- 1Department of Agricultural Microbiology, Faculty of Agriculture, Zagazig University, Zagazig, Egypt
- 2Key Laboratory of Tropical Translational Medicine of Ministry of Education, School of Pharmacy, Hainan Medical University, Haikou, China
- 3Department of Food Science, Faculty of Agriculture, Zagazig University, Zagazig, Egypt
- 4Department of Biochemistry, Faculty of Agriculture, Zagazig University, Zagazig, Egypt
- 5Department of Soils and Water, Faculty of Agriculture, Fayoum University, Fayoum, Egypt
- 6Department of Clinical Laboratory Sciences, College of Applied Medical Sciences, Jouf University, Sakaka, Saudi Arabia
- 7Department of Hematology/Oncology, Yousef Abdulatif Jameel Scientific Chair of Prophetic Medicine Application, Faculty of Medicine, King Abdulaziz University, Jeddah, Saudi Arabia
- 8Department of Poultry Diseases, Faculty of Veterinary Medicine, Cairo University, Giza, Egypt
- 9Department of Veterinary Sciences, Faculty of Health Sciences, Higher Colleges of Technology, Al Ain, United Arab Emirates
- 10Department of Medicine and Infectious Diseases, Faculty of Veterinary Medicine, Cairo University, Giza, Egypt
- 11Department of Entomology, Faculty of Science, Ain Shams University, Cairo, Egypt
- 12Plant Production Department (Horticulture-Pomology), Faculty of Agriculture Saba Basha, Alexandria University, Alexandria, Egypt
- 13Department of Agricultural Microbiology, Faculty of Agriculture, Benha University, Benha, Egypt
- 14Department of Clinical Microbiology, Infection and Immunology, Umeå University, Umeå, Sweden
- 15Department of Radiation Sciences, Oncology, Umeå University, Umeå, Sweden
- 16Department of Poultry Diseases, Faculty of Agriculture, Zagazig University, Zagazig, Egypt
- 17Department of Biology, College of Science, United Arab Emirates University, Al Ain, United Arab Emirates
- 18Khalifa Center for Genetic Engineering and Biotechnology, United Arab Emirates University, Al Ain, United Arab Emirates
- 19Harry Butler Institute, Murdoch University, Murdoch, WA, Australia
The yellow polyphenolic pigment known as curcumin, originating from the rhizome of the turmeric plant Curcuma longa L., has been utilized for ages in ancient medicine, as well as in cooking and food coloring. Recently, the biological activities of turmeric and curcumin have been thoroughly investigated. The studies mainly focused on their antioxidant, antitumor, anti-inflammatory, neuroprotective, hepatoprotective, and cardioprotective impacts. This review seeks to provide an in-depth, detailed discussion of curcumin usage within the food processing industries and its effect on health support and disease prevention. Curcumin’s bioavailability, bio-efficacy, and bio-safety characteristics, as well as its side effects and quality standards, are also discussed. Finally, curcumin’s multifaceted uses, food appeal enhancement, agro-industrial techniques counteracting its instability and low bioavailability, nanotechnology and focused drug delivery systems to increase its bioavailability, and prospective clinical use tactics are all discussed.
Introduction
Medicinal herbs could be the best source for various medicines (1). Due to their different therapeutic properties, medicinal herbs have been considered by many researchers worldwide (2). In modern medicine, several studies have been conducted to find the potential effects of various extracts of medicinal herbs that have a pivotal role in the health of people and animals (3–5). Plant-based drugs may be much more appropriate in biochemical terms when compared to synthetic drugs. However, modern medicine does not essentially support natural products for medicinal uses (6–8), such as growth inhibitors of some tumors (9). Moreover, different compounds (including colchicine, vincristine, vinblastine, podophyllotoxin, and taxol) have been isolated from various herbs, and have been used against different types of tumors (10).
One of the most studied medicinal herbs is turmeric. Turmeric is the dried rhizome powder of the Curcuma longa plant, composed of many phytochemicals (11, 12). Concerning the approximate composition, turmeric is composed of water (80–90%), followed by carbohydrates (around 13%), proteins (2%), minerals (2%), and lipids (<1%) (13). Among the minor components of turmeric, curcuminoids have a central role and may compose up to 10% of dry turmeric powder. This category mainly comprises curcumin, dimethoxy-curcumin, and bisdemethoxycurcumin, which can compose 62–90, 9–23, and 0.3–14 mg/g of commercial turmeric products (extracts and powders), respectively. Additionally, more than 50 curcuminoids (such as bisabocurcumin, curcumalongin, cyclocurcumin, and terpecurcumin) have been identified in turmeric (14, 15), which produce the yellow color of turmeric.
While turmeric, one of the basic elements of curry powder, is used as a spice in the west, it has been used as a natural remedy in Asia for a long period, e.g., for the treatment of stomach and liver problems. Alternatively, turmeric, which entered Turkish cuisine in the 16th century, was used as a natural dye to give yellow color to the saffron rice dessert. Because of the dye substance’s resemblance to saffron, it is used in the production of zerde as a low-price alternative. Even though the first application of turmeric in history was as a dyestuff for fabrics and yarns, its current use for health purposes has largely surpassed any other application, including spices (16).
New uses of turmeric shifted from textile dying and ancient proposed medicinal properties to exploring potential health effects, including anti-carcinogenic, anti-inflammatory, anticoagulant, antimicrobial, and antioxidant impacts (17). The medicinal properties of turmeric over the centuries have had many proposed benefits, such as aiding in wound healing, allergy, asthma, sinusitis, hepatic, and heart diseases (18). Few studies have examined the whole turmeric root as an agent to help control inflammation or other health concerns. Turmeric is a healthy and safe space for reasonable consumption (19).
The most significant curcuminoid, to which the most healing properties of turmeric are attributed, is curcumin, which was first extracted from turmeric in 1815, and its molecular formula was discovered in 1910. Curcumin, with the molecular formula C21H20O6 and the chemical name diferuloylmethane, is the most considerable molecule isolated from plants that have been investigated in recent years (12). This molecule is inherently hydrophobic and does not dissolve in water but in substances such as dimethyl sulfoxide, acetone, ethanol, and oil (20). Turmeric contains about 3–8% curcumin (depending on the growing season). A dessert spoon of turmeric powder (an average of 3 g) will contain an average of 30–90 mg of curcumin, although other plant species also contain some curcumin (21). In various research and studies, turmeric’s antiseptic, anti-inflammatory and antioxidant properties have been proven and proposed as a complementary treatment for Alzheimer’s, diabetes, asthma, stomach ulcers, etc. (22). Over the few current decades, considerable studies have been conducted on curcumin due to its beneficial health properties, including potent antioxidant properties (23, 24), antimicrobial (25), anti-inflammation (26), anticancer effects (27), cardio-protectiveness (28), and hypoglycemic action (29).
In this review, to collect the data, we used keywords such as “turmeric,” “curcumin,” “antioxidant,” “anticancer,” “antimicrobial,” “bioavailability,” and “Food applications,” on the web of science, ekb.eg, and Google Scholar, which has a total of 294 articles between 2004 and 2023.
Despite numerous cells, culture studies indicating that low doses of curcumin were sufficient to exhibit its biological action, many animal and clinical studies revealed that a hefty dose of curcumin is necessary to trigger its full impact since its poor bioavailability restricts its bio-efficacy. Therefore, this review concentrates on the way to increase curcumin bioavailability and its impacts of turmeric and curcumin on human health, metabolism, mechanisms of action, and their usage limits and food applications.
Extraction of bioactive compounds from turmeric
Obtaining curcuminoid-rich extracts is a crucial step in maximizing the utilization of turmeric in meals. Numerous studies have assessed the impact of traditional and innovative extraction methods (Table 1). Curcumin is the most important of turmeric’s medicinal components and makes up between 1 and 7 percent of the root (30). In terms of traditional solvent extraction, the recovery of curcumin employing a 70% hydroethanolic solution reached 69.1% (31). Due to its limited water solubility, curcumin must be extracted using organic solvents. In this context, solvent composition plays a crucial role in maximizing the extraction yield, and ethanol and acetone are the solvents of choice. In terms of the technique to separate curcumin from turmeric, continuous Soxhlet extraction might be recommended as a first step to increase the recovery of curcumin (1.3 and 6.9 g/100 g) from turmeric (32).
Research utilizing innovative and environmentally friendly methods, such as water extraction, demonstrated high curcumin recovery yields (between 76.0 and 90.6%) to increase curcumin extraction’s efficacy (33). Concerning the influence of supercritical carbon dioxide (SC-CO2) on curcumin extraction, Wakte et al. (34) attained a recovery efficiency of 69.4% by utilizing ethanol as a co-solvent and maintaining an extraction temperature of 50°C.
Considering these technologies and methods of extracting curcumin from turmeric, both traditional and developing technologies can be applied to create curcumin-rich extracts. Considering the lengthy extraction periods to obtain the extracts using conventional technologies (agitation and Soxhlet) and the use of organic solvents (particularly acetone), the use of alternative technologies and approaches that produce extracts using a less harmful and toxic solvent in a shorter period can be of great value to increase the use of curcumin in scientific studies and commercial applications in the food and pharmaceutical industries (33, 35).
Increasing curcumin bioavailability
Low bioavailability of any pharmaceutical agent within the body is due to; (1) poor gastrointestinal absorption, (2) high rates of metabolism, (3) inactivity of metabolic products, and (4) rapid elimination and clearance (36). Because of its tautomeric structure, high-molecular-weight, and aromatic groups, curcumin is extremely hydrophobic and, therefore, only partially absorbed through the gastrointestinal epithelium (37). One of the first studies to report this constraint, identified virtually undetectable plasma curcumin levels following a 1 g/kg oral dose in rats. Once absorbed, the liver predominantly metabolizes curcumin to form glucuronide and sulfate conjugates, representing about 99% of plasma curcumin (38). These metabolites have been reported to have low bioactivity compared to free curcumin (39). Lastly, the brief half-life of curcumin plays a crucial role in its low bioavailability. In rats, orally delivered curcuminoids reach a peak plasma concentration at 0.83 ± 0.05 h, with an elimination half-life of 1.70 ± 0.58 h (40).
Several delivery techniques have been developed to overcome the pharmaco-kinetics predisposing to poor bioavailability of orally ingested curcumin, including adjuvants, nanoparticles, liposomes, and a self-nanoemulsifying drug delivery system (SNEDDS) (41). The in vivo response has been varied (41), with several products posing a risk for drug–drug interactions due to their inhibition of the P-glycoprotein and CYP3A4 systems (42).
Regardless, curcumin’s substantial limitations have not stopped scientists from researching and enhancing its potential. Rather, they have created greater scope for developing innovative solutions to address issues with the native form. Curcumin formulations have come about because of research to improve bioavailability, permeability, circulation, half-life, and withstand metabolic processes. These formulations admit chemical curcumin derivatives and analogs with metabolic adjuvants, nanoparticles, liposomes, micelles, nanostructured lipid carriers (NLC), and phospholipid complexes (43).
Phospholipid complexes appear to have been effectively released into the worldwide market among the formulations that emerged from Longvida (Verdure Sciences, Noblesville, IN, USA), and Meriva (Indena, Milan, Italy). Longvida® with the bioavailability of a solid lipid formulation containing roughly 80 mg of curcumin was observed to be four times better than that of curcumin alone. Daily therapy with 400 mg of Longvida® for 4 weeks in an aged population resulted in better cognitive capabilities (44). Meriva®, another proprietary curcumin formulation from Indena, is used to treat osteoarthritis, diabetes mellitus, and microangiopathy in a 1:2 weight ratio of curcumin and soy lecithin (44).
Compared to Meriva, normal curcuminoid combination absorption was 29-fold lower in clinical studies. However, the C95 formulation contained 95% curcuminoid powder, which increased bioavailability fivefold. The curcumin cyclodextrin complex (CCC) is said to have a 45-fold higher bioavailability than C95 (45). The curcuminoid phospholipid complex (CPC) demonstrated a 20-fold better than curcumin bioavailability and 30-fold higher than total curcuminoids (46). Furthermore, Zeng et al. (47) examined the effect of piperine pre-administration on oral curcumin bioavailability. In this investigation, rats were given 20 mg/kg piperine first, followed by 200 mg/kg curcumin at intervals of 0.5–8 h after piperine treatment. The pre-treatment with piperine before curcumin administration significantly increased curcumin oral bioavailability in all tested rats. Curcumin permeability rose 1.85-fold when quercetin and resveratrol were combined, suggesting that resveratrol and quercetin had a cumulative impact on curcumin absorption (48). Other recent trends to improve oral bioavailability of curcumin are presented in Figure 1. Below is some recent techniques to increase the curcumin bioavailability.
LipiSperse
LipiSperse® is a novel delivery system designed to improve crystalline lipophilic agent dispersion in aqueous environments. Lipophilic active ingredients provide challenges from a formulation and bioavailability perspective. Often, improving bioavailability leads to a decreased dynamic load in final formulations (49).
LipiSperse® is a mixture of surfactants, polar lipids, and solvents specifically chosen for their ability to embed into the lipophilic crystal structure of the active ingredient while keeping the hydrophilic head on the surface, increasing the hydration of the crystal by lowering the surface tension, which allows it to disperse in water. Once dispersed in water, LipiSperse® then goes on to prevent the crystals from agglomeration (49).
Employing adjuvants
Although curcumin has several benefits, many preclinical studies have demonstrated that it cannot be utilized to treat human disorders due to its low bioavailability. Utilizing adjuvants to block detoxifying enzymes shown in curcumin metabolism is one of the most important methods for enhancing the oral bioavailability of curcumin (50). Among adjuvants, black pepper piperine is one of the most effective boosters of curcumin bioavailability. The simultaneous administration of curcumin and piperine to humans or animals boosted the serum levels by more than a thousandfold (51). Additionally, epigallocatechin-3-gallate (EGCG) was utilized as an adjuvant to curcumin, increasing the bioavailability of curcumin by many orders of magnitude (52). However, the adjuvants are not restricted to the substances listed above.
Several adjuvants have been employed to enhance curcumin’s oral bioavailability for various therapeutic uses, including cancer. Recent research suggests that oral administration of curcumin and piperine for symptomatic COVID-19 therapy might dramatically reduce mortality and morbidity (53). The conjugation between piperine and curcumin may be a safe and natural option for preventing post-COVID symptoms. According to another study, with curcumin nanoparticles, prolonged topical administration and improved bioavailability of curcumin were obtained, including the possibility of skin discoloration (54). A mouse model organism was utilized to evaluate skin inflammation with or without ultraviolet-B radiation exposure and with or without curcumin encapsulation in coconut oil. After 24 h of incubation, the experimental setup treated with encapsulated curcumin had less skin reddening than the control group (54). Moreover, inflammatory cytokine analyses and histology of the encapsulated curcumin-exposed skin revealed less skin cell damage and reduced inflammation (markers) compared to the control and non-encapsulated groups (54). Curcumin in nanocapsule or unencapsulated form efficiently restores host-microbe interactions and gut homeostasis (55).
Curcumin nano formulation: Nanoparticles, nanocomposites, hydrogels
The nanoformulation of curcumin has been proven to have extensive therapeutic application (56). The most common nanoformulations of curcumin are solid lipid nanoparticles, nano-composite, nano-suspension, nanoparticles, liposomes, micelles, polymeric nanoparticles, and hydrogels (56). Liposomes are vesicular structures containing one or more phospholipid bilayers capable of transporting potential therapeutic molecules to target cells. Liposomes attach to the lipid membrane of the target cell and release their contents into the cytoplasm. Liposomes may encapsulate both hydrophilic and lipophilic compounds, ensuring maximum efficacy and safety in the delivery of target-specific drugs. Micelles are nanostructures having a hydrophilic outer layer and a lipophilic inside. The self-assembly of amphiphilic co-polymers forms them at a critical micellar concentration. The hydrophobic core is an effective drug transporter (56).
Nanosuspensions are insoluble water medications dispersed in aqueous solutions without a carrier. The drugs have a colloidal size distribution of fewer than 1 m and are stabilized using surfactants and other chemicals (57). The combination of small particle size (PS), a large surface area, and high thermodynamic energy (57) supports rapid drug dispersion. Solid lipid nanoparticles (SLNs) are colloidal lipid carriers (50–1000 nm) made of biological lipids that are biocompatible and biodegradable. Unlike liposomes, SLNs are rigid particles that can only be loaded with hydrophobic substances, such as curcumin. SLNs are distinguished by their high drug-loading capacity, robust stability, exceptional bio-compatibility, and enhanced bio-availability. Due to their hydrophobic nature, they are excellent nano-carriers for controlled release and site/cell-directed medicine delivery to reticuloendothelial cells (58).
Nanoemulsions (NEs) are kinetically stable, transparent, or translucent dispersions of oil, emulsifier, and water with particle sizes of less than 100 nm. In contrast, nano and micro-emulsions do not form spontaneously; their low surfactant concentration needs a large amount of energy to generate. Because they are emulsions, they permit the incorporation of both hydrophobic and hydrophilic therapies and the enhancement of hydrophobic medicines according to the size of their vesicles (59).
Nano-sized quantum dots, manganese phosphate nanoparticles, noble metals, carbon nanotubes, silica nanoparticles, and magnetic nanoparticles are inorganic. They feature unique, size-dependent physical properties, including optical and electrical effects, effective contrasting effects, and magnetism. In addition, they are resistant to microorganisms and possess excellent storage properties. Curcumin-based inorganic nanoparticles have the potential to be utilized in several vital bioapplications (60). Polymeric nanoparticles are colloids with a diameter of less than one millimeter composed of biodegradable or non-biodegradable natural or manufactured polymers. They are either nano-capsules (polymer encases the drug) or nano-spheres (where the medication is distributed throughout the polymer) (60). Liposomes have more reactivity, surface area, sensitivity, and stability than polymeric nanoparticles. Because of their high membrane permeability (due to their tiny size) and capacity to target specific organs, they interact with drug carriers (61).
Nanocomposites are non-homogenous materials made at the nanoscale level by combining polymers with inorganic solids. Their frameworks have been discovered to be more complex than micro composites. Individual property structure, composition, interfacial interactions, and components significantly impact them. The method of in situ growth, bio-polymer, and inorganic matrix polymerization is the most common way to create nanocomposites (62).
Hydrogels are three-dimensional (3D) polymeric structures that are extremely swollen, hydrophilic, and capable of absorbing huge volumes of water-insoluble compounds in water due to cross-linked polymers, enmeshment, or crystalline areas in their composition. Natural, synthetic, or hybrid polymers can be used to make hydrogels. Biopolymer-based hydrogels have attracted much attention as promising options for medicinal applications, including therapeutic delivery (63). Therefore, nanotechnology is a very effective tool to increase the limits of native curcumin to improve its therapeutic potential due to the presence of other key features such as high cellular uptake, biodistribution, dissociation rates, stability in serum, and sustained drug release at the target site.
Structural analogs
Typically, curcumin analogs are divided into three groups: natural derivatives from turmeric, curcumin analogs from mother nature, and synthetic analogs (57) (Table 2). Curcumin, cyclocurcumin, bisdemethoxycurcumin, and demethoxycurcumin are curcuminoids that originate from turmeric. Bioactive compounds that occur naturally and possess structural similarity to curcumin are defined as curcumin analogs from mother nature. Synthetic analogs of curcumin compounds are produced by modifying the basic structure of curcumin using various chemical reactions. The major possibilities to modify curcumin structurally are making changes in the dike to functionality, aryl side chain, active methylene functionality, double bond, and metal complexes (64). Some of the synthetic analogs are EF24, (1E, 6Z) 1,7-bis(13-fluoro-9 -ethylcoumarin-8-yl)-5-hydroxy3-oxohepta-1,4,6-triene (65).
Liposomal curcumin
Curcumin is likely to be metabolized quickly with less photo-stability, making it an ineffective therapeutic agent in its natural form (73). According to several studies, solubilizing curcumin in a phospholipid bilayer and liposomes can increase its transport and bio-availability (74). It is well known that nanoparticles (liposomes, micelles) have been considered to improve curcumin’s intracellular and targeted drug delivery, which can cross inaccessible anatomical and physiological barriers. Thus, liposomes must be a successful method to increase the bioavailability and stability of curcumin (75). Major preparation methods to produce curcumin liposomes include an injection method, a reversed-phase evaporation method, a thin-film method, and a freeze-thawing method (76). Curcumin encapsulation, including liposomes for any cancer/disease, should enhance bioavailability compared to freely available curcumin. At the same time, it must be non-toxic to normal cells in the resulting environment. In this context, the synthesis of liposomes can be done using a solvent-free method (since solvents may be toxic). For instance, De Leo et al. (77) reported the encapsulation method of curcumin liposomes for drug delivery in pH-responsive polymers using an organic-free method. The pharmaco-dynamics and pharmaco-kinetics of liposomal curcumin were improved, resulting in higher anticancer and pharmacological activity. Especially, liposomal curcumin was prepared with various conjugates, including vitamin A, polyethylene glycol, hyaluronic acid, silica, and folic acid. Besides, the liposomal nanoparticles encapsulated with drug combinations could sensitize cancer cells in the OS cell line (human osteosarcoma cell line) (78). However, with the constant progress of various liposomes, curcumin liposomes should be more standardized for other diseases and cancer.
Curcumin phospholipid complex
Several studies have implied the crucial roles of phospholipids in improving the therapeutic efficiency of small molecules for those with poor oral bioavailability (79). Generally, amphipathic phospholipid complexes act as bioactive components to pass them through the gastrointestinal cells to the blood (80). Theoretically, phospholipid complexes are appropriate strategies for any small bioactive molecule. The curcumin molecule is found to have a high affinity toward biological membranes and tends to penetrate them rapidly to form dimeric biological complexes. Despite being a phenolic and poorly soluble compound, curcumin can link with phospholipids (particularly phosphatidylcholine) by forming non-covalent adducts (80).
