- 1Department of Gastroenterology, The Second Affiliated Hospital and Yuying Children’s Hospital of Wenzhou Medical University, Wenzhou, China
- 2Department of Pathophysiology, School of Basic Medicine Science, Wenzhou Medical University, Wenzhou, China
Background and aims: Alcoholic liver disease (ALD) is characterized by impaired liver function due to chronic alcohol consumption, even fatal in severe cases. We performed a meta-analysis to determine whether microbial agents have therapeutic potential for ALD and elucidate the underlying mechanisms.
Methods and results: Forty-one studies were eligible for this meta-analysis after searching the PubMed, Cochrane, and Embase databases. The combined analysis showed that microbial therapy significantly decreased hepatic enzymatic parameters, including alanine transaminase [standardized mean difference (SMD): –2.70, 95% confidence interval (CI): –3.33 to –2.07], aspartate aminotransferase (SMD: –3.37, 95% CI: –4.25 to –2.49), γ-glutamyl transpeptidase (SMD: -2.07, 95% CI: –3.01 to –1.12), and alkaline phosphatase (SMD: –2.12, 95% CI: –3.32 to –0.92). Microbial agents endotoxin to enter the portal circulation and increasing reduced total cholesterol (SMD = -2.75, 95%CI -4.03 to -1.46) and triglycerides (SMD = –2.64, 95% CI: –3.22 to –2.06). Microbial agents increased amounts of the beneficial flora Lactobacillus (SMD: 4.40, 95% CI: 0.97–7.84) and Bifidobacteria (SMD: 3.84, 95% CI: 0.22–7.45), Bacteroidetes (SMD: 2.51, 95% CI: 0.29–4.72) and decreased harmful Proteobacteria (SMD: –4.18, 95% CI: –6.60 to –1.77), protecting the integrity of the intestinal epithelium and relieving endotoxin (SMD: –2.70, 95% CI: -3.52 to –2.17) into the portal vein, thereby reducing the production of inflammatory factors such as tumor necrosis factor-α (SMD: –3.35, 95% CI: –4.31 to –2.38), interleukin-6 (SMD: –4.28, 95% CI: –6.13 to –2.43), and interleukin-1β (SMD: –4.28, 95% CI: –6.37 to –2.19). Oxidative stress was also relieved, as evidenced by decreased malondialdehyde levels (SMD: –4.70, 95% CI: –6.21 to –3.20). Superoxide dismutase (SMD: 2.65, 95% CI: 2.16–3.15) and glutathione levels (SMD: 3.80, 95% CI: 0.95–6.66) were elevated.
Conclusion: Microbial agents can reverse dysbiosis in ALD, thus significantly interfering with lipid metabolism, relieving inflammatory response and inhibiting oxidative stress to improve liver function.
Introduction
Alcoholic liver disease (ALD) is a spectrum of diseases, including steatohepatitis, alcoholic hepatitis, cirrhosis, and associated complications, which in severe cases can progress to liver failure and lead to multi-system dysfunction (1). The dynamics of ALD involve interactions between the direct toxicity of alcohol and its metabolites, oxidative stress, inflammatory cascades, and other complex alcohol-related consequences (2). The optimal management strategy for ALD is still debated due to the limited efficacy of current treatments (3).
Several lines of evidence suggested a link between intestinal flora and liver diseases. The overgrowth of gram-negative bacteria and subsequently elevated gut-derived endotoxin were found in patients with ALD, participating in the pathogenesis of ALD (4). Chronic heavy alcohol consumption damages the intestinal epithelial barrier. Consequently, it increases intestinal permeability, allowing intestinal endotoxin to enter the portal circulation and increasing the production of pro-inflammatory cytokine IL-1β, TNF-α, and IL-6 through the activated toll-like receptor (TLR) 4-nuclear factor kappa B (NF-κ B) pathway (5).
Probiotics are living microorganisms that offer health benefits to the host the host health benefits, whereas prebiotics is non-digestible food ingredients that selectively stimulate the growth or activity of probiotics (6, 7). Lactobacillus rhamnoses GG helped prevent chronic alcohol exposure-induced hepatic steatosis by increasing hepatic AMPK phosphorylation and Bax-regulated apoptosis (8). In a mouse model of ALD, the probiotic Akkermansia muciniphilak reinforces the gut vascular barrier (GVB) and thus protects against alcohol-induced liver damage (9). Most probiotics targeting ALD attenuate the barrier disruption caused by ethanol exposure (as seen by a reduction in intestinal leakiness) and restore tight junction protein expression as well as the thickness of the mucus layer (10). Bifidobacterium bifidum and Lactobacillus plantarum 8PA3 restore the normal intestinal flora in ALD, significantly enhancing liver function by mitigating liver-specific bio-enzymatic values (11). Prebiotic fructo-oligosaccharides increased the abundance of beneficial bacteria such as Lactobacillus and Bifidobacterium and improved alcoholic steatohepatitis (12). Pectin, as a prebiotic, restored intestinal homeostasis in mice with ALD, increasing the number of cupped cells and the expression of defensins Reg3β and Reg3γ (13). One study found that treatment by fecal transplantation from prebiotic (pectin)-fed mice prevented ALD (14).
Nevertheless, due to the diversity of microbial agents, the therapeutic effects of microbes on ALD have not been comprehensively described. In the present study, we collected data from published clinical research and preclinical studies and systematically assessed serum biochemical parameters, serum inflammatory parameters, and blood lipids to determine the effectiveness of microbial agents in ALD treatment. We also screened studies foroxidative stress parameters and intestinal barrier function to elucidate the underlying mechanisms.
Materials and methods
Literature search
Meta-analysis was conducted according to the Preferred Reporting Items for Systematic Reviews and Meta-Analysis (PRISMA) guidelines (15). PubMed, the Cochrane Library, and Embase were searched for studies in English up to November 2021. The search strategy was devised using medical subject headings and synonyms, as follows: (“prebiotics” OR “yogurt” OR “inulin” OR “oligosaccharide” OR “galactose oligosaccharide” OR “fructose oligosaccharide” OR “probiotics” OR “Lactobacillus” OR “Bifidobacterium” OR “Enterococcus” OR “Streptococcus” OR “Saccharomyces” AND disease “liver injury” OR “alcohol-induced liver injury” OR “Alcoholic liver disease”). After filtering the titles, abstracts, full texts and eliminating duplicates, appropriate studies were retained if they matched the inclusion criteria. Two reviewers carried out these screenings independently, and disagreements were resolved by discussion and consensus.
Study selection
The literature included basic animal experiments and clinical trials. Animal experiments followed the PICOS principles (i.e., participants, interventions, comparisons, outcomes, and study design). Participants (P): Animal models of ALD, usually shaped by feeding with large amounts of alcohol. Intervention (I): The intervention group received microbial preparations, including probiotics and prebiotics. Comparison (C): the comparison group uses no microbial agents. Outcome (O): outcome indicators include several key components: (1) liver enzymes, alkaline phosphatase, γ-glutamyl transpeptidase (GGT); (2) blood lipids like triglyceride (TG), total cholesterol (TC); (3) inflammatory indicators such as TNF-α, IL-6, IL-10, endotoxin, malondialdehyde (MDA), superoxide dismutase (SOD). Study design (S): randomized controlled studies. The inclusion criteria for clinical trials were similar to those for animal trials Cohort studies, case-control studies, and cross-sectional studies were excluded during the selection process.
Quality evaluation and data extraction
We included preclinical and clinical studies. For preclinical studies, methodological quality was assessed according to the Systematic Risk of Bias Evaluation Center for Laboratory Animal Experiments (SYRCLE) tool (16). Clinical studies were assessed using the Cochrane Risk Assessment Scale in the Cochrane Handbook. All experimental data were extracted independently and cross-checked by two authors. Graphical data were generated using Get Data software. The following information was extracted: (1) first author and publication year; (2) participant characteristics. It should be noted that, for animal experiments, we recorded animal species, modeling approach, and sample size. We recorded the age, the number of participants, and nationality for clinical experiments. (3) route of administration, dosage, and duration of treatment; (4) outcome variables. Discrepant opinions were resolved through third-party discussions.
Statistical analysis
The 95% confidence interval (CI) and standard deviation of the combined mean difference were used to determine differences in continuous variables. We also used the Cochran Q-test and I2 statistic (I2 < 25%, low heterogeneity; 25–50%, moderate heterogeneity; I2 > 50%, high heterogeneity). Because animal studies and clinical trials are exploratory, a random effects model was used. A statistically significant difference was defined as p < 0.05. Seventeen indicators were analyzed in subgroups to explore the sources of heterogeneity based on the type of microbes, strains, animal models, and modeling approaches. A meta-regression was conducted to determine potential heterogeneity origins. A sensitivity analysis was conducted to identify studies that significantly influenced the results by eliminating them one by one. Publication bias was estimated quantitatively using Egger’s test. Contour-enhanced funnel plots obtained using the trim-and-fill method help to distinguish asymmetries caused by publication bias or heterogeneity, among other factors (17). If the missing study is in a non-significant region, the asymmetry is attributable to publication bias. Alternatively, the observed asymmetry could be attributed to factors other than publication bias. The statistical analysis was performed using R and R Studio software.
