- 1Department of Clinical Nutrition, Faculty of Nutrition and Food Sciences, Tabriz University of Medical Sciences, Tabriz, Iran
- 2Nutrition Research Center, Faculty of Nutrition and Food Sciences, Tabriz University of Medical Sciences, Tabriz, Iran
- 3Department of Community Nutrition, School of Nutritional Sciences and Dietetics, Tehran University of Medical Sciences, Tehran, Iran
- 4Student Research Committee, Department of Clinical Nutrition, School of Nutrition and Food Sciences, Isfahan University of Medical Sciences, Isfahan, Iran
- 5Department of Anesthesiology and Intensive Care, Faculty of Medicine, Tabriz University of Medical Sciences, Tabriz, Iran
- 6Research Center for Integrative Medicine in Aging, Aging Research Institute, Tabriz University of Medical Sciences, Tabriz, Iran
- 7Department of Immunology, School of Medicine, Tarbiat Modares University, Tehran, Iran
Introduction: In sepsis, the immune system is overreacting to infection, leading to organ dysfunction and death. The purpose of this study was to investigate the impacts of curcumin nanomicelles on clinical outcomes and cellular immune responses in critically ill sepsis patients.
Method: For 10 days, 40 patients in the intensive care units (ICU) were randomized between the nano curcumin (NC) and placebo groups in a randomized study. We evaluated serum levels of biochemical factors, inflammatory biomarkers, the mRNA expression levels of FOXP3, NLRP-3, IFN-γ, and NF-κp genes in the PBMCs, and clinical outcomes before the beginning of the supplementation and on days 5 and 10.
Results: NLR family pyrin domain containing 3 (NLRP3), interferon gamma (IFN-γ), and nuclear factor kappa-light-chain-enhancer of activated B cells (NF-κB) mRNA expression levels significantly P = 0.014, P = 0.014, and P = 0.019, respectively) decreased, but forkhead box P3 (FOXP3) mRNA expression levels increased significantly (P = 0.008) in the NC group compared to the placebo group after 10 days. NC supplementation decreased serum levels of IL-22, IL-17, and high mobility group box 1 (HMGB1) (P < 0.05). Nevertheless, biochemical factors and nutritional status did not differ significantly (P > 0.05). NC supplementation resulted in decreased sequential organ failure assessment and multiple organ dysfunction syndromes scores, while it did not have significant impacts on length of stay in the ICU, systolic blood pressure, diastolic blood pressure, a saturation of oxygen (%), and respiratory rate (breaths/min) PaO2/FiO2 (p > 0.05).
Conclusion: For critically ill patients with sepsis, NC supplementation may be an effective therapeutic strategy. More randomized clinical trials involving longer follow-up periods and different doses are needed to achieve the best results.
Introduction
Sepsis is a complex and severe disorder that is caused by a strong response of the body’s immune system to an infection. The disease is by far the most serious medical problem related to acute organ dysfunction and the high hazard of death in the intensive care unit (ICU) (1). The immune system’s excessive response leads to an enhancement in inflammatory oxidative stress and an increase in organ failure (2, 3). In the world, disease continues to be the leading cause of death (2, 3). Globally, sepsis affects an estimated 30 million people worldwide, and this number has increased annually by nine to 13 percent. According to global statistics, sepsis affected 48.9 million people worldwide in 2017 and caused 11.0 million deaths (4).
There are two parts to the human immune system: innate and adaptive (5). The innate immune system responds non-specifically to infections (6). Adaptive immunity is slower than innate immunity but may recognize unique antigens and establish immunity after multiple exposures. Innate system cells include basophils, mast cells, eosinophils, natural killer (NK) cells, dendritic cells, macrophages, and neutrophils (7). B and T cells comprise the adaptive immune system responding to pathogens (8). B cells generate antibodies and plasma cells for long-term immunity, whereas T cells, namely gamma delta (γδ), CD8+, CD4+, and regulatory T cells (Tregs), create plasma cells and antibodies for long-term immunity (9). In addition to activating immune responses, the entry of pathogens into the body leads to the activation of various inflammatory pathways, namely NLRs, HMGB-1, and NF-κB (10). The NLRs play a significant role in recognizing invading bacteria and initiating the innate immune response. Inflammasomes can be activated during sepsis to augment inflammatory responses (11). As a result of NLRP3 inflammasome activation, caspase-1 is activated, producing pro-inflammatory cytokines, IL-18, and IL-1β (12).
On the other hand, the activation of NLRP3 leads to up-regulated NF-κB pathway, which can cause the production of various swelling factors. The nuclear protein HMGB1 regulates innate immune responses both intracellularly and extracellularly and is found ubiquitously in almost all cells (13). HMGB1 also functions as an acute-phase cytokine during infection. Serum and tissue HMGB1 levels rise during infection, particularly in sepsis, and play a crucial role in systemic inflammation (14). On the other hand, the enhancement in the serum level of inflammatory cytokines causes a decrease in the level of albumin, urea, and BUN bilirubin, LDH, in sepsis patients (15). Impaired nutritional variables (energy intake and serum albumin) are expected to exacerbate clinical outcomes, namely sequential organ failure assessment (SOFA) and multiple organ dysfunction syndromes (MODS) score, PaO2/FiO2, duration of mechanical ventilation, and duration of ICU stay (16). In addition, sepsis is associated with an excessive reduction of forkhead box P3 (FOXP3) (12). FOXP3 regulates the development and activity of CD25+ CD4+ Treg cells, which play an important role in the immune response (12).
Notwithstanding the complexity of sepsis in people admitted to the ICU, a variety of treatments, such as corticosteroids and broad-spectrum antibiotics, are used today, but efforts to find effective treatment with minimal side effects are still ongoing (17). Accordingly, various studies indicate that natural immunomodulatory agents might ameliorate bacterial and virus diseases when combined with routine treatment (18). Rolta et al. (19), Rolta et al. (20) showed that herbal compounds can be used as an adjunctive treatment in corona. In other studies Rolta et al. (21) phytocompounds indicated (emodin, rhein13c6, chrysophenol dimethyl ether and resveratrol) have antibacterial and antifungal properties.
A hydrophobic polyphenol and an active component in turmeric is curcumin, derived from Curcuma longa rhizomes. Curcuminoids are comprised of three components, including bisdemethoxycurcumin (10 to 15%), demethoxycurcumin (20 to 27%), and curcumin (60 to 70%) (22). Numerous pieces of evidence show that curcumin has many pharmacological and therapeutic activities, including antimicrobial, antioxidant, and antiviral effects, anti-cancer, and anti-inflammatory (23). Curcumin works by targeting multiple biochemical pathways, such as reducing lipid peroxidation, increasing the expression of antioxidant-producing genes, attenuating NF-κB and NRLP3 signaling pathways, and, most importantly, modulating the immune system response (24). Although curcumin has many medicinal benefits, it is unfortunately absorbed in very small amounts due to its low bioavailability and rapid metabolism, which is exacerbated in patients admitted to the ICU (23). However, today, through effective methods such as the use of liposomes, the use of nanoparticles, including non-polar sandwich technology, nano micelles, complexing with phospholipids or piperine and solid lipid particle formulations leads to a substantial rise in the absorption of hydrophobic substances such as curcumin (25). Also, due to the proven advantageous impacts of curcumin in cell lines and on models of septic rats, as well as some human studies on sepsis (24), this randomized clinical trial (RCT) aims to the effects of NC on immune system responses and clinical outcomes in critically ill patients with sepsis.