At last, the formation of these curcumin–phospholipid complexes could enhance the curcumin pharmacokinetics by stabilizing intestinal pH values and shielding curcumin in terms of retro-Claisen hydrolysis (36). Liu et al. (36) reported that a curcumin–phospholipid complex enhanced the oral bioavailability of curcumin compared with curcumin suspension to fivefold. In another study, in vivo absorption of a phospholipid–curcumin complex in Wistar rats showed that the bio-availability was significantly more enhanced than the kinetically free curcumin. In addition, pharmacokinetic study results revealed that a phospholipid–curcumin complex implied significantly high plasma concentrations and was found to be more stable when compared to natural curcumin (81). Various research findings suggest that the phospholipid–curcumin complex is one of the most precious methods for making curcumin more stable and improving its bioavailability (82).
Biochemical transformation of curcumin
The biochemical transformation of curcumin may occur during the processing, storage, or preparation of food products through a variety of mechanisms, including oxidation, pH-induced instability, and photodegradation (82, 83).
Oxidation
In the presence of oxygen, curcumin in phosphate buffer undergoes a rapid transformation into dicyclopentadiene, a major curcumin degradation product. This oxidation process was reported to be pH-dependent, with peak transformation at pH = 8, and catalyzed by cyclooxygenase-2 (COX-2) (39). Despite the rapid oxidation of curcumin into its degradation products, curcumin still exhibits its biological activities strongly; therefore, the activities may be because of the parent compound or degradation products. Nevertheless, a recent study comparing the anti-proliferative abilities of curcumin and its derivative, dicyclopentadiene, indicated that the derivative is significantly less active than the parent compound (83).
Furthermore, the co-addition of redox-active antioxidants (like ascorbate, TBHQ, and Trolox) dramatically increased curcumin stability, expanding its activities. With increasing chemical stability, curcumin efficacy significantly inhibits MC38 colon cancer cell proliferation. These data propose the biological impacts of curcumin are rather due to the parent compound, not the degradation. Furthermore, the co-administration of antioxidants increased curcumin concentration in plasma by approximately sixfold in an animal model (84). These results further supported the importance of stabilizing curcumin to increase its efficacies.
pH-induced instability
Curcumin is also important as a pH indicator or biosensor, which can be applied in smart packaging. Curcumin can monitor food spoilage by indicating changes in food pH and packaging headspace and providing information about food quality. This curcumin molecule is due to its high sensitivity in detecting acid-base reactions with visible color changes in food to the naked eye, which the consumer can visualize through the packaging without the need to open it. The curcumin molecule at pH 3.0–7.0 usually has a yellow color. However, with the pH increase from 7 to 8 (alkaline conditions) there is a strong change from yellow to red. This change occurs because the phenolic hydroxyl group readily reacts with OH- to form the phenoxide anion and causes a color change (43, 85).
Therefore, when adding curcumin to 0.1 M phosphate buffer at pH 7.2 and keeping at 37°C, more than 90% of the molecules rapidly degraded within 1 h, producing ferulic acid, vanillin, and feruloyl methane as its degradation products (Figure 2). HPLC analysis done by Nimiya et al. (84) showed that 80–90% of curcumin in phosphate buffer (pH 7.4) degraded post-12-min incubation. Another stability study showed that 80–90% of curcumin in phosphate buffer (pH 7.4) was degraded post-12-min incubation. Another stability study by Kharat et al. (86) at different pH values indicated that curcumin chemically degraded into its respective degradation products at alkaline pH values (pH ≥ 7.0), but crystallized, leading to a limitation of curcumin bioactivities at acidic pH values (pH < 7.0).
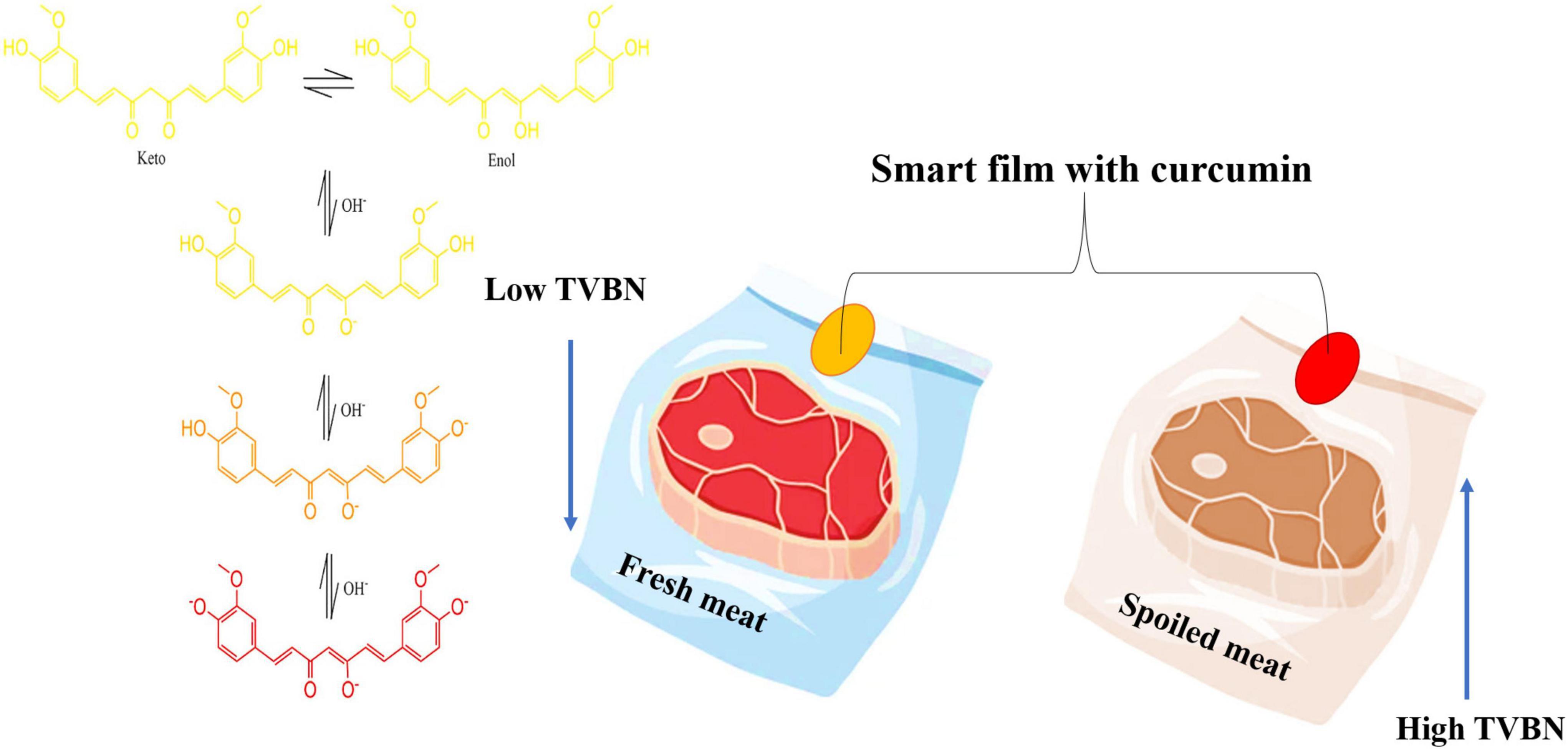
Figure 2. The effect of pH on curcumin color and its application in food shelf-life. TVBN, Total volatile base nitrogen.
Photodegradation
As curcumin undergoes degradation, the yellow color intensity decreases due to the formation of colorless products like vanillin, ferulic acid, and other small phenolic compounds. With exposure to sunlight, curcumin undergoes much faster degradation (87). High-performance thin layer chromatography analysis revealed that exposure to UV (254 nm) caused the degradation of curcumin into three products, whereas exposure to sunlight produced five degradation products (88). Few studies reported that singlet oxygen and superoxide were photogenerated by curcumin in biological systems, probably triggering curcumin phototoxicity. More studies on the mechanisms underlying curcumin photodegradation are required to understand the possible detrimental effects of curcumin phototoxicity (89).
Biochemical transformation
Human bodies have been designed to metabolize and deactivate any foreign compounds entering their systems, including curcumin. So, when curcumin enters the body, it will be metabolized, degraded, and deactivated to prevent it from reacting with the body’s systems. A pharmacokinetic study of curcumin was conducted where healthy human volunteers were given a single oral dose of 10 and 12 g of curcumin. Then serum samples were evaluated for free curcumin, glucuronide conjugate, and sulfate conjugate. The data showed that free curcumin levels peaked in the plasma for 10 and 12 g doses at times of 3.29 ± 0.43 h and 6.77 ± 0.83 h, with peak concentrations of 2.3 ± 0.26 μg/mL and 1.73 ± 0.19 μg/mL respectively (90).
Most of the curcumin detected in the plasma was in its conjugate forms, where the ratio of glucuronide: to sulfate was 1.92:1 (90), indicating curcumin has poor metabolic stability. After compounds manage to be absorbed by the gastrointestinal tract inside our body, two types of metabolism processes occur to deactivate foreign compounds trying to enter our body. The absorbed compounds will first undergo phase 1 metabolism in the gut epithelium cells. After passing through the epithelium cells, some compounds will be pumped back into the lumen due to efflux. The remaining compounds will be transported through the blood circulation to the liver, where they undergo phase 2 metabolism (90).
Phase 1 metabolism
As seen in Figure 3, during the phase 1 metabolism process in the epithelium cells, curcumin undergoes reduction catalyzed by NADPH-reductase, yielding dihydro curcumin, tetrahydro curcumin, hexahydro curcumin, and octa-hydro curcumin (phase 1 metabolites); autoxidation process yielding dicyclopentadiene and alkaline hydrolysis reaction yielding minor hydrolysis products ferulic acid, ferulaldehyde, vanillin, and feruloyl methane (91).
Phase 2 metabolism
This metabolism process includes conjugation reactions catalyzed by conjugative enzymes primarily found in the liver, kidney, and intestinal mucosa. Through this process, curcumin and its phase 1 metabolites are further metabolized, forming glucuronide and sulfate conjugates, making it bulkier and more water soluble. A recent study revealed that these conjugated products had fewer activations in HMVEC-dLy cells than curcumin due to their hydrophilicity (92).
Numerous animal and clinical studies have also found these metabolites to be the major curcumin form in serum. They are largely excreted out through feces and urine (93), indicating the deactivation of curcumin bioactivities through phase 2 metabolism and the rapid excretion of curcumin, leading to poor bioavailability of curcumin (93).
Metabolic regulation by gut microbiota
Curcumin metabolism pathways in the intestinal and liver cells have been intensively studied. Recent findings have shown the high importance of gut microorganisms in health, however, the fate of curcumin by intestinal microorganisms is largely unknown. Hassaninasab et al. (94) discovered a new metabolic pathway of curcumin involving a unique enzyme produced by the gut microorganism named CurA (NADPH-dependent curcumin or dihydro curcumin reductase). They were capable of isolating the curcumin-metabolizing microorganism from human feces and identifying it as Escherichia coli.
The 16S rRNA sequence of the E. coli H10407 strain, O55:H7 strain, CB9615, BW2952 strain, and K-12 strain DH10B sub-strain indicated their curcumin converting ability. CurA was responsible for converting curcumin through two reaction steps into dihydro curcumin and tetrahydro curcumin. However, the absence of further reduction products than tetrahydrocurcumin (THC) indicates that catalytic reduction by CurA is only effective for compounds with C = C double bonds (95). Future investigations are essential to understand the importance of gut microbiota in curcumin metabolic fate in the colon (96).
Health benefits and biological impacts of curcumin
As seen in Table 3 and Figure 4, curcumin has many different impacts on human health. Many researchers have studied the biological activities of curcumin in molecular, cellular, animal, and clinical studies to learn more about its contribution to health benefits. The obtained data showed that the actions of curcumin were involved in multiple pathways and mechanisms, demonstrating its beneficial effects. Curcumin has been studied for over three decades with numerous identified health benefits related to improvements in slowing the existence and progression of inflammatory disorders, cardiovascular disorders, and diabetes (97). For example, in vivo study when 50 patients with knee osteoarthritis were provided with a commercial compound containing curcumin (Theracurmin) (180 mg/day over 8 weeks) or a placebo, knee pain was significantly reduced by Theracurmin treatment when compared to the placebo (98). In addition, when another product containing curcumin (Algocur) was administered in pill form every 12 h for 5 or 10 days to rugby players suffering from osteo-muscular pain conditions, there were reductions in pain and improvements in physical function compared to the baseline condition (99). Curcumin has also been tested in cases with cardiovascular disorders, improving serum lipid levels (100).
Intake of curcumin extract (650 mg three times daily for 12 weeks) in patients with metabolic syndrome exhibited a lowering effect on the low-density lipoprotein cholesterol and an elevating one on the high-density lipoprotein cholesterol compared to placebo (101). The use of curcumin in treating type 2 diabetes was also intriguing. At a dose of 0.2/100 g or 1.0 g/100 g, a curcumin extract was fed to type 2 diabetic KK-A mice for month, compared to a basal control diet. The supplemented groups could resist the increased blood glucose observed in the control group, referring to turmeric as a functional food in type 2 diabetes mellitus (102). Similar findings have been noticed in human subjects. For example, in vivo ingestion of 6 g of C. longa elevated postprandial serum insulin levels at the 30- and 60-min intervals during an oral glucose tolerance test (19). Altogether, these studies, and others, showed that curcumin may be effective in controlling circulating blood glucose concentrations and has the potential to slow the progression of type 2 diabetes (15, 70–72). Turmeric is a safe food that healthy people can eat in moderation without getting sick (16).
Even if all the nutrients and substances in nature are beneficial for human health, they can become harmful when consumed in excess. There is a maximum level that should not be exceeded. Different investigations have referred to the safety of polyphenols at high doses (up to 8 g per day), but a clear dose has not yet been determined or decided. The Oxygen Radical Absorption Capacity (ORAC) value, which shows the antioxidant capacity of foods, is 44.776 in turmeric. With this value, turmeric ranks first in the list of spices with the highest antioxidant capacity. ORAC is a scale called free radicals in our body that indicates the absorption of substances that cause many diseases, especially cancer. That is, it is used for nutrients that indicate the absorption value. A food with a high ORAC value is more antioxidants, protects against cancer, and slows aging (16).
For adults, turmeric can be used as capsules, liquid essence, and tinctures containing powder. The cut root of turmeric can be consumed daily at 1.5–3 g, and the dried powdered turmeric root at 1–3 g. A standard powder (curcumin) can be taken thrice daily at 400–600 mg. Liquid extract (1:1) is recommended for daily consumption at 30–90 drops, one dose in the morning and one in the evening (1 part is 5 ml). Fresh turmeric can be stored in a cool, dry place for several weeks (103).
Curcumin can be used in salads, rice, and meat dishes to increase the flavor and consistency of food, giving it an attractive yellow color. It can be added to the dishes by mixing them with honey. It is also added to fish soup, cold cuts, and various vegetable dishes as a seasoning. It is used in the Spanish’s famous “paella” dish and the “curry” sauce of the Indians. Turmeric can also be used as a tea, which is very popular in Asian countries, especially Japan (103). Curcuma, the active ingredient of turmeric, prevents cancer and Alzheimer’s, protects from heavy metals, heals the liver, and is also a powerful antioxidant. However, for this effect of turmeric, the doses that will provide the same therapeutic effect at different times should be correct. Curcuma is great when it is pure and in small quantities, but its taste is bitter and less enjoyable when taken in larger doses (104).
Scientific data reveal that curcumin, is a difficult substance to absorb, and accordingly, it has poor bioavailability as well. Curcumin is absorbed very little in the human body when taken alone and is rapidly excreted from the intestines. For this reason, many studies have been conducted on the substances that will increase the bioavailability of curcumin, and it has been recommended that the piperine contained in black pepper can improve the uptake of curcumin by 2,000% (20 times). The vast metabolism of turmeric in the hepatic tissues and intestinal walls increased its bioavailability, which improved through piperine, which increases the absorption of all nutrients (104).
If such an improvement is not used, very little curcumin is absorbed, and even doses up to 4,000 mg can be completely inactive. Scientific research also shows that piperine, the active ingredient of black pepper, increases the absorption of substances that are difficult to absorb. Taking these two substances with an oil rich in unsaturated fatty acids further strengthens this benefit (74). With the extensive use of natural flavors in the food industry, the attention to natural antioxidants in plants worldwide is elevating daily. Since natural ingredients are used a lot in the food industry, people worldwide are becoming increasingly interested in natural antioxidants in herbs (105).
India has significant potential in terms of the production and export of some herbs and spices. Knowing these herbs and spices’ antioxidant and antimicrobial activities will make an essential contribution to extending the shelf life of food products (105, 106). Foods rich in polyunsaturated fatty acids are exposed to oxidative deterioration, which limits the shelf life of food products and causes quality loss. In industrial processes, synthetic antioxidants are mainly employed to prolong the preservation of nutrients. Many researchers state that some synthetic antioxidants used in food processing are carcinogenic and teratogenic to living organisms. Thus, consumers generally prefer natural antioxidants over synthetic ones (106).
Indian aromatic herbs used as additives to increase the sensorial properties of nutrients such as smell, and taste have become increasingly important. The antioxidant effect of phenolic materials found in the composition of these plants is based on their biochemical activities, e.g., cleaning free radicals, compounding metal ions, and preventing the formation of single oxygen. Some herbs and spices have been proven to have more antioxidant capacity than synthetic antioxidants (107). Because of the specific flavors and aromas, as well as the antimicrobial and antioxidant properties, herbs and spices have a broad bioactivity profile and can be utilized as alternatives in the food industry. Since, the prevention of lipid oxidation in foods is crucial for the producer and consumer, turmeric represents a good chose choice, being a strong natural antioxidant (108).
Curcumin’s antioxidant activity
The accumulation of reactive oxygen species (ROS) in cells has been tied to many disease cases, including the damage of the nucleic acids and DNA, potentially causing mutations and leading to tumor formation. ROS are free radicals involving superoxide hydroxyl radicals, anion radicals, hydrogen peroxide, and singlet oxygen. The adverse effects of ROS can be mitigated by natural antioxidants. The experiments on the in vitro stability and antioxidant capacity showed that when curcumin is embedded in the O/W SFME, its storage stability, light stability, thermal stability and antioxidant activity are significantly improved. This research is of great significance for the practical application of curcumin and other biomolecules (138). Also, in this study, glycated soy β-conglycinin (β-CG) stabilized curcumin (Cur) composites which were fabricated by a unique reversible self-assembly character of β-conglycinin-dextran conjugates (β-CG-DEX). Intrinsic fluorescence and far-UV CD spectra revealed that glycation did not affect the self-assembly property of β-CG in the pH-shifting treatment. The structure of β-CG-DEX could be unfolded at pH 12.0 and reassembled during acidification (from pH 12.0 to 7.0). Meanwhile, β-CG-DEX-3d, which was incubated at 60°C for 3 days, exhibited a high loading capacity (123.4 mg/g) for curcumin, which far exceeds that (74.90 mg/g) of β-CG-Cur. Moreover, the reassembled β-CG-DEX-3d-Cur showed eminent antioxidant activity of approximately 1.5 times higher than that of free curcumin. During the simulated gastrointestinal condition, compared with β-CG-Cur, β-CG-DEX-3d-Cur nanoparticles showed a more stable and sustained release of curcumin. Thus, β-CG-DEX has immense potential to become a new delivery carrier for hydrophobic food components by means of a self-assembly strategy (139). Turmeric is beneficial for the liver. It strengthens the liver and helps remove toxins from the liver (140–142).
Anti-microbial impacts of curcumin
The natural components from plants are considerable antimicrobial agents may that can substitute the current antibiotics, which are facing increasingly evolving resistance by microorganisms (Figure 5) (143–155). In an in vitro study, curcumin inhibited the growth of antibiotic-resistant Pseudomonas aeruginosa, Acinetobacter baumannii, and Klebsiella pneumoniae isolated from burn wound infections and showed synergism with meropenem (156). On gastrointestinal bacteria of human and bovine origin, Cur inhibited Firmicutes [Clostridioides difficile and Acetoanaerobium (Clostridium) sticklandii] but did not affect Bacteroidetes (Bacteroides fragilis and Prevotella bryantii) (157). Cur was conjugated to triphenyl phosphonium resulting in a compound named Mitocurcumin, which inhibited the growth of Bacillus subtilis, E. coli, Staphylococcus carnosus, and Mycobacterium smegmatis, and induced morphological changes in B. subtilis (158). Seventeen synthesized monocarbonyl curcuminoids showed high antibacterial activity against MSSA and MRSA and moderate activity against E. coli (158). The four most effective curcuminoids were bacteriostatic at low concentrations and bactericidal at high concentrations against MRSA, which showed membrane damage. In an ex vivo mammalian co-culture infection model, two curcuminoids decreased the viability of MSSA internalized in the fibroblasts (158). One of 13 synthesized curcuminoids, 3,3’-dihydroxycurcumin, showed antibacterial activity against S. aureus, B. subtilis, Enterococcus faecalis, and Mycobacterium tuberculosis, and produced membrane damage on B. subtilis (159). Nonetheless, all the synthesized curcuminoids were not effective against Gram-negative species (P. aeruginosa and E. coli). Cur analogs (monocurcuminoids, MC) were synthesized and showed higher, lower, or similar antimicrobial activity than Cur against Aeromonas hydrophila, E. coli, E. faecalis, K. pneumoniae, P. aeruginosa, S. aureus, and the yeast Candida albicans. Two MC and turmeric powder presented synergism against A. hydrophila, P. aeruginosa, and C. albicans. When aPDT was performed with UV light, two MC-mediated aPDT decreased the growth of E. faecalis, E. coli, and S. aureus, while aPDT with another MC and Cur increased the growth of A. hydrophila, E. faecalis, S. aureus, C. albicans, and P. aeruginosa (160). Cur was more effective than other natural biomolecules (quercetin and resveratrol) in inhibiting the growth of E. faecalis in spermatozoa from rabbits, but less effective than antibiotics (161). Cur-mediated aPDT also reduced the viability of E. faecalis biofilms grown in bovine bone cavities for 14 days by 1.92 log10 (162). The aPDT and the combination of a nanobubble solution and ultrasound reduced the viability of the aquatic pathogens A. hydrophila and Vibrio parahaemolyticus (163). The curcumin mode of action as an antimicrobial agent is summarized in Figures 5, 6.
