- 1College of Light Industry and Food Engineering, Guangxi University, Nanning, China
- 2School of Pharmacy, Hainan Medical University, Haikou, China
Fermented wine refers to alcoholic beverages with complex flavor substances directly produced by raw materials (fruit or rice) through microbial fermentation (yeast and bacteria). Its production steps usually include saccharification, fermentation, filtration, sterilization, aging, etc., which is a complicated and time-consuming process. Pulsed electric field (PEF) is a promising non-thermal food processing technology. Researchers have made tremendous progress in the potential application of PEF in the fermented wine industry over the past few years. The objective of this paper is to systematically review the achievements of PEF technology applied to the winemaking and aging process of fermented wine. Research on the application of PEF in fermented wine suggests that PEF treatment has the following advantages: (1) shortening the maceration time of brewing materials; (2) promoting the extraction of main functional components; (3) enhancing the color of fermented wine; (4) inactivating spoilage microorganisms; and (5) accelerating the formation of aroma substances. These are mainly related to PEF-induced electroporation of biomembranes, changes in molecular structure and the occurrence of chemical reactions. In addition, the key points of PEF treatments for fermented wine are discussed and some negative impacts and research directions are proposed.
Introduction
Fermented wine, known as original juice wine, is made of raw materials containing starch and sugar (fruit or rice) through the fermentation of yeast and bacteria to produce complex flavor substances and alcohol, such as red wine, white wine, and rice wine. The production of fermented wine is a complex and time-consuming process, including saccharification, fermentation, filtration, sterilization, and a series of continuous technological procedures. Any operation introduced in the brewing process can affect the physicochemical properties and sensory characteristics of the finished wine (1, 2). Some freshly fermented wines are not suitable for immediate drinking due to their spicy taste, pungent smell, precarious color, and aroma. In order to improve their stability, quality and sensory characteristics, these freshly fermented wines are customarily required to undergo post-processing, i.e., aging (also known as maturation) (3). However, several disadvantages of using oak barrels, pottery or other modern containers for naturally mature fermented wines, such as red wine and rice wine, are worth investigating, including long time, large storage space, and the presence of undesirable microorganisms that cause food contamination and produce unpleasant tastes (4). Therefore, it is necessary to adopt innovative technologies to optimize or accelerate the winemaking and aging of fermented wine.
Pulsed electric field (PEF) is a promising non-thermal food processing technology, which can effectively ensure the good quality of products (5–9). Recent technological developments, especially the use of continuous processing chambers, have provided more possibilities for scaling up the application of PEF technology, which has attracted widespread attention (10–12). The potential applications of PEF in the fermented wine industry have been extensively studied worldwide (13–16). Therefore, this review aims to systematically summarize the achievements of PEF technology in the fermented wine industry, and discuss the corresponding processing principles and some negative effects, so as to provide references for future research directions.
Principles of pulsed electric field
Pulsed electric field is a promising non-thermal technology, which applies a high-intensity electric field pulse for a short time (treatment time usually in the microsecond scale) to food or raw material between two electrodes (17–19). The exponentially decaying waves and square waves are commonly used in PEF processing, and square wave pulses are considered better than the exponential decay wave pulses because the former allows the material to be treated with a sustained and constant intensity for the total duration of the pulse (5). The factors affecting the efficiency of PEF treatment include electric field intensity, pulse number, pulse shape, pulse time/length, and initial temperature of processing medium (raw material), etc. (20). Among them, electric field intensity and treatment time (t = pulse duration × pulse number) are the main processing factors, and increasing the intensity of these two parameters generally improves the processing efficiency of PEF (5).
When an external electric field is applied, the charge accumulation on the membrane surface causes an increase in the transmembrane potential on both sides of the membrane. After the critical value of transmembrane potential is exceeded, the electroporation (reversible or irreversible) will be produced in cell membranes, resulting in a sharp increase in membrane permeability (21, 22). Animal and plant cells require a lower critical electric field intensity (0.5–2 kV/cm) for electroporation because their cell sizes are larger than microbial cells (10–14 kV/cm) (23). The electroporation dramatically increases membrane permeability which may affect mass transfer or cell rupture (Figure 1). Therefore, PEF technology has received extensive attention and research in a variety of food processing, such as food dehydration (24), sterilization (25), promotion of extraction (26), and reduction of pesticide residues (27). In addition, the PEF treatment promotes the ionization and polarization of molecules, changes the internal molecular arrangement, improves the effective collision rate between molecules, speeds up the chemical reaction rate in dynamic equilibrium, and reduces the activation energy required for the reaction. Hence, PEF is gradually used to accelerate the oxidation, reduction, association, hydrolysis, and other reactions in food (5, 25–28), especially liquid food such as fruit juices and alcoholic beverages (29). Meanwhile, compared with other non-thermal processes, such as high hydrostatic pressure (high equipment costs and discontinuous production) (30), the PEF technology also exhibits several advantages, such as shorter processing time, lower treatment temperature, and continuous flow processing (31).
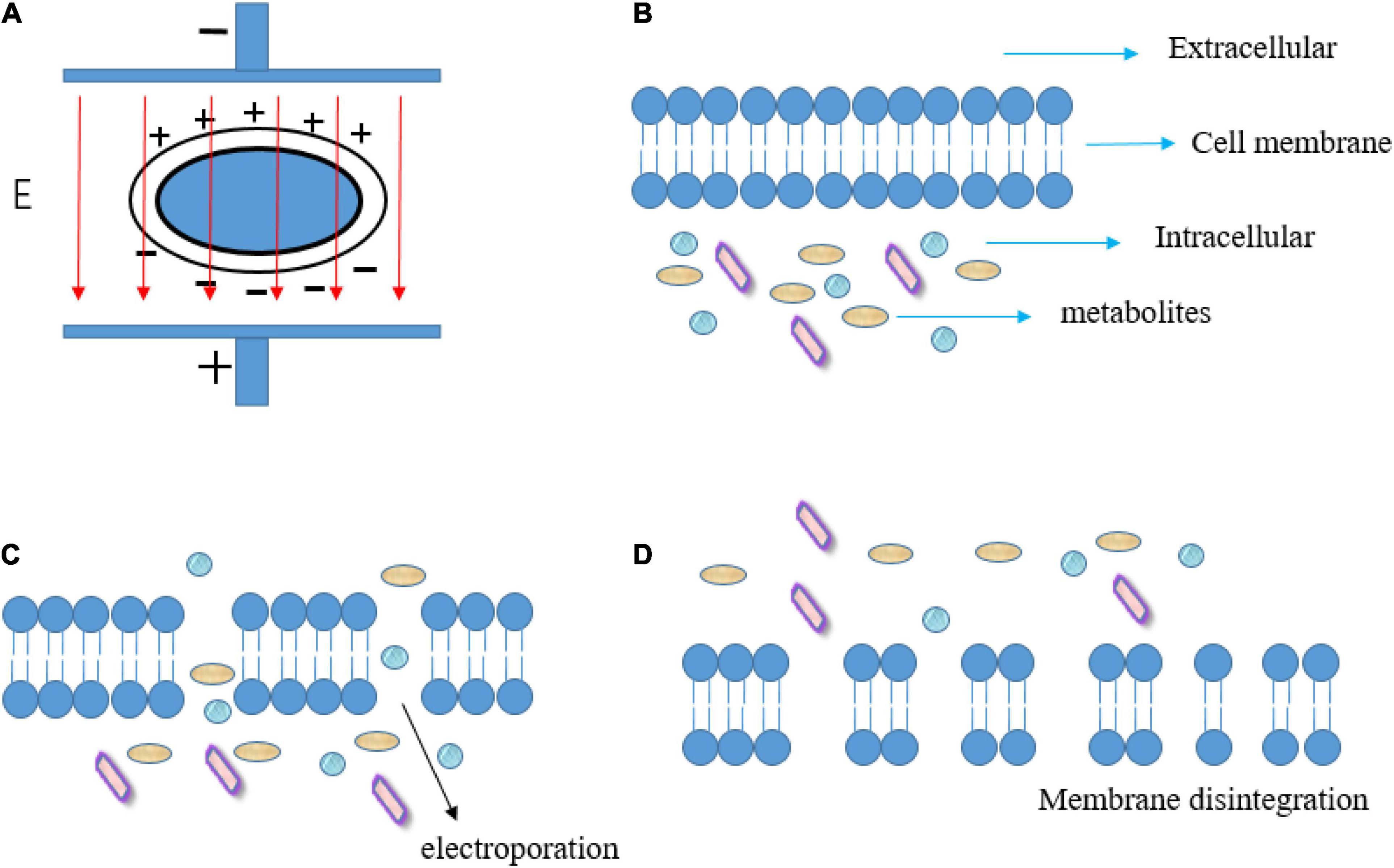
Figure 1. Schematic diagram of transmembrane potential induced by PEF (A), membrane before electroporation (B), electroporation of cell membrane (C), and membrane disintegration induced by excessive PEF treatment (D). Adapted from Mahesha et al. (96).
Applicability of pulsed electric field
Enhancement of primordial composition extraction
The efficient extraction of functional components from target tissues or cells is a major problem prior to fermentation. The raw materials are usually pressed and macerated for a long time to extract their active ingredients such as sugar and polyphenols in traditional brewing, thus improving the yield and quality of fermented wine (32, 33). PEF is adopted as a new way for promoting the extraction of active ingredients, shortening the immersion time, and promoting alcohol fermentation. This is mainly attributed to electroporation induced by PEF treatment (Figure 1), which increases the permeability of cell membrane to ions and macromolecules, thus promoting the release of intracellular substances (18). For example, polyphenols, including phenolic acids, flavanols, flavonols, anthocyanins, and stilbenes, are the most important functional compounds in winemaking, since they have multiple biological effects and contribute to the wine’s distinctive color (34). Unfortunately, only 40% of anthocyanins and 20% of tannins from grape skins are transferred to wine in the case of traditional winemaking (35, 36). This relatively low extraction efficiency is the result of insufficient permeabilization of cell walls and cytoplasmic membranes. Hence, PEF treatment has great potential to promote the extraction of functional components in the maceration process. Interestingly, although PEF treatment can promote the dissolution of active ingredients during the maceration stage, there are other studies in which the PEF treatment of the samples did not affect some enological parameters of the grape juice samples (37). Clodoveo et al. (38) describe the mechanism that PEF treatment can destroy the cell membranes of grapes, and this treatment has no effect on alcohol content, total acidity, pH, volatile acidity and concentration in reducing sugar. It is worth noting that high voltage is usually required to produce electroporation, but high-intensity PEF treatment has a strong electrolytic effect, and its high electric field strength and long treatment time may lead to the degradation of some macromolecular substances.