Materials and methods
Study design
We conducted this study on the 40 hospitalized patients in the ICUs at Imam Reza and Shohada Hospitals (Tabriz University of Medical Sciences, Tabriz, Iran). Criteria for inclusion included critically ill patients feeding enteral nutrition and patients who had been in the ICU for at least 10 days. Furthermore, An exclusion criteria included participants with the following conditions: patients with intestinal ischemia, pancreatitis, intolerance to enteral feeding, short bowel syndrome, pregnant and lactating women, intestinal obstruction, and use of non-steroidal anti-inflammatory drugs (NSAIDs). Researchers registered the study at the Iranian Registry of Clinical Trials (IRCT) website (IRCT20110123005670N7). A nosocomial infection is defined by the most recent guidelines of the CDC (26).
Randomization and intervention
Patients in blocks arranged according to gender and age score were randomly divided into placebo or NC groups in a 1:1 ratio using RAS software. Both patients and researchers were blind to the allocation of the study. Patients in the supplementation group received routine therapy, namely antibiotics (Meropenem, Imipenem, Ciprofloxacin) with two 80 mg NC capsules, while the placebo group received routine therapy with a placebo for 10 days. Enteral feedings were administered through the nasogastric tube to all patients from their first 24 h of admission (Karen Company, Tehran, Iran; Table 1). Depending on each patient’s metabolic status and weight, the amount of energy required was calculated at 25 to 30 kcal/kg. Starting with 25 ml/h of enteral feeding, the rate was enhanced by 25 ml/h every 4 h until the aim rate was reached. In cases where the gastric residual volume exceeded 150 ml, prokinetic agents were administered. Exir-Nano-Sina company produced a placebo and NC capsules (batch number: 17003). In NC supplements, curcumin accounted for bis-desmethoxycurcumin for 3%, for 25%, and 72%, emethoxycurcumin. The NC formulation included polysorbate 80 as a component of the placebo capsules. A specialist evaluated patients according to inclusion criteria before enrolling them in the study. Nurses without knowing which is the placebo and the NC, every 12 h (9:00 a.m. and 9:00 p.m.), an hour later than enteral feeding (to prevent interaction with the contents of the formula received), NC capsules and placebo (in terms of form and size) were given to patients as a solution through a nasogastric tube.
Since the vast majority of patients with sepsis have a low Glasgow Coma Score, in this study, we obtained informed consent from first-degree (but legal) relatives such as mothers, fathers, sons, or daughters of patients before entering the study.
Laboratory evaluates
Before the intervention, 5th, and 10th, every day between 12:00 and 3:00 p.m., venous blood samples were taken from each patient. The biochemical factors, namely blood urea nitrogen (BUN), albumin, fasting blood sugar (FBS), hemoglobin, indirect bilirubin, direct bilirubin, lactate dehydrogenase (LDH) and total plasma protein, were specified using Abbott ALCYON-350 auto-analyzer kits. The blood samples of patients were centrifuged for 10 min at a speed of 2500 rpm (Beckman Avanti J-25 - Beckman Coulter, Brea, CA). The serum was stored at 70°C before biochemical assessments. According to dual biotin antibody sandwich technology, inflammatory markers (IL-17 and IL-22) were assessed using the enzyme-linked immunoassay (ELISA) method. In the present study, Human IL-17 and IL-22 ELISA kits made by the Assessment Technology Laboratory (Crystal Day Biotech Co., Ltd., Shanghai, China) and HMGB1 were used.
Peripheral blood mononuclear cells and RNA isolation
Whole blood samples were directly examined for isolation of peripheral blood mononuclear cells (PBMCs). Separating PBMCs by density gradient centrifugation was accomplished using Ficoll-Histopaque solution gradient centrifugation. To isolate total RNA from the blood, TRIzol was used (Sigma Aldrich, Germany). Quantitative and qualitative characteristics of extracted RNA were determined using a NanoDrop spectrophotometer (Nano-Drop One/Once, Thermo Scientific). Then, we performed reverse transcription with random hexamer primers and oligo (dT) to transform the total RNA into complementary DNA (cDNA) based on the producer’s instructions (BioFact, RTase, South Korea). Gel electrophoresis on 1% agarose gel was used to determine the RNA integration.
Real-time polymerase chain reaction for genes
Measuring the levels of mRNA expression levels of FOXP3, NLRP3, IFN-γ, and NF-kβ was done using real-time polymerase chain reaction (RT-PCR) (Sigma Aldrich, Germany). The manufacturer’s instructions were followed for the reverse transcription of 25 ng of total RNA and for constructing complementary DNA using reverse transcription reagent kits (Thermo Scientific, EU). With the Light-Cycler 480 instrument (Roche, Germany), qRT-PCR was performed in a volume of 10 μl using SYBR Green PCR Master Mix (Sigma-Aldrich, Germany). A three-phase thermal cycling procedure was conducted: phase one (primary denaturation: 95°C for two min), phase two (30 s at 63°C, and 30 s at 74°C, 34 to 42 cycles of 30 s at 96°C), and last phase to form the melt curve (5 min at 74°C). A Primer Bank sequence was used to design the primers. The characteristic primers for the human of FOXP3, NLRP-3, IFN-γ NF-kβ, and β-actin genes are resumed in Table 2. Using the 2–ΔΔCT method as the comparison of placebo/post-intervention, the relative expression levels for each gene were calculated for each reaction in triplicate.
Statistical analysis
In this study, sampling based on the standard equation (Pukak) and based on mean and standard deviation with a significance level test of 5% (α = 0.05) with considering 80% (β = 0.2) power and distance 95% confidence was performed. According to the method for calculating the sample size for clinical trials, each group’s sample size was 17 individuals (27). In addition, 20% of dropouts were taken into account, increasing this to 20 people. The data was analyzed using SPSS software version 24 (Chicago, IL, USA). For the assessment of the normal distribution of continuous variables, the Kolmogorov-Smirnov test was used. Quantitative data are presented as frequency (%). For normally distributed data, the means and standard deviations (SDs) are shown as a mean ± standard deviation; for non-normally distributed data, the Q1 and Q3. We used Mann–Whitney U, independent t-tests, and chi-square tests to compare group changes (endpoint minus baseline). A paired t-test was used to determine if there were significant differences between baseline and after the intervention. Analyzing covariance (ANCOVA) was used to eliminate confounding variables and examine differences between post-intervention groups.
Results
Characteristics of patients participating
In this clinical study, 81 patients were included in the study. Also, 41 patients were excluded from the study due to discharge, refusal to participate, and intolerance to enteral nutrition. A total of 20 patients in the NC group and 20 patients in the placebo group participated in the current study, as shown in Figure 1. Table 3 summarizes the demographic data of participants. The baseline characteristics of the participants did not significantly differ between the two groups.
