- 1School of Food Science and Engineering, Yangzhou University, Yangzhou, Jiangsu, China
- 2Industrial Engineering Center for Huaiyang Cuisine of Jiangsu Province, School of Tourism and Cuisine, Yangzhou University, Yangzhou, Jiangsu, China
- 3Engineering Laboratory for Agro-Products Processing, Anhui Agricultural University, Hefei, China
Marination is a common technology in meat processing with advantages of enhancing tenderness, water retention, and overall quality. This study was conducted to investigate the effect of vacuum tumbling and immersion marination on meat quality, microstructure, water mobility, protein changes, and denaturation of Xueshan chicken. Results showed that vacuum tumbling significantly increased the marinating rate of chicken, tenderness, meat texture, and water retention. Meanwhile, vacuum tumbling decreased total sulfhydryl content alongside an increased protein surface hydrophobicity and free sulfhydryl content, indicating that vacuum tumbling elevated the degree of protein denaturation. Further, the peak area corresponding to the relaxation time T22 after vacuum tumbling was significantly higher than that of immersion marination, suggesting that the stability of the immobilized water of chicken was reduced by vacuum tumbling. Compared to immersion marination, vacuum tumbling improved myofibril fragmentation index (MFI) presenting fewer myofibrillar protein bands in sodium dodecyl sulfate polyacrylamide gel electrophoresis (SDS-PAGE) gel and more damaged muscular cells. Overall, vacuum tumbling could improve the marination absorptivity, protein degradation, and denaturation, resulting in changes in myofibril structure and meat quality of Xueshan chicken.
Introduction
Chicken is a healthy food option that is high in protein and low in fat. It has gained increasing consumer preference due to its delicate texture and high unsaturated fatty acid content (1, 2). Inferior meat attributes are usually occurred in fast-growing broiler with pale-soft-exudative conditions, white striping, and woody breast, resulting in high water loss and low yield during processing (3, 4). Slowing-growing (SG) genotype of broiler has been perceived to have better meat quality and flavor characteristics in comparison to fast-growing broiler (5, 6). Xueshan chicken is a SG chicken crossbred by Tibetan chicken and Chahua chicken. It is a major local yellow-feathered breed for meat resource in China and shares a market value of 7.69 billion ¥ in 2020 (7). In recent years, people have become increasingly demanding of high-quality meat products. Correspondingly, the meat industry has become focused on improving quality and nutritional value of poultry meat products.
Marination has been known as an important step in meat processing. It is often used to tenderize, increase water retention, and improve overall meat quality (8, 9). Immersion marination is a traditional method of marinating and refers to the soaking of meat into marinades which are comprised of water, salts, phosphate, flavoring, and other ingredients. The immersion marination is slow and uneven in salt penetration, which affects the quality of the product. In order to make marination more efficient, a series of meat marination technologies have been developed, including muti-needle injection, ultrasound-assisted marination, massaging, tumbling, or vacuum tumbling (9–11). Tumbling is a well-known physical-mechanical treatment method, and it can rupture muscular cell membranes for marinade easily penetrated and shorten the marination time (12). A vacuum tumbler is usually utilized in the tumbling process to promote marinade penetration, leading to the more efficient entry of the marinade into the meat. Vacuum processing can not only expand the muscle fiber by different internal and external pressures, but also discharge of occluded gases of meat (13). The vacuum and mechanical movements of tumbling exhibit synergistic effects of dispersing liquids from the meat pores and extracting meat proteins to the surface. At the end of the vacuum tumbling of meat products, the atmospheric pressure would compress the residual gas in the pores and favor the infiltration of exogenous solution through the pores of meat (14). Thus, vacuum tumbling can promote the relaxation of muscle fiber structures, and make the distribution of the marinade in the meat more uniform and finally improve the quality of meat products (15, 16).
Protein denaturation generally occurs during meat processing, which has a great influence on the color and texture characteristics of meat (17). The denaturation of myofibrillar proteins may expose the internal sulfhydryl groups of protein to be oxidized to disulfide bonds, resulting in an increase in protein surface hydrophobicity and decreased protein solubility (18, 19). This affects the texture and the state of water of meat products, and these qualities play a decisive role in overall quality (20). Proteins undergo complex biochemical changes including protein degradation during the processing process which may also account for changes in the color, texture, and water retention capacity of the meat (21).
Vacuum tumbling marination has been achieved as a common technology in chicken meat processing. Previous studies were mainly focused on the various marinade ingredients and formulations (22, 23), processing variables such as carcass chilling (24), sub-sampling (25), and deboning time (26), and tumbler machine operating parameters (27) on quality attributes of chicken meat product. The swell of myofibrils and solubilization of myofibrillar proteins are thought to be responsible for the quality changes by vacuum tumbling (8) while no evidence has been found in chicken meat processing. To our knowledge, little information is available on the effect of vacuum tumbling on changes in protein degradation and denaturation, the microstructure of muscle cells and mobility of water, which were accounting for quality attributes of chicken. Thus, the aim of this study was to investigate the effect of vacuum tumbling on the quality characteristics, water holding capacity, and protein changes of Xueshan chicken compared with immersion marination, to explore the relationship between protein denaturation and quality characteristics.
Materials and methods
Sample preparation
The chilled Xueshan chicken (3-month-old, weight of 1.20 ± 0.05 kg) was purchased from Lihua livestock Co. Ltd. (Changzhou, Jiangsu, China) and was thawed at 4°C for 24 h. The marinade was prepared according to the weight of the chicken, and the volume ratio of liquid to meat was 1:1. The marinade ingredients included pepper juice (0.05%, w/w), spiced juice (0.08%, w/w), ethyl maltol (0.04%, w/w), sodium glutamate (0.15%, w/w), sand ginger powder (0.2%, w/w), sodium chloride (6.41%, w/w), and sodium tripolyphosphate (0.42%, w/w). The pepper juice and spiced juice were purchased from Tieling Hongyuan Food Co. Ltd. (Liaoning, China). There were 30 whole chicken carcasses which were randomly allocated into tumbling marination treatment and immersion marination treatment (15 chickens per treatment) and performed three batches (five chickens per batch) using a vacuum tumbler (ESK125, Vakona Gmbh Co., Ltd., Lienen, Germany). The parameters of tumbling marination were set from our trial tests considering the marinating absorptivity and sensory evaluation of Xueshan chicken. The marination time was set for 10 h at a speed of seven revolutions per min. The vacuum was set for −0.06 MPa, in a cycle of 20 min on and 10 min off at 4°C. The chicken by immersion marinating was immersed in the marinade for 15 h at 4°C, which was performed by the manufacturer’s recommendation from Lihua livestock Co. Ltd. After completion of marinating treatments, chicken breasts were obtained for quality determination and protein measurements.