Improvement of juice yield
The yield of fruit juice is an important index to measure the effect of maceration, and it obviously increases by PEF treatment in the brewing process. After applying 11 kJ/kg (1.5 kV/cm, 8 μs) and 22 kJ/kg (1.5 kV/cm, 16 μs) of PEF treatments, the yield of grape juice increased by 8.9 and 4.3% compared with 78.0% (w/w) of the untreated sample, respectively (14). Similarly, an increase in “Pinot Noir” grape juice yield was observed, which may be attributed to increased cell membrane permeability and accelerated mass transfer after PEF treatment (1.5 kV/cm, 1,033 pulses) (39). Meanwhile, PEF treatment (1.5 kV/cm, 1,033 pulses) of grapes increased the release rate of anthocyanins, thus achieving an adsorption-desorption equilibrium between the anthocyanins inside the skin and outside the juice within a shorter time. As a significant property, dry extract consists of fixed wine compounds, including sugars, glycerol, organic acids, phenolics, and minerals, contribute to the development of distinctive taste and in turn affect the sensory characteristics and quality of the wine (16). Compared with the control group, the dry extract content in jujube wine increased by 10% after PEF treatment (1.5 kV/cm, 50 pulses), which may be related to the improvement of mass transfer in pulp tissues, indicating that PEF pretreatment had a positive impact on jujube wine quality (Table 1). The increases in total acidity and phenolics could also explain the cause of the elevated dry extract contents, which was consistent with the previous study (1.5 kV/cm, 16 μs) (14). Skin maceration and maturation in wooden barrels have also been reported to improve dry extracts and obtain good mouth-feel properties, but require a long time-consuming (40).
Promoting extraction of phenolic compounds
Phenolic compounds are important components in determining the quality of fermented wine, which influence the color, mouthfeel, and aging ability of wines. The phenolic compounds in winemaking can be divided into flavonoids (12 major subclasses, mainly anthocyanidins, flavan-3-ols, flavonols, flavanones, isoflavones, and others) and non-flavonoids (mainly hydroxyl cinnamic acid derivatives) (41). The application of PEF in the maceration stage can greatly enhance the content of phenolic compounds in fermented wine. Previous studies have shown that PEF treatments can increase the contents of total phenols, total flavonoids and anthocyanins in wine using different voltages (7–8 kV) and different frequencies (178–344 Hz) (42). The PEF treatment (8 kV/cm, 344 Hz, 300 s pulses) resulted in a wine with a content of total phenols 2 times and 1.5 times higher than the control sample in the case of “Pinot Noir” and “Merlot” red wine, respectively. Apart from phenolic compounds localized in the grape skins, PEF treatment improved the extraction of phenolic compounds that primarily originate from the grape seeds such as flavanols e.g., (+)-catechin. PEF treatment (7.4 kV/cm) on crushed grape berries of Tempranillo and Grenache has also been shown effective in enhancing (+)-catechin and (−)-epicatechin concentrations in the grape juice (37). Particularly, PEF treatment can promote the release of components that are difficult to leach through normal pathways by inducing electroporation of the cell membrane. Arcena et al. (26) observed that the flavonol quercetin along with a few anthocyanins, such as delphinidin-3-O-glucoside and petunidin-3-O-glucoside, were detected exclusively in Merlot musts pre-treated with high energy treatments, which indicated that not all phenolic compounds were easy to be extracted, thus the application of PEF was needed to enhance their extractability. Notably, PEF can also significantly increase the extraction rate of phenols in other fermented wines. Xu et al. (16) took jujube pulp as the research object and carried out different PEF treatments with 10 exponential wave pulses at 1.5 kV/cm, 1 Hz to improve the extraction of phenolic compounds, especially caffeic acid, morin, and p-hydroxybenzoic acid, which significantly strengthened the floral and fruit flavor volatility of jujube wine. In a similar report by Li et al. (43), the phenolic content in the fermented jujube wine after 20 min with PEF (1.5 kV/cm, 10 μs, 0.1 kHz) increased by 45% compared with the control group.
The variation trend of phenolic content was not consistent with different PEF treatment conditions, which is closely related to the variety of raw materials. El Darra et al. (44) applied PEF treatments with medium and high field intensity (0.8 and 5 kV/cm) to improve the total polyphenols content of the pre-treated extract of Cabernet Sauvignon red grapes during low-temperature maceration, showing that color intensity, anthocyanins content, and the extraction kinetics of phenolic compounds were significantly enhanced. Other authors confirmed that the extraction rate of phenols from Cabernet Franc grapes increased by 51 and 62% after PEF treatment (0.8–5 kV/cm, 42–53 kJ/kg), respectively (45). A similar finding was reported by investigating the effect of PEF at different electric field strengths (up to 41.5 kV/cm) and energy inputs (up to 49.4 kJ/kg) on the volatilities and phenolic profiles of “Merlot” grapes at different stages of winemaking (26). PEF treatment increased the contents of anthocyanins, catechin, stilbenes, hydroxycinnamic acid, and hydroxybenzoic acid in the wine after alcoholic and malolactic fermentation. Compared to the untreated group, juice from PEF-treated grapes was found to be at least 7–49 times higher in individual anthocyanins. The stilbenoids, flavonols, and hydroxycinnamic acids at all applied PEF intensities also increased 2–5 times, 2–11 times, and 4–6 times, respectively (26). Interestingly, the content of phenols did not necessarily increase with increasing applied electric field strength. The effect of PEF (0.9–3 kV/cm) on the extractability of polyphenols in early-harvested Sangiovese red grapes from Emilia Romagna (Italy) was investigated by Ricci et al. (46). The results showed that the extraction rate of polyphenols in the PEF-treated groups (10.4, 23.8, 32.5 kJ/kg) increased by 22.9, 16.1, and 20.4%, respectively, compared with the control group. More notably, the total phenolic content of the PEF-treated groups (10.4, 23.8, 32.5 kJ/kg) was significantly increased by 49.0, 60.8, and 60.7%, respectively, after maceration (46). Therefore, when applying a PEF treatment, the transmembrane transport of bioactive compounds was regulated by pore dimensions produced during electroporation and by the size of the passing molecules, which means that the extent of PEF intensity may modulate the composition and amount of the polyphenol components (14).
In general, according to published research results, it is not difficult to find that PEF treatment increases the content of total phenolic compounds and effectively promotes the dissolution of components, and the main influencing factors are electric field intensity, treatment time, and raw material type. Due to the electroporation effect, there are three main trends in the pretreatment of raw materials by PEF. Firstly, the yield of juice during the maceration stage was increased. Secondly, the extraction efficiency of compounds from the skins and the seed of raw materials was improved, which was manifested by the increase of phenolic substances such as hydroxycinnamic acids, malvidin-3-O-glucoside and (+)-catechin. Finally, the higher applied electric field strength may lead to a decrease in the extraction rate of polyphenols, and there is an optimal value of PEF treatment conditions.
Decreasing maceration time
The extraction of pigment compounds from the cells of the skin during the maceration step is controlled by diffusion through the cell membranes in the winemaking process (46). The extraction requires that the pigment compounds leave both the membrane-bound vacuole and the cell itself, which is a slow process that requires several days or even a dozen days, while PEF treatment can significantly shorten the maceration time and ensure adequate pigment extraction. The influence of PEF treatment (5 kV/cm, 2.1 kJ/kg) on the grape pomace on quality parameters and anthocyanins content of Cabernet Sauvignon wines obtained after different maceration times has been investigated (47). Regardless of the maceration time, the freshly red wine was richer in color intensity, total polyphenols index, and tannins, and showed better visual characteristics with PEF treatment. Meanwhile, it was detected that the concentrations of malvidin-3-glucoside and malvidin-3-glucoside acetate, as the main anthocyanins in red wine, were higher than those in control wine. Similar results were observed in the study of Puértolas et al. (48), where the maceration time for PEF-wine was only 96 h, 33% less than the control wine that was macerated for 144 h. According to the results obtained, the application of PEF treatment can significantly reduce the maceration time during winemaking. Interestingly, when using a pre-fermentative maceration at 12°C for 6 h, PEF-wine (0.8 and 5 kV/cm) presented an anthocyanin concentration of 36% higher than the control (Figure 2C). In contrast, Ducasse et al. (49) using a maceration scheme consisting of 12 h at a temperature of 15°C, obtained an increment of 13% on the anthocyanin content of Monastrell rosé wines using an enzymatic preparation. This indicated that PEF treatment can effectively improve the extraction rate of anthocyanin than enzyme treatment.
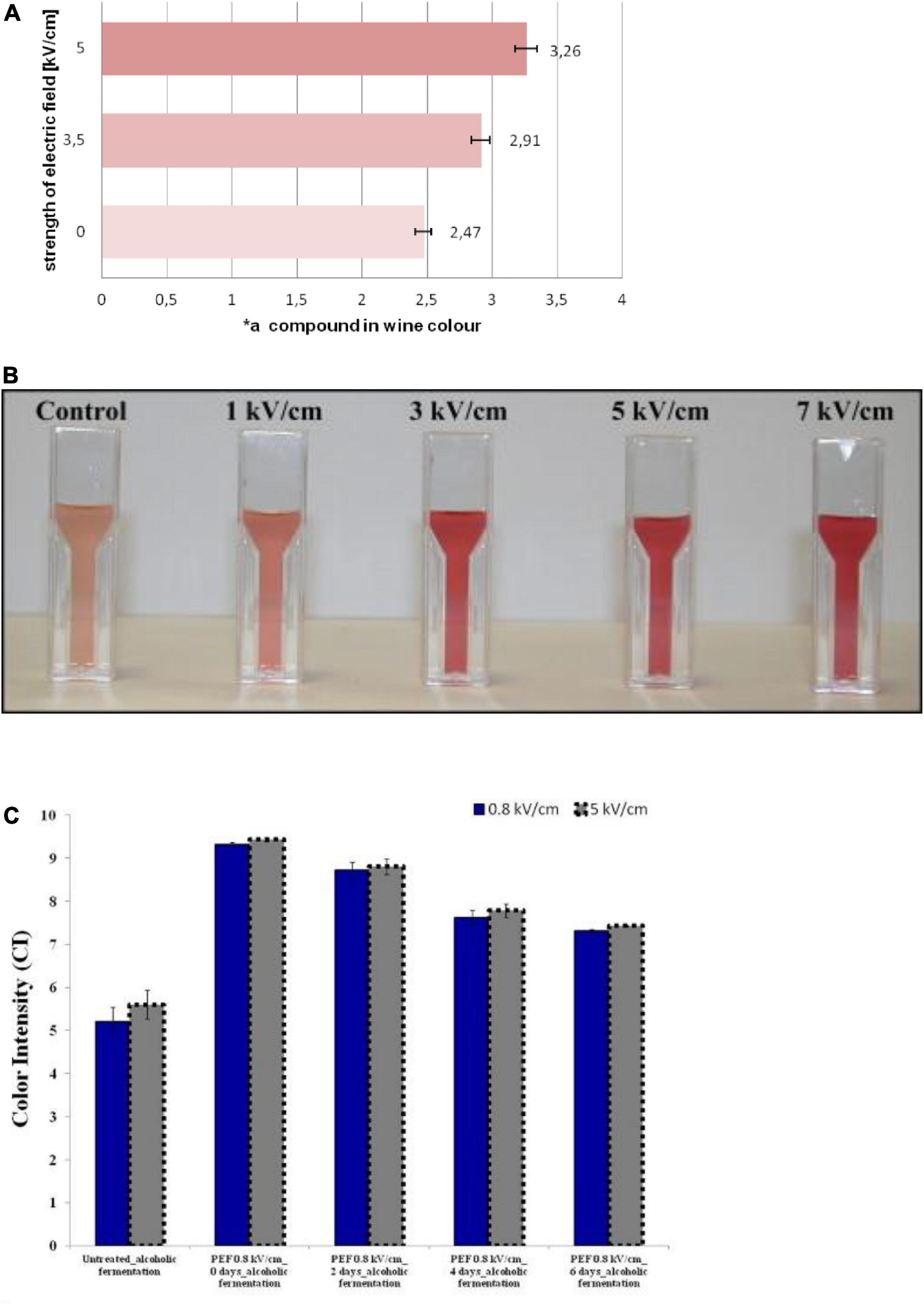
Figure 2. (A) Share of the red component (*a) in the color of the control group and PEF treatment (3.3 and 5 kV/cm) wines. Reprinted from Puértolas et al. (48). (B) The color of the Garnacha must significantly enhanced with the increase of PEF treatment (1–7 kV/cm, 0.4–4.1 kJ/kg, 50 pulses). Reprinted from Hui et al. (85). (C) Color intensity (CI) at t = 0, 2, 4, and 6 days of Alcoholic Fermentation using both high (5 kV/cm) and moderate (0.8 kV/cm) PEF. Reprinted from El Darra et al. (44).