Effect of nano curcumin on nutritional status
Between the NC group and placebo group, there were no considerable alterations in energy intake during the study period, as shown in Table 4.
Effect of nano curcumin on biochemical factors
In Table 5 the effect of curcumin on biochemical factors during the study stages is shown. Albeit serum levels of BUN, FBS, albumin, hemoglobin, total bilirubin, direct bilirubin, lactate, and total plasma protein decreased significantly in the NC group, the intergroup changes were not statistically significant.
Effect of nano curcumin on inflammatory factors
In Table 6, the effect of nano curcumin supplementation on inflammatory factors during the study stages is shown. There were no considerable alterations between the study groups in the baseline serum levels of HMGB-1, IL-17, and IL-22, however, compared to the placebo group, HMGB-1, IL-17, and IL-22 serum concentrations were significantly lower in the NC group after 10 days.
Effect of nano curcumin on forkhead box P3, NLR family pyrin domain containing 3, interferon gamma, and nuclear factor kappa B genes expression
Forkhead box P3 (FOXP3), NLR family pyrin domain containing 3 (NLRP-3), interferon gamma (IFN-γ), and nuclear factor kappa B (NF-kβ) levels did not change substantially after 5 days of supplementation with NC, as shown in Figure 2. In the NC group, FOXP3 expression considerably increased compared to the placebo group, after 10 days (fold change: 2.69 ± 0.99 vs. 3.24 ± 0.74 (P = 0.022) (Figure 2A). At the end of the study, NC supplementation significantly reduced mRNA expression of IFN-γ (fold change: 0.39 ± 0.11) compared to placebo (fold change: 1.26 ± 0.07). (P = 0.006) (Figure 2B). Also, on the 10th day, the NC group’s NF-kβ expression level (fold change: 1.13 ± 0.11) was higher than the placebo group’s fold change: 1.51 ± 0.07) (P = 0.014) (Figure 2C). Moreover, NC supplementation led to a significant decrease in NLRP-3 mRNA expression (fold change: 2.07 ± 0.79) than placebo (fold change: 2.99 ± 0.46), (P = 0.039) (Figure 2D).
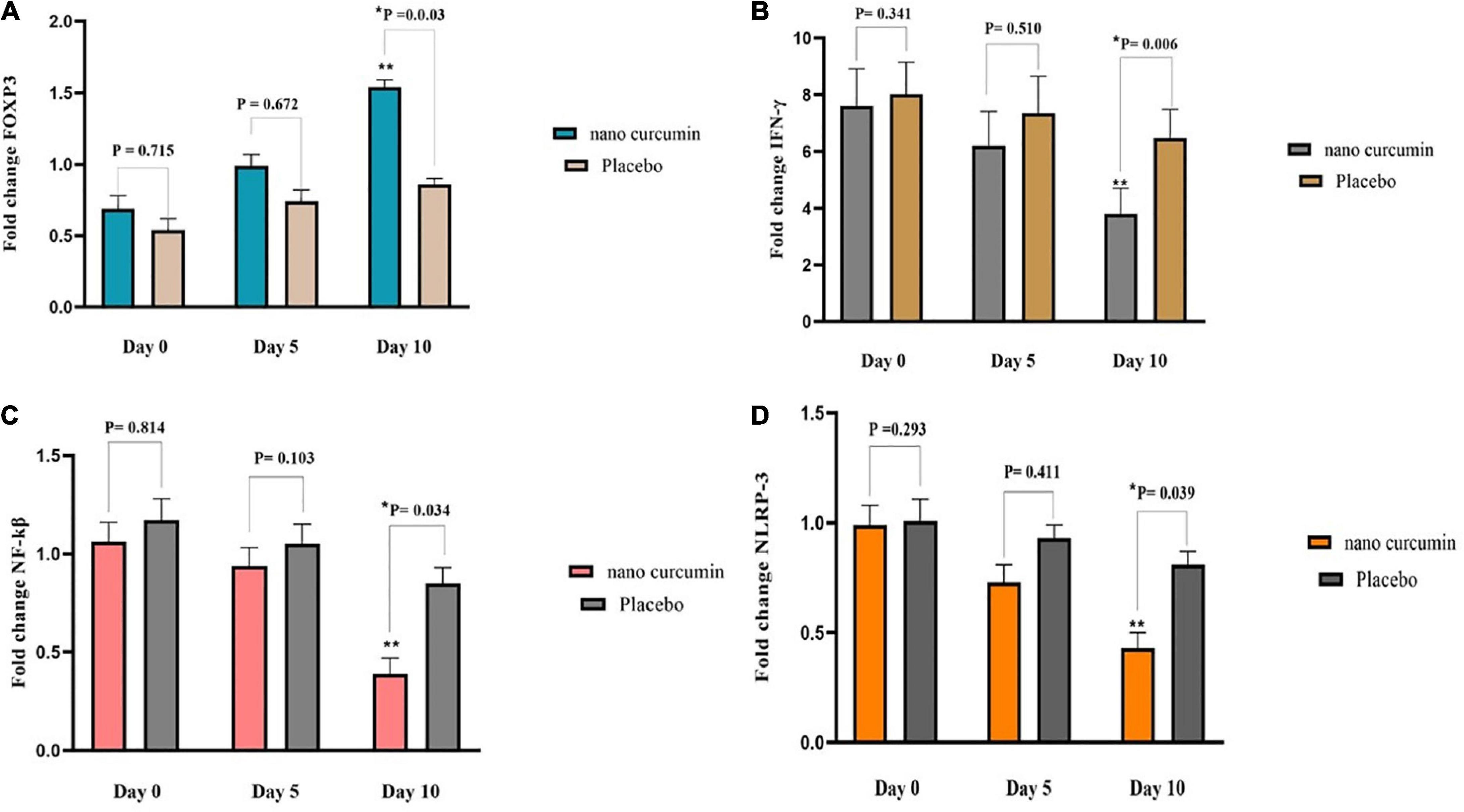
Figure 2. The effects of the intervention on FOXP3, NLRP3, IFN-γ, and NF-kβ expression in two study groups. (A) Fold change of FOXP3 (B) fold change of NLRP3 (C) fold change of IFN-γ (D) fold change of NF-kβ. Values are mean of fold change ± SEM. Data analysis was done using the ANCOVA test (adjusted for sex, age, type of disease, and baseline values; *p < 0.05 vs. placebo) and Repeated measures ANOVA (**p < 0.05 vs. baseline). P < 0.05, statistically significant. FOXP3, forkhead box P3; NF-κB, nuclear factor kappa B; NLRP3, NLR family pyrin domain containing 3; IFN-γ, interferon gamma.
Effect of nano curcumin on Clinical outcomes
In Table 7, effect of curcumin on clinical outcomes are presented for the participants. At the end of the study, the NC group’s MODS and SOFA scores decreased significantly compared to the placebo group (P < 0.05). Furthermore, there was no remarkable alteration between the two groups in the length of stay in the ICU, systolic blood pressure, dystopic blood pressure saturation (%), respiratory rate (breaths/min) PaO2/FiO2.
