- 1Spice and Beverage Research Institute, Chinese Academy of Tropical Agricultural Sciences, Wanning, China
- 2College of Food Science and Engineering, Hainan University, Haikou, China
- 3College of Food Science and Technology, Huazhong Agricultural University, Wuhan, China
- 4Key Laboratory of Processing Suitability and Quality Control of the Special Tropical Crops of Hainan Province, Wanning, China
- 5Department of Pharmacology, School of Basic Medicine and Life Science, Hainan Medical University, Haikou, China
Polysaccharides show protective effects on intestinal barrier function due to their effectiveness in mitigating oxidative damage, inflammation and probiotic effects. Little has been known about the effects of polysaccharides from Artocarpus heterophyllus Lam. pulp (jackfruit, JFP-Ps) on intestinal barrier function. This study aimed to investigate the effects of JFP-Ps on intestinal barrier function in high fat diet-induced obese rats. H&E staining and biochemical analysis were performed to measure the pathological and inflammatory state of the intestine as well as oxidative damage. Expression of the genes and proteins associated with intestinal health and inflammation were analyzed by RT-qPCR and western blots. Results showed that JFP-Ps promoted bowel movements and modified intestinal physiochemical environment by lowering fecal pH and increasing fecal water content. JFP-Ps also alleviated oxidative damage of the colon, relieved intestinal colonic inflammation, and regulated blood glucose transport in the small intestine. In addition, JFP-Ps modified intestinal physiological status through repairing intestinal mucosal damage and increasing the thickness of the mucus layer. Furthermore, JFP-Ps downregulated the inflammatory genes (TNF-α, IL-6) and up-regulated the free fatty acid receptors (GPR41 and GPR43) and tight junction protein (occludin). These results revealed that JFP-Ps showed a protective effect on intestinal function through enhancing the biological, mucosal, immune and mechanical barrier functions of the intestine, and activating SCFAs-GPR41/GPR43 related signaling pathways. JFP-Ps may be used as a promising phytochemical to improve human intestinal health.
Introduction
The intestine is the largest digestive and immune organ which closely linked to the body health, participates in the digestion and absorption of nutrients. The intestine also provides a favorable anaerobic environment for microbial colonization, plays an important role in defending the host from pathogens, and regulating the host’s immune system (1–4). The contents of short-chain fatty acids (SCFAs) and colonic water, and colonic pH value are basic indicators for intestinal health, which is closely linked to the body health (5). Changes in dietary composition can affect the development of gastrointestinal disease and the integrity of the gut by altering the growth and metabolism of the intestinal flora (6). Wang et al. (7) found that walnut green husk polysaccharides alleviated obesity, chronic inflammatory responses, non-alcoholic fatty liver disease and colonic tissue damage via regulating gut microbiota and SCFAs content.
Polysaccharides have been reported to improve gut health via enhancing intestinal barrier function and restoring intestinal homeostasis, and regulate intestinal function via mitigating oxidative damage and inflammation, and probiotic function (8, 9). Polysaccharides from Cyclocarya paliurus leaves, Dendrobium officinale, fruiting body of Hericium erinaceus and polysaccharide-rich sage weed extracts were found to maintain intestinal health through lowering fecal pH value, increasing fecal water content and SCFAs concentration (5, 6, 10, 11). Gao et al. (12) reported that polysaccharide fractions from okra improved intestinal function via increasing the contents of SCFAs and caecum moisture, thickness of mucosa and muscular layer. Dendrobium huoshanense polysaccharide improved intestinal mucosal barrier function by modifying intestinal mucosal structures, regulating the production of cytokines and promoting the expression of the tight junction proteins (8).
Polysaccharides from Artocarpus heterophyllus Lam (jackfruit) pulp (JFP-Ps) has been demonstrated to possess immunomodulatory effects (13). In the past few years, our team has investigated the isolation, purification, in vitro digestive properties, antioxidant activity and in vivo prebiotic effects of JFP-Ps (14–16). However, to our knowledge, little has been known about the protective effects of JFP-Ps on intestinal health. Therefore, the present study aimed to investigate the protective effects of JFP-Ps on intestinal function of obese rats induced by a high-fat diet.
Materials and methods
Materials and reagents
Jackfruit fruits were collected from Xinglong Tropical Botanical Garden (Wanning, Hainan, China). JFP-Ps was extracted from the Artocarpus heterophyllus Lam pulp using hot water extraction and ethanol precipitation as previously described by Zhu et al. (14). JFP-Ps was mainly composed of Rha, Ara, Gal, Glc, Xyl, and GalA, with an average molecular weight of approximately 1,668 kDa.
The normal-chow diet (D12450H, 10% calories from fat) and high-fat diet (D12451, 45% calories from fat) were obtained from Jiangsu Synergy Pharmaceutical and Biological Engineering Co., Ltd., (Jiangsu, China). Assay kits for the activity of superoxide dismutase (SOD), glutathione peroxidase (GSH-Px) and catalase (CAT) and the content of malondialdehyde (MDA) were obtained from Suzhou Grace Biotechnology Co., Ltd., (Jiangsu, China). ELISA kits for myeloperoxidase (MPO), tumor necrosis factor-α (TNF-α), interleukin-1β (IL-1β), interleukin-6 (IL-6), interleukin-10 (IL-10) and sodium-glucose cotransporter 1 (SGLT1) were purchased from Shanghai Enzyme-linked Biotechnology Co., Ltd., (Shanghai, China). The qPCR primers for TNF-α, IL-6, G protein-coupled receptor 43 (GPR43), G protein-coupled receptor 41 (GPR41) and β-actin were purchased from Sangon Biotech (Shanghai) Co., Ltd., (Shanghai, China). TriQuick reagent and bicinchoninic acid (BCA) assay kits were purchased from Beijing Solarbio Science & Technology Co., Ltd., (Beijing, China). SuperReal Premix Plus assay kits were purchased from Tiangen Biotech (Beijing) Co., Ltd., (Beijing, China). Rabbit-derived polyclonal antibodies against occludin (27260-1-AP) and secondary antibody (SA00001-2) were purchased from Proteintech Group, Inc., (Wuhan Sanying, Hubei, China). BeyoRT™ III first-strand synthesized kit, BeyoECL Plus reagent, poly (vinylidene fluoride) (PVDF) membrane, rabbit-derived monoclonal antibodies against β-actin (AF5003) and paraformaldehyde were purchased from Beyotime Biotechnology (Shanghai, China).
Animal experiments
Sprague-Dawley rats (SPF-grade, male), with body weights (BW) ranging from 180 to 200 g, were purchased from Hunan Silaikejingda Experimental Animal Co., Ltd., (Changsha, China) with the experimental animal production license SCXK (Xiang) 2019-0004. All rats were housed in an animal facility under controlled interior temperature (23 ± 2°C), relative humidity (55 ± 15%), noise (≤60 dB) and lighting cycle (12:12 h light-dark). After 1-week acclimation, the rats were divided into two groups: normal control (NC) group (n = 8) fed with normal-chow diet, obesity group (n = 45) fed with high fat diet (HFD). After 12 weeks, the average body weight (BW) of the obesity group was significantly higher than that in the normal group (p < 0.01). The animals in obesity group were further randomly divided into five groups: HFD group, inulin group, low-, medium- and high- dose JFP-Ps groups (JFP-Ps-L, JFP-Ps-M, JFP-Ps-H), and continually fed with the high fat diet. During the diet intervention, the NC group and HFD group were treated daily with an equivalent volume of distilled water by oral gavage. The inulin group was treated daily with inulin (1.5 g/kg BW) by oral gavage (17, 18). The JFP-Ps-L group, JFP-Ps-M group and JFP-Ps-H group were treated daily with 50, 100, and 200 mg/kg JFP-Ps by oral gavage, respectively. The diet intervention was lasted for 6 weeks. The recipes of the diets are listed in S1 and S2. All animal experimental procedures were approved by the Animal Ethics Committee of Hainan University and Hainan Medical University with experimental animal use permit SYXK (Qiong) 2017-0013.
