivar, environmental conditions, and processing on lunasin concentration in soybean and soy products. J AOAC Int. (2008) 91:936–46.
61. Iwaniak A, Minkiewicz P, Darewicz M. Food-originating ACE inhibitors, including antihypertensive peptides, as preventive food components in blood pressure reduction. Compr Rev Food Sci Food Saf. (2014) 13:114–34. doi: 10.1111/1541-4337.12051
62. Kuba M, Tana C, Tawata S, Yasuda M. Production of angiotensin I-converting enzyme inhibitory peptides from soybean protein with Monascus purpureus acid proteinase. Process Biochem. (2005) 40:2191–6. doi: 10.1016/j.procbio.2004.08.010
63. Chen JR, Okada T, Muramoto K, Suetsuna K, Yang SC. Identification of angiotensin I-converting enzyme inhibitory peptides derived from the peptic digest of soybean protein. J Food Biochem. (2002) 26:543–54. doi: 10.1111/j.1745-4514.2002.tb00772.x
64. Kodera T, Nio N. Identification of an angiotensin I-converting enzyme inhibitory peptides from protein hydrolysates by a soybean protease and the antihypertensive effects of hydrolysates in 4 spontaneously hypertensive model rats. J Food Sci. (2006) 71:C164–73. doi: 10.1111/j.1365-2621.2006.tb15612.x
65. Gu Y, Wu J. LC–MS/MS coupled with QSAR modeling in characterising of angiotensin I-converting enzyme inhibitory peptides from soybean proteins. Food Chem. (2013) 141:2682–90. doi: 10.1016/j.foodchem.2013.04.064
66. Rudolph S, Lunow D, Kaiser S, Henle T. Identification and quantification of ACE-inhibiting peptides in enzymatic hydrolysates of plant proteins. Food Chem. (2017) 224:19–25. doi: 10.1016/j.foodchem.2016.12.039
67. Kim SE, Kim HH, Kim JY, Im Kang Y, Woo HJ, Lee HJ. Anticancer activity of hydrophobic peptides from soy proteins. Biofactors. (2000) 12:151–5. doi: 10.1002/biof.5520120124
68. Wang W, De Mejia EG. A new frontier in soy bioactive peptides that may prevent age-related chronic diseases. Compr Rev Food Sci Food Saf. (2005) 4:63–78. doi: 10.1111/j.1541-4337.2005.tb00075.x
69. Ashaolu TJ. Health applications of soy protein hydrolysates. Int J Pept Res Therap. (2020) 26:2333–43. doi: 10.1007/s10989-020-10018-6
70. Daliri EBM, Ofosu FK, Chelliah R, Park MH, Kim JH, Oh DH. Development of a soy protein hydrolysate with an antihypertensive effect. Int J Mol Sci. (2019) 20:1496. doi: 10.3390/ijms20061496
71. Tomatsu M, Shimakage A, Shinbo M, Yamada S, Takahashi S. Novel angiotensin I-converting enzyme inhibitory peptides derived from soya milk. Food Chem. (2013) 136:612–6. doi: 10.1016/j.foodchem.2012.08.080
72. Kinoshita E, Yamakoshi J, Kikuchi M. Purification and identification of an angiotensin I-converting enzyme inhibitor from soy sauce. Biosci Biotechnol Biochem. (1993) 57:1107–10. doi: 10.1271/bbb.57.1107
73. Shin Z I, Yu R, Park SA, Chung DK, Ahn CW, Nam HS, et al. His-His-Leu, an angiotensin I converting enzyme inhibitory peptide derived from Korean soybean paste, exerts antihypertensive activity in vivo. J Agric Food Chem. (2001) 49:3004–9. doi: 10.1021/jf001135r
74. Li F, Liu W, Yamaki K, Liu Y, Fang Y, Li Z, et al. Angiotensin I-converting enzyme inhibitory effect of Chinese soypaste along fermentation and ripening: contribution of early soybean protein borne peptides and late Maillard reaction products. Int J Food Prop. (2016) 19:2805–16.
75. Ibe S, Yoshida K, Kumada K. Angiotensin I converting enzyme inhibitory activity of natto, a traditional Japanese fermented food. Nippon Shokuhin Kagaku Kogaku Kaishi. (2006) 53:189–92.