Discussion
The present RCT evaluated the impacts of NC supplementation on immune response in septic patients admitted to ICU. The current study revealed that 10 days of NC supplementation could significantly reduce IL-17, IL-22, SOFA, and MODS scores serum levels. It also decreased the mRNA expression of NLRP-3, NF-êB, HMGB-1, and IFN-γ genes and increased the mRNA expression of FOXP3. As far as we are aware, this is the first study to evaluate NC’s effect on immune response in patients with sepsis. Sepsis, which is defined as the excessive activity of the immune system in dealing with pathogenic factors, leads to systemic inflammatory response, coagulation disorders, endothelial function, and immune response (28), and it is a major cause of death in ICUs (29). We found that NC supplementation significantly decreased serum concentrations of IL-17 and IL-22 in septic patients after 10 days of intervention. Various studies have assessed the effect of curcumin on pro-inflammatory cytokines. A study conducted by Silva et al. (30) showed that treatment of septic rats with 100 mg/kg of curcumin remarkably lessened the pro-inflammatory cytokines, namely IL-1β and IL-6. In another study, Djalali et al. (31) reported that 2 months of NC supplementation declined the serum concentration of IL-17 and its mRNA expression in patients with episodic migraine. There is growing evidence that high concentrations of IL-17 are correlated to a higher peril of sepsis, which could provide a biomarker for the prognosis of sepsis (32). IL-17 interacts with various mediators, namely IL-1β, TNF-α, and IL-22 to exert its pro-inflammatory impact (33). Also, IL-22 plays a pivotal role in chronic inflammatory diseases and polymicrobial sepsis (34). In a trial conducted by Antiga et al. (27), curcumin (2g/day) supplementation considerably decreased the serum levels of IL-22 in participants with mild-to-moderate psoriasis Vulgaris. However, unlike the results of our study, curcumin did not significantly affect the serum level of IL-17 (27). This contrary finding might be due to the different underlying diseases of the participants and the distinct forms of curcumin used in the trials.
The inflammatory responses during sepsis might lead to the dysfunction of vital organs, including the lung, kidneys, heart, and liver, and thus, cause MODS (28). The number of organs engaged in MODS is positively correlated to the mortality of sepsis (35). Scores such as SOFA and MODS are used to properly identify septic patients at higher risk of mortality (29). In the present study, although NC supplementation did not affect the respiratory rate, PaO2/FiO2, and blood pressure, it significantly decreased the SOFA and MODS scores after 10 days. This finding is in line with the results of several animal studies (36, 37). Chen et al. (36) presented that supplementation with curcumin diminished tissue injury and improved survival rates in septic mice. Moreover, the results of another experimental study indicated that curcumin could prevent dysfunction of the kidneys, liver, and small bowel in rats with experimentally formed sepsis (37).
Additionally, the present study showed that septic patients who received NC for 10 days had significantly lower levels of NLRP-3. This finding was in agreement with an animal study that reported the suppression of NLRP-3 inflammasome activation in mice treated with a curcumin analog (38). Moreover, Gong et al. (39) indicated that curcumin could decrease the level of IL-1β by inhibiting the activation of NLRP-3 in an in vivo study. On the contrary, 12 weeks of curcumin supplementation among hemodialysis patients did not substantially influence NLRP-3 mRNA expression (40). This might be explained by different study sample sizes, carriers of the curcumin, as well as different participants of the studies. NLRP3 is a major component of the innate immune system that is prompted by pathogens and releases pro-inflammatory cytokines (41, 42).
Additionally, NC significantly reduced NF-kB expression in septic patients. In an animal study (43), both treatment and pretreatment with curcumin lessened NF-êB activation in renal tissues of septic rats. Xie et al. (44) also revealed that curcumin exerts its protective effects on lipopolysaccharide (LPS)/D-galactosamine (D-GalN)-induced acute liver injury in rats by up-regulating nuclear Nrf-2 and downregulate NF-êB. The activated NF-êB is a chief regulator of inflammatory gene expression, including NLRP-3 (Figure 3) (45).
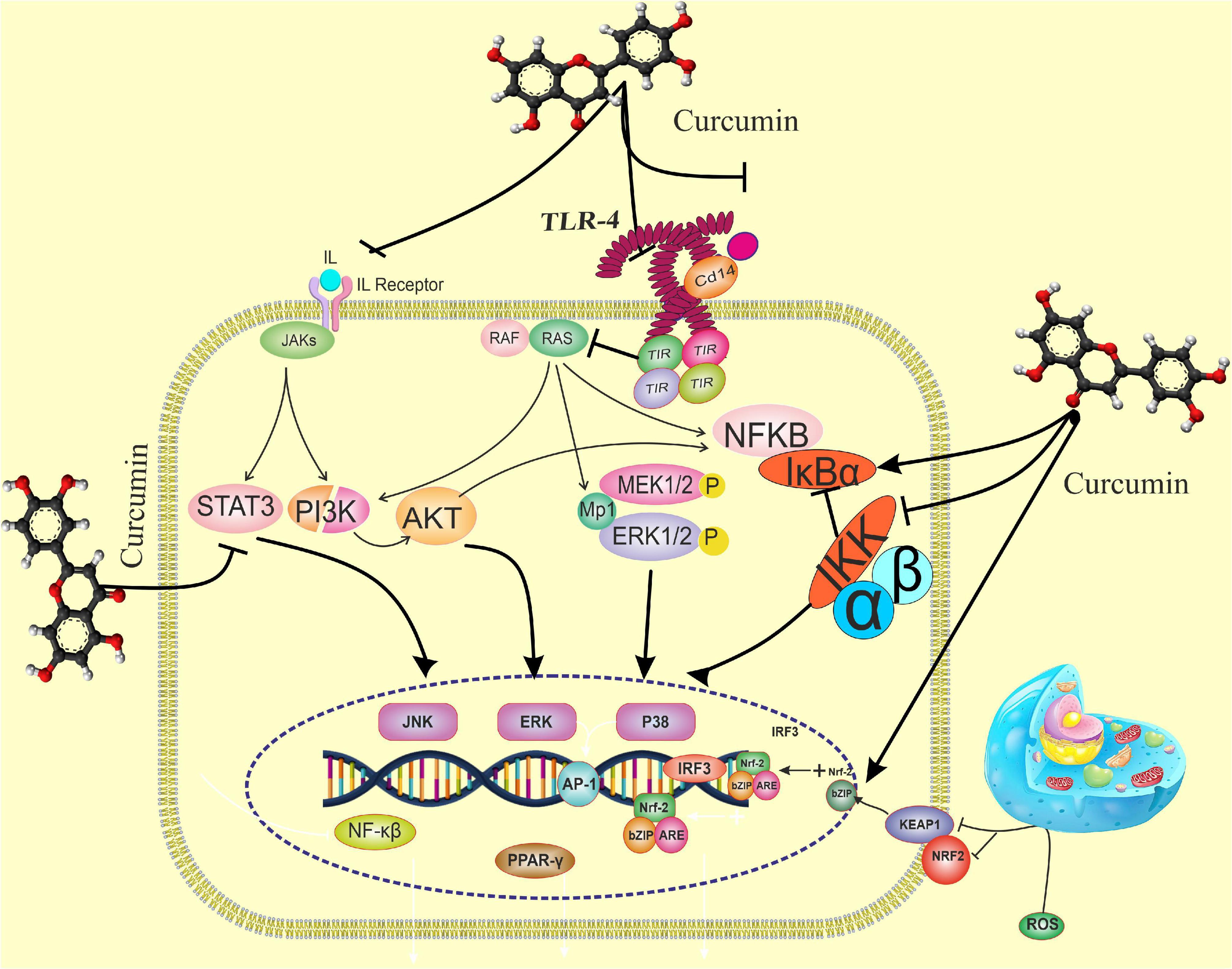
Figure 3. The effects of curcumin on the immune response pathway. Curcumin can bind directly to MD2 (protein appears to connect with toll-like receptor 4 on the cell surface). Curcumin also inhibits LPS-induced activation of MyD88- and TRIF-dependent TLR4 pathways, resulting in suppression of both IRF3 and NF-B. Curcumin promotes the expression of the Nrf-2 gene by boosting the antioxidant capacity and synthesis of antioxidant enzymes such as SOD, GPX CAT, and CAT. TANK-binding kinase 1; TRAF, TNF receptor-associated factor; TRAM, TRIF-related adaptor molecule; TRIF, TIR-domain- containing adapter-inducing interferon-β, Nrf-2, nuclear factor erythroid 2-related factor 2; p-IκBα, phosphorylated-IκBα; s; ROS, reactive oxygen species, STAT1, signal transducer and activator of transcription 1; TGF-1β, transforming growth factor-β1; TIRAP, toll-interleukin 1 receptor domain-containing adaptor protein; TLR4, toll-like receptor 4; TNF-α, tumor necrosis factor-α.
