- 1Department of Gastroenterology, Institute of Gastroenterology of Guangdong Province, Guangdong Provincial Key Laboratory of Gastroenterology, Nanfang Hospital, Southern Medical University, Guangzhou, China
- 2Guangzhou Zhiyi Biotechnology Co., Ltd., Guangzhou, China
Radiation-induced intestinal injury is characterized by intestinal barrier impairment. However, the therapeutic effects of probiotics for intestinal epithelial barrier repair in a mouse model of radiation-induced intestinal injury remain unclear. Previously, we isolated a strain of Bacteroides fragilis from the feces of a healthy infant and named it as B. fragilis strain ZY-312 (B. fragilis). In this study, we showed that B. fragilis can ameliorate radiation-induced intestinal injury in mice, manifested by decreased weight loss, intestinal length shortening, and intestinal epithelial cell (IEC) shedding. Moreover, we found that B. fragilis promoted IEC proliferation, stem cell regeneration, mucus secretion, and tight junction integrity by upregulating the STAT3 signaling pathway, through an experimental verification in Stat3△IEC mice (STAT3 defects in intestinal epithelial cells). Thus, the underlying protective mechanism of B. fragilis in radiation-induced intestinal injury is related to IEC proliferation, stem cell regeneration, goblet cell secretion, and tight junction repair via activation of the STAT3 signaling pathway. In addition, the therapeutic effects of B. fragilis were studied to provide new insights into its application as a functional and clinical drug for radiation-induced intestinal injury after radiotherapy.
Introduction
With the application of radiation delivery techniques in oncotherapy, 70% of cancer patients have benefited from radiotherapy, and 25% of them have become survivors (1, 2). However, gastrointestinal syndrome is a potentially serious complication of radiation therapy. Gastrointestinal syndrome is complex, characterized by gastrointestinal toxicity and radiation-induced intestinal injury. The major symptoms of radiation-induced intestinal injury in humans include diarrhea, abdominal pain, constipation, hematochezia, and weight loss (3). The acute radiation-induced intestinal injury occurs within 3 months, while chronic radiation-induced intestinal injury appears >3 months after radiotherapy (4, 5). It has been reported that 90% of cancer patients receiving abdominal radiotherapy develop radiation-induced intestinal injury within a few weeks (6) and life-threatening systemic infections after 3 months (7), thus compromising the patients’ quality of life. Therefore, it has become an urgent priority to seek effective therapies for side effects caused by radiotherapy.
Due to the fact that small intestinal epithelial cells (SI IECs) are major sensitive sites of abdominal radiotherapy (8), the healthy intestine is inevitably exposed to radiation during radiotherapy for abdominal and pelvic malignant tumors (9). Although the process of intestinal regeneration is initiated after radiation injury, there is also a rapid loss of LGR5 + intestinal stem cells (ISCs) and proliferating progenitor cells (10). The ionizing radiation will cause injury to basal epithelial cells and impede their renewal, leading to histologically detectable alterations of IECs such as decreased intestinal villous height and number, inflammation, bowel wall edema, and fibrin precipitation (11). In addition, manifestations such as diarrhea occur in humans and mice a few weeks after radiation injury due to the atrophy, ulceration, and high permeability of IECs (12). There is currently no effective therapeutic radioprotectant for general clinical application. Some clinical trials have shown that probiotics have the potential therapeutic effects to mitigate intestinal radiation injuries (13). A double-blind, placebo-controlled clinical trial including approximately 500 patients assigned to either a probiotic preparation (VSL#3) or placebo treatment after receiving adjuvant postoperative radiation therapy reported decreased incidence and severity of radiation-induced intestinal injury in the VSL#3 treatment group (14). Moreover, the application of Lactobacillus rhamnosus in radiation therapy improved stool consistency and reduced bowel movements and abdominal discomfort (15), suggesting that probiotics have the potential to become radioprotectants for clinical application.
Bacteroides fragilis strain ZY-312 (B. fragilis), belonging to the genus Bacteroides, was isolated from the feces of a healthy infant (16). O’Toole et al. (17) reported that B. fragilis has the potential to become a second-generation probiotic with biological applications. Using a metabolic engineering approach, our team previously found that B. fragilis mainly existed in the small intestine and colon (18), and promoted intestinal tissue proliferation, thus relieving antibiotic-related diarrhea in a rat model (19). In addition, we reported that B. fragilis inhibited intestinal epithelial cell apoptosis in a Cronobacter sakazakii-induced neonatal necrotizing enterocolitis model (20), and restored intestinal barrier to prevent diarrhea in a mouse model of Clostridium difficile infection (21). This suggests that B. fragilis has the potential to promote intestinal epithelium regeneration, thereby relieving diarrhea caused by abdominal radiotherapy. B. fragilis performs the probiotic therapeutic roles in intestinal disease. However, the effects and mechanism of B. fragilis in radiation-induced intestinal injury remain unclear. In this study, we aimed to evaluate the effects and underlying mechanism of B. fragilis in radiation-induced intestinal injury in mice. We hope that our findings will provide new insight into the treatment of the radiation-induced intestinal injury.
Materials and methods
Establishment of radiation-induced intestinal injury model
The mouse model of radiation-induced intestinal injury was constructed by previously described methods (22). The C57BL/6 mice and Stat3△IEC mice were gavaged with B. fragilis (1 × 109 CFU per mouse) or B. fragilis-derived capsular polysaccharide (PSA) (50 μg/mouse) for 14 days during the pretreatment stage, then administered to mice with radiation. Radiation was given using the irradiator (MultiRad, Faxitron, USA) at 26 Gy/min with 225 kVp X-rays using a 0.3 mm copper filter. (1) The control group is the following: Mice were treated with phosphate buffer (PBS) orally. (2) TAI group: Mice were treated with PBS, and exposed to 10 Gy total of abdominal irradiation. (3) TBI group: Mice were treated with PBS, and exposed to 4.5 Gy total body irradiation. (4) TAI/TBI + ZY-312 group: Mice were treated with B. fragilis (1 × 109 CFU per mouse) through an oral route, dissolved in sterile water in 0.2 ml volume per mice for 15 consecutive days after 4.5 Gy TBI or 10 Gy TAI. All experiments were performed using 6- to 8-week-old sex-matched mice. C57BL/6 mice were purchased from SPF (Beijing) Biotechnology Co., Ltd. (Beijing, China), and STAT3 conditional gene knockout mice (Stat3△IEC mice, the primers for gene identification were showed in Supplementary Table 2) were purchased from GemPharmatech Co., Ltd. (Jiangsu, China).
Culture of Bacteroides fragilis strain ZY-312
Bacteroides fragilis strain ZY-312 was cultured in 5% fetal bovine serum, 9.5 ml of tryptic soy broth, and 100 μl of passaging solution at 37°C for 24 h under anaerobic conditions.
Histological stain
The small intestinal tissue of mice was fixed in 4% paraformaldehyde for 24 h, embedded in paraffin, and cut into sections at a thickness of 5 μm (The histopathology associated index of intestine was evaluated though the criterion in Supplementary Table 1). For immunohistochemical staining, the intestinal sections were subjected to deparaffinization, hydration, antigen retrieval, quenching of endogenous peroxidase, and blocking procedures. All slices were then incubated with the primary antibodies against pSTAT3 (CST #9145) and Ki-67 (Abcam, England, Ab16667) at 4°C overnight followed by incubation with biotinylated secondary antibodies for 30 min and visualization using a 3,3′-Diaminobenzidine Kit (ZSGB-BIO, Beijing, China). For immunofluorescence staining for the detection of MUC2 (Abcam, England, Ab272692), Lgr5 (NBP1-28904SS), ZO-1 (Abcam, England, Ab221547), and Claudin-1 (Abcam, England, Ab242370), the colonic sections were processed by the methods described above. Periodic Acid-Schiff stain (PAS) staining (Biossci, China) was performed following the manufacturer’s protocols.
Small intestinal epithelial cells isolation
The small intestinal was isolated from mice. Then SI was washed with PBS and cut into 5 mm pieces, followed by digestion with buffer (5 mM EDTA and 2 mM dithiothreitol in Hanks balanced salt solution; Sigma, St. Louis, MO, USA) at 37°C for 30 min on a rotating platform. Through the digestion stopping stage with Hanks balanced salt solution, the solution was filtered through a 70 μm cell strainer. Lastly, the filtered solution was centrifuged for 10 min at 400 g (4°C) to obtain SI epithelial cells (23).
Western blot
Western blotting was performed followed by previous protocols (24). The proteins were separated by SDS–PAGE and analyzed by immunoblotting with rabbit polyclonal antiserum to pSTAT3 (CST #9145, Danvers, MA, USA), STAT3 (CST #4904), PCNA (CST #13110), P38 (CST #9212), pmTOR (CST #4511), mTOR (CST #4511), pJAK2 (CST #4695), pNF-KB (CST#4370), Glyceraldehyde-3-phosphate dehydrogenase (GAPDH) (CST #5174), ZO-1 (Proteintech 21773-1-AP), Claudin-1 (Proteintech 13050-1-AP), MUC2 (Abcam, England, 272692-10), Lgr5 (Abcam, England, 75850) antibodies were used to measure colonic epithelial protein expression.
16S rRNA analysis
The fecal microbiota composition of mice was determined by 16S rRNA gene amplification. Briefly, TGuide S96 Magnetic Soil/Stool DNA Kit (Tiangen Biotech Co., Ltd., Beijing, China) was used to extract genomic DNA from feces. DNA concentration and integrity were measured by a microplate reader (GeneCompang Limited, synergy HTX, United States) and agarose gel electrophoresis, respectively. The 16S rRNA gene V3–V4 region was amplified from the genomic DNA in a 25 μl reaction using the universal bacterial primers: (338F, 50–ACTCCTACGGGAGGCAGCA–30, and 806R, 50–GGACTACHVGGGTWTCTAAT–30). The PCR products were quantified by using a NanoDrop 2000 spectrophotometer (Thermo Fisher Scientific, Waltham, MA, United States) and purified with Monarch® DNA gel Extraction Kit (New England Biolabs, USA). Sequencing was performed on an Illumina NovaSeq 6000 with two paired-end read cycles of 250 bases each (Illumina Inc., San Diego, CA, United States; Biomarker Co., Ltd., Beijing, China). The Trimmomatic software v0.33 was used to analyze the raw data. After trimming, amplicon sequence variants (ASVs) were generated by using USEARCH 10.0 with a 97% similarity cutoff. The representative read of each ASV was selected by using the Quantitative Insights into Microbial Ecology (QIIME) package. All representative reads were annotated and blasted against the Silva database (Version 132) using the Ribosomal Database Project (RDP) classifier (confidence threshold was 70%). The microbial richness and diversity in fecal content samples were estimated using the alpha diversity that includes the Shannon index. The UniFrac distance matrix performed by QIIME software was used for the unweighted UniFrac Principal coordinates analysis (PCoA), and phylogenetic tree construction. Linear discriminant analysis effect size (LEfSe) based linear discriminant analysis (LDA) and cladogram were generated to assess differentially abundant microbial taxa. The 16S rRNA gene amplicon sequencing and analysis were conducted by Biomarker Technologies Co., Ltd. (Beijing, China).