Results
Identification of relevant studies
The flow diagram of this meta-analysis is displayed in Figure 1. A total of 9,858 records were initially obtained from the three databases, of which 3,484 were removed due to duplication. The initial screening of titles and abstracts yielded 87 articles after excluding 6,287 studies. A further 41 articles were rejected based on a detailed full-text evaluation. There were 41 studies (including 37 animal studies and four clinical studies) that met the inclusion criteria after a thorough screening of the full text (4, 11, 18–56).
Study characteristics and quality assessment
Tables 1, 2 display the characteristics of the 41 studies. All animal experiments were carried out using rodent models, mainly C57BL/6N mice and Wistar rats, the microbial agents in the intervention group were primarily probiotics (mostly Lactobacillus), and placebos were usually used in the control groups. In the clinical studies, subjects were patients of various nationalities with alcoholic hepatitis and cirrhosis caused by chronic heavy alcohol consumption.
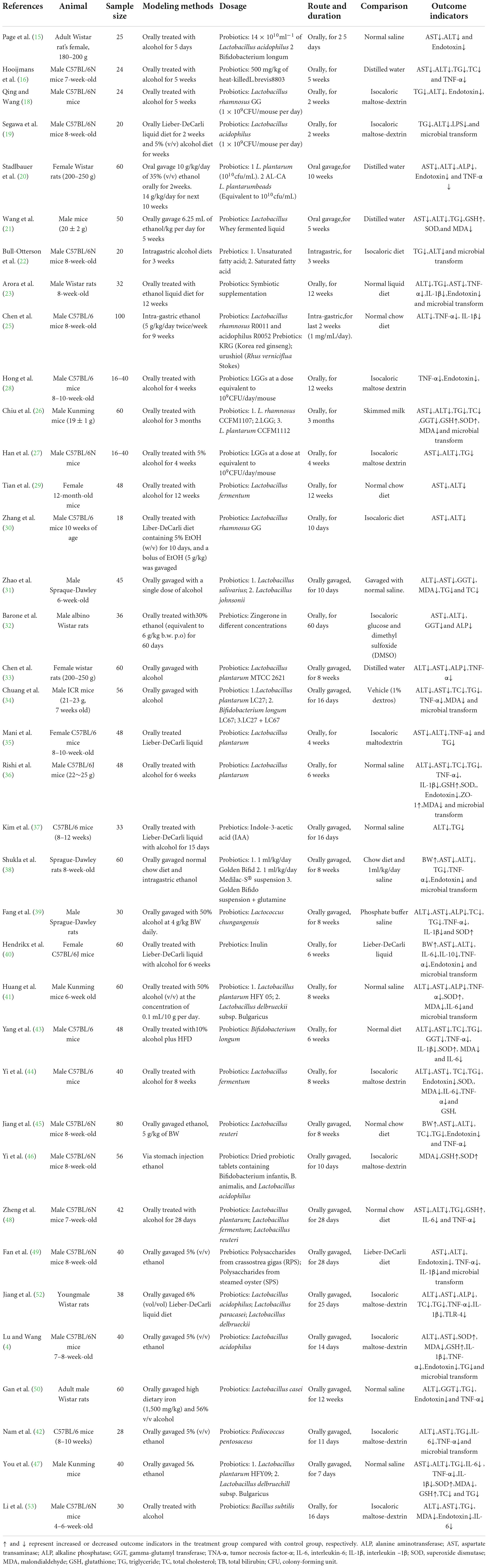
Table 1. Characteristics of included studies investigating the effects of probiotics and prebiotics on animal ALD.
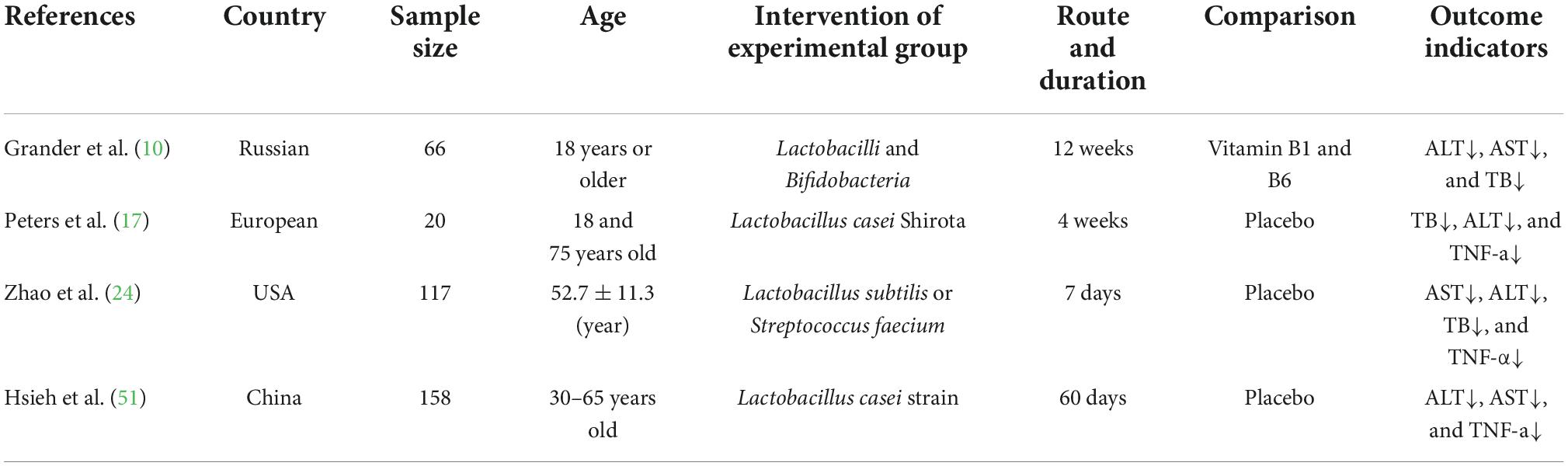
Table 2. Characteristics of included studies investigating the effects of probiotics and prebiotics on human ALD.
Two reviewers independently assessed method quality using the SYRCLE tool. Blinding caused most biases. The items were judged as low, unclear, or high risks. In most studies, blinding and allocation bias were unclear because no specific details of relevant information were provided. All studies were rated as having a low risk of reporting bias. Overall, the studies had similar high-quality evaluations with a low risk of bias (Supplementary Tables 1, 2). Differences in the quality evaluation process were addressed through discussion.
Effect of microbial agents on lipid control
Microbial therapy has a moderating effect on hyperlipemia, as indicated by a more dampened level of TC (SMD = -2.75, 95% CI –4.03 to –1.46, I2 = 81%) and TG (SMD = –2.64, 95% CI –3.22 to –2.06, I2 = 69%) (Figure 2). Subgroup analysis showed that biopsy tissue and mouse species are responsible for high TG heterogeneity (Supplementary Table 3). The contour-enhanced funnel plot with the trim-and-fill method indicates that publication bias was not the leading cause of asymmetry (Figure 3). Sensitivity analysis confirmed the robustness of the study. There were no clinical studies. About the effect of microbial agents on lipid control.
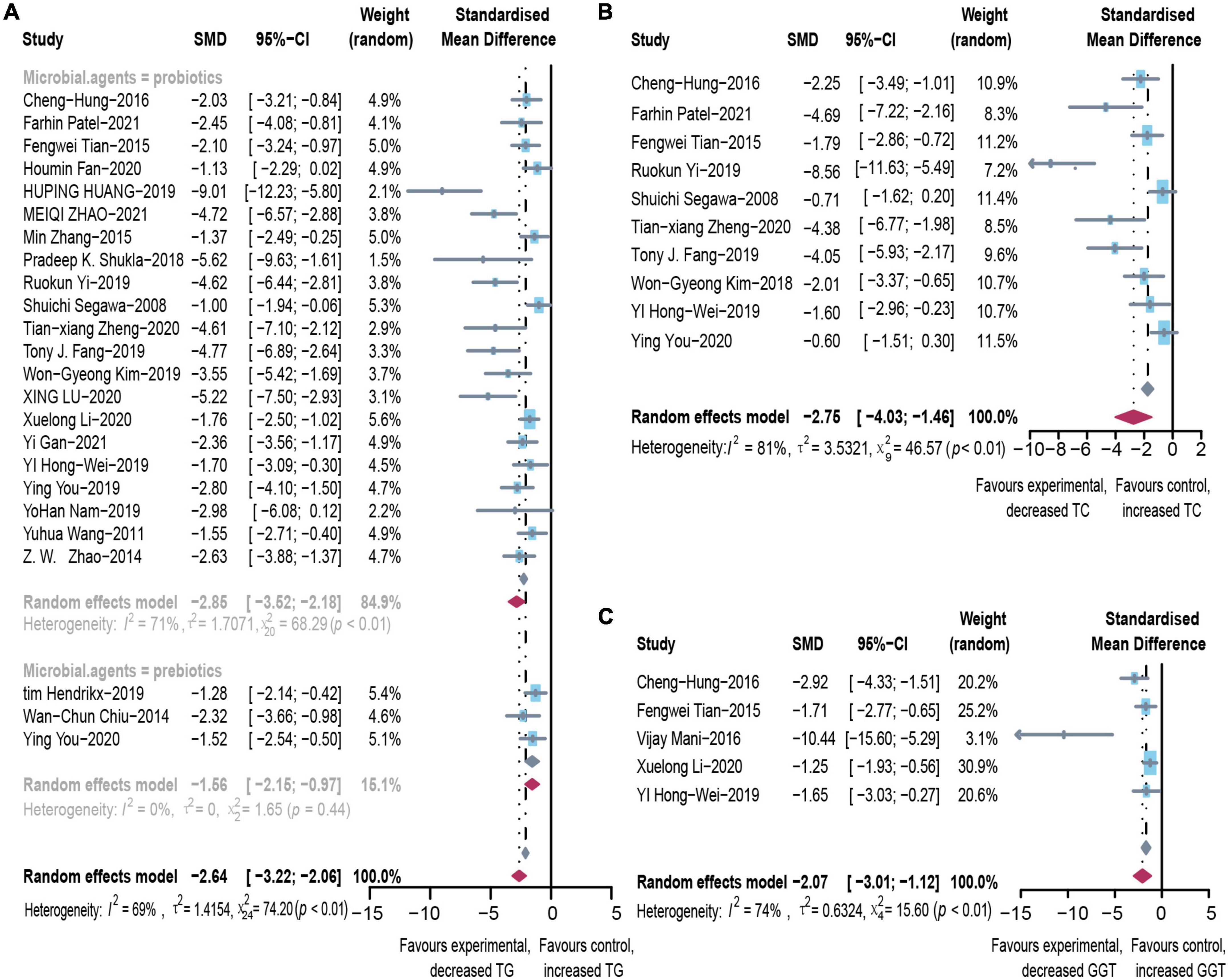
Figure 2. Effectiveness of microbial agents on lipid index. (A) The effect of microbial agents on TG, (B) TC. (C) The effect of microbial agents on GGT. SMD, Standardized mean difference; CI, Confidence interval.