Several mechanisms have been suggested for the effects of curcumin administration on the components of the immune response mentioned above. Curcumin can down-regulate the Th1 and Th17 cell pathways and help modulate T-helper immune responses (27). It is speculated that curcumin adjusts the Treg/Th17 rebalance by inhibiting the IL-23/Th17 pathway (46). Another hypothesis is that curcumin down-regulates the expression of IL-22 and IL-17 indirectly by repressing IL-1β and IL-6 due to their synergistic activities with IL-17 (47). In addition, curcumin blocks the phosphorylation and degradation of IêB, the inhibitor protein of NF-êB, and averts the nuclear translocation of NF-êB (30). By inhibiting the activation of NF-êB, the transcription of genes engaged in the expression of pro-inflammatory cytokines is suppressed (48). Additionally, curcumin increases the expression of peroxisome proliferator−activated receptor gamma (PPARγ), which contributes to the suppression of NF-êB and lowers the release of pro-inflammatory cytokines (49).
The inhibition of the NF-êB pathway can alleviate the severity of MODS (50). Also, myeloperoxidase which represents polymorphonucleocytes infiltration, is a major indicator of tissue damage (50). By blocking myeloperoxidase activity, curcumin decreases tissue injury (37, 50). Curcumin’s anti-inflammatory and antioxidant properties generally reduce organ dysfunction during sepsis (36, 37, 50).
The important role of NF-κB activation is the regulation of NLRP3, ASC, and caspase-1 gene expression as well as the production of pro-inflammatory cytokines (51, 52). Thus, curcumin inhibits NF-êB signaling and suppresses NLRP-3 activation (53). Besides, curcumin attaches to peroxiredoxin 1 (PRDX1), which interacts with pro-caspase-1 and suppresses the link between pro-caspase-1 and ASC; therefore, the assembly of NLRP-3 inflammasome discontinues (38).
The mRNA expression level of HMGB1 was considerably reduced after 10 days of NC supplementation in patients with sepsis. During sepsis, high concentrations of HMGB-1 stimulate the production of pro-inflammatory cytokines, which are associated with MOD and mortality (54, 55). In accordance with our study, Ahn et al. (56) reported inhibiting the production of HMGB1 in endotoxemia mice with curcumin longa extract-loaded nanoemulsion. In addition, in a study by Kim et al. (57), curcumin inhibited the LPS-mediated release of HMGB-1 by endothelial cells and down-regulated the expression of HMGB-1 receptors. The proposed mechanism for the effect of curcumin on HMGB-1 is the blocking of nitric oxide due to the suppression of c-Jun N-terminal kinase, which leads to the inhibited release of HMGB-1 by macrophages (56).
Another finding of the current study was that mRNA expression of IFN-γ was considerably reduced after 10 days of NC supplementation. An experimental study by Gao et al. (58) indicated that curcumin treatment markedly suppressed the IFN-γ gene expression by splenic T lymphocytes. In addition, Kang et al. (59) demonstrated that in macrophages stimulated with LPS or heat-killed Listeria monocytogenes, pretreatment with curcumin decreased the production of IFN-γ. When concentrations of IFN-γ exceed a particular level in sepsis, resistance to infections is impaired, leading to an increased lethality rate (60, 61). Therefore, suppressing IFN-γ to normal levels is beneficial to the host due to preventing bacterial outflow (62). The mechanism by which curcumin reduces IFN-γ is that curcumin decreases CD4+ and IFN-γ+ and thereby inhibits the Th1 response (63). In addition, curcumin inhibits the Th1 cytokine profile by inhibiting IL-12 production (59).
The other immune response factor that we assessed in the current study was FOXP3. The results showed that NC supplementation considerably increased the mRNA expression of FOXP3. In line with our finding, Chen et al. (36) reported that curcumin administration elevates the expression of FOXP3 in septic mice compared to mice treated with corn oil. FOXP3, a key regulator of T regulatory (Treg) cell development and function, is expressed on CD4+ CD25+ Treg cells (64, 65). In a study by Chai et al. (66), curcumin attenuated the acute lung injury of cecal ligation and puncture-induced mouse model by boosting the differentiation of naïve CD4+ T cells to CD4+ CD25+ FOXP3+ Tregs. Regarding the mechanism underlying the effect of curcumin on FOXP-3, it is suggested that curcumin increases the CD4+, CD25+, and FOXP3+ Treg cells (36), which in turn increases the expression of anti-inflammatory cytokine IL-10 and decreases the proliferation activity of CD4+, CD25–, and T cells (36, 67).
So far as we are aware, no previous research has assessed the effect of NC on immune response among septic patients in ICU. In addition, randomizing the participants minimized the possibility of confounding factors. However, this study is not without limitations. First, the results cannot be generalized since we did not include refractory septic shock patients. Second, a longer supplementation duration and higher NC doses might lead to greater efficacy.
In conclusion, our results indicated that NC supplementation for 10 days in ICU patients with sepsis significantly decreased pro-inflammatory cytokines, MODS, and SOFA scores, the mRNA expression of NF-êB, NLRP-3, IFN-γ, and increased the expression of FOXP3. Further trials with a longer intervention period and larger sample size are warranted to confirm these findings.