Anti-inflammatory impacts of curcumin
Inflammation is the reaction of the body’s immune system associated with many diseases (Figure 7) and is a significant risk factor for tumor progression, such as tumor development and metastasis (164). In mammalians, the nuclear factor kappa B (NFkB) pathway, which plays a significant action in intracellular activities, can be activated by various agents, including pro-inflammatory cytokines, e.g., tumor necrosis factor-alpha (TNF-α). Once activated, it will, in turn, activate the downstream inflammatory pathways such as cyclooxygenase (COX-2), lipoxygenase (LOX), and inducible nitric oxide synthase (iNOS). Curcumin has been shown to down-regulate these downstream pathways by suppressing NF-kB and thus decreasing inflammation. Curcumin, a natural compound, inhibits the expression of pro-inflammatory cytokines such as CXCL1 through the NF-kB signaling pathway, potentially reducing tumor metastasis (148, 152, 153, 165).
Zhang (166) found that Cur loaded tetrahedral framework nucleic acids (Cur-TFNAs) were synthesized to deliver Cur. Compared with free Cur, Cur-TFNAs exhibit a preferable drug stability, good biocompatibility (CCK-8 assay), ease of uptake (immunofluorescence), and higher tissue utilization (in vivo biodistribution). Most importantly, Cur-TFNAs present better anti-inflammatory effect than free Cur both in vivo and in vitro experiments through the determination of inflammation-related cytokines expression. Therefore, Cur-TFNAs have great prospects for the prevention of gout and similar inflammatory diseases.
Immune stimulant impact of curcumin
An in vivo study evaluated the impacts of turmeric as a dietary supply for the ornamental fish Green Terror on the development and performance, survival rate, and blood indices. A total of 144 samples with an average weight of 1.53 ± 0.22 (g) were collected, and the hypothesis was investigated with four isocaloric and iso-nitrogenous diets containing 0.1, 0.2, and 0.3% of turmeric powder. Over a 100 days, the fish were subjected to biometrics every 20 days. At the end of the experiment, blood samples were tested. Findings revealed that the fish fed with 0.3% turmeric powder showed better growth performance, feed conversion ratio (FCR), condition factor, and survival rate specification, but RBC, PCV, hemoglobin, and MCHC were not elevated significantly (p > 0.05) contrasted to the control fish (p > 0.05) (167).
In another study, 180 Nile tilapia fish were applied in 3 monthly growth trials to observe the impact of turmeric on growing tilapia. It was noticed that 0.50% turmeric might enhance growth performance and significantly keep fish versus P. fluorescens challenge (168). The impact of turmeric on the immune response of fish Labeo rohita has been judged an effective action (169). Also, in vivo study reported that curcumin had a protective impact on Bloch tissue and elevated the growth performance (170). In this regard, the consumption of turmeric by fantail guppy (Poecilia reticulate) caused the reduction of FCR and improved growth performance (171). Sand Goby (Oxyeleotris marmoratus) also showed a positive reaction to the consumption of turmeric powder by increments in the secretions of amylase, lipase, trypsin, and chemo-trypsin (172).
The effectiveness of turmeric powder in combination with ginger and garlic on the immunity response of Labeorohita to A. hydrophila and white shrimp (Litopenaeus vannamei boone) has been evaluated by Chowdhury et al. (173). The impact of turmeric on blood and immunological status of Mugil cephalus vaccinated with A. hydrophila bacterium has been assessed (174). The immune response of Japanese flounder and Nile Tilapia fingerlings was enhanced when fed a diet supplemented with curcumin (175, 176).
In a different study, the effect of dietary curcumin on Cyprinus carpio, Rohmah et al. (177) investigated the potentials of dietary curcumin and resveratrol on blood biochemistry, immune responses and resistance to the toxicity of the pesticide, abamectin. Common carps (540) (30.78 ± 0.17 g) were randomly distributed into 18 tanks (30 fish per tank), as six experimental groups (T1: non-supplemented and on-exposed fish, T2: 300 mg/kg curcumin, T3: 300 mg/kg resveratrol, T4: 12.5% LC50 of abamectin, T5: 300 mg/kg curcumin + 12.5% LC50 of abamectin, T6: 300 mg/kg resveratrol + 12.5% LC50 of abamectin). Use of 300 mg/kg resveratrol in the diet of non-abamectin exposed fish improved the growth performance (P < 0.05), while such effects were not observed for curcumin (P > 0.05). There were no differences in the final weight (FW), FCR and weight gain (WG) between control and fish of the treatments, resveratrol + abamectin and curcumin + abamectin (P < 0.05). The immune components in blood [lysozyme, complement activity, total immunoglobulin (total Ig), protease, myeloperoxidase (MPO), nitro-blue-tetrazolium (NBT), peroxidase, albumin] and mucus [acid phosphatase (ACP), alkaline phosphatase (ALP), esterase, antiprotease)] and antioxidant enzymes [(superoxide dismutase (SOD), glutathione peroxidase (GPx)] exhibited various change patterns compared to the control group, however, these components were almost all higher in fish supplemented with curcumin and resveratrol in an abamectin-free medium than in control and other groups (P < 0.05) (177). In most cases, the levels of immune and antioxidant components in the control did not show significant difference with the treatments, resveratrol + abamectin and curcumin + abamectin (P > 0.05). Abamectin induced oxidative stress in fish, as the malondialdehyde (MDA) levels significantly increased in the exposed fish compared to non-exposed groups (P < 0.05) (177). It appears that neither curcumin nor resveratrol were as effective in preventing oxidative stress, because MDA levels were higher in exposed fish (abamectin, curcumin + abamectin, resveratrol + abamectin) than in control and non-exposed individuals (P < 0.05). Curcumin and resveratrol also showed protective effects on liver, since the levels of liver metabolic enzymes [aspartate transaminase (AST), ALP, lactate dehydrogenase (LDH)] were lower in the supplemented fish in an abamectin-free medium than in control (P < 0.05) (177). Curcumin and resveratrol also mitigated the stress responses in the exposed fish, as cortisol and glucose levels showed significant decreases in the supplemented fish (P < 0.05). In conclusion, this study revealed that abamectin can depress the growth and immunity in the common carp. Although, both resveratrol and curcumin were mitigated the toxic effects of abamectin, it seems that resveratrol be more effective than curcumin (177).
Curcumin and Alzheimer
Progressive degeneration and dysfunction of the nervous system because of oxidative stress, aggregations of misfolded proteins, and neuroinflammation are the key pathological features of neurodegenerative diseases. Alzheimer’s disease (AD) is a chronic neurodegenerative disorder driven by uncontrolled extracellular deposition of β-amyloid (Aβ) in the amyloid plaques and intracellular accumulation of hyperphosphorylated tau protein. Many different signaling pathways, such as Wnt/β-catenin, Notch, ROS/JNK, and PI3K/Akt/mTOR are involved in Alzheimer’s disease and crosstalk between themselves. Curcumin is a hydrophobic polyphenol with noticeable neuroprotective and anti-inflammatory effects that can cross the blood-brain barrier. Therefore, it is widely studied for the alleviation of inflammatory and neurological disorders. However, the clinical application of curcumin is limited due to its low aqueous solubility and bioavailability because it has difficulty permeating the blood–brain barrier (BBB), it must be encapsulated by a drug carrier. To increase curcumin’s permeability across the blood–brain barrier, it was encapsulated and conjugated with different agents. The studies indicated that lipid-based carriers and poly lactic-co-glycolic acid (PLGA) increased its organ distribution tremendously (Table 3). The functionalization of curcumin with metallic nanoparticles also enhanced the uptake of curcumin in the brain. Furthermore, the conjugation of carriers with targeting agents, such as Tet-1 peptide, transferrin, lactoferrin, and chitosan increased the blood–brain barrier permeability of curcumin. However, research on the mechanism of curcumin with a delivery vehicle is limited. Therefore recently, nano-based curcumin delivery systems are developed to overcome these limitations effectively (178).
Also, some of the most frequently studied are lipid nanocarriers, liposomes, micelles and PLGA. These carriers are further conjugated with brain-targeting agents such as lactoferrin and transferrin. Curcumin was investigated heavily as a treatment for AD. It stimulated neurogenesis via the Notch and Wnt/β-catenin pathways, diminished the secretion of proinflammatory cytokines, and led to the deactivation of GSK-3β, which in turn reduced Aβ production and the buildup of plaques by downregulating the ROS/JNK pathway (178). Furthermore, downregulation of NF-κB signaling led to GSK3β-mediated inhibition of BACE1, which ultimately reduced Aβ plaques. However, the exact mechanism by which curcumin regulated these processes is still unknown. It would be beneficial to study the important signaling pathways after curcumin is encapsulated with nanocarriers to see if the action mechanism of curcumin is sustained (178). Overall, curcumin is a very promising antioxidant for the treatment of AD, and the use of carriers and targeting agents is very effective for enhancing delivery to the brain (179).
Furthermore, Campisi et al. (180) assessed the effect of the systemic administration of SLNs to facilitate Cur delivery on TG2 isoform expression levels in Wild Type (WT) and in TgCRND8 (Tg) mice. An experimental model of AD, which expresses two mutated human amyloid precursor protein (APP) genes, was used. Behavioral studies were also performed to evaluate the improvement of cognitive performance and memory function induced by all treatments (180). The expression levels of Bcl-2, Cyclin-D1, and caspase-3 cleavage were evaluated as well. The systemic administration of SLNs-CUR, both in WT and in Tg mice, allows one to differently modulate TG2 isoforms, which act either on apoptotic pathway activation or on the ability of the protein to repair cellular damage in the brains of Tg mice. In this study, it was also suggested that SLNs-CUR could be an innovative tool for the treatment of AD (180).
Anti-cancer impacts of curcumin
Many investigations have clarified that curcumin has potent anti-cancer impacts via suppressions of angiogenesis formation of new blood vessels from the preexisting vessels (181). There are multiple steps involved in angiogenesis, including activation, proliferation, invasion, and migration of the endothelial cells (182). Curcumin has been shown to prevent angiogenesis via multiple suppression of these steps in different cancers. Moreover, studies showed that curcumin also inhibited lymphangiogenesis, the formation of new lymphatic vessels, which has a serious function in cancer metastasis, in vivo through suppression of VEGF Receptor signaling (141, 155, 183). Indian diets contain a lot of fried foods, which also contribute to gastrointestinal tract cancers due to the cooking process, probably forming carcinogenic or mutagenic heterocyclic amines (HA). Some animal studies showed that feeding the mice with typical Indian dishes such as deep-fried vegetables showed a 20% increase in gastric carcinoma. However, despite all this, gastric tumor incidence rates are rated as moderate to low in India, in contrast to other countries. High use of natural compounds, e.g., turmeric, may explain why they protect against the cancer-causing bacterium Helicobacter pylori, a common cause of stomach tumors (184–186). The following some examples of tumors that enhanced by curcumin or curcumin composites and the anticancer mechanism of bioavailable curcumin illustrated in Figure 8.
Colon cancer
One of the significant issues of the anti-cancer effects of phytochemicals, bioactive compounds from foods, and other plants, is that the effective dosages of the phytochemicals are too high to be obtained by oral intake, particularly by food intake. In the study of Aromokeye and Si (187) a combination of curcumin and luteolin, two phytochemicals from food, at lower concentrations showed a higher inhibitory effect on colon cancer growth and investigated possible molecular mechanisms of this anti-colon cancer effect. By pairwise combination screening, the combination of curcumin (Cur) at 15 μM and luteolin (LUT) at 30 μM (C15L30) synergistically suppressed the proliferation of human colon cancer CL-188 cells, but the individual chemicals had a little inhibitory effect at the selected concentrations. This result was also confirmed in other colon cancer DLD-1cells, suggesting that this synergistic inhibitory effect of C15L30 applies to different colon cancer cells. The combination C15L30 synergistically suppressed the wound closure (wound healing assay) in CL-188 cells. The combination of Cur and LUT (at 20 mg/kg/day and 10 mg/kg/day, respectively, IP injection, 5 days for 2 weeks) synergistically suppressed tumor growth in CL-188 cell-derived xenograft mice (187). Western blot results showed that protein levels of Notch1 and TGF-β were synergistically reduced by the combination, both in CL-188 cells and xenograft tumors. Tumor pathological analysis revealed that combined Cur and LUT synergistically increased necrosis, but the individual treatment with Cur and LUT had no significant effect on tumor necrosis. Therefore, combined curcumin and luteolin synergically inhibited colon cancer development by suppressing cell proliferation, necrosis, and migration associated with Notch1 and TGF-β pathways. Colon cancer may be prevented/treated by consuming foods having high levels of luteolin and curcumin in humans (187).
Additionally, curcumin reverses doxorubicin resistance in colon cancer cells at the metabolic level, where Zhang et al. (188) examined the MDR reversal capability of Cur in drug sensitive-(SW620) and resistant-(SW620/Ad300) colon cancer cells, and elucidated the underlying molecular mechanisms at the metabolic level. It was found that Cur reversed P-gp-mediated resistance in SW620/Ad300 cells by enhancing the Dox-induced cytotoxicity and apoptosis. Further mechanistic studies indicated that Cur inhibited the ATP-dependent transport activity of P-gp, thereby increasing the intra-cellular accumulation of Dox in drug-resistant cells. Metabolomics analysis based on UPLC-MS/MS showed that the MDR phenomenon in SW620/Ad300 cells was closely correlated with the upregulation of spermine and spermidine synthesis and D-glutamine metabolism. Cur significantly inhibited the biosynthesis of spermine and spermidine by decreasing the expression of ornithine decarboxylase (ODC) and suppressed D-glutamine metabolism, which in turn decreased the anti-oxidative stress ability and P-gp transport activity of SW620/Ad300 cells, eventually reversed MDR. These findings indicated the MDR reversal activity and the related mechanism of action of Cur, suggesting that Cur could be a promising MDR reversal agent for cancer treatment.
Lung cancer
The application of traditional chemotherapy drugs for lung cancer has obvious limitations, such as toxic side effects, uncontrolled drug-release, poor bioavailability, and drug-resistance. Thus, to address the limitations of free drugs and improve treatment effects, novel T7 peptide-modified nanoparticles (T7-CMCS-BAPE, CBT) was developed based on carboxymethyl chitosan (CMCS), which is capable of targeted binding to the transferrin receptor (TfR) expressed on lung cancer cells and precisely regulating drug-release according to the pH value and ROS level. The results showed that the drug-loading content of docetaxel (DTX) and curcumin was approximately 7.82 and 6.48%, respectively. Good biosafety was obtained even when the concentration was as high as 500 μg/mL. More importantly, the T7-CMCS-BAPE-DTX/CUR (CBT-DC) complexes exhibited better in vitro and in vivo anti-tumor effects than DTX monotherapy and other nanocarriers loaded with DTX and Cur alone. Furthermore, CBT-DC ameliorated the immunosuppressive micro-environment to promote the inhibition of tumor growth. Collectively, the current findings help lay the foundation for combinatorial lung cancer treatment (189). Also, curcumin found to induce ferroptosis in non-small-cell lung cancer via activating autophagy, through inhibiting tumor growth and cell proliferation, but promoted cell death. Characteristic changes of ferroptosis were observed in curcumin group, including iron overload, GSH depletion and lipid peroxidation. Meanwhile, the protein level of ACSL4 was higher and the levels of SLC7A11 and GPX4 were lower in curcumin group than that in control group. Incubation of ferroptosis inhibitors ferrostatin-1 (Fer-1) or knockdown of iron-responsive element-binding protein 2 (IREB2) notably weakened curcumin-induced anti-tumor effect and ferroptosis in A549 and H1299 cells. Further investigation suggested that curcumin induced mitochondrial membrane rupture and mitochondrial cristae decrease, increased autolysosome, increased the level of Beclin1 and LC3, and decreased the level of P62. Curcumin-induced autophagy and subsequent ferroptosis were both alleviated with autophagy inhibitor chloroquine (CQ) or siBeclin1 (190).
Prostate cancer
Prostate cancer is the most widespread tumor in the U.S.A. The malignancy of this cancer is due to its ability to evolve into its refractory hormone stage. A major challenge in the clinical management of prostate cancer (PC) is to inhibit tumor growth and prevent metastatic spreading. In recent years, considerable efforts have been made to discover new compounds useful for PC therapy, and promising advances in this field were reached. Drugs currently used in PC therapy frequently induce resistance and PC progresses toward metastatic castration-resistant forms (mCRPC), making it virtually incurable. Curcumin, a commercially available nutritional supplement, represents an attractive therapeutic agent for mCRPC patients. The effects of chemotherapeutic drugs such as docetaxel, paclitaxel, and cisplatin, to curcumin, was compared on two PC cell lines displaying a different metastatic potential: DU145 (moderate metastatic potential) and PC-3 (high metastatic potential). The results revealed a dose-dependent reduction of DU145 and PC-3 cell viability upon treatment with curcumin similar to chemotherapeutic agents (paclitaxel, cisplatin, and docetaxel). Furthermore, the EGFR-mediated signaling effects on ERK activation in DU145 and PC-3 cells was explored (191). Results showed that DU145 and PC-3 cells overexpress EGFR, and the treatment with chemotherapeutic agents or curcumin reduced EGFR expression levels and ERK activation. Finally, chemotherapeutic agents and curcumin reduced the size of DU145 and PC-3 spheroids and have the potential to induce apoptosis and also in Matrigel. In conclusion, despite different studies being carried out to identify the potential synergistic curcumin combinations with chemopreventive/therapeutic efficacy for inhibiting PC growth, the results showed the ability of curcumin used alone, or in combinatorial approaches, to impair the size and the viability of PC-derived spheroids (191). Additionally, curcumin has considerable role in inhibiting PC progression by regulating the miR-30a-5p/PCLAF axis, where impede the proliferation, migration and invasion of PC cells, and promote their apoptosis in a time- and dose-dependent manner. Curcumin enhanced miR-30a-5p expression and inhibited PCLAF expression; furthermore, there was a negative correlation between miR-30a-5p and PCLAF expression in PC tissues. In addition, transfection of miR-30a-5p inhibitors partially reversed the function of curcumin on cell proliferation, migration, invasion and apoptosis. Overall, curcumin suppressed the malignant biological behaviors of PC cells by regulating the miR-30a-5p/PCLAF axis (192).
Pancreatic cancer
Pancreatic carcinoma is a malignant tumor with a high fatality rate, and the increased resistance of pancreatic carcinoma to chemotherapy has become a difficult problem in clinical practice. Hence, it is imperative to develop an effective treatment for pancreatic cancer. Sestrins are a class of stress-induced proteins that have antioxidation functions, regulating cell growth and metabolism. Curcumin is a natural pigment isolated from turmeric. Several studies have also suggested that this molecule has multiple pharmacological effects, such as anti-inflammatory, antioxidant, and antitumor effects. However, there are insufficient studies on curcumin cooperating with the sestrin family to inhibit tumors, and the mechanism is still unclear. A study carried out (193) observed the potential anticancer effects of curcumin combined with the sestrin family on pancreatic carcinoma and probe its possible molecular mechanisms. The results revealed that curcumin cooperated with sestrin2 to significantly suppress pancreatic cancer. In addition, sestrin2 cooperated with curcumin to inhibit pancreatic cancer by specifically targeting Nrf2/Keap1/HO-1/NQO-1. It is concluded that curcumin-mediated synergistic targeting of sestrin2 is a potentially valuable treatment for pancreatic cancer (193). In another study, a solid phase approach was proposed for the combinatorial synthesis of a mini library of the mimics of curcumin in good purity and yield. The non-effective findings in pancreatic cancer cells switched to strong growth inhibition and cell death efficacy for PC3 prostate cancer cells, and mimic 9, in which tyrosol (TYR) and homovanillyl alcohol (HVA) units were linked by a phosphodiester bond, was quite effective not only in cell growth inhibition but also in causing strong cell death under the study conditions and treatments that were not effective in PANC1 cells (194). Table 4 shows the biological activities of bioavailable curcumin and their mechanism.
Curcumin and exercise
Nutritional strategies, such as antioxidant-rich foods, which help regulate inflammation, immune function, and oxidative stress, may be beneficial during High-Intensity Interval Training (HIIT) (243). This HIIT exercise can increase the inflammatory response and hinder the body’s recovery process (140, 143, 244–247). Other supplements such as curcumin have emerged as agents with significant therapeutic potential. Curcumin supplementation improved recovery time in mice following an eccentric downhill running protocol in a model of exercise-induced muscle injury. Downhill running lowers both treadmill’s run time to fatigue and voluntary activity, while 10 mg of curcumin powder given three consecutive days before running offset these effects on performance. Lower creatine kinase, IL-6, and TNF-alpha concentrations were also observed in the soleus muscle (248). In a similar study, when a turmeric extract (100 mg/kg) was administered for 6 weeks to rats, the endurance time to exhaustion in the exercised group improved, and the concentrations of serum total cholesterol, high-density lipoprotein, triglycerides, and lactate levels in both the exercise and non-exercise groups improved (97). Similarly, in human models, other investigators have noticed that curcumin has the potential to prevent delayed-onset muscle soreness (DOMS) after downhill running and eccentric leg press exercises (249, 250).
In a human study, 20 healthy males took either 200 mg curcumin or a placebo twice a day for two days prior and 1-day after a 45-min, constant intensity (lactate 3.5-5 mmol/L) downhill run (grade –10%). The curcumin supplementation group experienced reductions in DOMS-related leg muscle pain at sites located on the anterior right thigh, posterior right thigh, anterior right leg, posterior right leg, anterior left thigh, posterior left thigh, anterior left leg, and posterior left leg. Magnetic resonance imaging (MRI) also revealed less muscle injury in both thighs’ posterior or medial compartments. The inflammatory marker, interleukin-8 (IL-8), was also significantly lower 2 h after the downhill running test contrasted to the placebo group (249).