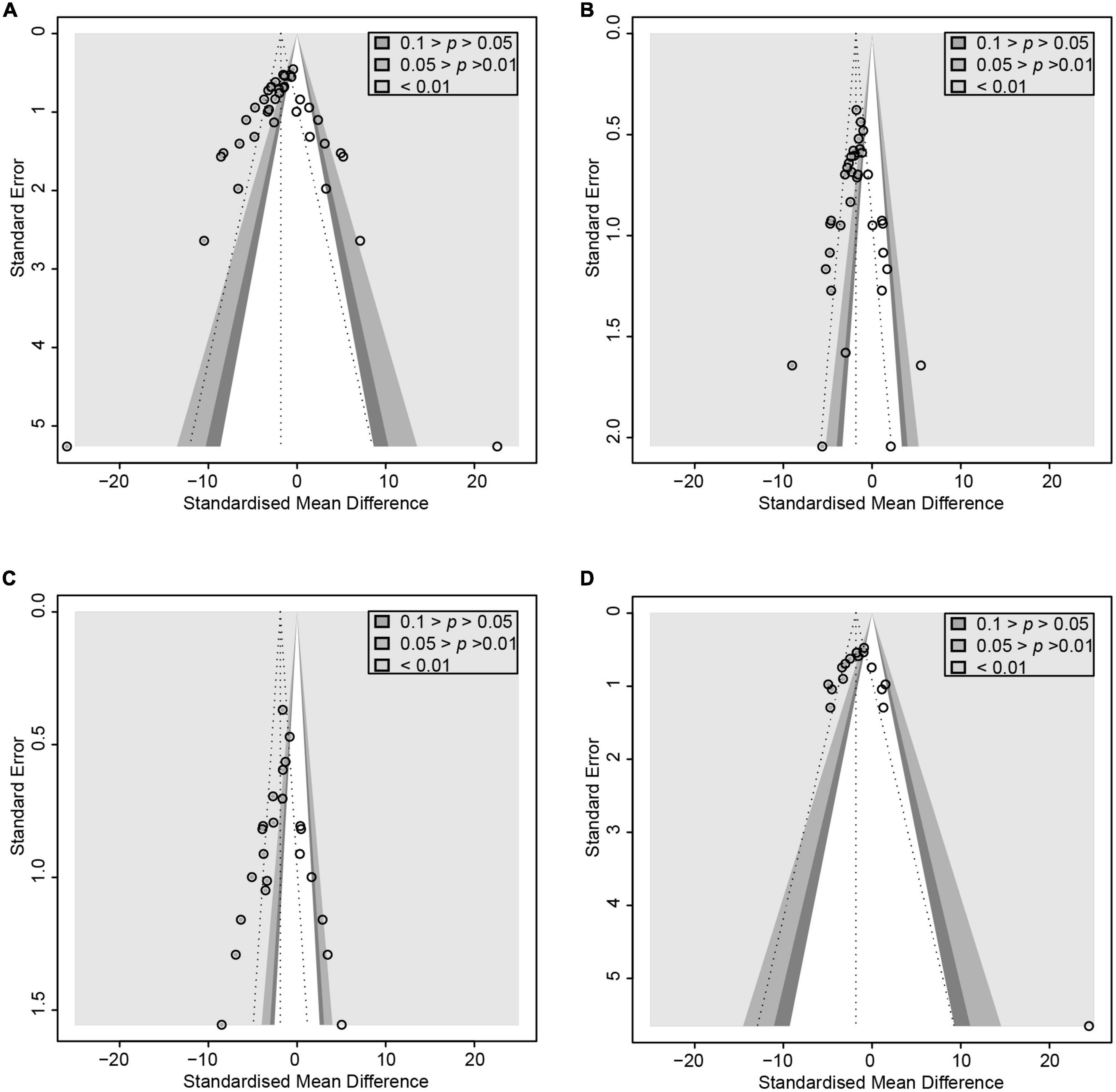
Figure 3. Contour-enhanced funnel plot with trim-and-fill method. (A) ALT. (B) TG (C) TNF-α (D) Endotoxin. If the missing studies were in the non-significant area, the asymmetry was due to publication bias. Otherwise, the observed asymmetry could be attributed to factors other than publication bias.
Effect of microbial agents on liver biochemical indicators
After pooled analysis of the data, there were significant differences in ALT (SMD: –2.70, 95% CI: –3.33 to –2.07, I2 = 78%), AST (SMD: –3.37, 95% CI: –4.25 to –2.49, I2 = 82%) and alkaline phosphatase (SMD: –2.12, 95% CI: –3.32 to –0.92, I2 = 66%), between the experimental and control groups (Figure 4). In addition, GGT (SMD: –1.8, 95% CI: –2.39 to –1.24, I2 = 68%), which is more specific to ALD, has also been addressed (Figure 2). Because of significant heterogeneity, the reasons for these differences were investigated by conducting subgroup analyses (Supplementary Table 4). The heterogeneity of ALT was slightly altered after considering probiotics and prebiotics separately; however, the heterogeneity changed more significantly when the variables were controlled for the animal model, flora type, and feeding pattern. This finding suggested that the more significant heterogeneity may be due to these factors. There was an inconspicuous asymmetry in the contour-enhanced funnel plot. The trim-and-fill method demonstrated that the asymmetry was caused by factors other than publication bias (Figure 3).