76. Okamoto A, Hanagata H, Kawamura Y, Yanagida F. Anti-hypertensive substances in fermented soybean, Natto. Plant Foods Hum Nutr. (1995) 47:39–47. doi: 10.1007/BF01088165
77. Fan J, Saito M, Tatsumi E, Ll L. Preparation of angiotensin I-converting enzyme inhibiting peptides from soybean protein by enzymatic hydrolysis. Food Sci Technol Res. (2003) 9:254–6. doi: 10.3136/fstr.9.254
78. Hernández-Ledesma B, Amigo L, Ramos M, Recio I. Angiotensin converting enzyme inhibitory activity in commercial fermented products. Formation of peptides under simulated gastrointestinal digestion. J Agric Food Chem. (2004) 52:1504–10. doi: 10.1021/jf034997b
79. Capriotti AL, Caruso G, Cavaliere C, Samperi R, Ventura S, Chiozzi RZ, et al. Identification of potential bioactive peptides generated by simulated gastrointestinal digestion of soybean seeds and soy milk proteins. J Food Compos Anal. (2015) 44:205–13. doi: 10.1016/j.jfca.2015.08.007
80. Nakahara T, Sano A, Yamaguchi H, Sugimoto K, Chikata H, Kinoshita E, et al. Antihypertensive effect of peptide-enriched soy sauce-like seasoning and identification of its angiotensin I-converting enzyme inhibitory substances. J Agric Food Chem. (2010) 58:821–7. doi: 10.1021/jf903261h
81. Nakahara T, Sugimoto K, Sano A, Yamaguchi H, Katayama H, Uchida R. Antihypertensive mechanism of a peptide-enriched soy sauce-like seasoning: the active constituents and its suppressive effect on renin–angiotensin–aldosterone system. J Food Sci. (2011) 76:H201–6. doi: 10.1111/j.1750-3841.2011.02362.x
82. Zhang JH, Tatsumi E, Ding CH, Li LT. Angiotensin I-converting enzyme inhibitory peptides in douchi, a Chinese traditional fermented soybean product. Food Chem. (2006) 98:551–7.
83. Fan J, Hu X, Tan S, Zhang Y, Tatsumi E, Li L. Isolation and characterisation of a novel angiotensin I-converting enzyme-inhibitory peptide derived from Douchi, a traditional Chinese fermented soybean food. J Sci Food Agric. (2009) 89:603–8.
84. Li FJ, Yin LJ, Cheng YQ, Yamaki K, Fan JF, Li LT. Comparison of angiotensin I-converting enzyme inhibitor activities of pre-fermented Douchi (a Chinese traditional fermented soybean food) started with various cultures. Int J Food Eng. (2009) 5:1556–3758.
85. Kuba M, Tanaka K, Tawata S, Takeda Y, Yasuda M. Angiotensin I-converting enzyme inhibitory peptides isolated from tofuyo fermented soybean food. Biosci Biotechnol Biochem. (2003) 67:1278–83. doi: 10.1271/bbb.67.1278
86. Wang H, Zhang S, Sun Y, Dai Y. ACE-inhibitory peptide isolated from fermented soybean meal as functional food. Int J Food Eng. (2013) 9:1–8. doi: 10.1515/ijfe-2012-0207
87. Rho SJ, Lee JS, Chung YI, Kim YW, Lee HG. Purification and identification of an angiotensin I-converting enzyme inhibitory peptide from fermented soybean extract. Process Biochem. (2009) 44:490–3. doi: 10.1016/j.procbio.2008.12.017
88. Chourasia R, Phukon LC, Abedin MM, Sahoo D, Rai AK. Production and characterization of bioactive peptides in novel functional soybean chhurpi produced using Lactobacillus delbrueckii WS4. Food Chem. (2022) 387:132889. doi: 10.1016/j.foodchem.2022.132889
89. Chalid SY, Hermanto S, Rahmawati A. Angiotensin converting enzyme inhibitor activity of the soybean tempeh protein as functional food. Int J Geomate. (2019) 16:73–8.
90. Kiriakidis S, Högemeier O, Starcke S, Dombrowski F, Hahne JC, Pepper M, et al. Novel tempeh (fermented soyabean) isoflavones inhibit in vivo angiogenesis in the chicken chorioallantoic membrane assay. Br J Nutr. (2005) 93:317–23. doi: 10.1079/bjn20041330
92. Balasuriya BN, Rupasinghe HV. Plant flavonoids as angiotensin converting enzyme inhibitors in regulation of hypertension. Funct Foods Health Dis. (2011) 1:172–88. doi: 10.31989/ffhd.v1i5.132
93. Wang T. Minor constituents and phytochemicals of soybeans. In: Johnson LA, White PJ, Galloway R editors. Soybeans: Chemistry, Production, Processing and Utilisation. Urbana, IL: AOCS Press (2008). p. 297–329. doi: 10.1016/B978-1-893997-64-6.50013-5
94. Xu YY, Yang C, Li SN. Effects of genistein on angiotensin-converting enzyme in rats. Life Sci. (2006) 79:828–37. doi: 10.1016/j.lfs.2006.02.035
95. Wu J, Muir AD. Isoflavone content and its potential contribution to the antihypertensive activity in soybean angiotensin I converting enzyme inhibitory peptides. J Agric Food Chem. (2008) 56:9899–904. doi: 10.1021/jf801181a
96. Montenegro MF, Pessa LR, Tanus-Santos JE. Isoflavone genistein inhibits the angiotensin-converting enzyme and alters the vascular responses to angiotensin I and bradykinin. Eur J Pharmacol. (2009) 607:173–7. doi: 10.1016/j.ejphar.2009.02.015
97. Choudhury A, Pai KV. Angiotensin converting enzyme inhibition activity of Daidzein. J Drug Deliv Therap. (2014) 4:92–8.