In a similar experiment, 28 males and females were provided either a placebo or 400 mg/day of curcumin before completing eccentric-only dual-leg press exercises. They then ingested either curcumin or a placebo 2 days prior to and 4 days after the protocol. Curcumin was found to reduce creatine kinase on days 1–4 following eccentric exercise. Additionally, IL-8 was significantly decreased in the curcumin group on days 1 and 2 following the exercise, and TNF-alpha was significantly lower in the curcumin group on days 1, 2, and 4 following the exercise placebo (250). Similar effects have been observed with lower doses of curcumin, but some speculate that this may decrease the magnitude of the anti-inflammatory response. When participants ingested 150 mg curcumin prior and 12 h after eccentric contractions of the elbow flexors of one arm on an isokinetic dynameter, maximal voluntary contraction torque was preserved, and recovery occurred four days sooner post-exercise in the curcumin group when contrasted to a placebo-controlled group. Interestingly, no significant differences were found in creatine kinase, IL-6, or TNF-alpha, suggesting that a larger dose of curcumin and a greater frequency of consumption on recovery days (post-exercise) may be needed to have more significant effects (251).
In a similar study, participants ingested 200 mg of curcumin and 20 mg of piperine three times a day and then completed 25 repetitions of 25-m, one-leg jumps on a downhill slope. Concentric and isometric peak torque for knee extension, one-leg 6-second sprint performance, countermovement jump performance, muscle soreness, and creatine kinase concentrations were measured. The results showed a moderately lower sprint mean power output 24 h post-exercise in the curcumin group contrasted to the placebo group. The dose in this study was higher than what was used in the previous studies; the length of supplementation may have been too short to find differences that matter (252).
Few studies examined the potential of curcumin to enhance endurance performance. Eleven recreational athletes took 500 mg/day of curcumin or placebo in a randomized, cross-over design for three days before and on the day of the exercise trial. The trial consisted of 2 h on a cycle ergometer at a power output of 95% of their lactate threshold. Curcumin was associated with a reduced exercise-induced inflammatory response. More specifically, curcumin was linked with lower IL-6 concentrations one hour after exercise compared to placebo (253). The outcomes of these studies were that curcumin could affect performance and the inflammatory response throughout short-term interventions, but more well-controlled investigations are still needed.
Curcumin and mental health
Curcumin is associated with better cognitive function and memory, as well as less stress and anxiety. Curcumin appears to be comparable with current pharmaceuticals as adjunctive therapy (254). Accumulating scientific evidence encourages using curcumin as a therapeutic agent for improving psychological health. In human studies, the most common psychological health assessments involve using questionnaires to quantify the signs and symptoms associated with mental health. In a sportive nutritional experiment, participants were supplemented with curcumin (3 days before and on the trial day) or placebo before completing a subjective daily analysis of life demands questionnaire to evaluate stress sources and stress signs before the exercise sessions. The curcumin group experienced “better than usual” results on the training days compared to the placebo on the second day of supply (253).
The obese patients witnessed by enhanced anxiety and depression, received 1,000 mg/day of a curcumin complex (curcumin, desmethoxycurcumin, and bisdemethoxycurcumin) or a placebo for 30 days. Curcumin treatment significantly reduced mean BAI scores compared to placebo, suggesting curcumin as an anti-anxiety therapy for individuals with obesity and major depressive disorder (255). Patients at a psychiatric outpatient department were enrolled in this study for 6 weeks. They were assigned to one of the three groups: 20 mg/day fluoxetine (n = 17), 1,000 mg/day curcumin (n = 10), or 1,000 mg/day and 20 mg/day of fluoxetine (n = 18). After 6 weeks of treatment, curcumin was equivalent to fluoxetine in terms of change in the Hamilton Depression Rating Scale score. The combination group showed a better response than the fluoxetine and curcumin groups alone. These results nominate curcumin as a powerful agent in treating major depressive disorder (256).
Cognition and memory are commonly explored in the older adult population. A computerized cognition test was used with 22 healthy older males and 38 healthy older females to evaluate the potential behavioral effects of curcumin. Participants received either curcumin (400 mg Longvida) or placebo once daily for four weeks and completed an array of computerized cognitive tasks preceding and following the assessment of the state of mood. A single dose of curcumin acutely improved cognitive processes and performance on a measure of sustained attention and working memory. After 4 weeks of curcumin supplementation, sustained attention and fatigue measures improved, proposing that curcumin may positively affect cognition in healthy elderly populations (254).
The official indications of the joint food and agriculture organization of the united nations/world health organization expert committee on food additives
The Joint FAO/WHO Expert Committee on Food Additives (JECFA) is an international scientific expert committee that is administered jointly by the Food and Agriculture Organization of the United Nations (FAO) and the World Health Organization (WHO). A turmeric rhizome extract is authorized as food additive (color) under the name curcumin (E 100) in the EU [Commission Regulation (EU) No 1129/20116]. According to Commission Regulation (EU) No 231/20127, the following definition is allocated to this food additive: ‘Curcumin is obtained by solvent extraction of turmeric, i.e., the ground rhizomes of strains of C. longa L. In order to obtain a concentrated curcumin powder, the extract is purified by crystallization. The product consists essentially of curcumin; i.e., the coloring principle [1,7-bis(4-hydroxy-3-methoxyphenyl)hepta-1,6-dien-3,5-dione8] and its two desmethoxy derivatives 9 in varying proportions. Minor amounts of oils and resins naturally occurring in turmeric may be present. Only the following solvents may be used in the extraction: ethyl acetate, acetone, carbon dioxide, dichloromethane, n-butanol, methanol, ethanol, hexane, propan-2-ol.’ The curcuminoids, including methoxy curcumin, curcumin, and bisdemethoxycurcumin, are a group of compounds found in turmeric (17), authorized by the US Food and Drug Administration (FDA) as GRAS, “Generally Recognized As Safe” at doses between 4,000 and 8,000 mg/day (257).
JECFA assessed the food additive curcumin (turmeric rhizome extract) in 2003 and established an acceptable daily intake (ADI) of 0–3 mg/kg body weight (bw) (258). In the European Food Safety Authority (EFSA) Panel on Food Additives and Nutrient Sources added to Food (ANS) adopted a scientific opinion on the re-evaluation of the food additive color curcumin (E 100) (turmeric rhizome extract) and concluded that the available data set supports the ADI allocated by JECFA based on the NOAEL of 250–320 mg/kg bw per day from the reproductive toxicity study in rats for a decreased body weight gain in the F2 generation observed at the highest dose level, and an uncertainty factor of 100 (259, 260). The EFSA took into account additional information on the use of curcumin (E 100) in foods and carried out a refined exposure assessment (261).
The European Medicines Agency (EMA) (262) assessed C. longa L., rhizoma, as herbal medicinal product in the form of powdered herbal substance, comminuted herbal substance, dry extract [13–25:1, extraction solvent: ethanol 96% (v/v)], dry extract [5.5–6.5:1, extraction solvent: ethanol 50% (v/v)] and tinctures (1:5 or 1:10, extraction solvent: ethanol 70% (v/v).
Applications of curcumin in food processing
Food preservation has been necessary for the continuity of life since the existence of humanity. Due to the changing eating habits over time and the increased number of employees, the development of ready-to-eat foods has become mandatory. Expanding food products’ shelf life and preserving their quality is a mandatory objective (263). The long-term preservation of seafood without spoiling is challenged by various requirements based on hygiene and sanitation rules, i.e., processing method, consuming seasons, processing wastes, making it ready to use the product and providing convenience for consumers and diversity for products. It has become important to utilize seafood processing in recent years.
Today, the increase in consumption and processing of food based on the relationship between the development of the industry and the consumption and production of food has made using food additives a technological must. The large number of people who work outside the home and do not have time to prepare food has encouraged the production of semi-finished or fully prepared foods commercially, and this situation has necessitated the use of food additives inevitable (264). Assuring food safety and security is one of the most important issues of today. Providing food security, improving food production, preventing nutrient losses, preserving their quality during the period between when the food is abundant and less, and extending its shelf life have gained significance. Thus, the usage of food additives has become inevitable in this case (5, 106, 265–271).
One of the food additives: Colorants
The use of plants by humanity as paint dates back centuries. Therefore, dye plants have become the main dyestuff of industrial products such as textiles, food, and leather. There are nearly 150 plant species used in natural dye production. These plants are turmeric, elecampane, licorice, common juniper, and sage (4). Food dyes, a type of food additive, are used in the industry for various purposes, including preserving, elevating, or modifying the existing or typical color, controlling color change and deterioration, standardizing the appearance, adding decorative features, and creating new products. They are additives used in confectionery, food eaten between meals, soft drinks, pastries, and many foods such as gelatin desserts (272). Color is one of the first characteristics of food that attracts people. A conventional color is desired in foods to be consumed. Colorants are substances added in food production to correct changes in a color loss that occur during processing or at the end of the process, that is, to restore the color of food or to color food. Color substances are also essential for creating a standard pigment in the product technologically (273).
The colorants are used to regain the natural color lost during processing and storage, to strengthen the weak color, to color the colorless food, and to meet consumer acceptance by hiding low quality (274). Since the additives are chemicals, their excess is harmful to health. Excessive use of these colorants may endanger one’s health. According to the way they are obtained, the colorants are divided into two natural and artificial colorants. Natural colorants are obtained from microbial, vegetable, animal, and mineral sources. The color stability of natural colorants is very low against physical and chemical effects. The majority of natural colorants are low in water solubility (275). According to the way they are obtained, the colorants are divided into two natural and artificial colorants. Natural colorants are obtained from microbial, vegetable, animal, mineral sources and agricultural wastes which are rich sources of natural colorants such as anthocyanin canthaxanthin, plain caramel, carotenes and chlorophylls (4, 276). Artificial colorants are more preferred in the food industry in terms of their physicochemical properties. Artificial colorants are easily soluble in water and oil (277). Curcumin is a powerful coloring agent widely used in the food industry. Its extraction from the plant C. longa is commonly done with aqueous solvent solutions. In contrast to the conventional extraction methods, two different green and bio-based surfactant-free microemulsion (SFME) extraction systems, which were approved for food and yield a higher extracting power of curcuminoids were compared. Two SFMEs, water/ethanol/triacetin and water/diacetin/triacetin, were investigated via dynamic light scattering. Curcumin solubility in binary mixtures consisting of ethanol/triacetin or diacetin/triacetin was studied both experimentally and theoretically using UV–Vis measurements and COSMO-RS. The SFMEs were further examined and compared to a common ethanol/water (80/20) extraction mixture with respect to their extracting ability using high performance liquid chromatography. The SFMEs containing ethanol were found to extract ∼18% more curcuminoids than the SFMEs containing diacetin and ∼53% more than the ordinary ethanol/water mixture (278). Also, (279) observed that using turmeric instead of Sunset yellow FCF can provide positive changes in the product’s appearance, taste, and texture that may appeal to the consumer. It has also been found to impact the increased consumption of smoked garfish positively. Thus, the use of turmeric, which is a natural colorant where more positive results are obtained, instead of artificial colorants that may be harmful to human health, has been suggested in terms of both making the color that is impulsive to the consumer in the product attractive and increasing the consumption of the smoked product (279).
Turmeric, one of the spices used in foods
Spices are usually cooked with food, but microorganisms in the form of spores remain alive during the cooking process, causing a proliferation in products stored in improper conditions during storage and distribution. The microflora in the spice reduces product shelf life and causes spoilage and foodborne diseases. These bacteria generally cause spoilage in products such as pickles, salami, sausage, and canned food. Turmeric is obtained from the C. longa plant’s root, a ginger family fiber plant. It is a plant with polyphenolic characteristics (279).
Dried turmeric is applied as a spice, and in making curry, it gives the curry a yellow color. With its bright yellow color, turmeric has been utilized as paint, medicine, and a spice since the 600s BC. Marko Polo has described turmeric as a vegetable that replaces saffron but is not saffron (279).
Turmeric is added in smoked foods, pickles, and some cakes. It is used in some dishes, the mixture of curry, mustard, and sauces for chicken meat, in some desserts, especially zerde, a dessert served at weddings in Anatolia, and gives it its yellow color. It is also used in seafood, fish soup, egg dishes, soups, rice, cold cuts, and numerous vegetable dishes. It is used especially in Indian and South Asian cuisine (279).
The scarce in vivo study explored the healthy benefits of curcumin in a functional food (280), which evaluated the effect of curcumin in combination with phytosterols in bread on the lipid profile. No significant effect was observed for curcumin addition in bread, whereas the incorporation of phytosterols reduced plasma cholesterol levels. Additionally, the authors indicated that this lack of effect observed for curcumin could be explained by the high temperatures used to process bread, which favored curcumin degradation. In vitro study of antimicrobial and antioxidant gelatin/curcumin composite films for active food packaging application, the authors found the addition of 1.5% of curcumin improved the UV blocking effect by more than 99% at a loss of 5.7% of transparency compared to neat gelatin films. The addition of curcumin (up to 1 wt%) significantly improved mechanical and water vapor barrier properties. Also, the gelatin/curcumin composite films exhibited remarkable antimicrobial activity against foodborne pathogenic bacteria, E. coli and L. monocytogenes, and showed strong antioxidant activity comparable to ascorbic acid. Antibacterial and antioxidant gelatin/curcumin composite films with improved UV protection, water vapor barrier and mechanical properties have high potential in active food packaging applications (281). Also, another in vitro study (282), found that poly lactic acid (PLA)/curcumin composite film showed excellent antioxidant and some antibacterial activity. The functional PLA/curcumin composite films with improved physical and functional properties can be used in active food packaging applications (282).
It is also worth mentioning that the incorporation of turmeric extract in beverages, bread, biscuits, snacks, pasta, milk, cheese, fresh sausage, and patties has been studied. Al-Obaidi (283) evaluated the influence of turmeric powder on the chemical composition, oxidative stability, and microbiology of the soft cheese. Different concentrations of turmeric powder (0, 0.1, 0.2, and 0.3%) represented as (T1, T2, T3, and T4) were added to the milk processed to the soft cheese, and then the cheese produced was stored at 5 ± 2°C for 9 days. The results showed no significant differences between the cheese of the different treatments (T2, T3, and T4) and the control cheese (T1) for moisture, protein, fat, ash, and pH. Evaluations of the cheese’s color, texture, and bitterness showed no significant differences between the cheese treated with turmeric powder (T2, T3, and T4) and the control cheese (T1). While for the flavor, the results showed that there was a significant difference between the cheese samples treated with turmeric powder 0.3% (w/v) (T4) and the untreated cheese sample (control) (T4). The results of peroxide value (PV) and acid value (AV) showed that the cheese treated with different concentrations of turmeric powder was lower than untreated cheese (control) (283). The microbiological results indicated that as the concentration of the turmeric powder increased, the total bacterial count decreased compared with the control treatment, which showed the highest total count after 9 days of storage at 5 ± 2°C. However, the coliform bacteria were increased during storage for control treatment only while it was undetectable for T2, T3, and T4 treatments after storage at 5 ± 2°C for 9 days. It could be concluded that turmeric powder was successfully used to improve the keeping quality of soft cheese (283).
Also, de Carvalho et al. (284) evaluated the in vitro effect of turmeric extract as a natural antioxidant on modified atmosphere-packaged fresh lamb sausages with fat replacement during storage (2°C). Five treatments were prepared: control without antioxidant (CONT); with 500 mg/kg sodium erythorbate (E500); and three batches with 250, 500, or 750 mg/kg turmeric extract (T250, T500, and T750). Turmeric extract improved the antioxidant capacity of lamb sausages and also slowed lipid oxidation and the generation of related volatile compounds. Moreover, the physicochemical parameters of lamb sausages were not greatly influenced by turmeric addition and concentration, except for the yellow color. All samples were considered acceptable by consumers. These findings showed that turmeric extract is effective against lipid oxidation and could be a good strategy to enhance the shelf life of lamb sausage (284).
Furthermore, Gómez-Estaca et al. (285) studied the effect of household practices (chilled storage and cooking) on quality attributes of low-fat PUFA-enriched pork burgers and the presence of curcumin as an antioxidant. Curcumin effectively reduced the lipid oxidation process derived from chilled storage or cooking regardless of the oleogelation method; the resulting products showed a lipid composition that meets recommendations for fatty acid intake. The samples formulated with beeswax oleogel presented adequate technological properties and overall sensory acceptability. Curcumin provided a yellow color that reduced sensory acceptance, regardless of the oleogelation method. Further studies are needed to adapt ethyl cellulose oleogels prepared with highly unsaturated lipids for the successful development of fresh meat products due to the extent of lipid oxidation during refrigeration and cooking and to the lower sensory acceptability.
Additionally, Ribeiro Oliveira et al. (286) developed extruded snacks from broken rice grains (BRG) and turmeric powder (TP). Snacks were in vitro evaluated for their physicochemical, microbial, and sensory characteristics and the selected savory snack formulation’s chemical composition, phenolic compounds, and antioxidant capacity. The selected snack, with the physical characteristics closest to the traditional corn product, was obtained with 6% substitution of BRG by TP and was constituted by 7.76% protein, 4.78% lipid, 5.84% dietary fiber, 75.3% available carbohydrates, and 174.75 mg of gallic acid equivalent per 100 g and 6.52% of DPPH scavenging capacity. The selected snack was shown to be feasible once TP was added as an ingredient that aggregated sensory, nutritional, and functional (antioxidant) values to the BRG-based snack, providing an alternative for the production of gluten-free snacks (286).
Three freshwater fish products (shell, taki, and tengra) were examined for their physicochemical (physical properties, proximate and chemical analyses), mineral, and bacteriological quality using turmeric powder, salt (dry), and the sun-drying procedure. The physicochemical properties such as the sensory properties, moisture, protein, fat, ash, salt, TVB-N, FFA, pH, certain mineral contents (Ca, Mg, Fe, Cu, Zn, and Mn), and bacterial load (SPC and HBC) of freshly processed turmeric and salt-treated sun-dried fish were examined. The dried fish product with the least moisture was also the most resistant to enzyme and microbiological activity (287).
Raw meat samples from pigs fed a control diet and a diet mixed with 4.5 g of turmeric powder per pig daily were used. Following the slaughter, raw meat was kept at 4°C for 7 days, and it was concluded that dietary turmeric powder induced an elevation in cooked meat quality, and color modifications in cooked meat were correlated with color parameters of raw samples. Dietary supplementation with C. longa powder did not change lipid oxidation, Warner Bratzler shear force, or the antioxidant capacity of cooked meat (288). In another study, the impacts of turmeric powder and ascorbic acid on lipid oxidation and antioxidant capacity in cooked rabbit burgers were estimated. The burgers were derived from 3 different formulations (C, control, with no additives; Tu with 3.5% of turmeric powder and AA with 0.1% of ascorbic acid) and were stored at 4°C for 0–7 days and cooked, and they concluded that the addition of 3.5% of turmeric powder in rabbit burgers exerts an antioxidant impact during storage. It appears more potent in limiting lipid oxidation than ascorbic acid post-cooking (289).
Curcumin usage limitation and future plans
Curcumin poses potential health risks despite its numerous advantages for human health and its well-established safety profile. Individuals’ side effects observed include abdominal pain, nausea, constipation, and hot flashes (290, 291). According to some accounts, curcumin may cause toxicity under certain circumstances. For instance, individuals receiving doses of curcumin between 0.45 and 12 g may suffer from vomiting, diarrhea, headaches, rushes, yellow stools, and elevated levels of both blood alkaline phosphatase and lactate dehydrogenase (292). For example, curcumin appears to cause DNA damage at both the mitochondrial and nuclear levels, suggesting that it may have a carcinogenic effect at doses near those that show positive results.
Additionally, mice fed different doses of turmeric oleoresin for 3 months and 2 years showed carcinogenic potential (292). Unsaturated ketones appear to be related to the mechanisms causing these effects, and these regions are known to form covalent bonds via a Michael addition reaction with the thiol groups of cysteine residues, and this also could be due to the antioxidant enzyme thioredoxin reductase that may be altered by this method, resulting in the formation of ROS (293). It can also inhibit the “guardian of the genome” “p53” and cause DNA damage caused by topoisomerase II. Curcumin was also discovered to inhibit the activities of cytochrome P450, glutathione S-transferase, UDP-glucuronosyltransferase, and chelate iron, which has an impact on how it is metabolized throughout the body. As xenobiotics build up when these three drug-metabolizing enzymes are blocked, toxicity may result (293). Numerous experiments on healthy subjects have confirmed the safety and effectiveness of curcumin. Despite this well-established safety, some negative side effects have been reported. Seven subjects receiving 500–12,000 mg in a dose-response study and followed for 72 h experienced diarrhea, headache, rash, and yellow stool (93). In another study, some subjects receiving 0.45–3.6 g/day curcumin for 1–4 months recorded nausea and diarrhea and elevated serum alkaline phosphatase and lactate dehydrogenase contents (294).