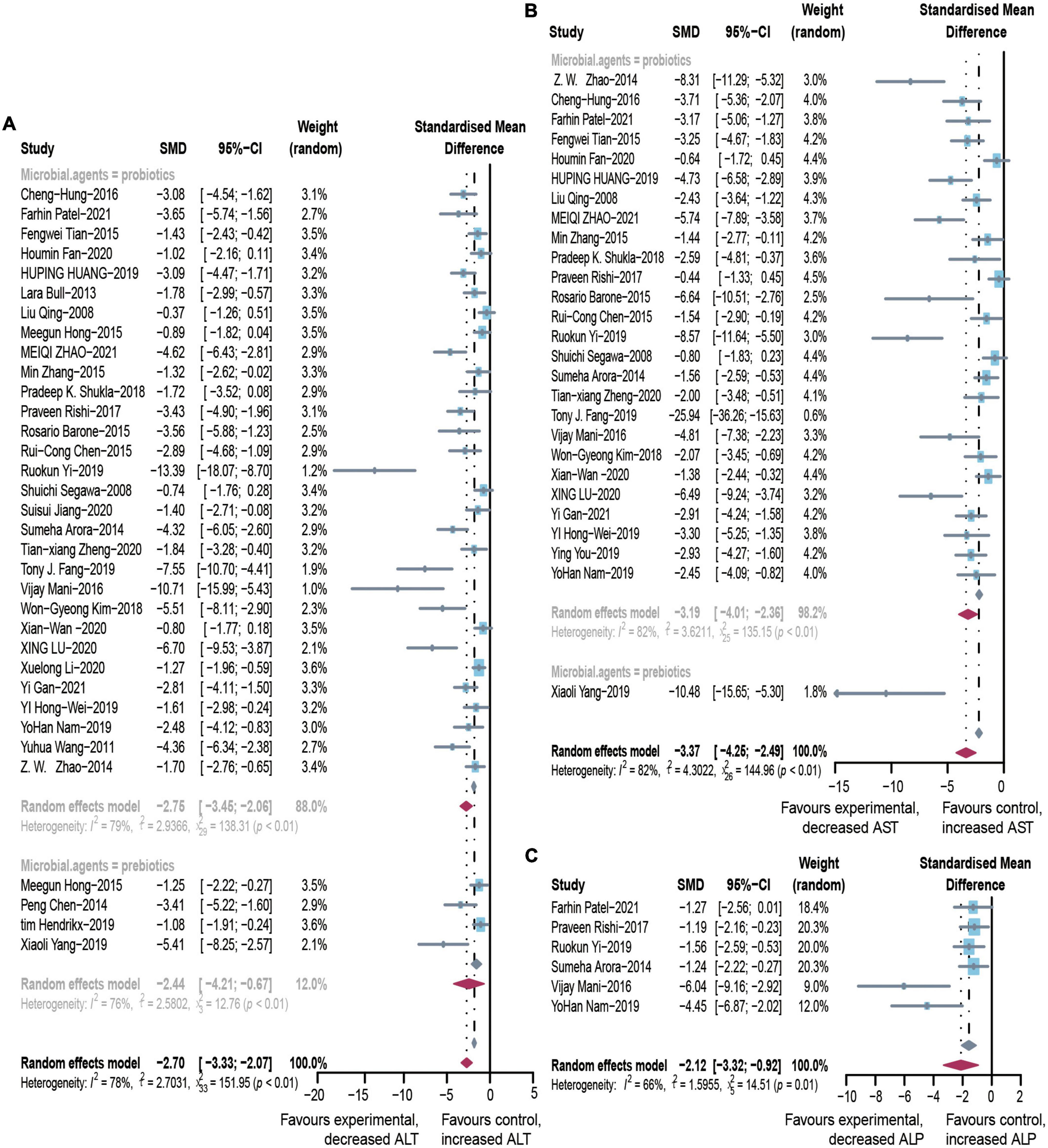
Figure 4. Effectiveness of microbial agents on liver biochemical index. (A) The effect of microbial agents on ALT, (B) AST, and (C) ALP. SMD, Standardized mean difference; CI, Confidence interval.
There were many reports investigating the role of probiotics in experimental ALD. Clinical studies were rare, but we have conducted a careful analysis, according to the clinical study data we have obtained: ALT (SMD: –0.95, 95% CI: –0.40 to –1.1, I2 = 69%), AST (SMD: –1.4, 95% CI: –3.2 to –0.4, I2 = 97%), and GGT (SMD: –0.63, 95% CI: –1.07 to –0.20, I2 = 70%) decreased significantly compared to the control group (Supplementary Figure 2).
Effect of microbial agents on inflammation mediators
In animal studies, TNF-α, IL-6, and IL-1β were used to assess inflammatory infiltration due to ALD (Figure 5). There were lower levels of TNF-α (SMD: –3.35, 95% CI: –4.31 to –2.38, I2 = 81%), IL-6 (SMD: –4.28, 95% CI: –6.13 to –2.43, I2 = 84%), and IL-1β (SMD: –4.28, 95% CI: –6.37 to –2.19, I2 = 87%), with evident heterogeneity. The analysis of subgroups was based on three items including animal models, tissues, and routes. The heterogeneity changed markedly among the animal models, suggesting that differences in animal species may be responsible for heterogeneity (Supplementary Table 5). The pooled analysis of TNF-α in serum and liver suggests that different tissues might not be the source of the heterogeneity. Egger’s test indicated publication bias and profile-enhanced funnel plots (drawn using the trim-and-fill method; Figure 3) showed that publication bias was not the leading cause of asymmetry. Sensitivity analysis revealed that no studies interfered significantly with the meta-analysis, implying good stability.
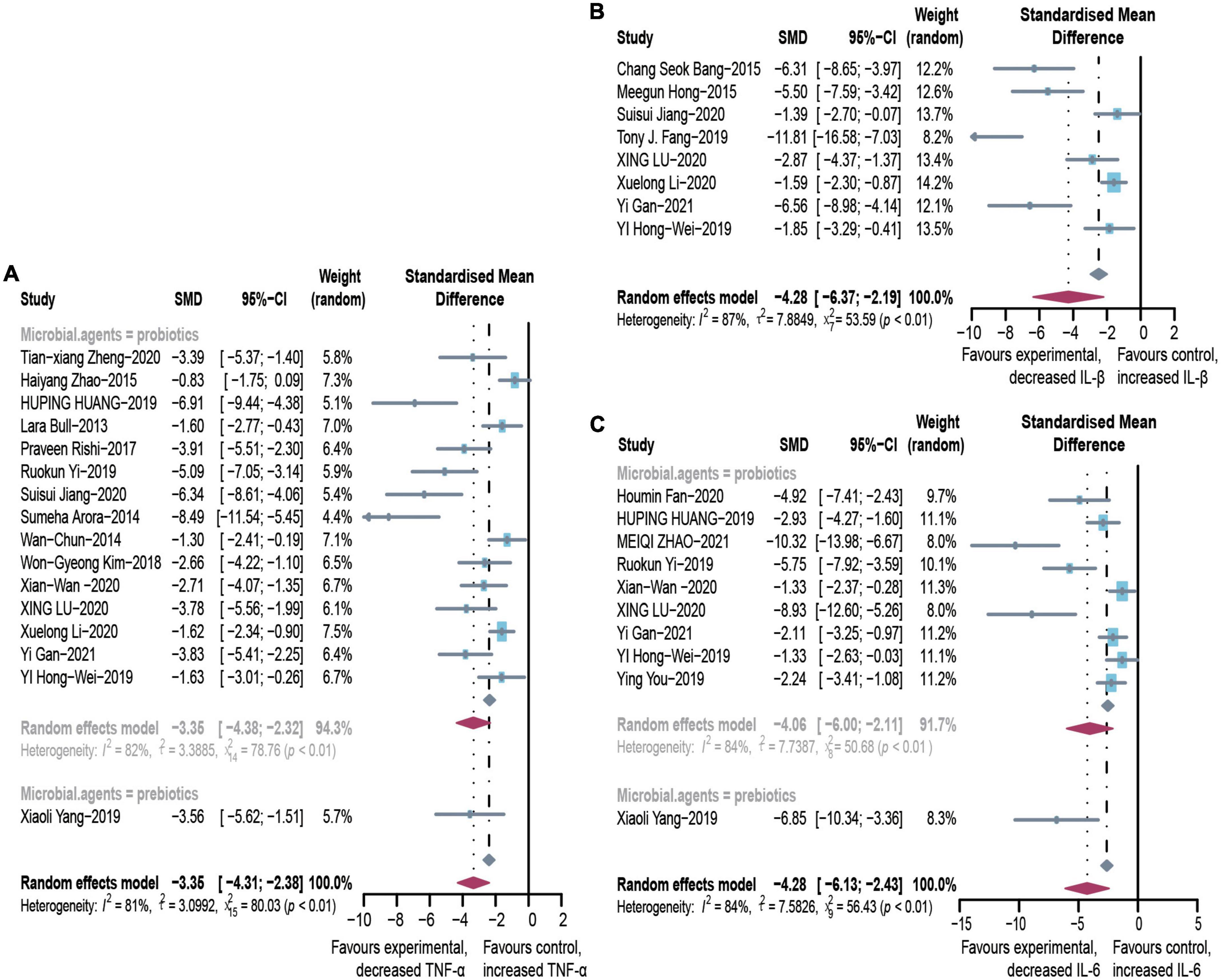
Figure 5. Effectiveness of microbial agents on inflammatory cytokines. (A) The effect of microbial agents on TNF-α. (B) The effect of microbial agents on IL-1β. (C) The effect of microbial agents on IL-6. SMD, Standardized mean difference; CI, Confidence interval.
Due to the paucity of clinical literature on indicators of inflammation, we only performed a pooled analysis of TNF-α (SMD: –1.7, 95% CI: –4.39 to 0.9, I2 = 89%). This finding suggests that probiotics moderate inflammation (Supplementary Figure 2).
Effect of microbial agents on floral translocation and endotoxin
A comprehensive study of intestinal flora translocation and endotoxin was conducted to evaluate the changes of each in patients with ALD. Intestinal flora underwent dramatic changes in response to alcohol (Supplementary Figure 1), with most flora showing an upward trend, including Lactobacillus (SMD: 4.40, 95% CI: 0.97–7.84, I2 = 85%), Bifidobacteria (SMD: 3.84, 95% CI: 0.22–7.45, I2 = 88%), and Bacteroidetes (SMD: 2.51, 95% CI: 0.29–4.72, I2 = 80%). Proteobacteria proliferated even more (SMD: –4.18, 95% CI: –6.60 to –1.77, I2 = 86%). Interestingly, most proliferating bacteria were beneficial to the intestinal tract (e.g., Lactobacillus); however, this finding could be because the microbial preparations administered in the animal models were Lactobacillus. We also explored the variation in endotoxin (SMD: –2.70, 95% CI: –3.52 to –1.88, I2 = 79%). Contour-enhanced funnel plots using the trim-and-fill method showed that publication bias was not the primary cause of asymmetry (Figure 3). The robustness of the results was demonstrated by sensitivity analysis. There were no clinical studies investigating floral translocation and endotoxin.