Enhancement of color
The color of fermented wine is usually an important index to measure its quality. For red wine, its color is generally determined by a mixture of pigment compounds (tannins, anthocyanins, flavonols, etc.), influenced by various factors such as variety, harvest year, grape ripeness, health, winemaking techniques, age, and storage conditions (50, 51). The color was determined using the CIELab method in the produced wines, obtaining L, * a, and * b values, which are the axes of a three-dimensional color space (52). According to research, PEF has a positive effect on the color of red wine, without affecting wine characteristics such as alcohol content, total acidity, pH, sugar concentration, and volatile acidity. As reported in Ilona et al. (53), in the maceration stage, the grapes were treated with a PEF treatment of 3.3 and 5 kV/cm for 10 s (Figure 2A). The share of the red parameter (*a) in wines is respectively higher by 15 and 24% than those of the samples without additional pretreatment (Table 1).
The color of the Garnacha must after maceration significantly enhanced with the increase of PEF treatment conditions (50 exponentially decaying pulses; 1–7 kV/cm, 0.4–4.1 kJ/kg) (Figure 2B). With PEF treatment (5 kV/cm, 122 Hz, 50 pulses) during aging in the bottle, the color intensity and Folin–Ciocalteu index in red wine from Cabernet Sauvignon grapes increased by 31 and 25%, respectively (48). Anthocyanin content in Cabernet Sauvignon rosé wines obtained from grapes treated with PEF (5 kV/cm, 50 pulses) was investigated. After 2 months of storage in bottles, varying extents of increases were observed in fourteen different anthocyanins (unacylated, acylated, and coumarylated) compared to the control group (54). The results showed that the extraction rate of wine-specific pigment compounds was higher under the support of PEF due to the effect of electroporation, which was beneficial because the color of darker wine indicated high levels of colored antioxidant beneficial compounds. Furthermore, the application of PEF on a semi-industrial scale has also achieved satisfactory results. The researchers examined the effect of PEF pretreatment (0.4–7 kV/cm, 100 μs–10 ms) on wine color at a scale of 2 tons per hour (55). Red wine produced by PEF-treated grapes had a 20–30% higher color intensity and a 7–17% higher total polyphenol index than the control group. Surprisingly, the total anthocyanin content of PEF wine was 34% higher than that of the control.
The purplish tones of young red wine typically develop into more stable brick-like colors of matured wines during storage (56–58). During the aging period after fermentation, the proanthocyanidins in wine will self-polymerize and form high molecular weight polymers with anthocyanins. In addition, the flavan-3-ols component unit of proanthocyanidins will form condensates with anthocyanins or acetaldehyde, resulting in the maturation of wine color (59). The content of proanthocyanidins, average degree of polymerization and component units of proanthocyanidins in Merlot red wine changed significantly after high voltage PEF treatment (6–24 kV/cm, 10 μs, 10 Hz, 0–300 pulses), and the trend of change was consistent with the natural aging effect (60). When the electric field strength was lower than 18 kV/cm, the treatment effect was closer to aging with the increase of the field strength. However, it is worth noting that when the field strength was up to 24 kV/cm, the excessive field strength would promote the participation of proanthocyanidin in the chemical reaction, and its degradation rate would exceed the formation rate, leading to the decrease of treatment effect. Therefore, moderate PEF treatment is beneficial to accelerate wine aging and stabilize wine color.
Inactivation of spoilage microorganisms
Yeasts and bacteria are common spoilage microorganisms in fermented wine, which negatively affect the quality and shelf life of wine and cause detrimental economic losses (61, 62). SO2 is generally used in the traditional winemaking process as an antioxidant and selective antibacterial additive to inhibit the growth of molds in the must during the early stage of wine production and the growth of undesirable bacteria and yeast during the fermentation process, to avoid microbial spoilage in the wine production and storage process (63). The problem is that SO2 can cause some adverse effects on consumers including allergic reactions, headaches, asthma, and abdominal pain. Therefore, the addition of SO2 is limited to a maximum of 150–350 mg/L. PEF leads to membrane electroporation and even disintegration due to its short and high electric field intensity pulses (Figure 3), which have a significant inactivation effect on microorganisms. Hence, the application of PEF to the microbial inactivation step of fermented wine can reduce or even replace the amount of SO2 addition in winemaking, while achieving the expected sterilization effect (64, 65).
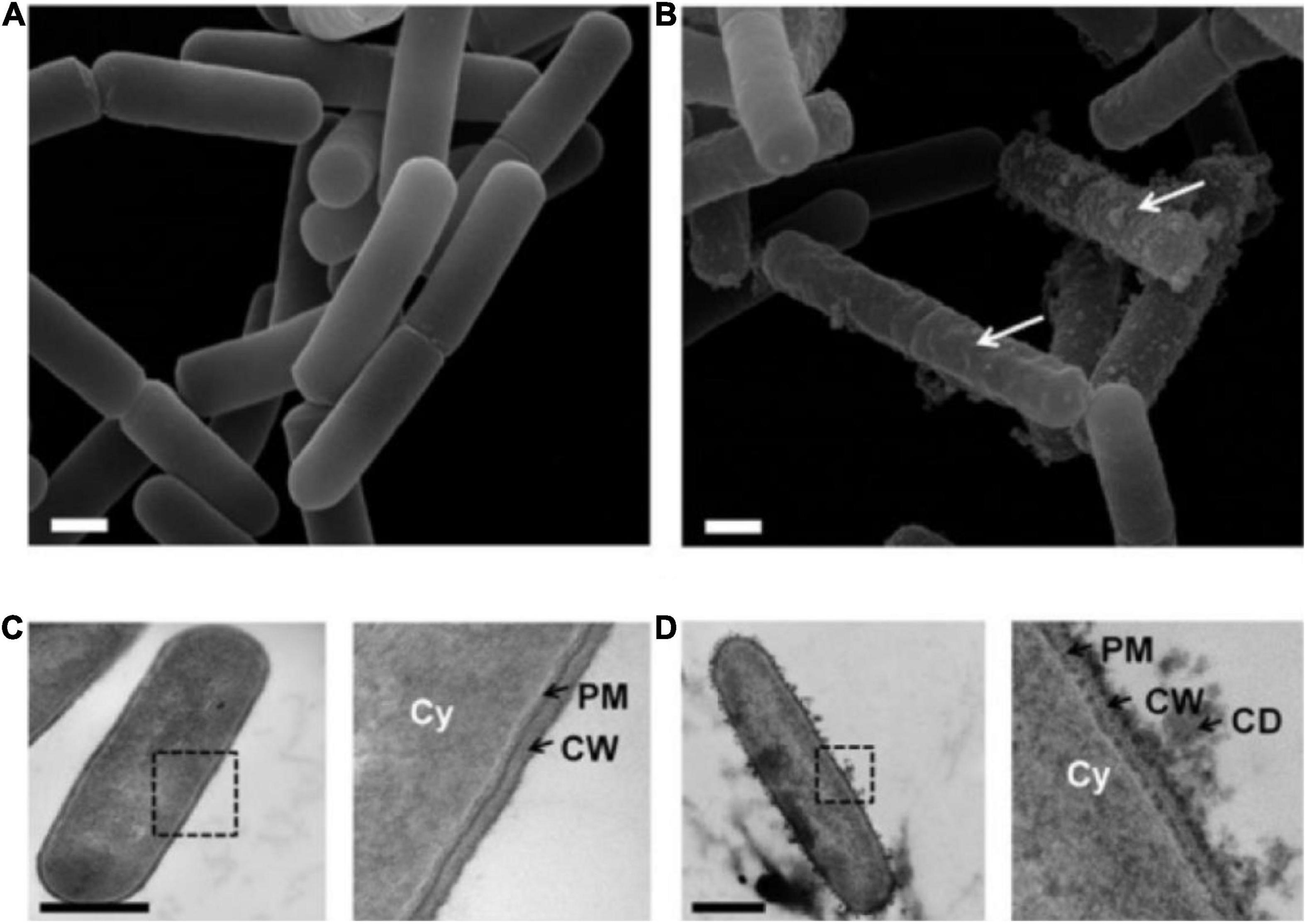
Figure 3. (A) SEM image of untreated bacteria. (B) After exposure to PEF (7.5 kV/cm, 1 ms), the SEM image showed bacterial surface damage. (C) TEM image of untreated Bacillus pumilus. Cy, cytoplasm; PM, plasma membrane; CW, cell wall. (D) TEM image of Bacillus pumilus after PEF. The illustration revealed the discharge of cell debris (CD) and the damage to PM and CW. Scale bars, 500 nm. Reprinted from Davaux et al. (71).
Lethal effects
Pulsed electric field treatment has proven to be a highly efficient wine pasteurization technique because it can inactivate key spoilage microorganisms in a short time, while retaining the distinctive flavors and aromas of the wines produced, without influencing taste and quality (66). There may be a variety of spoilage yeasts in winemaking, such as Saccharomyces cerevisiae, Saccharomyces bayanus, Zygosaccharomyces fermentati, and species of Candida, Pichia, and Hansenula, which may lead to the formation of thin-film growth on the surface of the wine, affecting the quality of wine (67) (pp. 535–676). A variety of bacteria can also be present in wine, such as Lactobacillus brevis, Oenococcus oeni, Lactobacillus buchneri, and Pediococcus, which can cause spoilage, pH rise, turbidity, discoloration, stale taste, and sediment formation (67) (pp. 535–676).