Conclusion
Curcumin has a prolonged history of usage in ancient medicine, where it was used in various medical treatments as well as food coloring and spice. Science has advanced over time, demonstrating curcumin’s many positive benefits for human health. The “golden spice” is still used in cooking today. Still, technological advances have made it possible to employ curcumin for various uses in the food and health industries. According to the results of preclinical and clinical studies conducted in vitro and in vivo, respectively, curcumin may be helpful in the prevention and treatment of many diseases, including cardiovascular diseases, diabetes mellitus, obesity, allergy, asthma, inflammatory diseases, and neurodegenerative disorders, e.g., Alzheimer’s, Parkinson’s, multiple sclerosis, and Huntington’s disease by affecting different molecular targets. Compared to other medications, curcumin is viewed as a very cost-effective and safe natural substance that can be used to prevent and treat many disorders. According to results from clinical trials, nano-formulations can increase curcumin bioavailability and are systemically safe. Nevertheless, these nano-formulations must be tested as therapeutic modalities for impending clinical studies and human application. Additionally, using curcumin nano-formulations in combination with other drugs is a good way to lower the dose of the primary therapeutic ingredient, which can increase therapeutic effectiveness while lowering systemic toxicity. Numerous curcumin nano-formulations have been developed, including dendrimers, polymeric nanoparticles, nanocrystals, micelles, liposomes, SLNs, and nanogels. These formulations are being investigated in several experimental and clinical studies and are suspected of being responsible for a few neurodegenerative diseases. Further research is recommended to assess the side impacts of different curcumin nano-formulations on human health and environmental significance.
Author contributions
All authors listed have made a substantial, direct, and intellectual contribution to the work, and approved it for publication.
Conflict of interest
The authors declare that the research was conducted in the absence of any commercial or financial relationships that could be construed as a potential conflict of interest.
Publisher’s note
All claims expressed in this article are solely those of the authors and do not necessarily represent those of their affiliated organizations, or those of the publisher, the editors and the reviewers. Any product that may be evaluated in this article, or claim that may be made by its manufacturer, is not guaranteed or endorsed by the publisher.
References
1. Wolf CP, Rachow T, Ernst T, Hochhaus A, Zomorodbakhsch B, Foller S, et al. Interactions in cancer treatment considering cancer therapy, concomitant medications, food, herbal medicine and other supplements. J Cancer Res Clin Oncol. (2022) 148:461–73. doi: 10.1007/s00432-021-03625-3
2. Zhang Y, McClain SA, Lee H-M, Elburki MS, Yu H, Gu Y, et al. A novel chemically modified curcumin “normalizes” wound-healing in rats with experimentally induced type I diabetes: initial studies. J Diabetes Res. (2016) 216:5782904. doi: 10.1155/2016/5782904
3. Abd El-Hack ME, El-Saadony MT, Swelum AA, Arif M, Abo Ghanima MM, Shukry M, et al. Curcumin, the active substance of turmeric: its effects on health and ways to improve its bioavailability. J Sci Food Agric. (2021) 101:5747–62. doi: 10.1002/jsfa.11372
4. El-Saadony MT, Saad AM, Elakkad HA, El-Tahan AM, Alshahrani OA, Alshilawi MS, et al. Flavoring and extending the shelf life of cucumber juice with aroma compounds-rich herbal extracts at 4°C through controlling chemical and microbial fluctuations. Saudi J Biol Sci. (2022) 29:346–54. doi: 10.1016/j.sjbs.2021.08.092
5. El-Saadony MT, Sitohy MZ, Ramadan MF, Saad AM. Green nanotechnology for preserving and enriching yogurt with biologically available iron (II). Innov Food Sci Emerg Technol. (2021) 69:102645. doi: 10.1016/j.ifset.2021.102645
6. Dias DA, Urban S, Roessner U. A historical overview of natural products in drug discovery. Metabolites. (2012) 2:303–36. doi: 10.3390/metabo2020303
7. Yaqoob MU, El-Hack MEA, Hassan F, El-Saadony MT, Khafaga AF, Batiha GE, et al. The potential mechanistic insights and future implications for the effect of prebiotics on poultry performance, gut microbiome, and intestinal morphology. Poult Sci. (2021) 100:101143. doi: 10.1016/j.psj.2021.101143
8. Abd El-Hack ME, El-Saadony MT, Shafi ME, Alshahrani OA, Saghir SA, Al-Wajeeh AS, et al. Prebiotics can restrict Salmonella populations in poultry: a review. Anim Biotechnol. (in press) (2021) 1–10. doi: 10.1080/10495398.2021.1883637
9. Zhang ZB, Ip SP, Cho WCS, Ng ACF, Hu Z, Huang YF, et al. Herb–drug interactions between androgenic Chinese herbal medicines and androgen receptor antagonist on tumor growth: studies on two xenograft prostate cancer animal models. Phytother Res. (2021) 35:2758–72. doi: 10.1002/ptr.7020
10. Rana A, Anand J, Tyagi M, Rai N. Forest-Based Medicinal Plants for Cancer Cure. Non-Timber Forest Products. Berlin: Springer (2021). p. 255–80. doi: 10.1007/978-3-030-73077-2_11
11. Soleimani V, Sahebkar A, Hosseinzadeh H. Turmeric (Curcuma longa) and its major constituent (Curcumin) as nontoxic and safe substances: review. Phytother Res. (2018) 32:985–95. doi: 10.1002/ptr.6054
12. Dumomangi Dorcas SL, Jireh Cosmas ON, Gborieneomie MF, Onyije FM. Histopathology and anticolon cancer effects of turmeric ethanolic extracts in wistar rats. Euro Sci J. (2021) 17:147–66. doi: 10.19044/esj.2021.v17n14p147
13. Hirun S, Utama-Ang N, Roach PD. Turmeric (Curcuma longa L.) drying: an optimization approach using microwave-vacuum drying. J Food Sci Technol. (2014) 51:2127–33. doi: 10.1007/s13197-012-0709-9
14. Meng F-C, Zhou Y-Q, Ren D, Wang R, Wang C, Lin L-G, et al. Turmeric: a review of its chemical composition, quality control, bioactivity, and pharmaceutical application. In: AM Grumezescu, AM Holban editors. Natural and Artificial Flavoring Agents and Food Dyes. Handbook of Food Bioengineering. Cambridge: Academic Press (2018). p. 299–350. doi: 10.1016/B978-0-12-811518-3.00010-7
15. Thangavel K, Dhivya K. Determination of curcumin, starch and moisture content in turmeric by Fourier transform near infrared spectroscopy (FT-NIR). Eng Agric Environ Food. (2019) 12:264–9. doi: 10.1016/j.eaef.2019.02.003
16. Liu S, Liu J, He L, Liu L, Cheng B, Zhou F, et al. A Comprehensive review on the benefits and problems of curcumin with respect to human health. Molecules. (2022) 27:4400. doi: 10.3390/molecules27144400
17. Cooksey C. Turmeric: old spice, new spice. Biotech Histochem. (2017) 92:309–14. doi: 10.1080/10520295.2017.1310924
18. Bange E, Timlin C, Kabel C, Svoboda J, Roeker L, Mato AR. Evidence for and against green tea and turmeric in the management of chronic lymphocytic leukemia. Clin Lymphoma Myeloma Leuk. (2018) 18:e421–6. doi: 10.1016/j.clml.2018.06.021
19. Wickenberg J, Ingemansson SL, Hlebowicz J. Effects of Curcuma longa (turmeric) on postprandial plasma glucose and insulin in healthy subjects. Nutr J. (2010) 9:34. doi: 10.1186/1475-2891-9-43
20. Beyene AM, Moniruzzaman M, Karthikeyan A, Min T. Curcumin nanoformulations with metal oxide nanomaterials for biomedical applications. Nanomaterials. (2021) 11:460. doi: 10.3390/nano11020460
21. Fabianowska-Majewska K, Kaufman-Szymczyk A, Szymanska-Kolba A, Jakubik J, Majewski G, Lubecka K. Curcumin from turmeric rhizome: a potential modulator of DNA methylation machinery in breast cancer inhibition. Nutrients. (2021) 13:332. doi: 10.3390/nu13020332
22. Esmaeili S, Berengi-Ardestani S, Khanniri E, Barzegar M, Sahari MA. Effect of storage time on the microbial and physicochemical properties of gamma irradiated turmeric powder under various atmospheres of packaging. Radiat Phys Chem. (2021) 187:109580. doi: 10.1016/j.radphyschem.2021.109580
23. de Sá IS, Peron AP, Leimann FV, Bressan GN, Krum BN, Fachinetto R, et al. In vitro and in vivo evaluation of enzymatic and antioxidant activity, cytotoxicity and genotoxicity of curcumin-loaded solid dispersions. Food Chem Toxicol. (2019) 125:29–37. doi: 10.1016/j.fct.2018.12.037
24. Guo X, Li W, Wang H, Fan Y-Y, Wang H, Gao X, et al. Preparation, characterization, release and antioxidant activity of curcumin-loaded amorphous calcium phosphate nanoparticles. J Non Cryst Solids. (2018) 500:317–25. doi: 10.1016/j.jnoncrysol.2018.08.015
25. Trigo-Gutierrez JK, Vega-Chacón Y, Soares AB, Mima EGDO. Antimicrobial activity of curcumin in nanoformulations: a comprehensive review. Int J Mol Sci. (2021) 22:7130. doi: 10.3390/ijms22137130
26. Wang Y, Li Y, He L, Mao B, Chen S, Martinez V, et al. Commensal flora triggered target anti-inflammation of alginate-curcumin micelle for ulcerative colitis treatment. Colloids Surf B Biointerfaces. (2021) 203:111756. doi: 10.1016/j.colsurfb.2021.111756
27. Abd Wahab NA, Lajis NH, Abas F, Othman I, Naidu R. Mechanism of anti-cancer activity of curcumin on androgen-dependent and androgen-independent prostate cancer. Nutrients. (2020) 12:679. doi: 10.3390/nu12030679
28. Ma R-H, Ni Z-J, Zhu Y-Y, Thakur K, Zhang F, Zhang Y-Y, et al. A recent update on the multifaceted health benefits associated with ginger and its bioactive components. Food Funct. (2021) 12:519–42. doi: 10.1039/D0FO02834G
29. El-Hadary A, Sitohy M. Safely effective hypoglycemic action of stevia and turmeric extracts on diabetic Albino rats. J Food Biochem. (2021) 45:e13549. doi: 10.1111/jfbc.13549
30. Sahne F, Mohammadi M, Najafpour GD, Moghadamnia AA. Extraction of bioactive compound curcumin from turmeric (Curcuma longa L.) via different routes: a comparative study. Pak J Biotechnol. (2016) 13:173–80.
31. Paulucci VP, Couto RO, Teixeira CC, Freitas LAP. Optimization of the extraction of curcumin from Curcuma longa rhizomes. Rev Bras Farmacogn. (2013) 23:94–100. doi: 10.1590/S0102-695X2012005000117
32. Shirsath SR, Sable SS, Gaikwad S, Sonawane S, Saini D, Gogate P. Intensification of extraction of curcumin from Curcuma amada using ultrasound assisted approach: effect of different operating parameters. Ultrason Sonochem. (2017) 38:437–45. doi: 10.1016/j.ultsonch.2017.03.040
33. Mottahedin P, Haghighi Asl A, Khajenoori M. Extraction of curcumin and essential oil from Curcuma longa L. by subcritical water via response surface methodology. J Food Process Preserv. (2017) 41:e13095. doi: 10.1111/jfpp.13095
34. Wakte PS, Sachin B, Patil A, Mohato D, Band T, Shinde D. Optimization of microwave, ultra-sonic and supercritical carbon dioxide assisted extraction techniques for curcumin from Curcuma longa. Sep Purif Technol. (2011) 79:50–5. doi: 10.1016/j.seppur.2011.03.010
35. Kiamahalleh MV, Najafpour-Darzi G, Rahimnejad M, Moghadamnia AA, Kiamahalleh MV. High performance curcumin subcritical water extraction from turmeric (Curcuma longa L.). J Chromatogr B Analyt Technol Biomed Life Sc. (2016) 1022:191–8. doi: 10.1016/j.jchromb.2016.04.021
36. Liu W, Zhai Y, Heng X, Che FY, Chen W, Sun D, et al. Oral bioavailability of curcumin: problems and advancements. J Drug Target. (2016) 24:694–702. doi: 10.3109/1061186X.2016.1157883
37. Zhang S, Asghar S, Yu F, Hu Z, Ping Q, Chen Z, et al. The enhancement of N-acetylcysteine on intestinal absorption and oral bioavailability of hydrophobic curcumin. Eur J Pharm Sci. (2020) 154:105506. doi: 10.1016/j.ejps.2020.105506
38. Khursheed R, Wadhwa S, Kumar B, Gulati M, Gupta S, Chaitanya M, et al. Development and validation of RP-HPLC based bioanalytical method for simultaneous estimation of curcumin and quercetin in rat’s plasma. S Afr J Bot. (2022) 149:870–7. doi: 10.1016/j.sajb.2021.12.009
39. Tsuda T. Curcumin as a functional food-derived factor: degradation products, metabolites, bioactivity, and future perspectives. Food Funct. (2018) 9:705–14. doi: 10.1039/C7FO01242J
40. Singh RP, Gangadharappa H, Mruthunjaya K. Phytosome complexed with chitosan for gingerol delivery in the treatment of respiratory infection: in vitro and in vivo evaluation. Eur J Pharm Sci. (2018) 122:214–29. doi: 10.1016/j.ejps.2018.06.028
41. Jamróz E, Cabaj A, Tkaczewska J, Kawecka A, Krzyściak P, Szuwarzyński M, Juszczak L. Incorporation of curcumin extract with lemongrass essential oil into the middle layer of triple-layered films based on furcellaran/chitosan/gelatin hydrolysates–In vitro and in vivo studies on active and intelligent properties. Food Chem. (2023) 402:134476. doi: 10.1016/j.foodchem.2022.134476
42. Yang Z, Yan J, Duan Y, Dai L, Wang Y, Sun Q, Xu X. Hydrolyzed rice glutelin nanoparticles as particulate emulsifier for pickering emulsion: structure, interfacial properties, and application for encapsulating curcumin. Food Hydrocoll. (2023) 134:108105. doi: 10.1016/j.foodhyd.2022.108105
43. de Oliveira Filho JG, Bertolo MRV, Rodrigues MÁV, Marangon CA, da Cruz Silva G, Odoni FCA, et al. Curcumin: A multifunctional molecule for the development of smart and active biodegradable polymer-based films. Trends Food Sci Technol. (2021) 118:840–849. doi: 10.1016/j.tifs.2021.11.005
44. Shen R, Yang X, Lin D. PH sensitive double-layered emulsions stabilized by bacterial cellulose nanofibers/soy protein isolate/chitosan complex enhanced the bioaccessibility of curcumin: in vitro study. Food Chem. (2023) 402:134262. doi: 10.1016/j.foodchem.2022.134262
45. Dragan ES, Ghiorghita CA, Dinu MV, Duceac IA, Coseri S. Fabrication of self-antibacterial chitosan/oxidized starch polyelectrolyte complex sponges for controlled delivery of curcumin. Food Hydrocoll. (2023) 135:108147. doi: 10.1016/j.foodhyd.2022.108147
46. Olotu F, Agoni C, Soremekun O, Soliman ME. An update on the pharmacological usage of curcumin: has it failed in the drug discovery pipeline? Cell Biochem Biophys. (2020) 78:267–289. doi: 10.1007/s12013-020-00922-5
47. Zhang Z, Li X, Sang S, McClements DJ, Chen L, Long J, Qiu C. Preparation, properties and interaction of curcumin loaded zein/HP-β-CD nanoparticles based on electrostatic interactions by antisolvent co-precipitation. Food Chem. (2023) 403:134344. doi: 10.1016/j.foodchem.2022.134344
48. Guo H, Feng Y, Deng Y, Yan T, Liang Z, Zhou Y, Wang W. Continuous flow modulates zein nanoprecipitation solvent environment to obtain colloidal particles with high curcumin loading. Food Hydrocoll. (2023) 134:108089. doi: 10.1016/j.foodhyd.2022.108089
49. Briskey D, Sax A, Mallard A, Rao A. Increased bioavailability of curcumin using a novel dispersion technology system (LipiSperse®). Eur J Nutr. (2019) 58:2087–2097. doi: 10.1007/s00394-018-1766-2
50. Cai D, Chen Q, Han J, Song Y, Meng Z, Zheng Y, et al. Effects of curcumin on the bioavailability of dioxin-like pollutants in rats. Sci Rep. (2021) 11:12461. doi: 10.1038/s41598-021-92085-3
51. Hatab HM, Hamid FFA, Soliman AF, Al-Shafie TA, Ismail YM, El-Houseini ME. A combined treatment of curcumin, piperine, and taurine alters the circulating levels of IL-10 and miR-21 in hepatocellular carcinoma patients: a pilot study. J Gastrointest Oncol. (2019) 10:766–76. doi: 10.21037/jgo.2019.03.07
52. Ruzicka J, Urdzikova LM, Svobodova B, Amin AG, Karova K, Dubisova J, et al. Does combined therapy of curcumin and epigallocatechin gallate have a synergistic neuroprotective effect against spinal cord injury? Neural Regen Res. (2018) 13:119–27. doi: 10.4103/1673-5374.224379
53. Pawar KS, Mastud RN, Pawar SK, Pawar SS, Bhoite RR, Bhoite RR, et al. Oral curcumin with piperine as adjuvant therapy for the treatment of COVID-19: a randomized clinical trial. Front Pharmacol. (2021) 12:669362. doi: 10.3389/fphar.2021.669362
54. Adusumilli NC, Mordorski B, Nosanchuk J, Friedman JM, Friedman AJ. Curcumin nanoparticles as a photoprotective adjuvant. Exp Dermatol. (2021) 30:705–9. doi: 10.1111/exd.14282
55. Zhong Y-B, Kang Z-P, Wang M-X, Long J, Wang H-Y, Huang J-Q, et al. Curcumin ameliorated dextran sulfate sodium-induced colitis via regulating the homeostasis of DCs and Treg and improving the composition of the gut microbiota. J Funct Foods. (2021) 86:104716. doi: 10.1016/j.jff.2021.104716
56. Jiang Z, Gan J, Wang L, Lv C. Binding of curcumin to barley protein Z improves its solubility, stability and bioavailability. Food Chem. (2023) 399:133952. doi: 10.1016/j.foodchem.2022.133952
57. Zhao Y, Liu JG, Chen WM, Yu AX. Efficacy of thermosensitive chitosan/ β-glycerophosphate hydrogel loaded with β-cyclodextrin-curcumin for the treatment of cutaneous wound infection in rats. Exp Ther Med. (2018) 15:1304–1313. doi: 10.3892/etm.2017.5552
58. Ban C, Jo M, Park YH, Kim JH, Han JY, Lee KW, et al. Enhancing the oral bioavailability of curcumin using solid lipid nanoparticles. Food Chem. (2020) 302:125328. doi: 10.1016/j.foodchem.2019.125328
59. Jiang T, Liao W, Charcosset C. Recent advances in encapsulation of curcumin in nanoemulsions: a review of encapsulation technologies, bioaccessibility and applications. Food Res Int. (2020) 132:109035. doi: 10.1016/j.foodres.2020.109035
61. Nayak SP, Ventrapragada LK, Rao AM, Kumar JK. Porous gold-curcumin nanocomposite for enhanced electrooxidation of glycerol and ethylene glycol. Mater Lett. (2023) 330:133212. doi: 10.1016/j.matlet.2022.133212
62. Wang Z, Zhao Z, Khan NR, Hua Z, Huo J, Li Y. Microwave assisted chitosan-polyethylene glycol hydrogel membrane synthesis of curcumin for open incision wound healing. Die Pharmazie. (2020) 75:118–123.
63. Desai PP, Patravale VB. Curcumin cocrystal micelles—Multifunctional nanocomposites for management of neurodegenerative ailments. J Pharm Sci. (2018) 107:1143–1156. doi: 10.1016/j.xphs.2017.11.014
64. Ullah MA, Johora FT, Sarkar B, Araf Y, Rahman MH. Curcumin analogs as the inhibitors of TLR4 pathway in inflammation and their drug like potentialities: a computer-based study. J Recept Signal Transduct Res. (2020) 40:324–338. doi: 10.1080/10799893.2020.1742741
65. He Y, Li W, Hu G, Sun H, Kong Q. Bioactivities of EF24, a novel curcumin analog: a review. Front Oncol. (2018) 8:614. doi: 10.3389/fonc.2018.00614
66. Khudhayer Oglah M, Fakri Mustafa Y. Curcumin analogs: synthesis and biological activities. Med Chem Res. (2020) 29:479–86. doi: 10.1007/s00044-019-02497-0
67. Lopes-Rodrigues V, Sousa E, Vasconcelos MH. Curcumin as a modulator of P-glycoprotein in cancer: challenges and perspectives. Pharmaceuticals. (2016) 9:71. doi: 10.3390/ph9040071
68. Fu M, Chen L, Zhang L, Yu X, Yang Q. Cyclocurcumin, a curcumin derivative, exhibits immune-modulating ability and is a potential compound for the treatment of rheumatoid arthritis as predicted by the MM-PBSA method. Int J Mol Med. (2017) 39:1164–72. doi: 10.3892/ijmm.2017.2926
69. Mapoung S, Suzuki S, Fuji S, Naiki-Ito A, Kato H, Yodkeeree S, et al. Dehydrozingerone, a curcumin analog, as a potential anti-prostate cancer inhibitor in vitro and in vivo. Molecules. (2020) 25:2737. doi: 10.3390/molecules25122737
70. Nkambeu B, Salem JB, Beaudry F. Capsaicin and its analogues impede nocifensive response of Caenorhabditis elegans to noxious heat. Neurochem Res. (2020) 45:1851–9. doi: 10.1007/s11064-020-03049-4
71. Udeni Gunathilake TMS, Ching YC, Chuah CH. Enhancement of curcumin bioavailability using nanocellulose reinforced chitosan hydrogel. Polymers. (2017) 9:64. doi: 10.3390/polym9020064
72. Singh A, Duggal S, Singh J, Katekhaye S. Experimental advances in pharmacology of gingerol and analogues. Pharmacy Global. (2010) 2:1–5.