Effect of microbial agents on oxidative stress
To evaluate the free radical-mediated lipid peroxidation damage and the antioxidant status of tissues, we measured levels of glutathione (GSH), SOD, and MDA (Figure 6). Microbial agent treatment contributed to increased levels of SOD (SMD: 2.65, 95% CI: 2.16–3.15, I2 = 44%) and GSH (SMD: 3.80, 95% CI: 0.95–6.66, I2 = 87%), while there was a significant decrease in MDA (SMD: –4.70, 95% CI: –6.21 to –3.20, I2 = 83%) with considerable heterogeneity. We performed a subgroup analysis of MDA according to mice models and feeding practices and obtained no meaningful results. Asymmetry was present in the contour-enhanced funnel plot (Figure 3), demonstrating that publication bias was not the leading cause of asymmetry. There were no clinical studies.
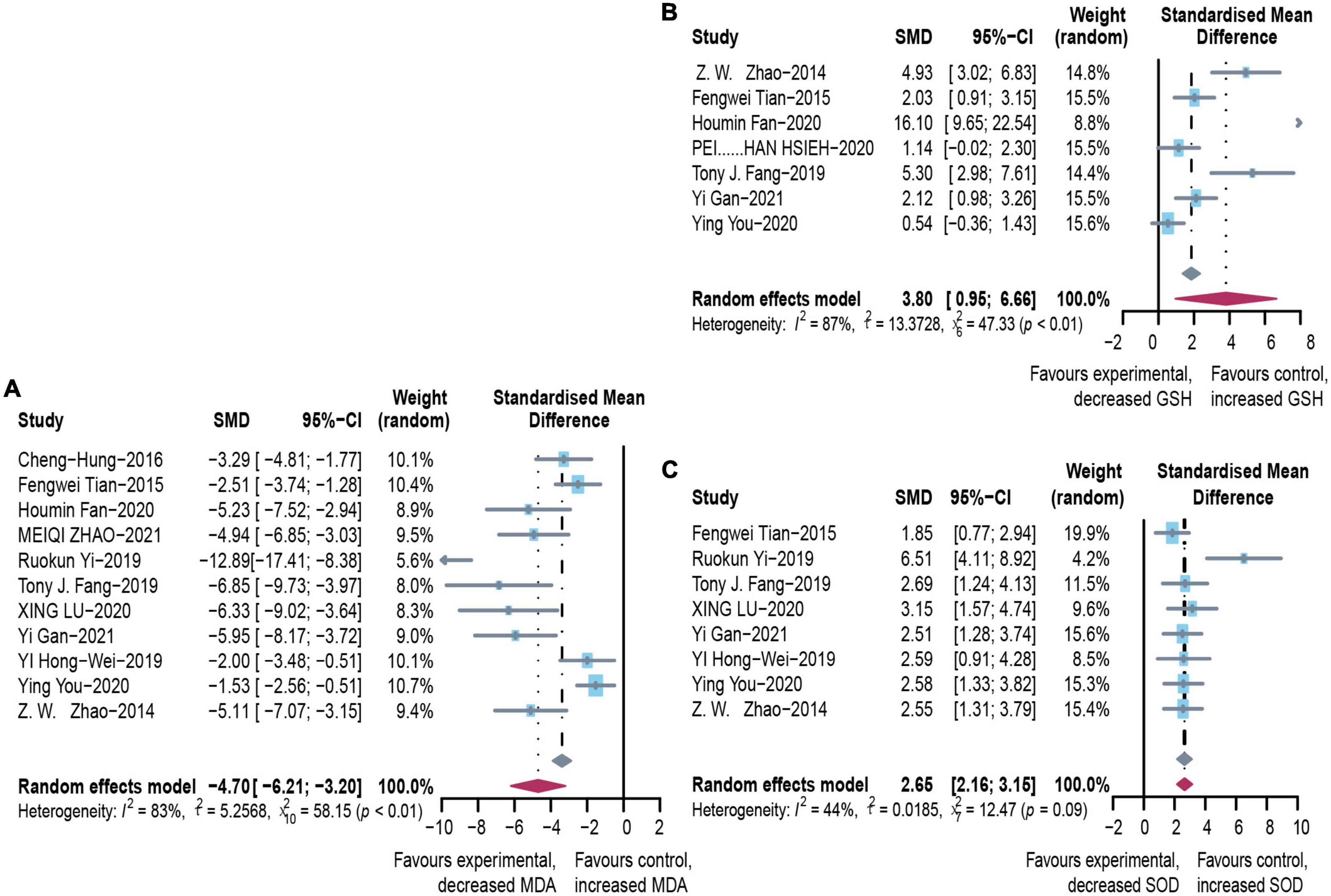
Figure 6. Effectiveness of microbial agents on oxidative stress. (A) The effect of microbial agents on MDA to evaluate free radical mediated lipid peroxidation injury. (B,C) The effect of microbial agents on GSH and SOD to evaluate antioxidant status of tissues. SMD, Standardized mean difference; CI, Confidence interval.
Discussion
We identified convincing evidence for the use of microbial treatment of ALD through careful analysis of clinical trials and animal studies. To our knowledge, this is the first meta-analysis of such therapies. Although alcohol is primarily metabolized in the liver, alcohol consumption causes ecological dysregulation of bacteria in the intestine, damage to the intestinal mucosa, and increased intestinal permeability, resulting in increased transport of bacteria and their products (e.g., endotoxins) into the portal circulation. The increased inflammatory cytokine levels during liver injury reach the intestine through the circulation to damage the intestinal mucosal barrier. This phenomenon disrupts the balance of the intestinal flora, creating a vicious cycle (57). Once the dynamic balance is disturbed under the onslaught of pathogenic factors, intestinal and hepatic dysfunction is triggered. Fortunately, probiotics and prebiotics can effectively maintain intestinal homeostasis (58).
Lactobacillus and Bifidobacterium are the most predominant antimicrobial genera among probiotics. Most studies chose probiotics containing Lactobacillus, Bifidobacterium, or a mixture of the two. These beneficial bacteria compete with pathogenic bacteria for binding sites in the intestinal epithelium, and they effectively reduce the pathogenic microorganisms by releasing antibacterial substances such as lactic acid and hydrogen peroxide (59). We found that, after the probiotic intervention, the dysbiosis in ALD mice improved with increased abundance of Bifidobacterium, decreased Proteobacteria, and a corresponding decrease in the rate of intestinal infections was also observed. Bifidobacteria and Lactobacilli are thought to promote mucosal immunity through the intestinal microbiota (60). Other potentially beneficial flora such as Lachnospiraceae might promote intestinal mucosal integrity through the metabolite butyrate, a short-chain fatty acid (61). Our findings demonstrated that the intestinal epithelial barrier (IEB) was more consolidated than the model groups, suggesting that probiotics and prebiotics can reinforce each other and work together to maintain intestinal immunity (62).
In addition to active modification of the intestinal flora, studies reported that probiotics protect the intestine and liver from alcohol stimulation by regulating the synthesis, catabolism and lipid transport, mitigating oxidative stress, and reinforcing the IEB (63). Sterol regulatory element binding proteins (SREBPs) are transcriptional mediators of lipid homeostasis that are upregulated in response to alcohol abuse, raising hepatic steatosis and plasma TG levels. SREBP-1c is the primary regulator of hepatic fatty acid and TG synthesis, and SREBP-2 regulates cholesterol synthesis (64). Alcohol reduces the expression of PPAR-α and MTP, critical participants in the transfer of TG and TC in the liver, leading to increased lipid accumulation (65). Probiotics inhibit weight gain, epiphyseal adipose tissue expansion, and partially reverse fructose (FRD)-induced adipocyte hypertrophy (66). Probiotics prevent the elevation of plasma triglycerides, leptin, and hepatic TG levels (67). Prebiotic fermentation products increased the production of hepatic mucin and modulated the action of hepatic lipogenic enzymes. Our findings suggest a significant decrease in TG and TC in the liver and serum compared to the model group (68).
Alcohol-induced inflammatory reactions should also be considered in the liver and throughout the body. The oxidative pathway of alcohol metabolism mediated by ethanol dehydrogenase and acetaldehyde dehydrogenase produces large amounts of acetaldehyde, which is thought to be the primary mediator of alcohol toxicity in the liver (69). Excess acetaldehyde displaces the intestinal flora and damages the intestinal mucosal barrier (70). Intestinal bacteria-derived endotoxins function through pattern recognition receptors such as TLRs, expressed in hepatic cells such as Kupffer cells. Lipopolysaccharide-induced inflammation boosts inflammatory cytokines, including TNF-α and IL-1β, which stimulate Stimulates NF-κB activation through the MAPK (mitogen-activated protein kinase) pathway, and the activated NF-κB enters the nucleus, forming a cytokine-NF-κB loop and causing a series of inflammatory responses in the cells (71). Consistent with these findings, we found that human trials and animal studies showed a considerable increase in inflammatory parameters in ALD model compared to the control group.
