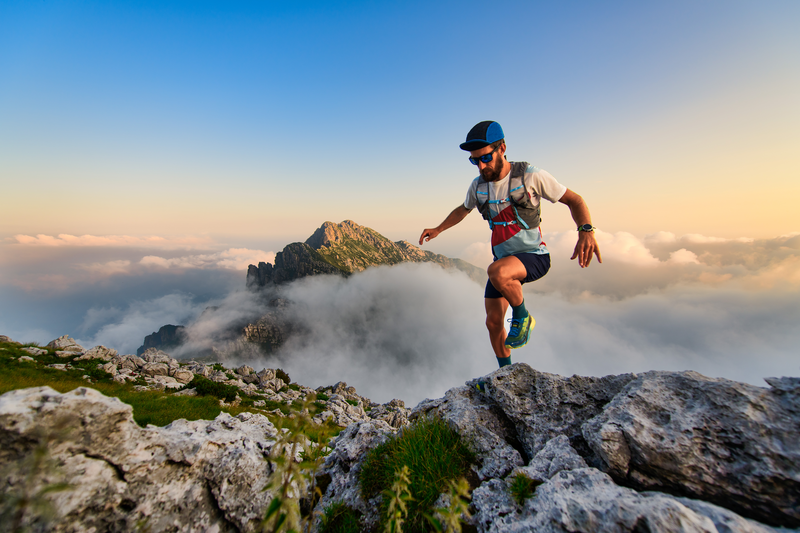
95% of researchers rate our articles as excellent or good
Learn more about the work of our research integrity team to safeguard the quality of each article we publish.
Find out more
ORIGINAL RESEARCH article
Front. Nutr. , 04 November 2022
Sec. Clinical Nutrition
Volume 9 - 2022 | https://doi.org/10.3389/fnut.2022.1042300
This article is part of the Research Topic Nutrition and Oral Biology in Health and Disease View all 6 articles
Background: Heavy metal(loid)s are frequently detected in vegetables posing potential human health risks, especially for those grown around mining areas. However, the oral bioaccessibility and gingival cytotoxicity of heavy metals in wild vegetables remain unclear.
Methods: In this study, we assessed the total and bioaccessible Cr, As, Cd, Pb, and Ni in four wild vegetables from mining areas in Southwest China. In addition, the cytotoxicity and underlying mechanisms of vegetable saliva extracts on human gingival epithelial cells (HGEC) were studied.
Results: The Plantago asiatica L. (PAL) showed the highest bioaccessible Cr, As, Cd, and Pb, while the greatest bioaccessible Ni was in Taraxacum mongolicum (TMM). The Pteridium aquilinum (PAM), Chenopodium album L. (CAL), and TMM extracts decreased cell viability, induced apoptosis, caused DNA damage, and disrupted associated gene expressions. However, PAL extracts which have the highest bioaccessible heavy metals did not present adverse effects on HGEC, which may be due to its inhibition of apoptosis by upregulating p53 and Bcl-2.
Conclusion: Our results indicated that polluted vegetable intake caused toxic effects on human gingiva. The heavy metals in vegetables were not positively related to human health risks. Collectively, both bioaccessibility and toxic data should be considered for accurate risk assessment.
Heavy metal(loid)s are wildly distributed in the environment, such as soil, and plants (1). Anthropogenic activities are the main contributors to heavy metal(loid)s in the environment (2). Smelting and mining operations are the most important anthropogenic sources of heavy metal(loid)s. Mining of polymetallic ores associated with sulfide minerals, lead–zinc, and copper can release Cr, As, Cd, Pb, and Ni into the environment (3, 4), which may result in their accumulation in the surrounding plants such as wild vegetables.
Wild vegetables are uncultivated but edible plants and distributed around the world. By supplying the body with protein, minerals, vitamins, and certain hormone precursors, wild vegetables play an important role in the human daily diet, especially in some developing countries such as China, Russia, and Thailand (5). Also, some of them provide important health benefits because of their nutritional and pharmacological characteristics (6). Plantago asiatica L. and Taraxacum mongolicum are traditional medicinal and representative wild vegetables in China for their variously beneficial functions, such as anti-oxidative, anti-inflammatory, and anti-microbial properties. In addition, Pteridium aquilinum and Chenopodium album L. are popular wild vegetables as well due to affluent nutrients such as polyunsaturated fatty acids, vitamin C, and carotene (7–9). Since the wild vegetables are uncultivated by humans and the heavy metal(loid)s in them are not regulated and monitored, consuming the wild vegetables that grew up on contaminated sites would lead to human health risks (4). In addition, the bioaccessibility of heavy metal(loid)s was employed to accurately assess exposure health risks as an in vitro assay by predicting the in vivo bioavailable fractions of the heavy metal(loid)s (10, 11).
Among exposure pathways, oral ingestion is an important contributor. The oral cavity is the original phase of the alimentary system, which contains various organs, such as teeth, tongue, and salivary glands. Ingesting heavy-metal(loid)-contaminated foods could lead to acute toxicities or chronic accumulations in human tissues and contribute to certain diseases (12). For instance, periodontitis is a representative inflammatory disease in the gingival tissues (13). Gingival epithelial cells play important roles in maintaining oral immunologic homeostasis and protecting oral organs from inflammatory damage (14). Ingestion of heavy-metal(loid)-contaminated wild vegetables may expose gingival epithelial cells to heavy metal(loid)s, especially during the chewing procedure. As such, the adverse effects of heavy metal(loid)s on human gingival epithelial cells and the underlying molecular mechanisms are still unclear. However, heavy metal(loid)s have been demonstrated to cause cytotoxicity, induce apoptosis and DNA damage, and alter related genes expressions (e.g., Caspase-3, p53, and GADD45α) to human oral mucosa cells, gastric adenocarcinoma cells, and colonic epithelial cells (15–17).
As one of the known vegetable kingdoms, Yunnan has abundant plant resources such as wild vegetables. Residents consume wild vegetables purchased from markets or collected by themselves, especially in spring. To evaluate the oral health risk of heavy metal(loid)s consumption of wild vegetables from mining areas, in this study, we investigated the pollution levels of Cr, As, Cd, Pb, and Ni in four common edible wild vegetables as well as their corresponding growing soils from mining areas in Yunnan, Southwest China. Also, the toxic effects of wild vegetable extracts on human gingival epithelial cells were assessed by analyzing cell morphology, viability, apoptosis, intracellular DNA damage, and the expressions of related genes. Overall, this study aims to provide a refining health risk assessment method of the wild vegetable extracts including heavy metal(loid)s on human gingiva via ingestion exposure.