Statistical analysis
Statistical analyses were performed using GraphPad Prism 8.0 (GraphPad Software). All variables are expressed as Means ± SEM, as noted in the figure legends. Two-way ANOVA was used to compare body weights. The one-way ANOVA with Tukey’s tests was used for comparisons among three or more groups. While non-parametric data were analyzed with a Mann–Whitney U-test. P < 0.05 was considered as significance.
Results
Bacteroides fragilis alleviated radiation-induced intestinal injury in mice through Bacteroides fragilis-derived polysaccharide
To evaluate the effect of B. fragilis strain ZY-312 on radiation-induced intestinal injury, we constructed the corresponding mouse model for total body radiation (TBI) and administered the mice with B. fragilis orally (Figures 1A,B). Compared with the TBI group, mice receiving B. fragilis (TBI + ZY-312 group) had significantly lower weight loss (Figure 1C) and slower SI and colon length shortening (Figure 1D). To explore the effect of B. fragilis on the IECs, the histopathological results revealed that B. fragilis supplementation attenuated SI epithelial cell damage (Figure 1E), reduced overall HAI, and resulted in longer lengths of SI crypts (Figure 1F). Furthermore, we found that B. fragilis promoted SI mucus secretion in radiation-induced intestinal injury (Figure 1G). Concurrently, we constructed a radiation-induced intestinal injury mouse model for total abdominal radiation (TAI) and administered the mice with B. fragilis and B. fragilis-derived capsular polysaccharide (PSA) (Figures 1A,B). Compared with the TAI group, mice receiving B. fragilis (TAI + ZY-312 group) had significantly lower weight loss (Figure 2A) and slower intestinal (SI and colon) length shortening (Figures 2B–C). To explore the effect of B. fragilis and PSA on the IECs, the histopathological results revealed that B. fragilis supplementation and PSA obviously attenuated SI epithelial cell damage (Figure 2D), reduced overall histopathology-associated index (HAI) (Figure 2E), and resulted in longer lengths of SI crypts (Figure 2F). Thus, B. fragilis may alleviate abdominal radiation-induced intestinal injury in mice through B. fragilis-derived PSA. And it suggested that B. fragilis promoted the integrity of SI epithelium to alleviate radiation-induced intestinal injury in mice.
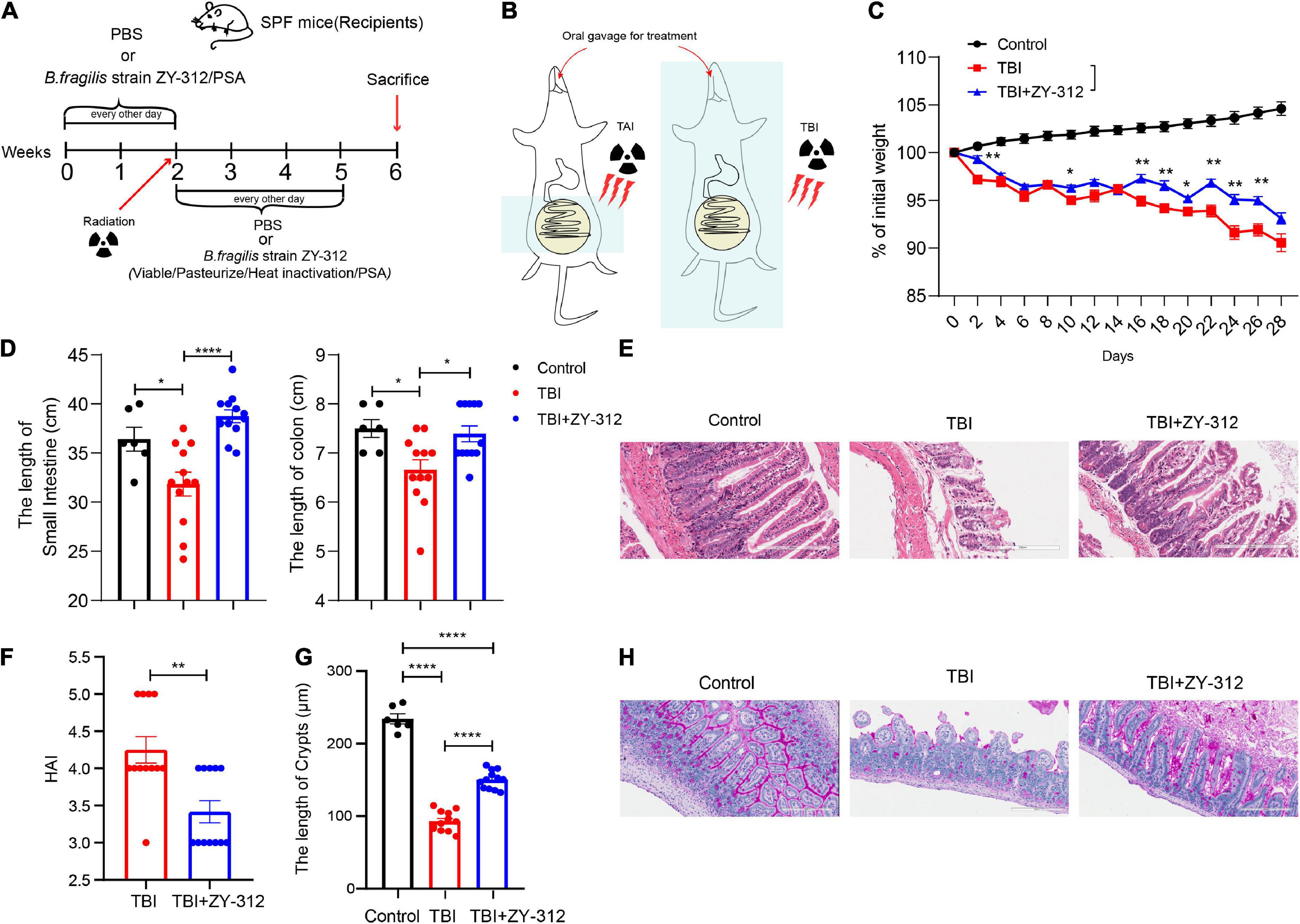
Figure 1. Bacteroides fragilis alleviated total body radiation-induced intestinal injury in mice. (A,B) The model of radiation-induced intestinal injury in mice. (C) The p-value of TAI group vs. TAI + ZY-312 group in percent (%) of weight loss is following: percent (%) of weight loss p-value (TBI group vs. TBI + ZY-312 group), Day2 0.0025, Day10 0.0483, Day16 0.0064, Day18 0.0017, Day20 0.0236, Day22 0.0017, Day24 0.0024, and Day26 0.0020. (D) The p-value between groups are following: p-value the small intestine length the colon length (Control group vs. TBI group) 0.0261 0.0263 (Control group vs. TBI + ZY-312 group) 0.3468 0.9326 (TBI group vs. TBI + ZY-312 group) <0.0001 and 0.0171. (E) The histopathology- associated index (HAI) between groups. (F) The p-value between groups are following: p-value HAI SI crypts (Control group vs. TBI group)/<0.0001 (Control group vs. TBI + ZY-312 group)/<0.0001 (TBI group vs. TBI + ZY-312 group) 0.0017 and <0.0001. (G) The length of SI crypts between groups. (H) Periodic acid-Schiff stain (PAS) staining of SI. Control group (N = 6), TBI group (N = 12), TBI + ZY-312 group (N = 12). N, the number of mice. The data are presented as mean ± SEM, and *p < 0.05, **p < 0.01, **p < 0.001, **p <0.0001.