Data availability statement
The original contributions presented in this study are included in the article/supplementary material, further inquiries can be directed to the corresponding author.
Ethics statement
The studies involving human participants were reviewed and approved by Tabriz University of Medical Sciences (IR.TBZMED.REC.1396.762). The patients/participants provided their written informed consent to participate in this study.
Author contributions
AK, SP, MV, AM, SS, and MK designed the first hypothesis of the work and searched the data. AK and MV read and extracted the data. AK and AT-E wrote the draft of the manuscript. All authors have read and approved the final manuscript.
Funding
We thank the Tabriz University of Medical Sciences, Tabriz, Iran, for provided the study grant to conduct this research.
Conflict of interest
The authors declare that the research was conducted in the absence of any commercial or financial relationships that could be construed as a potential conflict of interest.
Publisher’s note
All claims expressed in this article are solely those of the authors and do not necessarily represent those of their affiliated organizations, or those of the publisher, the editors and the reviewers. Any product that may be evaluated in this article, or claim that may be made by its manufacturer, is not guaranteed or endorsed by the publisher.
References
1. Dolin H, Papadimos T, Chen X, Pan Z. Characterization of pathogenic sepsis etiologies and patient profiles: a novel approach to triage and treatment. Microbiol Insights. (2019) 12:1178636118825081. doi: 10.1177/1178636118825081
3. Karimi A, Naeini F, Azar V, Hasanzadeh M, Niazkar H, Tutunchi H. A comprehensive systematic review of the therapeutic effects and mechanisms of action of quercetin in sepsis. Phytomedicine. (2021) 86:153567. doi: 10.1016/j.phymed.2021.153567
4. Rudd K, Johnson S, Agesa K, Shackelford K, Tsoi D, Kievlan D, et al. Global, regional, and national sepsis incidence and mortality, 1990-2017: analysis for the global burden of disease study. Lancet. (2020) 395:200–11.
5. Gloria Y, Fuchs K, Chang T, Engels P, Rusch E, Gouttefangeas C, et al. Chitin oligomers directly promote lymphoid innate and adaptive immune cell activation. bioRxiv. (2022) [Preprint]. doi: 10.1101/2022.04.06.487356
6. Wu C, Xu Y, Zhao Y. Two kinds of macrophage memory: innate and adaptive immune-like macrophage memory. Cell Mol Immunol. (2022) 19:852–4. doi: 10.1038/s41423-022-00885-y
7. Nalos M, Santner-Nanan B, Parnell G, Tang B, McLean AS, Nanan R. Immune effects of interferon gamma in persistent staphylococcal sepsis. Am J Respir Crit Care Med. (2012) 185:110–2. doi: 10.1164/ajrccm.185.1.110
8. Vos Q, Lees A, Wu Z, Snapper C, Mond J. B-cell activation by T-cell-independent type 2 antigens as an integral part of the humoral immune response to pathogenic microorganisms. Immunol Rev. (2000) 176:154–70. doi: 10.1034/j.1600-065x.2000.00607.x
9. Pati S, Chowdhury A, Mukherjee S, Guin A, Mukherjee S, Sa G. Regulatory lymphocytes: the dice that resolve the tumor endgame. Appl Cancer Res. (2020) 40:1–9.
10. Castellheim A, Brekke O, Espevik T, Harboe M, Mollnes T. Innate immune responses to danger signals in systemic inflammatory response syndrome and sepsis. Scand J Immunol. (2009) 69:479–91.
11. Wiersinga W, Leopold S, Cranendonk D, van Der Poll T. Host innate immune responses to sepsis. Virulence. (2014) 5:36–44.
12. Karimi A, Ghodsi R, Kooshki F, Karimi M, Asghariazar V, Tarighat-Esfanjani A. Therapeutic effects of curcumin on sepsis and mechanisms of action: a systematic review of preclinical studies. Phytother Res. (2019) 33:2798–820. doi: 10.1002/ptr.6467
13. Karimi A, Naeini F, Asghari Azar V, Hasanzadeh M, Ostadrahimi A, Niazkar H, et al. A comprehensive systematic review of the therapeutic effects and mechanisms of action of quercetin in sepsis. Phytomedicine. (2021) 86:153567.
14. Yang H, Wang H, Andersson U. Targeting inflammation driven by HMGB1. Front Immunol. (2020) 11:484. doi: 10.3389/fimmu.2020.00484
15. Gustot T, Durand F, Lebrec D, Vincent J, Moreau R. Severe sepsis in cirrhosis. Hepatology. (2009) 50:2022–33.
16. Sharath C. Use of SOFA (Sequential Organ Failure Assessment) Scoring in Assessing the Incidence and Severity of Organ Dysfunction and Predicting the Outcome in Patients with Sepsis in Surgical Unit. Coimbatore: Coimbatore Medical College (2016).
17. Warunek J, Jin R, Blair S, Garis M, Marzullo B, Wohlfert E. Tbet expression by regulatory T cells is needed to protect against Th1-mediated immunopathology during toxoplasma infection in mice. Immunohorizons. (2021) 5:931–43. doi: 10.4049/immunohorizons.2100080
18. Liew K, Hafiz M, Chong Y, Harith H, Israf D, Tham C. A review of Malaysian herbal plants and their active constituents with potential therapeutic applications in sepsis. Evid Based Complement Alternat Med. (2020) 2020:8257817. doi: 10.1155/2020/8257817
19. Rolta R, Salaria D, Sharma P, Sharma B, Kumar V, Rathi B, et al. Phytocompounds of Rheum emodi, Thymus serpyllum, and Artemisia annua inhibit spike protein of SARS-CoV-2 binding to ACE2 receptor: in silico approach. Curr Pharmacol Rep. (2021) 7:135–49. doi: 10.1007/s40495-021-00259-4
20. Rolta R, Yadav R, Salaria D, Trivedi S, Imran M, Sourirajan A, et al. In silico screening of hundred phytocompounds of ten medicinal plants as potential inhibitors of nucleocapsid phosphoprotein of COVID-19: an approach to prevent virus assembly. J Biomol Struct Dyn. (2021) 39:7017–34. doi: 10.1080/07391102.2020.1804457
21. Rolta R, Kumar V, Sourirajan A, Upadhyay N, Dev K. Bioassay guided fractionation of rhizome extract of Rheum emodi wall as bio-availability enhancer of antibiotics against bacterial and fungal pathogens. J Ethnopharmacol. (2020) 257:112867. doi: 10.1016/j.jep.2020.112867
22. Karimi A, Mahmoodpoor A, Kooshki F, Niazkar H, Shoorei H, Tarighat-Esfanjani A. Effects of nanocurcumin on inflammatory factors and clinical outcomes in critically ill patients with sepsis: a pilot randomized clinical trial. Eur J Integr Med. (2020) 36:101122.
