- 1Department of Hematology and Oncology, International Cancer Center, Shenzhen Key Laboratory, Shenzhen University General Hospital, Shenzhen University Clinical Medical Academy, Shenzhen University Health Science Center, Shenzhen University, Shenzhen, China
- 2School of Medicine, Shenzhen University, Shenzhen, China
Advanced glycation end products (AGEs) are generated by the nonenzymatic glycation of proteins or lipids. Diabetic retinopathy (DR) is one common complication in patients with diabetes. The accumulation of AGEs in retinal cells is strongly associated with the development of DR. AGEs can induce the breakdown of redox balance and then cause oxidative stress in retinal cells, exerting cytopathic effects in the progression of DR. The interaction between AGEs and the receptor for AGE (RAGE) is involved in multiple cellular pathological alterations in the retina. This review is to elucidate the pathogenetic roles of AGEs in the progression of DR, including metabolic abnormalities, lipid peroxidation, structural and functional alterations, and neurodegeneration. In addition, disorders associated with AGEs can be used as potential therapeutic targets to explore effective and safe treatments for DR. In this review, we have also introduced antioxidant phytochemicals as potential therapeutic strategies for the treatment of DR.
Introduction
Advanced glycation end products (AGEs), a group of heterogeneous complexes, are produced from non-enzymatic glycation that happens between reducing sugars, a free amino group, nucleic acids, proteins, or lipids through the Maillard reaction (1). The formation of AGEs occurs either from foods or metabolized biological process in the physiological system. Foods containing high protein, sugar, fat, moisture, etc., easily result in the formation and accumulation of AGEs during processing or storage. Long-term AGE intake can lead to their cumulation in body fluids, contributing to the outbreak of chronic disorders and toxic pathogenesis in our body (2), especially diabetic complications. In addition, the generation of AGEs produces free radicals—reactive oxygen species (ROS) to facilitate redox imbalance, finally resulting in oxidative stress, and ROS can in turn prompt the formation of AGEs. ROS attacks functional biomacromolecules such as deoxyribonucleic acid (DNA), proteins, and lipids, subsequently affecting normal biological activity and inducing physiological dysfunction.
Diabetic retinopathy (DR) can impose a sight-threatening effect on the eyes, and it is generally classified as a microvasculature complication in diabetes (3). It is commonly recognized that DR is the main reason for vision loss or visual damage among elderly people or working-age adults worldwide (4). The amount of patients influenced by DR will ascend to 191 million in 2030 (5). Despite numerous studies, the mechanism underlying hyperglycemia-induced pathology in the retina still remains elusive. It has been shown that hyperglycemia-mediated progression of retinopathy is tightly associated with the abnormality of multiple metabolic pathways, in which the accumulation of AGEs plays a critical role (5). AGEs, such as carboxyethyllysine (CEL), carboxymethyllysine (CML), and pentosidine, have been well chemically characterized in the body. The occurrence of these AGEs is closely correlated with the severity of DR. For example, CML has been usually found in the retinal blood vessels of patients with diabetes (6). In addition, in the formation process of AGEs, proteins are structurally impaired by this non-enzymatic cross-link between amino groups and reducing sugars. One instance is the nonenzymatic glycation of elastin and collagen that are deeply involved in the formation of the stiffer blood vessels in DR (7).
Formation mechanism of AGEs
The reaction between proteins and reducing sugars or carbonyl groups is known as the Maillard reaction, which non-enzymatically alters the function and morphology of these biological molecules (8). It mainly includes three levels (Figure 1). The primary level is the formation of the Schiff base in the reaction of free amino groups and glucose. In the existence of acid–base catalysis, the Schiff base is unstable and undergoes rearrangements to generate stable glycation—Amadori products that are early glycosylation products and mainly carbonyl compounds (Figure 1) (9). The second level produces reactive dicarbonyl compounds through chemical reactions of oxidation and dehydration, generating the precursors of AGEs (Figure 1). These reactive dicarbonyl compounds, such as glyoxal and deoxyglucosones compounds, react with free amino groups, proteins, DNA, or lipids (9) and undergo further cyclization reactions (the third level) to produce irreversible AGEs (Figure 1).
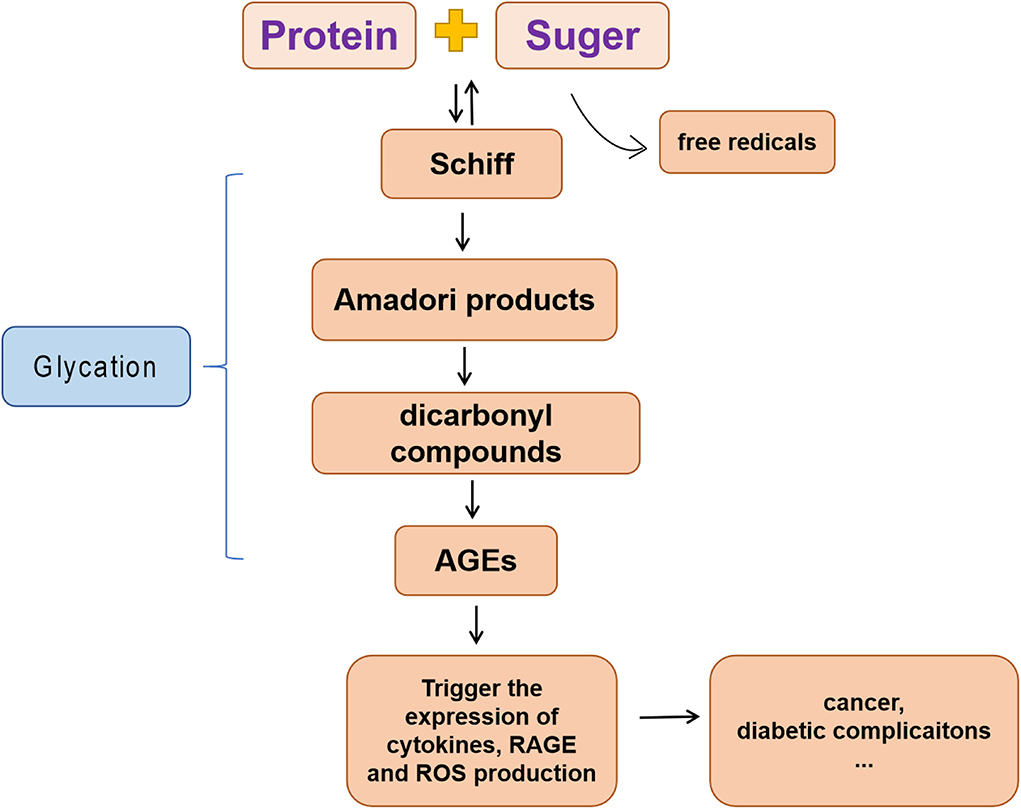
Figure 1. The formation of advanced glycation end products (AGEs). A schematic representation of the formation of AGE and the interaction with the receptor for AGE (RAGE). Glycation includes three main levels and promotes various diseases, such as diabetic complications, cancer, and so on.