The study area is an industrial district in the central region of Yunnan Province, Southwest China, which as a site for Cu mining and smelting (Figure 1), it is influenced by a subtropical plateau monsoon climate, holding an annual average temperature of 21.2°C, the average annual precipitation is 560.9 mm with a relative humidity of 76%. The rainy season comes in May every year, the plants will have a better living environment since then. The Cu mining and smelting sites had more than 2,000 years, which caused intermittent water and soil loss, along with discharged/emitted wastewater, solid wastes, and dust. Heavy metal(loid)s would be accumulated in soils and plants surrounding the mines. However, there are few studies on the pollution status and health risk of heavy metal(loid)s in local wild edible vegetables.
Four sampling sites (S1–S4) were selected in the vicinity of the mines in Xiaojiang River Basin, Dongchuan District, Kunming city, Yunnan province, Southwest China. And four common types of local wild edible vegetables (eight samples) including Pteridium aquilinum (PAM), Plantago asiatica L. (PAL), Chenopodium album L. (CAL), and Taraxacum mongolicum (TMM), as well as their corresponding growing soils (eight samples) were collected from S1–S4 in June 2021. All wild vegetable samples were washed three times with distilled water and dried to constant weight at 75°C, then crushed with a porcelain mortar and pestle, and kept in a refrigerator before analyses. All soil samples were air-dried and grounded to pass through a 100-mesh nylon sieve before analyses.
The total contents of heavy metal(loid)s in soils were analyzed by an X-ray fluorescence spectrometer (XRF, E-max 500). Due to XRF with a high limit of detection, the total and bioaccessible concentrations of heavy metal(loid)s in wild vegetables were determined by inductively coupled plasma mass spectrometry (ICP-MS, ICAPQR, Thermo Fisher Scientific, USA) (18). For total contents, wild vegetable samples (0.10 g, dry weight) were digested with concentrated HNO3 (Guaranteed Reagent) and 30% H2O2 (Guaranteed Reagent), then analyzed by ICP-MS. Based on total concentrations of heavy metal(loid)s in all wild vegetables, we selected four wild vegetable samples for the following experiments.
Bioaccessible heavy metal(loid)s in wild vegetables were extracted using artificial saliva (pH = 7, Fusayama, Shanghai Lianshuo Biological Technology, China) (19). The compositions of the artificial saliva include NaCl, KCl, CaCl2, NaH2PO4, and urea. Briefly, wild vegetable samples (0.50 g, dry weight) were dissolved in 20 mL artificial saliva and extracted at 150 rpm and 37°C in an oscillator for 24 h. After that, each extract was centrifuged and the supernatant was passed through a 0.22 μm sterile syringe filter (Corning, NY, USA), and collected in two sterile tubes, respectively. The content of the supernatant in one tube was applied for ICP-MS analysis, while the supernatant in another tube was used for cell experiments.
Human gingival epithelial cells (HGEC) were purchased from the American type culture collection (Manassas, VA, USA). HGEC were grown in Dulbecco's Modified Eagle Medium (DMEM) supplemented with 10% fetal bovine serum (FBS) and 1% penicillin-streptomycin (PS) in a humidified atmosphere with 5% CO2 at 37°C, and the media was substituted with fresh medium every alternate day.
Before each exposure, the logarithmic growth phase HGEC was trypsinized and reseeded into 6/24/96-well plates overnight to allow cell attachment for use. Each wild vegetable extract was mixed with DMEM 1:4 to obtain the exposure solution. The diluted extracts were added to the 6/24/96-well plates and incubated for 24 h, with a blank artificial saliva solution as the control.
To determine the impacts of extracts on HGEC proliferation, HGEC were cultured in a 96-well plate after overnight incubation at the density of 8 × 103 cells/100 μL/well. After exposure to the extracts for 24 h, cellular morphology was recorded by an inverted microscope (TS-100, Nikon, Japan). Simultaneously, a cell counting kit-8 assay kit (CCK-8, Yi Fei Xue Biotechnology, China) was employed to analyse cell proliferation. To calculate cell viability, the absorbance was measured at 450 nm with a microplate reader (Molecular Devices LLC, USA).
Annexin V-FITC/PI apoptosis assay kit was used for cell apoptosis analysis. In brief, HGEC were seeded in 6-well plates (1 × 106 cells per well) with DMEM for 24 h. After that, the spent DMEM was replaced with the exposure solution and treated for 24 h. After the exposures, cells were harvested and washed with cold phosphate buffer saline (PBS) three times and then immersed in 500 μL of PBS binding buffer (containing 5 μL Annexin V-FITC and 5 μL of PI-staining solution) for 15 min at room temperature in the dark. Approximately 1 × 104 cells were loaded with a CyFlow® Cube 6 Flow cytometer (Sysmex Partec, Germany) to assess cell apoptosis, and then analyzed by Flow Jo Version 10.0.6 software (BD Biosciences, USA).
Direct immunofluorescence staining was employed to assay the DNA damage of HGEC induced by extracts. Briefly, HGEC were replated into 24-well plates at 5 × 104 cells/well for 1 day and then exposed to extracts for 24 h at 37°C. Cells were rinsed three times with PBS, fixed by 4% poly-formaldehyde for 30 min, and permeabilized by 10% Triton X-100 for 15 min. After being washed three times with PBS, cells were blocked with 1% bovine serum albumin (BSA) for 60 min. Subsequently, cells were incubated overnight at 4°C with the rabbit monoclonal antibody anti-γ-H2AX (ab81299, Abcam, USA). After being maintained at room temperature for 1 h, cells were immersed in PBS for 5 min, then incubated by Goat Anti-Rabbit IgG (H+L) Fluor488-conjugated (Affinity Biosciences, USA) at room temperature for another 1 h. Last, the cells had a 10 min dark incubation at 37°C with 4', 6-diamidino-2-phenylindole (DAPI, Service bio, China) for nuclear counterstaining. Immunofluorescent images were observed and photographed with an inverted microscope system (IX73, Olympus, Japan).
