- 1Preclinical Department, Faculty of Medicine and Defence Health, Universiti Pertahanan Nasional Malaysia, Kuala Lumpur, Malaysia
- 2Department of Nursing, Faculty of Medicine, Universiti Kebangsaan Malaysia, Kuala Lumpur, Malaysia
- 3Department of Physiology, Faculty of Medicine, Universiti Kebangsaan Malaysia, Kuala Lumpur, Malaysia
- 4Centre of Shariah, Faculty of Islamic Studies, Universiti Kebangsaan Malaysia, Bangi, Selangor, Malaysia
- 5Insitute of Islam Hadhari, Universiti Kebangsaan Malaysia, Bangi, Selangor, Malaysia
- 6Bioaromatic Research Centre, Universiti Malaysia Pahang, Gambang, Pahang, Malaysia
- 7Faculty of Forestry and Environment, Universiti Putra Malaysia, Serdang, Selangor, Malaysia
This review aims to gather and summarize up-to-date information on the potential health benefits of Nigella sativa (NS) on diabetes mellitus (DM) and its complications from different animal models, clinical trials and in vitro studies. DM is one of the most prevalent metabolic disorders resulting from chronic hyperglycaemia due to problems in insulin secretion, insulin action or both. It affects people regardless of age, gender and race. The main consequence of DM development is the metabolic dysregulation of glucose homeostasis. Current treatments for DM include pharmacological therapy, insulin and diabetic therapy targeting β cells. Some of these therapeutic approaches are promising; however, their safety and effectiveness remain elusive. Since ancient times, medicinal plants have been used and proven effective against diseases. These plants are believed to be effective and benefit physiological and pathological processes, as they can be used to prevent, reduce or treat multiple diseases. Nigella sativa Linn. is an annual indigenous herbaceous plant belonging to Ranunculaceae, the buttercup family. NS exhibits multifactorial activities; it could ameliorate oxidative, inflammatory, apoptotic and insulinotropic effects and inhibit carbohydrate digestive enzymes. Thus, this review demonstrates the therapeutic potential of NS that could be used as a complement or adjuvant for the management of DM and its complications. However, future research should be able to replicate and fill in the gaps of the study conducted to introduce NS safely to patients with DM.
Introduction
Diabetes mellitus (DM) is one of the most prevalent metabolic disorders resulting from chronic hyperglycaemia due to problems in insulin secretion, insulin action, or both (1, 2). It affects people regardless of age, gender and race. The main consequence of DM development and the considerable morbidity and mortality it causes is the metabolic dysregulation of glucose homeostasis (3). The following classical classifications of DM were proposed by the American Diabetes Association in 1997: type 1 diabetes mellitus (T1DM), type 2 diabetes mellitus (T2DM), gestational DM, and specific types of diabetes due to other causes (4). T1DM and T2DM are the most common types of DM (2). Approximately 90–95% of those with DM develop T2DM (4). Global prevalence data from the International Diabetes Federation Atlas 2013 showed that the prevalence of DM in adults aged 20–79 years is 8.3% (382 million) of the worldwide population (2).
T2DM is characterized predominantly by insulin resistance with relative insulin deficiency, which increases the insulin demand of insulin-target tissues (4–6). Insulin levels may appear normal or elevated with normal β cell function, but their secretion is defective and insufficient to compensate for insulin resistance (5). People with T2DM are usually obese, and obesity is able to cause some degree of insulin resistance (5, 6). Additionally, those with an increased percentage of body fat distributed in the abdominal region are also affected by T2DM (5, 6). Nevertheless, diabetes-related health conditions can be prevented with pharmacological treatment and insulin. For example, metformin remains essential in treating T2DM, particularly for obese and overweight people (7). Metformin is necessary, as it increases insulin sensitivity and suppresses hepatic gluconeogenesis (8). Other therapeutic approaches include gene therapy, diabetic therapy targeting β cells and β cell regeneration, stem cells and enhancing the self-replication of β cells. Some of these therapeutic approaches are promising; however, their safety and effectiveness remain elusive (7).
Since ancient times, medicinal plants have been used and proven effective against diseases. These plants are believed to be effective and benefit physiological and pathological processes, as they can prevent, reduce or treat multiple diseases (9). Nigella sativa Linn. (NS; Figure 1) is an annual indigenous herbaceous plant that belongs to the buttercup family Ranunculaceae, a family of flowering plants, and the genus Nigella with 14 species, including N. arvensis, N. ciliaris, N. damascene, N. hispanica, N. integrifolia, N. nigellastrum, N. orientalis, and NS (10). Previously, NS is used only as a spice and in traditional medicine. However, nowadays, it is used extensively in the pharmaceutical and food industries (11).
NS constitutes a broad spectrum of therapeutic activities, including antidiabetic, diuretic, antihypertensive, anticancer, immunomodulatory, analgesic, antimicrobial, anthelmintic, anti-inflammatory, spasmolytic, bronchodilatory, gastroprotective, hepatoprotective, renal protective, antifungal, and antioxidant properties; increases maternal lactation and fertility and boosts the immune system (12, 13). NS's antidiabetic effects could improve clinical and glycaemic control in DM (14). In addition, numerous clinical studies have demonstrated the efficacy of NS on DM (15).
Several reviews have been conducted pertaining to the broad pharmacological effects of NS. However, very little literature has been done specifically on DM and its complications.
Thus, this review aims to gather and summarize up-to-date information on the potential health benefits of NS on DM and its complications from different animal models, clinical trials and in vitro studies. We also highlight the utilization of NS as the precursor to synthesize nanoparticles, which are beneficial in managing DM. Besides that, this review also specifies the different DM animal models and study designs, as well as the type of NS used in the study to perceive how NS influences the outcome in DM management. The findings could help provide a basis for developing new complementary antidiabetic medications with fewer side effects and excellent efficacy.
Methods
A literature search was performed to identify and map out relevant articles related to the effects of NS on DM. Peer-reviewed, full-text English articles up to May 2022 were collected from electronic databases, including Scopus, MEDLINE via EBSCOhost and Google Scholar. The following set of keywords was used: (1) “Nigella sativa” or “Nigella sativa oil” or “Nigella sativa extracts” and (2) “diabetes mellitus.” The literature search was further supplemented by referencing related review articles and scientific reports found in the search results. All available in vitro, in vivo and human studies that reported the effects of NS or NS oil or NS seed on DM were included in this review. Meanwhile, studies on the effects of an individual bioactive component of NS, the mixture of NS with other herbs and studies on gestational diabetes (GDM) were excluded.
Physicochemical and phytochemical contents of NS
NS is composed of remarkable levels of iron, copper, zinc, phosphorus, calcium, thiamin, niacin, pyridoxine, and folic acid (12, 16, 17). Additionally, it has a maximal nutritional value, including substantial amounts of vegetable protein (20–85%), fiber (7–94%), fat (38.02%), and carbohydrate (31.94%) (18). NS also has various amino acid components, including glutamate, arginine and aspartate, as well as cysteine and methionine (18). In addition, NS seeds contain 26–34% fixed oil from major fatty acids, such as linoleic acid (64.6%) and palmitic acid (20.4%) (17). Furthermore, NS seed oil comprises 0.4–2.5% essential oil (19).
Various factors can affect NS's phytochemical content, including the growing areas, maturation stage, processing procedures and isolation techniques (20). Using different extraction methods or solvents, some researchers also found different compounds that other studies have not report. For instance, in oil samples extracted with a cold press, hexane, tetrahydrofuran, ethanol, dichloromethane, and methanol produced various amounts of thymoquinone (21). Many studies have been conducted to determine the bioactive components of NS. Table 1 summarizes the various phytochemical compounds of NS that have been identified from different origins.
Thymoquinone, thymohydroquinone, dithymoquinone, p-cymene, carvacrol, terpinen-4-ol, t-anethol, sesquiterpene longifolene, α-pinene, and thymol are the major phytochemical compounds found in NS (Figure 2) (12). Thymoquinone is the major active principle of NS oil and exhibits antidiabetic and antitumour activity against breast, lung, prostate, liver, colon and pancreatic cancers (31). Findings from previous studies revealed that thymoquinone accounts for 38–40% of total NS bioactive components (32). On the other hand, Singh et al. (33) reported p-cymene as the major component with 36.2% in the NS essential oil and linoleic acid as the major component with 53.60% using acetone extract. Besides these compounds, other compounds are found in trace amounts, such as camphene, sabinene, phenol, 2-methyl-5-(1-methyl ethyl), α-terpinene, β-terpinene, γ-terpinene, limonene, and many others.
Effect of NS on DM
One of the most explored properties of NS is its antidiabetic potential. Studies involving different animal models, clinical trials, and in vitro studies have been conducted widely. This study reviewed 50 studies, including 31 animal studies, six in vitro studies, and 13 clinical trials. Both NS oil and extracts possess antidiabetic properties, as summarized in Table 2, and their proposed mechanism is illustrated in Figure 3.