The inactivation effect is closely related to the species of microorganisms, due to the different sensitivity of microorganisms to PEF treatment. S. cerevisiae can cause a secondary fermentation of the wine when residual sugar is present, eventually forming a precipitate in the wine. Abca and Evrendilek (68) found that a 3 μs square bipolar pulse with a field intensity of 31 kV/cm resulted in 4.5 log reductions of S. cerevisiae in red wine. When the same electric field intensity was applied to S. bayanus in red wine, the inactivation rate was significantly increased, reaching 5.4 log reductions. The PEF resistance of different wine spoilage microorganisms such as Dekkera anomala, Dekkera bruxellensis, Lactobacillus hilgardii, and Lactobacillus plantarum both must and wine was investigated by applying treatments ranging from 16 to 31 kV/cm and from 10 to 350 kJ/kg at 24°C (69). The optimal treatment with a specific energy of 186 kJ/kg has been established which permitted to reduce the 99.9% of the spoilage flora of must and wine at the field strength of 29 kV/cm, limiting the risk of alteration of these products by microorganisms of genera Brettanomyces and Lactobacillus. Concerning bacteria in wine, Gonzalez-Arenzana et al. (65) treated 12 lactic acid bacteria and 4 acetic acid bacteria in Tempranillo red wine under four different PEF conditions with specific energies of 60, 62, 72, and 95 kJ/kg, respectively. The inactivation rate was around 1.93 log reductions (Pediococcus pentosaceus) and 3.56 log reductions (Acetobacter pasteurianus) at PEF treatment of 62 kJ/kg (22 kV/cm, 154 μs), (Table 1). The inactivation cycles obtained with the 72 kJ/kg PEF treatment (27 kV/cm, 123 μs), were between 0.64 log reductions (Acetobacter aceti) and 2.44 log reductions (Metschnikowia pulcherrima). The 95 kJ/kg PEF treatment (33 kV/cm, 105 μs) managed inactivations between 1.96 log reductions (O. oeni strain O46) and 4.94 log reductions (A. pasteurianus).
A similar effect was achieved with PEF treatment in rice wine. Lyu et al. (77) found that 2.88 log reductions of Saccharomyces cerevisiae were inactivated when PEF treatment with moderate conditions (35°C, 12 kV/cm, 120 μs) was applied to Chinese rice wine. Further, Huang et al. (70) used PEF technology to evaluate the inactivation effect of S. cerevisiae at the initial temperature of 25–35°C, electric field intensity of 12–21 kV/cm and treatment time of 30–180 μs. The highest inactivation values, corresponding to approximately 5.5 log reductions, were obtained at 21 kV/cm and 180 μs with the initial treatment temperatures of 35°C. Davaux et al. (71) investigated the effects of the PEF (7 kV/cm) on the microbiological stability of wines on a semi-industrial scale from 500 to 1,200 L/h. When the temperature of wine without adding SO2 did not exceed 50°C, PEF had a significant inactivation effect on yeast in wine (71). The results obtained showed an excellent efficiency of the yeast treatment with an instant cessation of alcoholic fermentation and a decrease in the yeast population ranging from 3 to 5 log reduction.
Sublethal effects
Recently, the sublethal effects of PEF on microorganisms have attracted more and more attention. There are many factors affecting the microbial inactivation by PEF and one of the key contributing factors is critical electric field intensity, which is defined as the minimum electric field intensity required for microbial inactivation. Previous studies have shown that the intensity of the applied electric field must exceed a critical electric field intensity for electroporation (reversible or irreversible) of the cell membrane to occur (72, 73). Notably, when the cell membrane undergoes reversible electroporation after PEF treatment, a sublethal state of the cells may be induced (73). In general, the critical electric field strength value depends on microbial characteristics, especially on cell size and shape, and the characteristics of the growing medium (72). Critical electric field intensity was lowest for the yeast (14.83 kV/cm) and it was 18.64 kV/cm for the aerobic bacteria (73). Whereas, the highest critical electric field intensity value of 19.46 kV/cm was noted for the lactic acid bacteria (73). This variation in PEF sensitivity of the microorganisms could be due to the differences in cell size since the relatively large-sized cells (yeast) inactivated at the lowest electric field intensity. Generally, with the increase of voltage amplitude, capacitance capacity, and discharge times, the bactericidal rate keeps improving. When the injection energy is more than 12 J/ml at normal temperature, the bactericidal rate of PEF (15–25 kV/cm) is more than 97%, and the maximum 2-log reduction inactivation rate can be achieved (74).
Brettanomyces bruxellensis is mainly found in barrel-aged red wines with low SO2 content and high pH (75). The researchers inoculated the wine with B. bruxellensis and then treated it with continuous PEF. The concentration of B. bruxellensis in wine was determined after inoculation, ranging from 2.3 × 105 to 5.9 × 105 CFU/ml. The B. bruxellensis concentration decreased to 9.2 × 104 CFU/ml (0.8 log reduction) after PEF treatment (32 kV/cm, 250 Hz, 51.2 μs). However, the yeast concentration had returned to the same level as the untreated wine after 2 months. Therefore, moderate PEF treatment conditions which could have induced a sublethal state instead of cell death, are not sufficient to inactivate B. bruxellensis to produce microbiologically stable wines (66). To completely inactivate B. bruxellensis in wine, more demanding PEF conditions are required, including higher electric field intensities and longer treatment times. A recent study found that multiple PEF treatments (50 kV/cm, 100 Hz, 78 μs) achieved the expected level of B. bruxellensis inactivation in Cabernet Sauvignon red wine (>6 log reductions) (25).
It is worth noting that the efficiency of PEF inactivating microorganisms is also closely related to food medium (processed raw materials) factors such as alcohol and temperature. For example, Acetobacter sp. is also one of the spoilage microorganisms during winemaking, converting ethanol produced by yeast into acetic acid, which increases the volatile acidity of the wine. The effect of ethanol as a growth substrate on PEF resistance in Acetobacter sp. cells was investigated by Niu et al. (22). The inactivation rate of Acetobacter sp. (109 CFU/ml) cultivated with 9% ethanol by PEF treatment (20.0 kV/cm, 6.0 ms) could reach 5.17 log reductions, which was significantly higher than that cultivated without ethanol (3.22 log). According to the report, the combination of eugenol and PEF has a strong synergistic bactericidal effect. The inactivation level of Escherichia coli was increased from 0.39 to 0.96 log when the added eugenol concentration was increased from 0 to 0.64 mg/ml and PEF treatment intensity was 20.0 kV/cm (76). Besides, increasing the initial temperature of fermented wine during PEF treatment had a significant effect on microbial inactivation. The survival yeast decreased with 4.09 log reductions when the Chinese rice wine was preheated to 35°C and followed by PEF treatment (18 kV/cm, 150 μs). In comparison, the yeast inactivation was 2.05 log reductions at an initial temperature of 25°C and the same PEF treatment (77).
In summary, PEF used for microbial inactivation can maximize the sterilization effect, inactivate enzymes, protect the nutritional components in wine, and require low processing temperature, a short processing time of several decades microsecond to sterilize. However, it was very difficult to summarize and systematically classify resistance to PEF based on size, shape or membrane structure due to the high number of different microorganisms. The inactivation of microorganisms associated with fermented wine depends on the PEF conditions applied and the characteristics of the microorganisms (78). The diversity of microbial species which are PEF resistant indicates PEF sterilization needs strict verification before application.
Formation of aroma substances during aging
Freshly fermented wines usually have some disadvantages such as sourness, thin taste, stimulating taste, and many other negative impacts due to the high content of fusel oil, acetal, and free alcohol. Hence, aging is needed to stabilize the wine quality, while various biological and chemical reactions occur during the aging process, resulting in complex aromas and full-bodied mouthfeel (79). It is reported that high-intensity PEF treatment (usually >15 kV/cm) can accelerate the chemical reaction rate in dynamic equilibrium and reduce the activation energy required for the reactions (Figures 4, 5), which can significantly promote the formation of various aroma components such as thiol, acids, polyphenols, and esters in fermented wine (28). For example, it was found that Merlot red wine vinified with PEF-treated grapes possessed higher thiol concentrations and higher intensities of blackcurrant flavor character at different electric field strengths (up to 41.5 kV/cm) and energy inputs (up to 49.4 kJ/kg) (26).
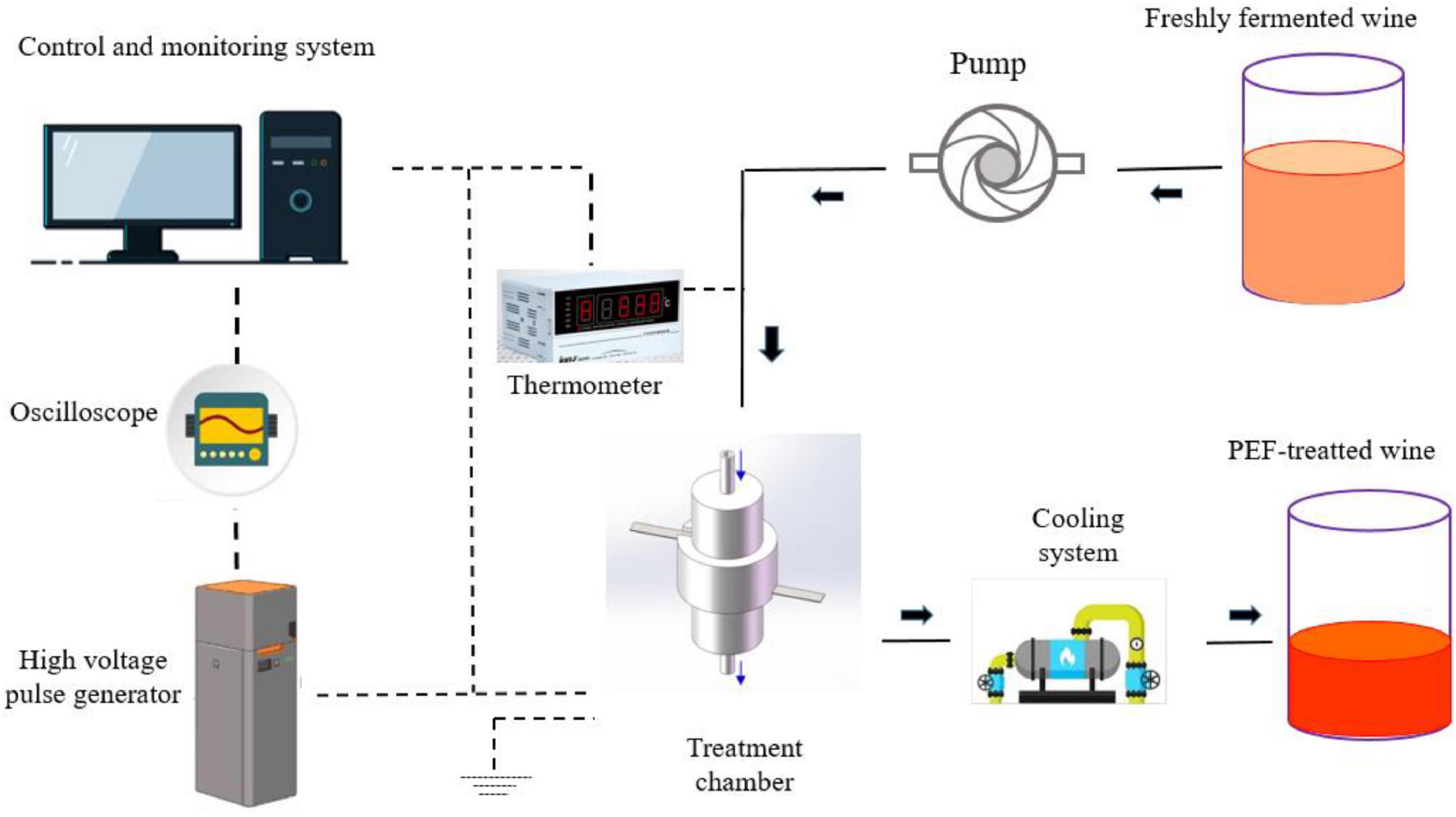
Figure 4. Schematics diagram of a PEF treatment system for fermented wines. Adapted from Wang et al. (10).