AGEs and the receptor for AGE (RAGE) axis in DR
Advanced glycation end products and the receptor for AGE (RAGE) axis comprises AGE and RAGE. AGE-mediated damage occurs mainly through its interaction with RAGE on the cell membrane. The binding of AGEs and RAGE activates downstream signaling pathways, such as nuclear factor-κB (NF-κB) signaling pathway, transforming growth factor-β (TGF-β) pathway, Jak-STAT pathway, PI3K-Akt pathway, and so on, which are involved in the cellular processes of inflammation, apoptosis, autophagy, carcinogenesis, angiogenesis, and nephropathy and vasculopathy (10) (Figure 2). The binding of RAGE with AGEs can prompt the activation of the inflammatory factor NF-κB, which subsequently leads to pericyte apoptosis, and can also augment the expression of vascular endothelial growth factor (VEGF) to incur vascular endothelial permeability in the retina (6, 11). AGEs and the RAGE axis can also boost the expression of RAGE through enhanced downstream cellular signaling pathways (5).
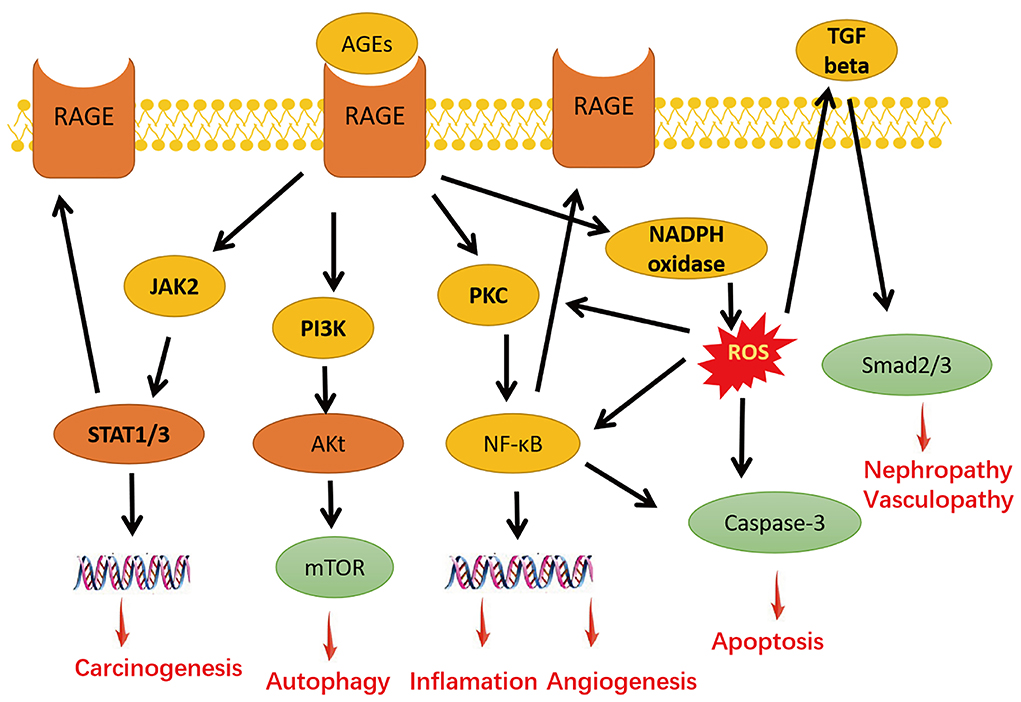
Figure 2. The AGE and RAGE axis and its downstream signaling pathways. The interaction of AGE–RAGE activates downstream signaling, including nuclear factor-κB (NF-κB) signaling pathway, transforming growth factor-β (TGF-β) pathway, Jak-STAT pathway, PI3K-Akt pathway, to promote inflammation, apoptosis, autophagy, angiogenesis, and nephropathy and vasculopathy, and induces the generation of reactive oxygen species (ROS) through nicotinamide adenine dinucleotide phosphate (NADPH) oxidase.
The interaction of AGEs and RAGE generates ROS via activating nicotinamide adenine dinucleotide phosphate (NADPH) oxidase, which induces the activation of NF-κB and the elevation of proinflammatory cytokines. Proinflammatory cytokines upregulate the expression of NADPH oxidase (Nox) and augment the production of ROS (12). The production of AGE-induced ROS also participates and plays an important role in the pathophysiological progression of DR (13, 14). Periodic injection of AGEs into rats can cause retinal hyperpermeability and leukostasis while augmenting the level of RAGE and ROS; however, concurrent injection of pigment epithelium-derived factor (PEDF) can block this process via suppressing AGE-mediated ROS generation, NF-κB activity, and VEGF levels (15–17). Additionally, it has been suggested that the production of ROS can also be stimulated by AGEs via mitochondrial electron transport chain (18). As a consequence, the enrichment of ROS contributes to the accumulation of AGE (19) and the upregulation of RAGE (20), to aggravate all AGE-induced damages.
Pathogenetic roles
AGEs and metabolic abnormalities in DR
Metabolic disorders play a critical role in the progression of DR, including enhanced glucose influx via hexosamine and polyol pathways and hyperactivated protein kinase C (PKC) pathway. These disorders promote the accumulation of AGEs in retinal cells and interact with AGEs to amplify these metabolic disorders, leading to the dysfunction of retinal tissues (Figure 3).