Determination of marinating absorptivity and pH
The marination absorptivity was measured according to the method of Peiretti et al. (28). The weight of chicken breast before marination was recorded as m1 and the weight of marinated chicken breast was recorded as m2. The marination absorptivity was calculated as the percentage of difference of m2 and m1 over m1. The pH of the sample was measured using a portable pH meter (FE20K; Mettler Toledo, Zurich, Switzerland). The probe was inserted into the chicken breast, and each sample was measured at four different points and averaged.
Meat quality measurements
The color attributes including L*, a*, and b* values on the chicken breast surface were measured by a colorimeter (CR-400; Konica Minolta, Tokyo, Japan). The press loss and cooking loss were measured according to the method of Kauffman et al. (29) and Xia et al. (30), respectively, and expressed as the percentage of weight difference over the initial weight. The shear force was measured by the method described by Chen et al. (31). After the determination of cooking loss, the sample was cut along the direction of the muscle fiber, and the meat slices were cut with a C-LM3B digital display tenderness meter (Northeast Agricultural University, Haerbin, China). The texture profile analysis was conducted using a texture analyzer (TA-XT Plus, Stable Micro System, Surrey, UK) according to Malila et al. (32) with slight modifications. The cooked chicken breast was cooled and cut into 1 × 1 × 1 cm3 pieces along the direction of the muscle fibers. The texture profiles including hardness, springiness, cohesiveness, chewiness, and resilience were analyzed by Texture Expert Exceed 2.64a.
Nuclear magnetic resonance transverse relaxation (T2) analysis
The water distribution and proportion in the chicken breast meat samples were determined using a micro nuclear magnetic resonance (NMR) instrument (Meso MR23, Niumag Electric Corporation, Shanghai, China) by the method of Kang et al. (33) with some modifications. The meat sample was cut into a 1 cm diameter with a length of 2 cm along the direction of the muscle fiber and placed in a glass magnetic resonance tube with a diameter of 1 cm. The spin-selective relaxation time (T2) was measured using the Carr–Purcell–Meiboom–Gill (CPMG) pulse sequence, and the instrument temperature was stable at 32°C. The measurement parameters were set as follows: TR = 4,500 ms, SW = 100 KHz, NECH = 4,000, NS = 32, τ = 200 μs, and the obtained attenuation curve was inverted by Multi-Exp Inv analysis software. Statistical analysis was performed on the proportion of moisture content, and each test was repeated three times.
Analysis of microstructure
The microstructures of chicken breast were examined by the scanning electron microscopy (GeminiSEM 300, Carl Zeiss, Oberkochen, Germany). The marinated sample was cut into 2 cm × 0.5 cm × 0.5 cm pieces vertical to the muscle fiber direction and fixed in 2.5% glutaraldehyde dissolved in 0.1 M phosphate buffer (pH 7.2) overnight at 4°C. The specimens were washed twice with 0.1 M phosphate buffer (pH 7.2) and each for 15 min. The samples were dehydrated with a gradient of 30%, 50%, 70%, 80%, 90%, 95%, 100% (two times), 100% (with anhydrous sodium sulfate) ethanol, each for 15 min. The specimens were dried with a critical point dryer (CPD-300, Leica, Heerbrugg, Switzerland) and coated with gold (SCD 500, BAL-TEC, Vienna, Austria).
Determination of myofibrillar protein solubility
Meat sample (2 g) was homogenized three times with 40 ml of 0.1 M ice-cold potassium phosphate buffer (pH 7.2, 1.1 M potassium iodide) to extract total protein. The samples were then placed on a shaker and extracted at 4°C for 12 h. The samples were then centrifuged (1,500 g, 20 min) at 4°C and the BCA protein assay kit (Thermo Scientific, Rockford, USA) was used to determine the concentration of supernatant protein. Sarcoplasmic protein solubility was measured by 0.025 M ice-cold potassium phosphate buffer (pH 7.2), and performed the same extraction and BCA measurement protocol. The myofibrillar protein solubility was expressed as the difference between total protein solubility and sarcoplasmic protein solubility.
Preparation of myofibrillar protein
The extraction of myofibrillar protein was carried out according to Park and Xiong (34) with slight modifications. The chopped meat (10 g) was mixed with 4 vol of separation buffer (10 mM phosphate buffer, 2 mM MgCl2, 0.1 M NaCl, and 1 mM EGTA, pH 7.0), homogenized for 30 s and centrifuged (4°C, 2,000 × g, 15 min). The supernatant was discarded and the pellet was washed two more times with 4 vol of the separation buffer. The pellet was then washed three times with 4 vol of 0.1 M NaCl. The supernatant was discarded and the myofibrillar protein was obtained to detect protein concentration by Biuret protein assay. Then, the myofibrillar protein was stored at 4°C for use within 24 h.
Determination of protein surface hydrophobicity
The myofibrillar protein was dissolved in 20 mM phosphate buffer (containing 0.01 M K2HPO4, pH 7.0) and diluted to a concentration of 1.5 mg/ml. The 20 μl of 8 mM 8-Anilino-1-naphthalene sulfonic acid (ANS) was added to 2 ml of the diluted solution and incubated at room temperature for 10 min. The fluorescence intensity of the protein was determined at an excitation wavelength of 380 nm and an emission wavelength of 490 nm. ANS was not added to the blank. The different protein concentrations were taken as the abscissa and the corresponding fluorescence intensity was plotted as the ordinate. The surface hydrophobicity of protein was represented by the slope of the regression fit curve, and the initial slope was called S0ANS.
Determination of protein sulfhydryl content
Protein sulfhydryl content was determined by the method described by Fu et al. (35), with minor modifications. The 1 ml of 2 mg/ml myofibrillar protein solution was mixed with 9 ml of 50 mM PBS (0.01 M EDTA, 0.6 M KCl, 8 M urea, 0.02 M phosphate buffer, pH 7.0) and 0.4 ml of 10 mM 5,5′-dithiobis (2-nitrobenzoic acid) DTNB. The mixture was incubated at 40°C for 25 min. The absorbance of the sample was measured at 412 nm with a molar extinction coefficient of 13,600 M–1 cm–1. The free sulfhydryl content was determined by incubation at 4°C for 1 h in a urea-free reaction mixture. Protein sulfhydryl content was expressed in nmol/mg protein.