Sample collection
All the rats were fasted for 12 h and anesthetized with chloral hydrate by intraperitoneal injection, and then dissected. Fecal samples were collected, immersed immediately in liquid nitrogen, and stored at −80°C for water content and pH value analysis. The length of the colon was measured and the intestinal tissue was rinsed with pre-cooled saline, blotted on filter paper, and divided into three portions for further analysis.
Water content and pH value of feces
A portion of fecal sample was heated in an air-oven at 105 ± 2°C to a constant weight. The water content of the feces was calculated from the mass of the feces before and after drying. The other part of fecal sample was diluted with distilled water at a ratio of 1:10 (w/v) and the pH value was determined using a SevenCompact™ S220 pH meter (Mettler Toledo, Switzerland).
Antioxidant activities analysis
One hundred milligram colon tissue were mixed with pre-cooled saline (4°C, 0.9%, w/w) at a ratio of 1:10 (w/v), homogenized over ice for 3 min, and centrifuged (12,000 × g, 15 min, 4°C) to gather supernatants. The activities of SOD, GSH-Px and CAT and the content of MDA were determined using the biochemical kits following the manufacturer’s protocols.
Enzyme-linked immunoassay
One hundred milligram of colon tissue were homogenized with 1.0 mL pre-cooled saline (4°C, 0.9%, w/w) over ice for 5 min. Then tissue homogenate was centrifuged (12,000 × g, 20 min, 4°C) to collect supernatants. The activity of MPO and the concentrations of TNF-α, IL-1β, IL-6, and IL-10 in the colon were measured by ELISA assay kits following the manufacturer’s instructions. The activity of SGLT1 in the small intestine was analyzed following the manufacturer’s instructions.
Histological examination
The small intestine tissue was fixed in 4% paraformaldehyde overnight, dehydrated with graded alcohol, imbedded in a paraffin wax, and whittled down to 4 μm thickness. Then the sections were mounted onto clean glass slides, soaked in graded xylene and alcohol, stained with hematoxylin and eosin. Lastly, slides were sealed with neutral balsam for inspection under a binocular microscope.
RT-qPCR analysis
The mRNA levels of TNF-α, IL-6, GPR43, and GPR41 were determined by RT-qPCR. Total RNA was extracted from the small intestine tissue with the TriQuick reagent according to the manufacturer’s protocol. The purity and concentration of total RNA was determined by Thermo Scientific™ NanoDrop™ 2000C spectrophotometer. cDNA was generated by reverse transcription of RNA using BeyoRT™ III first-strand synthesized kit. RT-qPCR analysis of the target genes was performed on the Bio-Rad® CFX96 Real Time PCR System using a SuperReal PreMix Color (SYBR Green). The relative expression levels of the genes were calculated according to the 2–ΔΔ Ct method and normalized to the housekeeping gene, β-actin. Primer information is listed in Table 1.
Western blot analysis
Fifty milligram of small intestine tissue were homogenized with 0.5 mL pre-cooled RIPA buffer, 5 μL PMSF lysis buffer and 5 μL protease inhibitor cocktail over ice for 5 min, and centrifuged (12,000 × g, 10 min, 4°C) to collect supernatants. The protein concentrations were quantified by BCA Protein Assay Kit. Denatured protein samples were fractionated on a 10% SDS-PAGE and transferred onto 0.45 μm PVDF membranes. After the membranes were blocked with 5% skimmed milk at room temperature for 60 min, the membranes were incubated overnight with primary antibodies (1:1,300) at 4°C, and then HRP-conjugated secondary antibody (1:1,000) following the manufacturer’s instructions. Protein bands were developed using an ultrasensitive ECL chemiluminescence kit and visualized using a Tanon 5200 Multi chemiluminescent imaging system, and lastly quantified using Image J software.
Statistical analysis
Results are expressed as means ± standard error of the mean (SEM). Data were analyzed by one-way analysis of variance (ANOVA), followed by Duncan’s multiple comparison tests with SPSS Statistics 26 software (IBM, USA). p < 0.05 indicated a statistically significant difference.
Results
Effects of JFP-Ps on water content and pH value of feces
The water content of feces was significantly lower in the HFD group (56.75 ± 2.64%) than that in the NC group (64.68 ± 1.28%) (Figure 1A). JFP-Ps and inulin treatments increased water content as compared with HFD treatment. Moreover, the water content in the JFP-Ps-H group (67.05 ± 1.34%) was close to that in the inulin group (67.47 ± 2.02%), which was slightly higher than that in the normal group. As shown in Figure 1B, the pH value of feces in the HFD group was significantly higher than the NC group (p < 0.05). After feeding with JFP-Ps and inulin, the pH values of feces were significantly decreased in obese rats compared with the HFD treatment. The results indicated that JFP-Ps significantly increased fecal water content and decreased fecal pH value in obese rats.
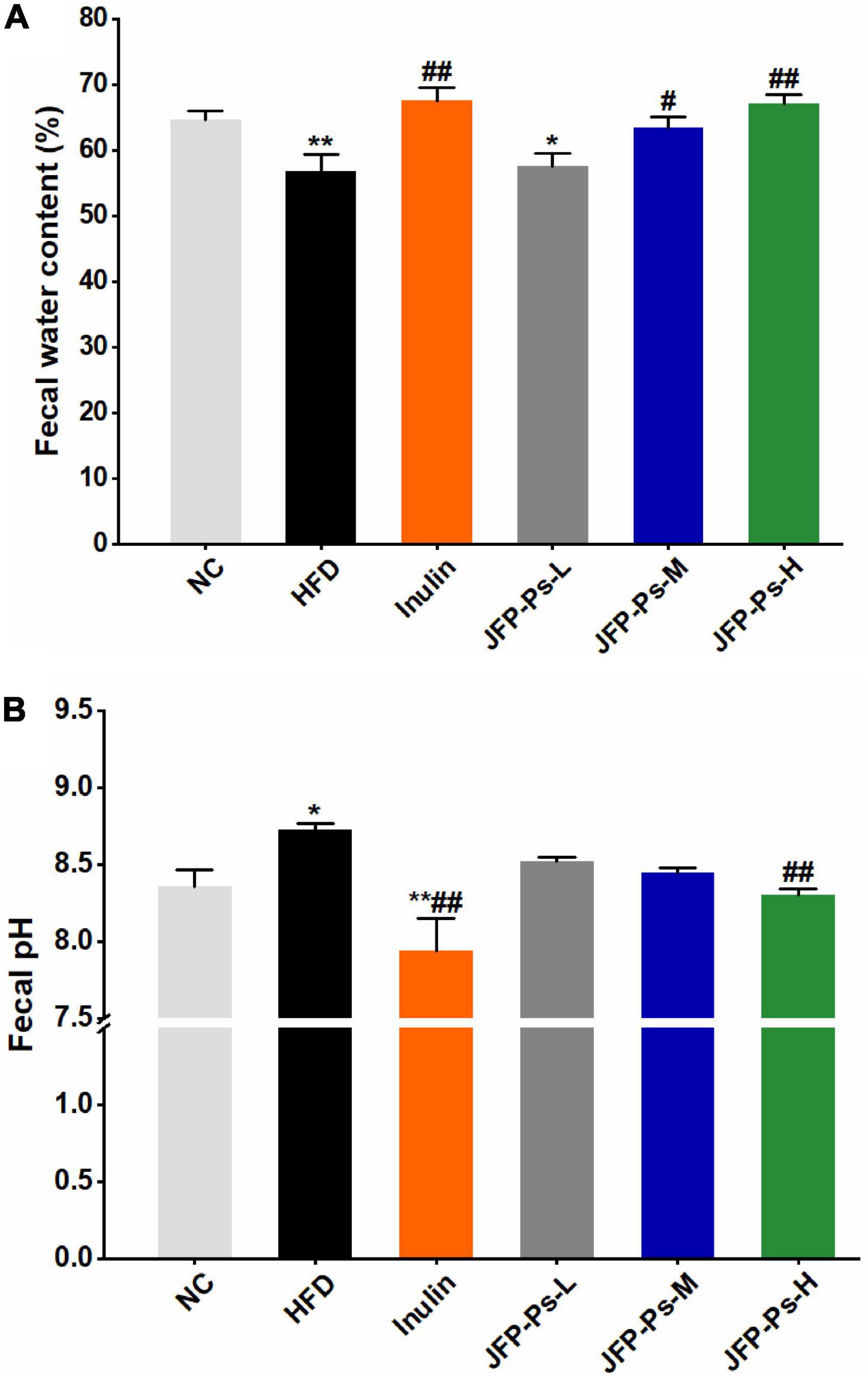
Figure 1. Effect of JFP-Ps on fecal water content (A) and fecal pH value (B) in obese rats. Data are expressed as mean ± SEM (n = 6 for each group) and analyzed using one-way ANOVA. *p < 0.05, **p < 0.01 compared to the NC group; #p < 0.05, ##p < 0.01 compared with the HFD group.