In the inflammatory cascade, unsaturated fatty acids are driven by reactive oxygen species (ROS) to produce lipid peroxidases, which trigger fatty acid side chain reactions. Oxidative metabolites of ethanol, such as acetaldehyde and ROS, play essential roles in the clinical and pathological spectra ALD’s. At the same time, excess acetaldehyde entering the bloodstream is converted to superoxide by p-xanthine oxidase, producing MDA, the end product of free radical-mediated lipid peroxidation, which is used as a marker of oxidative stress (72). The antioxidants SOD and GSH reflect antioxidant levels. Ethanol-induced oxidative stress damage was confirmed by the decreased levels of SOD and GSH and the high content of MDA in alcohol-fed mice (73). The presence of microorganisms not only ameliorates oxidative stress by suppressing ROS and significantly reducing cytokine levels by inhibiting TLR-mediated endotoxins (21). Our findings showed that probiotics or prebiotics could reduce MDA levels by inhibiting the inflammatory response and the oxidative effect of alcohol. Meanwhile, there were increased concentrations of the antioxidants SOD and GSH.
The toxic effects of alcohol on the liver are mediated by interfering with lipid metabolism, disrupting the mucosal barrier, enhancing the inflammatory response and promoting oxidative stress. In contrast, microbial treatment can lead to significant changes in liver-specific biological enzymes. In clinical trials, we could observe a greater decrease in AST, ALT, and GGT compared to the control group. Interestingly, In patients with alcoholic liver disease, elevations in AST were more pronounced than in ALT and serum AST concentrations are usually more than twice as high as ALT because alcohol induces mitochondrial dysfunction through activation of the CYP2E1 enzyme and because of the massive release of AST from the mitochondrial matrix (74). And in animal experiments we obtained the same results, which fully illustrates the incredible effect of microbial treatment for ALD.
Our meta-analysis of 41 studies showed that microbial agents could help to treat ALD; nevertheless, there were still some limitations. First, due to the exploratory nature of this study, heterogeneity was inevitable when combining specific indicators, even when using random effects models and subgroup analysis. Nevertheless, sensitivity analysis supported the robustness of our results. Asymmetry appeared in the funnel plot and Egger’s test, indicating publication bias. Contour-enhanced funnel plots using the trim-and-fill method demonstrated that heterogeneity was the primary cause of asymmetry. In addition, although the number of clinical studies is limited and the data used for statistical analysis is small, we have conducted a careful analysis to summaries while hoping that more clinical studies will be available to support our conclusion. In this light, the conclusions we drew from 41 articles remain valid.
Conclusion
Prebiotics and probiotics exert hepatoprotective effects by regulating intestinal flora, maintaining the integrity of the intestinal mucosa, reducing the entry of endotoxins released by pathogenic microorganisms into the portal system, and inhibiting oxidative stress as well as pro-inflammatory factors. Our study provides new insights into the management of ALD. Nevertheless, clinical studies are still needed to translate microbial therapy into practical clinical applications.
Data availability statement
The original contributions presented in this study are included in the article/Supplementary material, further inquiries can be directed to the corresponding author/s.
Author contributions
ZX conceived the idea and designed the study strategy. QW, JS, and MZ conducted reference search. QW and CX summarized the data. QW, GR, YX, and OY drafted the manuscript. QW conducted data acquisition and statistical analyses. FW provided critical revisions of the manuscript for important intellectual content, administrative and funding support, and supervision. All authors contributed to the article and approved the submitted version.
Funding
This work was funded by the Natural Science Foundation of Zhejiang Province (LY20H180010) and Zhejiang Medical Association (2022ZYC-A14).
Conflict of interest
The authors declare that the research was conducted in the absence of any commercial or financial relationships that could be construed as a potential conflict of interest.
Publisher’s note
All claims expressed in this article are solely those of the authors and do not necessarily represent those of their affiliated organizations, or those of the publisher, the editors and the reviewers. Any product that may be evaluated in this article, or claim that may be made by its manufacturer, is not guaranteed or endorsed by the publisher.
Supplementary material
The Supplementary Material for this article can be found online at: https://www.frontiersin.org/articles/10.3389/fnut.2022.1054265/full#supplementary-material
References
1. Singal AK, Bataller R, Ahn J, Kamath PS, Shah VH. ACG clinical guideline: alcoholic liver disease. Am J Gastroenterol. (2018) 113:175–94. doi: 10.1038/ajg.2017.469
2. Nibourg GA, Chamuleau RA, van der Hoeven TV, Maas MA, Ruiter AF, Lamers WH, et al. Liver progenitor cell line HepaRG differentiated in a bioartificial liver effectively supplies liver support to rats with acute liver failure. PLoS One. (2012) 7:e38778. doi: 10.1371/journal.pone.0038778
3. Ki SH, Park O, Zheng M, Morales-Ibanez O, Kolls JK, Bataller R, et al. Interleukin-22 treatment ameliorates alcoholic liver injury in a murine model of chronic-binge ethanol feeding: role of signal transducer and activator of transcription 3. Hepatology. (2010) 52:1291–300. doi: 10.1002/hep.23837
4. Lu X, Wang F. Lactobacillus acidophilus and vitamin C attenuate ethanol-induced intestinal and liver injury in mice. Exp Therap Med. (2021) 22:1005. doi: 10.3892/etm.2021.10438
5. Chen P, Stärkel P, Turner JR, Ho SB, Schnabl B. Dysbiosis-induced intestinal inflammation activates tumor necrosis factor receptor I and mediates alcoholic liver disease in mice. Hepatology. (2015) 61:883–94. doi: 10.1002/hep.27489
6. Milosevic I, Vujovic A, Barac A, Djelic M, Korac M, Radovanovic Spurnic A, et al. Gut-liver axis, gut microbiota, and its modulation in the management of liver diseases: a review of the literature. Int J Mol Sci. (2019) 20:395. doi: 10.3390/ijms20020395
7. Catry E, Bindels LB, Tailleux A, Lestavel S, Neyrinck AM, Goossens JF, et al. Targeting the gut microbiota with inulin-type fructans: preclinical demonstration of a novel approach in the management of endothelial dysfunction. Gut. (2018) 67:271–83. doi: 10.1136/gutjnl-2016-313316
8. Forsyth CB, Farhadi A, Jakate SM, Tang Y, Shaikh M, Keshavarzian A. Lactobacillus GG treatment ameliorates alcohol-induced intestinal oxidative stress, gut leakiness, and liver injury in a rat model of alcoholic steatohepatitis. Alcohol. (2009) 43:163–72. doi: 10.1016/j.alcohol.2008.12.009
9. Grander C, Grabherr F, Spadoni I, Enrich B, Oberhuber G, Rescigno M, et al. The role of gut vascular barrier in experimental alcoholic liver disease and A. muciniphila supplementation. Gut Microbes. (2020) 12:1851986. doi: 10.1080/19490976.2020.1851986
10. Grander C, Adolph TE, Wieser V, Lowe P, Wrzosek L, Gyongyosi B, et al. Recovery of ethanol-induced Akkermansia muciniphila depletion ameliorates alcoholic liver disease. Gut. (2018) 67:891–901. doi: 10.1136/gutjnl-2016-313432
11. Kirpich IA, Solovieva NV, Leikhter SN, Shidakova NA, Lebedeva OV, Sidorov PI, et al. Probiotics restore bowel flora and improve liver enzymes in human alcohol-induced liver injury: a pilot study. Alcohol. (2008) 42:675–82. doi: 10.1016/j.alcohol.2008.08.006
12. Yan AW, Fouts DE, Brandl J, Stärkel P, Torralba M, Schott E, et al. Enteric dysbiosis associated with a mouse model of alcoholic liver disease. Hepatology. (2011) 53:96–105. doi: 10.1002/hep.24018