73. Zhao S, Pi C, Ye Y, Zhao L, Wei Y. Recent advances of analogues of curcumin for treatment of cancer. Eur J Med Chem. (2019) 180:524–35. doi: 10.1016/j.ejmech.2019.07.034
74. Chang M, Wu M, Li H. Antitumor activities of novel glycyrrhetinic acid-modified curcumin-loaded cationic liposomes in vitro and in H22 tumor-bearing mice. Drug Deliv. (2018) 25:1984–95. doi: 10.1080/10717544.2018.1526227
75. Sinjari B, Pizzicannella J, D’Aurora M, Zappacosta R, Gatta V, Fontana A, et al. Curcumin/liposome nanotechnology as delivery platform for anti-inflammatory activities via NFkB/ERK/pERK pathway in human dental pulp treated with 2-hydroxyethyl methacrylate (HEMA). Front Physiol. (2019) 10:633. doi: 10.3389/fphys.2019.00633
76. Bulboacă AE, Boarescu PM, Bolboacă SD, Blidaru M, Feştilă D, Dogaru G, et al. Comparative effect of curcumin versus liposomal curcumin on systemic pro-inflammatory cytokines profile, MCP-1 and RANTES in experimental diabetes mellitus. Int J Nanomed. (2019) 14:8961–72. doi: 10.2147/IJN.S226790
77. De Leo V, Milano F, Mancini E, Comparelli R, Giotta L, Nacci A, et al. Encapsulation of curcumin-loaded liposomes for colonic drug delivery in a pH-responsive polymer cluster using a pH-driven and organic solvent-free process. Molecules. (2018) 23:739. doi: 10.3390/molecules23040739
78. Feng T, Wei Y, Lee RJ, Zhao L. Liposomal curcumin and its application in cancer. Int J Nanomed. (2017) 12:6027. doi: 10.2147/IJN.S132434
79. Yu F, Li Y, Chen Q, He Y, Wang H, Yang L, et al. Monodisperse microparticles loaded with the self-assembled berberine-phospholipid complex-based phytosomes for improving oral bioavailability and enhancing hypoglycemic efficiency. Eur J Pharm Biopharm. (2016) 103:136–48. doi: 10.1016/j.ejpb.2016.03.019
80. Lu M, Qiu Q, Luo X, Liu X, Sun J, Wang C, et al. Phyto-phospholipid complexes (phytosomes): a novel strategy to improve the bioavailability of active constituents. Asian J Pharm Sci. (2019) 14:265–74. doi: 10.1016/j.ajps.2018.05.011
81. Mirzaei H, Shakeri A, Rashidi B, Jalili A, Banikazemi Z, Sahebkar A. Phytosomal curcumin: a review of pharmacokinetic, experimental and clinical studies. Biomed Pharmacother. (2017) 85:102–12. doi: 10.1016/j.biopha.2016.11.098
82. Slika L, Patra D. A short review on chemical properties, stability and nano-technological advances for curcumin delivery. Expert Opin Drug Deliv. (2020) 17:61–75. doi: 10.1080/17425247.2020.1702644
83. Sanidad KZ, Zhu J, Wang W, Du Z, Zhang G. Effects of stable degradation products of curcumin on cancer cell proliferation and inflammation. J Agric Food Chem. (2016) 64:9189–95. doi: 10.1021/acs.jafc.6b04343
84. Nimiya Y, Wang W, Du Z, Sukamtoh E, Zhu J, Decker E, et al. Redox modulation of curcumin stability: redox active antioxidants increase chemical stability of curcumin. Mol Nutr Food Res. (2016) 60:487–94. doi: 10.1002/mnfr.201500681
85. Oliveira Filho JG, Egea MB. Edible bioactive film with curcumin: a Potential “Functional” Packaging? Int J Mol Sci. (2022) 23:5638. doi: 10.3390/ijms23105638
86. Kharat M, Du Z, Zhang G, McClements DJ. Physical and chemical stability of curcumin in aqueous solutions and emulsions: impact of pH, temperature, and molecular environment. J Agric Food Chem. (2017) 65:1525–32. doi: 10.1021/acs.jafc.6b04815
87. Seidi Damyeh M, Mereddy R, Netzel ME, Sultanbawa Y. An insight into curcumin-based photosensitization as a promising and green food preservation technology. Compr Rev Food Sci Food Saf. (2020) 19:1727–59. doi: 10.1111/1541-4337.12583
88. Chagas PA, Schneider R, dos Santos DM, Otuka AJ, Mendonça CR, Correa DS. Bilayered electrospun membranes composed of poly (lactic-acid)/natural rubber: a strategy against curcumin photodegradation for wound dressing application. React Funct Polym. (2021) 163:104889. doi: 10.1016/j.reactfunctpolym.2021.104889
89. Lima AR, Silva CM, da Silva LM, Machulek A Jr., De Souza AP, de Oliveira KT, et al. Environmentally safe photodynamic control of Aedes aegypti using sunlight-activated synthetic curcumin: photodegradation, aquatic ecotoxicity, and field trial. Molecules. (2022) 27:5699. doi: 10.3390/molecules27175699
90. Sukamtoh E. Biological Effects and Action Mechanisms of Dietary Compounds Masters Theses. Amherst, MA: University of Massachusetts Amherst (2018). 666 p.
91. Luis PB, Gordon ON, Nakashima F, Joseph AI, Shibata T, Uchida K, et al. Oxidative metabolism of curcumin-glucuronide by peroxidases and isolated human leukocytes. Biochem Pharmacol. (2017) 132:143–149. doi: 10.1016/j.bcp.2017.03.002
92. Wei H, Jiang D, Yu B, Ni D, Li M, Long Y, Cai W. Nanostructured polyvinylpyrrolidone-curcumin conjugates allowed for kidney-targeted treatment of cisplatin induced acute kidney injury. Bioact Mater. (2023) 19:282–291. doi: 10.1016/j.bioactmat.2022.04.006
93. Lao CD, Ruffin MT, Normolle D, Heath DD, Murray SI, Bailey JM, et al. Dose escalation of a curcuminoid formulation. BMC Complement Altern Med. (2006) 6:10. doi: 10.1186/1472-6882-6-10
94. Hassaninasab A, Hashimoto Y, Tomita-Yokotani K, Kobayashi M. Discovery of the curcumin metabolic pathway involving a unique enzyme in an intestinal microorganism. Proc Natl Acad Sci U.S.A. (2011) 108:6615–6620. doi: 10.1073/pnas.1016217108
95. Di Meo F, Margarucci S, Galderisi U, Crispi S, Peluso G. Curcumin, gut microbiota, and neuroprotection. Nutrients. (2019) 11:2426. doi: 10.3390/nu11102426
96. Scazzocchio B, Minghetti L, D’Archivio M. Interaction between gut microbiota and curcumin: a new key of understanding for the health effects of curcumin. Nutrients. (2020) 12:2499. doi: 10.3390/nu12092499
97. Sahin K, Pala R, Tuzcu M, Ozdemir O, Orhan C, Sahin N, et al. Curcumin prevents muscle damage by regulating NF-κB and Nrf2 pathways and improves performance: an in vivo model. J Inflamm Res. (2016) 9:147–54. doi: 10.2147/JIR.S110873
98. Nakagawa Y, Mukai S, Yamada S, Matsuoka M, Tarumi E, Hashimoto T, et al. Short-term effects of highly-bioavailable curcumin for treating knee osteoarthritis: a randomized, double-blind, placebo-controlled prospective study. J Orthop Sci. (2014) 19:933–9. doi: 10.1007/s00776-014-0633-0
99. Di Pierro F, Zacconi P, Bertuccioli A, Togni S, Eggenhoffner R, Giacomelli L, et al. A naturally-inspired, curcumin-based lecithin formulation (Meriva formulated as the finished product Algocur) alleviates the osteo-muscular pain conditions in rugby players. Eur Rev Med Pharm Sci. (2017) 21:4935–40.
100. Qin S, Huang L, Gong J, Shen S, Huang J, Ren H, et al. Efficacy and safety of turmeric and curcumin in lowering blood lipid levels in patients with cardiovascular risk factors: a meta-analysis of randomized controlled trials. Nutr J. (2017) 16:68. doi: 10.1186/s12937-017-0293-y
101. Yang YS, Su YF, Yang HW, Lee YH, Chou JI, Ueng KC. Lipid-lowering effects of curcumin in patients with metabolic syndrome: a randomized, double-blind, placebo-controlled trial. Phytother Res. (2014) 28:1770–7. doi: 10.1002/ptr.5197
102. Den Hartogh DJ, Gabriel A, Tsiani E. Antidiabetic properties of curcumin I: Evidence from in vitro studies. Nutrients. (2020) 12:118. doi: 10.3390/nu12010118
103. Aggarwal BB, Yuan W, Li S, Gupta SC. Curcumin-free turmeric exhibits anti-inflammatory and anticancer activities: identification of novel components of turmeric. Mol Nutr Food Res. (2013) 57:1529–42. doi: 10.1002/mnfr.201200838
105. Çoban ÖEPB. Use of cereals and spices with antioxidant effects in foods. J Food Technol. (2010) 5:7–19.
106. Saad AM, Sitohy MZ, Ahmed AI, Rabie NA, Amin SA, Aboelenin SM, et al. Biochemical and functional characterization of kidney bean protein alcalase-hydrolysates and their preservative action on stored chicken meat. Molecules. (2021) 26:4690. doi: 10.3390/molecules26154690
107. Abdel-Shafi S, Al-Mohammadi A-R, Sitohy M, Mosa B, Ismaiel A, Enan G, et al. Antimicrobial activity and chemical constitution of the crude, phenolic-rich extracts of Hibiscus sabdariffa, Brassica oleracea and Beta vulgaris. Molecules. (2019) 24:4280. doi: 10.3390/molecules24234280
108. Altun T, Usta F, Çelik F, Danabaş D. The Benefits of Seafood for Human Health. Izmir: National Water Days (2004). p. 11–8.
109. Ide H, Tokiwa S, Sakamaki K, Nishio K, Isotani S, Muto S, et al. Combined inhibitory effects of soy isoflavones and curcumin on the production of prostate-specific antigen. Prostate. (2010) 70:1127–33. doi: 10.1002/pros.21147
110. Kim SG, Veena MS, Basak SK, Han E, Tajima T, Gjertson DW, et al. Curcumin treatment suppresses IKKβ kinase activity of salivary cells of patients with head and neck cancer: a pilot study. Clin Cancer Res. (2011) 17:5953–61. doi: 10.1158/1078-0432.CCR-11-1272
111. Bayet-Robert M, Kwiatowski F, Leheurteur M, Gachon F, Planchat E, Abrial C, et al. Phase I dose escalation trial of docetaxel plus curcumin in patients with advanced and metastatic breast cancer. Cancer Biol Ther. (2010) 9:8–14. doi: 10.4161/cbt.9.1.10392
112. Howells LM, Iwuji CO, Irving GR, Barber S, Walter H, Sidat Z, et al. Curcumin combined with FOLFOX chemotherapy is safe and tolerable in patients with metastatic colorectal cancer in a randomized phase IIa trial. J Nutr. (2019) 149:1133–9. doi: 10.1093/jn/nxz029
113. Pastorelli D, Fabricio AS, Giovanis P, D’Ippolito S, Fiduccia P, Soldà C, et al. Phytosome complex of curcumin as complementary therapy of advanced pancreatic cancer improves safety and efficacy of gemcitabine: results of a prospective phase II trial. Pharmacol Res. (2018) 132:72–9. doi: 10.1016/j.phrs.2018.03.013
114. Lambring C, Varga K, Livingston K, Lorusso N, Dudhia A, Basha R. Therapeutic applications of curcumin and derivatives in colorectal cancer. Onco Ther. (2022) 9:51–62. doi: 10.1615/OncoTherap.2022044575
115. Song W, Wei L, Du Y, Wang Y, Jiang S. Protective effect of ginsenoside metabolite compound K against diabetic nephropathy by inhibiting NLRP3 inflammasome activation and NF-κB/p38 signaling pathway in high-fat diet/streptozotocin-induced diabetic mice. Int Immunopharmacol. (2018) 63:227–38. doi: 10.1016/j.intimp.2018.07.027
116. Yao Z, Le TH, Du Q, Mu H, Liu C, Zhu Y. The potential clinical value of curcumin and its derivatives in colorectal cancer. AntiCancer Agents Med Chem. (2021) 21:1626–37. doi: 10.2174/1871520620999201113095821
117. Salehi B, Stojanoviæ-Radiæ Z, Matejiæ J, Sharifi-Rad M, Kumar NVA, Martins N, et al. The therapeutic potential of curcumin: a review of clinical trials. Eur J Med Chem. (2019) 163:527–45. doi: 10.1016/j.ejmech.2018.12.016
118. Tomeh MA, Hadianamrei R, Zhao X. A review of curcumin and its derivatives as anticancer agents. Int J Mol Sci. (2019) 20:1033. doi: 10.3390/ijms20051033
119. Bilia AR, Bergonzi MC, Isacchi B, Antiga E, Caproni M. Curcumin nanoparticles potentiate therapeutic effectiveness of acitrein in moderate-to-severe psoriasis patients and control serum cholesterol levels. J Pharm Pharmacol. (2018) 70:919–28. doi: 10.1111/jphp.12910
120. Farkhondeh T, Samarghandian S, Pourbagher-Shahri AM, Sedaghat M. The impact of curcumin and its modified formulations on Alzheimer’s disease. J Cell Physiol. (2019) 234:16953–65. doi: 10.1002/jcp.28411
121. Vo TS, Vo TTBC, Vo TTTN, Lai TNH. Turmeric (Curcuma longa L.): chemical components and their effective clinical applications. J Turkish Chem Soc A Chem. (2021) 8:883–98. doi: 10.18596/jotcsa.913136
122. Li H, Sureda A, Devkota HP, Pittalà V, Barreca D, Silva AS, et al. Curcumin, the golden spice in treating cardiovascular diseases. Biotechnol Adv. (2020) 38:107343. doi: 10.1016/j.biotechadv.2019.01.010
123. Karthikeyan A, Young KN, Moniruzzaman M, Beyene AM, Do K, Kalaiselvi S, et al. Curcumin and its modified formulations on inflammatory bowel disease (IBD): the story so far and future outlook. Pharmaceutics. (2021) 13:484. doi: 10.3390/pharmaceutics13040484
124. Goulart RDA, Barbalho SM, Lima VM, Souza GAD, Matias JN, Araújo AC, et al. Effects of the use of curcumin on ulcerative colitis and Crohn’s disease: a systematic review. J Med Food (2021) 24:675–85. doi: 10.1089/jmf.2020.0129
125. Pourhabibi-Zarandi F, Shojaei-Zarghani S, Rafraf M. Curcumin and rheumatoid arthritis: a systematic review of literature. Int J Clin Pract (2021) 75:e14280. doi: 10.1111/ijcp.14280
126. Miserocchi E, Giuffrè C, Cicinelli MV, Marchese A, Gattinara M, Modorati G, et al. Oral phospholipidic curcumin in juvenile idiopathic arthritis-associated uveitis. Eur J Ophthalmol. (2020) 30:1390–6. doi: 10.1177/1120672119892804
127. Kwiecien S, Magierowski M, Majka J, Ptak-Belowska A, Wojcik D, Sliwowski Z, et al. Curcumin: a potent protectant against esophageal and gastric disorders. Int J Mol Sci. (2019) 20:1477. doi: 10.3390/ijms20061477
128. Memarzia A, Khazdair MR, Behrouz S, Gholamnezhad Z, Jafarnezhad M, Saadat S, et al. Experimental and clinical reports on anti-inflammatory, antioxidant, and immunomodulatory effects of Curcuma longa and curcumin, an updated and comprehensive review. BioFactors. (2021) 47:311–50. doi: 10.1002/biof.1716
129. Radbakhsh S, Barreto GE, Bland AR, Sahebkar A. Curcumin: a small molecule with big functionality against amyloid aggregation in neurodegenerative diseases and type 2 diabetes. Biofactors. (2021) 47:570–86. doi: 10.1002/biof.1735
130. Yang J, Miao X, Yang F-J, Cao J-F, Liu X, Fu J-L, et al. Therapeutic potential of curcumin in diabetic retinopathy. Int J Mol Med. (2021) 47:75. doi: 10.3892/ijmm.2021.4908
131. Ezati M, Ghavamipour F, Adibi H, Pouraghajan K, Arab SS, Sajedi RH, et al. Design, synthesis, spectroscopic characterizations, antidiabetic, in silico and kinetic evaluation of novel curcumin-fused aldohexoses. Spectrochim Acta A Mol Biomol Spectrosc. (2023) 285:121806. doi: 10.1016/j.saa.2022.121806
132. Zhang P, Fang J, Zhang J, Ding S, Gan D. Curcumin inhibited podocyte cell apoptosis and accelerated cell autophagy in diabetic nephropathy via regulating Beclin1/UVRAG/Bcl2. Diabetes Metab Syndr Obes. (2020) 13:641–652. doi: 10.2147/DMSO.S237451
133. Cui X, Lin L, Sun X, Wang L, Shen R. Curcumin protects against renal ischemia/reperfusion injury by regulating oxidative stress and inflammatory response. Evid based Complement Altern Med. (2021) 2021:8490772. doi: 10.1155/2021/8490772
134. Mohammadi E, Tamaddoni A, Qujeq D, Nasseri E, Zayeri F, Zand H, et al. An investigation of the effects of curcumin on iron overload, hepcidin level, and liver function in β−thalassemia major patients: a double-blind randomized controlled clinical trial. Phytother Res. (2018) 32:1828–1835. doi: 10.1002/ptr.6118
135. Markova E, Goldina I, Savkin I, Knyazheva M, Smyk A. Efficiency of the original flavonoids composition based on curcumin in chronic alcohol intoxication. Eur Psychiatry. (2022) 65(Suppl. 1):S825–S826. doi: 10.1192/j.eurpsy.2022.2137
136. Lin K, Chen H, Chen X, Qian J, Huang S, Huang W. Efficacy of curcumin on aortic atherosclerosis: a systematic review and meta-analysis in mouse studies and insights into possible mechanisms. Oxid Med Cell Longev. (2020) 2020:1520747. doi: 10.1155/2020/1520747
137. Memarzia A, Saadat S, Behrouz S, Boskabady MH. Curcuma longa and curcumin affect respiratory and allergic disorders, experimental and clinical evidence: a comprehensive and updated review. Biofactors. (2022) 48:521–51. doi: 10.1002/biof.1818
138. Liu W, Pan N, Han Y, Li D, Chai J. Solubilization, stability and antioxidant activity of curcumin in a novel surfactant-free microemulsion system. LWT Food Sci Technol. (2021) 147:111583. doi: 10.1016/j.lwt.2021.111583
139. Wang Z-J, Xu J-J, Ji F-Y, Luo S-Z, Li X-J, Mu D-D, et al. Fabrication and characterization of soy β-conglycinin-dextran-polyphenol nanocomplexes: Improvement on the antioxidant activity and sustained-release property of curcumin. Food Chem. (2022) 395:133562. doi: 10.1016/j.foodchem.2022.133562
140. Abd El-Hack A, Mohamed E, El-Shall NA, El-Kasrawy NI, El-Saadony MT, Shafi ME, et al. The use of black pepper (Piper guineense) as an ecofriendly antimicrobial agent to fight foodborne microorganisms. Environ Sci. Pollut Res. (2022) 29:10894–907. doi: 10.1007/s11356-021-17806-7
141. Abd El-Hack ME, El-Saadony MT, Salem HM, El-Tahan AM, Soliman MM, Youssef GB, et al. Alternatives to antibiotics for organic poultry production: types, modes of action and impacts on bird’s health and production. Poult Sci. (2022) 101:101696. doi: 10.1016/j.psj.2022.101696
142. Shehata AM, Paswan VK, Attia YA, Abougabal MS, Khamis T, Alqosaibi AI, et al. In ovo inoculation of Bacillus subtilis and raffinose affects growth performance, cecal microbiota, volatile fatty acid, ileal morphology and gene expression, and sustainability of broiler chickens (Gallus gallus). Front Nutr. (2022) 9:903847. doi: 10.3389/fnut.2022.903847
143. Ashour EA, Farsi RM, Alaidaroos BA, Abdel-Moneim A-ME, El-Saadony MT, Osman AO, et al. Impacts of dietary supplementation of pyocyanin powder on growth performance, carcase traits, blood chemistry, meat quality and gut microbial activity of broilers. Ital J Anim Sci. (2021) 20:1357–72. doi: 10.1080/1828051X.2021.1924087
144. Abd El-Hack A, Mohamed E, El-Saadony MT, Shehata AM, Arif M, Paswan VK, et al. Approaches to prevent and control Campylobacter spp. colonization in broiler chickens: a review. Environ Sci Pollut Res. (2021) 28:4989–5004. doi: 10.1007/s11356-020-11747-3
145. El-Saadony MT, Salem HM, El-Tahan AM, Abd El-Mageed TA, Soliman SM, Khafaga AF, et al. The control of poultry salmonellosis using organic agents: an updated overview. Poult Sci. (2022) 101:101716. doi: 10.1016/j.psj.2022.101716
146. El-Saadony MT, Zabermawi NM, Zabermawi NM, Burollus MA, Shafi ME, Alagawany M, et al. Nutritional aspects and health benefits of bioactive plant compounds against infectious diseases: a review. Food Rev Int. (in press) (2021) 1–23. doi: 10.1080/87559129.2021.1944183
147. El-Tarabily KA, El-Saadony MT, Alagawany M, Arif M, Batiha GE, Khafaga AF, et al. Using essential oils to overcome bacterial biofilm formation and their antimicrobial resistance. Saudi J Biol Sci. (2021) 28:5145–56. doi: 10.1016/j.sjbs.2021.05.033
148. El-Shall NA, Abd El-Hack ME, Albaqami NM, Khafaga AF, Taha AE, Swelum AA, et al. Phytochemical control of poultry coccidiosis: a review. Poult Sci. (2022) 101:101542. doi: 10.1016/j.psj.2021.101542
149. Radwan MH, Alaidaroos BA, Jastaniah SD, Abu El-Naga MN, El-Gohary E-GE, Barakat EM, et al. Evaluation of antibacterial activity induced by Staphylococcus aureus and Ent A in the hemolymph of Spodoptera littoralis. Saudi J Biol Sci. (2022) 29:2892–903. doi: 10.1016/j.sjbs.2022.01.025
150. Reda F, El-Saadony M, El-Rayes T, Farahat M, Attia G, Alagawany M. Dietary effect of licorice (Glycyrrhiza glabra) on quail performance, carcass, blood metabolites and intestinal microbiota. Poult Sci. (2021) 100:101266. doi: 10.1016/j.psj.2021.101266
151. Reda FM, El-Saadony MT, Elnesr SS, Alagawany M, Tufarelli V. Effect of dietary supplementation of biological curcumin nanoparticles on growth and carcass traits, antioxidant status, immunity and caecal microbiota of Japanese quails. Animals. (2020) 10:754. doi: 10.3390/ani10050754
152. Salem HM, Khattab MS, Yehia N, Abd El-Hack ME, El-Saadony MT, Alhimaidi AR, et al. Morphological and molecular characterization of Ascaridia columbae in the domestic pigeon (Columba livia domestica) and the assessment of its immunological responses. Poult Sci. (2022) 101:101596. doi: 10.1016/j.psj.2021.101596
153. Salem HM, Salaeh NM, Ragni M, Swelum AA, Alqhtani AH, Abd El-Hack ME, et al. Incidence of gastrointestinal parasites in pigeons with an assessment of the nematocidal activity of chitosan nanoparticles against Ascaridia columbae. Poult Sci. (2022) 101:101820. doi: 10.1016/j.psj.2022.101820
154. Swelum AA, Elbestawy AR, El-Saadony MT, Hussein EO, Alhotan R, Suliman GM, et al. Ways to minimize bacterial infections, with special reference to Escherichia coli, to cope with the first-week mortality in chicks: an updated overview. Poult Sci. (2021) 100:101039. doi: 10.1016/j.psj.2021.101039
155. Swelum AA, El-Saadony MT, Abdo M, Ombarak RA, Hussein EO, Suliman G, et al. Nutritional, antimicrobial and medicinal properties of Camel’s milk: a review. Saudi J Biol Sci. (2021) 28:3126–36. doi: 10.1016/j.sjbs.2021.02.057
156. Xue K, Tan PH, Zhao ZH, Cui LY, Kannan MB, Li SQ, Zeng RC. In vitro degradation and multi-antibacterial mechanisms of β-cyclodextrin@ curcumin embodied Mg (OH) 2/MAO coating on AZ31 magnesium alloy. J Mater Sci Technol. (2023) 132:179–192. doi: 10.1016/j.jmst.2022.04.053
157. Lakes JE, Richards CI, Flythe MD. Inhibition of bacteroidetes and firmicutes by select phytochemicals. Anaerobe. (2020) 61:102145. doi: 10.1016/j.anaerobe.2019.102145
158. Wang M, Yi N, Fang K, Zhao Z, Xie R, Chen W. Deep colorful antibacterial wool fabrics by high-efficiency pad dyeing with insoluble curcumin. Chem Eng J. (2023) 452:139121. doi: 10.1016/j.cej.2022.139121
159. Polaquini CR, Morao LG, Nazare AC, Torrezan GS, Dilarri G, Cavalca LB, et al. Antibacterial activity of 3, 3’-dihydroxycurcumin (DHC) is associated with membrane perturbation. Bioorg Chem. (2019) 90:103031. doi: 10.1016/j.bioorg.2019.103031
160. Cerveira MM, Vianna HS, Ferrer EMK, da Rosa BN, de Pereira CMP, Baldissera MD, et al. Bioprospection of novel synthetic monocurcuminoids: Antioxidant, antimicrobial, and in vitro cytotoxic activities. Biomed Pharmacother. (2021) 133:111052. doi: 10.1016/j.biopha.2020.111052
161. Duracka M, Lukac N, Kacaniova M, Kantor A, Hleba L, Ondruska L, et al. Antibiotics versus natural biomolecules: the case of in vitro induced bacteriospermia by Enterococcus faecalis in rabbit semen. Molecules. (2019) 24:4329. doi: 10.3390/molecules24234329
162. Rocha MP, Santos MS, Rodrigues PLF, Araújo TSD, de Oliveira JM, Rosa LP, et al. Photodynamic therapry with curcumin in the reduction of Enterococcus faecalis biofilm in bone cavity: rMicrobiological and spectral fluorescense analysis. Photodiagnosis Photodyn Ther. (2021) 33:102084. doi: 10.1016/j.pdpdt.2020.102084
163. Rafeeq S, Shiroodi S, Schwarz MH, Nitin N, Ovissipour R. Inactivation of Aeromonas hydrophila and Vibrio parahaemolyticus by curcumin-mediated photosensitization and nanobubble-ultrasonication approaches. Foods. (2020) 9:1306. doi: 10.3390/foods9091306
164. Kronski E, Fiori ME, Barbieri O, Astigiano S, Mirisola V, Killian PH, et al. miR181b is induced by the chemopreventive polyphenol curcumin and inhibits breast cancer metastasis via down-regulation of the inflammatory cytokines CXCL1 and-2. Mol Oncol. (2014) 8:581–95. doi: 10.1016/j.molonc.2014.01.005
165. Killian PH, Kronski E, Michalik KM, Barbieri O, Astigiano S, Sommerhoff CP, et al. Curcumin inhibits prostate cancer metastasis in vivo by targeting the inflammatory cytokines CXCL1 and-2. Carcinogenesis. (2012) 33:2507–19. doi: 10.1093/carcin/bgs312
166. Zhang M, Zhang X, Tian T, Zhang Q, Wen Y, Zhu J, et al. Anti-inflammatory activity of curcumin-loaded tetrahedral framework nucleic acids on acute gouty arthritis. Bioact Mater. (2022) 8:368–80. doi: 10.1016/j.bioactmat.2021.06.003
167. Batmany Y, Zoriehzahra S, Kakoolaki S. Evaluating the effect of using turmeric (Curcuma longa) on growth performance and hematological parameters of the ornamental fish, Green Terror (Andinocara rivulatus). J Surv Fish Sci. (2019) 5:37–47. doi: 10.18331/SFS2019.5.2.5
168. Mahmoud MMA, El-Lamie MM, Dessouki AA, Yusuf MS. Effect of turmeric (Curcuma longa) supplementation on growth performance, feed utilization, and resistance of nile tilapia (Oreochromis niloticus) to Pseudomonas fluorescens challenge. Glob Res J Fish Sci Aquac. (2014) 1:26–33.