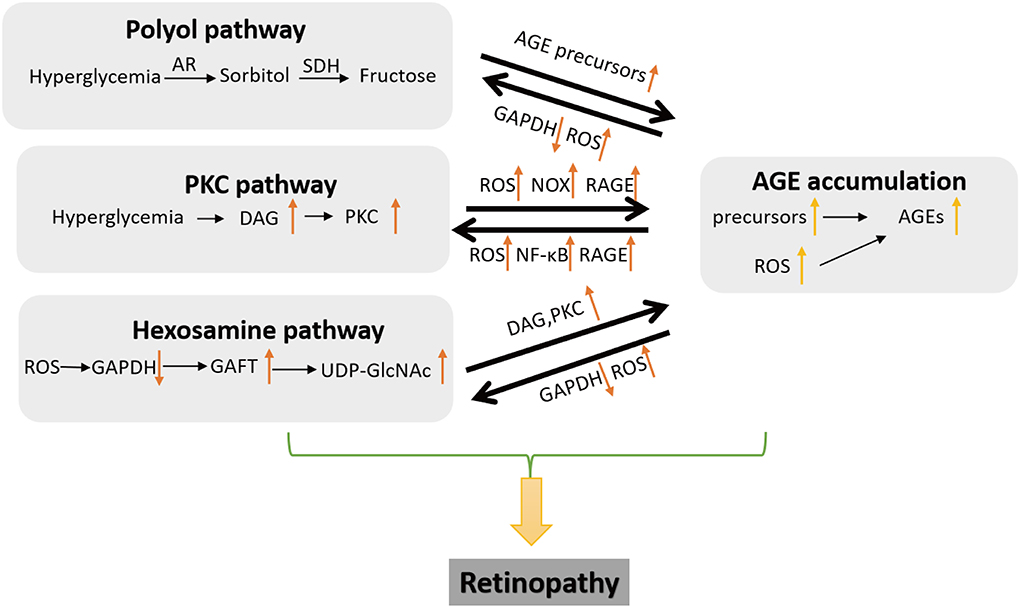
Figure 3. Metabolic abnormalities in a diabetic complication. Major disorders are polyol pathway, hexosamine biosynthesis pathway, and the activation of protein kinase C (PKC). Polyol pathway promotes the generation of AGE precursors. The activation of PKC induces the expression of vascular endothelial growth factor (VEGF) and RAGE and increase of ROS. Hexosamine pathway causes glycosylation of transcription factors and the generation of diphosphate uracil-N-acetylglucosamine (UDP-GlcNAc). These disorders interact with AGEs to promote the pathogenesis of diabetic retinopathy (DR).
AGEs and polyol pathway
Glucose metabolism in polyol pathway is active in the hyperglycemic situation, which facilitates the formation of AGEs (21). In this pathway, glucose is catalyzed by aldose reductase, the first and rate-limiting enzyme, for its conversion into sorbitol with NADPH as the electron donor. Then, the sorbitol dehydrogenase oxidizes sorbitol into fructose, combined with the transfer of NAD into NADH (22, 23). In this process, the intermediate sorbitol, as hydrophilic alcohol, is difficult to diffuse through the cellular membrane, which brings about cell hypertonicity and the augmented osmotic pressure to finally induce osmotic damage of a retinal capillary (5). Notably, fructose produced as a byproduct can be phosphorylated into fructose-3-phosphate and then degenerated into 3-deoxyglucosone, both of which can be used as precursors to generate AGEs through non-enzymatic glycosylation (24).
AGEs and PKC pathway
Protein kinase C pathway is also deeply involved in the modulation of multiple physiological alterations in retinal tissues, including endothelial permeability, the upregulation of VEGF, retinal hemodynamics, and the adhesion and hyper-activation of leukocytes (leukostasis) in retinal cells (23, 25, 26). In addition, Nox can be positively modulated by PKC, which facilitates the generation of ROS in numerous vascular cells, such as pericytes, endothelial cells, and others (27, 28). The enrichment of ROS production in turn prompts the formation of AGE. Extracellular AGEs can combine its transmembrane receptor RAGE to activate PKC pathway to intensify this process (29).
AGEs and hexosamine pathway
Patients with diabetes have higher levels of hexosamine in their retinal cells. Glucose in hexosamine pathway is transferred into fructose-6-phosphate through phosphorylation. Subsequently, fructose-6-phosphate can be catalyzed into glucosamine-6-phosphate (5). Glucosamine-6-phosphate can be acetylated and isomerized to generate N-acetylglucosamine-6-phosphate and then transferred into the end products—diphosphate uracil-N-acetylglucosamine (UDP-GlcNAc). The end products can be utilized as substrates to produce glycosyl side chains for modifying lipids or proteins, which is called O-GlcNAc Modification—one particular type of glycosylation occurred in multiple chronic diseases (30, 31).
All metabolic disorders in DR interact with each other through the corresponding mid-components, AGEs or ROS (32). An elevated ROS level suppresses the activity of glyceraldehydes-3-phosphate dehydrogenase (GAPDH), which triggers the increased glucose influx into polyol pathway and increases intracellular AGEs (33). Meanwhile, the repression of GAPDH also leads to the activation of PKC and NF-κB, and the initiation of hexosamine pathway (34). Fructose-6-phosphate amidotransferase (GFAT) in hexosamine pathway is correlated with diacylglycerol- (DAG-) stimulated PKC activity (35). Excess glucose is metabolized in polyol pathway to form sorbitol and subsequently converted into fructose, which prompt the formation of strong glycosylating precursors for the generation of AGEs (36). Correspondingly, excessive AGEs interrupt redox balance, leading to oxidative stress and activating PKC pathway (37).
AGEs and lipid peroxidation in DR
It has been discovered that tissues from DR contain a variety of metabolic components of lipid peroxidation (38), which are positively correlated to the duration and severity of diabetic complications (39). AGE-induced ROS generation brings about the breakdown of redox balance and prompts the rise of lipid peroxidation in DR. The abundance of polyunsaturated fatty acids (PUFAs) in retinal outer photoreceptor segment membranes makes the retina susceptible to ROS attack (40), and these PUFAs play an important structural and functional role in the retina. In the retina, arachidonic acid (AA), docosahexaenoic acid (DHA), and oleic acid (OA) are the main PUFAs, respectively, accounting for about 8, 50, and 10% in total fatty acids (41). Retinal lipid peroxidation not only jeopardizes photoreceptor membrane cells but also influences the normal physiological activity. The components in the process of lipid peroxidation, hydroxynonenal (HNE) and hydroxyhexenal (HHE), can chemically interact with cellular macromolecules (proteins or DNA), subsequently resulting in photoreceptor membrane damage and retinal pigment epithelial disorders (42). Additionally, ROS-induced mitochondrial dysfunction facilitates lipid accumulation in glia cells, which can be oxidized to render retinal neurodegeneration (43). As a result, one component in the production of AGEs is peroxidized lipids, and another method in the production of AGEs is by incubating lipid peroxidation products with proteins is (44).