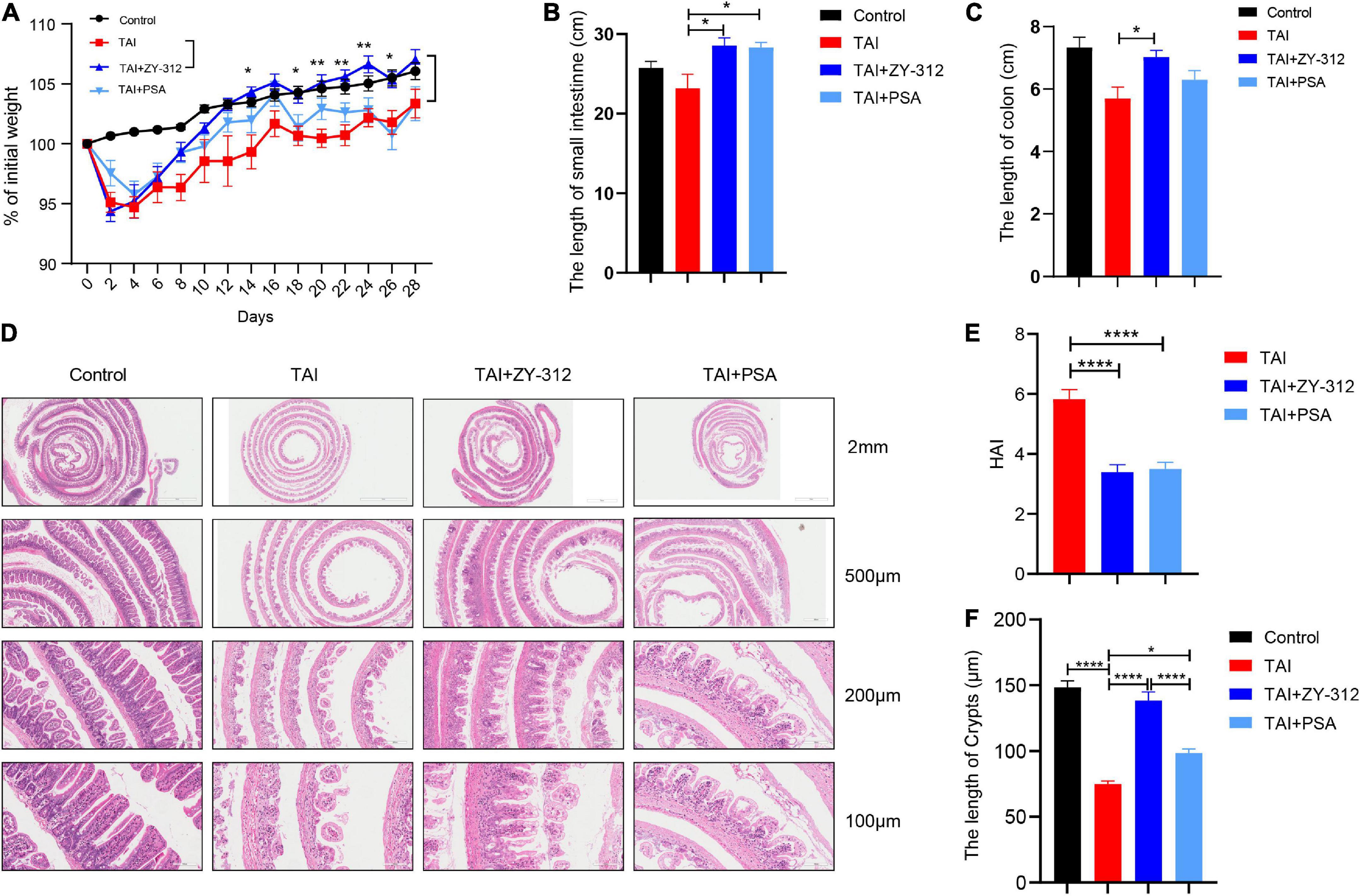
Figure 2. Bacteroides fragilis alleviated abdominal radiation-induced intestinal injury in mice through B. fragilis-derived polysaccharide (PSA). (A) The p-value between groups are following: percent (%) of weight loss p-value (TAI group vs. TAI + ZY-312 group), Day14 0.0323, Day18 0.0254, Day20 0.0014, Day22 0.0017, Day24 0.0027, and Day26 0.0431. (B) The p-value between groups are following: p-value the small intestine length (TAI group vs. TAI + ZY-312 group) 0.0149 and (TAI group vs. TAI + PSA group) 0.0202. (C) The p-value between groups are following: p-value the colon length (TAI group vs. TAI + ZY-312 group) 0.0319. (D) The histopathology of small intestinal at different magnifications. (E) The p-value between groups are following: p-value HAI (TAI group vs. TAI + ZY-312 group) <0.0001 and (TAI group vs. TAI + PSA group) <0.0001. (F) The p-value between groups are following: p-value SI crypts (Control group vs. TAI group) <0.0001, (TAI group vs. TAI + ZY-312 group) <0.0001, (TAI group vs. TAI + PSA group) 0.014, and (TAI + ZY-312 group vs. TAI + PSA group) <0.0001. The data are presented as mean ± SEM, and *p < 0.05, **p < 0.01, **p < 0.001, **p <0.0001.
Bacteroides fragilis promoted stem cell and goblet cell regeneration and accelerated tight junction repair
Leucine-rich repeat-containing G-protein coupled receptor 5 (LGR5+) ISCs cells are considered active multipotent intestinal stem cells because they have the capacity to divide and differentiate into different types of intestinal epithelial cells, such as goblet cells and enterocytes (11). Thus the degree of stem cell injury is an important indicator for damage repair after radiation. In addition, intestinal epithelial tight junctions, including zonula occludens-1 (ZO-1), occludins, and claudins, are also highly sensitive to ionizing radiation with the disruption of IECs (25). Next, we attempted to determine whether B. fragilis has the capacity to alleviate the destruction of SI IECs after radiation. B. fragilis was found to promote SI IECs proliferation (Figure 3A). Regarding the types of IECs affected, the small intestinal histological results showed that B. fragilis promoted stem cell proliferation (Figure 3B), goblet cell secretion (Figure 3C), and tight junction protein expression (Figure 3D). This indicated that B. fragilis promoted stem cell and goblet cell regeneration, and accelerated tight junction repair in radiation-induced intestinal injury in mice.
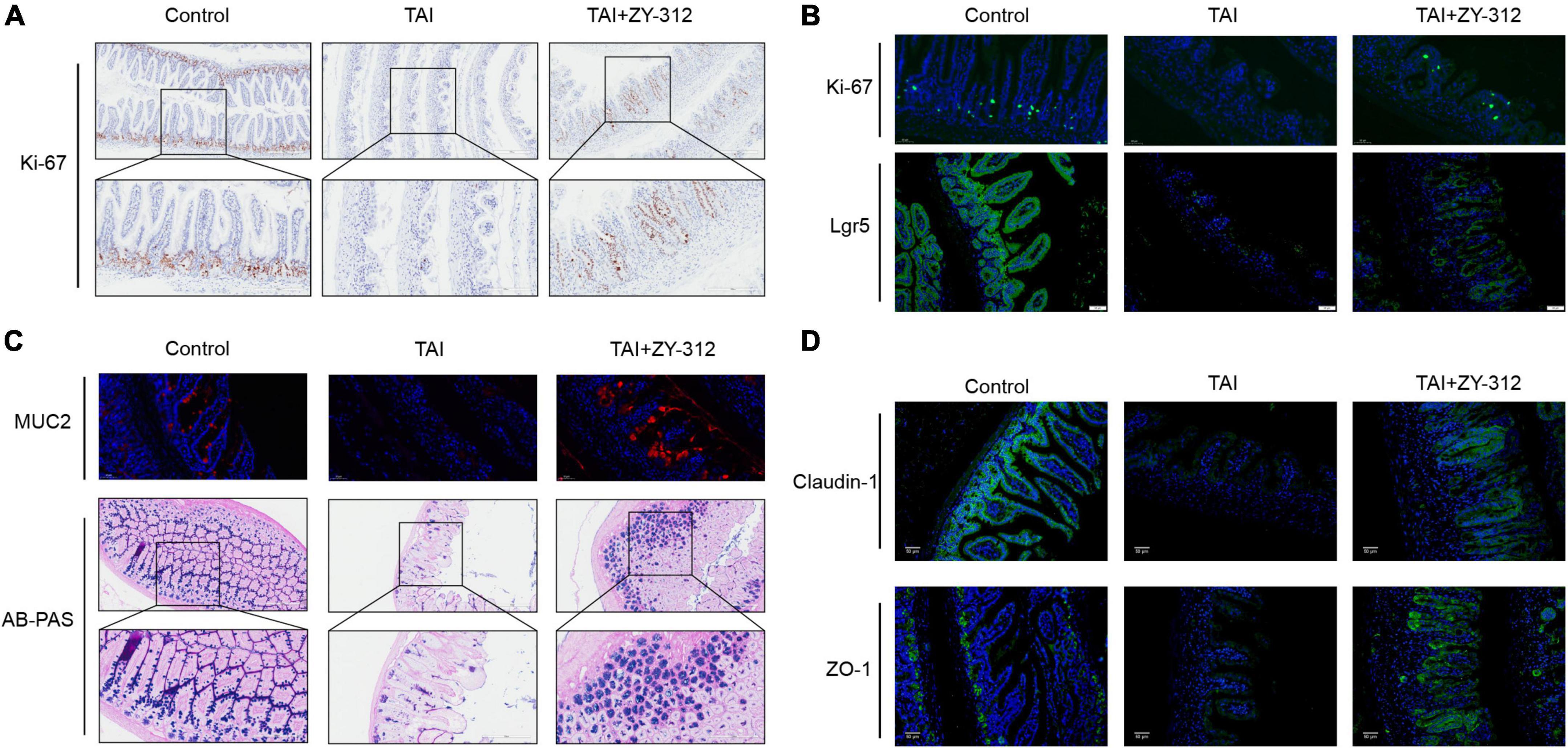
Figure 3. Bacteroides fragilis promoted intestinal epithelial cell proliferation, stem cell regeneration, goblet cell secretion, and tight junction repair. (A) The immunohistochemical staining of Ki-67 in slower intestinal (SI) between groups. (B) The immunofluorescence staining of Ki-67 and Lgr5. (C) The immunofluorescence and Alcian blue-periodic acid-Schiff staining (AB-PAS) of SI goblet cells. (D) The immunofluorescence staining of Claudin-1 and ZO-1.
Bacteroides fragilis did not significantly alter the intestinal microbiota to relieve radiation-induced intestinal injury in mice
Certain types of intestinal microbiota reside in the mucus layer (26), and mucus has the capacity of resisting pathogenic bacteria in colitis (27). B. fragilis has a positive influence on mucus secretion, which suggests that it may alter the intestinal microbiota, thus relieving radiation-induced intestinal injury in mice. Hence we analyzed intestinal microbiota by 16S rRNA gene sequencing. The main intestinal microbiota phyla were Firmicutes, Bacteroidetes, and Proteobacteria. And the microbial community bar plot showed the relative abundance of phylum Firmicutes increased and that of phylum Bacteroidetes decreased gradually in the TAI + ZY-312 group compared to the TAI group, although there was no statistically significant difference (Figures 4A,B). At the genus level, the genera of the Lachnospiraceae_NK4A136_group producing butyrate increased in the TAI + ZY-312 group compared to the TAI group, despite it did not show a statistically significant difference (Figures 4C,D). Furthermore, we analyzed the microbiota diversity (Shannon), and conducted principal component analysis (PCA), which reflects the difference in gut microbiota between and within groups. We found that the microbiota diversity between the TAI and TAI + ZY-312 groups did not change significantly (Figures 4E,F). And the BugBase results showed that the abundance of anaerobic microbiota was increased in the TAI and TAI + ZY-312 groups compared to the control group (Figure 4G). LEfSe and phylogenetic tree are analytical methods that could screen the statistical difference biomarker among different groups. The LEfSe results showed that the abundance of the Clostridiales_vadinBB60_group was increased in the TAI + ZY-312 group (Figures 4H,I). It indicated that B. fragilis participated in modulating intestinal microbiota, although it did not significantly alter the intestinal microbiota in microbiota abundance and diversity.