To further investigate the mechanisms of cell apoptosis and DNA damage, the associated regulatory gene expression levels were estimated. After each exposure, the total RNA of HGEC was extracted using the total RNA isolation kit (Yi Fei Xue Biotechnology, China). The concentration and quality of RNA were determined using a UV–Vis Spectrophotometer (Q5000, Quawell, USA). The cDNA was synthesized from total RNA by the commercial reverse transcription kit. The quantitative real-time polymerase chain reaction (qRT-PCR) was performed to detect related gene expression levels (Bax, Bcl-2, p53, p21, GADD45α, Caspase-3, Caspase-8, and Caspase-9) by applying SYBR Green qPCR Master Mix and Roche LightCycler 480II Real-Time PCR system (Roche, Swiss). The reaction cycle condition started at 95°C for 15 min, followed by 40 cycles for 15 s, then maintained at 60°C for 1 min, and β-Actin was utilized as an internal reference. The data were calculated by the 2−ΔΔCT method (20), with the primer sequences being listed in Table 1.
Reagent blank, artificial saliva solution, and certified reference materials (GBW07405 and GBW10015a, Institute of Geophysical & Geochemical Exploration, China) were used to ensure quality control and assurance. The recovery rates were all above 80%. All the samples had triplicate determinations to ensure precise results. The accuracy of the measurements is assured within 10% RSD. ICP-MS detection limits of Cr, As, Cd, Pb, and Ni are 0.0002, 0.0002, 0.0003, 0.0001, and 0.002 mg/kg, respectively. XRF detection limits of Cr, As, Cd, Pb, and Ni are 5, 0.2, 0.05, 0.8, and 1 mg/kg, respectively. Statistical analysis was conducted by GraphPad Prism Version 8.0 software (GraphPad Software LLC, CA, USA). Data are presented as the mean ± standard deviation, and differences among exposure groups and the control group were analyzed by one-way ANOVA at p < 0.05.
Total concentrations of Cr, As, Cd, Pb, and Ni (mg/kg, dry weight) in the wild vegetables were shown in Figure 2A. Generally, Ni or Pb had the highest concentrations among all heavy metal(loid)s in PAL (13.7 ± 0.83 mg/kg), TMM (3.99 ± 1.49 mg/kg), CAL (3.24 ± 1.65 mg/kg), and PAM (1.42 ± 0.51 mg/kg). Interestingly, Pearson correlation analyses indicated that Ni contents in wild vegetables were negatively correlated with those in soils (r = −0.99, p < 0.05). These results were consistent with the concentrations found in cabbages that grew up in the same areas, and atmospheric deposition of the sampling site probably increased the absorption of metal(loid)s in the overground part of wild vegetables, which affected the results (21, 22). Cr, As, and Cd revealed varied levels in different wild vegetables, with the lowest concentrations of Cr (0.19 ± 0.06 mg/kg) and As (0.13 ± 0.00 mg/kg) found in PAM, and Cd (0.31 ± 0.12 mg/kg) in CAL. The results also showed lower levels of Cr, Cd, and Pb in TMM compared with the previous study (23), which could be explained by the fact that lower contamination levels of the corresponding soils in the current study (Table 2). In addition, PAL was contaminated by the highest levels of Cr, As, Cd, Pb, and Ni among four wild vegetables, with average concentrations being 5.72 ± 0.61, 7.07 ± 0.48, 0.96 ± 0.14, 13.7 ± 0.83, and 6.20 ± 0.90 mg/kg, respectively. Cr and Cd levels were lower when compared with Abbasi et al. (6) study, but Pb was higher in our study, which may be attributed to the different industrial activities. In addition, PAL corresponding soil did not have the highest concentrations of metal(loid)s compared with the other three soils (Table 2), indicating that PAL easily accumulated the above-mentioned metal(loid)s, especially for As, which is consistent with previous studies (24, 25). Although the concentrations of As, Cd, Pb, and Ni in PAM corresponding soils were higher compared to the other three vegetable soils (Table 2), the levels of heavy metal(loid)s in PAM were lower than those in the others. The differences in metal(loid)s contents of wild vegetables and their corresponding soils may be caused by the different contents of metal(loid) in environmental matrices as well as uptake capacities and preferences of various plant species (2, 24, 26). Furthermore, bioaccumulation coefficients of metal(loid)s, soil physical and chemical characteristics, and environmental parameters also play important roles in the transportation and accumulation of heavy metal(loid)s from soils to plants (1, 27). In addition, the average concentrations of heavy metal(loid)s in wild vegetables exceeded the leafy vegetables regulatory limit (GB2762−2017, China; WHO/FAO, 2019).
Figure 2. Total (A) and bioaccessible (B) concentrations of heavy metal(loid)s in wild vegetables. Error bars represent the mean ± SD of values from triplicate experiments. Different letters indicate significant differences (p < 0.05).
Table 2. Total concentrations (mg/kg) of heavy metal(loid)sa in wild vegetables and their corresponding growing soils.
Bioaccessible concentrations of Cr, As, Cd, Pb, and Ni in wild vegetables were presented in Figure 2B. Among all the wild vegetables, the highest bioaccessible concentrations of Cr (0.04 ± 0.008 mg/kg), As (1.76 ± 0.13 mg/kg), Cd (0.045 ± 0.006 mg/kg), and Pb (0.03 ± 0.004 mg/kg) were found in PAL, consistent with the results of total concentrations. As a bioremediation plant, PAL can accumulate various heavy metal(loid)s (24). The highest bioaccessible Ni (1.47 ± 0.23 mg/kg) was found in TMM, which is consistent with a previous study (7). Especially, the bioaccessible Pb in PAL was reduced to 0.032 ± 0.003 mg/kg compared with its total concentration of 13.7 ± 0.83 mg/kg, similar to the other study (28). Since inorganic Pb (II) is the main lead speciation in food (29) and it is barely soluble in alkaline solutions, the soluble fraction of Pb in extracts (pH = 7.5–9.3) should be low. Although the bioaccessible concentrations of heavy metal(loid)s in wild vegetables were much lower than the total concentrations, we further conducted cytotoxicity experiments to investigate whether these bioaccessible extracts have adverse effects on HGEC.
The observations of cell morphology and viability are significant visualized indicators to evaluate cytotoxicity, which were widely employed to assay cell survival and proliferation (16, 30). Nevertheless, the impacts of bioaccessible metal(loid)s in vegetables on the morphology and viability of human gingival cells were not reported. Our previous study indicated gastric bioaccessible metals in cabbage did not cause the alteration of cell viability and morphology in human gastric adenocarcinoma cells (31). In this study, transformed cell morphologies were observed under all exposures excluded PAL, and cell viabilities were decreased across all exposures (Figures 3A–F). Specifically, HGEC exhibited varied levels of cell viability inhibition from 12 to 29% after being exposed to the extracts, especially after being exposed to TMM extracts. TMM extracts had the highest bioaccessible concentration of Ni among all vegetables (Figure 2B), and Ni was demonstrated to decrease cell viability and elicit cytotoxicity in oral mucosa cells and gingival fibroblasts (15, 32). Consistently, bioaccessible Ni was negatively correlated with the cell viability (r = −0.92, p < 0.05) in this study among all vegetables (Table 3).