AGEs and structural and functional changes in DR
Advanced glycation end products-associated metabolic disorders give rise to various pathophysiological changes in the structures and functions of retinal microvasculature. Major alterations in DR include the thickened retinal capillary basement membrane (CBM), the breakdown of the blood–retinal barrier (BRB), and the formation of acellular and occluded capillaries.
One typical characteristic of DR is the thickening of the CBM, which is due to the disruption of the balance between the expression and degeneration of extracellular matrix (ECM) proteins (45). Hyperglycemic state, as a key feature in diabetes, is regarded as a principal contributor to thicken CBM in DR, and AGEs and excessive ROS play dominant roles in this thickening process (46). The generation of AGEs on the collagen incurs the cross-linking among collagen proteins, leading to structural stiffness and limiting the transmembrane conveyance of multiple growth factors, and all these pathological alterations finally cause retinal endothelial cell and pericyte death (47). The treatment of aminoguanidine, one AGE inhibitor, plays a protective role to antagonize the thickening of retinal CBM in diabetic rats, which further proves the role of AGEs in this progression (48). The generation of AGEs promotes the accumulation of ROS to indirectly activate the corresponding transcription factors and cytokines that ultimately enhance the levels of ECM proteins in the retinal endothelial cells such as collagen and fibronectin (FN) (45). Fibrosis and thickened CBMs can be attributed to ROS-mediated upregulation of ECM genes (49).
The blood–retinal barrier controls the substance exchange between circulating blood vessels and neural retinal cells. It is regarded as one barrier of high selectivity that can provide essential nutrients and eliminate metabolic waste to maintain the normal function of neural retina. It has been documented that the dysfunction of BRB is closely associated with AGEs (50). AGEs can augment the adhesion of leukocytes that are deeply involved in the dysfunction of the BRB (51). Additionally, AGEs contribute to the breakdown of redox balance and prompt oxidative stress in retinal cells. Dysfunction of the BRB is partly attributed to excessive accumulation of ROS in retinal cells, which induces retinal pericyte loss—a histopathologic hallmark of DR (5).
The formation of acellular and occluded capillaries in DR can be ascribed to the elevated ability of angiogenesis. AGEs can enhance the transcription activities of activator protein-1 and NF-κB to upregulate the expression of angiogenesis-associated genes such as VEGF and angiopoietin-2 (50). In multiple pathological statuses, angiogenesis and inflammation are tightly cohesive processes. AGEs, as the booster for inflammation (52), indirectly prompt the formation of acellular and occluded capillaries. The interaction between AGEs and the RAGE promotes the production of ROS and the activation of NF-κB. The cross talk of ROS and NF-κB signaling is common in retinal cells, and they mutually modulate each other to augment inflammation (5).
AGEs and neurodegeneration in DR
Retinal neurons, glial cells, and vascular endothelium form a retinal neurovascular unit, a complicated entity of functional and physical coupling, and each component in this entity is closely synchronized to unite retinal blood flow and the metabolic system (53–55). Long-term hyperglycemia facilitates the formation of AGEs and ROS, disrupts the balance of the metabolic system, and then causes the production of inflammatory mediators and cellular damages, which constitute a vicious cycle. A vicious cycle in retinal neurons or vascellum contributes to the dysfunction of this neurovascular unit (56), which can be exemplified by the fact that excessive accumulation of AGEs has been discovered in retinal glial cells, the axons of retinal ganglion cells (RGCs), and the neurons near the retina inner surface (57, 58). The expression of RAGE is also abnormally upregulated in glial cells and RGCs, making these cells susceptible to AGE-involved processes, such as ROS formation and the activation of NF-κB and PKC pathways (57). AGE-induced activation of PKC functions as an upstream positive regulator of NOX, which brings about superabundant NOX-mediated ROS formation and subsequently causes ischemic loss of RGCs, to facilitate neurodegeneration (59). Additionally, early activation of the innate immune and complement systems and microglia also jeopardize a retinal neurovascular unit (60). AGEs can prompt the generation of proinflammatory cytokines and act as persistent antigenic stimulus to be immunostimulatory and impair the retinal neuron (60).
Therapeutic potential of phytochemicals in DR
Abnormalities associated with AGEs, such as inflammation and oxidative stress, can be used as potential therapeutic targets for DR. Antioxidant phytochemicals are well known for their anti-inflammatory, antioxidant, or anticarcinogenic properties, and their functions in DR treatment have also been investigated by many studies (5, 61, 62). Here, we described some antioxidant phytochemicals and their therapeutic potentials against AGE-mediated abnormality in DR.
Epigallocatechin-3-gallate (EGCG) is the primary polyphenol in green tea, with strong antioxidant capacity. EGCG blocks the formation of AGEs and exerts a curative effect on AGEs-induced collagen cross-linking (63). EGCG can also repress the activity of NF-κB, which plays an antagonistic role in vascular inflammation and apoptosis of retinal cells (64). In addition to the alleviation of oxidative stress, it can also protect the retinal nervous system, and ameliorate the injuries occurring in the BRB and the damage of electroretinograms and basement membrane thickening (64, 65).
Quercetin, a natural flavonoid, can prompt the upregulation of an ROS eraser, e.g., catalase and superoxide-dismutase (SOD), suppress AGE-induced NF-κB activity, and effectively guard against neurodegeneration and ROS-mediated impairments in the retina (66).
Resveratrol, a polyphenol of nonflavonoid phytochemical, is an excellent scavenger to eliminate ROS and exert protective effects against DR (67). It can block ROS-mediated cellular apoptosis in capillary endothelial cells of the retina (68), and also dose-dependently suppress AGE-associated factors, VEGF, TGF-β1, and PKC-β (69).
Curcumin, a polyphenol in Curcuma longa, possesses antioxidant, hypoglycemic, and anti-inflammatory capacity and also exhibits therapeutic effects in the treatment of DR (70). It can prevent structural alterations in the retina and block the thickness of CBM in DR (71).
Besides polyphenols, other antioxidants also exert effective effects in DR. Astaxanthin, as a xanthophyll carotenoid, possesses robust antioxidant capacity against oxidative stress. Notably, astaxanthin blocks the formation of endogenous N(ε)-CML, a representative member of AGEs, via suppressing ROS. Microalgae extracts containing astaxanthin ameliorate AGEs-induced impairments in retinal pigment epithelial (RPE) cells such as the abnormal expression of VEGF and matrix metalloproteinases (72). A relevant clinical study has revealed that lutein, one phytochemical of the carotenoid family, exerts safe and protective effects on visual function in patients of age-related macular degeneration (73). Lutein ameliorates ischemia-reperfusion injury and represses the apoptosis of retinal pigment epithelial and ganglion cells (74, 75).