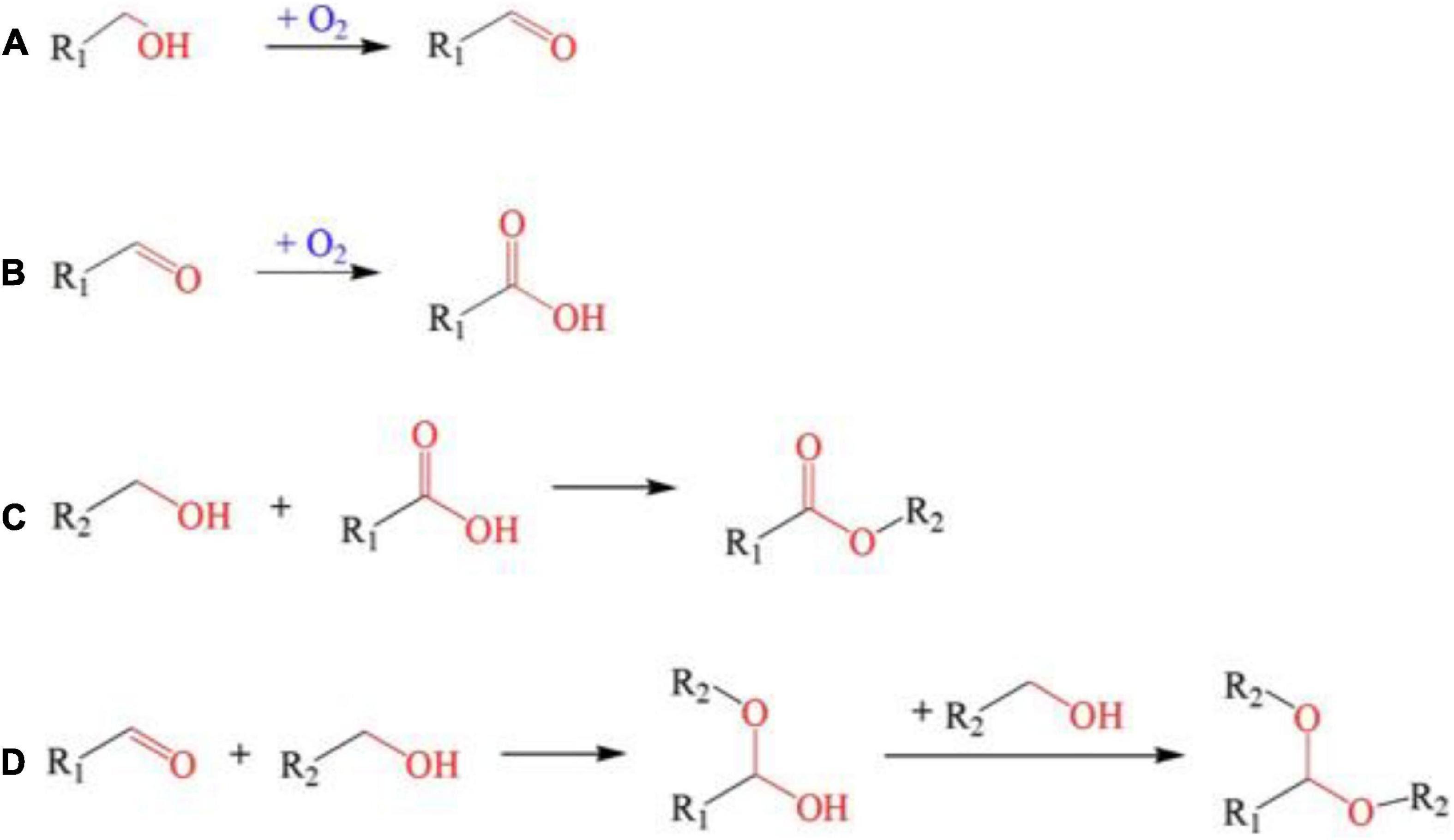
Figure 5. The reactions promoted by PEF treatment. (A) Alcohols oxidized into aldehydes; (B) aldehydes oxidized to acids; (C) Esterification reaction; (D) Polymerization reaction of alcohols and aldehydes could condensate into acetal. Reprinted from Ma et al. (97).
Fatty acids
Moderate PEF markedly increased the fatty acid content of fermented wine because it enhanced the oxidation of ethanol to aldehydes and further to acids (80). Different moderate PEF treatments (6–24 kV/cm) and pulse numbers (100–300 pulses) with red wine resulted in the evolution of L-malic acid, citric acid, and succinic acid concentrations following similar trends to bottle aging (10). The variation trends of citric acid, oxalic acid, and succinic acid in naturally aged white wines, were similar to those of white wines treated by PEF (6 kV/cm, 100 pulses) (Table 1). The amount of L-malic acid in the wine treated by PEF of 6 kV/cm and 100 pulse numbers was near to that of bottle storage for 90 days. The phenomenon revealed that the application of external PEF affected the molecular structure and chemical reactions among the substances in wine (81). Similarly, the fatty acids such as isovaleric acid, hexanoic acid, and octanoic acid in Chinese rice wine treated with PEF (2 kV/cm, 0.5 Hz, 36 pulses) could reach the level of natural aging for 3 years (82).
It is noteworthy that the variation trend of different fatty acids after PEF treatment was significantly different. For example, PEF was able to decline the content of tartaric acid in white wine and then decreased more steeply with a further increment of PEF strength. This trend is consistent with the change in bottle storage and may be related to chemical reactions between tartaric acid and other components. High levels of PEF allow the rapid evolution of tartaric acids, forming caffeoyltartaric acid, and ρ-coumaroyl tartaric acid (10). Furthermore, high-intensity PEF may have some negative effects on the changes of fatty acids in fermented wine. Succinic acid, for example, is created as a by-product of the sucrose fermentation process in wine and enables the taster to perceive the acidity, bitterness and acidity of wine in high concentrations (83). Zeng et al. (81) reported that the succinic acid content was lower than that of the untreated group due to the conversion of succinate to ethyl succinate after PEF treatment at a lower intensity. However, high-intensity PEF treatment at 18 and 24 kV/cm significantly promoted the hydrolysis of diethyl succinate, and the content of succinic acid increased (10). Therefore, the change in fatty acids depends on the strength of PEF, and there may be an optimal treatment condition for different acids.
Free amino acids
The taste characteristics of free amino acids can be divided into several categories, which were MSG-like (Asp and Glu), sweet (Ala, Gly, Ser, and Thr), and bitter (Arg, His, Ile, Leu, Met, Phe, and Val), based on the study of Azevedo et al. (84). The sweet and MSG-like components of Shaoxing Huangjiu (Chinese rice wine) treated with PEF (2 kV/cm, 0.5 Hz, 36 pulses) could reach the level of aging for 3 years (82). In terms of bitter amino acids, the contents of Huangjiu treated with accelerated aging showed a general trend of decline (Table 1). Compared with the naturally aged Huangjiu, PEF treatment reduced the bitterness of the wine and tempered the natural flavor of Huangjiu. This result was consistent with the research of Xu et al. (16), which found that Asp, Glu, Gly, and Ser increased after PEF treatment (1.5 kV/cm, 1 Hz, 50 pulses) in jujube wine. Free amino acids are precursors of flavor compounds (11), these free amino acids may be highly correlated to the complex synthesis of flavor compounds, which is of great significance to the overall aroma of Chinese rice wine. It is worth noting that high-intensity PEF treatment may promote the Maillard reaction between sugar and amino acids in rice wine, thus reducing the content of bitter-type amino acids (44, 84, 85).
Polyphenols
The content of polyphenols in fermented wine after PEF treatment was increased, such as flavan-3-ols, flavonols, hydroxycinnamic acids, and their derivatives, which is due to the high concentration of precursors in PEF-wine (86). For example, high concentrations of tartrate esters are hydrolyzed into their corresponding free acids such as caffeic acid and coumaric acids, leading to an increase in hydroxyl cinnamic acid. The surge in hydroxybenzoic acids, particularly gallic acid, protocatechuic acid, and syringic acid, may have resulted from the cleavage of anthocyanins during wine storage (e.g., malvidin 3-O-glucoside may lead to the production of syringic acid) (87). However, PEF treatment may also lead to a reduction in the evolution of some specific phenolic substances during aging. For red wines treated with PEF at specific energy (49.40 kJ/kg), prolonged storage at 4°C significantly reduced the amount of 11 Phenolic compounds, including anthocyanins, flavonols, and stilbenes (80). As mentioned earlier, this reduction may be due to degradation, oxidation, and cleavage auto association, as well as co-pigmentation, polymerization, and formation of new pigments (88). Due to the high initial anthocyanin content throughout the winemaking process, the level is still above that of naturally aged wines, despite the loss of large amounts of anthocyanin during storage. Moreover, the condensation reaction between (+)-catechin and acetaldehyde was enhanced by the PEF treatment (Figure 5). With the increase of PEF intensity ranging from 0 to 50 kV/cm, the decrease rate of (+)-catechin increased obviously. It was reported that at 40 kV/cm, the content of (+)-catechin after 31.12 ms reaction was roughly equivalent to the control group for 62.23 ms reaction without PEF treatment. Meanwhile, PEF treatment could significantly reduce the activation energy of the condensation reaction from 41.59 to 28.98 kJ/mol (89). Similarly, polyphenol flavan-3-ol monomers catechin and epicatechin promoted polymerization to form tannins under PEF treatment (4 kV/cm, 100 μs, 6.2 kJ/kg), which was similar to naturally aged Grenache wines (13).
Esters composition
Esters are the main source of aroma in wine (90) and PEF treatment can promote the esterification reaction in fermented wine (Table 1). The reaction rate and product concentration of the esterification reaction increase with the increase of electric field intensity. The effect of PEF (0–35 kV/cm, 1.0 kHz, 30 μs) on ethanol esterification of propionate was investigated by Lin et al. (91), while ethyl propionate had a distinctive fruity aroma. The content of ethyl propionate produced by PEF treatment was 1.30, 1.80, and 1.9 times higher than that without PEF treatment when the reaction temperature was 20°C and the field strength was 6.6, 13.3, and 20 kV/cm, respectively. Similarly, ethyl lactate possessed a distinctive rum, fruit and cream aroma. When 20 kV/cm PEF was applied and the reaction temperature was 25, 30, and 35°C, the yield of ethyl lactate was 1.7, 1.8, and 1.4 times of the sample without PEF (92). In addition, PEF can reduce the activation energy of esterification under certain conditions. When the electric field intensity is 13.3 kV/cm, the activation energy of esterification is 62.85 kJ/mol, which is 18.4% lower than the 77.05 kJ/mol of the control sample. In practical application, Shen et al. (82) treated Chinese rice wine samples with PEF (2 kV/cm, 0.5 Hz, 36 pulses) to explore the effect of PEF on the aroma substances of rice wine. The oxidation and esterification reaction could be promoted and the ethanol content would be greatly reduced in the PEF-treated Chinese rice wine. Fatty acid ethyl esters treated with PEF (2 kV/cm) could reach the level of natural aging for 3 years, especially in medium-chain (C6–C12) and long-chain (C13–C18) fatty acid ethyl esters. Among them, ethyl 2-hydroxypropionate, ethyl butyrate, ethyl valerate, and ethyl palmitate increased most significantly (82). The technical principle of PEF promoting aging is to use the energy provided by high-voltage to change the wine body into a strong oxidation state and accelerate a series of reaction processes such as oxidation-reduction and esterification (Figure 5).