Figure 3. Alterations of HGEC morphology and viability after being exposed to the extracts of wild vegetables for 24 h. Normal HGEC morphology in control (A; CK) and altered cell morphology and viability after exposures to the extracts of PAM (B), PAL (C), CAL (D), and TMM (E). Cell viability was determined by the CCK-8 assay kit and was normalized as the percentage of control (F). Typical cobblestone, round, or floated appearances of HGEC were clear in the control group and exposure groups (A–E) at 200 × magnification (bar = 100 μm). Error bars represent the mean ± SD of values from triplicate experiments. Different letters indicate significant differences (p < 0.05).
Table 3. Pearson correlation coefficients between wild vegetable bioaccessible metal(loid)s and various toxicity indexes (cell viability, apoptosis, and genes) in HGEC after exposures to four extracts for 24 h.
The changes in cellular morphology were consistent with the results of cell viability besides the exposure to PAL extract (Figures 3A–E). As one of the most important traditional Chinese herbs, PAL is rich in beneficial nutrients such as PAL polysaccharides, which could alleviate the cytotoxicity caused by environmental pollutants (8). We speculated PAL polysaccharides play an important role in mediating the extract-induced cytotoxicity of PAL. Specifically, the typical cobblestone and polygonal appearances of confluent monolayers were clear in the control group (Figure 3A) and the PAL exposure group (Figure 3C). However, HGEC emerged with loose and irregular morphologies along with increased floated and round cells under the exposures of PAM, CAL, and TMM extracts (Figures 3B,D,E), revealing cell deaths. Since these results cannot firmly confirm that bioaccessible heavy metal(loid)s are the causes of cell death, further experiments are crucial to elucidate the mechanisms of the observed cytotoxicity.
As a gene-controlled cell death under stress conditions, apoptosis plays an indispensable role in maintaining tissue homeostasis by eliminating cells, but disordered apoptosis induced by xenobiotics is a pathological phenomenon (33). Apoptosis of HGEC after being exposed to extracts for 24 h are shown in (Figure 4). In this study, Annexin V-FITC/PI double staining tests detected cell apoptosis after exposing HGEC to PAM, CAL, and TMM extracts (Figures 4C,E,F), which was consistent with the corresponding decreases in cell viability (Figure 3F) and changes in cell morphology (Figures 3B,D,E). Similarly, studies reported that heavy metal(loid)s in environmental matrices (e.g., dust, air particulate matter, and foods) caused cytotoxicity and induced apoptosis of human cell lines (34–36). Furthermore, the most elevated apoptotic ratio (39.7%) was observed under the exposure of CAL extract compared with the control group (1.82%), implicating CAL extract was more potent to induce cytotoxicity and apoptosis than that of other extracts (37). However, the PAL extract, which had the highest bioaccessible Cr, As, Cd, and Pb, did not decrease cell viability, change cell morphology, and induce cell apoptosis. It is possibly due to the protective effects of PAL polysaccharides, such as alleviating the cytotoxicity caused by environmental pollutants and inducing the maturation of dendritic cells (8, 38). Thus, we speculated PAL polysaccharides extracted by the artificial saliva may play pivotal roles in inhibiting PAL-extract-induced apoptosis. These results indicated the nutrients in the vegetables may also influence the contaminant-induced toxicity, thus the human health risk assessments simply based on the total concentrations of heavy metal(loid)s may not be accurate enough (39).
Figure 4. Apoptosis of HGEC after being exposed to extracts for 24 h. FL1 represents Annexin V-FITC staining and FL2 represents PI staining. HGEC populations (A; black circle) and apoptosis in the control group (B; CK). Apoptosis of HGEC after being exposed to PAM (C), PAL (D), CAL (E), and TMM (F) extracts: necrotic cells were shown in the Q1 quadrant, viable (late) apoptotic cells and non-viable (early) apoptotic cells were distributed in Q2 and Q3 quadrant, Q4 quadrant was living cells. The statistical graph showed cell apoptosis rate under different treatments (Q2 + Q3 quadrant) (G). Data were presented in mean ± SD. The different letters indicate significant differences (p < 0.05).
To further elucidate how extracts triggered HGEC apoptosis, a DNA damage assay was performed (40). The damage and repair of DNA play important roles in cell transformation and cell death in the human oral cavity (41), while DNA damage as a blockage in DNA replication could lead to cell apoptosis (42). Histone H2AX phosphorylation on serine 139 is an early signaling event triggered by DNA double-strand breaks. Thus, as the phosphorylated version of histone H2AX, γ-H2AX is a sensitive marker for DNA damage (43).
To further confirm our hypothesis that the observed HGEC apoptosis was caused by extract-induced DNA damage, we assessed DNA damages after the exposures to extracts by using immunofluorescence staining (Figure 5). The results showed that the exposure groups of PAM, CAL, and TMM extracts presented more H2AX phosphorylation focal points (Figures 5C,F,L,O) compared with that in the control group, while the level of DNA damages in the PAL exposure group and control group are similar (Figures 5C,I). These results indicated the extracts of PAM, CAL, and TMM induced DNA damage in HGEC, which is consistent with the results of cell apoptosis (Figure 4G), morphology (Figures 3B,D,E), and viability (Figure 3F).
Figure 5. Effects of extracts (PAM: D–F, PAL: G–I, CAL: J–L, and TMM: M–O) on γ-H2AX protein in HGEC after being exposed for 24 h. Background control (CK) was artificial saliva alone (A–C). The immunofluorescent photographs of the phosphorylation of histone H2AX in γ-H2AX-stained HGEC (Green: B,E,H,K,N) and the counterstained nuclei with DAPI (Blue: A,D,G,J,M) were captured. Blue dots were cell nuclei, green dots indicated DNA damage. The magnification was 60 × and the scale bar (A–O: yellow, located in the lower right corner) is 20 μm.