Although multiple studies support the therapeutic functions of antioxidant phytochemicals against the progression of DR, the function of one single phytochemical compound is still limited. In patients with diabetes, the multiplex nutritional formula (including resveratrol, the extract of turmeric root and green tea, and other components) can ameliorate visual function and inhibit serum inflammatory factors, which confirms the therapeutic potential of these antioxidants (76). However, some studies show no alleviation of the severity in patients with retinopathy supplied with antioxidants (77). Thus, there is still no explicit conclusion for these antioxidants that has been drawn from the clinical trials. Clinical outcomes are impacted by multiple factors, e.g., the antioxidant dose, the duration of administration, and BRB-influenced transport. Despite these difficulties, it is still encouraging to push the research forward.
Conclusion
Diabetic retinopathy is a common diabetic complication in patients with diabetes, and the accumulation of AGEs is tightly associated with multiple disorders in the progression of DR, such as metabolic abnormalities, lipid peroxidation, structural and functional alterations, neurodegeneration, and so on. AGEs induce the disruption of the balance between the formation and elimination of ROS and cause oxidative stress in retinal cells, exerting cytopathic effects in this pathophysiology. Antioxidant phytochemicals, as AGE formation inhibitors, are a class of chemicals with reductive and biological activities and possess therapeutic potential in DR treatment, which provide a promising way to control this vision-damaging complication.
Author contributions
QK conceptualized the topic, researched and analyzed the literature, and wrote the manuscript. HD and SJ constructed the figures. LY revised the manuscript critically. All authors approved the final version of the manuscript, ensure the accuracy and integrity of the work, and agree to be accountable for all aspects of the work.
Funding
This review is supported by the Chinese National Major Project for New Drug Innovation (2019ZX09201002003), National Natural Science Foundation of China (82030076, 82070161, 81970151, 81670162, and 81870134), Shenzhen Science and Technology Foundation (JCYJ20190808163601776 and JCYJ20200109113810154), Shenzhen Key Laboratory Foundation (ZDSYS20200811143757022), and Sanming Project of Medicine in Shenzhen (SZSM202111004).
Acknowledgments
We thank LY for a critical reading of the review and helpful suggestions. We would like to acknowledge the support provided by Instrumental Analysis Center of Shenzhen University (Xili Campus).
Conflict of interest
The authors declare that the research was conducted in the absence of any commercial or financial relationships that could be construed as a potential conflict of interest.
Publisher's note
All claims expressed in this article are solely those of the authors and do not necessarily represent those of their affiliated organizations, or those of the publisher, the editors and the reviewers. Any product that may be evaluated in this article, or claim that may be made by its manufacturer, is not guaranteed or endorsed by the publisher.
Abbreviations
DR, diabetic retinopathy; ROS, reactive oxygen species; Nox, NADPH oxidase; AGEs, advanced glycation end products; DAG, diacylglycerol; VEGF, vascular endothelial growth factor; PKC, protein kinase C; GAPDH, glyceraldehydes-3-phosphate dehydrogenase; RAGEs, receptor for AGEs; GFAT, fructose-6-phosphate amidotransferase; UDP-GlcNAc, diphosphate uracil-N-acetylglucosamine; HHE, hydroxyhexenal; HNE, hydroxynonenal; PUFAs, polyunsaturated fatty acids; DHA, docosahexaenoic acid; AA, arachidonic acid; OA, oleic acid; RGCs, retinal ganglion cells; RPE, retinal pigment epithelium; CBM, capillary basement membrane; ECM, extracellular matrix; NADPH, nicotinamide adenine dinucleotide phosphate; BRB, blood–retinal barrier.
References
1. Ott C, Jacobs K, Haucke E, Navarrete Santos A, Grune T, Simm A, et al. Role of advanced glycation end products in cellular signaling. Redox Biol. (2014) 2:411–29. doi: 10.1016/j.redox.2013.12.016
2. Chaudhuri J, Bains Y, Guha S, Kahn A, Hall D, Bose N, et al. The role of advanced glycation end products in aging and metabolic diseases: bridging association and causality. Cell Metab. (2018) 28:337–52. doi: 10.1016/j.cmet.2018.08.014
3. Beli E, Yan Y, Moldovan L, Vieira CP, Gao R, Duan Y, et al. Restructuring of the gut microbiome by intermittent fasting prevents retinopathy and prolongs survival in db/db mice. Diabetes. (2018) 67:1867–79. doi: 10.2337/db18-0158
4. Wong TY, Cheung CM, Larsen M, Sharma S, Simo R. Diabetic retinopathy. Nat Rev Dis Primers. (2016) 2:16012. doi: 10.1038/nrdp.2016.12
5. Kang Q, Yang C. Oxidative stress and diabetic retinopathy: molecular mechanisms, pathogenetic role and therapeutic implications. Redox Biol. (2020) 37:101799. doi: 10.1016/j.redox.2020.101799
6. Stitt AW. Advanced glycation: an important pathological event in diabetic and age related ocular disease. Br J Ophthalmol. (2001) 85:746–53. doi: 10.1136/bjo.85.6.746
7. Kass DA, Shapiro EP, Kawaguchi M, Capriotti AR, Scuteri A, deGroof RC, et al. Improved arterial compliance by a novel advanced glycation end-product crosslink breaker. Circulation. (2001) 104:1464–70. doi: 10.1161/hc3801.097806
8. Bolt HM. High complexity of toxic reactions: parallels between products of oxidative stress and advanced glycation end products. Arch Toxicol. (2020) 94:1373–4. doi: 10.1007/s00204-020-02727-0
9. Abdallah HM, El-Bassossy H, Mohamed GA, El-Halawany AM, Alshali KZ, Banjar ZM, et al. Phenolics from garcinia mangostana inhibit advanced glycation endproducts formation: effect on amadori products, cross-linked structures and protein thiols. Molecules. (2016) 21:251. doi: 10.3390/molecules21020251
10. Zhou Q, Cheng KW, Xiao J, Wang M. The multifunctional roles of flavonoids against the formation of advanced glycation end products (AGEs) and AGEs-induced harmful effects. Trends Food Sci Technol. (2020) 103:333–47. doi: 10.1016/j.tifs.2020.06.002
11. Yamagishi S, Nakamura K, Matsui T, Inagaki Y, Takenaka K, Jinnouchi Y, et al. Pigment epithelium-derived factor inhibits advanced glycation end product-induced retinal vascular hyperpermeability by blocking reactive oxygen species-mediated vascular endothelial growth factor expression. J Biol Chem. (2006) 281:20213–20. doi: 10.1074/jbc.M602110200