Determination of myofibril fragmentation index
The myofibril fragmentation index (MFI) was determined according to the method of Culler et al. (36). The myofibrillar protein solution was adjusted to 0.5 mg/ml and its absorbance at 540 nm was measured using a microplate reader (Spectra Max M3; Molecular Devices, Sunnyvale, USA). The MFI was obtained by an absorbance value multiplying by 200.
Sodium dodecyl sulfate polyacrylamide gel electrophoresis
The concentration of myofibrillar protein was adjusted to 4 mg/ml and mixed with the loading buffer [10 mM Tris–HCl, 2.5% (m/v) SDS, 1% (v/v) β-mercaptoethanol, 10% glycerol (v/v), and 0.01% (m/v) bromophenol blue, pH 6.8] in an equal volume, followed by heating in a water bath at 95°C for 5 min. The separating gel was 10%. The initial electrophoresis voltage was 90 V, and after 30 min, the voltage was increased to 120 V for 60 min. After electrophoresis, the gel was stained with a staining buffer containing 45% methanol, 10% glacial acetic acid, and 0.1% (m/v) coomassie brilliant blue, and then de-stained with distilled water before scanning on a gel imager (GT-800F, Epson, Nagano, Japan).
Statistical analysis
All analytical tests were performed in triplicate. The data were statistically analyzed using SPSS 16.0 software and the results were expressed as mean ± standard error (SE). The t-test was used to compare two treatments, and the significant difference level was P < 0.05.
Results and discussion
Water holding capacity and pH
The water retention of chicken after marination is shown in Table 1. The marination absorptivity of the chicken breast marinated by vacuum tumbling was higher than that of immersion marinating (P < 0.01). It was previously reported that the addition of sodium tripolyphosphate promoted the dissociation of actomyosin to myosin in meat, which had a strong water retention capacity, thereby increasing the water retention of chicken (8, 37). The mechanical action of tumbling marination promoted relaxation of the muscle tissue, and the fascia tissue was partially destroyed, allowing the marinade to penetrate into the meat more quickly (9, 13). The widening of muscle fiber gaps allowed the chicken to maintain more water. A large amount of the marination liquid was absorbed by the chicken, which caused the cooking loss of the tumbling marinated chicken to be significantly higher than that of immersion marination (P < 0.05). However, with respect to press loss, there was no significant difference between immersion marination and vacuum tumbling (P > 0.05), indicating a comparable effect of water holding capacity in chicken meat after two marination treatments. The pH value has a great influence on the quality of the meat. Compared to immersion marination, tumbling marination significantly increased the pH value of chicken breast (P < 0.05) possibly due to the higher absorptivity of marinade, which contained alkaline tripolyphosphate (38, 39). During the marination process, sodium chloride and sodium tripolyphosphate in the marinade diffused into the muscle tissue due to osmotic pressure, resulting in an increase in the pH of the chicken breast.
Color
Color is an important sensory quality of meat. The effect of marination methods on the color of chicken breast meat is shown in Table 1. The L* of the chicken meat marinated by vacuum tumbling was higher (P < 0.01) than after immersion marination. The L* is related to the water retention of the meat. An increase in the L* by vacuum tumbling was due to the fact that muscle cells absorb a large amount of water during the marination process, which changes the reflection characteristics of the meat, resulting in a higher L* of marinated chicken (40).
Vacuum tumbling group showed a significantly lower a* value and higher b* value than that of the immersion margination group (Table 1, P < 0.01). During the vacuum tumbling process, the mechanical effect of the tumbling treatment may disrupt the muscle fibers, resulting in the change in myoglobin content in muscle cell (41). Moreover, the myoglobin in the chicken might be oxidized to oxygenated myoglobin, and the long-term vacuum tumbling marination caused the oxygenated myoglobin further oxidized to form brown metmyoglobin, leading to a decrease in the observed a* value. In addition, as the tumbling time increased, the residual blood in the meat gradually flowed out, which also caused a* of chicken breast meat to decrease. The b* of tumbling marinated chicken breast was shown to be significantly higher than that of the immersion treatment in the current study, which may be caused by protein denaturation during the rolling process (40).
Texture and tenderness of chicken breast
Texture and tenderness are important indicators for evaluating meat quality and have a great influence on the taste of meat. The tenderness of meat is usually expressed by shear force. As presented in Table 1, hardness and chewiness of the chicken prepared by vacuum tumbling were lower than after immersion marination (P < 0.01). Consistently, the shear force of tumble-marinated chicken breast was lower than immersion marination (P < 0.01). The mechanical effect of vacuum tumbling treatment may disrupt the muscle fibers and cause the muscle tissue to be loosen, thereby promoting the diffusion of the marinade into the muscle tissue. In addition, under vacuum conditions, the muscle fibers swelled due to the difference between internal and external pressure, which increased the gap between the muscle fibers and promoted the structural relaxation of the muscle fibers (13). It was shown that the cohesiveness of meat was related to the integrity of muscle fiber structure and greater cohesiveness indicated less damaged muscle fiber. The cohesiveness of chicken marinated by vacuum tumbling was significantly lower than after immersion marination (P < 0.01), which indicated more damage to muscle fibers by vacuum tumbling. Moreover, mechanical effects and vacuum may cause the release of endogenous proteases such as cathepsins and calpains in meat, thereby further increasing the tenderness of chicken (42, 43). As a result, chicken marinated by vacuum tumbling had significantly higher tenderness compared to immersion marination.
NNR transverse relaxation (T2)
Nuclear magnetic resonance transverse relaxation can reflect the mobility of water in food matrix (44). As shown in Figure 1, four water groups were observed and included T20 (0–0.1 ms), T21 (1–10 ms), T22 (10–100 ms), and T23 (100–1,000 ms). T20 and T21 represented water that was tightly bound to macromolecules, where T20 was strongly bound water and T21 was weakly bound water. T22 indicated the immobilized water inside the organized protein structure, and T23 was referred to as the free water outside the myofibril.