Unpleasant substances
Pulsed electric field treatment can reduce the concentration of some irritant volatile substances below the reported odor threshold, potentially having a positive effect on overall wine perception. The fatty acids such as octanoic acid, capric acid, butyric acid, and 3-methylbutyric acid significantly decreased in the presence of PEF (11, 22 kJ/kg) (14). The concentrations of the last three compounds were lower than the odor threshold (15, 10, and 3 mg/L, respectively) after PEF treatment, which could cause a cheese-like, rancid and pungent odor at high concentrations (93). In addition to fatty acids, the concentrations of some volatile phenols (such as 4-vinylphenol and 4-vinylguaiacol) were also significantly reduced by PEF processing. The presence of these two compounds in white wine and red wine is due to enzymatic decarboxylation of cinnamic acids by yeasts and is generally considered a defect in wine because they have unpleasant smells similar to drugs and paints (94). Most notably, excessive fusel oils which are a mixture of higher alcohols in freshly wine will make wine bitter, spicy, and rough taste. Appropriate high voltage PEF treatment (5–20 kV/cm) can promote the oxidation of fusel oil in wine, so that it can transform into aldehydes or acids, resulting in a decrease and corresponding reduction of fresh wine irritation (95). Similarly, some researchers pretreated jujube pulp with PEF (1.5 kV/cm, 10 μs, 0.1 kHz) and found that within a certain range, along with the increase of PEF treatment time, the methanol content in the fermented jujube wine decreased significantly, and the maximum reduction reached 30.7% compared with the control group after 140 min of treatment (43).
Challenges and future trends
In previous studies, the potential of PEF in the raw material extraction, sterilization and aging of fermented wine has been extensively explored in laboratory studies, and gradually applied in pilot-scale and semi-industrial production. Nevertheless, further investigations should be carried out for some controversial results about the application of PEF in fermented wine.
For commercial application, PEF needs to achieve breakthroughs in the core components of equipment such as high-power supply and intelligent control systems. The focus should be on the applicability of PEF for broad-spectrum processing of different materials such as high conductivity or high viscosity, the uniformity under the action of the physical field, and the controllability of the processing temperature. At the same time, the stability, processing capacity and production efficiency of PEF system should be greatly improved to realize the large-scale application, continuous processing and intelligent control of PEF in the fermented wine industry. Moreover, since the electrode is in direct contact with the wine, the electrochemical reaction at the interface may cause corrosion and migration of the electrode material, resulting in the flavor of the PEF-treated wine being affected. Some researchers even tasted metallic in some PEF-treated samples (15). Therefore, it is necessary to develop more suitable or more durable electrode materials to replace the commonly used stainless steel electrode.
On the other hand, the principle of accelerated aging of fermented wine after PEF treatment and the optimal application parameters of PEF are still unclear. The complex reactions of multiple compounds occur under the action of PEF, and flavor in wine is affected by the combination of all the compounds, which means that some trace substances may have a unique effect on flavor. It is difficult to capture the effects of trace substances in conventional analysis methods, making it difficult for researchers to detect subtle changes caused by PEF. More importantly, for specific compounds, such as phenols, alcohols, acids, esters, and other aroma components, the effect of PEF is not all positive. The effects of PEF on some desired substances have disparate trends for different fermented wines. However, most current research on PEF mainly focus on the application of pre-maceration and the evolution of phenolic components during red wine aging while other kinds of fermented wine are rarely reported. Furthermore, various aging conditions such as container material and aging temperature, give the wine a kaleidoscope of styles during aging. PEF treatment alone cannot completely achieve the complex aroma and taste of the natural aging process. Therefore, combining PEF technology with other processing technologies such as ultrasound, microwave, and micro-oxygenation to obtain fermented wines that are closer to natural aging may be a direction worth exploring.
Conclusion
Research on the applicability of PEF in the fermented wine industry suggests that PEF can not only shorten the maceration time of brewing raw materials and promote the extraction of main functional components, but also enhance the color of fermented wines, inactivate spoilage microorganisms, and accelerate the formation of aroma substances during the aging process. Additionally, appropriate PEF treatment can reduce the levels of some unpleasant substances, especially fusel oil in freshly fermented wines. Furthermore, some laboratory and semi-industrial studies on the application of PEF technology have achieved the expected results. However, it is worth noting that there are still some bottlenecks that need to be solved urgently, such as the development of corrosion-resistant electrodes, high-power supplies, and intelligent control systems, which hinder the large-scale industrial application of PEF technology in the fermented wine industry.
Author contributions
YF: writing—original draft, software, formal analysis, and visualization. TY: writing—review and editing and validation. YZ: conceptualization and data curation. AZ: investigation and resources. LG: writing—review and editing and investigation. DN: funding acquisition, supervision, writing—review and editing, resources, and project administration. All authors contributed to the article and approved the submitted version.
Funding
This work was supported by the National Natural Science Foundation of China (32102133), the Natural Science Foundation of Guangxi Province (2021JJA130374), and the Scientific Research Staring Foundation of Hainan Medical University (XRC202129).
Conflict of interest
The authors declare that the research was conducted in the absence of any commercial or financial relationships that could be construed as a potential conflict of interest.
Publisher’s note
All claims expressed in this article are solely those of the authors and do not necessarily represent those of their affiliated organizations, or those of the publisher, the editors and the reviewers. Any product that may be evaluated in this article, or claim that may be made by its manufacturer, is not guaranteed or endorsed by the publisher.
References
1. Setford PC, Jeffery DW, Grbin PR, Muhlack RA. Mathematical modelling of anthocyanin mass transfer to predict extraction in simulated red wine fermentation scenarios. Food Res Int. (2018) 121:705–13. doi: 10.1016/j.foodres.2018.12.044
2. Petrozziello M, Torchio F, Piano F, Giacosa S, Ugliano M, Bosso A, et al. Impact of increasing levels of oxygen consumption on the evolution of color, phenolic, and volatile compounds of Nebbiolo wines. Front Chem. (2018) 6:137. doi: 10.3389/fchem.2018.00137
3. Dong ZY, Liu Y, Xu M, Zhang TH, Ren H, Liu W, et al. Accelerated aging of grape pomace vinegar by using additives combined with physical methods. J. Food Process Eng. (2020) 43:e13398. doi: 10.1111/jfpe.13398
4. de-Villiers A, Alberts P, Tredoux AGJ, Nieuwoudt HH. Analytical techniques for wine analysis: an African perspective; a review. Anal Chim Acta. (2012) 730:2–23. doi: 10.1016/j.aca.2011.11.064
5. Niu D, Zeng XA, Ren EF, Xu FY, Li J, Wang MS, et al. Review of the application of pulsed electric fields (PEF) technology for food processing in China. Food Res Int. (2020) 137:109715. doi: 10.1016/j.foodres.2020.109715
6. Zhang SM, Sun LZ, Ju HP, Bao ZJ, Zeng XA, Lin SY. Research advances and application of pulsed electric field on proteins and peptides in food. Food Res Int. (2021) 139:109914. doi: 10.1016/j.foodres.2020.109914
7. Aadil RM, Zeng XA, Ali A, Zeng F, Farooq MA, Han Z, et al. Influence of different pulsed electric field strengths on the quality of the grapefruit juice. Int J Food Sci Technol. (2015) 50:2290–6. doi: 10.1111/ijfs.12891
8. Arshad RN, Adul-Malek Z, Munir A, Buntat Z, Ahmad MH, Jusoh YMM, et al. Electrical systems for pulsed electric field applications in the food industry: an engineering perspective. Trends Food Sci Technol. (2020) 104:1–13. doi: 10.1016/j.tifs.2020.07.008
9. Arshad RN, Abdul-Malek Z, Roobab U, Munir MA, Naderipour A, Qureshi MI, et al. Pulsed electric field: a potential alternative towards a sustainable food processing. Trends Food Sci Technol. (2021) 111:43–54. doi: 10.1016/j.tifs.2021.02.041
10. Wang XQ, Su HN, Zhang QH, Yang PP. The effects of pulsed electric fields applied to red and white wines during bottle ageing on organic acid contents. J Food Sci Tech Mys. (2015) 52:171–80. doi: 10.1007/s13197-013-0984-0
11. Krivoruchko A, Nielsen J. Production of natural products through metabolic engineering of Saccharomyces cerevisiae. Curr Opin Biotech. (2015) 35:7–15. doi: 10.1016/j.copbio.2014.12.004
12. Zhang ZH, Wang LH, Zeng XA, Han Z, Brennan CS. Non-thermal technologies and its current and future application in the food industry: a review. Int J Food Sci Tech. (2019) 54:1–13. doi: 10.1111/ijfs.13903
13. Maza MA, Martínez JM, Cebrián G, Sánchez-Gimeno AC, Camargo A, Álvarez I, et al. Evolution of polyphenolic compounds and sensory properties of wines obtained from grenache grapes treated by pulsed electric fields during aging in bottles and in oak barrels. Foods. (2020) 9:542. doi: 10.3390/foods9050542
14. Comuzzo P, Marconi M, Zanella G, Querze M. Pulsed electric field processing of white grapes (cv. Garganega): effects on wine composition and volatile compounds. Food Chem. (2018) 264:16–23. doi: 10.1016/j.foodchem.2018.04.116
15. Yang NN, Huang K, Lyu C, Wang JP. Pulsed electric field technology in the manufacturing processes of wine, beer, and rice wine: a review. Food Control. (2016) 61:28–38. doi: 10.1016/j.foodcont.2015.09.022
16. Xu LF, Tang ZS, Wen QH, Zeng XA, Brennan C, Niu D. Effects of pulsed electric fields pretreatment on the quality of jujube wine. Int J Food Sci Tech. (2019) 54:3109–17. doi: 10.1111/ijfs.14226