Moreover, PAM and CAL extracts induced more severe DNA damage compared with TMM extract (Figures 5F,L,O), even though TMM extract had higher bioaccessible Ni (Figure 2B). Although a previous study showed that Ni did not induce DNA damage in gingival cells (44), Hafez et al. (32) found that DNA damages of oral mucosa cells were correlated with the cellular Ni concentrations. Ni subsulfide and nickel oxides had also been demonstrated to induce DNA damage (45). In this study, the extracts with high Ni contents (exclude PAL) only slightly exhibited DNA damage on HGEC (Figures 2B, 5F,L,O). These inconsistent results could be attributed to the differences in cellular physiology as well as the constituents of the exposure and exposed systems (46, 47). As such, these comparisons further demonstrated that the cell apoptosis and DNA damages may not be correlated with the levels of heavy metal(loid)s in edible vegetables, but are also impacted by the other factors in each specific exposure scenario.
Measuring gene expression is an effective approach to evaluating the underlying mechanism of cell apoptosis (41, 48). Extrinsic (e.g., caspase-3, -8, and -9, Bcl-2 genes) and intrinsic (e.g., Bax, Bcl-2, caspase-9, p53 genes) related pathways were recognized as two primary modes of apoptosis (49, 50). Specifically, caspases play important roles in the initiation (caspase-8, -9, and -10) and effector (caspase-3, -6, and -7) phases of apoptosis. Furthermore, the Bcl-2 family of proto-oncogenes is the key factor to regulate apoptosis, involving the anti-apoptotic gene (Bcl-2) and pro-apoptotic gene (Bax) (49). Tumor suppressor gene p53 controls genomic integrity, cell proliferation, and cell death through complex signaling networks. Some studies also demonstrated that p53 is vital to induce cell apoptosis (51, 52). As important downstream genes of p53, GADD45α and p21 are involved in growth arrest and apoptosis (53).
To further explore the underlying molecular mechanism of HGEC apoptosis induced by extracts, we assessed the genotoxicity of these extracts by measuring the alterations of regulatory gene expressions in HGEC after each exposure. The results showed that after being exposed to the PAM extract for 24 h, the mRNA levels of Bax, Bcl-2, p21, GADD45α, Caspase-3, -8, -9 in HGEC were up-regulated from 1.6 to 6.6 folds (Figures 6A–F,H), while p53 was maintained at the same level (Figure 6G). This result explained the observations of HGEC apoptosis under PAM extract exposure (Figure 4C) since the activations of Caspase-3, -8, and−9 induce DNA damage and lead to cell apoptosis (15, 54). After being exposed to CAL extract, p21 and GADD45α were significantly up-regulated (p < 0.05), which indicated CAL-induced apoptosis was probably due to the induced DNA damage pathways. In the TMM extract exposure group, only p21 was up-regulated (Figure 6H), while Bax, Caspase-9, and p53 were significantly down-regulated (p < 0.05) (Figures 6A,D,G), and the rest of the measured genes being barely altered (Figures 6B,C,E,F). The up-regulation of the p21 gene usually causes growth arrest and promotes DNA damage, eventually leading to cell apoptosis (30). In contrast, the expression levels of all measured genes were up-regulated in the exposure group of PAL extract (Figures 6A–H) but did not lead to HGEC apoptosis (Figure 4D). It implicates certain chemicals in PAL extracts such as PAL polysaccharides restrained extract-induced apoptosis, which is consistent with the prior cytotoxicity results and previous studies (55, 56). In addition, Pearson correlation analyses between bioaccessible metal(loid)s in wild vegetables and gene expressions of HGEC after exposures were performed. We found bioaccessible As was positively correlated with the mRNA expression of p53 (r = 0.99, p < 0.05) (Table 3), indicating As induced HGEC apoptosis may relate to the expression of p53, consistent with a previous study (57). In contrast, bioaccessible Cd was negatively correlated with the mRNA expression of p53 (r = −0.96, p < 0.05) (Table 3), our study found that a low concentration of Cd accelerated human gastric epithelial cells proliferation, which may decrease the mRNA expression of p53 (16).
Figure 6. The mRNA expression levels of cell apoptosis regulatory genes (A: Bax, B: Bcl-2, C: Caspase-8, D: Caspase-9, E: Caspase-3, F: GADD45α, G: p53, and H: p21) in HGEC after being exposed to four extracts compared with the control group. The control group (CK) was treated by DMEM only. Results were represented as mean ± SD of triplicate experiments. The different letters represent significant differences at p < 0.05.
In brief, our results showed the observed HGEC apoptosis induced by the extracts may mainly attribute to the DNA damage pathways. Furthermore, higher expression levels of GADD45α and p21 genes showed DNA damage is the key pathway that induced the HGEC apoptosis under exposure to PAM and CAL extracts. However, the alterations of GADD45α expressions were inconsistent with the results of DNA damage, HGEC morphology, and HGEC viability under the exposures of PAL and TMM extracts, and polygenic regulation is probably the main reason for that inconsistency (43, 58). Overall, three extracts (PAM, CAL, and TMM) induced HGEC apoptosis and DNA damage as well as altered the expressions of p53, GADD45α, and p21 genes. These results demonstrated DNA damage may play an important role in the observed HGEC apoptosis induced by the three extracts.
In this study, Ni or Pb was predominant in all vegetable samples, with Plantago asiatica L. (PAL), Taraxacum mongolicum (TMM), Chenopodium album L. (CAL), and Pteridium aquilinum (PAM) being 13.7, 3.99, 3.24, and 1.42 mg/kg, respectively. PAL had the highest bioaccessible Cr, As, Cd, and Pb, while the greatest bioaccessible Ni was found in TMM. The PAM, CAL, and TMM extracts decreased cell viability and triggered cellular apoptosis. The bioaccessible Ni was negatively correlated with cell viability. Increased DNA damage and abnormal related mRNA expression were also observed. Interestingly, PAL extract with the highest bioaccessible heavy metal(loid)s did not correlate with higher cytotoxicity, indicating beneficial nutrients such as PAL polysaccharides may play an important role in mediating the extract-induced-cytotoxicity of PAL. However, it is not clear which chemical composition and cytotoxicity are causal, and only eight wild vegetable species were harvested in this study due to the season limitation, more samples from the same site or different species would be collected in our follow-up study to further validate our conclusions. Taken together, consuming wild vegetables from mining areas may cause adverse health effects on the human oral cavity. Both oral bioaccessibility and cytotoxicity data should be considered for accurate human risk assessments.
The original contributions presented in the study are included in the article/supplementary material, further inquiries can be directed to the corresponding author/s.
WT: conceptualization, investigation, formal analysis, and writing—original draft. PG: investigation, conceptualization, formal analysis, and writing—review and editing. D-PZ, M-YZ, C-CW, and J-JL: investigation, validation, and formal analysis. Z-XW and J-MW: data analysis. Y-YN: supervision and conceptualization. PX: project administration, supervision, conceptualization, funding acquisition, and writing—review and editing. All authors contributed to the article and approved the submitted version.