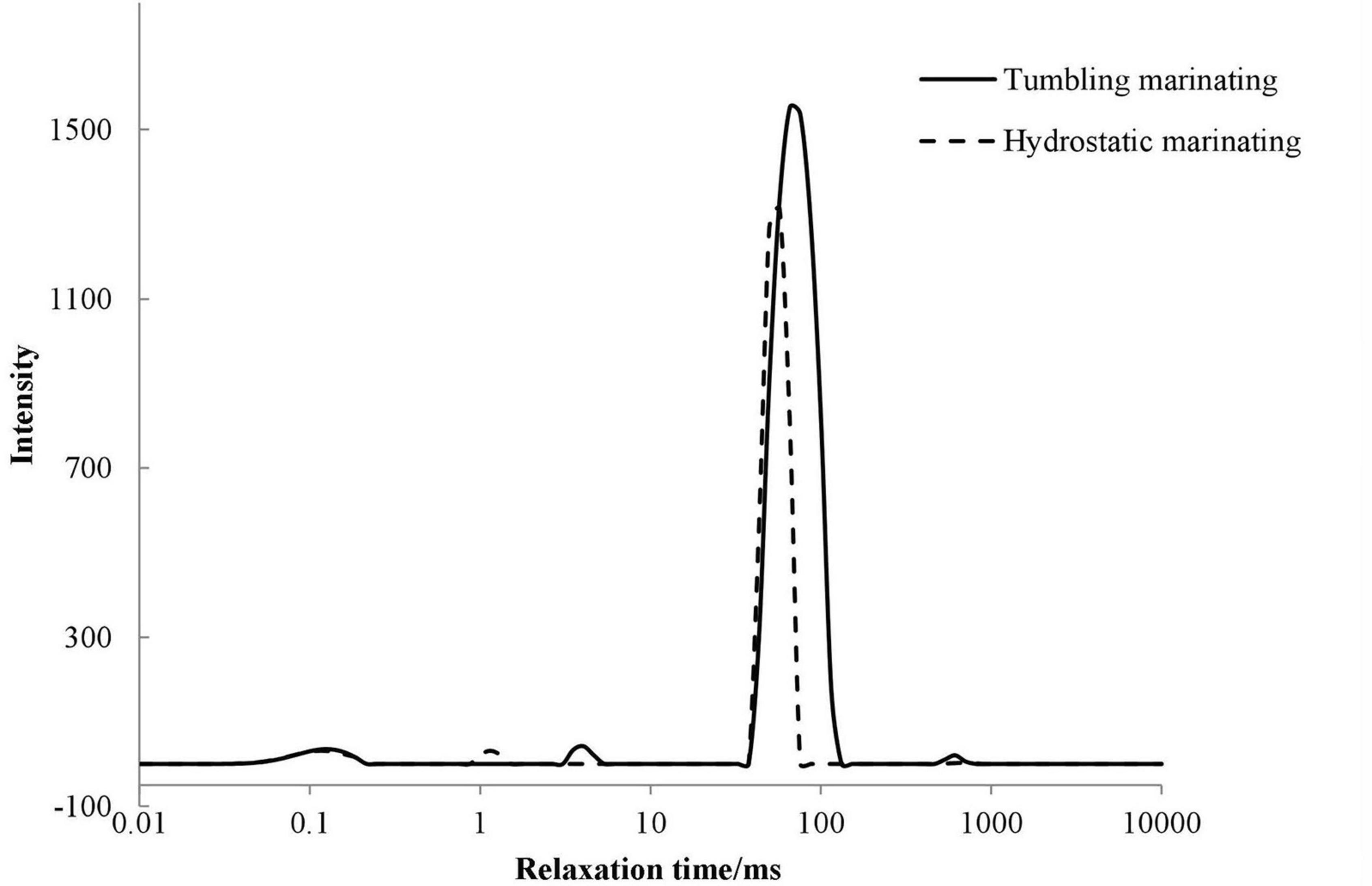
Figure 1. Effects of vacuum tumbling and immersion marinations on the T2 relaxation time (ms) of prepared chicken breast.
As shown in Table 2, no significant difference was found in the peak time of T20 between immersion and vacuum tumbling marination (P > 0.05). However, vacuum tumbling marination significantly decreased the combined water peak area ratio of P20 and P21 (P < 0.05), which may be related to the change in protein spatial structure. Compared with immersion marination, the vacuum tumbling marination significantly increased the relaxation time of T21, T22, and T23, and the peak area of T22 (P < 0.05). It was suggested that vacuum tumbling marination significantly increased the immobilized water content of the chicken. This may be due to the mechanical effect of vacuum tumbling, which led to the increase in muscle fiber gap and more water absorption inside muscular cells, thereby raising the content of the immobilized water. The vacuum tumbling group had a higher T22 value than the immersion group. The vacuum tumbling marination treatment might destroy the intact structure of the muscle fiber and reduce the stability of the immobilized water. In addition, vacuum tumbling marination was able to absorb much-marinating liquid and then increased moisture content and water mobility (33).

Table 2. Effect of vacuum tumbling and immersion marination on water mobility and distribution of chicken breast.
Protein properties
The MFI is inversely related to the integrity of the internal structure of myofibrils and affects the tenderness of the meat (45). As seen in Table 3, the MFI value of the chicken marinated by vacuum tumbling significantly increased (P < 0.05) compared to immersion marination. During the vacuum tumbling process, the mechanical effects caused the breakage of muscle fiber and improved tenderness, which was consistent with the shear force measurement (Table 1).
The surface hydrophobicity of the protein reflects the relative content of hydrophobic residues on the surface of the protein molecule and is related to protein functional properties (46, 47). The greater surface hydrophobicity of myofibrillar proteins indicated more exposed the hydrophobic residues inside the protein molecules. As shown in Table 3, the protein surface hydrophobicity of the chicken marinated by vacuum tumbling was significantly higher than that by immersion marination (P < 0.05). Protein denaturation can lead to the exposure of hydrophobic groups inside the protein (19, 48). It was implied that vacuum tumbling caused denaturation of myofibrillar proteins, leading to the exposure of hydrophobic residues inside the protein, which increased the surface hydrophobicity of the protein.
The total sulfhydryl content of the vacuum tumbling marination group was lower than immersion marination (P < 0.05). The continuously physico-mechanical action (e.g., friction and beating) of tumbling marination as well as the catalysis of metal ions in the chamber wall of a tumbling machine were considered as important factors for the oxidation of meat proteins. Free sulfhydryl groups are closely related to the distribution and content of sulfhydryl groups on the surface of proteins. As shown in Table 3, the free sulfhydryl content of the vacuum tumbling marination group was higher than immersion marnation group (P < 0.05), indicating that vacuum tumbling marination increased the degree of protein denaturation. It was shown that the free sulfhydryl content of protein was positively correlated with surface hydrophobicity (49). Vacuum tumbling marination was speculated to denature meat proteins, causing structural changes, and exposing hydrophobic groups which were embedded inside the protein. Moreover, the change of protein spatial structures led to the exposure of sulfhydryl groups, increasing the content of free sulfhydryl groups, and this change of free sulfhydryl content was consistent with the surface hydrophobicity.