Effects of JFP-Ps on colon length and intestinal micromorphology
As shown in Figures 2A,B, the colon of the HFD group was significantly shorter than the NC group (p < 0.05). JFP-Ps and inulin significantly inhibited the decrease of colon length compared with the HFD group (p < 0.01).
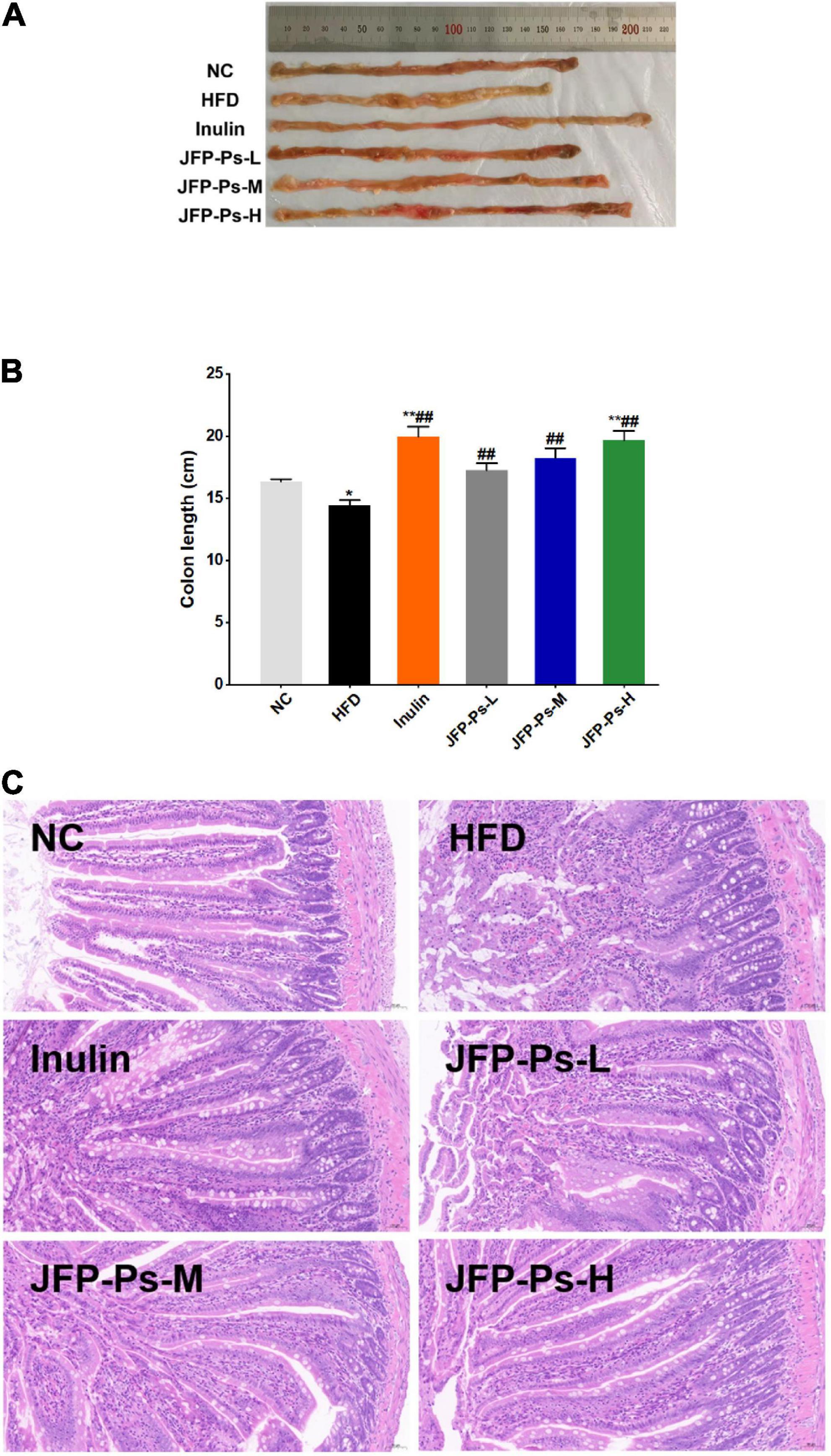
Figure 2. Effect of JFP-Ps on colon length and intestinal micromorphology in obese rats. (A) Representatives of colonic tissue in each group, (B) colon length, (C) jejunal micromorphology (original magnification 20X). Data are expressed as mean ± SEM (n = 6 for each group) and analyzed using one-way ANOVA. *p < 0.05, **p < 0.01 compared to the NC group; ##p < 0.01 compared with the HFD group.
Morphological changes in jejunal tissue between the groups were visualized by H&E staining. As shown in Figure 2C, the jejunum in the NC group showed clear tissue structure, with finger-shaped villi closely and evenly arranged, a large number of cup-shaped cells within the columnar epithelium, and a clear demarcation between the submucosa and the muscular layer. In the HFD group, the mucosal layer of jejunum was disorganized; the shape of the villi was not clear; and the tips of the villi were mixed and accompanied by severe inflammatory infiltration, with cup-shaped cells visible only at the base of the villi. Comparison to the HFD group, the jejunum of the JFP-Ps-L group and JFP-Ps-M group showed clearer structure, with the villi gradually becoming clearer in shape and more uniformly arranged, with less mixing of the villi apices and less inflammatory infiltration, and a gradual increase in the distribution of cupped cells within the columnar epithelium. The morphology and structure of the jejunal tissue in the JFP-Ps-H group was similar to that in the NC group, but the length and number of villi increased. Moreover, villi were more closely arranged; the volume of the intestinal lumen decreased; more cup-shaped cells were distributed within the columnar epithelium and the thickness of the mucosal layer increased. The results showed that JFP-Ps restored intestinal mucosal damage induced by a high-fat diet in obese rats, increased the thickness of the mucus layer and protected the barrier function of the intestinal mucosa, which in turn had a positive effect on intestinal health.
Effects of JFP-Ps on antioxidant activities in colon
As shown in Table 2, in comparison to the NC group, the activities of SOD, GSH-Px, and CAT in the HFD group were decreased and the content of MDA in the HFD group was increased (p < 0.01). Compared with HFD group, JFP-Ps treatment increased the activities of SOD, GSH-Px and CAT, and the activities of GSH-Px increased significantly (p < 0.01). Moreover, JFP-Ps decreased the content of MDA significantly (p < 0.01). The results indicated that JFP-Ps may alleviate oxidative damage in the colon of obese rats and improve integrity of the intestinal epithelium by enhancing the activities of antioxidant enzymes in the colon.