169. Behera T, Swain P, Sahoo S, Mohapatra D, Das BK. Immunostimulatory effects of curcumin in fish, Labeo rohita (H.). Indian J Nat Prod Resour. (2011) 2:184–8.
170. Manju M, Akbarsha MA, Oommen OV. In vivo protective effect of dietary curcumin in fish Anabas testudineus (Bloch). Fish Physiol Biochem. (2012) 38:309–18. doi: 10.1007/s10695-011-9508-x
171. Mukherjee A, Mandal B, Banerjee S. Turmeric as a carotenoid source on pigmentation and growth of fantail guppy Poecilia reticulata. Proc Zool Soc. (2009) 62:119–23. doi: 10.1007/s12595-009-0013-5
172. Rojtinnakorn J, Rittiplang S, Tongsiri S, Chaibu P. Tumeric extract inducing growth biomarker in sand goby (Oxyeleotris marmoratus). Proceedings of the 2nd International Conference on Chemical, Biological and Environment Sciences (ICCEBS 2012). Bali: (2012).
173. Chowdhury DK, Sahu NP, Sardar P, Deo AD, Bedekar MK, Singha KP, et al. Feeding turmeric in combination with ginger or garlic enhances the digestive enzyme activities, growth and immunity in Labeo rohita fingerlings. Anim Feed Sci Technol. (2021) 277:114964. doi: 10.1016/j.anifeedsci.2021.114964
174. Saad T, Abou El-Geit E, El-Hammady A Zaki MS. Effect of black cumin seeds (Nigella sativa) and/or turmeric (curcumin) on hematological, biochemical and immunological parameters of sea bass vaccinated with Pseudomonas fluorescens Bacterin. Life Sci J. (2013) 10:1292–303.
175. Anene A, Okorie EO, Ajima MN, Onyemaonwu J. Dietary supplement of turmeric (Curcuma longa) powder: Impact on haematological and biochemical responses in clarias gariepinus (Burchell, 1822) fingerlings. Aquac Stud. (2021) 22:714. doi: 10.4194/AQUAST714
176. El Basuini MF, Zaki MA, El-Hais AM, Elhanafy MG, El-Bilawy EH, Zaineldin AI, et al. Microbial, immune and antioxidant responses of Nile tilapia with dietary nano-curcumin supplements under chronic low temperatures. Aquac Fish. (in press) (2022). doi: 10.1016/j.aaf.2022.03.011
177. Rohmah MK, Salahdin OD, Gupta R, Muzammil K, Qasim MT, Al-Qaim ZH, et al. Modulatory role of dietary curcumin and resveratrol on growth performance, serum immunity responses, mucus enzymes activity, antioxidant capacity and serum and mucus biochemicals in the common carp, Cyprinus carpio exposed to abamectin. Fish Shellfish Immunol. (2022) 129:221–30. doi: 10.1016/j.fsi.2022.08.042
178. Shahbaz SK, Koushki K, Sathyapalan T, Majeed M, Sahebkar A. PLGA-Based curcumin delivery system: an interesting therapeutic approach in the treatment of Alzheimer’s disease. Curr Neuropharmacol. (2022) 20:309–23. doi: 10.2174/1570159X19666210823103020
179. Ege D. Action mechanisms of curcumin in Alzheimer’s disease and its brain targeted delivery. Materials. (2021) 14:3332. doi: 10.3390/ma14123332
180. Campisi A, Sposito G, Pellitteri R, Santonocito D, Bisicchia J, Raciti G, et al. Effect of unloaded and curcumin-loaded solid lipid nanoparticles on tissue transglutaminase isoforms expression levels in an experimental model of Alzheimer’s disease. Antioxidants. (2022) 11:1863. doi: 10.3390/antiox11101863
181. Chatterjee S, Sinha S, Molla S, Hembram KC, Kundu CN. PARP inhibitor Veliparib (ABT-888) enhances the anti-angiogenic potentiality of Curcumin through deregulation of NECTIN-4 in oral cancer: Role of nitric oxide (NO). Cell Signal. (2021) 80:109902. doi: 10.1016/j.cellsig.2020.109902
182. Caban M, Lewandowska U. Polyphenols and posterior segment eye diseases: effects on angiogenesis, invasion, migration and epithelial-mesenchymal transition. Food Rev Int. (in press) (2021) 1–29. doi: 10.1080/87559129.2021.2012792
183. Abd El-Hack ME, Alqhtani AH, Swelum AA, El-Saadony MT, Salem HM, Babalghith AO, et al. Pharmacological, nutritional and antimicrobial uses of Moringa oleifera Lam. leaves in poultry nutrition: an updated knowledge. Poult Sci. (2022) 101:102031. doi: 10.1016/j.psj.2022.102031
184. Wilken R, Veena MS., Wang MB, Srivatsan ES. Curcumin: a review of anti-cancer properties and therapeutic activity in head and neck squamous cell carcinoma. Mol Cancer. (2011) 10:12. doi: 10.1186/1476-4598-10-12
185. Abd El-Hack ME, El-Saadony MT, Elbestawy AR, Nahed A, Saad AM, Salem HM, et al. Necrotic enteritis in broiler chickens: disease characteristics and prevention using organic antibiotic alternatives–a comprehensive review. Poult Sci. (2022) 101:101590. doi: 10.1016/j.psj.2021.101590
186. Abd El-Hack ME, El-Saadony MT, Saad AM, Salem HM, Ashry NM, Ghanima MMA, et al. Essential oils and their nanoemulsions as green alternatives to antibiotics in poultry nutrition: a comprehensive review. Poult Sci. (2021) 101:101584. doi: 10.1016/j.psj.2021.101584
187. Aromokeye R, Si H. Combined curcumin and luteolin synergistically inhibit colon cancer associated with notch1 and TGF-β signaling pathways in cultured cells and xenograft mice. Cancers. (2022) 14:3001. doi: 10.3390/cancers14123001
188. Zhang N, Gao M, Wang Z, Zhang J, Cui W, Li J, et al. Curcumin reverses doxorubicin resistance in colon cancer cells at the metabolic level. J Pharm Biomed Anal. (2021) 201:114129. doi: 10.1016/j.jpba.2021.114129
189. Zhu X, Yu Z, Feng L, Deng L, Fang Z, Liu Z, et al. Chitosan-based nanoparticle co-delivery of docetaxel and curcumin ameliorates anti-tumor chemoimmunotherapy in lung cancer. Carbohydr Polym. (2021) 268:118237. doi: 10.1016/j.carbpol.2021.118237
190. Tang X, Ding H, Liang M, Chen X, Yan Y, Wan N, et al. Curcumin induces ferroptosis in non-small-cell lung cancer via activating autophagy. Thoracic Cancer. (2021) 12:1219–30. doi: 10.1111/1759-7714.13904
191. Boccellino M, Ambrosio P, Ballini A, De Vito D, Scacco S, Cantore S, et al. The role of curcumin in prostate cancer cells and derived spheroids. Cancers. (2022) 14:3348. doi: 10.3390/cancers14143348
192. Pan L, Sha J, Lin W, Wang Y, Bian T, Guo J. Curcumin inhibits prostate cancer progression by regulating the miR-30a-5p/PCLAF axis. Exp Ther Med. (2021) 22:969. doi: 10.3892/etm.2021.10401
193. Fu H, Ni X, Ni F, Li D, Sun H, Kong H, et al. Study of the mechanism by which curcumin cooperates with Sestrin2 to inhibit the growth of pancreatic cancer. Gastroenterol Res Pract. (2021) 2021:7362233. doi: 10.1155/2021/7362233
194. Romanucci V, Giordano M, Pagano R, Agarwal C, Agarwal R, Zarrelli A, et al. Solid-phase synthesis of curcumin mimics and their anticancer activity against human pancreatic, prostate, and colorectal cancer cell lines. Bioorg Med Chem. (2021) 42:116249. doi: 10.1016/j.bmc.2021.116249
195. Panahi Y, Hosseini MS, Khalili N, Naimi E, Majeed M, Sahebkar A. Antioxidant and anti-inflammatory effects of curcuminoid-piperine combination in subjects with metabolic syndrome: a randomized controlled trial and an updated meta-analysis. Clin Nutr. (2015) 34:1101–8. doi: 10.1016/j.clnu.2014.12.019
196. Panahi Y, Alishiri GH, Parvin S, Sahebkar A. Mitigation of systemic oxidative stress by curcuminoids in osteoarthritis: results of a randomized controlled trial. J Diet Suppl. (2016) 13:209–220. doi: 10.3109/19390211.2015.1008611
197. Menon VP, Sudheer AR. Antioxidant and anti-inflammatory properties of curcumin. The molecular targets and therapeutic uses of curcumin in health and disease. Adv Exp Med Biol. (2007) 595:105–125. doi: 10.1007/978-0-387-46401-5_3
198. Marchiani A, Rozzo C, Fadda A, Delogu G, Ruzza P. Curcumin and curcumin-like molecules: from spice to drugs. Curr Med Chem. (2014) 21:204–222. doi: 10.2174/092986732102131206115810
199. Lin YG, Kunnumakkara AB, Nair A, Merritt WM, Han LY, Armaiz-Pena GN, et al. Curcumin inhibits tumor growth and angiogenesis in ovarian carcinoma by targeting the nuclear factor-κB pathway. Clin Cancer Res. (2007) 13:3423–3430. doi: 10.1158/1078-0432.CCR-06-3072
200. Pontes-Quero GM, Benito-Garzón L, Cano JP, Aguilar MR, Vázquez-Lasa B. Amphiphilic polymeric nanoparticles encapsulating curcumin: Antioxidant, anti-inflammatory and biocompatibility studies. Mater Sci Eng. (2021) 121:111793. doi: 10.1016/j.msec.2020.111793
201. Chuengsamarn S, Rattanamongkolgul S, Luechapudiporn R, Phisalaphong C, Jirawatnotai S. Curcumin extract for prevention of type 2 diabetes. Diabetes care. (2012) 35:2121–7. doi: 10.2337/dc12-0116
202. Hlavaèková L, Janegová A, Ulièná O, Janega P, Èerná A, Babál P. Spice up the hypertension diet-curcumin and piperine prevent remodeling of aorta in experimental L-NAME induced hypertension. Nutr Metab. (2011) 8:72. doi: 10.1186/1743-7075-8-72
203. Sahebkar A. Are curcuminoids effective C-reactive protein-lowering agents in clinical practice? Evidence from a meta-analysis. Phytother Res. (2014) 28:633–42. doi: 10.1002/ptr.5045
204. Sahebkar A, Serban M-C, Ursoniu S, Banach M. Effect of curcuminoids on oxidative stress: a systematic review and meta-analysis of randomized controlled trials. J Funct Foods. (2015) 18:898–909. doi: 10.1016/j.jff.2015.01.005
205. Soni K, Kutian R. Effect of oral curcumin administranon on serum peroxides and cholesterol levels in human volunteers. Indian J Physiol Pharmacol. (1992) 36:273–5.
206. Kuptniratsaikul V, Dajpratham P, Taechaarpornkul W, Buntragulpoontawee M, Lukkanapichonchut P, Chootip C, et al. Efficacy and safety of Curcuma domestica extracts compared with ibuprofen in patients with knee osteoarthritis: a multicenter study. Clin Interv Aging. (2014) 9:451–8. doi: 10.2147/CIA.S58535
207. Foryst-Ludwig A, Neumann M, Schneider-Brachert W, Naumann M. Curcumin blocks NF-κB and the motogenic response in Helicobacter pylori-infected epithelial cells. Biochem Biophys Res Commun. (2004) 316:1065–72. doi: 10.1016/j.bbrc.2004.02.158
208. Kundu P, De R, Pal I, Mukhopadhyay AK, Saha DR, Swarnakar S. Curcumin alleviates matrix metalloproteinase-3 and-9 activities during eradication of Helicobacter pylori infection in cultured cells and mice. PLoS One. (2011) 6:e16306. doi: 10.1371/journal.pone.0016306
209. Cullen BR, Greene WC. Regulatory pathways governing HIV-1 replication. Cell. (1989) 58:423–6. doi: 10.1016/0092-8674(89)90420-0
210. Chandran B, Goel A. A randomized, pilot study to assess the efficacy and safety of curcumin in patients with active rheumatoid arthritis. Phytothe Res. (2012) 26:1719–25. doi: 10.1002/ptr.4639
211. Daily JW, Yang M, Park S. Efficacy of turmeric extracts and curcumin for alleviating the symptoms of joint arthritis: a systematic review and meta-analysis of randomized clinical trials. J Med Food. (2016) 19:717–729. doi: 10.1089/jmf.2016.3705
212. Ghasemi F, Shafiee M, Banikazemi Z, Pourhanifeh MH, Khanbabaei H, Shamshirian A, et al. Curcumin inhibits NF-kB and Wnt/β-catenin pathways in cervical cancer cells. Pathol Res Pract. (2019) 215:152556. doi: 10.1016/j.prp.2019.152556
213. O’Sullivan-Coyne G, O’sullivan G, O’Donovan T, Piwocka K, McKenna S. Curcumin induces apoptosis-independent death in oesophageal cancer cells. Br J Cancer. (2009) 101:1585–1595. doi: 10.1038/sj.bjc.6605308
214. Anand P, Sundaram C, Jhurani S, Kunnumakkara AB, Aggarwal BB. Curcumin and cancer: an “old-age” disease with an “age-old” solution. Cancer Lett. (2008) 267:133–164. doi: 10.1016/j.canlet.2008.03.025
215. Liang S, Du J, Hong Y, Cheng L, Gu Z, Li Z, Li C. Octenyl succinate anhydride debranched starch-based nanocarriers for curcumin with improved stability and antioxidant activity. Food Hydrocoll. (2023) 135:108118. doi: 10.1016/j.foodhyd.2022.108118
216. Kuo Y-C, Wang L-J, Rajesh R. Targeting human brain cancer stem cells by curcumin-loaded nanoparticles grafted with anti-aldehyde dehydrogenase and sialic acid: colocalization of ALDH and CD44. Mater Sci Eng C. (2019) 102:362–72. doi: 10.1016/j.msec.2019.04.065
217. Guimarães MR, Coimbra LS, de Aquino SG, Spolidorio LC, Kirkwood KL, Rossa C Jr. Potent anti-inflammatory effects of systemically administered curcumin modulate periodontal disease in vivo. J Periodontal Res. (2011) 46:269–79. doi: 10.1111/j.1600-0765.2010.01342.x
218. Han Z, Zhang J, Zhang K, Zhao Y. Curcumin inhibits cell viability, migration, and invasion of thymic carcinoma cells via downregulation of microRNA-27a. Phytother Res. (2020) 34:1629–37. doi: 10.1002/ptr.6629
219. Chen H-W, Lee J-Y, Huang J-Y, Wang C-C, Chen W-J, Su S-F, et al. Curcumin inhibits lung cancer cell invasion and metastasis through the tumor suppressor HLJ1. Cancer Res. (2008) 68:7428–38. doi: 10.1158/0008-5472.CAN-07-6734
220. Wang M, Jiang S, Zhou L, Yu F, Ding H, Li P, et al. Potential mechanisms of action of curcumin for cancer prevention: focus on cellular signaling pathways and miRNAs. Int J Biol Sci. (2019) 15:1200–14. doi: 10.7150/ijbs.33710
221. Zhao M-D, Li J-Q, Chen F-Y, Dong W, Wen L-J, Fei W-D, et al. Co-delivery of curcumin and paclitaxel by “core-shell” targeting amphiphilic copolymer to reverse resistance in the treatment of ovarian cancer. Int J Nanomed. (2019) 14:9453–67. doi: 10.2147/IJN.S224579
222. Kouhpeikar H, Butler AE, Bamian F, Barreto GE, Majeed M, Sahebkar A. Curcumin as a therapeutic agent in leukemia. J Cell Physiol. (2019) 234:12404–14. doi: 10.1002/jcp.28072
223. Divya CS, Pillai MR. Antitumor action of curcumin in human papillomavirus associated cells involves downregulation of viral oncogenes, prevention of NFkB and AP-1 translocation, and modulation of apoptosis. Mol Carcinog. (2006) 45:320–32. doi: 10.1002/mc.20170
224. Khan K, Quispe C, Javed Z, Iqbal MJ, Sadia H, Raza S, et al. Resveratrol, curcumin, paclitaxel and miRNAs mediated regulation of PI3K/Akt/mTOR pathway: go four better to treat bladder cancer. Cancer Cell Int. (2020) 20:560. doi: 10.1186/s12935-020-01660-7
225. Hashemi M, Mirzaei S, Barati M, Hejazi ES, Kakavand A, Entezari M, et al. Curcumin in the treatment of urological cancers: therapeutic targets, challenges and prospects. Life Sci. (2022) 309:120984. doi: 10.1016/j.lfs.2022.120984
226. Termini D, Den Hartogh DJ, Jaglanian A, Tsiani E. Curcumin against prostate cancer: current evidence. Biomolecules. (2020) 10:1536. doi: 10.3390/biom10111536
227. Nagaraju GP, Benton L, Bethi SR, Shoji M, El-Rayes BF. Curcumin analogs: their roles in pancreatic cancer growth and metastasis. Int J Cancer. (2019) 145:10–19. doi: 10.1002/ijc.31867
228. Soni VK, Shukla D, Kumar A, Vishvakarma NK. Curcumin circumvent lactate-induced chemoresistance in hepatic cancer cells through modulation of hydroxycarboxylic acid receptor-1. Int J Biochem Cell Biol. (2020) 123:105752. doi: 10.1016/j.biocel.2020.105752
229. Cai X-Z, Wang J, Xiao-Dong L, Wang G-L, Liu F-N, Cheng M-S, et al. Curcumin suppresses proliferation and invasion in human gastric cancer cells by down-regulation of PAK1 activity and cyclin D1 expression. Cancer Biol Ther. (2009) 8:1360–8. doi: 10.4161/cbt.8.14.8720
230. Shishodia S, Chaturvedi MM, Aggarwal BB. Role of curcumin in cancer therapy. Curr Probl Cancer. (2007) 31:243–305. doi: 10.1016/j.currproblcancer.2007.04.001
231. Pricci M, Girardi B, Giorgio F, Losurdo G, Ierardi E, Di Leo A. Curcumin and colorectal cancer: from basic to clinical evidences. Int J Mol Sci. (2020) 21:2364. doi: 10.3390/ijms21072364
232. Niamsa N, Sittiwet C. Antimicrobial activity of Curcuma longa aqueous extract. J Pharmacol Toxicol. (2009) 4:173–177. doi: 10.3923/jpt.2009.173.177
233. Lawhavinit O-A, Kongkathip N, Kongkathip B. Antimicrobial activity of curcuminoids from Curcuma longa L. on pathogenic bacteria of shrimp and chicken. Agric Nat Resour. (2010) 44:364–371.