12. Prasad K, Bhanumathy KK. AGE-RAGE Axis in the Pathophysiology of Chronic Lower Limb Ischemia and a Novel Strategy for Its Treatment. Int J Angiol. (2020) 29:156–67. doi: 10.1055/s-0040-1710045
13. Guimaraes EL, Empsen C, Geerts A, van Grunsven LA. Advanced glycation end products induce production of reactive oxygen species via the activation of NADPH oxidase in murine hepatic stellate cells. J Hepatol. (2010) 52:389–97. doi: 10.1016/j.jhep.2009.12.007
14. Zhang M, Kho AL, Anilkumar N, Chibber R, Pagano PJ, Shah AM, et al. Glycated proteins stimulate reactive oxygen species production in cardiac myocytes: involvement of Nox2 (gp91phox)-containing NADPH oxidase. Circulation. (2006) 113:1235–43. doi: 10.1161/CIRCULATIONAHA.105.581397
15. Cheung CYY, Lee CH, Tang CS, Xu A, Au KW, Fong CHY, et al. Genetic regulation of pigment epithelium-derived factor (PEDF): an exome-chip association analysis in chinese subjects with type 2 diabetes. Diabetes. (2019) 68:198–206. doi: 10.2337/db18-0500
16. Safi SZ, Qvist R, Kumar S, Batumalaie K, Ismail IS. Molecular mechanisms of diabetic retinopathy, general preventive strategies, and novel therapeutic targets. Biomed Res Int. (2014) 2014:801269. doi: 10.1155/2014/801269
17. Sheikpranbabu S, Haribalaganesh R, Gurunathan S. Pigment epithelium-derived factor inhibits advanced glycation end-products-induced cytotoxicity in retinal pericytes. Diabetes Metab. (2011) 37:505–11. doi: 10.1016/j.diabet.2011.03.006
18. Lin N, Zhang H, Su Q. Advanced glycation end-products induce injury to pancreatic beta cells through oxidative stress. Diabetes Metab. (2012) 38:250–7. doi: 10.1016/j.diabet.2012.01.003
19. Moldogazieva NT, Mokhosoev IM, Mel'nikova TI, Porozov YB, Terentiev AA. Oxidative stress and advanced lipoxidation and glycation end products (ALEs and AGEs) in aging and age-related diseases. Oxid Med Cell Longev. (2019) 2019:3085756. doi: 10.1155/2019/3085756
20. Yao D, Brownlee M. Hyperglycemia-induced reactive oxygen species increase expression of the receptor for advanced glycation end products (RAGE) and RAGE ligands. Diabetes. (2010) 59:249–55. doi: 10.2337/db09-0801
21. Dagher Z, Park YS, Asnaghi V, Hoehn T, Gerhardinger C, Lorenzi M, et al. Studies of rat and human retinas predict a role for the polyol pathway in human diabetic retinopathy. Diabetes. (2004) 53:2404–11. doi: 10.2337/diabetes.53.9.2404
22. Yang S, Zhao Y, Yu J, Fan Z, Gong ST, Tang H, et al. Sugar alcohols of polyol pathway serve as alarmins to mediate local-systemic innate immune communication in drosophila. Cell Host Microbe. (2019) 26:240–51.e8. doi: 10.1016/j.chom.2019.07.001
23. Yuan T, Yang T, Chen H, Fu D, Hu Y, Wang J, et al. New insights into oxidative stress and inflammation during diabetes mellitus-accelerated atherosclerosis. Redox Biol. (2019) 20:247–60. doi: 10.1016/j.redox.2018.09.025
24. Tarr JM, Kaul K, Chopra M, Kohner EM, Chibber R. Pathophysiology of diabetic retinopathy. ISRN Ophthalmol. (2013) 2013:343560. doi: 10.1155/2013/343560
25. Titchenell PM, Antonetti DA. Using the past to inform the future: anti-VEGF therapy as a road map to develop novel therapies for diabetic retinopathy. Diabetes. (2013) 62:1808–15. doi: 10.2337/db12-1744
26. Aiello LP, Clermont A, Arora V, Davis MD, Sheetz MJ, Bursell SE, et al. Inhibition of PKC beta by oral administration of ruboxistaurin is well tolerated and ameliorates diabetes-induced retinal hemodynamic abnormalities in patients. Invest Ophthalmol Vis Sci. (2006) 47:86–92. doi: 10.1167/iovs.05-0757
27. Birkenfeld AL, Shulman GI. Nonalcoholic fatty liver disease, hepatic insulin resistance, and type 2 diabetes. Hepatology. (2014) 59:713–23. doi: 10.1002/hep.26672
28. George A, Pushkaran S, Konstantinidis DG, Koochaki S, Malik P, Mohandas N, et al. Erythrocyte NADPH oxidase activity modulated by Rac GTPases, PKC, and plasma cytokines contributes to oxidative stress in sickle cell disease. Blood. (2013) 121:2099–107. doi: 10.1182/blood-2012-07-441188
29. Burr SD, Stewart JA. Rap1a overlaps the AGE/RAGE signaling cascade to alter expression of alpha-SMA, p-NF-kappaB, and p-PKC-zeta in cardiac fibroblasts isolated from type 2 diabetic. Mice Cells. (2021) 10. doi: 10.3390/cells10030557
30. Nagy T, Fisi V, Frank D, Katai E, Nagy Z, Miseta A, et al. Hyperglycemia-induced aberrant cell proliferation; A metabolic challenge mediated by protein O-GlcNAc modification. Cells. (2019) 8:999. doi: 10.3390/cells8090999
31. Hart GW, Housley MP, Slawson C. Cycling of O-linked beta-N-acetylglucosamine on nucleocytoplasmic proteins. Nature. (2007) 446:1017–22. doi: 10.1038/nature05815
32. Nishikawa T, Edelstein D, Du XL, Yamagishi S, Matsumura T, Kaneda Y, et al. Normalizing mitochondrial superoxide production blocks three pathways of hyperglycaemic damage. Nature. (2000) 404:787–90. doi: 10.1038/35008121
33. Beisswenger PJ, Howell SK, Smith K, Szwergold BS. Glyceraldehyde-3-phosphate dehydrogenase activity as an independent modifier of methylglyoxal levels in diabetes. Biochim Biophys Acta. (2003) 1637:98–106. doi: 10.1016/S09254439(02)00219-3
34. Brownlee M. The pathobiology of diabetic complications: a unifying mechanism. Diabetes. (2005) 54:1615–25. doi: 10.2337/diabetes.54.6.1615
35. Schleicher ED, Weigert C. Role of the hexosamine biosynthetic pathway in diabetic nephropathy. Kidney Int Suppl. (2000) 77:S13–8. doi: 10.1046/j.1523-1755.2000.07703.x
36. Lorenzi M. The polyol pathway as a mechanism for diabetic retinopathy: attractive, elusive, and resilient. Exp Diabetes Res. (2007) 2007:61038. doi: 10.1155/2007/61038
37. Scivittaro V, Ganz MB, Weiss MF. AGEs induce oxidative stress and activate protein kinase C-beta(II) in neonatal mesangial cells. Am J Physiol Renal Physiol. (2000) 278:F676–83. doi: 10.1152/ajprenal.2000.278.4.F676
38. Robles-Rivera RR, Castellanos-Gonzalez JA, Olvera-Montano C, Flores-Martin RA, Lopez-Contreras AK, Arevalo-Simental DE, et al. Adjuvant therapies in diabetic retinopathy as an early approach to delay its progression: the importance of oxidative stress and inflammation. Oxid Med Cell Longev. (2020) 2020:3096470. doi: 10.1155/2020/3096470
39. Gupta MM, Chari S. Lipid peroxidation and antioxidant status in patients with diabetic retinopathy. Indian J Physiol Pharmacol. (2005) 49:187–92.