Effects of JFP-Ps on inflammation-related indicators in colon
As shown in Table 3, the MPO activity in the HFD group was significantly increased (p < 0.05), and the contents of pro-inflammatory cytokines (TNF-α, IL-1β, and IL-6) increased, and the content of anti-inflammatory cytokine (IL-10) decreased, compared to that in the NC group. However, compared with HFD group, the MPO activity in the JFP-Ps group was significantly decreased (p < 0.01), the contents of pro-inflammatory cytokines were decreased in a dose-dependent manner, and the level of the anti-inflammatory cytokine was increased. The results indicated that JFP-Ps may decrease the inflammation in the colon of obese rats induced by high-fat diet.
Effects of JFP-Ps on glucose transport in the small intestine
As shown in Figure 3, SGLT1 activity in the small intestine of rats from the HFD group was decreased compared with the NC group. The JFP-Ps intervention reduced SGLT1 activity in HFD group. The results showed that JFP-Ps may inhibit glucose transport in the small intestine by reducing SGLT1 activity in the intestinal epithelium of obese rats.
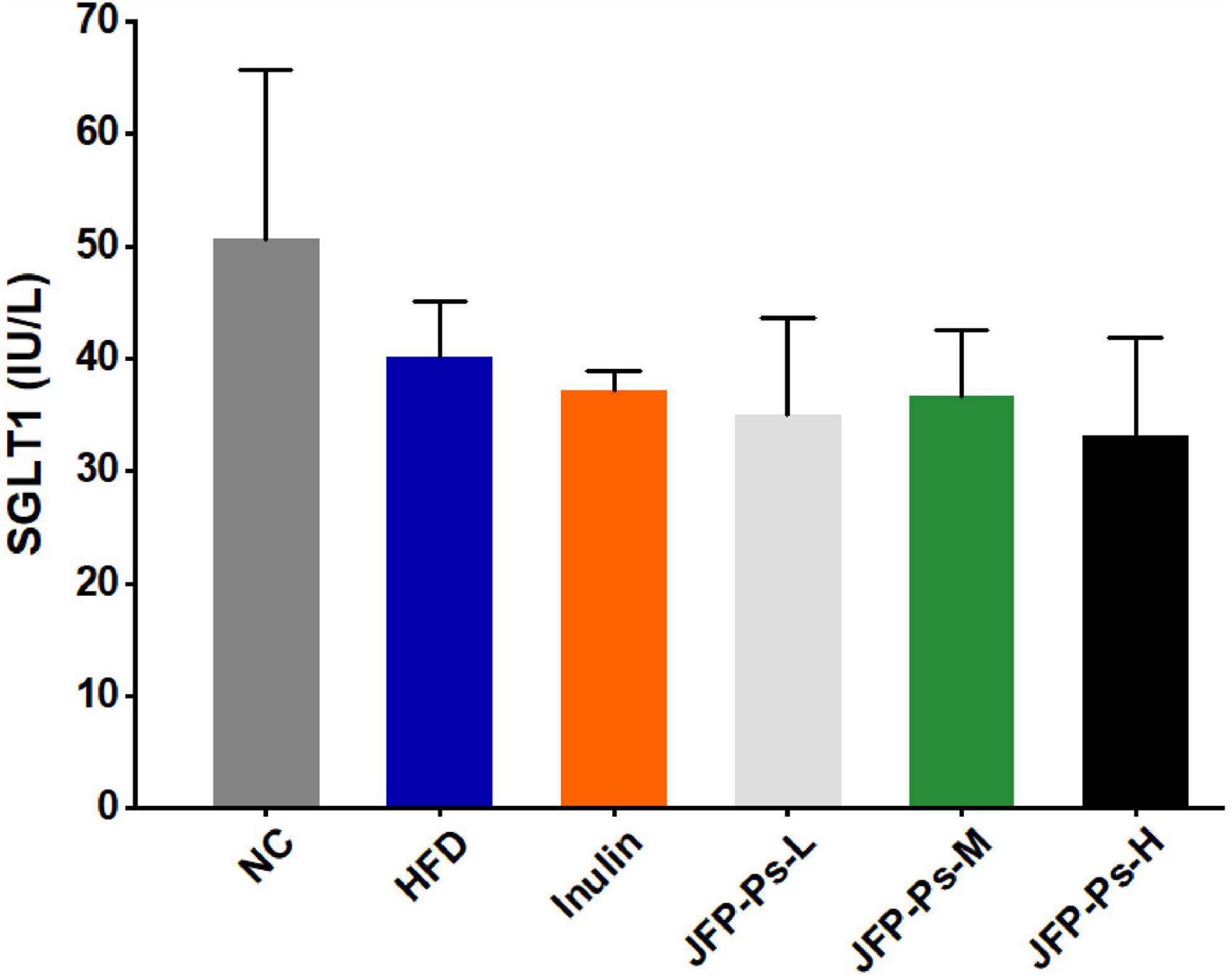
Figure 3. Effect of JFP-Ps on SGLT1 activity in small intestinal tissues. Data are expressed as mean ± SEM (n = 6 for each group) and analyzed using one-way ANOVA. No significant difference was observed.
Effects of JFP-Ps on the expression of gut barrier function-related genes in small intestine
The levels of TNF-α and IL-6 mRNA expression were significantly increased in the small intestine of the HFD group, and the gene expression levels of GPR41 and GPR43 were slightly down-regulated (Figures 4A–D). Interestingly, treatment with JFP-Ps decreased the expression of TNF-α and IL-6, while increased the gene expression of GPR43 and GPR41 in a dose-dependent manner. These results showed that JFP-Ps may inhibit inflammation and enhance immune function in the small intestine of obese rats.
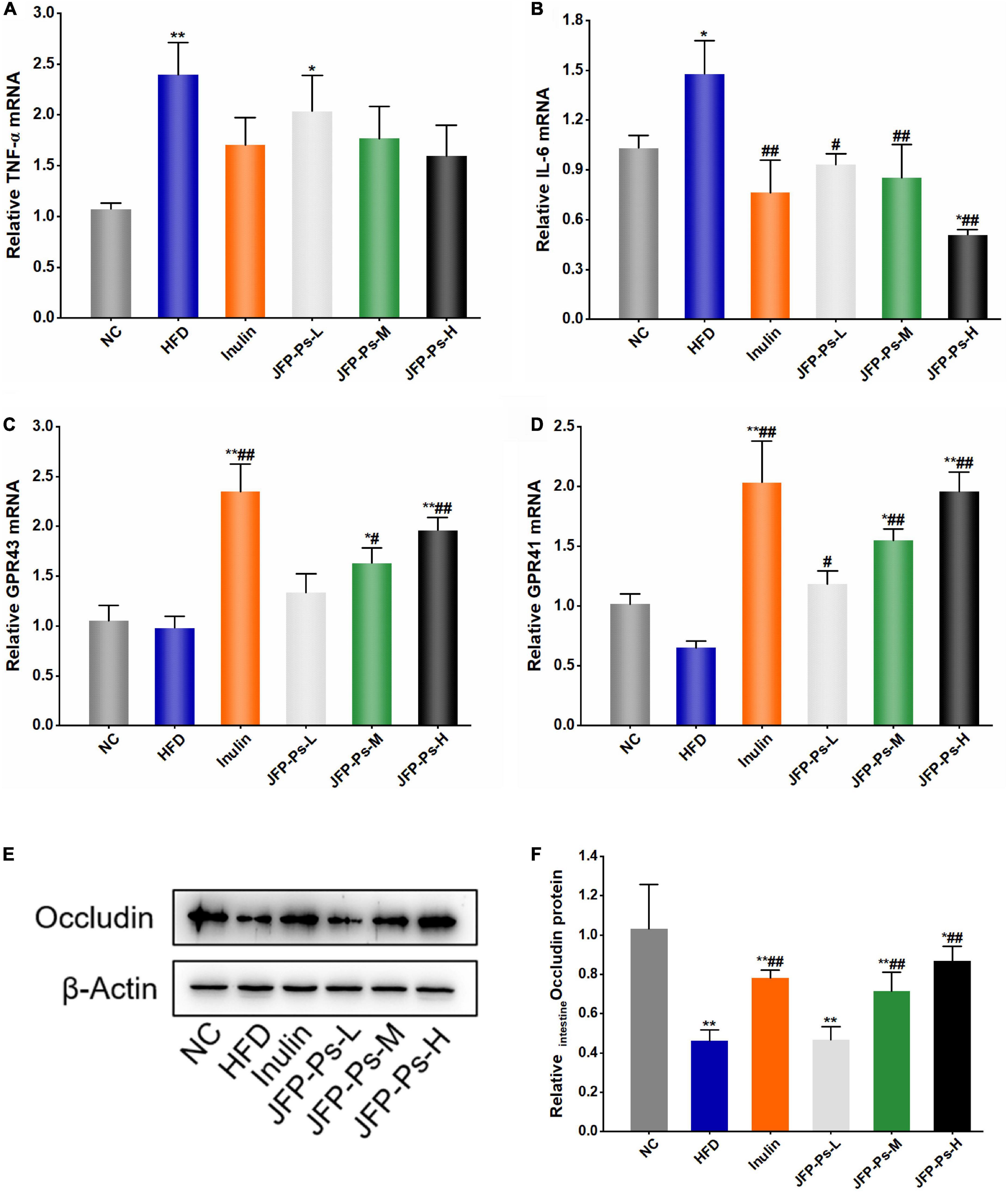
Figure 4. Effect of JFP-Ps on the expression of inflammatory genes, free fatty acid receptor genes and tight junction protein in the small intestine of obese rats. (A) TNF-α, (B) IL-6, (C) GPR43, (D) GPR41, (E) protein expression of occludin, (F) relative band intensities of occludin. Data are expressed as mean ± SEM (n = 6 for each group) and analyzed using one-way ANOVA. *p < 0.05, **p < 0.01 compared to the NC group; #p < 0.05, ##p < 0.01 compared with the HFD group.