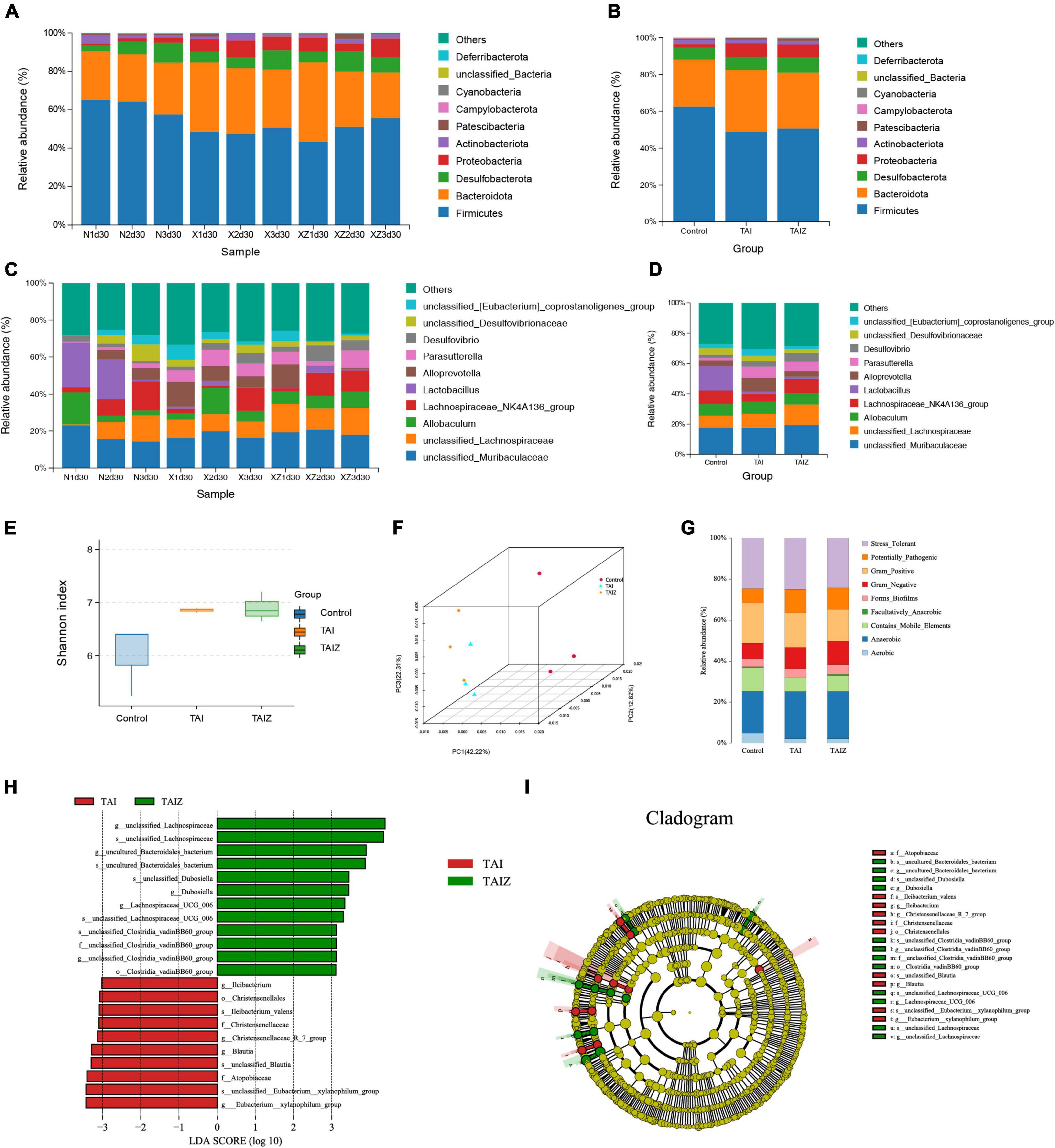
Figure 4. Bacteroides fragilis did not significantly alter intestinal microbiota to relieve radiation-induced intestinal injury in mice. (A,B) The phylum level of microbiota composition and comparison of microbiota in mice between samples and groups. (C,D) The genus level of microbiota composition and comparison of microbiota in mice between samples and groups. (E) The abundance and diversity of microbiota (Shannon index) in mice. (F) The principal coordinate analysis of microbiota. (G) The bug base analysis of microbiota. (H) The Linear discriminant analysis effect size (LEfSe) analysis of differential bacteria in slower intestinal (SI) meeting a significant linear discriminant analysis (LDA) threshold value of >3.5 between groups with different levels. (I) The cladogram based on LEfSe shows differential bacteria of the gut microbiota between groups with different levels. Nd30, control group; xd30 and TAI, TAI group; xzd30 and TAIZ, TAI + ZY-312 group.
Bacteroides fragilis upregulated STAT3 signaling pathway in intestinal epithelial cells
It has been reported that the short-chain fatty acids (SCFAs), including butyrate, can activate downstream JAK/STAT (28), p38 (29), NF-κB (30), PI3K/AKT (31), Wnt/β-catenin (32), and other signaling pathways to promote intestinal mucosa proliferation and repair. B. fragilis increased the abundance of the genera of the butyrate-producing Lachnospiraceae_NK4A136_group, which are beneficial for intestinal mucosa regeneration. Next, we attempted to explore the underlying mechanism of B. fragilis in the intestinal mucosa repair after radiation injury. Thus, we detected some classic proliferation pathways including signal transducer and activator of transcription (STAT3) (33), pmTOR, pNF-κB, and p38 signaling. The western blot results showed that B. fragilis activated STAT3 phosphorylation in the intestinal tissue, while B. fragilis did not affect the other classic proliferation pathways, such as pmTOR, pNF-κB, and p38 (Figure 5A). Meanwhile, we found that B. fragilis increased the expression of the proliferation index PCNA, goblet cell marker MUC2, stem cell indicator Lgr5, and tight junction protein claudin-1 (Figure 5A).
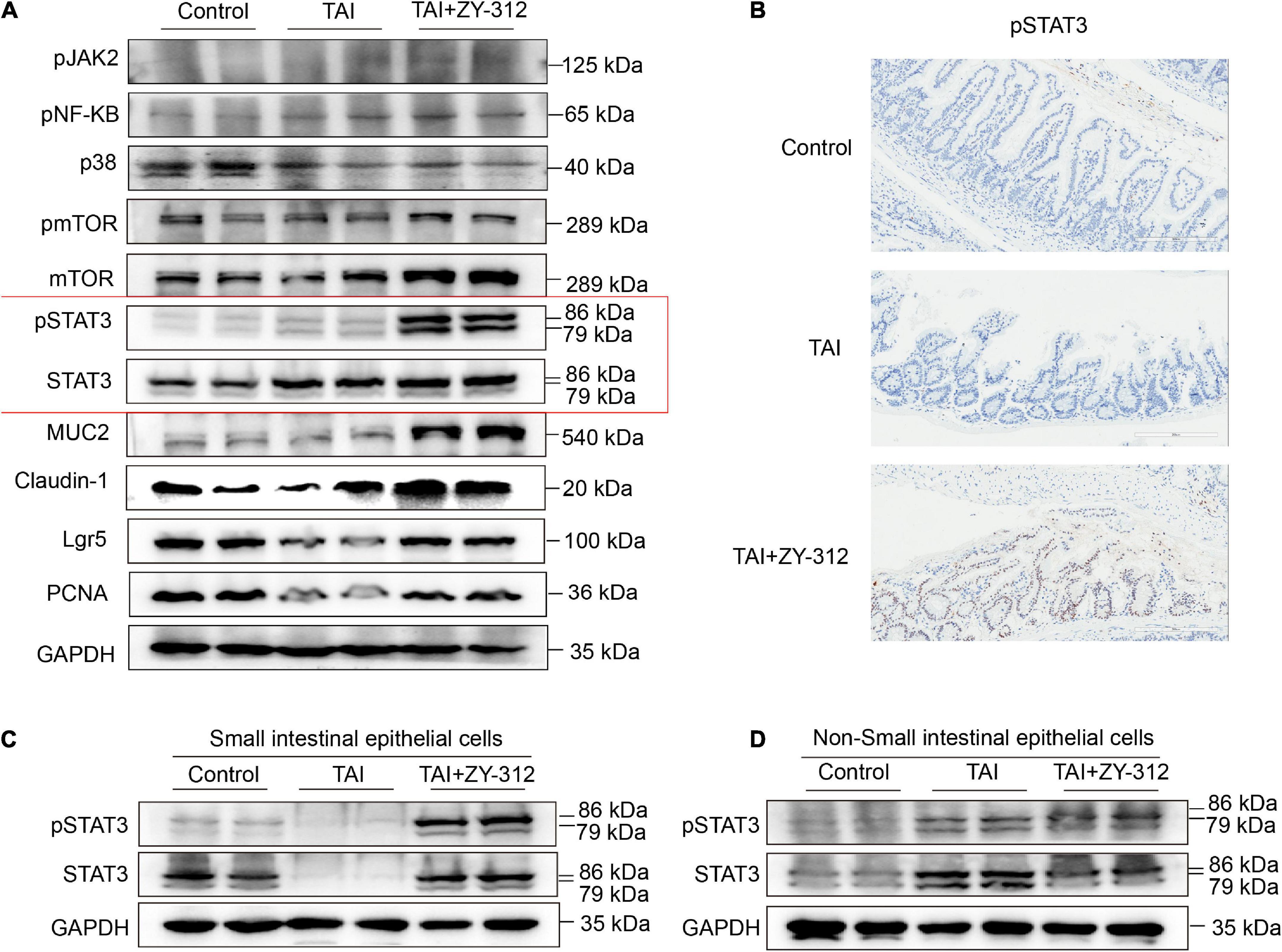
Figure 5. Bacteroides fragilis upregulated the signal transducer and activator of transcription 3 (STAT3) signaling pathway in intestinal epithelial cells. (A) The western blot analysis of pJAK2, pNF-KB, p38, pmTOR, mTOR, pSTAT3, STAT3, MUC2, Claudin-1, Lgr5, and PCNA in slower intestinal (SI) tissue between groups. GAPDH was regarded as an internal reference. (B) The immunohistochemical staining of pSTAT3 in SI between groups. (C) The western blot analysis of pSTAT3, STAT3 in SI intestinal epithelial cells (IECs). (D) The western blot analysis of pSTAT3, STAT3 in Non-SI epithelial cells.