The solubility of proteins is closely related to their functional properties, reflecting some extent the degree of denaturation of proteins. The effect of two marination methods on protein solubility is shown in Table 3. The total protein solubility of the vacuum tumbling group was lower (P < 0.05) than that of immersion group. Vacuum tumbling had a greater effect on the solubility of total protein, indicating more denaturation of chicken protein compared to immersion marination. During the vacuum tumbling marination process, salt and sodium tripolyphosphate can promote the dissolution of salt-soluble proteins, resulting in a decrease in the solubility of myofibrillar proteins. Further, the vacuum conditions and the mechanical effects of tumbling accelerated the dissolution of salt-soluble proteins, which may also result in a significantly lower myofibrillar solubility in the vacuum tumbling group.
Microstructure
As it was shown in Figure 2, the muscular cells in the immersion group were closely arranged, and the gap between muscle fibers was small, indicating that the degree of damage to the muscle fibers by immersion was limited. However, the muscular cells in vacuum tumbling marination group was seen to be severely damaged and the muscle bundle membrane was broken to disrupt the integrity of muscle fibers. Moreover, the gap between the muscle fibers was visibly larger by vacuum tumbling marination, compared to immersion marination. This indicates that vacuum tumbling destroyed the structure of the muscle fibers, increased the gap of the muscle fibers, and loosened the structure of the muscle fibers, thereby attributing to the tenderness promotion and also the protein denaturation of chicken meat by vacuum tumbling (50).
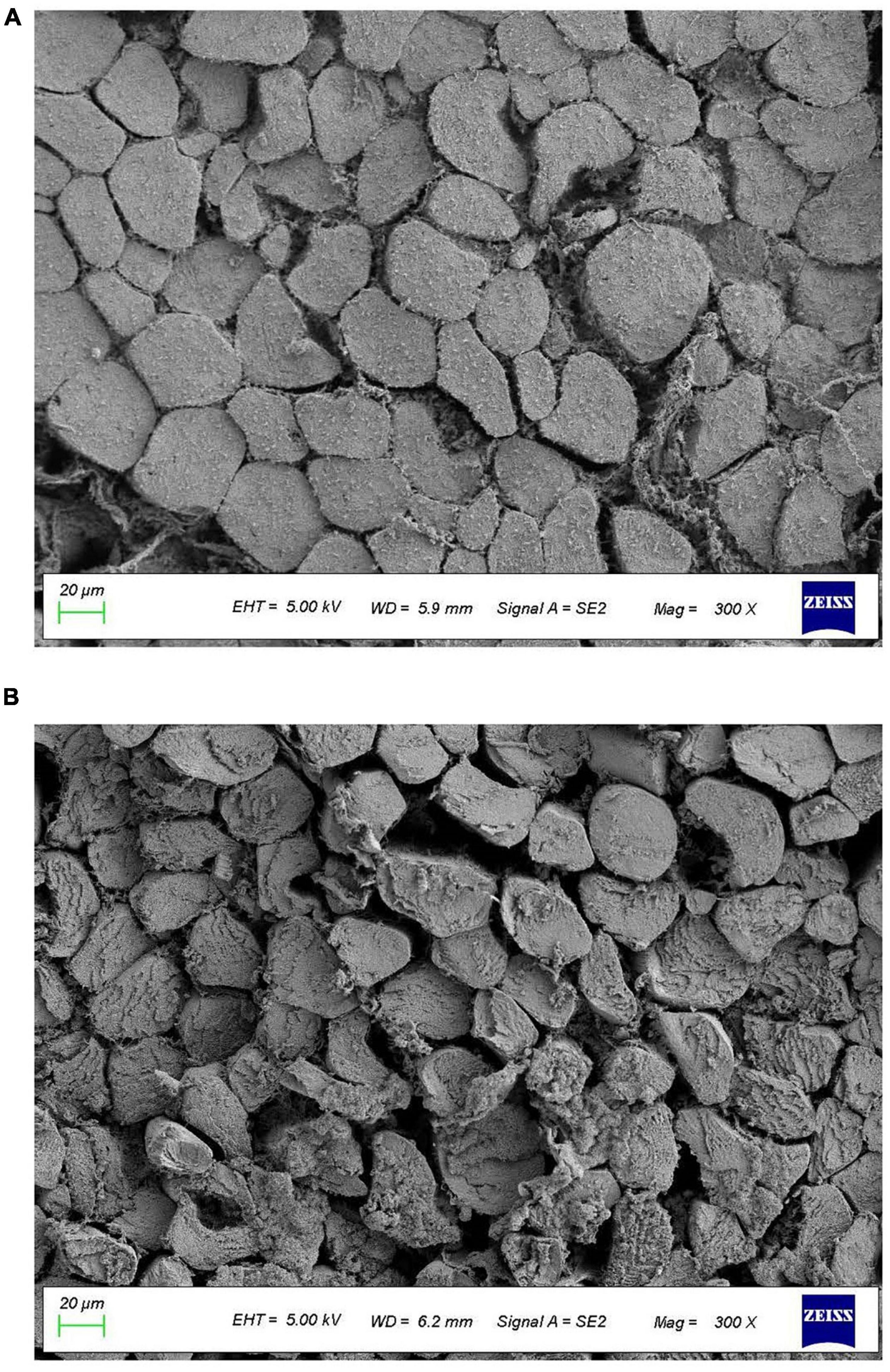
Figure 2. Effects of vacuum tumbling (A) and immersion marinations (B) on the microstructure of chicken.
SDS-PAGE
The effect of marination on the myofibrillar protein bands is shown in Figure 3. Compared with immersion marination, the intensity of the protein bands at the Band 1 (95–130 kDa, e.g., C-protein or α-actinin) and Band 2 (36–55 kDa, e.g., tropomyosin or troponin-T) in the vacuum tumbling marination group significantly reduced, among which Band 2 was less detectable. The difference in the relative intensity of the myofibrillar protein bands maybe due to denaturation of the protein during vacuum tumbling marination, resulting in protein fragmentation. Combined with the results of total sulfhydryl content and surface hydrophobicity in the current study, it appears that vacuum tumbling promotes protein degradation and increases the degree of protein denaturation, finally affecting the quality attributes of marinated chicken meat.