Effects of JFP-Ps on the protein expression level of occludin in small intestine
Western blot analysis showed that protein expression level of occludin in the HFD group was significantly lower than the NC group (p < 0.01) (Figures 4E,F). JFP-Ps significantly increased the protein expression of occludin in a concentration-dependent manner (p < 0.01). The result showed that JFP-Ps may enhance mechanical barrier function in the small intestine.
Discussion
Intestine is the largest digestive and immune organ in the body, provides a favorable and anaerobic environment for microbial colonization and performs an important role in nutrient absorption, detoxification and immune regulation (2, 3). Intestinal dysfunction is closely associated with obesity and other related metabolic diseases (19, 20). Natural polysaccharides could prevent and treat intestinal diseases caused by various factors via restoring intestinal barrier function (21). The results of this study indicated that JFP-Ps possessed a protective effect on the intestine via improving intestinal barrier function and alleviating intestinal inflammation.
The higher water content in the feces is accompanied by an increase in the volume and looseness of the feces, which facilitates the body’s bowel movements (22). A high water content in feces also indicates a high moisture environment in the intestine, which may help the exchange and transport of substances and the intestinal mucus layer in dissolving mucins, immune factors, electrolytes, etc., thus maintaining the integrity of the intestinal mucosal layer and the balance of osmotic pressure and protecting the intestinal barrier (23–25). In addition, water in the intestinal lumen enters the enterocytes in the villi by osmosis, causing the cells to swell and the tissue to thicken, leading to spontaneous bending of adjacent tissue, promoting the formation of crypt foci in the intestine, and reducing the lumen volume (26). Do et al. (27) reported that polysaccharide fraction from greens of Raphanus sativus increased water content in the feces of obese mice induced by high-fat diet. JFP-Ps increased the water-holding capacity of feces and promoted the body’s bowel movements, implying that the intake of JFP-Ps may contribute to the integrity of the intestinal mucosal layer, formation of the crypt, and protection of the intestinal barrier.
The pH value of the intestinal contents is an important parameter in measuring intestinal health (28). An increased intestinal pH value is associated with a decrease in the abundance of probiotic bacteria (such as Lactobacillus and Bifidobacterium) and an increase in the abundance of pathogenic bacteria. Oligosaccharides were fermented by intestinal microorganisms to produce SCFAs, lowering intestinal pH value and promoting the growth of probiotic bacteria (29). In the study, JFP-Ps was found to decrease intestinal pH value, suggesting that JFP-Ps may be fermented by intestinal microorganisms to produce SCFAs, creating an acidic intestinal environment, inhibiting the growth of harmful bacteria, promoting the growth of probiotic bacteria and the balance of intestinal flora, thereby enhancing the biological barrier function of the intestine in the obese rats. The results were consistent with our previous study (16).
The colon acts as an important site for absorbing water and salt from food residues and provides a habitat for intestinal flora. Dietary fibers are fermented in the colon, and the timing and effect of fermentation is influenced by the growth state of the colon (30). The colon length may be shortened due to intestinal diseases. However, the butyric acid produced by intestinal probiotics (e.g., Lactobacillus, Bifidobacterium, etc.) from the fermentation of dietary polysaccharides provides 60–70% energy for the colon cells, promoting the regeneration and growth of colon epithelial cells, which in turn increases colon length (30, 31). Polysaccharide fraction from greens of Raphanus sativus was reported to increase colon length and restore intestinal barrier function in high-fat diet induced obese mice (27). In the study, JFP-Ps increased colon length in a dose-dependent manner, restored intestinal mucosal damage and increased the thickness of the mucus layer in obese rats induced by a high-fat diet, suggesting that JFP-Ps may enhance intestinal barrier function and reduce the risk of intestinal diseases.
The antioxidant enzymes SOD, GSH-Px and CAT constitute the body’s enzymatic antioxidant system, play an important role in protecting the body from oxidative damage, and are regarded as the first line of defense against oxidative damage (32). MDA is a product of lipid peroxidation in tissues and organs, and its level reflects the degree of tissue damage. A high-fat diet causes oxidative damage in the body and promotes the development of obesity. Wang et al. (7) reported that walnut green husk polysaccharides could prevent colonic oxidative stress and inflammation damage caused by high-fat diets. Our previous study has found that JFP-Ps exhibited a strong free radical scavenging activity (14). Consistent with these reports, JFP-Ps increased the activities of SOD, GSH-Px and CAT and decreased the content of MDA, suggesting that JFP-Ps may maintain the integrity of intestinal epithelium by increasing the activities of antioxidant enzymes in the colon of obese rats induced by a high-fat diet.
Weakening of the intestinal mucosal barrier allows a large number of foreign antigens to enter the intestinal wall, inducing an inflammatory response in the gut and accumulation of inflammatory cells and inflammatory cytokines, and triggering an immune response and damage (33). MPO is a hemoglobin enriched in neutrophils, and MPO activity was used to reflect neutrophil aggregation and tissue inflammation. TNF-α, IL-1β and IL-6 are typical pro-inflammatory cytokines that promote the inflammatory cascade; IL-10 is an anti-inflammatory cytokine that inhibits the inflammatory response. Liu et al. (34) reported that Rheum tanguticum polysaccharide significantly reduced MPO activity in the colonic mucosa of rats with colitis. A polysaccharide purified from Arctium lappa inhibited the increase of pro-inflammatory factors in the colon of mice with colitis (35). Angelica sinensis polysaccharide increased IL-10 level in the colon of rats with immune colonic injury (36). Consistent with these reports, JFP-Ps decreased the contents of TNF-α, IL-1β and IL-6, and increased the content of IL-10, suggesting that JFP-Ps may alleviate colonic inflammation in high-fat diet-induced obesity rats.