The regulation of STAT3 is complex, as it is involved in signal transduction pathways in numerous cell types under various conditions. Pickert et al. (34) reported that interleukin (IL)-22 motivated STAT3 signaling pathway in IECs to repair mucosal wound in mice with experimental colitis. Notably, STAT3 phosphorylation in immune cells may exert different inflammation effects in colitis (35–37). We attempted to identify the specific layer in the small intestines where STAT3 phosphorylation is triggered after B. fragilis administration in radiation-induced intestinal injury. We found that B. fragilis mainly promoted STAT3 phosphorylation in the SI IECs but not in the non-epithelial layer (Figures 5B–D), suggesting that B. fragilis upregulated the STAT3 signaling pathway in SI IECs. It suggested that B. fragilis activated STAT3 phosphorylation with the upregulation with PCNA, MUC2, Lgr5, and claudin-1 in SI IECs.
Bacteroides fragilis promoted slower intestinal intestinal epithelial cell proliferation, stem cell regeneration, goblet cell secretion, and tight junction repair through STAT3 signaling pathway
To verify whether B. fragilis promoted SI IECs regeneration through the STAT3 signaling pathway, we constructed a radiation-induced intestinal injury model in Stat3△IEC (STAT3 defects in SI epithelium) mice and administered the mice B. fragilis orally (Supplementary Figure 1). Compared with the Stat3△IEC mice receiving B. fragilis (TAI + ZY-312/Stat3△IEC group), wild-type mice receiving B. fragilis (TAI + ZY-312/WT group) had significantly lower weight loss (Figure 6A), slower intestinal (SI and colon) length shortening (Figure 6B), attenuated SI epithelial cells damage (Figure 6C), reduced overall HAI (Figure 6D), and had longer lengths of SI crypts (Figure 6E). This suggested that B. fragilis relieved radiation-induced intestinal injury through the STAT3 signaling pathway in SI IECs.
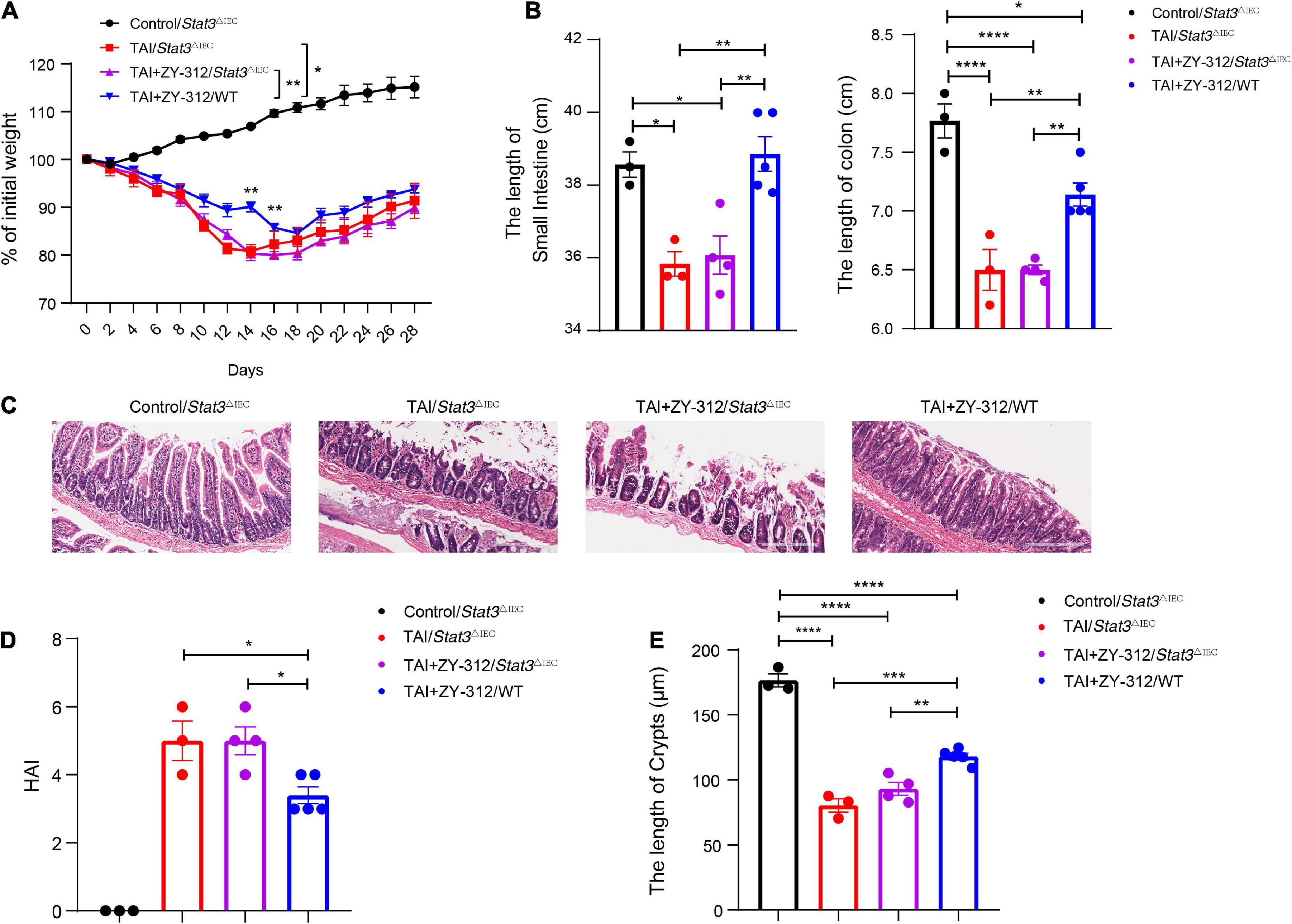
Figure 6. (A) The p-value between groups are following: percent (%) of weight loss p-value (TAI+ZY-312/Stat3△IEC vs. TAI+ZY-312 group/WTgroup), Day14 0.0059 and Day16 0.0037. (B) The p-value between groups are following: p-value the small intestine length the colon length, (Control/Stat3△IEC group vs. TAI/Stat3△IEC group) 0.0171 <0.0001, (Control/Stat3△IEC group vs. TAI+ZY-312/Stat3△IEC group) 0.02 <0.0001, (TAI/Stat3△IEC group vs. TAI+ZY-312/WT group) 0.0041 0.009, and (TAI+ZY-312/Stat3△IEC group vs. TAI+ZY-312/WT group) 0.0041 0.005. (C) The statistical analysis of colon length. (D) The p-value between groups are following: p-value HAI (TAI/Stat3△IEC group vs. TAI+ZY-312/WT group) 0.0372 and (TAI+ZY-312/Stat3△IEC group vs. TAI+ZY-312/WT group) 0.0232. (E) The p-value between groups are following: p-value SI Crypts (Control/Stat3△IEC group vs. TAI/Stat3△IEC group) <0.0001, (Control/Stat3△IEC group vs. TAI+ZY-312/Stat3△IEC group) <0.0001, (Control/Stat3△IEC group vs. TAI+ZY-312/WT group) <0.0001, (TAI/Stat3△IEC group vs. TAI+ZY-312/WT group) 0.0003, and (TAI+ZY-312/Stat3△IEC group vs. TAI+ZY-312/WT group) 0.0041. The length of SI crypts between groups. Control group/Stat3ΔIEC (N = 3), TAI/Stat3ΔIECgroup (N = 3), TAI + ZY-312/Stat3ΔIEC group (N = 4), TAI + ZY-312/WT group (N = 5). The data are presented as mean ± SEM, and *p < 0.05, **p < 0.01, **p < 0.001, **p <0.0001.
We found that SI IECs in Stat3△IEC mice after abdominal radiation injury had a very low expression of pSTAT3, while the SI IECs from wild-type mice receiving B. fragilis after abdominal radiation injury had a higher expression of pSTAT3 (Figure 7A). In this context, we discovered that mice receiving B. fragilis in WT mice (TAI + ZY-312/WT group) upregulated the expression of PCNA, MUC2, Lgr5, ZO-1, and claudin-1 (Figures 7A,B), while mice receiving B. fragilis in Stat3△IEC mice (TAI + ZY-312/Stat3△IEC group) showed little expression of the markers above (Figures 7A,B). This suggested that B. fragilis requires the STAT3 signaling pathway to promote SI IECs proliferation, stem cell regeneration, mucus secretion, and tight junction proteins expression.
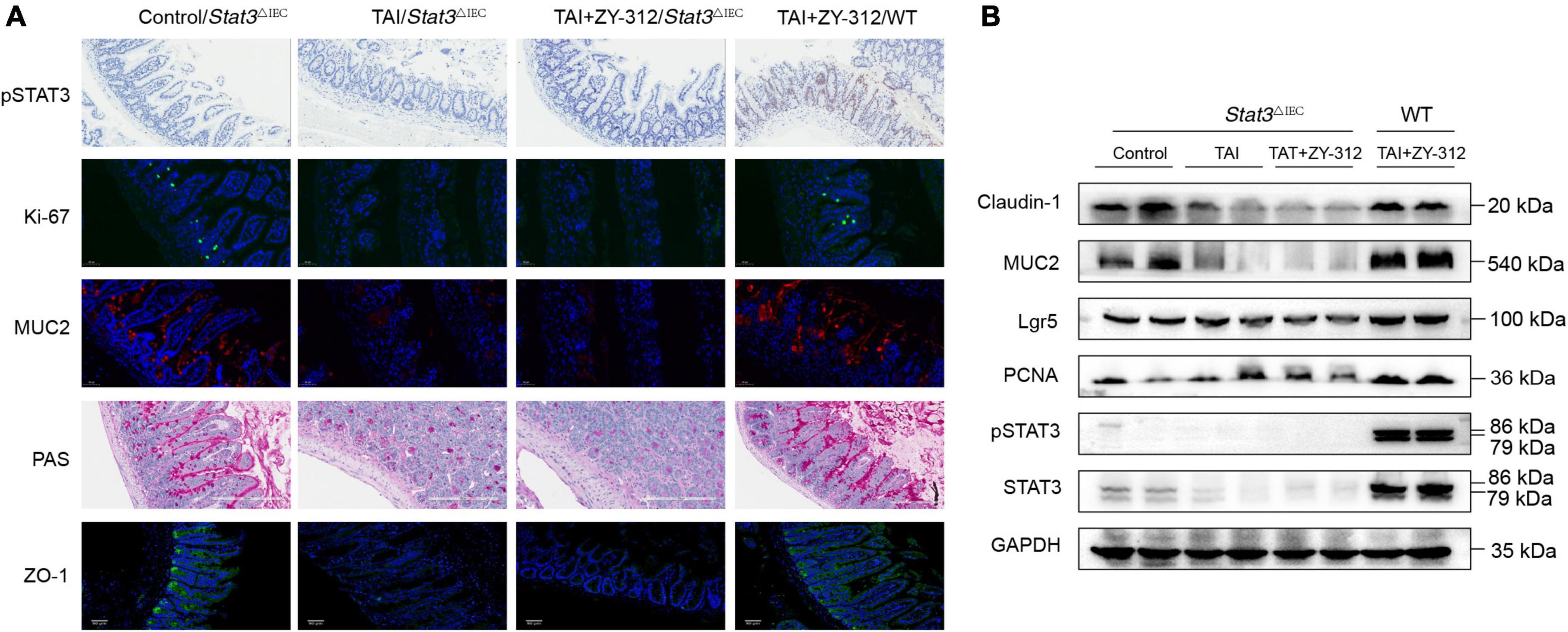
Figure 7. Bacteroides fragilis promoted intestinal epithelial cell proliferation, stem cell regeneration, goblet cell secretion, and tight junction repair through the signal transducer and activator of transcription 3 (STAT3) signaling pathway. (A) The histological staining of pSTAT3, Ki-67, MUC2, periodic acid-Schiff stain (PAS), and ZO-1 in SI IECs. (B) The western blot analysis of pSTAT3, STAT3, MUC2, Claudin-1, Lgr5, and PCNA in intestinal epithelial cell (SI) intestinal epithelial cell (IECs) between groups. GAPDH was regarded as an internal reference.