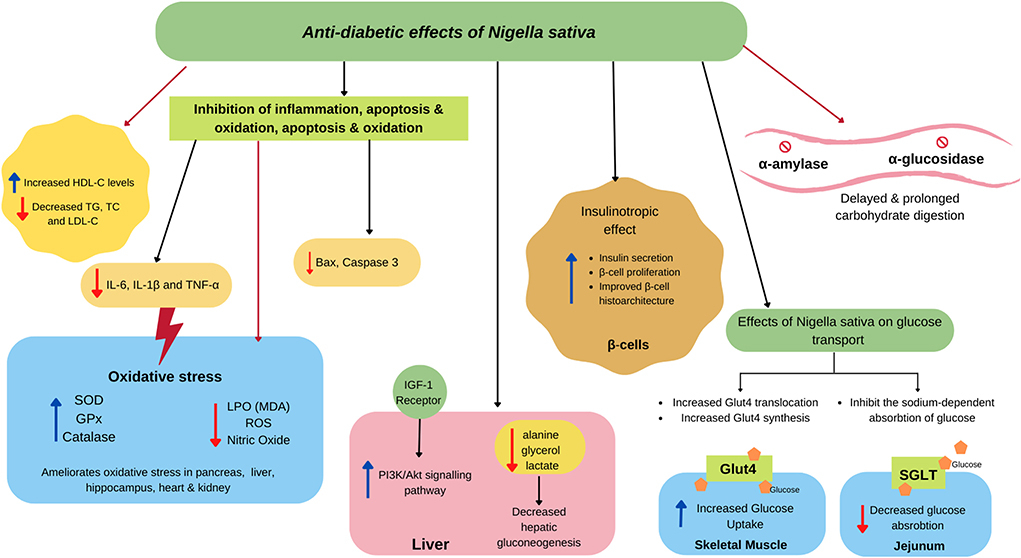
Figure 3. Proposed antidiabetic effects of NS. NS attenuates insulin secretion by ameliorating β cell proliferation and improves the structure of the islet of the pancreas. In the small intestine, NS agents delay the digestion and absorption of food because of the inhibitory activity of α-amylase and α-glucosidase. NS also modulate SGLT causing less glucose absorption in the jejunum epithelium and activating GLUT4, which is associated with increased glucose absorption in skeletal muscle. NS also alleviates insulin resistance by activating the PI3K/Akt signaling pathway, which promotes GLUT4 biogenesis and translocation. NS also decreases hepatic gluconeogenesis by reducing its precursors. Besides, NS exerts a potential in scavenging , HO·, DPPH, and other free radicals to lighten oxidative stress, resulting in the less production of malondialdehyde (MDA) and nitric oxide. NS also increase the contents and activity of antioxidant enzymes GSHPx, SOD, and CAT. NS improves dyslipidaemia, which could reduce inflammatory markers, such as IL-6, IL-1β, and TNF-α, along with the reduction of apoptosis markers, such as Bax and caspase-3.
In vitro and in vivo studies
DM is a complex multifactorial metabolic disorder characterized by hyperglycaemia and lack of insulin release or insulin resistance. The pathophysiology of DM is closely associated with impaired redox homeostasis, activation of the inflammatory signaling cascade, and dyslipidaemia. Insulin, the key regulator hormone released by the β cell of the pancreas, is essential in maintaining glucose homeostasis. Hence, restoring insulin release and its sensitivity is warranted as one of the therapeutic strategies in managing DM.
Reports from recent studies have shown NS manage to alleviate the plasma glucose level due to its insulinotropic effects. In these studies, the proposed mechanisms for this effect are through the modulation of insulin receptor genes, increasing the insulin/insulin receptor ratio (42), enhancing the brain's insulin signaling pathway (41) and increased in insulin release from the increment of β cell activity (38).
The potential effect of NS in promoting insulin release has been studied extensively in T2DM animal models using a combination of streptozotocin (STZ) and nicotinamide or a combination of low-dose STZ with a high-fat diet (HFD). Rchid et al. further validated these findings in their in vitro study. They found that NS can stimulate glucose-induced insulin release, which is the highest in the basic fraction compared to the acidic and neutral fractions (66). The basic fraction of NS contains amino acids and some basic compounds, which could contribute to this particular effect of NS. This result signifies that the hypoglycaemic effect of NS is partially mediated by the potentiation of insulin release by the β cell.
Interestingly, El-Dakhakhny et al. reported that the hypoglycaemic effect of NS is not parallel to the stimulation of insulin release. In this study, they found that NS oil and its bioactive compounds, nigellone and thymoquinone, could stimulate insulin secretion in isolated rat pancreatic islets in the presence of glucose. However, as the insulin release does not reflect the blood glucose level, they postulate that NS possesses an extrapancreatic role in causing the hypoglycaemic effect. However, in this study, they used a much higher STZ (75 mg/kg) dose to induce the experimental animal, signifying more extensive damage to the β cell, which mimics T1DM rather than T2DM, as reported in the previous study (40).
Pancreatic tissue are vulnerable to diabetes-induced oxidative stress due to low expression of antioxidant enzyme and high production of endogenous ROS (70). This tissue damage could further impair the insulin released, deteriorating the diabetes condition. The effect of NS ameliorating insulin release from the β cell was further supported by the improvement in the β cell histoarchitecture findings. Studies revealed that administration of NS causes an increase in insulin immunoreactive percentage and mean pancreatic islet diameter, the ability to preserve the pancreatic β cell integrity, and increased area of insulin immunoreactive β cells in diabetic rats (43, 53). Additionally, Kanter et al. reported that treatment with 0.2 mL NS volatile oil for 4 weeks could lead to partial regeneration or proliferation of the pancreatic β cells in STZ-induced female diabetic rats (45). These improvements in β cell structure and functions could restore insulin release, which helps in overall blood glucose control in both T1DM and T2DM.
The hypoglycaemic effect seen in NS could also be attributed to the role of glucose transport. In a study by Meddah et al., 2 g/kg/day NS extract caused a reduction in glucose reabsorption from the intestine by modulating SGLT1, the primary glucose transporter in the intestine (60). The inhibition of SGLT1 not only improves glucose absorption but can also induce the release of incretin, such as glucagon-like peptide (GLP-1), which augments glucose-induced insulin secretion and prevents the release of glucagon (71). Thus, by acting on SGLT1, NS could help maintain blood glucose homeostasis. Normally, insulin facilitates glucose uptake in skeletal muscle and adipocytes through GLUT4 activity. The translocation of the GLUT4 to the cell surface helps in glucose transport into the cell, which reflects insulin sensitivity (72). In diabetes, the normal harmony between GLUT4 and insulin activity is disrupted. The prolonged hyperinsulinemia and hyperglycaemia worsen insulin resistance, which causes the glucose to be unable to enter the cell. Administration of 48 mg/kg/day of NS ethanolic extract in STZ-induced diabetes rats for 4 weeks shows upregulation in GLUT4 activity and synthesis in the skeletal muscle. This finding corresponds to the other parameter, such as improvement in blood glucose level, insulin and glucose tolerance following NS treatment.
Hypolipidemic effects
In diabetes, hyperglycaemia cause reduction in lipoprotein lipase activity which leads to hyperlipidaemia (73). Diabetic dyslipidaemia, characterized by increased fasting and post-prandial blood glucose, low-density lipoprotein (LDL-C), and decreased high-density lipoprotein (HDL-C), represents the major link with increased cardiovascular risk of diabetic patients (74). Fat accumulation has also been linked to systemic oxidative stress in animal and human studies due to the generation of inflammation and oxidative stress. NS has been shown to cause improvement in dyslipidaemia and high blood glucose levels in various diabetes studies. A study by Bensiameur-Touati et al. reported that the supplementation of 0.2 g/kg NS improved dyslipidaemia in diabetic rats by increasing the HDL-C and decreasing the triglycerides (TGs), cholesterol and low-density lipoprotein (LDL-C) (48). This finding is supported by Mohebbati et al. as they found administration of NS hydroalcoholic extracts for 6 weeks decreased TC, TGs, and VLDL-C (50). The essential fatty acid content in the NS itself could be among the factors that augment the improvement of dyslipidemia and its complications (75). Interestingly, utilization of NS seed polysaccharide also revealed similar improvement in lipid profile following treatment for 4 weeks in the T2DM rat model (54).
Antioxidants effects
Hyperglycaemia control remains one of the focal therapeutic strategies in managing DM. Uncontrol chronic hyperglycaemia triggers the overactivity of the NADPH oxidase enzyme, increases adipocyte expansion and mitochondrial production of the reactive oxygen species (ROS). When the production of ROS supersedes the antioxidant activity, it will result in the formation of oxidative stress (76).
A plethora of studies have proven NS's pharmacological potential is mainly due to its antioxidant property. As a potential source of antioxidants, NS is shown to cause a reduction in ROS formation and lipid peroxidation (LPO), evidenced by a decrease in its by-product, malondialdehyde (MDA). NS also upregulates the activity of the antioxidant enzymes such as catalase (CAT), superoxide dismutase (SOD), glutathione peroxidase (GPx), or molecules such as glutathione (GSH) in both in vitro and in vivo studies, which include both serum and the target tissue.