SGLT1 is a key transporter mainly expressed in small intestinal tissues, which involved in glucose absorption in the intestinal lumen, and closely associated with metabolic diseases, such as obesity and diabetes (37). In obese patients with type 2 diabetes, the overexpression of SGLT1 caused an abnormal increase in blood glucose in the body (38). Inhibition of SGLT1 expression can reduce glucose absorption in small intestine, the escaped glucose transferred into the large intestine and fermented to produce SCFAs, which in turn reduces the occurrence of obesity and type 2 diabetes (37, 39). Consistent with the previous study, JFP-Ps reduced SGLT1 activity in the intestinal epithelial cells of obese rats, suggesting that JFP-Ps may alleviate the development of obesity.
The expression levels of TNF-α and IL-6 in small intestinal tissues may reflect the inflammatory status of small intestinal tissues in obese rats. Han et al. (40) found that polysaccharide from Gracilaria Lemaneiformise reduced intestinal inflammation and prevented colitis in mice by decreasing the levels of pro-inflammatory factors in the mouse colon. GPR41 and GPR43 is a group of free fatty acid receptors that are activated by SCFAs. Intestinal microbes can ferment indigestible dietary fiber to produce SCFAs, which in turn activate GPR41 and GPR43, and mediate immune function in the small intestine (41–43). Consistent with these reports, our results showed that JFP-Ps down-regulated the expression of pro-inflammatory genes (TNF-α, IL-6), up-regulated the expression of GPR41 and GPR43. These results suggested that JFP-Ps could alleviate inflammation and enhance immune barrier function of the small intestine in obese rats via inhibiting the expression of pro-inflammatory genes and activating SCFA- and GPR41/GPR43-related signaling pathways in the small intestine of obesity rats.
Occludin is a tight junction protein and is believed to be directly involved in the barrier and fence functions of tight junctions (44). Occludin has been identified as an important component of the intestinal mechanical barrier and regulates macromolecule flux across the intestinal epithelial tight junction barrier (45). Sang et al. (46) reported that polysaccharide from sporoderm-broken spore of Ganoderma lucidum up-regulated the expression of occludin protein in the ileum of mice fed with high-fat diet. In line with this report, JFP-Ps increased the protein expression of occludin in a concentration-dependent manner, suggesting that JFP-Ps may enhance mechanical barrier function in the small intestine of obese rats.
Conclusion
In conclusion, JFP-Ps exhibited a protective effect on intestinal function and was beneficial to intestinal health. As shown in Figure 5, JFP-Ps promoted bowel movements and modified intestinal physiochemical environment by lowering fecal pH value and increasing fecal water content. Meanwhile, JFP-Ps was found to increase the length of the colon, alleviate oxidative damage of the colon, relieve intestinal colonic inflammation, and inhibit glucose transport in the small intestine. In addition, JFP-Ps repaired intestinal mucosal damage, and increased the thickness of the mucus layer. The potential mechanism of JFP-Ps improved intestinal barrier functions involved in inhibiting the expression of the inflammatory genes (TNF-α, IL-6), promoting the expression of the tight junction protein (occludin), and activating SCFA-GPR41/GPR43 related signaling pathways. Our findings would provide theoretical support for the development of JFP-Ps as a promising phytochemical to improve human health.
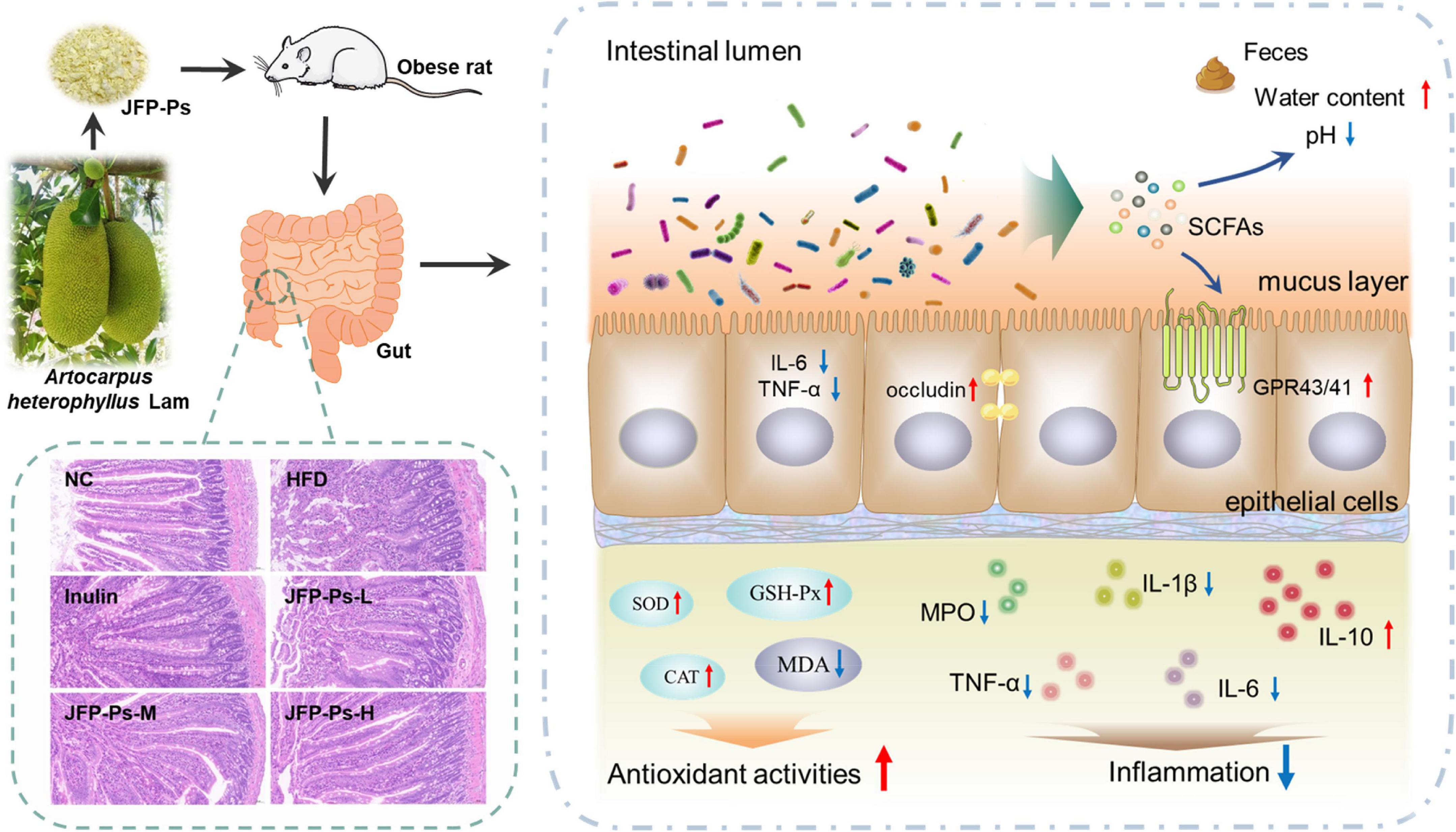
Figure 5. The beneficial effects of JFP-Ps on intestinal barrier function in high fat diet-induced obese rats.
Data availability statement
The data presented in this study are included in the article/Supplementary material, further inquiries can be directed to the corresponding authors.
Ethics statement
This animal study was approved by the Animal Ethics Committee of Hainan Medical University.
Author contributions
SZ, JC, KZ, QL, and LT designed the experiments. SZ and YC conducted the experiments. CL, GW, XC, and FX analyzed the data. SZ, JC, KZ, and LT wrote and revised the manuscript and gave the final approval. All authors contributed to the article and approved the submitted version.
Funding
This work was financially supported by the National Natural Science Foundation of China (No. 31901649) and the Central Public-interest Scientific Institution Basal Research Fund for Chinese Academy of Tropical Agricultural Sciences (No. 1630142022009).