Overall, our results showed that B. fragilis promoted intestinal barrier integrity through the STAT3 signaling pathway in a mouse model of radiation-induced intestinal injury (Figure 8).
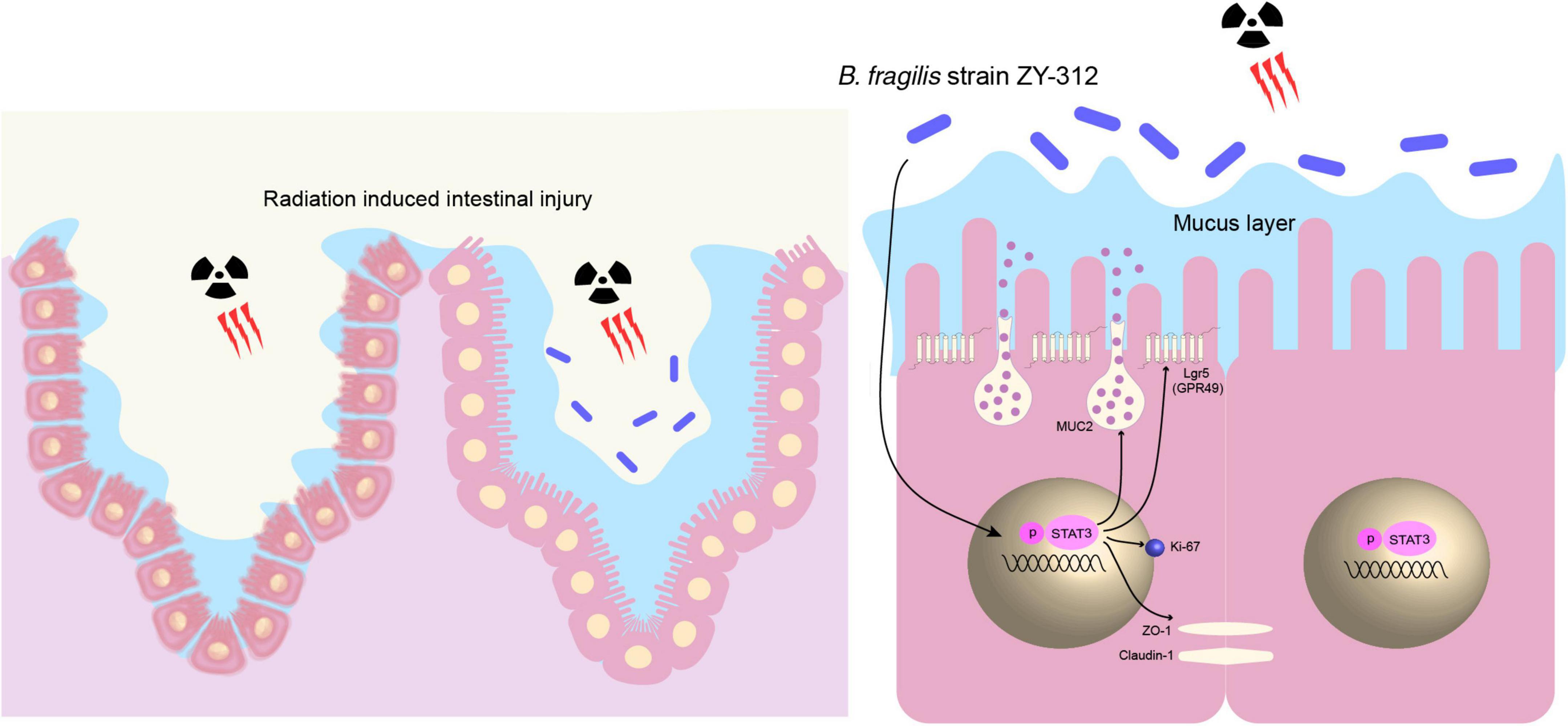
Figure 8. B. fragilis promoted intestinal barrier integrity through the STAT3 signaling pathway in a mouse model of radiation-induced intestinal injury. B. fragilis promoted SI IEC proliferation, stem cell regeneration, goblet cell secretion, and tight junction repair through the STAT3 signaling pathway hence relieving radiation-induced intestinal injury in mice.
Discussion
Gut microbiota dysbiosis plays a crucial role in the pathogenesis of radiation-induced intestinal injury. The abundance of most bacteria belonging to the phyla Actinobacteria and Proteobacteria has increased, while that of microorganisms from Firmicutes and Bacteroides has decreased (38, 39). This suggests that the replenishment of Firmicutes and Bacteroides play protective roles in restoring the gut microbiota structure and relieving radiation-induced intestinal injury. Cui et al. (40) reported that fecal microbiota transplantation could ameliorate radiation-induced toxicity and improve the prognosis of patients after radiotherapy. Furthermore, a randomized, double-blind, controlled trial showed that Lactobacillus acidophilus LAC-361 and Bifidobacterium longum BB-536 belonging to the phylum Firmicutes decreased diarrhea caused by radiation enteritis (41, 42). However, the effect and mechanism of the probiotic B. fragilis, belonging to the phylum Bacteroides, on radiation-induced intestinal injury remains unclear.
In this study, we found that B. fragilis promoted IEC repair to relieve radiation-induced intestinal injury in mice. It was similar to a study that reported that L. rhamnosus promoted IEC survival in the crypt region of mesenchymal stem cells and reduced epithelial cell apoptosis in radiation-induced intestinal injury (43). The intestinal barrier is the first defense line in the gastrointestinal tract, which consists of a mucus layer and a subjacent epithelium monolayer with tight junction proteins (44, 45). At the bottom of crypts, intestinal stem cells (ISCs) differentiate into goblet cells and promote epithelial regeneration against radiation-induced intestinal injury (46). It has been reported that the mucus secreted from goblet cells performs an important role in radiation-induced intestinal injury (47). While at the top of crypts, the most important intercellular tight junction is mainly composed of claudin and ZO-1, which exert a vital role in sustaining cell polarity and the intestinal epithelial barrier (48, 49). A clinical study reported that patients receiving radiotherapy had increased intestinal permeability and tight junction disruption (50). Besides, the following radiotherapy patients express limited amounts of tight junction proteins in the intestinal epithelium (51). It suggests that the level of stem cells, goblet cells, and tight junction may be potential markers of the degree of intestinal epithelium repair after abdominal radiation. In our study, we found that B. fragilis promoted IEC proliferation, stem cell regeneration, goblet cell secretion, and tight junction repair in radiation-induced intestinal injury. It was reported that a part of the symbiotic microbiota resides in the mucus layer to absorb the nourishment, and mucus has a scouring effect on pathogenic bacteria to relieve colitis (52). Based on the results that B. fragilis increased mucus expression in radiation-induced intestinal injury, it suggests that B. fragilis may have an influence on intestinal microbiota through mucus secretion. In this study, we analyzed the gut microbiota in mice through 16S rRNA gene sequencing. we found that B. fragilis strain ZY-312 did not lead to a significant increase in the abundance of phylum Firmicutes as well as the bacterial alpha and beta diversity. However, It is noteworthy that B. fragilis increased the general abundance of the Lachnospiraceae_NK4A136_group, which are butyrate-producing bacteria that are beneficial for intestinal mucosa protection, immune regulation, and inflammation inhibition (53). In addition, B. fragilis increased the abundance of the Clostridiales_vadinBB60_group in LEfSe analysis, which was related to the rise of vitamin D in the human body after ultraviolet b (UVB) radiation exposure (54). B. fragilis has no significant influence on the intestinal microbiota after radiation-induced intestinal injury in mice, it suggests that B. fragilis may promote intestinal epithelium repair through underlying molecular-mediated mechanisms. In the study, we found that B. fragilis motivated the STAT3 phosphorylation in SI IECs, it indicated that B. fragilis required the participation of the STAT3 signaling pathway to promote intestinal mucosa regeneration.
STAT3 plays an important role in the intracellular signal transduction pathway for cell proliferation and differentiation (55). The phosphorylation of STAT3 will translocate to the nucleus and activates downstream genes involved in proliferation and mucosa defense (56). It has been reported that the motivation of the STAT3 signaling pathway along the crypt-villus axis of the intestinal epithelium is mainly involved in the repair response to damage (57). And STAT3-disrupted intestinal organoids were unable to survive after suffering irradiation (58). It suggests that STAT3 is required for intestinal mucosa regeneration after radiation damage. STAT3 participates in intestinal epithelial cell regeneration including stem cells and goblet cell proliferation, and tight junction repair. STAT3 is an important regulator of intestinal epithelial stem cells (59). And probiotics have the capacity to motivate the STAT3 signaling to promote repair after damage. Yu et al. revealed that the probiotic Lactobacillus could activate STAT3 signaling to promote stem cell proliferation in DSS-induced colitis model mice (60). Another study reported that the upregulation of the STAT3 signaling pathway is related to mucus secretion in mice with experimental colitis (61). In addition, the activation of the STAT3 signaling pathway increased the expression of the tight junction protein ZO-1 and occludin (62). In our study, we found that B. fragilis promoted intestinal epithelial cell proliferation, stem cell regeneration, goblet cell secretion, and tight junction repair through the STAT3 signaling pathway. It is consistent with those studies that support the feasible relationship between the upstream STAT3 signaling pathway and the downstream activity of IECs including stem cells, goblet cells, and tight junctions.