Seflek et al. reported treatment with 0.2 mg NS oil daily for 30 days caused a significant reduction in total oxidant status (TOS) and MDA and an increase in the antioxidant enzyme, SOD, along with total antioxidant status (TAS) in STZ-induced rats (34). Similar findings have been reported in a study on diabetic myopathy. They found that administration of 1 mL/kg NS oil restores the antioxidant enzyme such as CAT, GSH, GST, and cardiac enzyme CK-MB and reduces the MDA level, which signifies the improvement of the oxidant balance (37). Balbaa et al. further supported this finding in their study using the T2DM animal model, which they reported a significant reduction in brain oxidative stress evidenced by a decrease in TBARS whilst increasing the GSH, GPx, GST, and SOD (41). They also found the NS administration for 21 days able to deplete one of the crucial enzymes in generating oxidative stress, xanthine oxidase (XO). XO utilized xanthine and hypoxanthine as reducing substrates and causes electron reduction of an oxygen molecule to yield ROS such as superoxide and hydrogen peroxidase (77). Thus, ROS generation can be controlled and minimized by lowering the XO activity. Another critical finding in this study is the NS potential in reducing the advanced glycation end products (AGE). AGE formed by the non-enzymatic reaction secondary to chronic hyperglycaemia propagates the oxidative stress reaction by activation of NADPH oxidase enzyme and increases the drive of mitochondrial ROS production (78). Similarly, Abbasnezhad et al. also reported a reduction in AGE following treatment for 6 weeks in STZ-induced rats (46).
In a more recent study, Alkhalaf et al. utilized the NS extract to synthesize the silver nanoparticles (AgNPs) and reported their antioxidant potential in reducing of both AGE and MDA (55). The study that ran for 21 days in STZ-induced rats also reported an increase in the antioxidant enzymes, SOD and GSH along with an increase in nitrotyrosine. The liver and kidney also benefit from the antioxidant effects of the NS as they cause an increase in TBARS, hydroperoxides, CAT and SOD levels and reduced glutathione and glutathione peroxidase following treatment with 300 mg/kg/day ethanol extract of NS for 4 weeks (61). A similar antioxidant potential is reported in the NS gold nanoparticle, which increased the antioxidant activity in the DPPH assay (27).
A comparison study between NS oil and the NS aqueous on the pancreas reported that both NS preparation protects against oxidative stress by decreasing COX-2, LPO, and MDA and increasing the antioxidant enzyme SOD, hence, providing pancreatic-protective effects. However, treatment with NS oil could not ameliorate the serum glucose level to normal value despite restoring the insulin level in diabetic rats (58). In another study, hydroalcoholic extracts of NS with doses of 200 and 400 mg/kg for 42 days were shown to improve oxidative status by increasing the thiol content and decreasing the MDA level in the hippocampus of diabetic rats (47).
Anti-inflammatory effects
Diabetes induced-oxidative damage may create ripple effects that trigger widespread inflammatory reactions, which further exacerbate the damage to the tissues. Studies have shown that the pro-inflammatory molecules such as interleukin 6 (IL-6), interleukin-1beta (IL-1β), and tumor necrosis factor (TNF-α) are significantly elevated in diabetic humans and experimental models (79). Furthermore, these pro-inflammatory markers serve a crucial role in metabolic inflammation caused by the impairment of serine phosphorylation of insulin receptor substrate 1 (IRS-1), which regulates insulin receptor signaling and consequently modulates insulin action (80).
Studies have reported that IL-1β, IL-6, and TNF-α were significantly reduced in the serum of HFD-STZ-induced diabetic rats treated with NS seed polysaccharides (NSSP) for 4 weeks (54). This may relate to the NS potential to significantly downregulate the gene and protein expression of inflammatory chemokines, as well as repress the activation of NFκB and MAPK pathways, which are crucial for cellular inflammatory responses (76). The green synthesis of silver nanoparticles (SeNPs) using NS seed extract also revealed similar effects in causing a reduction in the TNF-α and NFκB in diabetic nephropathy animal models (55). The generation of the massive pro-inflammatory cytokines further exacerbates the inflammatory reaction, which could potentiate insulin resistance by altering the insulin signaling pathways. NS administration was shown to reverse these effects by upregulating the gene expression of insulin growth factor 1 (IGF-1) and phosphoinositide-3 kinase (PI3K) (42). The increase in IGF-1 and PI3K helps promote insulin sensitivity which is crucial in maintaining blood glucose control, particularly in T2DM.
Vascular inflammation is another critical issue, which causes a high chance of morbidity and mortality in diabetic patients. Insulin resistance disrupted nitric oxide synthesis by reducing the activity of endothelial NO synthase (eNOS) along with increased production of a vasoconstrictor such as endothelin-1 (81). NS was found to reverse this effect by increasing the eNOS, which helps improve endothelial dysfunction (46). They also reported that NS treatment with ethanolic extracts for 6 weeks caused a reduction in vascular cell adhesion molecule 1 (VCAM-1), mainly used to allow firm adhesion of leukocytes to endothelial cells and promotes vascular inflammation (82). A previous study reported that NS bioactive compound thymoquinone disrupts the link between TNF- α signaling pathway that regulates the ICAM/VCAM expression in arthritis in vitro (83). This means that NS mediates its effects by modulating the adhesion molecule as well as controlling the inflammatory markers.
Interestingly, Balbaa et al. reported that the combination of NS oil and hypoglycaemic drugs such as metformin and glibenclamide inhibited the inducible nitric oxide synthase (iNOS) and NO formation in the brain tissue (41). As iNOS is considered one of the important regulators for insulin resistance, its inhibition minimizes the risk of disease progression. In an earlier study, Balbaa et al. also revealed a reduced serum NO in T2DM rats (42). Similarly, Hamdy and Taha also reported a significant reduction in NO levels in both heart and brain samples (37). Nitric oxide works like double edge swords, yielding different outcomes in various studies. The lack of NO leads to vascular damage by causing endothelial dysfunction, as mentioned earlier, and NO in excess causes the propagation of oxidative stress and inflammation. Hence, the NS's ability to restore NO homeostasis is warranted in managing DM.
Anti-apoptotic effects
The abundance generation of ROS and pro-inflammatory cytokines opens the gate for the activation of the apoptosis cascade and leakage of cytochrome-c, which lead to more detrimental effects. The extrinsic apoptotic pathway is initiated by binding the Fas ligand to the death receptor to activate the caspase complex. Whereas, the intrinsic apoptotic pathway is derived from the regulated B-cell lymphoma (BCL-2), which expresses both pro- and anti-apoptotic signals (84). In response to apoptotic stimuli, upregulation of BCL-2-associated X protein (BAX) and downregulation of BCL2 and has reported promoting cell death in diabetic models (85).
NS significantly increases the expression BCL2 in cardiac tissue following NS oil administration for 21 days (35). Cuce et al. also found a similar result as they reported a reduction in BAX and caspase 3 expression in the abdominal and thoracic aortic section, preventing apoptosis in the vessels of STZ-induced diabetic rats (57). The antiapoptotic effect of NS is not only beneficial for preventing cellular damage in DM but also resourceful in managing cancerous cells, as reported widely (86). These studies highly suggest that NS treatment could modulate both the intrinsic and extrinsic pathways of hyperglycaemia-induced cell death.
Hepatoprotective effects
Hyperglycaemia and insulin resistance in DM affect the carbohydrates, fats and protein metabolism, potentially leading to the formation of non-alcoholic fatty liver disease. If there is no proper intervention, there is a risk of progression to hepatitis, cirrhosis, and eventually hepatocellular carcinoma (87). Among the main triggers responsible for this deteriorating effect are oxidative stress and liver inflammation. Various studies have reported that high lipid peroxidation (LPO) could damage the hepatocyte, characterized by raised liver enzyme and alteration of the histoarchitecture of the liver (78). Administration of the NS oil for 30 days improved hepatic antioxidant enzymes such as CAT and GSH while preventing the LPO in the liver of STZ-induced rats (43).
Similar hepatoprotective effects were shown by Kaleem et al. using NS ethanol extract treatment for 4 weeks (61). They found that 300 mg/kg NS ethanol extract improved the antioxidant enzymes, such as CAT, SOD, and LPO products [thiobarbituric acid reactive substance (TBARS) and hydroperoxides] and reduced glutathione (GSH) and glutathione peroxidase (GSHPx) in both liver and kidney (61) of STZ-induced rats. Interestingly, a study on alloxan-induced rabbits also demonstrated improved GSH and ceruloplasmin concentrations whilst preventing LPO-induced liver damage in diabetic rabbits (44).
The hepatoprotective effect of NS is not limited to its antioxidant properties as they are also shown to improve the hepatic glycogen content (43). Furthermore, they postulated that the improvement of the hepatic glycogen content is due to the advancement of insulin secretion, which promotes glycogenesis in the liver.
Fararh et al. supported this finding and reported NS oil extracts could decrease hepatic gluconeogenesis by reducing its precursors (alanine, glycerol, and lactate) (39) and prevent the formation of glucose.