17. Buckow R, Ng S, Toepfl S. Pulsed electric field processing of orange juice: a review on microbial, enzymatic, nutritional, and sensory quality and stability. Compr Rev Food Sci Food Saf. (2013) 12:455–67. doi: 10.1111/1541-4337.12026
18. Niu D, Ren EF, Li J, Zeng XA, Li SL. Effects of pulsed electric field-assisted treatment on the extraction, antioxidant activity and structure of naringin. Sep Purif Technol. (2021) 265:118480. doi: 10.1016/j.seppur.2021.118480
19. Zhang ZH, Han Z, Zeng XA, Wang MS. The preparation of Fe-glycine complexes by a novel method (pulsed electric fields). Food Chem. (2017) 219:468–76. doi: 10.1016/j.foodchem.2016.09.129
20. Gabric D, Barba F, Roohinejad S, Gharibzahedi SMT, Radojcin M, Putnik P, et al. Pulsed electric fields as an alternative to thermal processing for preservation of nutritive and physicochemical properties of beverages: a review. J. Food Process Eng. (2018) 41:e12638. doi: 10.1111/jfpe.12638
21. Barba FJ, Brianceau S, Turk M, Boussetta N, Vorobiev E. Effect of alternative physical treatments (ultrasounds, pulsed electric fields, and high-voltage electrical discharges) on selective recovery of bio-compounds from fermented grape pomace. Food Bioprocess Tech. (2015) 8:1139–48. doi: 10.1007/s11947-015-1482-3
22. Niu D, Wang LH, Zeng XA, Wen QH, Brennan CS, Tang ZS, et al. Effect of ethanol adaption on the inactivation of Acetobacter sp. by pulsed electric fields. Innov Food Sci Emerg Technol. (2019) 52:25–33. doi: 10.1016/j.ifset.2018.11.009
23. Bhat ZF, Morton JD, Mason SL, Bekhit AEA. Pulsed electric field operates enzymatically by causing early activation of calpains in beef during ageing. Meat Sci. (2019) 153:144–51. doi: 10.1016/j.meatsci.2019.03.018
24. Wiktor A, Sledz M, Nowacka M, Chudoba T, Witrowa-Rajchert D. Pulsed electric field pretreatment for osmotic dehydration of apple tissue: experimental and mathematical modeling studies. Drying Technol. (2014) 32:408–17. doi: 10.1080/07373937.2013.834926
25. Sanelle VW, Silva FVM, Farid MM. Pulsed electric field treatment of red wine: inactivation of Brettanomyces and potential hazard caused by metal ion dissolution. Innov Food Sci Emerg Technol. (2019) 52:57–65. doi: 10.1016/j.ifset.2018.11.001
26. Arcena MR, Leong SY, Then S, Hochberg M, Sack M, Mueller G, et al. The effect of pulsed electric fields pre-treatment on the volatile and phenolic profiles of Merlot grape musts at different winemaking stages and the sensory characteristics of the finished wines. Innov Food Sci Emerg Technol. (2021) 70:102698. doi: 10.1016/j.ifset.2021.102698
27. Shinde GP, Kumar R, Reddy KR, Nadanasabhapathi S, Semwal AD. Impact of pulsed electric field processing on reduction of benzylpenicillin residue in milk. J Environ Health Sci Eng. (2021) 19:1143–51. doi: 10.1007/s40201-021-00680-3
28. Wang MS, Wang SN. Study of the mechanism of wine aging accelerated by frequency conversion electric field. Farm Products Process. (2021) 4:16–9. doi: 10.16693/j.cnki.1671-9646(X).2021.04.004
29. Delsart C, Cholet C, Ghidossi R, Grimi N, Gontier E, Geny L, et al. Effects of pulsed electric fields on Cabernet Sauvignon grape berries and on the characteristics of wines. Food Bioprocess Tech. (2014) 7:424–36. doi: 10.1007/s11947-012-1039-7
30. Nabi BG, Mukhtar K, Arshad RN, Radicetti E, Tedeschi P, Shahbaz MU, et al. High-pressure processing for sustainable food supply. Sustainability. (2022) 13:13908. doi: 10.3390/su132413908
31. Ranjha MMAN, Kanwal R, Shafique B, Arshad RN, Irfan S, Kieliszek M, et al. A critical review on pulsed electric field: a novel technology for extraction of phytoconstituents. Molecules. (2021) 26:4893. doi: 10.3390/molecules26164893
32. Al Daccache M, Koubaa M, Salameh D, Vorobiev E, Maroun RG, Louka N. Control of the sugar/ethanol conversion rate during moderate pulsed electric field-assisted fermentation of a Hanseniaspora sp. strain to produce low-alcohol cider. Innov Food Sci Emerg Technol. (2020) 59:102258. doi: 10.1016/j.ifset.2019.102258
33. Li XL, Zhang ZH, Qi XH, Li L, Zhu J, Brennan CS, et al. Application of nonthermal processing technologies in extracting and modifying polysaccharides: a critical review. Compr Rev Food Sci Food Saf. (2021) 20:4367–89. doi: 10.1111/1541-4337.12820
34. Xia EQ, Deng GF, Guo YJ, Li HB. Biological activities of polyphenols from grapes. Int J Mol Sci. (2010) 11:622–46. doi: 10.3390/ijms11020622
35. Bindon KA, Kassara S, Smith PA. Towards a model of grape tannin extraction under wine-like conditions: the role of suspended mesocarp material and anthocyanin concentration. Aust J Grape Wine Res. (2017) 23:22–32. doi: 10.1111/ajgw.12258
36. Cerpa-Calderon FK, Kennedy JA. Berry integrity and extraction of skin and seed proanthocyanidins during red wine fermentation. J Agric Food Chem. (2008) 56:9006–14. doi: 10.1021/jf801384v
37. Lopez-Giral N, Gonzalez-Arenzana L, Gonzalez-Ferrero C, Lopez R, Santamaria P, Lopez-Alfaro I, et al. Pulsed electric field treatment to improve the phenolic compound extraction from Graciano, Tempranillo and Grenache grape varieties during two vintages. Innov Food Sci Emerg Technol. (2015) 28:31–9. doi: 10.1016/j.ifset.2015.01.003
38. Clodoveo ML, Dipalmo T, Rizzello CG, Corbo F, Crupi P. Emerging technology to develop novel red winemaking practices: an overview. Innov Food Sci Emerg Technol. (2016) 38:41–56. doi: 10.1016/j.ifset.2016.08.020
39. Leong SY, Burritt DJ, Oey I. Evaluation of the anthocyanin release and health-promoting properties of Pinot Noir grape juices after pulsed electric fields. Food Chem. (2016) 196:833–41. doi: 10.1016/j.foodchem.2015.10.025
40. Segade SR, Pace C, Torchio F, Giacosa S, Gerbi V, Rolle L. Impact of maceration enzymes on skin softening and relationship with anthocyanin extraction in wine grapes with different anthocyanin profiles. Food Res Int. (2015) 71:50–7. doi: 10.1016/j.foodres.2015.02.012
41. Galanakis CM, Kotanidis A, Dianellou M, Gekas V. Phenolic content and antioxidant capacity of Cypriot wines. Czech J Food Sci. (2015) 33:126–36. doi: 10.17221/335/2014-CJFS
42. Teusdea AC, Bandici L, Kordiaka R, Bandici GE, Vicas SI. The effect of different pulsed electric field treatments on producing high quality red wines. Not Bot Horti Agrobot Cluj Napoca. (2017) 45:540–7. doi: 10.15835/nbha45210890
43. Li J, Meng J, Zeng XA, Li XJ. Effects of pulsed electric field-assisted extraction on the quality of jujube wine. Liquor Mak Sci Tech. (2019) 5:61–4. doi: 10.13746/j.njkj.2018316
44. El Darra N, Rajha HN, Ducasse MA, Turk MF, Grimi N, Maroun RG, et al. Effect of pulsed electric field treatment during cold maceration and alcoholic fermentation on major red wine qualitative and quantitative parameters. Food Chem. (2016) 213:352–60. doi: 10.1016/j.foodchem.2016.06.073
45. El Darra N, Grimi N, Maroun RG, Louka N, Vorobiev E. Pulsed electric field, ultrasound, and thermal pretreatments for better phenolic extraction during red fermentation. Eur Food Res Technol. (2013) 236:47–56. doi: 10.1007/s00217-012-1858-9
46. Ricci A, Parpinello GP, Banfi BA, Olivi F, Versari A. Preliminary study of the effects of pulsed electric field (PEF) treatments in wines obtained from early-harvested sangiovese grapes. Beverages. (2020) 6:34. doi: 10.3390/beverages6020034
47. Lopez N, Puertolas E, Hernandez-Orte P, Alvarez I, Raso J. Effect of a pulsed electric field treatment on the anthocyanins composition and other quality parameters of Cabernet Sauvignon freshly fermented model wines obtained after different maceration times. LWT-Food Sci Technol. (2009) 42:1225–31. doi: 10.1016/j.lwt.2009.03.009
48. Puértolas E, Saldana G, Condón S, Álvarez I, Raso J. Evolution of polyphenolic compounds in red wine from Cabernet Sauvignon grapes processed by pulsed electric fields during aging in bottle. Food Chem. (2010) 119:1063–70. doi: 10.1016/j.foodchem.2009.08.018
49. Ducasse MA, Canal-Llauberes RM, de Lumley M, Williams P, Souquet JM, Fulcrand H, et al. Effect of macerating enzyme treatment on the polyphenol and polysaccharide composition of red wines. Food Chem. (2010) 118:369–76. doi: 10.1016/j.foodchem.2009.04.130
50. Lopez N, Puertolas E, Condon S, Alvarez I, Raso J. Application of pulsed electric fields for improving the maceration process during vinification of red wine: influence of grape variety. Eur Food Res Technol. (2008) 227:1099–107. doi: 10.1007/s00217-008-0825-y
51. Rinaldi A, Picariello L, Soares S, Brandão E, de Freitas V, Moio L, et al. Effect of oxidation on color parameters, tannins, and sensory characteristics of Sangiovese wines. Eur Food Res Technol. (2021) 247:2977–91. doi: 10.1007/s00217-021-03851-6
52. Castellanos ER, Jofre VP, Fanzone ML, Assof MV, Catania AA, Diaz-Sambueza AM, et al. Effect of different closure types and storage temperatures on the color and sensory characteristics development of Argentinian Torrontes Riojano white wines aged in bottles. Food Control. (2021) 130:108343. doi: 10.1016/j.foodcont.2021.108343
53. Ilona G, Ewa K, Andrzej C. Impact of pulsed electric field on the colour of wine made from grapes Marechal Foch variety. Proceedings of the 2018 Applications of Electromagnetics in Modern Techniques and Medicine (PTZE). Raclawice: (2018). p. 33–6.
54. Puértolas E, Saldana G, Álvarez I, Raso J. Experimental design approach for the evaluation of anthocyanin content of rosé wines obtained by pulsed electric fields. Influence of temperature and time of maceration. Food Chem. (2011) 126:1482–7. doi: 10.1016/j.foodchem.2010.11.164
55. Davaux, F, Leroy JB, Royant L, Marchand S. Increased diffusion kinetics of red and white grape skin compounds by pulsed electric fields. BIO Web Conf. (2019) 12:02008. doi: 10.1051/bioconf/20191202008
56. Han G, Ugliano M, Currie B, Vidal S, Diéval J, Waterhouse AL. Influence of closure, phenolic levels and microoxygenation on Cabernet Sauvignon wine composition after 5 years’ bottle storage. J Sci Food Agric. (2015) 95:36–43. doi: 10.1002/jsfa.6694
57. Jackson RS. 18-Shelf life of wine. In: Kilcast D, Subramaniam P editors. Book Food and Beverage Stability and Shelf Life. Sawston: Woodhead Publishing (2011). p. 540–70.
58. Marquez A, Serratosa MP, Merida J. Influence of bottle storage time on colour, phenolic composition and sensory properties of sweet red wines. Food Chem. (2014) 146:507–14. doi: 10.1016/j.foodchem.2013.09.103
59. Arapitsas P, Perenzoni D, Guella G, Mattivi F. Improving the phloroglucinolysis protocol and characterization of sagrantino wines proanthocyanidins. Molecules. (2021) 26:1087. doi: 10.3390/molecules26041087
60. Su HN, Huang WD, Zhan JC, Wang XQ. Effect of high-voltage pulsed electric field on proanthocyanidins content in freshly brewed dry red wine. Food Sci. (2010) 31:39–43.