In conclusion, our results suggested that the probiotic B. fragilis promoted SI IEC proliferation, stem cell regeneration, goblet cell secretion, and tight junction repair through the STAT3 signaling pathway in a mouse model of radiation-induced intestinal injury. In our next study, we will further explore the underlying mechanisms through which B. fragilis mediates intestinal metabolism and immunity. Our results may provide new insights for its application as a functional and clinical drug for radiation-induced intestinal injury after radiotherapy.
Conclusion
Bacteroides fragilis strain ZY-312 promoted small intestinal epithelial cell proliferation, stem cell regeneration, goblet cells secretion, and tight junction repair through the STAT3 signaling pathway in a mouse model of radiation-induced intestinal injury.
Data availability statement
The original contributions presented in this study are publicly available. The data presented in the study are deposited in the Figshare repository, accession number: doi: 10.6084/m9.figshare.21310536.
Ethics statement
This animal study was reviewed and approved by L2018053.
Author contributions
QZ was responsible for bacteria strain culture, animal experiment, data analysis, and manuscript writing. BS, RH, HL, WZ, MS, KL, XL, and SC were in charge of molecular detection. YL and YW provided the B. fragilis strain ZY-312, revised the manuscripts, and offered financial assistance. FZ was responsible for the project design and acquired funding. All authors contributed to the article and approved the submitted version.
Funding
This research work was supported by the National Natural Science Foundation of China (Project Nos. 81770530, 81970465, and 82172729), Guangdong Science and Technology Project (No. 2017B020209003), Natural Science Foundation of Guangdong Province (No. 2022A1515012649), Innovation Leader Team Program of Guangzhou (No. 201809010014), and R&D Plan for Key Areas in Guangdong Province (No. 2019B020204003).
Conflict of interest
Authors YL and YW were employed by Guangzhou Zhiyi Biotechnology Co., Ltd., and they provided fund assistance for our research project.
The remaining authors declare that the research was conducted in the absence of any commercial or financial relationships that could be construed as a potential conflict of interest.
Publisher’s note
All claims expressed in this article are solely those of the authors and do not necessarily represent those of their affiliated organizations, or those of the publisher, the editors and the reviewers. Any product that may be evaluated in this article, or claim that may be made by its manufacturer, is not guaranteed or endorsed by the publisher.
Supplementary material
The Supplementary Material for this article can be found online at: https://www.frontiersin.org/articles/10.3389/fnut.2022.1063699/full#supplementary-material
References
1. Hauer-Jensen, M, Denham JW, Andreyev HJ. Radiation enteropathy–pathogenesis, treatment and prevention. Nat Rev Gastroenterol Hepatol. (2014) 11:470–9. doi: 10.1038/nrgastro.2014.46
2. Citrin DE. Recent developments in radiotherapy. N Engl J Med. (2017) 377:2200–1. doi: 10.1056/NEJMc1713349
3. Andreyev J. Gastrointestinal symptoms after pelvic radiotherapy: a new understanding to improve management of symptomatic patients. Lancet Oncol. (2007) 8:1007–17. doi: 10.1016/S1470-2045(07)70341-8
4. Reis Ferreira M, Andreyev HJN, Mohammed K, Truelove L, Gowan SM, Li J, et al. Microbiota- and radiotherapy-induced gastrointestinal side-effects (MARS) study: A large pilot study of the microbiome in acute and late-radiation enteropathy. Clin Cancer Res. (2019) 25:6487–500. doi: 10.1158/1078-0432.CCR-19-0960
5. Chater C, Saudemont A, Zerbib P. Chronic radiation enteritis. J Visc Surg. (2019) 156:175–6. doi: 10.1016/j.jviscsurg.2018.09.002
6. Lalla RV, Bowen J, Barasch A, Elting L, Epstein J, Keefe DM, et al. MASCC/ISOO clinical practice guidelines for the management of mucositis secondary to cancer therapy. Cancer. (2014) 120:1453–61. doi: 10.1002/cncr.28592
7. Marin M, Gudiol C, Ardanuy C, Garcia-Vidal C, Calvo M, Arnan M, et al. Bloodstream infections in neutropenic patients with cancer: differences between patients with haematological malignancies and solid tumours. J Infect. (2014) 69:417–23. doi: 10.1016/j.jinf.2014.05.018
8. Tian H, Biehs B, Warming S, Leong KG, Rangell L, Klein OD, et al. A reserve stem cell population in small intestine renders Lgr5-positive cells dispensable. Nature. (2011) 478:255–9. doi: 10.1038/nature10408
9. Kumagai T, Rahman F, Smith AM. The microbiome and radiation induced-bowel injury: Evidence for potential mechanistic role in disease pathogenesis. Nutrients. (2018) 10:1405. doi: 10.3390/nu10101405
10. Hua G, Wang C, Pan Y, Zeng Z, Lee SG, Martin ML, et al. Distinct Levels of Radioresistance in Lgr5+ Colonic Epithelial Stem Cells versus Lgr5+ Small Intestinal Stem Cells. Cancer Res. (2017) 77:2124–33. doi: 10.1158/0008-5472.CAN-15-2870
11. Hua G, Thin TH, Feldman R, Haimovitz-Friedman A, Clevers H, Fuks Z, et al. Crypt base columnar stem cells in small intestines of mice are radioresistant. Gastroenterology. (2012) 143:1266–76. doi: 10.1053/j.gastro.2012.07.106
12. Zihni C, Mills C, Matter K, Balda MS. Tight junctions: from simple barriers to multifunctional molecular gates. Nat Rev Mol Cell Biol. (2016) 17:564–80. doi: 10.1038/nrm.2016.80
13. Touchefeu Y, Montassier E, Nieman K, Gastinne T, Potel G, Bruley des Varannes S, et al. Systematic review: the role of the gut microbiota in chemotherapy- or radiation-induced gastrointestinal mucositis - current evidence and potential clinical applications. Aliment Pharmacol Ther. (2014) 40:409–21. doi: 10.1111/apt.12878
14. Delia P, Sansotta G, Donato V, Messina G, Frosina P, Pergolizzi S, et al. Prevention of radiation-induced diarrhea with the use of VSL#3, a new high-potency probiotic preparation. Am J Gastroenterol. (2002) 97:2150–2. doi: 10.1111/j.1572-0241.2002.05946.x
15. Osterlund P, Ruotsalainen T, Korpela R, Saxelin M, Ollus A, Valta P, et al. Lactobacillus supplementation for diarrhoea related to chemotherapy of colorectal cancer: a randomised study. Br J Cancer. (2007) 97:1028–34. doi: 10.1038/sj.bjc.6603990
16. Liu Y, Zhang W, Bai Y, Zhi F. Isolation and identification of a non-enterotoxigenic strain of Bacteroides fragilis from a healthy term infant. Zhonghua Yi Xue Za Zhi. (2014) 94:2372–4.
17. O’Toole PW, Marchesi JR, Hill C. Next-generation probiotics: the spectrum from probiotics to live biotherapeutics. Nat Microbiol. (2017) 2:17057. doi: 10.1038/nmicrobiol.2017.57
18. Xu W, Su P, Zheng L, Fan H, Wang Y, Liu Y, et al. In vivo imaging of a novel strain of Bacteroides fragilis via metabolic labeling. Front Microbiol. (2018) 9:2298. doi: 10.3389/fmicb.2018.02298
19. Zhang W, Zhu B, Xu J, Liu Y, Qiu E, Li Z, et al. Bacteroides fragilis protects against antibiotic-associated diarrhea in rats by modulating intestinal defenses. Front Immunol. (2018) 9:1040. doi: 10.3389/fimmu.2018.01040
20. Fan H, Chen Z, Lin R, Liu Y, Wu X, Puthiyakunnon S, et al. Bacteroides fragilis Strain ZY-312 defense against cronobacter sakazakii-induced necrotizing enterocolitis in vitro and in a neonatal rat model. mSystems. (2019) 4:e305–19. doi: 10.1128/mSystems.00305-19
21. Deng H, Yang S, Zhang Y, Qian K, Zhang Z, Liu Y, et al. Bacteroides fragilis prevents clostridium difficile infection in a mouse model by restoring gut barrier and microbiome regulation. Front Microbiol. (2018) 9:2976. doi: 10.3389/fmicb.2018.02976
22. Riehl TE, Alvarado D, Ee X, Zuckerman A, Foster L, Kapoor V, et al. Lactobacillus rhamnosus GG protects the intestinal epithelium from radiation injury through release of lipoteichoic acid, macrophage activation and the migration of mesenchymal stem cells. Gut. (2019) 68:1003–13. doi: 10.1136/gutjnl-2018-316226
23. Sato T, Vries RG, Snippert HJ, van de Wetering M, Barker N, Stange DE, et al. Single Lgr5 stem cells build crypt-villus structures in vitro without a mesenchymal niche. Nature. (2009) 459:262–5. doi: 10.1038/nature07935
24. Zeineldin M, Neufeld K. Isolation of epithelial cells from mouse gastrointestinal tract for western blot or RNA analysis. Bio Protoc. (2012) 2:e292. doi: 10.21769/bioprotoc.292
25. Shukla PK, Gangwar R, Manda B, Meena AS, Yadav N, Szabo E, et al. Rapid disruption of intestinal epithelial tight junction and barrier dysfunction by ionizing radiation in mouse colon in vivo: protection by N-acetyl-l-cysteine. Am J Physiol Gastrointest Liver Physiol. (2016) 310:G705–15. doi: 10.1152/ajpgi.00314.2015
26. Johansson ME, Hansson GC. Immunological aspects of intestinal mucus and mucins. Nat Rev Immunol. (2016) 16:639–49. doi: 10.1038/nri.2016.88