Acknowledgments
We are grateful to Wuhan Servicebio Technology Co., Ltd., and Shanghai Majorbio Bio-Pharm Technology Co., Ltd., (Shanghai, China) for their outstanding technical assistance they provided.
Conflict of interest
The authors declare that the research was conducted in the absence of any commercial or financial relationships that could be construed as a potential conflict of interest.
The handling editor declared a shared parent affiliation with the several authors KZ and LT at the time of review.
Publisher’s note
All claims expressed in this article are solely those of the authors and do not necessarily represent those of their affiliated organizations, or those of the publisher, the editors and the reviewers. Any product that may be evaluated in this article, or claim that may be made by its manufacturer, is not guaranteed or endorsed by the publisher.
Supplementary material
The Supplementary Material for this article can be found online at: https://www.frontiersin.org/articles/10.3389/fnut.2022.1035619/full#supplementary-material
Abbreviations
JFP-Ps, polysaccharides from Artocarpus heterophyllus Lam. (jackfruit) pulp; SOD, superoxide dismutase; GSH-Px, glutathione peroxidase; CAT, catalase; MDA, malondialdehyde; MPO, myeloperoxidase; TNF-α, tumor necrosis factor-α; IL-1β, interleukin-1β; IL-6, interleukin-6; IL-10, interleukin-10; SGLT1, sodium-glucose cotransporter 1; GPR43, G protein-coupled receptor 43; GPR41, G protein-coupled receptor 41; SCFAs, short-chain fatty acids.
References
1. Sekirov, I, Russell SL, Antunes LCM, Finlay BB. Gut microbiota in health and disease. Physiol Rev. (2009) 90:859–904. doi: 10.1152/physrev.00045.2009
3. Zhou CB, Zhou YL, Fang JY. Gut microbiota in cancer immune response and immunotherapy. Trends Cancer. (2021) 7:647–60. doi: 10.1016/j.trecan.2021.01.010
4. Wu YB, Wan JW, Choe U, Pham Q, Schoene NW, He Q, et al. Interactions between food and gut microbiota: impact on human health. Annu Rev Food Sci Technol. (2019) 10:389–408. doi: 10.1146/annurev-food-032818-121303
5. Zhang GY, Nie SP, Huang XJ, Hu JL, Cui SW, Xie MY, et al. Study on dendrobium officinale o-acetyl-glucomannan (dendronan). 7. improving effects on colonic health of mice. J Agr Food Chem. (2016) 64:2485–91. doi: 10.1021/acs.jafc.5b03117
6. Huang YL, Chau CF. Improvement in intestinal function of hamsters as influenced by consumption of polysaccharide-rich sage weed extracts. Food Chem. (2012) 133:1618–23. doi: 10.1016/j.foodchem.2012.02.062
7. Wang GL, Yang XY, Wang J, Zhong DY, Zhang RG, Zhang YN, et al. Walnut green husk polysaccharides prevent obesity, chronic inflammatory responses, nonalcoholic fatty liver disease and colonic tissue damage in high-fat diet fed rats. Int J Biol Macromol. (2021) 182:879–98. doi: 10.1016/j.ijbiomac.2021.04.047
8. Xie SZ, Liu B, Ye HY, Li QM, Pan LH, Zha XQ, et al. Dendrobium huoshanense polysaccharide regionally regulates intestinal mucosal barrier function and intestinal microbiota in mice. Carbohyd Polym. (2019) 206:149–62. doi: 10.1016/j.carbpol.2018.11.002
9. Huo JY, Wu ZY, Sun WZ, Wang ZH, Wu JH, Huang MQ, et al. Protective effects of natural polysaccharides on intestinal barrier injury: a review. J Agr Food Chem. (2022) 70:711–35. doi: 10.1021/acs.jafc.1c05966
10. Min FF, Wan YJ, Nie SP, Xie MY. Study on colon health benefit of polysaccharide from Cyclocarya paliurus leaves in vivo. J Funct Foods. (2014) 11:203–9. doi: 10.1016/j.jff.2014.10.005
11. Wang XY, Yin JY, Nie SP, Xie MY. Isolation, purification and physicochemical properties of polysaccharide from fruiting body of Hericium erinaceus and its effect on colonic health of mice. Int J Biol Macromol. (2018) 107:1310–9. doi: 10.1016/j.ijbiomac.2017.09.112
12. Gao H, Zhang WC, Wu ZY, Wang HY, Hui AL, Meng L, et al. Preparation, characterization and improvement in intestinal function of polysaccharide fractions from okra. J Funct Foods. (2018) 50:147–57. doi: 10.1016/j.jff.2018.09.035
13. Tan YF, Li HF, Lai WY, Zhang JQ. Crude dietary polysaccharide fraction isolated from jackfruit enhances immune system activity in mice. J Med Food. (2013) 16:663–8. doi: 10.1089/jmf.2012.2565
14. Zhu KX, Zhang YJ, Nie SP, Xu F, He SZ, Gong D, et al. Physicochemical properties and in vitro antioxidant activities of polysaccharide from Artocarpus heterophyllus lam. pulp. Carbohyd Polym. (2017) 155:354–61. doi: 10.1016/j.carbpol.2016.08.074
15. Zhu KX, Yao SW, Zhang YJ, Liu QB, Xu F, Wu G, et al. Effects of in vitro saliva, gastric and intestinal digestion on the chemical properties, antioxidant activity of polysaccharide from Artocarpus heterophyllus lam. (Jackfruit) pulp. Food Hydrocolloid. (2019) 87:952–9. doi: 10.1016/j.foodhyd.2018.09.014
16. Zhu KX, Fan HF, Zeng SJ, Nie SP, Zhang YJ, Tan LH, et al. Polysaccharide from Artocarpus heterophyllus lam. (jackfruit) pulp modulates gut microbiota composition and improves short-chain fatty acids production. Food Chem. (2021) 364:130434. doi: 10.1016/j.foodchem.2021.130434
17. Thøgersen R, Castro-Mejía JL, Kræmer Sundekilde U, Hansen LH, Gray N, Kuhnle G, et al. Inulin and milk mineral fortification of a pork sausage exhibits distinct effects on the microbiome and biochemical activity in the gut of healthy rats. Food Chem. (2020) 331:127291. doi: 10.1016/j.foodchem.2020.127291
18. Massot-Cladera M, Azagra-Boronat I, Franch À, Castell M, Rodríguez-Lagunas MJ, Pérez-Cano FJ. Gut health-promoting benefits of a dietary supplement of vitamins with inulin and acacia fibers in rats. Nutrients. (2020) 12:2196. doi: 10.3390/nu12082196
19. Winer DA, Winer S, Dranse HJ, Lam TKT. Immunologic impact of the intestine in metabolic disease. J Clin Invest. (2017) 127:33–42. doi: 10.1172/JCI88879
20. Khan S, Luck H, Winer S, Winer DA. Emerging concepts in intestinal immune control of obesity-related metabolic disease. Nat Commun. (2021) 12:2598. doi: 10.1038/s41467-021-22727-7
21. Ma S, Xu JX, Lai T, Xu WN, Zhang J, Zhang HC, et al. Inhibitory effect of fermented flammulina velutipes polysaccharides on mice intestinal inflammation. Front Nutr. (2022) 9:934073. doi: 10.3389/fnut.2022.934073
22. Roberfroid M. Dietary fiber, inulin, and oligofructose: a review comparing their physiological effects. Crit Rev Food Sci Nutr. (1993) 33:103–48. doi: 10.1080/10408399309527616
23. Okazaki Y, Katayama T. Dietary phytic acid modulates characteristics of the colonic luminal environment and reduces serum levels of proinflammatory cytokines in rats fed a high-fat diet. Nutr Res. (2014) 34:1085–91. doi: 10.1016/j.nutres.2014.09.012