98. Yang HY, Chen JR, Chang LS. Effects of soy protein hydrolysate on blood pressure and angiotensin-converting enzyme activity in rats with chronic renal failure. Hypertens Res. (2008) 31:957–63. doi: 10.1291/hypres.31.957
99. Rossi GP, Sanga V, Barton M. Potential harmful effects of discontinuing ACE-inhibitors and ARBs in COVID-19 patients. Elife. (2020) 9: e57278.
100. Karimi A, Majlesi M, Rafieian-Kopaei M. Herbal versus synthetic drugs; beliefs and facts. J Nephropharmacol. (2015) 4:27.
101. Farooq S, Ngaini Z. Natural and synthetic drugs as potential treatment for coronavirus disease 2019 (COVID-2019). Chem Afr. (2021) 4:1–13. doi: 10.1080/07391102.2020.1790425
102. Wu J, Ding X. Hypotensive and physiological effect of angiotensin converting enzyme inhibitory peptides derived from soy protein on spontaneously hypertensive rats. J Agric Food Chem. (2001) 49:501–6. doi: 10.1021/jf000695n
103. Wu J, Ding X. Characterization of inhibition and stability of soy-protein-derived angiotensin I-converting enzyme inhibitory peptides. Food Res Int. (2002) 35:367–75.
104. Chatterjee C, Gleddie S, Xiao CW. Soybean bioactive peptides and their functional properties. Nutrients. (2018) 10:1211.
105. Lo WM, Li-Chan EC. Angiotensin I converting enzyme inhibitory peptides from in vitro pepsin- pancreatin digestion of soy protein. J Agric Food Chem. (2005) 53:3369–76.
106. Erdmann K, Cheung BW, Schröder H. The possible roles of food-derived bioactive peptides in reducing the risk of cardiovascular disease. J Nutr Biochem. (2008) 19:643–54. doi: 10.1016/j.jnutbio.2007.11.010
107. Vallabha VS, Tiku PK. Antihypertensive peptides derived from soy protein by fermentation. Int J Peptide Res Therap. (2014) 20:161.
Keywords: soy products, angiotensin-converting enzyme I (ACE I), cardiovascular diseases (CVDs), natural drugs, renin-angiotensin-aldosterone system (RAAS)
Citation: Ramlal A, Nautiyal A, Baweja P, Kumar V, Mehta S, Mahto RK, Tripathi S, Shanmugam A, Pujari Mallikarjuna B, Raman P, Lal SK, Raju D and Rajendran A (2022) Angiotensin-converting enzyme inhibitory peptides and isoflavonoids from soybean [Glycine max (L.) Merr.]. Front. Nutr. 9:1068388. doi: 10.3389/fnut.2022.1068388
Received: 12 October 2022; Accepted: 03 November 2022;
Published: 24 November 2022.
Edited by:
Elena Ibañez, Institute of Food Science Research (CSIC), SpainReviewed by:
Alejandro Cifuentes, Foodomics Lab, CIAL (CSIC), SpainBlanca Hernandez-Ledesma, Spanish National Research Council (CSIC), Spain
Copyright © 2022 Ramlal, Nautiyal, Baweja, Kumar, Mehta, Mahto, Tripathi, Shanmugam, Pujari Mallikarjuna, Raman, Lal, Raju and Rajendran. This is an open-access article distributed under the terms of the Creative Commons Attribution License (CC BY). The use, distribution or reproduction in other forums is permitted, provided the original author(s) and the copyright owner(s) are credited and that the original publication in this journal is cited, in accordance with accepted academic practice. No use, distribution or reproduction is permitted which does not comply with these terms.
*Correspondence: Ambika Rajendran, rambikarajendran@gmail.com
†Present address: Ayyagari Ramlal, School of Biological Sciences, Universiti Sains Malaysia (USM), Penang, Malaysia
‡ORCID: Ayyagari Ramlal, orcid.org/0000-0002-1093-9877; Ambika Rajendran, orcid.org/0000-0002-2223-9309