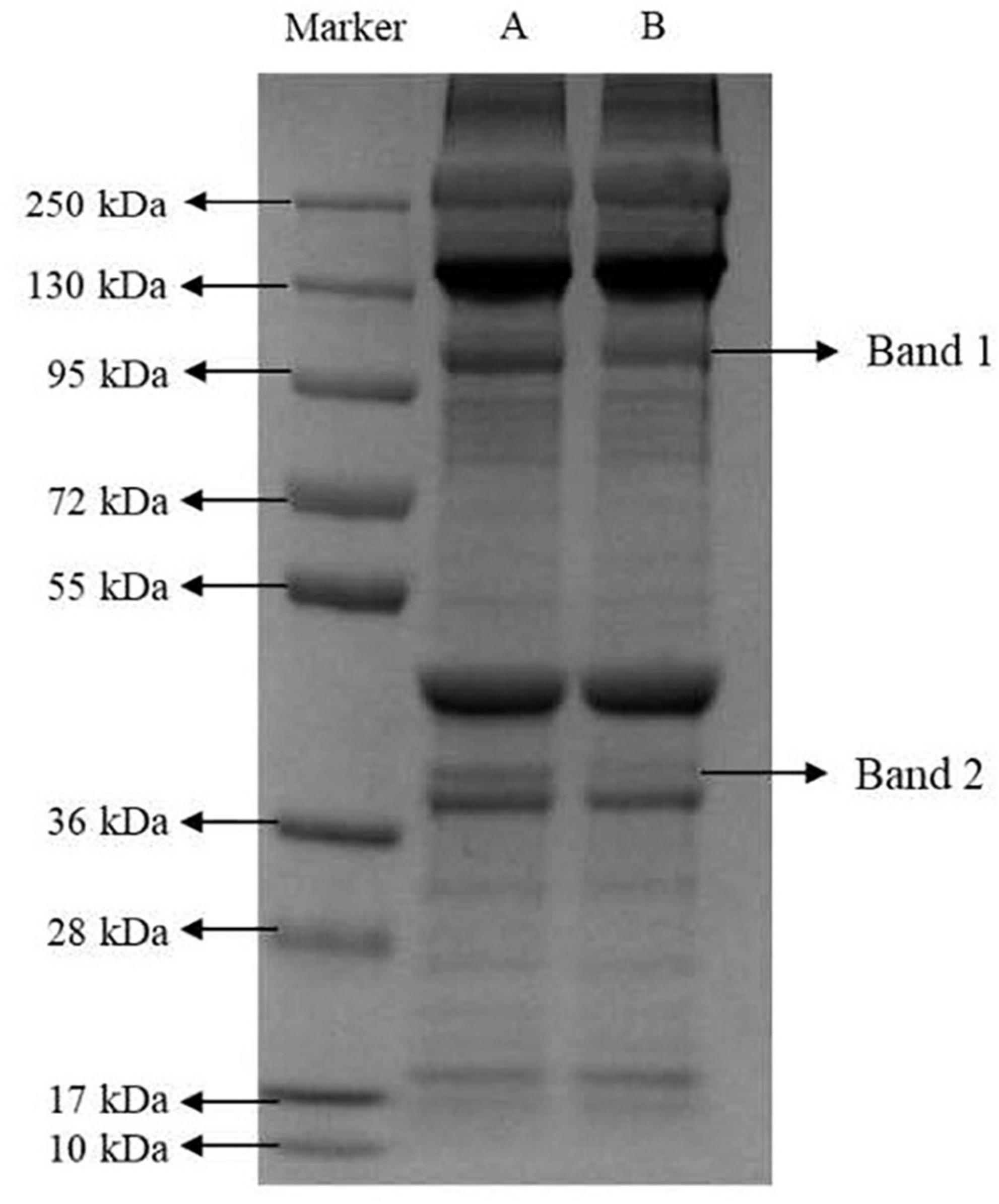
Figure 3. Representative image of SDS-PAGE gels of myofibrillar proteins in chicken breast by marinating treatment. The chicken breasts treated with immersion and vacuum tumbling marinating were denoted as A and B, respectively.
Conclusion
The study explored the effects of vacuum tumbling and immersion marination on the overall quality, microstructure, water mobility, protein changes, and denaturation of Xueshan chicken. Overall, the vacuum tumbling had more damaged muscle fiber and higher marination absorption rate than that of immersion marination, resulting in desired meat tenderness, water retention, and texture characteristics of chicken. Accordingly, vacuum tumbling significantly decreased the total sulfhydryl content and protein solubility, and increased the surface hydrophobicity and free sulfhydryl content compared to immersion marination. These results demonstrated that vacuum tumbling significantly increased the degree of protein denaturation and potentially improved the quality of marinated chicken.
Data availability statement
The original contributions presented in this study are included in the article/supplementary material, further inquiries can be directed to the corresponding author.
Author contributions
QG and RL: conceptualization, resources, and writing – review and editing. SC: methodology. ZJ: software. SG and YW: validation. GX: formal analysis. SC and SG: investigation. ZK: data curation. QG: writing – original draft preparation, project administration, and funding acquisition. MW: visualization. HY: supervision. All authors have read and agreed to the published version of the manuscript.
Funding
This research was funded by Jiangsu Key Research and Development Program (BE2020400 and BE2021320), Natural Science Foundation of Jiangsu Province (BK20190888) and National Natural Science Foundation of China (No. 31901610), High Level Talent Support Program of Yangzhou University, Cooperation of Yangzhou and Yangzhou University in Construction of Science and Technology Innovation Platform Project (YZ2020267).
Acknowledgments
The technical support of Opening Research of Jiangsu Key Laboratory of Animal Genetic Breeding and Molecular Design (AGBMD2020) is acknowledged.
Conflict of interest
The authors declare that the research was conducted in the absence of any commercial or financial relationships that could be construed as a potential conflict of interest.
Publisher’s note
All claims expressed in this article are solely those of the authors and do not necessarily represent those of their affiliated organizations, or those of the publisher, the editors and the reviewers. Any product that may be evaluated in this article, or claim that may be made by its manufacturer, is not guaranteed or endorsed by the publisher.
References
1. Sáyago-ayerdi S, Brenes A, Viveros A, Goñi I. Antioxidative effect of dietary grape pomace concentrate on lipid oxidation of chilled and long-term frozen stored chicken patties. Meat Sci. (2009) 83:528–33. doi: 10.1016/j.meatsci.2009.06.038
2. Erdaw MM, Beyene WT. Trends, prospects and the socio-economic contribution of poultry production in sub-Saharan Africa: a review. Worlds Poult Sci J. (2022) 78:835–52.