13. Ferrere G, Wrzosek L, Cailleux F, Turpin W, Puchois V, Spatz M, et al. Fecal microbiota manipulation prevents dysbiosis and alcohol-induced liver injury in mice. J Hepatol. (2017) 66:806–15. doi: 10.1016/j.jhep.2016.11.008
14. Wang Q, Li Y, Lv L, Jiang H, Yan R, Wang S, et al. Identification of a protective Bacteroides strain of alcoholic liver disease and its synergistic effect with pectin. Appl Microbiol Biotechnol. (2022) 106:3735–49. doi: 10.1007/s00253-022-11946-7
15. Page MJ, McKenzie JE, Bossuyt PM, Boutron I, Hoffmann TC, Mulrow CD, et al. The PRISMA 2020 statement: an updated guideline for reporting systematic reviews. BMJ. (2021) 372:n71. doi: 10.1136/bmj.n71
16. Hooijmans CR, Rovers MM, de Vries RB, Leenaars M, Ritskes-Hoitinga M, Langendam MW. SYRCLE’s risk of bias tool for animal studies. BMC Med Res Methodol. (2014) 14:43. doi: 10.1186/1471-2288-14-43
17. Peters JL, Sutton AJ, Jones DR, Abrams KR, Rushton L. Contour-enhanced meta-analysis funnel plots help distinguish publication bias from other causes of asymmetry. J Clin Epidemiol. (2008) 61:991–6. doi: 10.1016/j.jclinepi.2007.11.010
18. Qing L, Wang T. Lactic acid bacteria prevent alcohol-induced steatohepatitis in rats by acting on the pathways of alcohol metabolism. Clin Exp Med. (2008) 8:187–91. doi: 10.1007/s10238-008-0002-4
19. Segawa S, Wakita Y, Hirata H, Watari J. Oral administration of heat-killed Lactobacillus brevis SBC8803 ameliorates alcoholic liver disease in ethanol-containing diet-fed C57BL/6N mice. Int J Food Microbiol. (2008) 128:371–7. doi: 10.1016/j.ijfoodmicro.2008.09.023
20. Stadlbauer V, Mookerjee RP, Hodges S, Wright GA, Davies NA, Jalan R. Effect of probiotic treatment on deranged neutrophil function and cytokine responses in patients with compensated alcoholic cirrhosis. J Hepatol. (2008) 48:945−51. doi: 10.1016/j.jhep.2008.02.015
21. Wang Y, Kirpich I, Liu Y, Ma Z, Barve S, McClain CJ, et al. Lactobacillus rhamnosus GG treatment potentiates intestinal hypoxia-inducible factor, promotes intestinal integrity and ameliorates alcohol-induced liver injury. Am J Pathol. (2011) 179:2866–75. doi: 10.1016/j.ajpath.2011.08.039
22. Bull-Otterson L, Feng W, Kirpich I, Wang Y, Qin X, Liu Y, et al. Metagenomic analyses of alcohol induced pathogenic alterations in the intestinal microbiome and the effect of Lactobacillus rhamnosus GG treatment. PLoS One. (2013) 8:e53028. doi: 10.1371/journal.pone.0053028
23. Arora S, Kaur IP, Chopra K, Rishi P. Efficiency of double layered microencapsulated probiotic to modulate proinflammatory molecular markers for the management of alcoholic liver disease. Mediators Inflamm. (2014) 2014:715130. doi: 10.1155/2014/715130
24. Zhao ZW, Pan DD, Wu Z, Sun YY, Guo YX, Zeng XQ. Antialcoholic liver activity of whey fermented by Lactobacillus casei isolated from koumiss. J Dairy Sci. (2014) 97:4062–71. doi: 10.3168/jds.2014-7954
25. Chen P, Torralba M, Tan J, Embree M, Zengler K, Stärkel P, et al. Supplementation of saturated long-chain fatty acids maintains intestinal eubiosis and reduces ethanol-induced liver injury in mice. Gastroenterology. (2015) 148:203–14.e16. doi: 10.1053/j.gastro.2014.09.014
26. Chiu WC, Huang YL, Chen YL, Peng HC, Liao WH, Chuang HL, et al. Synbiotics reduce ethanol-induced hepatic steatosis and inflammation by improving intestinal permeability and microbiota in rats. Food Funct. (2015) 6:1692–700. doi: 10.1039/C5FO00104H
27. Han SH, Suk KT, Kim DJ, Kim MY, Baik SK, Kim YD, et al. Effects of probiotics (cultured Lactobacillus subtilis/Streptococcus faecium) in the treatment of alcoholic hepatitis: randomized-controlled multicenter study. Eur J Gastroenterol Hepatol. (2015) 27:1300–6. doi: 10.1097/MEG.0000000000000458
28. Hong M, Kim SW, Han SH, Kim DJ, Suk KT, Kim YS, et al. Probiotics (Lactobacillus rhamnosus R0011 and acidophilus R0052) reduce the expression of toll-like receptor 4 in mice with alcoholic liver disease. PLoS One. (2015) 10:e0117451. doi: 10.1371/journal.pone.0117451
29. Tian F, Chi F, Wang G, Liu X, Zhang Q, Chen Y, et al. Lactobacillus rhamnosus CCFM1107 treatment ameliorates alcohol-induced liver injury in a mouse model of chronic alcohol feeding. J Microbiol. (2015) 53:856–63. doi: 10.1007/s12275-015-5239-5
30. Zhang M, Wang C, Wang C, Zhao H, Zhao C, Chen Y, et al. Enhanced AMPK phosphorylation contributes to the beneficial effects of Lactobacillus rhamnosus GG supernatant on chronic-alcohol-induced fatty liver disease. J Nutr Biochem. (2015) 26:337–44. doi: 10.1016/j.jnutbio.2014.10.016
31. Zhao H, Zhao C, Dong Y, Zhang M, Wang Y, Li F, et al. Inhibition of miR122a by Lactobacillus rhamnosus GG culture supernatant increases intestinal occludin expression and protects mice from alcoholic liver disease. Toxicol Lett. (2015) 234:194–200. doi: 10.1016/j.toxlet.2015.03.002
32. Barone R, Rappa F, Macaluso F, Caruso Bavisotto C, Sangiorgi C, Di Paola G, et al. Alcoholic liver disease: a mouse model reveals protection by Lactobacillus fermentum. Clin Transl Gastroenterol. (2016) 7:e138. doi: 10.1038/ctg.2015.66
33. Chen RC, Xu LM, Du SJ, Huang SS, Wu H, Dong JJ, et al. Lactobacillus rhamnosus GG supernatant promotes intestinal barrier function, balances Treg and TH17 cells and ameliorates hepatic injury in a mouse model of chronic-binge alcohol feeding. Toxicol Lett. (2016) 241:103–10. doi: 10.1016/j.toxlet.2015.11.019
34. Chuang CH, Tsai CC, Lin ES, Huang CS, Lin YY, Lan CC, et al. Heat-killed Lactobacillus salivarius and Lactobacillus johnsonii reduce liver injury induced by alcohol in vitro and in vivo. Molecules. (2016) 21:1456. doi: 10.3390/molecules21111456
35. Mani V, Siddique AI, Arivalagan S, Thomas NS, Namasivayam N. Zingerone ameliorates hepatic and renal damage in alcohol-induced toxicity in experimental rats. Int J Nutr Pharmacol Neurol Dis. (2016) 6:125–32. doi: 10.4103/2231-0738.184585
36. Rishi P, Arora S, Kaur UJ, Chopra K, Kaur IP. Better management of alcohol liver disease using a ‘microstructured synbox’ system comprising L. plantarum and EGCG. PLoS One. (2017) 12:e0168459. doi: 10.1371/journal.pone.0168459
37. Kim WG, Kim HI, Kwon EK, Han MJ, Kim DH. Lactobacillus plantarum LC27 and Bifidobacterium longum LC67 mitigate alcoholic steatosis in mice by inhibiting LPS-mediated NF-κB activation through restoration of the disturbed gut microbiota. Food Funct. (2018) 9:4255–65. doi: 10.1039/C8FO00252E
38. Shukla PK, Meena AS, Manda B, Gomes-Solecki M, Dietrich P, Dragatsis I, et al. Lactobacillus plantarum prevents and mitigates alcohol-induced disruption of colonic epithelial tight junctions, endotoxemia, and liver damage by an EGF receptor-dependent mechanism. FASEB J. (2018) 32:fj201800351R. doi: 10.1096/fj.201800351R
39. Fang TJ, Guo JT, Lin MK, Lee MS, Chen YL, Lin WH. Protective effects of Lactobacillus plantarum against chronic alcohol-induced liver injury in the murine model. Appl Microbiol Biotechnol. (2019) 103:8597–608. doi: 10.1007/s00253-019-10122-8
40. Hendrikx T, Duan Y, Wang Y, Oh JH, Alexander LM, Huang W, et al. Bacteria engineered to produce IL-22 in intestine induce expression of REG3G to reduce ethanol-induced liver disease in mice. Gut. (2019) 68:1504–15. doi: 10.1136/gutjnl-2018-317232
41. Huang H, Lin Z, Zeng Y, Lin X, Zhang Y. Probiotic and glutamine treatments attenuate alcoholic liver disease in a rat model. Exp Therap Med. (2019) 18:4733–9. doi: 10.3892/etm.2019.8123
42. Nam Y, Kim JH, Konkit M, Kim W. Hepatoprotective effects of Lactococcus chungangensis CAU 1447 in alcoholic liver disease. J Dairy Sci. (2019) 102:10737–47. doi: 10.3168/jds.2019-16891
43. Yang X, He F, Zhang Y, Xue J, Li K, Zhang X, et al. Inulin ameliorates alcoholic liver disease via suppressing LPS-TLR4-Mψ axis and modulating gut microbiota in mice. Alcohol Clin Exp Res. (2019) 43:411–24. doi: 10.1111/acer.13950