23. Naeini F, Tutunchi H, Razmi H, Mahmoodpoor A, Vajdi M, Sefidmooye Azar P, et al. Does nano-curcumin supplementation improve hematological indices in critically ill patients with sepsis? a randomized controlled clinical trial. J Food Biochem. (2022) 46:e14093. doi: 10.1111/jfbc.14093
24. Karimi A, Naeini F, Niazkar H, Tutunchi H, Musazadeh V, Mahmoodpoor A, et al. Nano-curcumin supplementation in critically ill patients with sepsis: a randomized clinical trial investigating the inflammatory biomarkers, oxidative stress indices, endothelial function, clinical outcomes and nutritional status. Food Funct. (2022) 13:6596–612. doi: 10.1039/d1fo03746c
25. Ferguson J, Abbott K, Garg M. Anti-inflammatory effects of oral supplementation with curcumin: a systematic review and meta-analysis of randomized controlled trials. Nutr Rev. (2021) 79:1043–66.
26. Mayhall C. Hospital Epidemiology and Infection Control. Philadelphia, PA: Lippincott Williams & Wilkins (2012).
27. Antiga E, Bonciolini V, Volpi W, Del Bianco E, Caproni M. Oral curcumin (Meriva) Is effective as an adjuvant treatment and is able to reduce IL-22 serum levels in patients with psoriasis vulgaris. Biomed Res Int. (2015) 2015:283634. doi: 10.1155/2015/283634
28. Chen F, Jiang Y, Liu S, Zou L, Cao Y, Zhu Y. The expression changes and correlation analysis of high mobility group box-1 and tissue factor in the serum of rats with sepsis. Eur Rev Med Pharmacol Sci. (2019) 23:1634–40. doi: 10.26355/eurrev_201902_17123
29. Cai J, Lin Z. Correlation of blood high mobility group box-1 protein with mortality of patients with sepsis: a meta-analysis. Heart Lung. (2021) 50:885–92. doi: 10.1016/j.hrtlng.2021.07.010
30. Silva L, Catalão C, Felippotti T, Oliveira-Pelegrin G, Petenusci S, de Freitas L, et al. Curcumin suppresses inflammatory cytokines and heat shock protein 70 release and improves metabolic parameters during experimental sepsis. Pharm Biol. (2017) 55:269–76. doi: 10.1080/13880209.2016.1260598
31. Djalali M, Abdolahi M, Hosseini R, Miraghajani M, Mohammadi H, Djalali M. The effects of nano-curcumin supplementation on Th1/Th17 balance in migraine patients: a randomized controlled clinical trial. Complement Therap Clin Pract. (2020) 41:101256. doi: 10.1016/j.ctcp.2020.101256
32. Ge Y, Huang M, Yao Y. Biology of Interleukin-17 and its pathophysiological significance in sepsis. Front Immunol. (2020) 11:1558. doi: 10.3389/fimmu.2020.01558
33. Cua D, Tato C. Innate IL-17-producing cells: the sentinels of the immune system. Nat Rev Immunol. (2010) 10:479–89.
34. Weber G, Schlautkötter S, Kaiser-Moore S, Altmayr F, Holzmann B, Weighardt H. Inhibition of interleukin-22 attenuates bacterial load and organ failure during acute polymicrobial sepsis. Infect Immun. (2007) 75:1690–7. doi: 10.1128/IAI.01564-06
35. Vachharajani V, Wang S, Mishra N, El Gazzar M, Yoza B, McCall C. Curcumin modulates leukocyte and platelet adhesion in murine sepsis. Microcirculation. (2010) 17:407–16. doi: 10.1111/j.1549-8719.2010.00039.x
36. Chen L, Lu Y, Zhao L, Hu L, Qiu Q, Zhang Z, et al. Curcumin attenuates sepsis-induced acute organ dysfunction by preventing inflammation and enhancing the suppressive function of Tregs. Int Immunopharmacol. (2018) 61:1–7. doi: 10.1016/j.intimp.2018.04.041
37. Memis D, Hekimoglu S, Sezer A, Altaner S, Sut N, Usta U. Curcumin attenuates the organ dysfunction caused by endotoxemia in the rat. Nutrition. (2008) 24:1133–8. doi: 10.1016/j.nut.2008.06.008
38. Liu W, Guo W, Zhu Y, Peng S, Zheng W, Zhang C, et al. Targeting peroxiredoxin 1 by a curcumin analogue, AI-44, Inhibits NLRP3 inflammasome activation and attenuates lipopolysaccharide-induced sepsis in mice. J Immunol. (2018) 201:2403–13. doi: 10.4049/jimmunol.1700796
39. Gong Z, Zhou J, Li H, Gao Y, Xu C, Zhao S, et al. Curcumin suppresses NLRP3 inflammasome activation and protects against LPS-induced septic shock. Mol Nutr Food Res. (2015) 59:2132–42. doi: 10.1002/mnfr.201500316
40. Alvarenga L, Salarolli R, Cardozo L, Santos R, de Brito J, Kemp J, et al. Impact of curcumin supplementation on expression of inflammatory transcription factors in hemodialysis patients: a pilot randomized, double-blind, controlled study. Clin Nutr. (2020) 39:3594–600.
41. Danielski L, Giustina A, Bonfante S, Barichello T, Petronilho F. The NLRP3 inflammasome and its role in sepsis development. Inflammation. (2020) 43:24–31.
42. Chen B, Li H, Ou G, Ren L, Yang X, Zeng M. Curcumin attenuates MSU crystal-induced inflammation by inhibiting the degradation of IκBα and blocking mitochondrial damage. Arthritis Res Ther. (2019) 21:193. doi: 10.1186/s13075-019-1974-z
43. Abd-Elrazek A, Mahmoud S, Abd ElMoneim A. The comparison between curcumin and propolis against sepsis-induced oxidative stress, inflammation, and apoptosis in kidney of adult male rat. Future J Pharm Sci. (2020) 6:1–13.