Interestingly, Sobhi et al. reported that treatment with NS seed extract did not show a hepatoprotective effect on acetaminophen following treatment for 4 weeks (52). Acetaminophen is an analgesic and antipyretic agent with a direct hepatotoxic effect in a large dose. Even though NS did not possess a protective effect on the liver from acetaminophen, no hepatotoxicity was found when tested alone in STZ-NA-induced rats. The liver plays a huge role in maintaining blood glucose levels; therefore, preserving its function is warranted as a treatment modality for DM.
Digestive enzyme inhibition
Another issue faced in T2DM patients is a spike in blood sugar levels following a meal, referred to as postprandial blood glucose. Hydrolysis of carbohydrates by a digestive enzyme, such as α-amylase and α-glucosidase, contributes to the increase of blood glucose levels in the body (88). Therefore, one therapeutic strategy aimed at maintaining the blood glucose level is inhibiting these digestive enzymes. Currently, a few inhibitors are available in the market, such as voglibose and acarbose. However, the issue with these medications is the disturbing side effect, such as flatulence and digestive disorders. NS's role as an inhibitor carbohydrate digestive enzyme was studied in 2019 by Hannan et al. (65). The methanolic extract of NS causes a reduction or delaying carbohydrate digestion and absorption by improving glucose tolerance and reducing disaccharidase enzyme activity in STZ-induced diabetic rats (65).
Tiji et al. also reported similar findings. NS extraction reveals to cause a reduction in both intestinal α-glucosidase and pancreatic α-amylase activity, with NS acetone extract showing the greatest inhibitory effect. This suggests the possibility of the polyphenol compounds, such as catechin and gallic acid, as the main contributors to digestive enzyme inhibitory effects (67). A different finding is reported by Dalli et al. as they found that the in vitro α-amylase percentage of inhibition is greatest in the aqueous fraction (92.24%), which is similar to the positive control used in this study, Acarbose; followed by methanol (86%), ethanol (81%), dichloromethane and n-hexane fractions; both are showing 64% inhibition. In vivo assessment of the α-amylase inhibition also revealed that aqueous and an ethanolic fractions of NS reduced the glucose 30 min after the oral sucrose load. However, in the in situ glucose absorption, methanolic extract caused better control compared to the aqueous fraction. The various degrees of inhibition in different fractions suggest that the α-amylase inhibitory effect of NS is attributed to different bioactive compounds such as quercetin, kaempferol, thymoquinone and others, as shown in their HPLC and GC-MS findings (68).
Interestingly, the NS digestive enzyme inhibition was also evaluated using different preparations of nanoparticles.
Due to its high antioxidant potential, NS has been utilized as a capping or reducing agent in producing nanoparticles. Vijayakumar et al. reported that the silver nanoparticles synthesized using NS (NS AgNPs) possessed a high degree of inhibition in both α-amylase and α-glucosidase enzymes. They also reported an increase in the inhibition of the dipeptylpeptidase-IV enzyme (DPP-IV). DPP-IV acts by inhibiting the incretins such as GLP-1 and GIP. As the incretins stimulate insulin release, inactivation of the incretins by the DPP-IV enzyme exerts a negative response toward high blood glucose control, especially in T2DM. Inhibition of this DPP-IV enzyme could further potentiate better blood glucose control, as reported in this study. It can work similarly to gliptin, the available DPP-IV inhibitor in the market (69). In a more recent study, Veeramani et al. reported that NS-coated gold nanoparticles (AuNPs) show potent antioxidant activity in in vitro DPPH assay, as well as causing significant inhibition on α-amylase and α-glucosidase in a dose-dependent manner (27). Similarly, the microencapsulation of the hydroacetone extract of NS also reported a high α-amylase enzyme inhibition compared to acarbose, signifying that the microencapsulation of NS enhances the inhibitory effect against the carbohydrate digestive enzyme (89).
Clinical trials
Due to the promising NS antidiabetic potential shown in preclinical studies, clinical trials have been conducted to translate the findings into clinical use. In this review, 13 clinical trials were selected, as summarized in Table 3. However, in these clinical studies, patients are on the standard regime of an oral hypoglycaemic agent (OHA) or other medications (antihypertensive and antihyperlipidemic agents).
Similar to in vitro and in vivo studies, the clinical trials revealed that NS has the potential as a supplementary treatment for T2DM. The duration used for the clinical trials ranges from 8 weeks (96, 98) up to 1 year (93–95). The treatment of T2DM for 8 weeks with NS oil has shown marked improvement in terms of glycaemic control (decreased in FBG and HbA1c and increased insulin level) and the amelioration of the lipid profile (considerable reduction in T-cholesterol, LDL and TG and an increase in HDL). The improvement in these parameters could help minimize the cardiometabolic risk factors in T2DM (96, 98). Furthermore, hyperlipidaemia is considered as one of the important triggers of the oxidative inflammatory cascade in T2DM (76); therefore, NS's hypolipidaemic property could reduce the risk of T2DM progression and complications (98–100).
Also, ameliorating dyslipidaemia could help improve cardiovascular function in patients with T2DM. Hadi et al. further reported that along with the improvement in lipid profile, patients had shown a substantial decrease in BMI, waist circumference, systolic blood pressure (SBP) and diastolic blood pressure (DBP) at a dose of 500 mg NS daily (96). This finding is supported further by Bamosa et al., who found that treatment with 2 g powdered NS for a year could reduce blood pressure, protect the heart from diastolic dysfunction and improve left ventricular (LV) systolic function (95). In this study, an echocardiogram was performed at baseline and repeated at 6 and 12 months follow-ups to evaluate the cardiac function of patients with T2DM. They found that NS could preserve diastolic function by monitoring the transmitral flow ratio, which was substantially reduced in the placebo group. In addition, NS also protects the heart from the development of LV hypertrophy and improves systolic function.
The cardioprotective effect of NS was consistent with the animal studies, which showed a reduction in oxidative stress parameters in the aorta and cardiac tissue following the treatment with hydroalcoholic extract of NS for 6 weeks in STZ-induced diabetic rats (47). This result suggests that ameliorating oxidative stress is one of the NS's potential actions in causing improvement in cardiac function in DM. This antioxidant effect is further supported by Kaatabi et al., who reported a considerable increase in total antioxidant capacity (TAC), CAT, GSH and SOD and a remarkable reduction in TBARS following treatment with 500 mg NS powdered capsule in T2DM patients (94). This finding is consistent with a study by Kooshki et al., which showed a reduction in serum MDA, the LPO by-product, in patients with T2DM treated with 1,000 mg NS for 8 weeks (98). The improvement in antioxidant enzymes could help minimize oxidative stress progression in T2DM and could be a mechanism contributing to the hypoglycaemic effect of NS.
Kaatabi et al. also reported increased pancreatic β cell activity with considerably lower insulin resistance after a year of NS treatment (94). A similar finding was reported by Bamosa et al. who demonstrated that treatment with NS oil for 12 weeks helps in the reduction of insulin resistance calculated by HOMA-IR and the improvement of β cell function in a non-controlled trial study (91). Another study reported that the effects of NS on fasting insulin and insulin resistance in newly diagnosed patients with T2DM were comparable to the standard oral hypoglycaemic agent (OHA) metformin following treatment for 3 months (97). However, different studies reported that NS causes a reduction in FBG, but no remarkable differences in insulin secretion and HOMA-IR after adjusting for changes in body weight, dietary intake and baseline value (102). This result suggests that the clinical study outcome is heavily influenced by the dosage, duration, type of clinical study, dietary intake, baseline glucose, ethnicity and activity.
The improvement in pancreatic β-cell function has also reported widely in in vitro (66) and in vivo diabetes studies (38, 41, 43, 45, 53, 62, 63). The amelioration of β-cell function could explain the improvement of insulin levels and the overall fasting and post-prandial blood glucose levels in clinical trials (91–93). The improvement in post-prandial blood glucose could be attributed partially to the inhibition of the carbohydrate digestion enzyme by NS (52, 67, 68). Post-prandial blood glucose control helps prevent the post-meal spike in blood glucose levels, which is crucial in preventing T2DM progression and complications. After NS treatment, improvement in glycated hemoglobin levels resulted a well-controlled blood glucose level (91–95, 102). The organ-protecting effect was also observed in the liver and kidney, in which treatment with NS caused a reduction in serum creatinine and urea with improvement in glomerular filtration rate and 24 h urinary volume (101) and a reduction in ALT (90), AST and total serum bilirubin (92).