44. Yi R, Tan F, Liao W, Wang Q, Mu J, Zhou X, et al. Isolation and identification of Lactobacillus plantarum HFY05 from natural fermented yak yogurt and its effect on alcoholic liver injury in mice. Microorganisms. (2019) 7:530. doi: 10.3390/microorganisms7110530
45. Jiang XW, Li YT, Ye JZ, Lv LX, Yang LY, Bian XY, et al. New strain of Pediococcus pentosaceus alleviates ethanol-induced liver injury by modulating the gut microbiota and short-chain fatty acid metabolism. World J Gastroenterol. (2020) 26:6224–40. doi: 10.3748/wjg.v26.i40.6224
46. Yi HW, Zhu XX, Huang XL, Lai YZ, Tang Y. Selenium-enriched Bifidobacterium longum protected alcohol and high fat diet induced hepatic injury in mice. Chin J Nat Med. (2020) 18:169–77. doi: 10.1016/S1875-5364(20)30018-2
47. You Y, Liu YL, Ai ZY, Wang YS, Liu JM, Piao CH, et al. Lactobacillus fermentum KP-3-fermented ginseng ameliorates alcohol-induced liver disease in C57BL/6N mice through the AMPK and MAPK pathways. Food Funct. (2020) 11:9801–9. doi: 10.1039/D0FO02396E
48. Zheng TX, Pu SL, Tan P, Du YC, Qian BL, Chen H, et al. Liver metabolomics reveals the effect of Lactobacillus reuteri on alcoholic liver disease. Front Physiol. (2020) 11:595382. doi: 10.3389/fphys.2020.595382
49. Fan H, Shen Y, Ren Y, Mou Q, Lin T, Zhu L, et al. Combined intake of blueberry juice and probiotics ameliorate mitochondrial dysfunction by activating SIRT1 in alcoholic fatty liver disease. Nutr Metab. (2021) 18:50. doi: 10.1186/s12986-021-00554-3
50. Gan Y, Tong J, Zhou X, Long X, Pan Y, Liu W, et al. Hepatoprotective effect of Lactobacillus plantarum HFY09 on ethanol-induced liver injury in mice. Front Nutr. (2021) 8:684588. doi: 10.3389/fnut.2021.684588
51. Hsieh PS, Chen CW, Kuo YW, Ho HH. Lactobacillus spp. reduces ethanol-induced liver oxidative stress and inflammation in a mouse model of alcoholic steatohepatitis. Exp Therap Med. (2021) 21:188. doi: 10.3892/etm.2021.9619
52. Jiang S, Ma Y, Li Y, Liu R, Zeng M. Mediation of the microbiome-gut axis by oyster (Crassostrea gigas) polysaccharides: a possible protective role in alcoholic liver injury. Int J Biol Macromol. (2021) 182:968–76. doi: 10.1016/j.ijbiomac.2021.04.050
53. Li X, Han J, Liu Y, Liang H. Lactobacillus casei relieves liver injury by regulating immunity and suppression of the enterogenic endotoxin-induced inflammatory response in rats cotreated with alcohol and iron. Food Sci Nutr. (2021) 9:5391–401. doi: 10.1002/fsn3.2486
54. Li X, Liu Y, Guo X, Ma Y, Zhang H, Liang H. Effect of Lactobacillus casei on lipid metabolism and intestinal microflora in patients with alcoholic liver injury. Eur J Clin Nutr. (2021) 75:1227–36. doi: 10.1038/s41430-020-00852-8
55. Patel F, Parwani K, Patel D, Mandal P. Metformin and probiotics interplay in amelioration of ethanol-induced oxidative stress and inflammatory response in an in vitro and in vivo model of hepatic injury. Mediators Inflamm. (2021) 2021:6636152. doi: 10.1155/2021/6636152
56. Zhao M, Chen C, Yuan Z, Li W, Zhang M, Cui N, et al. Dietary Bacillus subtilis supplementation alleviates alcohol-induced liver injury by maintaining intestinal integrity and gut microbiota homeostasis in mice. Exp Therap Med. (2021) 22:1312. doi: 10.3892/etm.2021.10747
57. Thurman RG, Bradford BU, Iimuro Y, Knecht KT, Arteel GE, Yin M, et al. The role of gut-derived bacterial toxins and free radicals in alcohol-induced liver injury. J Gastroenterol Hepatol. (1998) 13(Suppl.):S39–50. doi: 10.1111/jgh.1998.13.s1.39
58. Bajaj JS. Alcohol, liver disease and the gut microbiota. Nat Rev Gastroenterol Hepatol. (2019) 16:235–46. doi: 10.1038/s41575-018-0099-1
59. Roodposhti PM, Dabiri N. Effects of probiotic and prebiotic on average daily gain, fecal shedding of Escherichia coli, and immune system status in newborn female calves. Asian Australas J Anim Sci. (2012) 25:1255–61. doi: 10.5713/ajas.2011.11312
60. Vitetta L, Saltzman ET, Thomsen M, Nikov T, Hall S. Adjuvant probiotics and the intestinal microbiome: enhancing vaccines and immunotherapy outcomes. Vaccines. (2017) 5:50. doi: 10.3390/vaccines5040050
61. Xiao X, Nakatsu G, Jin Y, Wong S, Yu J, Lau JY. Gut microbiota mediates protection against enteropathy induced by indomethacin. Sci Rep. (2017) 7:40317. doi: 10.1038/srep40317
62. Markowiak P, Śliżewska K. Effects of probiotics, prebiotics, and synbiotics on human health. Nutrients. (2017) 9:1021. doi: 10.3390/nu9091021
63. Wang Y, Liu L, Moore DJ, Shen X, Peek RM, Acra SA, et al. An LGG-derived protein promotes IgA production through upregulation of APRIL expression in intestinal epithelial cells. Mucosal Immunol. (2017) 10:373–84. doi: 10.1038/mi.2016.57
64. Wei Q, Zhou B, Yang G, Hu W, Zhang L, Liu R, et al. JAZF1 ameliorates age and diet-associated hepatic steatosis through SREBP-1c -dependent mechanism. Cell Death Dis. (2018) 9:859. doi: 10.1038/s41419-018-0923-0
65. Tang CC, Huang HP, Lee YJ, Tang YH, Wang CJ. Hepatoprotective effect of mulberry water extracts on ethanol-induced liver injury via anti-inflammation and inhibition of lipogenesis in C57BL/6J mice. Food Chem Toxicol. (2013) 62:786–96. doi: 10.1016/j.fct.2013.10.011
66. Nakamura MT, Yudell BE, Loor JJ. Regulation of energy metabolism by long-chain fatty acids. Prog Lipid Res. (2014) 53:124–44. doi: 10.1016/j.plipres.2013.12.001
67. Kumar M, Nagpal R, Kumar R, Hemalatha R, Verma V, Kumar A, et al. Cholesterol-lowering probiotics as potential biotherapeutics for metabolic diseases. Exp Diabetes Res. (2012) 2012:902917. doi: 10.1155/2012/902917
68. Sekiya M, Yahagi N, Matsuzaka T, Najima Y, Nakakuki M, Nagai R, et al. Polyunsaturated fatty acids ameliorate hepatic steatosis in obese mice by SREBP-1 suppression. Hepatology. (2003) 38:1529–39. doi: 10.1016/j.hep.2003.09.028
69. Lieber CS. Role of oxidative stress and antioxidant therapy in alcoholic and nonalcoholic liver diseases. Adv Pharmacol. (1997) 38:601–28. doi: 10.1016/S1054-3589(08)61001-7
70. Seitz HK, Bataller R, Cortez-Pinto H, Gao B, Gual A, Lackner C, et al. Alcoholic liver disease. Nat Rev Dis Primers. (2018) 4:16. doi: 10.1038/s41572-018-0014-7
71. Inokuchi S, Tsukamoto H, Park E, Liu ZX, Brenner DA, Seki E. Toll-like receptor 4 mediates alcohol-induced steatohepatitis through bone marrow-derived and endogenous liver cells in mice. Alcohol Clin Exp Res. (2011) 35:1509–18. doi: 10.1111/j.1530-0277.2011.01487.x
72. Na HK, Lee JY. Molecular basis of alcohol-related gastric and colon cancer. Int J Mol Sci. (2017) 18:1116. doi: 10.3390/ijms18061116
73. Wang M, Ma LJ, Yang Y, Xiao Z, Wan JB. n-3 polyunsaturated fatty acids for the management of alcoholic liver disease: a critical review. Crit Rev Food Sci Nutr. (2019) 59:S116–29. doi: 10.1080/10408398.2018.1544542
Keywords: alcoholic liver disease, microbial agents, probiotics, prebiotics, gut-liver axis, meta-analysis
Citation: Wang Q, Shi J, Zhao M, Ruan G, Dai Z, Xue Y, Shi D, Xu C, Yu O, Wang F and Xue Z (2022) Microbial treatment of alcoholic liver disease: A systematic review and meta-analysis. Front. Nutr. 9:1054265. doi: 10.3389/fnut.2022.1054265
Received: 26 September 2022; Accepted: 03 November 2022;
Published: 21 November 2022.
Edited by:
George Grant, University of Aberdeen, United KingdomReviewed by:
Palash Mandal, Charotar University of Science and Technology, IndiaAmira Kamil Mohammed, University of Baghdad, Iraq
Copyright © 2022 Wang, Shi, Zhao, Ruan, Dai, Xue, Shi, Xu, Yu, Wang and Xue. This is an open-access article distributed under the terms of the Creative Commons Attribution License (CC BY). The use, distribution or reproduction in other forums is permitted, provided the original author(s) and the copyright owner(s) are credited and that the original publication in this journal is cited, in accordance with accepted academic practice. No use, distribution or reproduction is permitted which does not comply with these terms.
*Correspondence: Fangyan Wang, ZmFuZ3lhbl93YW5nQHdtdS5lZHUuY24=; Zhanxiong Xue, eHVlemhhbnhpb25nQDEyNi5jb20=
†These authors have contributed equally to this work