3. Huang X, Ahn D. The incidence of muscle abnormalities in broiler breast meat -a review. Korean J Food Sci Anim Resour. (2018) 38:835–50.
4. Wang YH, Lin J, Wang J, Wu SG, Qiu K, Zhang HJ, et al. The role of incubation conditions on the regulation of muscle development and meat quality in poultry. Front Physiol. (2022) 13:883134. doi: 10.3389/fphys.2022.883134
5. Weng KQ, Huo WR, Li Y, Zhang Y, Zhang Y, Chen GH, et al. Fiber characteristics and meat quality of different muscular tissues from slow-and fast-growing broilers. Poult Sci. (2022) 101:101537. doi: 10.1016/j.psj.2021.101537
6. Weng KQ, Li Y, Huo WR, Zhang Y, Cao ZF, Zhang Y, et al. Comparative phosphoproteomic provides insights into meat quality differences between slow- and fast-growing broilers. Food Chem. (2022) 373:131408. doi: 10.1016/j.foodchem.2021.131408
7. Wang LD, Kong LL, Hu XD, Bai H, Wang ZX, Jiang Y, et al. Effect of stocking density on performance, meat quality and cecal bacterial communities of yellow feather broilers. Anim Biotechnol. (2021) 22:1–11. doi: 10.1080/10495398.2021.1898413
8. Alvarado C, McKee S. Marination to improve functional properties and safety of poultry meat. J Appl Poult Res. (2007) 16:113–20.
9. Zhou YJ, Hu MQ, Wang L. Effects of different curing methods on edible quality and myofibrillar protein characteristics of pork. Food Chem. (2022) 387:132872. doi: 10.1016/j.foodchem.2022.132872
10. Xiong GY, Fu XY, Pan DM, Qi J, Xu XL, Jiang XJ. Influence of ultrasound-assisted sodium bicarbonate marination on the curing efficiency of chicken breast meat. Ultrason Sonochem. (2020) 60:104808. doi: 10.1016/j.ultsonch.2019.104808
11. Yusop SM, O’Sullivan MG, Kerry JP. Marinating and enhancement of the nutritional content of processed meat products. 1st ed. In: JP Kerry, JF Kerry editors. Processed Meats: improving Safety, Nutrition and Quality. (Vol. 66) (Philadelphia, PA: Woodhead Publishing Limited) (2011). p. 421–49. doi: 10.1016/j.dib.2019.104801
12. Conde E, Gaillard J, Núñez C, Piai M, Ramallo AV. Towards the string dual of tumbling and cascading gauge theories. Phys Lett B. (2012) 709:385–9.
13. Zhu C, Yin F, Tian W, Zhu Y, Zhao L, Zhao G. Application of a pressure-transform tumbling assisted curing technique for improving the tenderness of restructured pork chops. LWT Food Sci Technol. (2019) 111:125–32.
14. Bosse R, Thiermann N, Gibis M, Schmidt H, Weiss J. Effect of mechanical curing treatments on particle distribution to simulate non-motile bacteria migration in cured raw ham. J Food Eng. (2017) 194:58–66. doi: 10.1016/j.jfoodeng.2016.09.005
15. Szerman N, Gonzalez C, Sancho AM, Grigioni G, Carduza F, Vaudagna SR. Effect of whey protein concentrate and sodium chloride addition plus tumbling procedures on technological parameters, physical properties and visual appearance of sous vide cooked beef. Meat Sci. (2007) 76:463–73. doi: 10.1016/j.meatsci.2007.01.001
16. Bosse R, Müller A, Gibis M, Weiss A, Schmidt H, Weiss J. Recent advances in cured raw ham manufacture. Crit Rev Food Sci Nutr. (2018) 58:610–30. doi: 10.1080/10408398.2016.1208634
17. Zhan X, Sun D, Zhu Z, Wang Q. Improving the quality and safety of frozen muscle foods by emerging freezing technologies: a review. Crit Rev Food Sci Nutr. (2018) 58:2925–38. doi: 10.1080/10408398.2017.1345854
18. Benjakul S, Visessanguan W, Thongkaew C, Tanaka M. Comparative study on physicochemical changes of muscle proteins from some tropical fish during frozen storage. Food Res Int. (2003) 36:787–95.
19. Li YP, Zhang XH, Lu F, Kang ZL. Effect of sodium bicarbonate and sodium chloride on aggregation and conformation of pork myofibrillar protein. Food Chem. (2021) 350:129233. doi: 10.1016/j.foodchem.2021.129233
20. Ishiwatari N, Fukuoka M, Sakai N. Effect of protein denaturation degree on texture and water state of cooked meat. J Food Eng. (2013) 117:361–9.
21. Yu Q, Wu W, Tian X, Jia F, Xu L, Dai R, et al. Comparative proteomics to reveal muscle-specific beef color stability of holstein cattle during post-mortem storage. Food Chem. (2017) 229:769–78. doi: 10.1016/j.foodchem.2017.03.004
22. Petracci M, Laghi L, Rimini S, Rocculi P, Capozzi F, Cavani C. Chicken breast meat marinated with increasing levels of sodium bicarbonate. J Poult Sci. (2014) 51:206–12. doi: 10.3382/ps/peu063
23. Popat DS, Manohar GR, Ramamurthy N, Babu RN, Mir NA, Jaywant RJ. Effect of vacuum tumbling on quality and economy of tandoori chicken prepared from commercial broiler chicken meat. Indian J Poult Sci. (2017) 52:322–6.
24. Perumalla AVS, Saha A, Lee Y, Meullenet JF, Owens CM. Marination properties and sensory evaluation of breast fillets from air-chilled and immersion-chilled broiler carcasses. Poult Sci. (2011) 90:671–9. doi: 10.3382/ps.2010-00845
25. Bowker B, Zhuang H. Freezing-thawing and sub-sampling influence the marination performance of chicken breast meat. Poult Sci. (2017) 96:3482–8. doi: 10.3382/ps/pex117
26. Saha A, Perumalla A, Lee Y, Meullenet JF, Owens CM. Tenderness, moistness, and flavor of pre- and postrigor marinated broiler breast fillets evaluated by consumer sensory panel. Poult Sci. (2009) 88:1250–6. doi: 10.3382/ps.2008-00236
27. Bowker BC, Callahan JA, Solomon MB. Effects of hydrodynamic pressure processing on the marination and meat quality of turkey breasts. Poult Sci. (2010) 89:1744–9. doi: 10.3382/ps.2009-00484
28. Peiretti P, Gai F, Rotolo L, Brugiapaglia A, Gasco L. Effects of tomato pomace supplementation on carcass characteristics and meat quality of fattening rabbits. Meat Sci. (2013) 95:345–51. doi: 10.1016/j.meatsci.2013.04.011
29. Kauffman R, Eikelenboom G, van der Wal PG, Engel B, Zaar M. A comparison of methods to estimate water-holding capacity in post-rigor porcine muscle. Meat Sci. (1986) 18:307–22. doi: 10.1016/0309-1740(86)90020-3
30. Xia X, Kong B, Liu J, Diao X, Liu Q. Influence of different thawing methods on physicochemical changes and protein oxidation of porcine longissimus muscle. LWT Food Sci Technol. (2012) 46:280–6.