Discussion
NS belongs to the genus Nigella in the family Ranunculaceae. Some of the plants belonging to this genus include N. damascene, N. arvensis, N. hispanica, N. nigellastrum and N. orientalis. Compared to the other plants in the same genus, only NS has reported antidiabetic properties. However, phytochemical evaluations of these plants show that they possess bioactive compounds, which could be beneficial in managing DM, such as quercetin, quercitrin, hyperoside, carvacrol, thymohydroquinone, and kaempferol, to name a few (103). These compounds have been studied extensively and show potential antioxidant, anti-inflammatory and antiapoptotic properties. N. damascene, one of the plants from the same genus, was reported to have antioxidant effects owing to its quercetin and its derivative content. The polar extract of NS was also shown to possess a wide range of phenolic compounds, which were found to have high antioxidant capacity. However, the potential effect of nigella genus plants is not only limited to the antioxidant activity as they are also reported to ameliorate dyslipidaemia. Kokdil et al. reported the administration of N. orientalis and N. segetalis fixed oils orally (1 mL/kg/day) on Wistar Kyoto rats for 4 weeks increased HDL-C and N. orientalis also caused a decrease in LDL-C and VLDL-C. Both plants also increased the total antioxidant status in the experimental rats (104). Due to the high potential of NS, the other plant of the same genus should be explored to unravel their antidiabetic potential further.
The in vivo and in vitro trials have shown a wide array of NS potential to be utilized as hypoglycaemic agents. Some NS actions considered the main attributes of its antidiabetic potentials are antioxidant, anti-inflammatory and insulin-mimetic properties. Additionally, the action of NS in lowering blood glucose levels mimics the currently available OHAs in the market. For instance, similar to Metformin, NS is shown to activate the AMPK pathway (59), enhance insulin sensitivity and glucose uptake and fatty acid oxidation, and reduce intestinal glucose absorption (60). Not only that, NS has proven potential in the modulation of the carbohydrate digestive enzyme to prevent and control the fluctuations of postprandial blood glucose level, similar to the actions of miglitol, voglibose, and acarbose, currently available antidiabetic drugs that are prescribed to T2DM patients. Other than its individual effects, NS could potentiate the effect of these antidiabetic drugs, and synergise the effect, which could help in more holistic control in managing the blood glucose level. Furthermore, the dosage of the medication and the toxicity could be reduced when applied in combination, hence improving the patients' overall outcome.
Based on the clinical trials, several DM biomarkers could be assessed to trace the effects of NS and its bioactive compound in causing the desired effect. The potential biosensing marker could be the basis of DM parameters such as fasting and post-prandial blood glucose level and glycated hemoglobin; to evaluate the long-term blood glucose control as well as compliance toward the treatment and the plasma insulin level. Additionally, the cardiometabolic biosensing marker, such as lipid profile, could be monitored to prevent progression toward diabetic cardiomyopathy (105). However, it should not be left unnoticed that in these clinical trials, patients are still on the OHA's, which means the effects seen in these patients are synergistic effects with the medication they took rather than individual effects of NS. Future studies must be conducted to evaluate the NS effects in the combination of OHA and its pharmacological and toxicological profile for future clinical applications.
However, despite numerous studies showing NS promising results to be utilized in DM management, it is still bound to several limitations that require attention before clinical application. Similar to other plant resources, the NS's bioavailability in terms of solubility, stability and half-life remains the main issue in elucidating the warranted effects. Modulation of the structure and different doses and strategies have been studied extensively in preclinical settings, which gives a wide array of information before safely being conducted in the clinical trial. Additionally, the drug-herb interaction is another important issue to be further validated to ensure clinical therapeutic efficacy. It may cause risks of unwanted pharmacokinetic and pharmacodynamic interactions when co-administered. Hence, a more structured and extensive human trial is needed to further verify the mechanism of action, pharmacological effect, the range of safe doses and toxicological profile before utilizing NS as an antidiabetic agent.
Conclusion
This review article demonstrates the therapeutic potential of NS could be used as a complement or adjuvant for the management of DM and its complications. Various preparations of NS have been studied to provide therapeutic strategies for maximizing the antidiabetic effects of NS. NS exhibits multifactorial activities that target several tissues at the same time owing to its phytochemical properties. The proposed mechanism includes ameliorating oxidative, inflammatory, apoptotic, and insulinotropic effects and inhibiting carbohydrate-digesting enzymes, to name a few. Similar promising hypoglycaemic effects have been found in clinical studies involving real patients with T2DM, further validating the potential of NS. As a whole, NS appears to play a role as a complement or adjuvant in managing DM and its complications. However, future research should be able to replicate and fill in the gaps to introduce NS safely in patients with DM.
Author contributions
MM, SA, and MK: conceptualization, methodology, and writing—review and editing. SA, NM, EA, and MA: writing—original draft preparation. SA and EA: visualization. All authors have read and agreed to the published version of the manuscript.
Conflict of interest
The authors declare that the research was conducted in the absence of any commercial or financial relationships that could be construed as a potential conflict of interest.
Publisher's note
All claims expressed in this article are solely those of the authors and do not necessarily represent those of their affiliated organizations, or those of the publisher, the editors and the reviewers. Any product that may be evaluated in this article, or claim that may be made by its manufacturer, is not guaranteed or endorsed by the publisher.
References
1. Artasensi A, Pedretti A, Vistoli G, Fumagalli L. Type 2 diabetes mellitus: a review of multi-target drugs. Molecules. (2020) 25:81987. doi: 10.3390/molecules25081987
2. Kharroubi AT, Darwish HM. Diabetes mellitus: the epidemic of the century. World J Diabet. (2015) 6:850–67. doi: 10.4239/wjd.v6.i6.850
3. Jiang S, Young JL, Wang K, Qian Y, Cai L. Diabeticinduced alterations in hepatic glucose and lipid metabolism: the role of type 1 and type 2 diabetes mellitus (Review). Mol Med Rep. (2020) 22:603–11. doi: 10.3892/mmr.2020.11175
4. American Diabetes Association C. Diagnosis and classification of diabetes mellitus. Diabet Care. (2010) 33(Suppl.1):S62–9. doi: 10.2337/dc10-S062
5. Roden M, Shulman GI. The integrative biology of type 2 diabetes. Nature. (2019) 576:51–60. doi: 10.1038/s41586-019-1797-8
6. Galicia-Garcia U, Benito-Vicente A, Jebari S, Larrea-Sebal A, Siddiqi H, Uribe KB, et al. Pathophysiology of type 2 diabetes mellitus. Int J Mol Sci. (2020) 21:176275. doi: 10.3390/ijms21176275
7. Tan SY, Mei Wong JL, Sim YJ, Wong SS, Mohamed Elhassan SA, Tan SH, et al. Type 1 and 2 diabetes mellitus: a review on current treatment approach and gene therapy as potential intervention. Diabetes Metab Syndr. (2019) 13:364–72. doi: 10.1016/j.dsx.2018.10.008
8. Lin SH, Cheng PC, Tu ST, Hsu SR, Cheng YC, Liu YH. Effect of metformin monotherapy on serum lipid profile in statin-naive individuals with newly diagnosed type 2 diabetes mellitus: a cohort study. PeerJ. (2018) 6:e4578. doi: 10.7717/peerj.4578
9. Kooti W, Hasanzadeh-Noohi Z, Sharafi-Ahvazi N, Asadi-Samani M, Ashtary-Larky D. Phytochemistry, pharmacology, and therapeutic uses of black seed (Nigella sativa). Chin J Nat Med. (2016) 14:732–45. doi: 10.1016/S1875-5364(16)30088-7
10. Padhye S, Banerjee S, Ahmad A, Mohamad R, Sarkar FH. From here to eternity - the secret of Pharaohs: therapeutic potential of black cumin seeds and beyond. Cancer Ther. (2008) 6:495–510.
11. Hosseini SS, Nadjafi F, Asareh MH, Rezadoost H. Morphological and yield related traits, essential oil and oil production of different landraces of black cumin (Nigella sativa) in Iran. Sci Hortic. (2018) 233:1–8. doi: 10.1016/j.scienta.2018.01.038
12. Ahmad A, Husain A, Mujeeb M, Khan SA, Najmi AK, Siddique NA, et al. review on therapeutic potential of Nigella sativa: a miracle herb. Asian Pac J Trop Biomed. (2013) 3:337–52. doi: 10.1016/S2221-1691(13)60075-1
13. Areefa A, Mohd A, Shah CS. A review on Nigella sativa (Kalonji) seeds: a universal healer. CellMed. (2020) 10:1–14. doi: 10.5667/CellMed.2020.001
14. Barakat EM, El Wakeel LM, Hagag RS. Effects of Nigella sativa on outcome of hepatitis C in Egypt. World J Gastroenterol. (2013) 19:2529–36. doi: 10.3748/wjg.v19.i16.2529
15. Maideen NMP. Antidiabetic activity of Nigella sativa (black seeds) and its active constituent (thymoquinone): a review of human and experimental animal studies. Chonnam Med J. (2021) 57:169–75. doi: 10.4068/cmj.2021.57.3.169
16. Takruri HRH, Dameh MAF. Study of the nutritional value of black cumin seeds (Nigella sativa L.). J Sci Food Agric. (1998) 76:404–10. doi: 10.1002/(SICI)1097-0010(199803)76:3<404::AID-JSFA964>3.0.CO;2-L
17. Ramadan MF. Nutritional value. Functional properties and nutraceutical applications of black cumin (Nigella sativa L.): an overview. Int J Food Sci Technol. (2007) 42:1208–18. doi: 10.1111/j.1365-2621.2006.01417.x
18. Yimer EM, Tuem KB, Karim A, Ur-Rehman N, Anwar F. Nigella sativa L. (Black Cumin): a promising natural remedy for wide range of illnesses. Evid Based Complement Alternat Med. (2019) 2019:1528635. doi: 10.1155/2019/1528635
19. Mamun MA. Major nutritional compositions of black cumin seeds – cultivated in Bangladesh and the physicochemical characteristics of its oil. Int Food Res J. (2018) 25:2634–9.