27. Desai MS, Seekatz AM, Koropatkin NM, Kamada N, Hickey CA, Wolter M, et al. A dietary fiber-deprived gut microbiota degrades the colonic mucus barrier and enhances pathogen susceptibility. Cell. (2016) 167:1339–53. doi: 10.1016/j.cell.2016.10.043
28. Postler TS, Ghosh S. Understanding the holobiont: how microbial metabolites affect human health and shape the immune system. Cell Metab. (2017) 26:110–30. doi: 10.1016/j.cmet.2017.05.008
29. Feng Q, Chen WD, Wang YD. Gut microbiota: An integral moderator in health and disease. Front Microbiol. (2018) 9:151. doi: 10.3389/fmicb.2018.00151
30. Spiljar M, Merkler D, Trajkovski M. The immune system bridges the gut microbiota with systemic energy homeostasis: Focus on TLRs, Mucosal Barrier, and SCFAs. Front Immunol. (2017) 8:1353. doi: 10.3389/fimmu.2017.01353
31. Mármol I, Sánchez-de-Diego C, Pradilla Dieste A, Cerrada E, Rodriguez Yoldi MJ. Colorectal carcinoma: A general overview and future perspectives in colorectal cancer. Int J Mol Sci. (2017) 18:197. doi: 10.3390/ijms18010197
32. Lee YS, Kim TY, Kim Y, Lee SH, Kim S, Kang SW, et al. Microbiota-Derived lactate accelerates intestinal stem-cell-mediated epithelial development. Cell Host Microbe. (2018) 24:833–46. doi: 10.1016/j.chom.2018.11.002
33. Chen J, Zhao KN, Vitetta L. Effects of intestinal microbial–elaborated butyrate on oncogenic signaling pathways. Nutrients. (2019) 11:1026. doi: 10.3390/nu11051026
34. Pickert G, Neufert C, Leppkes M, Zheng Y, Wittkopf N, Warntjen M, et al. STAT3 links IL-22 signaling in intestinal epithelial cells to mucosal wound healing. J Exp Med. (2009) 206:1465–72. doi: 10.1084/jem.20082683
35. Zhang M, Zhou L, Xu Y, Yang M, Xu Y, Komaniecki GP, et al. A STAT3 palmitoylation cycle promotes TH17 differentiation and colitis. Nature. (2020) 586:434–9. doi: 10.1038/s41586-020-2799-2
36. Oweida AJ, Darragh L, Phan A, Binder D, Bhatia S, Mueller A, et al. STAT3 modulation of regulatory T cells in response to radiation therapy in head and neck cancer. J Natl Cancer Inst. (2019) 111:1339–49. doi: 10.1093/jnci/djz036
37. Zou S, Tong Q, Liu B, Huang W, Tian Y, Fu X. Targeting STAT3 in Cancer Immunotherapy. Mol Cancer. (2020) 19:145. doi: 10.1186/s12943-020-01258-7
38. Wang Z, Wang Q, Wang X, Zhu L, Chen J, Zhang B, et al. Gut microbial dysbiosis is associated with development and progression of radiation enteritis during pelvic radiotherapy. J Cell Mol Med. (2019) 23:3747–56. doi: 10.1111/jcmm.14289
39. Jian Y, Zhang D, Liu M, Wang Y, Xu ZX. The impact of gut microbiota on radiation-induced enteritis. Front Cell Infect Microbiol. (2021) 11:586392. doi: 10.3389/fcimb.2021.586392
40. Cui M, Xiao H, Li Y, Zhou L, Zhao S, Luo D, et al. Faecal microbiota transplantation protects against radiation-induced toxicity. EMBO Mol Med. (2017) 9:448–61. doi: 10.15252/emmm.201606932
41. Cui M, Xiao H, Li Y, Zhang S, Dong J, Wang B, et al. Sexual dimorphism of gut microbiota dictates therapeutics efficacy of radiation injuries. Adv Sci (Weinh). (2019) 6:1901048. doi: 10.1002/advs.201901048
42. Demers M, Dagnault A, Desjardins J. A randomized double-blind controlled trial: impact of probiotics on diarrhea in patients treated with pelvic radiation. Clin Nutr. (2014) 33:761–7. doi: 10.1016/j.clnu.2013.10.0150
43. Ciorba MA, Riehl TE, Rao MS, Moon C, Ee X, Nava GM, et al. Lactobacillus probiotic protects intestinal epithelium from radiation injury in a TLR-2/cyclo-oxygenase-2-dependent manner. Gut. (2012) 61:829–38. doi: 10.1136/gutjnl-2011-300367
44. Paone P, Cani PD. Mucus barrier, mucins and gut microbiota: the expected slimy partners? Gut. (2020) 69:2232–43. doi: 10.1136/gutjnl-2020-322260
45. Otani T, Furuse M. Tight junction structure and function revisited. Trends Cell Biol. (2020) 30:805–17. doi: 10.1016/j.tcb.2020.08.004
46. Gehart H, Clevers H. Tales from the crypt: new insights into intestinal stem cells. Nat Rev Gastroenterol Hepatol. (2019) 16:19–34. doi: 10.1038/s41575-018-0081-y
47. Jang H, Kim S, Kim H, Oh SH, Kwak SY, Joo HW, et al. Metformin protects the intestinal barrier by activating goblet cell maturation and epithelial proliferation in radiation-induced enteropathy. Int J Mol Sci. (2022) 23:5929. doi: 10.3390/ijms23115929
48. Bazzoni G, Martinez-Estrada OM, Orsenigo F, Cordenonsi M, Citi S, Dejana E. Interaction of junctional adhesion molecule with the tight junction components ZO-1, cingulin, and occludin. J Biol Chem. (2000) 275:20520–6. doi: 10.1074/jbc.M905251199
49. Lerner A, Matthias T. Changes in intestinal tight junction permeability associated with industrial food additives explain the rising incidence of autoimmune disease. Autoimmun Rev. (2015) 14:479–89. doi: 10.1016/j.autrev.2015.01.009
50. Nejdfors P, Ekelund M, Weström BR, Willén R, Jeppsson B. Intestinal permeability in humans is increased after radiation therapy. Dis Colon Rectum. (2000) 43:1582–8. doi: 10.1007/BF02236743
51. Ulluwishewa D, Anderson RC, McNabb WC, Moughan PJ, Wells JM, Roy NC. Regulation of tight junction permeability by intestinal bacteria and dietary components. J Nutr. (2011) 141:769–76. doi: 10.3945/jn.110.135657
52. Johansson ME, Sjövall H, Hansson GC. The gastrointestinal mucus system in health and disease. Nat Rev Gastroenterol Hepatol. (2013) 10:352–61. doi: 10.1038/nrgastro.2013.35
53. Ma L, Ni Y, Wang Z, Tu W, Ni L, Zhuge F, et al. Spermidine improves gut barrier integrity and gut microbiota function in diet-induced obese mice. Gut Microbes. (2020) 12:1–19. doi: 10.1080/19490976.2020.1832857
54. Bosman ES, Albert AY, Lui H, Dutz JP, Vallance BA. Skin exposure to narrow band ultraviolet (UVB) light modulates the human intestinal microbiome. Front Microbiol. (2019) 10:2410. doi: 10.3389/fmicb.2019.02410
55. Verhoeven Y, Tilborghs S, Jacobs J, De Waele J, Quatannens D, Deben C, et al. The potential and controversy of targeting STAT family members in cancer. Semin Cancer Biol. (2020) 60:41–56. doi: 10.1016/j.semcancer.2019.10.002
56. Ernst M, Thiem S, Nguyen PM, Eissmann M, Putoczki TL. Epithelial gp130/Stat3 functions: an intestinal signaling node in health and disease. Semin Immunol. (2014) 26:29–37. doi: 10.1016/j.smim.2013.12.006
57. Grivennikov S, Karin E, Terzic J, Mucida D, Yu GY, Vallabhapurapu S, et al. IL-6 and Stat3 are required for survival of intestinal epithelial cells and development of colitis-associated cancer. Cancer Cell. (2009) 15:103–13. doi: 10.1016/j.ccr.2009.01.001
58. Oshima H, Kok SY, Nakayama M, Murakami K, Voon DC, Kimura T, et al. Stat3 is indispensable for damage-induced crypt regeneration but not for Wnt-driven intestinal tumorigenesis. FASEB J. (2019) 33:1873–86. doi: 10.1096/fj.201801176R
59. Galoczova M, Coates P, Vojtesek B. STAT3, stem cells, cancer stem cells and p63. Cell Mol Biol Lett. (2018) 23:12. doi: 10.1186/s11658-018-0078-0
60. Hou Q, Ye L, Liu H, Huang L, Yang Q, Turner JR, et al. Lactobacillus accelerates ISCs regeneration to protect the integrity of intestinal mucosa through activation of STAT3 signaling pathway induced by LPLs secretion of IL-22. Cell Death Differ. (2018) 25:1657–70. doi: 10.1038/s41418-018-0070-2
61. Mizoguchi A, Yano A, Himuro H, Ezaki Y, Sadanaga T, Mizoguchi E. Clinical importance of IL-22 cascade in IBD. J Gastroenterol. (2018) 53:465–74. doi: 10.1007/s00535-017-1401-7
Keywords: Bacteroides fragilis strain ZY-312, probiotics, radiation-induced intestinal injury, stem cells, goblet cells, tight junction, STAT3 signaling pathway
Citation: Zhou Q, Shen B, Huang R, Liu H, Zhang W, Song M, Liu K, Lin X, Chen S, Liu Y, Wang Y and Zhi F (2022) Bacteroides fragilis strain ZY-312 promotes intestinal barrier integrity via upregulating the STAT3 pathway in a radiation-induced intestinal injury mouse model. Front. Nutr. 9:1063699. doi: 10.3389/fnut.2022.1063699
Received: 07 October 2022; Accepted: 14 November 2022;
Published: 15 December 2022.
Edited by:
Hengyi Xu, Nanchang University, ChinaReviewed by:
Xiaoxi Liu, Guangdong Ocean University, ChinaShuangshuang Guo, Wuhan Polytechnic University, China
Copyright © 2022 Zhou, Shen, Huang, Liu, Zhang, Song, Liu, Lin, Chen, Liu, Wang and Zhi. This is an open-access article distributed under the terms of the Creative Commons Attribution License (CC BY). The use, distribution or reproduction in other forums is permitted, provided the original author(s) and the copyright owner(s) are credited and that the original publication in this journal is cited, in accordance with accepted academic practice. No use, distribution or reproduction is permitted which does not comply with these terms.
*Correspondence: Fachao Zhi, emhpZmM0MTUzMkAxNjMuY29t; orcid.org/0000-0001-8674-4737