234. Hosny I, El Kholy W, Murad H, El Dairouty R. Antimicrobial activity of curcumin upon pathogenic microorganisms during manufacture and storage of a novel style cheese ‘Karishcum’. Am J Sci. (2011) 7:611–618.
235. Tajbakhsh S, Mohammadi K, Deilami I, Zandi K, Fouladvand M, Ramedani E, et al. Antibacterial activity of Indium curcumin and Indium diacetylcurcumin. Afr J Biotechnol. (2008) 7:3832–3835.
236. De R, Kundu P, Swarnakar S, Ramamurthy T, Chowdhury A, Nair GB, et al. Antimicrobial activity of curcumin against Helicobacter pylori isolates from India and during infections in mice. Antimicrob Agents Chemother. (2009) 53:1592–1597. doi: 10.1128/AAC.01242-08
237. Upendra R, Pratima K, Manjunatha Reddy A. Turmeric powder (Curcuma longa Linn.) as an antifungal agent in plant tissue culture studies. Int J Eng Sci Technol. (2011) 3:1578–1581.
238. Ungphaiboon S, Supavita T, Singchangchai P, Sungkarak S, Rattanasuwan P, Itharat A. Study on antioxidant and antimicrobial activities of turmeric clear liquid soap for wound treatment of HIV patients. Songklanakarin J Sci Technol. (2005) 27:569–578.
239. Martins CVB, Da Silva DL, Neres ATM, Magalhaes TFF, Watanabe GA, Modolo LV, De Resende MA. Curcumin as a promising antifungal of clinical interest. J Antimicrob Chemother. (2009) 63:337–339. doi: 10.1093/jac/dkn488
240. Wang Y, Xu S, Han C, Wang L, Zheng Q, Wang S, Qin Q. Curcumin inhibits Singapore grouper iridovirus infection through multiple antiviral mechanisms. Aquaculture. (2023) 562:738870. doi: 10.1016/j.aquaculture.2022.738870
241. Balasubramanyam K, Varier RA, Altaf M, Swaminathan V, Siddappa NB, Ranga U, et al. Curcumin, a novel p300/CREB-binding protein-specific inhibitor of acetyltransferase, represses the acetylation of histone/nonhistone proteins and histone acetyltransferase-dependent chromatin transcription. J Biol Chem. (2004) 279:51163–51171. doi: 10.1074/jbc.M409024200
242. Si X, Wang Y, Wong J, Zhang J, McManus BM, Luo H. Dysregulation of the ubiquitin-proteasome system by curcumin suppresses coxsackievirus B3 replication. J Virol. (2007) 81:3142–3150. doi: 10.1128/JVI.02028-06
243. Li Z, Hu W, Dong J, Azi F, Xu X, Tu C, Dong M. The use of bacterial cellulose from kombucha to produce curcumin loaded Pickering emulsion with improved stability and antioxidant properties. Food Sci Hum Wellness. (2023) 12:669–679. doi: 10.1016/j.fshw.2022.07.069
244. Machado M, Pereira R, Sampaio-Jorge F, Knifis F, Hackney A. Creatine supplementation: effects on blood creatine kinase activity responses to resistance exercise and creatine kinase activity measurement. Braz J Pharm Sci. (2009) 45:751–757. doi: 10.1590/S1984-82502009000400020
245. Abd El-Hack ME, Alaidaroos BA, Farsi RM, Abou-Kassem DE, El-Saadony MT, Saad AM, et al. Impacts of supplementing broiler diets with biological curcumin, zinc nanoparticles and Bacillus licheniformis on growth, carcass traits, blood indices, meat quality and cecal microbial load. Animals. (2021) 11:1878. doi: 10.3390/ani11071878
246. Abd El-Hack ME, El-Saadony MT, Elbestawy AR, Gado AR, Nader MM, Saad AM, et al. Hot red pepper powder as a safe alternative to antibiotics in organic poultry feed: an updated overview. Poult Sci. (2022) 101:101684. doi: 10.1016/j.psj.2021.101684
247. Abdelnour SA, Swelum AA, Salama A, Al-Ghadi MQ, Qattan SY, Abd El-Hack ME, et al. The beneficial impacts of dietary phycocyanin supplementation on growing rabbits under high ambient temperature. Ital J Anim Sci. (2020) 19:1046–56. doi: 10.1080/1828051X.2020.1815598
248. Davis JM, Murphy EA, Carmichael MD, Zielinski MR, Groschwitz CM, Brown AS, et al. Curcumin effects on inflammation and performance recovery following eccentric exercise-induced muscle damage. Am J Physiol Regul Integr Comp Physiol. (2007) 292:2168–73. doi: 10.1152/ajpregu.00858.2006
249. Drobnic F, Riera J, Appendino G, Togni S, Franceschi F, Valle X, et al. Reduction of delayed onset muscle soreness by a novel curcumin delivery system (Meriva§): a randomised, placebo-controlled trial. J Int Soc Sports Nutr. (2014) 11:31–40. doi: 10.1186/1550-2783-11-31
250. McFarlin BK, Venable AS, Henning AL, Sampson JNB, Pennel K, Vingren JL, et al. Reduced inflammatory and muscle damage biomarkers following oral supplementation with bioavailable curcumin. BBA Clin. (2016) 5:72–8. doi: 10.1016/j.bbacli.2016.02.003
251. Tanabe Y, Maeda S, Akazawa N, Zempo-Miyaki A, Choi Y, Ra S-G, et al. Attenuation of indirect markers of eccentric exercise-induced muscle damage by curcumin. Eur J Appl Physiol. (2015) 115:1949–57. doi: 10.1007/s00421-015-3170-4
252. Delecroix B, Leduc C, Dawson B, Dupont G. Curcumin and piperine supplementation and recovery following exercise induced muscle damage: a randomized controlled trial. J Sports Sci Med. (2017) 16:147–53.
253. Sciberras JN, Galloway SD, Fenech A, Grech G, Farrugia C, Duca D, et al. The effect of turmeric (curcumin) supplementation on cytokine and inflammatory marker responses following 2 hours of endurance cycling. J Int Soc Sports Nutr. (2015) 12:5. doi: 10.1186/s12970-014-0066-3
254. Cox KH, Pipingas A, Scholey AB. Investigation of the effects of solid lipid curcumin on cognition and mood in a healthy older population. J Psychopharmacol. (2015) 29:642–51. doi: 10.1177/0269881114552744
255. Esmaily H, Sahebkar A, Iranshahi M, Ganjali S, Mohammadi A, Ferns G, et al. An investigation of the effects of curcumin on anxiety and depression in obese individuals: a randomized controlled trial. Chin J Integr Med. (2015) 21:332–8. doi: 10.1007/s11655-015-2160-z
256. Sanmukhani J, Satodia V, Trivedi J, Patel T, Tiwari D, Panchal B, et al. Efficacy and safety of curcumin in major depressive disorder: a randomized controlled trial. Phytother Res. (2014) 28:579–85. doi: 10.1002/ptr.5025
257. Hewlings SJ, Kalman DS. Curcumin: a review of its’ effects on human health. Foods. (2017) 6:10–92. doi: 10.3390/foods6100092
258. World Health Organization. Evaluation of Certain Food Additives and Contaminants. Sixty-First Report of the Joint FAO/WHO Expert Committee on Food Additives. WHO Technical Report Series 922. Geneva: World Health Organization (2004).
259. EFSA Scientific Committee, More S, Bampidis V, Benford D, Boesten J, Bragard C, et al. Genotoxicity assessment of chemical mixtures. EFSA J. (2019) 17:5519. doi: 10.2903/j.efsa.2019.5519
260. EFSA Panel on Food Additives and Nutrient Sources added to Food. Scientific opinion on the re-evaluation of curcumin (E 100) as a food additive. EFSA J. (2010) 8:1679. doi: 10.2903/j.efsa.2010.1679
261. European Food Safety Authority (EFSA). Refined exposure assessment for curcumin (E 100). EFSA J. (2014) 12:3876. doi: 10.2903/j.efsa.2014.3876
262. European Medicines Agency (EMA). Committee on Herbal Medicinal Products. Herbal Monograph on Curcuma longa L. rhizoma. EMA/507445/2018. Amsterdam: EMA (2018).
263. Jafarzadeh S, Nafchi AM, Salehabadi A, Oladzad-Abbasabadi N, Jafari SM. Application of bio-nanocomposite films and edible coatings for extending the shelf life of fresh fruits and vegetables. Adv Colloid Interface Sci. (2021) 291:102405. doi: 10.1016/j.cis.2021.102405
264. Güneri N. A review on turmeric (Curcuma longa L.) and usage in seafood. Mar Sci Tech Bull. (2021) 10:71–84. doi: 10.33714/masteb.771756
265. Delikanlı Akbay G, Pekcan AG. Turmeric: evaluation in terms of health and nutrition. Beslenme ve Diyet Dergisi. (2016) 44:68–72.
266. El-Saadony MT, Abd El-Hack ME, Swelum AA, Al-Sultan SI, El-Ghareeb WR, Hussein EO, et al. Enhancing quality and safety of raw buffalo meat using the bioactive peptides of pea and red kidney bean under refrigeration conditions. Ital J Anim Sci. (2021) 20:762–76. doi: 10.1080/1828051X.2021.1926346
267. El-Saadony MT, Khalil OS, Osman A, Alshilawi MS, Taha AE, Aboelenin SM, et al. Bioactive peptides supplemented raw buffalo milk: biological activity, shelf life and quality properties during cold preservation. Saudi J Biol Sci. (2021) 28:4581–91. doi: 10.1016/j.sjbs.2021.04.065
268. Namir M, Iskander A, Alyamani A, Sayed-Ahmed ETA, Saad AM, Elsahy K, et al. Upgrading common wheat pasta by fiber-rich fraction of potato peel byproduct at different particle sizes: Effects on physicochemical, thermal, and sensory properties. Molecules. (2022) 27:2868. doi: 10.3390/molecules27092868
269. Saad AM, El-Saadony MT, Mohamed AS, Ahmed AI, Sitohy MZ. Impact of cucumber pomace fortification on the nutritional, sensorial and technological quality of soft wheat flour-based noodles. Int J Food Sci Technol. (2021) 56:3255–68. doi: 10.1111/ijfs.14970
270. Saad AM, Mohamed AS, El-Saadony MT, Sitohy MZ. Palatable functional cucumber juices supplemented with polyphenols-rich herbal extracts. LWT Food Sci Technol. (2021) 148:111668. doi: 10.1016/j.lwt.2021.111668
271. Sayed-Ahmed ETA, Salah KBH, El-Mekkawy RM, Rabie NA, Ashkan MF, Alamoudi SA, et al. The preservative action of protein hydrolysates from legume seed waste on fresh meat steak at 4°C: limiting unwanted microbial and chemical fluctuations. Polymers. (2022) 14:3188. doi: 10.3390/polym14153188
272. Gholami Z, Firouzi R, Rashedinia M. Evaluating the food colors in traditional confectionaries and beverages in Shiraz city. Trends Pharmacol Sci. (2021) 7:111–6.
273. Ghosh S, Sarkar T, Das A, Chakraborty R. Natural colorants from plant pigments and their encapsulation: An emerging window for the food industry. LWT Food Sci Technol. (2022) 153:112527. doi: 10.1016/j.lwt.2021.112527
274. Benucci I, Lombardelli C, Mazzocchi C, Esti M. Natural colorants from vegetable food waste: Recovery, regulatory aspects, and stability—a review. Compr Rev Food Sci Food Saf. (2022) 21:2715–37. doi: 10.1111/1541-4337.12951
275. Batu A, Molla E. Additives used in Turkish delight production. J Food Technol Elect. (2008) 27:33–6.
276. Saad AM, El-Saadony MT, El-Tahan AM, Sayed S, Moustafa MA, Taha AE, et al. Polyphenolic extracts from pomegranate and watermelon wastes as substrate to fabricate sustainable silver nanoparticles with larvicidal effect against Spodoptera littoralis. Saudi J Biol Sci. (2021) 28:5674–5683. doi: 10.1016/j.sjbs.2021.06.011
277. Abou-Kassem DE, Mahrose KM, El-Samahy RA, Shafi ME, El-Saadony MT, Abd El-Hack ME, Ashour EA. Influences of dietary herbal blend and feed restriction on growth, carcass characteristics and gut microbiota of growing rabbits. Ital J Anim Sci. (2021) 20:896–910. doi: 10.1080/1828051X.2021.1926348
278. Degot P, Huber V, Hofmann E, Hahn M, Touraud D, Kunz W. Solubilization and extraction of curcumin from Curcuma longa using green, sustainable, and food-approved surfactant-free microemulsions. Food Chem. (2021) 336:127660. doi: 10.1016/j.foodchem.2020.127660
279. Özer Ö. Determination of Some Quality Criteria hot Smoked Zargana Fish (Belone Belone euxini Günther, 1866) Preserved In Refrigerator Conditions by Using Natural and Artificial Colors. MSc. Thesis, Sinop University: Sinop. 108 p. 2010.
280. Ferguson JJ, Wolska A, Remaley AT, Stojanovski E, MacDonald-Wicks L, Garg ML. Bread enriched with phytosterols with or without curcumin modulates lipoprotein profiles in hypercholesterolaemic individuals. A randomised controlled trial. Food Funct. (2019) 10:2515–2527. doi: 10.1039/C8FO02512F
281. Roy S, Rhim J-W. Preparation of antimicrobial and antioxidant gelatin/curcumin composite films for active food packaging application. Colloids Surf B Biointerfaces. (2020) 188:110761. doi: 10.1016/j.colsurfb.2019.110761
282. Roy S, Rhim J-W. Preparation of bioactive functional poly (lactic acid)/curcumin composite film for food packaging application. Int J Biol Macromol. (2020) 162:1780–9. doi: 10.1016/j.ijbiomac.2020.08.094
283. Al-Obaidi LFH. Effect of adding different concentrations of turmeric powder on the chemical composition, oxidative stability and microbiology of the soft cheese. Plant Arch. (2019) 19:317–21.
284. de Carvalho FAL, Munekata PES, de Oliveira AL, Pateiro M, Domínguez R, Trindade MA, et al. Turmeric (Curcuma longa L.) extract on oxidative stability, physicochemical and sensory properties of fresh lamb sausage with fat replacement by tiger nut (Cyperus esculentus L.) oil. Food Res Int. (2020) 136:109487. doi: 10.1016/j.foodres.2020.109487
285. Gómez-Estaca J, Pintado T, Jiménez-Colmenero F, Cofrades S. The effect of household storage and cooking practices on quality attributes of pork burgers formulated with PUFA-and curcumin-loaded oleogels as healthy fat substitutes. LWT Food Sci Technol. (2020) 119:108909. doi: 10.1016/j.lwt.2019.108909
286. Ribeiro Oliveira A, Chaves Ribeiro AE, Resende Oliveira É, Oliveira Ribeiro K, Costa Garcia M, Careli-Gondim Í, et al. Physicochemical, microbiological and sensory characteristics of snacks developed from broken rice grains and turmeric powder. Int J Food Sci. (2020) 55:2719–29. doi: 10.1111/ijfs.14525
287. Farid F, Latifa G, Chakraborty S, Nahid M, Begum M. Combine effect of Curcuma longa (turmeric) powder and dry-salt with sun-drying in quality changes of three freshwater fish species of Meghna River. Eur J Biotechnol Biosci. (2016) 4:42–7.
288. Mancini S, Paci G, Preziuso G. Effect of dietary turmeric powder (Curcuma longa L.) on cooked pig meat quality. J Food Process Preserv. (2017) 41:e12878. doi: 10.1111/jfpp.12878
289. Mancini S, Preziuso G, Paci G. Effect of turmeric powder (Curcuma longa L.) and ascorbic acid on antioxidant capacity and oxidative status in rabbit burgers after cooking. World Rabbit Sci. (2016) 24:121–7. doi: 10.4995/wrs.2016.4207
290. Amin F, Islam N, Anila N, Gilani A. Clinical efficacy of the co-administration of turmeric and black seeds (Kalongi) in metabolic syndrome–A double blind randomized controlled trial–TAK-MetS trial. Complement Ther Med. (2015) 23:165–74. doi: 10.1016/j.ctim.2015.01.008
291. Chuengsamarn S, Rattanamongkolgul S, Phonrat B, Tungtrongchitr R, Jirawatnotai S. Reduction of atherogenic risk in patients with type 2 diabetes by curcuminoid extract: a randomized controlled trial. J Nutr Biochem. (2014) 25:144–50. doi: 10.1016/j.jnutbio.2013.09.013
292. Kocaadam B, Şanlier N. Curcumin, an active component of turmeric (Curcuma longa), and its effects on health. Crit Rev Food Sci Nutr. (2017) 57:2889–95. doi: 10.1080/10408398.2015.1077195
293. Burgos-Morón E, Calderón-Montaño JM, Salvador J, Robles A, López-Lázaro M. The dark side of curcumin. Int J Cancer. (2010) 126:1771–5. doi: 10.1002/ijc.24967
Keywords: bioavailability, cancer, curcumin, dietary additives, herbal treatment, polyphenolic pigment, metabolism
Citation: El-Saadony MT, Yang T, Korma SA, Sitohy M, Abd El-Mageed TA, Selim S, Al Jaouni SK, Salem HM, Mahmmod Y, Soliman SM, Mo’men SAA, Mosa WFA, El-Wafai NA, Abou-Aly HE, Sitohy B, Abd El-Hack ME, El-Tarabily KA and Saad AM (2023) Impacts of turmeric and its principal bioactive curcumin on human health: Pharmaceutical, medicinal, and food applications: A comprehensive review. Front. Nutr. 9:1040259. doi: 10.3389/fnut.2022.1040259
Received: 09 September 2022; Accepted: 09 November 2022;
Published: 10 January 2023.
Edited by:
Domenico Sergi, University of Ferrara, ItalyReviewed by:
Roberta Masella, National Institutes of Health (ISS), ItalyMariana Buranelo Egea, Goiano Federal Institute (IFGOIANO), Brazil
Copyright © 2023 El-Saadony, Yang, Korma, Sitohy, Abd El-Mageed, Selim, Al Jaouni, Salem, Mahmmod, Soliman, Mo’men, Mosa, El-Wafai, Abou-Aly, Sitohy, Abd El-Hack, El-Tarabily and Saad. This is an open-access article distributed under the terms of the Creative Commons Attribution License (CC BY). The use, distribution or reproduction in other forums is permitted, provided the original author(s) and the copyright owner(s) are credited and that the original publication in this journal is cited, in accordance with accepted academic practice. No use, distribution or reproduction is permitted which does not comply with these terms.
*Correspondence: Tao Yang, taoyang@hainmc.edu.cn; Khaled A. El-Tarabily, ktarabily@uaeu.ac.ae
†These authors have contributed equally to this work and share first authorship