20. Al-Jasass FM, Al-Jasser MS. Chemical composition and fatty acid content of some spices and herbs under Saudi Arabia conditions. ScientificWorldJournal. (2012) 2012:859892. doi: 10.1100/2012/859892
21. Alrashidi M, Derawi D, Salimon J, Firdaus Yusoff M. An investigation of physicochemical properties of Nigella sativa L. seed oil from Al-Qassim by different extraction methods. J King Saud Univ Sci. (2020) 32:3337–42. doi: 10.1016/j.jksus.2020.09.019
22. Silva AFC, Haris PI, Serralheiro ML, Pacheco R. Mechanism of action and the biological activities of Nigella sativa oil components. Food Bioscience. (2020) 38:100783. doi: 10.1016/j.fbio.2020.100783
23. Piras A, Rosa A, Marongiu B, Porcedda S, Falconieri D, Dessì MA, et al. Chemical composition and in vitro bioactivity of the volatile and fixed oils of Nigella sativa L. extracted by supercritical carbon dioxide. Indus Crops Prod. (2013) 46:317–23. doi: 10.1016/j.indcrop.2013.02.013
24. Jrah Harzallah H, Kouidhi B, Flamini G, Bakhrouf A, Mahjoub T. Chemical composition, antimicrobial potential against cariogenic bacteria and cytotoxic activity of Tunisian Nigella sativa essential oil and thymoquinone. Food Chem. (2011) 129:1469–74. doi: 10.1016/j.foodchem.2011.05.117
25. Bourgou S, Ksouri R, Bellila A, Skandrani I, Falleh H, Marzouk B. Phenolic composition and biological activities of Tunisian Nigella sativa L. shoots and roots. C R Biol. (2008) 331:48–55. doi: 10.1016/j.crvi.2007.11.001
26. Kabir Y, Akasaka-Hashimoto Y, Kubota K, Komai M. Volatile compounds of black cumin (Nigella sativa L.) seeds cultivated in Bangladesh and India. Heliyon. (2020) 6:e05343. doi: 10.1016/j.heliyon.2020.e05343
27. Veeramani S, Narayanan AP, Yuvaraj K, Sivaramakrishnan R, Pugazhendhi A, Rishivarathan I, et al. Nigella sativa flavonoids surface coated gold NPs (Au-NPs) enhancing antioxidant and antidiabetic activity. Process Biochem. (2022) 114:193–202. doi: 10.1016/j.procbio.2021.01.004
28. Gnanasekaran P, Roy A, Sirpu Natesh N, Raman V, Ganapathy P, Arumugam MK. Removal of microbial pathogens and anticancer activity of synthesised nano-thymoquinone from Nigella sativa seeds. Environ Technol Innov. (2021) 24:102068. doi: 10.1016/j.eti.2021.102068
29. Ciesielska-Figlon K, Daca A, Kokotkiewicz A, Łuczkiewicz M, Zabiegała B, Witkowski JM, et al. The influence of Nigella sativa essential oil on proliferation, activation, and apoptosis of human T lymphocytes in vitro. Biomed Pharmacother. (2022) 153:113349. doi: 10.1016/j.biopha.2022.113349
30. Wajs A, Bonikowski R, Kalemba D. Composition of essential oil from seeds of Nigella sativa L. cultivated in Poland. Flavour Fragr J. (2008) 23:126–32. doi: 10.1002/ffj.1866
31. Akram Khan M, Afzal M. Chemical composition of Nigella sativa Linn: part 2 recent advances. Inflammopharmacology. (2016) 24:67–79. doi: 10.1007/s10787-016-0262-7
32. Srinivasan K. Cumin (Cuminum cyminum) and black cumin (Nigella sativa) seeds: traditional uses, chemical constituents, and nutraceutical effects. Food Qual Saf. (2018) 2:1–16. doi: 10.1093/fqsafe/fyx031
33. Singh G, Marimuthu P, de Heluani CS, Catalan C. Chemical constituents and antimicrobial and antioxidant potentials of essential oil and acetone extract of Nigella sativa seeds. J Sci Food Agric. (2005) 85:2297–306. doi: 10.1002/jsfa.2255
34. Seflek HN, Kalkan S, Cuce G, Kilinc I, Sozen ME. Effects of Nigella sativa oil on ovarian volume, oxidant systems, XIAP and NF-kB expression in an experimental model of diabetes. Biotech Histochem. (2019) 94:325–33. doi: 10.1080/10520295.2019.1566571
35. Altun E, Avci E, Yildirim T, Yildirim S. Protective effect of Nigella sativa oil on myocardium in streptozotocin-induced diabetic rats. Acta Endocrinol. (2019) 15:289–94. doi: 10.4183/aeb.2019.289
36. Al-Trad B, Al-Batayneh K, El-Metwally S, Alhazimi A, Ginawi I, Alaraj M, et al. Nigella sativa oil and thymoquinone ameliorate albuminuria and renal extracellular matrix accumulation in the experimental diabetic rats. Eur Rev Med Pharmacol Sci. (2016) 20:2680–8.
37. Hamdy NM, Taha RA. Effects of Nigella sativa oil and thymoquinone on oxidative stress and neuropathy in streptozotocin-induced diabetic rats. Pharmacology. (2009) 84:127–34. doi: 10.1159/000234466
38. Fararh KM, Atoji Y, Shimizu Y, Takewaki T. Isulinotropic properties of Nigella sativa oil in Streptozotocin plus Nicotinamide diabetic hamster. Res Vet Sci. (2002) 73:279–82. doi: 10.1016/S0034-5288(02)00108-X
39. Fararh KM, Atoji Y, Shimizu Y, Shiina T, Nikami H, Takewaki T. Mechanisms of the hypoglycaemic and immunopotentiating effects of Nigella sativa L. oil in streptozotocin-induced diabetic hamsters. Res Vet Sci. (2004) 77:123–9. doi: 10.1016/j.rvsc.2004.03.002
40. El-Dakhakhny M, Mady N, Lembert N, Ammon HP. The hypoglycemic effect of Nigella sativa oil is mediated by extrapancreatic actions. Planta Med. (2002) 68:465–6. doi: 10.1055/s-2002-32084
41. Balbaa M, Abdulmalek SA, Khalil S. Oxidative stress and expression of insulin signaling proteins in the brain of diabetic rats: role of Nigella sativa oil and antidiabetic drugs. PLoS ONE. (2017) 12:e0172429. doi: 10.1371/journal.pone.0172429
42. Balbaa M, El-Zeftawy M, Ghareeb D, Taha N, Mandour AW. Nigella sativa relieves the altered insulin receptor signaling in streptozotocin-induced diabetic rats fed with a high-fat diet. Oxid Med Cell Longev. (2016) 2016:2492107. doi: 10.1155/2016/2492107
43. Abdelrazek HMA, Kilany OE, Muhammad MAA, Tag HM, Abdelazim AM. Black seed thymoquinone improved insulin secretion, hepatic glycogen storage, and oxidative stress in streptozotocin-induced diabetic male wistar rats. Oxid Med Cell Longev. (2018) 2018:8104165. doi: 10.1155/2018/8104165
44. Meral I, Yener Z, Kahraman T, Mert N. Effect of Nigella sativa on glucose concentration, lipid peroxidation, anti-oxidant defence system and liver damage in experimentally-induced diabetic rabbits. J Vet Med A Physiol Pathol Clin Med. (2001) 48:593–9. doi: 10.1046/j.1439-0442.2001.00393.x
45. Kanter M, Akpolat M, Aktas C. Protective effects of the volatile oil of Nigella sativa seeds on beta-cell damage in streptozotocin-induced diabetic rats: a light and electron microscopic study. J Mol Histol. (2009) 40:379–85. doi: 10.1007/s10735-009-9251-0
46. Abbasnezhad A, Niazmand S, Mahmoudabady M, Rezaee SA, Soukhtanloo M, Mosallanejad R, et al. Nigella sativa L. seed regulated eNOS, VCAM-1 and LOX-1 genes expression and improved vasoreactivity in aorta of diabetic rat. J Ethnopharmacol. (2019) 228:142–7. doi: 10.1016/j.jep.2018.09.021
47. Abbasnezhad A, Hayatdavoudi P, Niazmand S, Mahmoudabady M. The effects of hydroalcoholic extract of Nigella sativa seed on oxidative stress in hippocampus of STZ-induced diabetic rats. Avicenna J Phytomed. (2015) 5:333–40.