40. Catala A. Lipid peroxidation of membrane phospholipids in the vertebrate retina. Front Biosci (Schol Ed). (2011) 3:52–60. doi: 10.2741/s131
41. Liu Y, Zhang D, Hu J, Liu G, Chen J, Sun L, et al. Lipid peroxidation of unsaturated fatty acids in the retina and the inhibitory effects of blueberry polyphenols. J Agric Food Chem. (2015) 63:9295–305. doi: 10.1021/acs.jafc.5b04341
42. Liu Y, Zhang D, Wu Y, Ji B. Docosahexaenoic acid aggravates photooxidative damage in retinal pigment epithelial cells via lipid peroxidation. J Photochem Photobiol B. (2014) 140:85–93. doi: 10.1016/j.jphotobiol.2014.07.016
43. Liu L, Zhang K, Sandoval H, Yamamoto S, Jaiswal M, Sanz E, et al. Glial lipid droplets and ROS induced by mitochondrial defects promote neurodegeneration. Cell. (2015) 160:177–90. doi: 10.1016/j.cell.2014.12.019
44. Kuzan A. Toxicity of advanced glycation end products (Review). Biomed Rep. (2021) 14:46. doi: 10.3892/br.2021.1422
45. Khan ZA, Chakrabarti S. Cellular signaling and potential new treatment targets in diabetic retinopathy. Exp Diabetes Res. (2007) 2007:31867. doi: 10.1155/2007/31867
46. Roy S, Kim D. Retinal capillary basement membrane thickening: Role in the pathogenesis of diabetic retinopathy. Prog Retin Eye Res. (2021) 82:100903. doi: 10.1016/j.preteyeres.2020.100903
47. Goh SY, Cooper ME. Clinical review: The role of advanced glycation end products in progression and complications of diabetes. J Clin Endocrinol Metab. (2008) 93:1143–52. doi: 10.1210/jc.2007-1817
48. Gardiner TA, Anderson HR, Stitt AW. Inhibition of advanced glycation end-products protects against retinal capillary basement membrane expansion during long-term diabetes. J Pathol. (2003) 201:328–33. doi: 10.1002/path.1429
49. Mason RM, Wahab NA. Extracellular matrix metabolism in diabetic nephropathy. J Am Soc Nephrol. (2003) 14:1358–73. doi: 10.1097/01.ASN.0000065640.77499.D7
50. Stitt AW, Bhaduri T, McMullen CB, Gardiner TA, Archer DB. Advanced glycation end products induce blood-retinal barrier dysfunction in normoglycemic rats. Mol Cell Biol Res Commun. (2000) 3:380–8. doi: 10.1006/mcbr.2000.0243
51. Moore TC, Moore JE, Kaji Y, Frizzell N, Usui T, Poulaki V, et al. The role of advanced glycation end products in retinal microvascular leukostasis. Invest Ophthalmol Vis Sci. (2003) 44:4457–64. doi: 10.1167/iovs.02-1063
52. Garay-Sevilla ME, Rojas A, Portero-Otin M, Uribarri J. Dietary AGEs as exogenous boosters of inflammation. Nutrients. (2021) 13. doi: 10.3390/nu13082802
53. Metea MR, Newman EA. Signalling within the neurovascular unit in the mammalian retina. Exp Physiol. (2007) 92:635–40. doi: 10.1113/expphysiol.2006.036376
54. Hamel E. Perivascular nerves and the regulation of cerebrovascular tone. J Appl Physiol. (1985) 100:1059–64. doi: 10.1152/japplphysiol.00954.2005
55. Iadecola C. Neurovascular regulation in the normal brain and in Alzheimer's disease. Nat Rev Neurosci. (2004) 5:347–60. doi: 10.1038/nrn1387
56. Wu MY, Yiang GT, Lai TT, Li CJ. The oxidative stress and mitochondrial dysfunction during the pathogenesis of diabetic retinopathy. Oxid Med Cell Longev. (2018) 2018:3420187. doi: 10.1155/2018/3420187
57. Tezel G, Luo C, Yang X. Accelerated aging in glaucoma: immunohistochemical assessment of advanced glycation end products in the human retina and optic nerve head. Invest Ophthalmol Vis Sci. (2007) 48:1201–11. doi: 10.1167/iovs.06-0737
58. Takeuchi M, Bucala R, Suzuki T, Ohkubo T, Yamazaki M, Koike T, et al. Neurotoxicity of advanced glycation end-products for cultured cortical neurons. J Neuropathol Exp Neurol. (2000) 59:1094–105. doi: 10.1093/jnen/59.12.1094
59. Dvoriantchikova G, Grant J, Santos AR, Hernandez E, Ivanov D. Neuronal NAD(P)H oxidases contribute to ROS production and mediate RGC death after ischemia. Invest Ophthalmol Vis Sci. (2012) 53:2823–30. doi: 10.1167/iovs.12-9526
60. Feng Y, Busch S, Gretz N, Hoffmann S, Hammes HP. Crosstalk in the retinal neurovascular unit - lessons for the diabetic retina. Exp Clin Endocrinol Diabetes. (2012) 120:199–201. doi: 10.1055/s-0032-1304571
61. Yu J, Jiang Q, Liu N, Fan D, Wang M, Zhao Y, et al. Apigenin and apigenin-7, 4.'-O-dioctanoate protect against acrolein-aggravated inflammation via inhibiting the activation of NLRP3 inflammasome and HMGB1/MYD88/NF-kappaB signaling pathway in Human umbilical vein endothelial cells (HUVEC). Food Chem Toxicol. (2022) 168:113400. doi: 10.1016/j.fct.2022.113400