24. Da Silva JK, Cazarin CBB, Bogusz Junior S, Augusto F, Maróstica Junior MR. Passion fruit (Passiflora edulis) peel increases colonic production of short-chain fatty acids in wistar rats. LWT Food Sci Technol. (2014) 59:1252–7. doi: 10.1016/j.lwt.2014.05.030
25. Hughes MR. Regulation of salt gland, gut and kidney interactions. Comparative biochemistry and physiology. Comput Biochem Physiol Mol Integr Physiol. (2003) 136:507–24. doi: 10.1016/j.cbpb.2003.09.005
26. Yang QT, Xue SL, Chan CJ, Rempfler M, Vischi D, Maurer-Gutierrez F, et al. Cell fate coordinates mechano-osmotic forces in intestinal crypt formation. Nat Cell Biol. (2021) 23:733–44. doi: 10.1038/s41556-021-00700-2
27. Do MH, Lee HB, Oh MJ, Jhun H, Choi SY, Park HY. Polysaccharide fraction from greens of Raphanus sativus alleviates high fat diet-induced obesity. Food Chem. (2021) 343:128395. doi: 10.1016/j.foodchem.2020.128395
28. Chung YC, Hsu CK, Ko CY, Chan YC. Dietary intake of xylooligosaccharides improves the intestinal microbiota, fecal moisture, and pH value in the elderly. Nutr Res. (2007) 27:756–61. doi: 10.1016/j.nutres.2007.09.014
29. Mehta S, Huey SL, McDonald D, Knight R, Finkelstein JL. Nutritional interventions and the gut microbiome in children. Annu Rev Nutr. (2021) 41:479–510. doi: 10.1146/annurev-nutr-021020-025755
30. Jädert C, Phillipson M, Holm L, Lundberg JO, Borniquel S. Preventive and therapeutic effects of nitrite supplementation in experimental inflammatory bowel disease. Redox Biol. (2013) 2:73–81. doi: 10.1016/j.redox.2013.12.012
31. Rivière A, Selak M, Lantin D, Leroy F, De Vuyst L. Bifidobacteria and butyrate-producing colon bacteria: importance and strategies for their stimulation in the human gut. Front Microbiol. (2016) 7:979. doi: 10.3389/fmicb.2016.00979
32. Wang XY, Yin JY, Hu JL, Nie SP, Xie MY. Gastroprotective polysaccharide from natural sources: review on structure, mechanism, and structure-activity relationship. Food Front. (2022) 172:1–32. doi: 10.1002/fft2.172
33. Korzenik JR, Podolsky DK. Evolving knowledge and therapy of inflammatory bowel disease. Nat Rev Drug Discov. (2006) 5:197–209. doi: 10.1038/nrd1986
34. Liu L, Guo ZJ, Lv ZG, Sun Y, Cao W, Zhang R, et al. The beneficial effect of Rheum tanguticum polysaccharide on protecting against diarrhea, colonic inflammation and ulceration in rats with TNBS-induced colitis: the role of macrophage mannose receptor in inflammation and immune response. Int Immunopharmacol. (2008) 8:1481–92. doi: 10.1016/j.intimp.2008.04.013
35. Wang Y, Zhang NF, Kan J, Zhang X, Wu XN, Sun R, et al. Structural characterization of water-soluble polysaccharide from Arctium lappa and its effects on colitis mice. Carbohydr Polym. (2019) 213:89–99. doi: 10.1016/j.carbpol.2019.02.090
36. Liu SP, Dong WG, Wu DF, Luo HS, Yu JP. Protective effect of angelica sinensis polysaccharide on experimental immunological colon injury in rats. World J Gastroenterol. (2003) 9:2786–90. doi: 10.3748/wjg.v9.i12.2786
37. Lehmann A, Hornby PJ. Intestinal SGLT1 in metabolic health and disease. Am J Physiol Gastrointest Liver Physiol. (2016) 310:G887–98. doi: 10.1152/ajpgi.00068.2016
38. Zambrowicz B, Ding ZM, Ogbaa I, Frazier K, Banks P, Turnage A, et al. Effects of LX4211, a dual SGLT1/SGLT2 inhibitor, plus sitagliptin on postprandial active GLP-1 and glycemic control in type 2 diabetes. Clin Ther. (2013) 35:273. doi: 10.1016/j.clinthera.2013.01.010
39. Zhang YJ, Xie QT, You LJ, Cheung PC, Zhao ZG. Behavior of non-digestible polysaccharides in gastrointestinal tract: a mechanistic review of its anti-obesity effect. eFood. (2021) 2:59–72. doi: 10.2991/efood.k.210310.001
40. Han R, Wang L, Zhao ZG, You LJ, Pedisić S, Kulikouskaya V, et al. Polysaccharide from gracilaria lemaneiformis prevents colitis in Balb/c mice via enhancing intestinal barrier function and attenuating intestinal inflammation. Food Hydrocolloid. (2020) 109:106048. doi: 10.1016/j.foodhyd.2020.106048
41. Brown AJ, Goldsworthy SM, Barnes AA, Eilert MM, Tcheang L, Daniels D, et al. The orphan G protein-coupled receptors GPR41 and GPR43 are activated by propionate and other short chain carboxylic acids. J Biol Chem. (2003) 278:11312–9. doi: 10.1074/jbc.M211609200
42. Kimura I, Ichimura A, Ohue-Kitano R, Igarashi M. Free fatty acid receptors in health and disease. Physiol Rev. (2020) 100:171–210. doi: 10.1152/physrev.00041.2018
43. Kim MH, Kang SG, Park JH, Yanagisawa M, Kim CH. Short-chain fatty acids activate GPR41 and GPR43 on intestinal epithelial cells to promote inflammatory responses in mice. Gastroenterology. (2013) 145:e1–10. doi: 10.1053/j.gastro.2013.04.056
44. Saitou M, Fujimoto K, Doi Y, Itoh M, Fujimoto T, Furuse M, et al. Occludin-deficient embryonic stem cells can differentiate into polarized epithelial cells bearing tight junctions. J Cell Biol. (1998) 141:397–408. doi: 10.1083/jcb.141.2.397
45. Saitoh Y, Suzuki H, Tani K, Nishikawa K, Irie K, Ogura Y, et al. Tight junctions. Structural insight into tight junction disassembly by Clostridium perfringens enterotoxin. Science. (2015) 347:775–8. doi: 10.1126/science.1261833
Keywords: Artocarpus heterophyllus Lam. polysaccharide, intestinal function, inflammation, protective effect, obese rats
Citation: Zeng S, Cao J, Chen Y, Li C, Wu G, Zhu K, Chen X, Xu F, Liu Q and Tan L (2022) Polysaccharides from Artocarpus heterophyllus Lam. (jackfruit) pulp improves intestinal barrier functions of high fat diet-induced obese rats. Front. Nutr. 9:1035619. doi: 10.3389/fnut.2022.1035619
Received: 03 September 2022; Accepted: 07 October 2022;
Published: 03 November 2022.
Edited by:
Yanjun Zhang, Chinese Academy of Tropical Agricultural Sciences, ChinaReviewed by:
Fuqiang Zhao, Chongqing University, ChinaQiuting Zhang, Nanjing Normal University, China
Yan Zhou, Guizhou Medical University, China
Copyright © 2022 Zeng, Cao, Chen, Li, Wu, Zhu, Chen, Xu, Liu and Tan. This is an open-access article distributed under the terms of the Creative Commons Attribution License (CC BY). The use, distribution or reproduction in other forums is permitted, provided the original author(s) and the copyright owner(s) are credited and that the original publication in this journal is cited, in accordance with accepted academic practice. No use, distribution or reproduction is permitted which does not comply with these terms.
*Correspondence: Kexue Zhu, emh1a2V4dWUxNjNAMTYzLmNvbQ==; Lehe Tan, dGxoMzY4N0AxNjMuY29t
†These authors have contributed equally to this work and share first authorship