48. Bensiameur-Touati K, Kacimi G, Haffaf EM, Berdja S, Aouichat-Bouguerra S. In vivo subacute toxicity and antidiabetic effect of aqueous extract of Nigella sativa. Evid Based Complement Alternat Med. (2017) 2017:8427034. doi: 10.1155/2017/8427034
49. Sultan MT, Butt MS, Karim R, Iqbal SZ, Ahmad S, Zia-Ul-Haq M, et al. Effect of Nigella sativa fixed and essential oils on antioxidant status, hepatic enzymes, and immunity in streptozotocin induced diabetes mellitus. BMC Complement Altern Med. (2014) 14:193. doi: 10.1186/1472-6882-14-193
50. Mohebbati R, Abbasnezhad A, Havakhah S, Mousavi M. The effect of Nigella Sativa on renal oxidative injury in diabetic rats. Saudi J Kidney Dis Transpl. (2020) 31:775–86. doi: 10.4103/1319-2442.292311
51. Sultan MT, Butt MS, Karim R, Zia-Ul-Haq M, Batool R, Ahmad S, et al. Nigella sativa fixed and essential oil supplementation modulates hyperglycemia and allied complications in streptozotocin-induced diabetes mellitus. Evid Based Complement Alternat Med. (2014) 2014:826380. doi: 10.1155/2014/826380
52. Sobhi W, Stevigny C, Duez P, Calderon BB, Atmani D, Benboubetra M. Effect of lipid extracts of Nigella sativa L. seeds on the liver ATP reduction and alpha-glucosidase inhibition. Pak J Pharm Sci. (2016) 29:111–7.
53. Abdelmeguid NE, Fakhoury R, Kamal SM, Al Wafai RJ. Effects of Nigella sativa and thymoquinone on biochemical and subcellular changes in pancreatic beta-cells of streptozotocin-induced diabetic rats. J Diabetes. (2010) 2:256–66. doi: 10.1111/j.1753-0407.2010.00091.x
54. Dong J, Liang Q, Niu Y, Jiang S, Zhou L, Wang J, et al. Effects of Nigella sativa seed polysaccharides on type 2 diabetic mice and gut microbiota. Int J Biol Macromol. (2020) 159:725–38. doi: 10.1016/j.ijbiomac.2020.05.042
55. Alkhalaf MI, Hussein RH, Hamza A. Green synthesis of silver nanoparticles by Nigella sativa extract alleviates diabetic neuropathy through anti-inflammatory and antioxidant effects. Saudi J Biol Sci. (2020) 27:2410–9. doi: 10.1016/j.sjbs.2020.05.005
56. Somboonwong J, Yusuksawad M, Keelawat S, Thongruay S, Poumsuk U. Minimization of the risk of diabetic microangiopathy in rats by Nigella sativa. Pharmacogn Mag. (2016) 12:S175–80. doi: 10.4103/0973-1296.182169
57. Cuce G, Sozen ME, Cetinkaya S, Canbaz HT, Seflek H, Kalkan S. Effects of Nigella sativa L. seed oil on intima-media thickness and Bax and Caspase 3 expression in diabetic rat aorta. Anatol J Cardiol. (2016) 16:460–6. doi: 10.5152/AnatolJCardiol.2015.6326
58. Al Wafai RJ. Nigella sativa and thymoquinone suppress cyclooxygenase-2 and oxidative stress in pancreatic tissue of streptozotocin-induced diabetic rats. Pancreas. (2013) 42:841–9. doi: 10.1097/MPA.0b013e318279ac1c
59. Benhaddou-Andaloussi A, Martineau L, Vuong T, Meddah B, Madiraju P, Settaf A, et al. The in vivo antidiabetic activity of Nigella sativa is mediated through activation of the AMPK pathway and increased muscle glut4 content. Evid Based Complement Alternat Med. (2011) 2011:538671. doi: 10.1155/2011/538671
60. Meddah B, Ducroc R, El Abbes Faouzi M, Eto B, Mahraoui L, Benhaddou-Andaloussi A, et al. Nigella sativa inhibits intestinal glucose absorption and improves glucose tolerance in rats. J Ethnopharmacol. (2009) 121:419–24. doi: 10.1016/j.jep.2008.10.040
61. Kaleem M, Kirmani D, Asif M, Ahmed Q, Bano B. Biochemical effects of Nigella sativa L. seeds in diabetic rats. Indian J Exp Biol. (2006) 44:745–8.
62. Kanter M, Coskun O, Korkmaz A, Oter S. Effects of Nigella sativa on oxidative stress and beta-cell damage in streptozotocin-induced diabetic rats. Anat Rec A Discov Mol Cell Evol Biol. (2004) 279:685–91. doi: 10.1002/ar.a.20056
63. Kanter M, Meral I, Yener Z, Ozbek H, Demir H. Partial regeneration/proliferation of the beta-cells in the islets of Langerhans by Nigella sativa L. in streptozotocin-induced diabetic rats. Tohoku J Exp Med. (2003) 201:213–9. doi: 10.1620/tjem.201.213
64. Widodo G, Herowati R, Perangin-Angin JM, Kamlasi JEY. Antihyperglycemic, antioxidant, and pancreas regeneration activities of black cumin (Nigella sativa L.) seeds ethanol extract in alloxan-induced diabetic rats. (2016) 8:37–40.
65. Hannan JMA, Ansari P, Haque A, Sanju A, Huzaifa A, Rahman A, et al. Nigella sativa stimulates insulin secretion from isolated rat islets and inhibits the digestion and absorption of (CH2O)n in the gut. Biosci Rep. (2019) 39:BSR20190723. doi: 10.1042/BSR20190723
66. Rchid H, Chevassus H, Nmila R, Guiral C, Petit P, Chokairi M, et al. Nigella sativa seed extracts enhance glucose-induced insulin release from rat-isolated Langerhans islets. Fundam Clin Pharmacol. (2004) 18:525–9. doi: 10.1111/j.1472-8206.2004.00275.x
67. Tiji S, Bouhrim M, Addi M, Drouet S, Lorenzo JM, Hano C, et al. Linking the Phytochemicals and the alpha-Glucosidase and alpha-amylase enzyme inhibitory effects of Nigella sativa seed extracts. Foods. (2021) 10:81818. doi: 10.3390/foods10081818
68. Dalli M, Daoudi NE, Azizi SE, Benouda H, Bnouham M, Gseyra N. Chemical composition analysis using HPLC-UV/GC-MS and inhibitory activity of different Nigella sativa fractions on pancreatic alpha-amylase and intestinal glucose absorption. Biomed Res Int. (2021) 2021:9979419. doi: 10.1155/2021/9979419
69. Vijayakumar S, Divya M, Vaseeharan B, Chen J, Biruntha M, Silva LP, et al. Biological compound capping of silver nanoparticle with the seed extracts of blackcumin (Nigella sativa): a potential antibacterial, antidiabetic, anti-inflammatory, and antioxidant. J Inorg Organomet Polym Mater. (2021) 31:624–35. doi: 10.1007/s10904-020-01713-4
70. Wang J, Wang H. Oxidative stress in pancreatic beta cell regeneration. Oxid Med Cell Longev. (2017) 2017:1930261. doi: 10.1155/2017/1930261
71. Song P, Onishi A, Koepsell H, Vallon V. Sodium glucose cotransporter SGLT1 as a therapeutic target in diabetes mellitus. Expert Opin Ther Targets. (2016) 20:1109–25. doi: 10.1517/14728222.2016.1168808
72. Gannon NP, Conn CA, Vaughan RA. Dietary stimulators of GLUT4 expression and translocation in skeletal muscle: a mini-review. Mol Nutr Food Res. (2015) 59:48–64. doi: 10.1002/mnfr.201400414
73. Vergès B. Pathophysiology of diabetic dyslipidaemia: where are we? Diabetologia. (2015) 58:886–99. doi: 10.1007/s00125-015-3525-8
74. Wu L, Parhofer KG. Diabetic dyslipidemia. Metabol Clin Exp. (2014) 63:1469–79. doi: 10.1016/j.metabol.2014.08.010
75. Hannan MA, Rahman MA, Sohag AAM, Uddin MJ, Dash R, Sikder MH, et al. Black cumin (Nigella sativa L): a comprehensive review on phytochemistry, health benefits, molecular pharmacology, and safety. Nutrients. (2021) 13:1784. doi: 10.3390/nu13061784
76. Evans JL, Goldfine ID, Maddux BA, Grodsky GM. Oxidative stress and stress-activated signaling pathways: a unifying hypothesis of type 2 diabetes. Endocr Rev. (2002) 23:599–622. doi: 10.1210/er.2001-0039
77. Kostić DA, Dimitrijević DS, Stojanović GS, Palić IR, 0ordević AS, Ickovski JD. Xanthine oxidase: isolation, assays of activity, and inhibition. J Chem. (2015) 2015:294858. doi: 10.1155/2015/294858
78. Tangvarasittichai S. Oxidative stress, insulin resistance, dyslipidemia and type 2 diabetes mellitus. World J Diabetes. (2015) 6:456–80. doi: 10.4239/wjd.v6.i3.456