61. Martorell P, Querol A, Fernaández-Espinar MT. Rapid identification and enumeration of saccharomyces cerevisiae cells in wine by real-time PCR. Appl Environ Microb. (2005) 71:6823–30. doi: 10.1128/AEM.71.11.6823-6830.2005
62. Marta SR, Kamal G, Amaury TR, Aantonio LG, Fulgencio MI. Control of native spoilage yeast on dealcoholized red wine by preservatives alone and in binary mixtures. J Food Sci. (2017) 82:2128–33. doi: 10.1111/1750-3841.13817
63. Guerrero RF, Cantos-Villar E. Demonstrating the efficiency of sulphur dioxide replacements in wine: a parameter review. Trends Food Sci Tech. (2015) 42:27–43. doi: 10.1016/j.tifs.2014.11.004
64. Milani EA, Alkhafaji S, Silva FVM. Pulsed electric field continuous pasteurization of different types of beers. Food Control. (2015) 50:223–9. doi: 10.1016/j.foodcont.2014.08.033
65. Gonzalez-Arenzana L, Portu J, Lopez R, Lopez N, Santamaria P, Garde-Cerdan T, et al. Inactivation of wine-associated microbiota by continuous pulsed electric field treatments. Innov Food Sci Emerg Technol. (2015) 29:187–92. doi: 10.1016/j.ifset.2015.03.009
66. Sanelle VW, Farid MM, Silva FVM. SO2, high pressure processing and pulsed electric field treatments of red wine: effect on sensory, Brettanomyces inactivation and other quality parameters during one year storage. Innov Food Sci Emerg Technol. (2018) 48:204–11. doi: 10.1016/j.ifset.2018.06.016
67. Jackson RS. 8-post-fermentation treatments and related topics. 4th ed. In: Jackson RS editor. Wine Science. Cambridge, MA: Academic Press (2014). p. 535–676.
68. Abca EE, Evrendilek GA. Processing of red wine by pulsed electric fields with respect to quality parameters. J.Food Proces Preserv. (2014) 39:758–67. doi: 10.1111/jfpp.12285
69. Puértolas E, López N, Condón S, Raso J, Alvarez I. Pulsed electric fields inactivation of wine spoilage yeast and bacteria. Int J Food Microbiol. (2009) 130:49–55. doi: 10.1016/j.ijfoodmicro.2008.12.035
70. Huang K, Lin Y, Liu DL, Ling G, Wang JP. Modeling of yeast inactivation of PEF-treated Chinese rice wine: effects of electric field intensity, treatment time and initial temperature. Food Res Int. (2013) 54:456–67. doi: 10.1016/j.foodres.2013.07.046
71. Davaux F, Leroy JB, Royant L. Microbiological stabilization of wines by use of pulsed electric fields. BIO Web Conf. (2019) 15:02001. doi: 10.1051/bioconf/20191502001
72. Mañas P, Pagán R. Microbial inactivation by new technologies of food preservation. J Appl Microbiol. (2005) 98:1387–99. doi: 10.1111/j.1365-2672.2005.02561.x
73. Puligundla P, Pyun YR, Mok C. Pulsed electric field (PEF) technology for microbial inactivation in low-alcohol red wine. Food Sci Biotechnol. (2018) 27:1691–6. doi: 10.1007/s10068-018-0422-1
74. Yi CW, Wu CD, Xu W, Chu JY, Lu CX. Study on sterilization by high voltage pulsed electric fields shock wave. High Voltage Eng. (2007) 33:109–11. doi: 10.13336/j.1003-6520.hve.2007.02.035
75. Lebleux M, Abdo H, Coelho C, Basmaciyan L, Albertin W, Maupeu J, et al. New advances on the Brettanomyces bruxellensis biofilm mode of life. Int J Food Microbiol. (2020) 318:108464. doi: 10.1016/j.ijfoodmicro.2019.108464
76. Niu D, Wang QY, Ren EF, Zeng XA, Wang LH, He TF, et al. Multi-target antibacterial mechanism of eugenol and its combined inactivation with pulsed electric fields in a hurdle strategy on Escherichia coli. Food Control. (2019) 106:106742. doi: 10.1016/j.foodcont.2019.106742
77. Lyu C, Huang K, Yang NN, Wang HJ, Wang JP. Combination of thermosonication and pulsed electric fields treatments for controlling Saccharomyces cerevisiae in Chinese rice wine. Food Bioproc Tech. (2016) 9:1854–64. doi: 10.1007/s11947-016-1769-z
78. Gonzalez-Arenzana L, Lopez-Alfaro I, Gutierrez AR, Lopez N, Santamaria P, Lopez R. Continuous pulsed electric field treatments’ impact on the microbiota of red Tempranillo wines aged in oak barrels. Food Biosci. (2019) 27:54–9. doi: 10.1016/j.fbio.2018.10.012
79. Hu WZ, Li M, Hao WY, Guo DX, Zhu RY, Shi Y, et al. Research progress on aging technology of fruit wine. China Brew. (2021) 40:1–4. doi: 10.11882/j.issn.0254-5071.2021.02.001
80. Arcena MR, Leong SY, Hochberg M, Sack M, Mueller G, Sigler J, et al. Evolution of volatile and phenolic compounds during bottle storage of merlot wines vinified using pulsed electric fields-treated grapes. Foods. (2020) 9:443. doi: 10.3390/foods9040443
81. Zeng XA, Yu SJ, Zhang L, Chen XD. The effects of AC electric field on wine maturation. Innov Food Sci Emerg Technol. (2008) 9:463–8. doi: 10.1016/j.ifset.2008.03.002
82. Shen C, Zhu H, Zhu W, Zhu Y, Peng Q, Elsheery NI, et al. The sensory and flavor characteristics of Shaoxing Huangjiu (Chinese rice wine) were significantly influenced by micro-oxygen and electric field. Food Sci Nutr. (2021) 9:6006–19. doi: 10.1002/fsn3.2531
83. Kritsunankul O, Pramote B, Jakmunee J. Flow injection on-line dialysis coupled to high performance liquid chromatography for the determination of some organic acids in wine. Talanta. (2009) 79:1042–9. doi: 10.1016/j.talanta.2009.03.001
84. Azevedo MS, Seraglio SKT, Rocha G, Balderas CB, Piovezan M, Gonzaga LV, et al. Free amino acid determination by GC-MS combined with a chemometric approach for geographical classification of bracatinga honeydew honey (Mimosa scabrella Bentham). Food Control. (2017) 78:383–92. doi: 10.1016/j.foodcont.2017.03.008
85. Hui M, Qiu C, Zhang H, Tian Q, Zhou T, Chen H. Study on the correlation between color and browning nutrient contents of Laohuzhou rice wine. J Henan Univ Tech. (2018) 39:15–6. doi: 10.16433/j.cnki.issn1673-2383.2018.02.003
86. Puértolas E, López N, Condón S, Álvarez I, Raso J. Potential applications of PEF to improve red wine quality. Trends Food Sci Tech. (2010) 21:247–55. doi: 10.1016/j.tifs.2010.02.002
87. Oliveira CM, Barros AS, Ferreira ACS, Silva AMS. Influence of the temperature and oxygen exposure in red port wine: a kinetic approach. Food Res Int. (2015) 75:337–47. doi: 10.1016/j.foodres.2015.06.024
88. Avizcuri JM, Sáenz-Navajas MP, Echávarri JF, Ferreira V, Fernández-Zurbano P. Evaluation of the impact of initial red wine composition on changes in color and anthocyanin content during bottle storage. Food Chem. (2016) 213:123–34. doi: 10.1016/j.foodchem.2016.06.050
89. Zhao D, Zeng XA, Sun DW, Liu D. Effects of pulsed electric field treatment on (+)-catechin–acetaldehyde condensation. Innov Food Sci Emerg Technol. (2013) 20:100–5. doi: 10.1016/j.ifset.2013.07.007
90. Hu K, Jin GJ, Mei WC, Li T, Tao YS. Increase of medium-chain fatty acid ethyl ester content in mixed H. uvarum/S. cerevisiae fermentation leads to wine fruity aroma enhancement. Food Chem. (2018) 239:495–501. doi: 10.1016/j.foodchem.2017.06.151
91. Lin ZR, Zeng XA, Yu SJ. Effect of pulsed electric field on esterification of propionic acid and ethanol. Sci Tech Food Ind. (2013) 34:140–3. doi: 10.13386/j.issn1002-0306.2013.04.052
92. Zeng XA, Liu XY. Effect of pulsed electric field on esterification of lactic acid with ethanol. J South China Univ Tech. (2011) 39:127–31. doi: 10.3969/j.issn.1000-565X.2011.12.022
93. Tao YS, Zhang L. Intensity prediction of typical aroma characters of cabernet sauvignon wine in Changli County (China). LWT-Food Sci Technol. (2010) 43:1550–6. doi: 10.1016/j.lwt.2010.06.003
94. Gao Y, Tian Y, Liu D, Li Z, Zhang XX, Li JM, et al. Evolution of phenolic compounds and sensory in bottled red wines and their co-development. Food Chem. (2015) 172:565–74. doi: 10.1016/j.foodchem.2014.09.115
95. Liu XJ, Yin YG, Fan SM, Zhu C. Effect of higher alcohols in wine treated with high voltage pulsed electric field. J Shenyang Agric Univ. (2007) 2:250–2. doi: 10.3969/j.issn.1000-1700.2007.02.031
96. Mahesha MP, Marianne NL, Francisco JB. 4-Pulsed electric field (PEF) as an efficient technology for food additives and nutraceuticals development. In: Barba FJ, Parniakov O, Wiktor A editors. Book Pulsed Electric Fields to Obtain Healthier and Sustainable Food for Tomorrow. Cambridge, MA: Academic Press (2020). p. 65–99.
Keywords: pulsed electric field, fermented wine, mechanism, aging, application
Citation: Feng Y, Yang T, Zhang Y, Zhang A, Gai L and Niu D (2022) Potential applications of pulsed electric field in the fermented wine industry. Front. Nutr. 9:1048632. doi: 10.3389/fnut.2022.1048632
Received: 19 September 2022; Accepted: 14 October 2022;
Published: 02 November 2022.
Edited by:
Monica Trif, Centre for Innovative Process Engineering, GermanyReviewed by:
Lina Cheng, Guangdong Academy of Agricultural Sciences, ChinaVeronica Sanda Chedea, Research Station for Viticulture and Enology Blaj (SCDVV Blaj), Romania
Rai Naveed Arshad, University of Technology Malaysia, Malaysia
Copyright © 2022 Feng, Yang, Zhang, Zhang, Gai and Niu. This is an open-access article distributed under the terms of the Creative Commons Attribution License (CC BY). The use, distribution or reproduction in other forums is permitted, provided the original author(s) and the copyright owner(s) are credited and that the original publication in this journal is cited, in accordance with accepted academic practice. No use, distribution or reproduction is permitted which does not comply with these terms.
*Correspondence: Debao Niu, happyndb@gxu.edu.cn
†These authors have contributed equally to this work