62. Zhang X, Ni L, Zhu Y, Liu N, Fan D, Wang M, et al. Quercetin inhibited the formation of lipid oxidation products in thermally treated soybean oil by trapping intermediates. J Agric Food Chem. (2021) 69:3479–88. doi: 10.1021/acs.jafc.1c00046
63. Babu PV, Sabitha KE, Shyamaladevi CS. Therapeutic effect of green tea extract on advanced glycation and cross-linking of collagen in the aorta of streptozotocin diabetic rats. Clin Exp Pharmacol Physiol. (2006) 33:351–7. doi: 10.1111/j.1440-1681.2006.04374.x
64. Meng JM, Cao SY, Wei XL, Gan RY, Wang YF, Cai SX, et al. Effects and mechanisms of tea for the prevention and management of diabetes mellitus and diabetic complications: an updated review. Antioxidants (Basel). (2019) 8:190. doi: 10.3390/antiox8060170
65. Silva KC, Rosales MA, Hamassaki DE, Saito KC, Faria AM, Ribeiro PA, et al. Green tea is neuroprotective in diabetic retinopathy. Invest Ophthalmol Vis Sci. (2013) 54:1325–36. doi: 10.1167/iovs.12-10647
66. Kumar B, Gupta SK, Nag TC, Srivastava S, Saxena R, Jha KA, et al. Retinal neuroprotective effects of quercetin in streptozotocin-induced diabetic rats. Exp Eye Res. (2014) 125:193–202. doi: 10.1016/j.exer.2014.06.009
67. Abu-Amero KK, Kondkar AA, Chalam KV. Resveratrol and Ophthalmic Diseases. Nutrients. (2016) 8:200. doi: 10.3390/nu8040200
68. Li J, Yu S, Ying J, Shi T, Wang P. Resveratrol Prevents ROS-Induced Apoptosis in High Glucose-Treated Retinal Capillary Endothelial Cells via the Activation of AMPK/Sirt1/PGC-1alpha Pathway. Oxid Med Cell Longev. (2017) 2017:7584691. doi: 10.1155/2017/7584691
69. Losso JN, Truax RE, Richard G. Trans-resveratrol inhibits hyperglycemia-induced inflammation and connexin downregulation in retinal pigment epithelial cells. J Agric Food Chem. (2010) 58:8246–52. doi: 10.1021/jf1012067
70. Aldebasi YH, Aly SM, Rahmani AH. Therapeutic implications of curcumin in the prevention of diabetic retinopathy via modulation of anti-oxidant activity and genetic pathways. Int J Physiol Pathophysiol Pharmacol. (2013) 5:194–202.
71. Gupta SK, Kumar B, Nag TC, Agrawal SS, Agrawal R, Agrawal P, et al. Curcumin prevents experimental diabetic retinopathy in rats through its hypoglycemic, antioxidant, and anti-inflammatory mechanisms. J Ocul Pharmacol Ther. (2011) 27:123–30. doi: 10.1089/jop.2010.0123
72. Sun Z, Liu J, Zeng X, Huangfu J, Jiang Y, Wang M, et al. Protective actions of microalgae against endogenous and exogenous advanced glycation endproducts (AGEs) in human retinal pigment epithelial cells. Food Funct. (2011) 2:251–8. doi: 10.1039/c1fo10021a
73. Ramkumar HL, Tuo J, Shen DF, Zhang J, Cao X, Chew EY, et al. Nutrient supplementation with n3 polyunsaturated fatty acids, lutein, and zeaxanthin decrease A2E accumulation and VEGF expression in the retinas of Ccl2/Cx3cr1-deficient mice on Crb1rd8 background. J Nutr. (2013) 143:1129–35. doi: 10.3945/jn.112.169649
74. Gong X, Draper CS, Allison GS, Marisiddaiah R, Rubin LP. Effects of the Macular Carotenoid Lutein in Human Retinal Pigment Epithelial Cells. Antioxidants (Basel). (2017) 6. doi: 10.3390/antiox6040100
75. Li SY, Lo AC. Lutein protects RGC-5 cells against hypoxia and oxidative stress. Int J Mol Sci. (2010) 11:2109–17. doi: 10.3390/ijms11052109
76. Chous AP, Richer SP, Gerson JD, Kowluru RA. The diabetes visual function supplement study (DiVFuSS). Br J Ophthalmol. (2016) 100:227–34. doi: 10.1136/bjophthalmol-2014-306534
Keywords: AGEs, diabetic retinopathy, RAGE, pathogenetic roles, antioxidant phytochemicals
Citation: Kang Q, Dai H, Jiang S and Yu L (2022) Advanced glycation end products in diabetic retinopathy and phytochemical therapy. Front. Nutr. 9:1037186. doi: 10.3389/fnut.2022.1037186
Received: 05 September 2022; Accepted: 31 October 2022;
Published: 18 November 2022.
Edited by:
Qian Wu, Hubei University of Technology, ChinaReviewed by:
Leilei Xu, National Institutes of Health (NIH), United StatesBin Zhou, Hubei University of Technology, China
Shuo Zhang, Karolinska Institutet (KI), Sweden
Copyright © 2022 Kang, Dai, Jiang and Yu. This is an open-access article distributed under the terms of the Creative Commons Attribution License (CC BY). The use, distribution or reproduction in other forums is permitted, provided the original author(s) and the copyright owner(s) are credited and that the original publication in this journal is cited, in accordance with accepted academic practice. No use, distribution or reproduction is permitted which does not comply with these terms.
*Correspondence: Li Yu, eXVsaUBzenUuZWR1LmNu