This work was supported in part by the Yunnan Agricultural Basic Research Joint Special Project (202101BD070001-023), the National Natural Science Foundation of China (41967026 and 21906134), the Yunnan Innovative Research Team (202005AE160017), the Natural Science Foundation of Hunan Province (2021JJ30479), Top Young Talents Project of National Forestry and Grassland Administration (2020132613), the Yunnan Fundamental Research Projects (2019FB014), the Yunnan Thousand Youth Talent Program (YNQR-QNRC-2018-049), the Open Project of Beijing Key Laboratory of Toxicological Research and Risk Assessment for Food Safety (KF-2020–01), and the Research Foundation of Yunnan Education Department (2022J0508, 2021Y231, and 2021Y237).
The authors declare that the research was conducted in the absence of any commercial or financial relationships that could be construed as a potential conflict of interest.
All claims expressed in this article are solely those of the authors and do not necessarily represent those of their affiliated organizations, or those of the publisher, the editors and the reviewers. Any product that may be evaluated in this article, or claim that may be made by its manufacturer, is not guaranteed or endorsed by the publisher.
1. Natasha, Shahid M, Khalid S, Bibi I, Bundschuh J, Khan Niazi N, et al. A critical review of mercury speciation, bioavailability, toxicity and detoxification in soil-plant environment: ecotoxicology and health risk assessment. Sci Total Environ. (2020) 711:134749. doi: 10.1016/j.scitotenv.2019.134749
2. Zhang J, Baralkiewicz D, Wang Y, Falandysz J, Cai C. Arsenic and arsenic speciation in mushrooms from China: a review. Chemosphere. (2020) 246:125685. doi: 10.1016/j.chemosphere.2019.125685
3. Li X, Zhang J, Gong Y, Liu Q, Yang S, Ma J, et al. Status of copper accumulation in agricultural soils across China (1985-2016). Chemosphere. (2020) 244:125516. doi: 10.1016/j.chemosphere.2019.125516
4. Nguyen TH, Sakakibara M, Sano S, Mai TN. Uptake of metals and metalloids by plants growing in a lead-zinc mine area, Northern Vietnam. J Hazard Mater. (2011) 186:1384–91. doi: 10.1016/j.jhazmat.2010.12.020
5. Chettri S, Manivannan S, Muddarsu VR. Nutrient and elemental composition of wild edible ferns of the Himalaya. Am Fern J. (2018) 108:95–106. doi: 10.1640/0002-8444-108.3.95
6. Abbasi AM, Iqbal J, Khan MA, Shah MH. Health risk assessment and multivariate apportionment of trace metals in wild leafy vegetables from Lesser Himalayas, Pakistan. Ecotoxicol Environ Saf. (2013) 92:237–44. doi: 10.1016/j.ecoenv.2013.02.011
7. Kovacik J, Bujdos M, Ketzer P, Babula P, Peterkova V, Krenn L. Dandelion is more tolerant to cadmium than to nickel excess. Chemosphere. (2019) 224:884–91. doi: 10.1016/j.chemosphere.2019.02.181
8. Li F, Huang D, Yang W, Liu X, Nie S, Xie M. Polysaccharide from the seeds of Plantago asiatica L. alleviates nonylphenol induced reproductive system injury of male rats via PI3K/Akt/mTOR pathway. J Funct Foods. (2020) 66:103828. doi: 10.1016/j.jff.2020.103828
9. Nekrasov EV, Svetashev VI. Edible far Eastern ferns as a dietary source of long-chain polyunsaturated fatty acids. Foods. (2021) 10:1220. doi: 10.3390/foods10061220
10. Guo J, Zhang Y, Liu W, Zhao J, Yu S, Jia H, et al. Incorporating in vitro bioaccessibility into human health risk assessment of heavy metals and metalloid (As) in soil and pak choi (Brassica chinensis L.) from greenhouse vegetable production fields in a megacity in Northwest China. Food Chem. (2022) 373:131488. doi: 10.1016/j.foodchem.2021.131488
11. Llorente-Mirandes T, Llorens-Muñoz M, Funes-Collado V, Sahuquillo À, López-Sánchez JF. Assessment of arsenic bioaccessibility in raw and cooked edible mushrooms by a PBET method. Food Chem. (2016) 194:849–56. doi: 10.1016/j.foodchem.2015.08.047
12. Gu B, Chen D, Yang Y, Vitousek P, Zhu YG. Soil-food-environment-health nexus for sustainable development. Research. (2021) 2021:9804807. doi: 10.34133/2021/9804807
13. Kawasaki H, Amano H. Antiinflammatory role of microRNA429 in human gingival epithelial cellsinhibition of IL8 production through direct binding to IKKbeta mRNA. Mol Med Rep. (2021) 24:581. doi: 10.3892/mmr.2021.12220
14. Kashiwagi Y, Takedachi M, Mori K, Kubota M, Yamada S, Kitamura M, et al. High glucose-induced oxidative stress increases IL-8 production in human gingival epithelial cells. Oral Dis. (2016) 22:578–84. doi: 10.1111/odi.12502
15. Buczko P, Szarmach I, Grycz M, Kasacka I. Caspase-3 as an important factor in the early cytotoxic effect of nickel on oral mucosa cells in patients treated orthodontically. Folia Histochem Cytol. (2017) 55:37–42. doi: 10.5603/FHC.a2017.0004
16. Wang K, Ma JY Li MY, Qin YS, Bao XC, Wang CC, et al. Mechanisms of Cd and Cu induced toxicity in human gastric epithelial cells: oxidative stress, cell cycle arrest and apoptosis. Sci Total Environ. (2021) 756:143951. doi: 10.1016/j.scitotenv.2020.143951
17. Yin N, Cai X, Du H, Zhang Z, Li Z, Chen X, et al. In vitro study of soil arsenic release by human gut microbiota and its intestinal absorption by Caco-2 cells. Chemosphere. (2017) 168:358–64. doi: 10.1016/j.chemosphere.2016.10.091
18. da Silva EB, Gao P, Xu M, Guan D, Tang X, Ma LQ. Background concentrations of trace metals As, Ba, Cd, Co, Cu, Ni, Pb, Se, and Zn in 214 Florida urban soils: Different cities and land uses. Environ Pollut. (2020) 264:114737. doi: 10.1016/j.envpol.2020.114737