31. Chen L, Zhou G, Zhang W. Effects of high oxygen packaging on tenderness and water holding capacity of pork through protein oxidation. Food Bioproc Tech. (2015) 8:2287–97.
32. Malila Y, Petracci M, Benjakul S, Visessanguan W. Effect of tumbling marination on marinade uptake of chicken carcass and parts quality. Rev Bras Cienc Avic. (2017) 19:61–8.
33. Kang D, Gao X, Ge Q, Zhou G, Zhang W. Effects of ultrasound on the beef structure and water distribution during curing through protein degradation and modification. Ultrason Sonochem. (2017) 38:317–25. doi: 10.1016/j.ultsonch.2017.03.026
34. Park D, Xiong YL. Oxidative modification of amino acids in porcine myofibrillar protein isolates exposed to three oxidizing systems. Food Chem. (2007) 103:607–16.
35. Fu Q, Liu R, Zhang W, Li Y, Wang J, Zhou G. Effects of different packaging systems on beef tenderness through protein modifications. Food Bioproc Tech. (2015) 8:580–8.
36. Culler R, Smith G, Cross H. Relationship of myofibril fragmentation index to certain chemical, physical and sensory characteristics of bovine longissimus muscle. J Food Sci. (1978) 43:1177–80.
37. Thangavelu KP, Kerry JP, Tiwari BK, Mcdonnell CK. Novel processing technologies and ingredient strategies for the reduction of phosphate additives in processed meat. Trends Food Sci Technol. (2019) 94:43–53. doi: 10.1016/j.tifs.2019.10.001
38. Baublits RT, Pohlman FW, Brown AH, Johnson ZB. Effects of sodium chloride, phosphate type and concentration, and pump rate on beef biceps femoris quality and sensory characteristics. Meat Sci. (2005) 70:205–14. doi: 10.1016/j.meatsci.2004.12.011
39. Gao T, Li JL, Zhang L, Jiang Y, Liu Y, Zhang X, et al. Effect of different tumbling marination methods and time on the quality characteristics of prepared pork chops. Food Sci Technol. (2015) 35:445–51.
40. Yusop SM, O’Sullivan MG, Kerry JF, Kerry JP. Influence of processing method and holding time on the physical and sensory qualities of cooked marinated chicken breast fillets. LWT Food Sci Technol. (2012) 46:363–70.
41. Lopez K, Schilling MW, Armstrong TW, Smith BS, Corzo A. Sodium chloride concentration affects yield, quality, and sensory acceptability of vacuum-tumbled marinated broiler breast fillets. Poult Sci. (2012) 91:1186–94. doi: 10.3382/ps.2011-01733
42. Gao T, Li JL, Zhang L, Jiang Y, Ma R. Effect of different tumbling marination treatments on the quality characteristics of prepared pork chops. Asian Australas J Anim Sci. (2015) 28:260–7. doi: 10.5713/ajas.14.0511
43. Anonymous. Tumbling and subsequent aging improves tenderness of beef steaks. J Anim Sci. (2022) 100:skac102.
44. Bertram HC, Kohler A, Backer U, Ofstad R, Andersen HJ. Heat-induced changes in myofibrillar protein structures and myowater of two pork qualities. a combined FT-IR spectroscopy and low-field NMR relaxometry study. J Agric Food Chem. (2006) 54:1740–6. doi: 10.1021/jf0514726
45. Kim HW, Yan FF, Hu JY, Cheng HW, Kim YHB. Effects of probiotics feeding on meat quality of chicken breast during postmortem storage. Poult Sci. (2016) 95:1457–64. doi: 10.3382/ps/pew055
46. Chandrapala J, Zisu B, Palmer M, Kentish S, Ashokkumar M. Effects of ultrasound on the thermal and structural characteristics of proteins in reconstituted whey protein concentrate. Ultrason Sonochem. (2011) 18:951–7. doi: 10.1016/j.ultsonch.2010.12.016
47. Riebroy S, Benjakul S, Visessanguan W, Erikson U, Rustad T. Acid-induced gelation of natural actomyosin from atlantic cod (Gadus morhua) and burbot (Lota lota). Food Hydrocoll. (2009) 23:26–39.
49. Gülseren İ, Güzey D, Bruce BD, Weiss J. Structural and functional changes in ultrasonicated bovine serum albumin solutions. Ultrason Sonochem. (2007) 14:173–83.
Keywords: vacuum tumbling, immersion, protein denaturation, meat texture, water retention
Citation: Ge Q, Guo S, Chen S, Wu Y, Jia Z, Kang Z, Xiong G, Yu H, Wu M and Liu R (2022) A comparative study of vacuum tumbling and immersion marination on quality, microstructure, and protein changes of Xueshan chicken. Front. Nutr. 9:1064521. doi: 10.3389/fnut.2022.1064521
Received: 08 October 2022; Accepted: 07 November 2022;
Published: 23 November 2022.
Edited by:
Zhaojun Wei, Hefei University of Technology, ChinaReviewed by:
Kang Dacheng, Linyi University, ChinaZhichao Xiao, Yunnan Agricultural University, China
Copyright © 2022 Ge, Guo, Chen, Wu, Jia, Kang, Xiong, Yu, Wu and Liu. This is an open-access article distributed under the terms of the Creative Commons Attribution License (CC BY). The use, distribution or reproduction in other forums is permitted, provided the original author(s) and the copyright owner(s) are credited and that the original publication in this journal is cited, in accordance with accepted academic practice. No use, distribution or reproduction is permitted which does not comply with these terms.
*Correspondence: Rui Liu, cnVpbGl1QHl6dS5lZHUuY24=