79. Tsalamandris S, Antonopoulos AS, Oikonomou E, Papamikroulis GA, Vogiatzi G, Papaioannou S, et al. The role of inflammation in diabetes: current concepts and future perspectives. Eur Cardiol. (2019) 14:50–9. doi: 10.15420/ecr.2018.33.1
80. Boura-Halfon S, Zick Y. Phosphorylation of IRS proteins, insulin action, and insulin resistance. Am J Physiol Endocrinol Metabolism. (2009) 296:E581–91. doi: 10.1152/ajpendo.90437.2008
81. Wang H, Wang AX, Aylor K, Barrett EJ. Nitric oxide directly promotes vascular endothelial insulin transport. Diabetes. (2013) 62:4030–42. doi: 10.2337/db13-0627
82. Kong D-H, Kim YK, Kim MR, Jang JH, Lee S. Emerging roles of vascular cell adhesion molecule-1 (VCAM-1) in immunological disorders and cancer. Int J Mol Sci. (2018) 19:1057. doi: 10.3390/ijms19041057
83. Umar S, Hedaya O, Singh AK, Ahmed S. Thymoquinone inhibits TNF-α-induced inflammation and cell adhesion in rheumatoid arthritis synovial fibroblasts by ASK1 regulation. Toxicol Appl Pharmacol. (2015) 287:299–305. doi: 10.1016/j.taap.2015.06.017
84. Van Opdenbosch N, Lamkanfi M. Caspases in cell death, inflammation, and disease. Immunity. (2019) 50:1352–64. doi: 10.1016/j.immuni.2019.05.020
85. Ronco MT, de Luján Alvarez M, Monti J, Carrillo MC, Pisani G, Lugano MC, et al. Modulation of balance between apoptosis and proliferation by lipid peroxidation (LPO) during rat liver regeneration. Mol Med. (2002) 8:808–17. doi: 10.1007/BF03402085
86. Majdalawieh AF, Fayyad MW. Recent advances on the anti-cancer properties of Nigella sativa, a widely used food additive. J Ayurveda Integr Med. (2016) 7:173–80. doi: 10.1016/j.jaim.2016.07.004
87. Mohamed J, Nazratun Nafizah AH, Zariyantey AH, Budin SB. Mechanisms of diabetes-induced liver damage: the role of oxidative stress and inflammation. Sultan Qaboos Univ Med J. (2016) 16:e132–41. doi: 10.18295/squmj.2016.16.02.002
88. Alqahtani AS, Hidayathulla S, Rehman MT, ElGamal AA, Al-Massarani S, Razmovski-Naumovski V, et al. Alpha-amylase and alpha-glucosidase enzyme inhibition and antioxidant potential of 3-oxolupenal and katononic acid isolated from Nuxia oppositifolia. Biomolecules. (2020) 10:61. doi: 10.3390/biom10010061
89. Varghese L, Mehrotra N. α-Amylase inhibitory activity of microencapsulated Nigella sativa L. and herb- drug interaction: an in vitro analysis. Ann Phytomed. (2020) 9:12. doi: 10.21276/ap.2020.9.1.12
90. Ahmad B, Masud T, Uppal A, Naveed A. Effects of Nigella sativa oil on some blood parameters in type 2 diabetes mellitus patients. Asian J Chem. (2009) 21:5373–81.
91. Bamosa AO, Kaatabi H, Lebdaa FM, Elq AM, Al-Sultanb A. Effect of Nigella sativa seeds on the glycemic control of patients with type 2 diabetes mellitus. Indian J Physiol Pharmacol. (2010) 54:344–54.
92. El-Shamy KA, Mosa MMA, El-Nabarawy SK, El-Qattan M. Effect of Nigella sativa tea in type 2-diabetic patients as regards glucose homeostasis, liver and kidney functions. J Appl Sci Res. (2011) 7:1982–91.
93. Hosseini MS, Mirkarimi SA, Amini M, Mohtashami R, Kianbakht S, Fallah Huseini H. Effects of Nigella sativa L. seed oil in type II diabetic patients: a randomized, double-blind, placebo - controlled clinical trial. J Medicinal Plants. (2013) 12:93–9.
94. Kaatabi H, Bamosa AO, Badar A, Al-Elq A, Abou-Hozaifa B, Lebda F, et al. Nigella sativa improves glycemic control and ameliorates oxidative stress in patients with type 2 diabetes mellitus: placebo controlled participant blinded clinical trial. PLoS ONE. (2015) 10:e0113486. doi: 10.1371/journal.pone.0113486
95. Bamosa A, Kaatabi H, Badar A, Al-Khadra A, Al Elq A, Abou-Hozaifa B, et al. Nigella sativa: a potential natural protective agent against cardiac dysfunction in patients with type 2 diabetes mellitus. J Family Community Med. (2015) 22:88–95. doi: 10.4103/2230-8229.155380
96. Hadi S, Daryabeygi-Khotbehsara R, Mirmiran P, McVicar J, Hadi V, Soleimani D, et al. Effect of Nigella sativa oil extract on cardiometabolic risk factors in type 2 diabetes: a randomised, double-blind, placebo-controlled clinical trial. Phytother Res. (2021) 35:3747–55. doi: 10.1002/ptr.6990
97. Moustafa HAM, El Wakeel LM, Halawa MR, Sabri NA, El-Bahy AZ, Singab AN. Effect of Nigella Sativa oil vs. metformin on glycemic control and biochemical parameters of newly diagnosed type 2 diabetes mellitus patients. Endocrine. (2019) 65:286–94. doi: 10.1007/s12020-019-01963-4
98. Kooshki A, Tofighiyan T, Rastgoo N, Rakhshani MH, Miri M. Effect of Nigella sativa oil supplement on risk factors for cardiovascular diseases in patients with type 2 diabetes mellitus. Phytother Res. (2020) 34:2706–11. doi: 10.1002/ptr.6707
99. Kaatabi H, Bamosa AO, Lebda FM, Al Elq AH, Al-Sultan AI. Favorable impact of Nigella sativa seeds on lipid profile in type 2 diabetic patients. J Family Community Med. (2012) 19:155–61. doi: 10.4103/2230-8229.102311
100. Badar A, Kaatabi H, Bamosa A, Al-Elq A, Abou-Hozaifa B, Lebda F, et al. Effect of Nigella sativa supplementation over a one-year period on lipid levels, blood pressure and heart rate in type-2 diabetic patients receiving oral hypoglycemic agents: nonrandomised clinical trial. Ann Saudi Med. (2017) 37:56–63. doi: 10.5144/0256-4947.2017.56
101. Ansari ZM, Nasiruddin M, Khan RA, Haque SF. Protective role of Nigella sativa in diabetic nephropathy: a randomised clinical trial. Saudi J Kidney Dis Transpl. (2017) 28:9–14. doi: 10.4103/1319-2442.198093
102. Heshmati J, Namazi N, Memarzadeh M-R, Taghizadeh M, Kolahdooz F. Nigella sativa oil affects glucose metabolism and lipid concentrations in patients with type 2 diabetes: a randomised, double-blind, placebo-controlled trial. Food Res Int. (2015) 70:87–93. doi: 10.1016/j.foodres.2015.01.030
103. Salehi B, Quispe C, Imran M, Ul-Haq I, Živković J, Abu-Reidah IM, et al. Nigella plants - traditional uses, bioactive phytoconstituents, preclinical and clinical studies. Front Pharmacol. (2021) 12:625386. doi: 10.3389/fphar.2021.625386
104. Kökdil G, Tamer L, Ercan B, Çelik M, Atik U. Effects of Nigella orientalis and N. segetalis fixed oils on blood biochemistry in rats. Phytother Res. (2006) 20:71–5. doi: 10.1002/ptr.1809
Keywords: Nigella sativa, thymoquinone, diabetes mellitus, antidiabetic, antioxidative, hypoglycaemic
Citation: Adam SH, Mohd Nasri N, Kashim MIAM, Abd Latib EH, Ahmad Juhari MAA and Mokhtar MH (2022) Potential health benefits of Nigella sativa on diabetes mellitus and its complications: A review from laboratory studies to clinical trials. Front. Nutr. 9:1057825. doi: 10.3389/fnut.2022.1057825
Received: 30 September 2022; Accepted: 25 October 2022;
Published: 10 November 2022.
Edited by:
Muwen Lu, South China Agricultural University, ChinaReviewed by:
Tabussam Tufail, University of Lahore, PakistanHassan Rasouli, Kermanshah University of Medical Sciences, Iran
Copyright © 2022 Adam, Mohd Nasri, Kashim, Abd Latib, Ahmad Juhari and Mokhtar. This is an open-access article distributed under the terms of the Creative Commons Attribution License (CC BY). The use, distribution or reproduction in other forums is permitted, provided the original author(s) and the copyright owner(s) are credited and that the original publication in this journal is cited, in accordance with accepted academic practice. No use, distribution or reproduction is permitted which does not comply with these terms.
*Correspondence: Mohd Helmy Mokhtar, aGVsbXkmI3gwMDA0MDt1a20uZWR1Lm15