19. Pappas RS, Stanfill SB, Watson CH, Ashley DL. Analysis of toxic metals in commercial moist snuff and alaskan iqmik. J Anal Toxicol. (2008) 32:281–91. doi: 10.1093/jat/32.4.281
20. Schmittgen TD, Livak KJ. Analyzing real-time PCR data by the comparative C(T) method. Nat Protoc. (2008) 3:1101–8. doi: 10.1038/nprot.2008.73
21. Kovacik J, Dudas M, Hedbavny J, Martonfi P. Dandelion Taraxacum linearisquameum does not reflect soil metal content in urban localities. Environ Pollut. (2016) 218:160–7. doi: 10.1016/j.envpol.2016.08.030
22. Zou L, Yang J, Cheng X, Wang W, Huang Q, Zhang X. Investigation of Farmland Soil and Chinese cabbage heavy metal pollution along banks of Xiaojiang in Dongchuan,Yunnan Province. Southwest China J Agri Sci. (2018) 31:754–8. doi: 10.16213/j.cnki.scjas.2018.4.019
23. Atikpo E, Okonofua ES, Uwadia NO, Michael A. Health risks connected with ingestion of vegetables harvested from heavy metals contaminated farms in Western Nigeria. Heliyon. (2021) 7:e07716. doi: 10.1016/j.heliyon.2021.e07716
24. Yang X, Mei Y, He J, Jiang R, Li Y, Li J. Comprehensive assessment for removing multiple pollutants by plants in bioretention systems. Chinese Sci Bull. (2014) 59:1446–53. doi: 10.1007/s11434-014-0200-2
25. Zuo TT, Jin HY, Zhang L, Liu YL, Nie J, Chen BL, et al. Innovative health risk assessment of heavy metals in Chinese herbal medicines based on extensive data. Pharmacol Res. (2020) 159:104987. doi: 10.1016/j.phrs.2020.104987
26. Fu J, Cui Y. In vitro digestion/Caco-2 cell model to estimate cadmium and lead bioaccessibility/bioavailability in two vegetables: the influence of cooking and additives. Food Chem Toxicol. (2013) 59:215–21. doi: 10.1016/j.fct.2013.06.014
27. Xu FF, Song J, Li YQ, Lai YF, Lin J, Pan JL, et al. Bioaccessibility and bioavailability adjusted dietary exposure of cadmium for local residents from a high-level environmental cadmium region. J Hazard Mater. (2021) 420:126550. doi: 10.1016/j.jhazmat.2021.126550
28. Saba D, Manouchehri N, Besancon S, El Samad O, Bou Khozam R, Nafeh Kassir L, et al. Bioaccessibility of lead in Dittrichia viscosa plants and risk assessment of human exposure around a fertilizer industry in Lebanon. J Environ Manage. (2019) 250:109537. doi: 10.1016/j.jenvman.2019.109537
29. Li J, Sun C, Zheng L, Jiang F, Yin X, Chen J, et al. Determination of lead species in algae by capillary electrophoresis-inductively coupled plasma-mass spectrometry. Chin J Anal Chem. (2016) 44:1659–64. doi: 10.1016/S1872-2040(16)60970-2
30. Xiang P, Wang K, Bi J, Li M, He RW, Cui D, et al. Organic extract of indoor dust induces estrogen-like effects in human breast cancer cells. Sci Total Environ. (2020) 726:138505. doi: 10.1016/j.scitotenv.2020.138505
31. Li MY, Qin YS, Wang CC, Wang K, Deng ZH, Xu WM, et al. Total and bioaccessible heavy metals in cabbage from major producing cities in Southwest China: health risk assessment and cytotoxicity. RSC Adv. (2021) 11:12306–14. doi: 10.1039/D1RA01440D
32. Hafez HS, Selim EM, Kamel Eid FH, Tawfik WA, Al-Ashkar EA, Mostafa YA. Cytotoxicity, genotoxicity, and metal release in patients with fixed orthodontic appliances: a longitudinal in-vivo study. Am J Orthod Dentofacial Orthop. (2011) 140:298–308. doi: 10.1016/j.ajodo.2010.05.025
33. Elmore S. Apoptosis: a review of programmed cell death. Toxicol Pathol. (2007) 35:495–516. doi: 10.1080/01926230701320337
34. Giani F, Masto R, Trovato MA, Malandrino P, Russo M, Pellegriti G, et al. Heavy metals in the environment and thyroid cancer. Cancers. (2021) 13:4052. doi: 10.3390/cancers13164052
35. Sun J, Yu J, Shen Z, Niu X, Wang D, Wang X, et al. Oxidative stress-inducing effects of various urban PM25 road dust on human lung epithelial cells among 10 Chinese megacities. Ecotoxicol Environ Saf. (2021) 224:112680. doi: 10.1016/j.ecoenv.2021.112680
36. Yuan Y, Wu Y, Ge X, Nie D, Wang M, Zhou H, et al. In vitro toxicity evaluation of heavy metals in urban air particulate matter on human lung epithelial cells. Sci Total Environ. (2019) 678:301–8. doi: 10.1016/j.scitotenv.2019.04.431
37. Jiang N, Wen H, Zhou M, Lei T, Shen J, Zhang D, et al. Low-dose combined exposure of carboxylated black carbon and heavy metal lead induced potentiation of oxidative stress, DNA damage, inflammation, and apoptosis in BEAS-2B cells. Ecotoxicol and Environ Saf. (2020) 206:111388. doi: 10.1016/j.ecoenv.2020.111388
38. Gao J, Zhang YN, Cui J, Zhang J, Ming Y, Hao Z, et al. A polysaccharide from the whole plant of Plantago asiatica L. enhances the antitumor activity of dendritic cell-based immunotherapy against breast cancer. Front Pharmacol. (2021) 12:678865. doi: 10.3389/fphar.2021.678865
39. Gao P. The exposome in the era of one health. Environ Sci Technol. (2021) 55:2790–9. doi: 10.1021/acs.est.0c07033
40. Norbury CJ, Zhivotovsky B. DNA damage-induced apoptosis. Oncogene. (2004) 23:2797–808. doi: 10.1038/sj.onc.1207532
41. Gao H, Prasad GL, Zacharias W. Combusted but not smokeless tobacco products cause DNA damage in oral cavity cells. Environ Toxicol Pharmacol. (2014) 37:1079–89. doi: 10.1016/j.etap.2014.03.022
42. Sarma P, Ramaiah MJ, Kamal A, Bhadra U, Bhadra MP. A novel bisindole-PBD conjugate causes DNA damage induced apoptosis via inhibition of DNA repair pathway. Cancer Biol Ther. (2014) 15:1320–32. doi: 10.4161/cbt.29705