44. Xie Y, Chu J, Jian X, Dong J, Wang L, Li G, et al. Curcumin attenuates lipopolysaccharide/d-galactosamine-induced acute liver injury by activating Nrf2 nuclear translocation and inhibiting NF-kB activation. Biomed Pharmacother. (2017) 91:70–7. doi: 10.1016/j.biopha.2017.04.070
45. Selvaraj V, Nepal N, Rogers S, Manne N, Arvapalli R, Rice K, et al. Inhibition of MAP kinase/NF-kB mediated signaling and attenuation of lipopolysaccharide induced severe sepsis by cerium oxide nanoparticles. Biomaterials. (2015) 59:160–71. doi: 10.1016/j.biomaterials.2015.04.025
46. Wei C, Wang J, Xiong F, Wu B, Luo M, Yu Z, et al. Curcumin ameliorates DSS-induced colitis in mice by regulating the Treg/Th17 signaling pathway. Mol Med Rep. (2021) 23:34. doi: 10.3892/mmr.2020.11672
47. Sun J, Zhao Y, Hu J. Curcumin inhibits imiquimod-induced psoriasis-like inflammation by inhibiting IL-1beta and IL-6 production in mice. PLoS One. (2013) 8:e67078. doi: 10.1371/journal.pone.0067078
48. Wang Q, Ye C, Sun S, Li R, Shi X, Wang S, et al. Curcumin attenuates collagen-induced rat arthritis via anti-inflammatory and apoptotic effects. Int Immunopharmacol. (2019) 72:292–300. doi: 10.1016/j.intimp.2019.04.027
49. Siddiqui A, Cui X, Wu R, Dong W, Zhou M, Hu M, et al. The anti-inflammatory effect of curcumin in an experimental model of sepsis is mediated by up-regulation of peroxisome proliferator-activated receptor-gamma. Crit Care Med. (2006) 34:1874–82. doi: 10.1097/01.CCM.0000221921.71300.BF
50. Liu S, Zhang J, Pang Q, Song S, Miao R, Chen W, et al. The protective role of curcumin in Zymosan-induced multiple organ dysfunction syndrome in mice. Shock. (2016) 45:209–19. doi: 10.1097/SHK.0000000000000502
51. Chen S, Tang C, Ding H, Wang Z, Liu X, Chai Y, et al. Maf1 ameliorates sepsis-associated encephalopathy by suppressing the NF-kB/NLRP3 inflammasome signaling pathway. Front Immunol. (2020) 11:594071. doi: 10.3389/fimmu.2020.594071
52. Luo M, Yan D, Sun Q, Tao J, Xu L, Sun H, et al. Ginsenoside Rg1 attenuates cardiomyocyte apoptosis and inflammation via the TLR4/NF-kB/NLRP3 pathway. J Cell Biochem. (2020) 121:2994–3004. doi: 10.1002/jcb.29556
53. Ran Y, Su W, Gao F, Ding Z, Yang S, Ye L, et al. Curcumin ameliorates white matter injury after ischemic stroke by inhibiting microglia/macrophage pyroptosis through NF-κB suppression and NLRP3 inflammasome inhibition. Oxid Med Cell Longev. (2021) 2021:1552127. doi: 10.1155/2021/1552127
54. Yang H, Ochani M, Li J, Qiang X, Tanovic M, Harris H, et al. Reversing established sepsis with antagonists of endogenous high-mobility group box 1. Proc Natl Acad Sci USA. (2004) 101:296–301. doi: 10.1073/pnas.2434651100
55. Yoo H, Im Y, Ko R, Lee J, Park J, Jeon K. Association of plasma level of high-mobility group box-1 with necroptosis and sepsis outcomes. Sci Rep. (2021) 11:9512. doi: 10.1038/s41598-021-88970-6
56. Ahn M, Hwang J, Lee S, Ham S, Hur J, Kim J, et al. Curcumin longa extract-loaded nanoemulsion improves the survival of endotoxemic mice by inhibiting nitric oxide-dependent HMGB1 release. PeerJ. (2017) 5:e3808. doi: 10.7717/peerj.3808
57. Kim D, Lee W, Bae J. Vascular anti-inflammatory effects of curcumin on HMGB1-mediated responses in vitro. Inflamm Res. (2011) 60:1161–8. doi: 10.1007/s00011-011-0381-y
58. Gao X, Kuo J, Jiang H, Deeb D, Liu Y, Divine G, et al. Immunomodulatory activity of curcumin: suppression of lymphocyte proliferation, development of cell-mediated cytotoxicity, and cytokine production in vitro. Biochem Pharmacol. (2004) 68:51–61. doi: 10.1016/j.bcp.2004.03.015
59. Kang B, Song Y, Kim K, Choe Y, Hwang S, Kim T. Curcumin inhibits Th1 cytokine profile in CD4+ T cells by suppressing interleukin-12 production in macrophages. Br J Pharmacol. (1999) 128:380–4. doi: 10.1038/sj.bjp.0702803
60. Souza-Fonseca-Guimaraes F, Parlato M, Philippart F, Misset B, Cavaillon J, Adib-Conquy M. Toll-like receptors expression and interferon-γ production by NK cells in human sepsis. Crit Care. (2012) 16:R206. doi: 10.1186/cc11838
61. Schütze S, Kaufmann A, Bunkowski S, Ribes S, Nau R. Interferon-gamma impairs phagocytosis of Escherichia coli by primary murine peritoneal macrophages stimulated with LPS and differentially modulates proinflammatory cytokine release. Cytokine. (2021) 3:100057. doi: 10.1016/j.cytox.2021.100057
62. Wang D, Zhong X, Huang D, Chen R, Bai G, Li Q, et al. Functional polymorphisms of interferon-gamma affect pneumonia-induced sepsis. PLoS One. (2014) 9:e87049. doi: 10.1371/journal.pone.0087049
63. Kanakasabai S, Casalini E, Walline C, Mo C, Chearwae W, Bright J. Differential regulation of CD4(+) T helper cell responses by curcumin in experimental autoimmune encephalomyelitis. J Nutr Biochem. (2012) 23:1498–507. doi: 10.1016/j.jnutbio.2011.10.002
64. Bao R, Hou J, Li Y, Bian J, Deng X, Zhu X, et al. Adenosine promotes Foxp3 expression in Treg cells in sepsis model by activating JNK/AP-1 pathway. Am J Transl Res. (2016) 8:2284–92.
65. Forward N, Conrad D, Power Coombs M, Doucette C, Furlong S, Lin T, et al. Curcumin blocks interleukin (IL)-2 signaling in T-lymphocytes by inhibiting IL-2 synthesis, CD25 expression, and IL-2 receptor signaling. Biochem Biophys Res Commun. (2011) 407:801–6. doi: 10.1016/j.bbrc.2011.03.103
66. Chai Y, Chen Y, Lin S, Xie K, Wang C, Yang Y, et al. Curcumin regulates the differentiation of naïve CD4+T cells and activates IL-10 immune modulation against acute lung injury in mice. Biomed Pharmacother. (2020) 125:109946. doi: 10.1016/j.biopha.2020.109946
Keywords: curcumin, immune response, clinical outcome, sepsis, inflammation
Citation: Karimi A, Pourreza S, Vajdi M, Mahmoodpoor A, Sanaie S, Karimi M and Tarighat-Esfanjani A (2022) Evaluating the effects of curcumin nanomicelles on clinical outcome and cellular immune responses in critically ill sepsis patients: A randomized, double-blind, and placebo-controlled trial. Front. Nutr. 9:1037861. doi: 10.3389/fnut.2022.1037861
Received: 06 September 2022; Accepted: 21 November 2022;
Published: 06 December 2022.
Edited by:
Nicola Fiotti, University of Trieste, ItalyReviewed by:
Israr Ahmad, University of Alabama at Birmingham, United StatesJyoti Mehta, Shoolini University of Biotechnology and Management Sciences, India
Copyright © 2022 Karimi, Pourreza, Vajdi, Mahmoodpoor, Sanaie, Karimi and Tarighat-Esfanjani. This is an open-access article distributed under the terms of the Creative Commons Attribution License (CC BY). The use, distribution or reproduction in other forums is permitted, provided the original author(s) and the copyright owner(s) are credited and that the original publication in this journal is cited, in accordance with accepted academic practice. No use, distribution or reproduction is permitted which does not comply with these terms.
*Correspondence: Ali Tarighat-Esfanjani, dGFyaWdoYXRhQHRiem1lZC5hYy5pcg==