43. Natale F, Rapp A, Yu W, Maiser A, Harz H, Scholl A, et al. Identification of the elementary structural units of the DNA damage response. Nat Commun. (2017) 8:15760. doi: 10.1038/ncomms15760
44. Lopez-Jornet P, Perrez FP, Calvo-Guirado JL, Ros-Llor I, Ramirez-Fernandez P. Metallic ion content and damage to the DNA in oral mucosa cells patients treated dental implants. J Mater Sci Mater Med. (2014) 25:1819–24. doi: 10.1007/s10856-014-5203-7
45. Shosuke Kawanishi S, Sumiko I, Nishino AK. Distinct mechanisms of oxidative dna damage induced by carcinogenic nickel subsulfide and nickel oxides. Environ Health Perspect. (2002) 110:789–91. doi: 10.1289/ehp.02110s5789
46. Patil S, Baeshen HA. Aqueous extract of tobacco induces mitochondrial potential dependent cell death and epithelial-mesenchymal transition in gingival epithelial cells. Saudi J Biol Sci. (2021) 28:4613–8. doi: 10.1016/j.sjbs.2021.04.068
47. Xiang P, Liu RY, Sun HJ, Han YH, He RW, Cui XY, et al. Molecular mechanisms of dust-induced toxicity in human corneal epithelial cells: water and organic extract of office and house dust. Environ Int. (2016) 92–3:348–56. doi: 10.1016/j.envint.2016.04.013
48. Gao H, Prasad GL, Zacharias W. Differential cell-specific cytotoxic responses of oral cavity cells to tobacco preparations. Toxicol In Vitro. (2013) 27:282–91. doi: 10.1016/j.tiv.2012.07.015
49. Pulido MD, Parrish AR. Metal-induced apoptosis: mechanisms. Mutat Res. (2003) 533:227–41. doi: 10.1016/j.mrfmmm.2003.07.015
50. Winter E, Chiaradia LD, Silva AH, Nunes RJ, Yunes RA, Creczynski-Pasa TB. Involvement of extrinsic and intrinsic apoptotic pathways together with endoplasmic reticulum stress in cell death induced by naphthylchalcones in a leukemic cell line: advantages of multi-target action. Toxicol In Vitro. (2014) 28:769–77. doi: 10.1016/j.tiv.2014.02.002
51. Aubrey BJ, Kelly GL, Janic A, Herold MJ, Strasser A. How does p53 induce apoptosis and how does this relate to p53-mediated tumour suppression? Cell Death Differ. (2018) 25:104–13. doi: 10.1038/cdd.2017.169
52. Hao Q, Chen J, Liao J, Huang Y, Larisch S, Zeng SX, et al. p53 induces ARTS to promote mitochondrial apoptosis Cell Death Dis. (2020) 12:204. doi: 10.1101/2020.05.14.096982
53. Zhang QZ, Wen F, Yang HL, Cao YY, Peng RG, Wang YM, et al. GADD45alpha alleviates the CDDP resistance of SK-OV3/cddp cells via redox-mediated DNA damage. Oncol Lett. (2021) 22:720. doi: 10.3892/ol.2021.12981
54. Zhao WJ Li X, Xu ZQ, Fang KM, Hong HC, Sun HJ, et al. Environmentally relevant concentrations of arsenic induces apoptosis in the early life stage of zebrafish. Ecotoxicol Environ Saf. (2021) 227:112883. doi: 10.1016/j.ecoenv.2021.112883
55. Fan W, Zhang B, Wu C, Wu H, Wu J, Wu S, et al. Plantago asiatica L. seeds extract protects against cardiomyocyte injury in isoproterenol- induced cardiac hypertrophy by inhibiting excessive autophagy and apoptosis in mice. Phytomedicine. (2021) 91:153681. doi: 10.1016/j.phymed.2021.153681
56. Kho MC, Park JH, Han BH, Tan R, Yoon JJ, Kim HY, et al. Plantago asiatica L. ameliorates puromycin aminonucleoside-induced nephrotic syndrome by suppressing inflammation and apoptosis. Nutrients. (2017) 9:386. doi: 10.3390/nu9040386
57. Ahamed M, Akhtar MJ, Alhadlaq HA. Co-exposure to SiO2 nanoparticles and arsenic induced augmentation of oxidative stress and mitochondria-dependent apoptosis in human cells. Int J Environ Res Public Health. (2019) 16:3199. doi: 10.3390/ijerph16173199
Keywords: wild vegetables, heavy metal(loid)s, oral bioaccessibility, gingival cytotoxicity, health risk
Citation: Tian W, Gao P, Zong D-P, Liu J-J, Zhang M-Y, Wang C-C, Wang Z-X, Wang J-M, Niu Y-Y and Xiang P (2022) The oral bioaccessibility and gingival cytotoxicity of metal(loid)s in wild vegetables from mining areas: Implication for human oral health. Front. Nutr. 9:1042300. doi: 10.3389/fnut.2022.1042300
Received: 12 September 2022; Accepted: 20 October 2022;
Published: 04 November 2022.
Edited by:
Elsa Lamy, University of Évora, PortugalReviewed by:
Arlette Setiawan, Universitas Padjadjaran, IndonesiaCopyright © 2022 Tian, Gao, Zong, Liu, Zhang, Wang, Wang, Wang, Niu and Xiang. This is an open-access article distributed under the terms of the Creative Commons Attribution License (CC BY). The use, distribution or reproduction in other forums is permitted, provided the original author(s) and the copyright owner(s) are credited and that the original publication in this journal is cited, in accordance with accepted academic practice. No use, distribution or reproduction is permitted which does not comply with these terms.
*Correspondence: You-Ya Niu, bml1eW91eWFAc2luYS5jb20=; Ping Xiang, eGlhbmdwaW5nQHN3ZnUuZWR1LmNu; cGluZ194aWFuZ0AxMjYuY29t
†These authors have contributed equally to this work
Disclaimer: All claims expressed in this article are solely those of the authors and do not necessarily represent those of their affiliated organizations, or those of the publisher, the editors and the reviewers. Any product that may be evaluated in this article or claim that may be made by its manufacturer is not guaranteed or endorsed by the publisher.
Research integrity at Frontiers
Learn more about the work of our research integrity team to safeguard the quality of each article we publish.