- 1Liggins Institute, The University of Auckland, Auckland, New Zealand
- 2Nestlé Research, Société des Produits Nestlé SA, Singapore, Singapore
- 3Department of Women's and Children's Health, Uppsala University, Uppsala, Sweden
- 4Environmental-Occupational Health Sciences and Non-Communicable Diseases Research Group, Research Institute for Health Sciences, Chiang Mai University, Chiang Mai, Thailand
- 5Department of Paediatrics: Child and Youth Health, Faculty of Medical and Health Sciences, School of Medicine, University of Auckland, Auckland, New Zealand
- 6Nestlé Research, Société des Produits Nestlé SA, Beijing, China
- 7Nestlé Research, Société des Produits Nestlé SA, Lausanne, Switzerland
- 8MRC Lifecourse Epidemiology Unit, University of Southampton, Southampton, United Kingdom
- 9NIHR Southampton Biomedical Research Centre, University Hospital Southampton, NHS Foundation Trust, Southampton, United Kingdom
- 10Department of Obstetrics and Gynaecology, Yong Loo Lin School of Medicine, National University of Singapore and National University Health System, Singapore, Singapore
- 11Singapore Institute for Clinical Sciences, Agency for Science, Technology and Research, Singapore, Singapore
- 12A Better Start – National Science Challenge, The University of Auckland, Auckland, New Zealand
Introduction: During pregnancy and lactation minerals such as zinc are required to support maternal and infant health. Zinc is involved in various cellular processes, with requirements increasing in pregnancy and lactation. In the setting of a randomized trial, we investigated the effects on human milk (HM) zinc concentrations of a micronutrient-containing supplement including zinc in the intervention (but not control) group, started preconception and taken throughout pregnancy until birth. Additionally, we characterized longitudinal changes in HM concentrations of zinc and other minerals (calcium, copper, iodine, iron, magnesium, manganese, phosphorus, potassium, selenium, and sodium).
Methods: HM samples were collected across 7 time points from 1 week to 12 months from lactating mothers from Singapore (n = 158) and New Zealand (n = 180). HM minerals were quantified using sector field inductively coupled plasma mass spectrometry. Potential intervention effects on HM mineral concentrations were assessed using linear mixed models with a repeated measures design and time-weighted area-under-the-curve analyses.
Results: Over the first 3 months of lactation, HM zinc concentrations were 11% higher in the intervention group compared to the control group (p = 0.021). Higher HM zinc concentrations were most evident at 6 weeks of lactation. The intervention had no effect on HM concentrations of other minerals, which were not differently supplemented to the control and intervention groups. Temporal changes in HM minerals over 12 months of lactation were studied in the New Zealand mothers; HM zinc and copper concentrations progressively decreased throughout 12 months, while iron, potassium, sodium, and phosphorus decreased until 6 months then plateaued. HM calcium and magnesium initially increased in early lactation and iodine remained relatively constant throughout 12 months. HM manganese and selenium fell over the initial months of lactation, with a nadir at 6 months, and increased thereafter. The contrasting patterns of changes in HM mineral concentrations during lactation may reflect different absorption needs and roles at different stages of infancy.
Discussion: Overall, this study indicates that HM zinc concentrations are influenced by maternal supplementation during preconception and pregnancy. Further studies are required to understand the associations between HM zinc and other minerals and both short- and long-term offspring outcomes.
Trial registration: ClinicalTrials.gov, identifier: NCT02509988, Universal Trial Number U1111-1171-8056. Registered on 16 July 2015. This is an academic-led study by the EpiGen Global Research Consortium.
1. Introduction
Adequate mineral status during pregnancy and lactation is essential for the health of the mother and optimal growth and development of the infant (1). Minerals play important roles in brain development (iron, zinc, copper, and iodine), bone health (calcium, phosphorus, and magnesium), and thyroid hormone metabolism (selenium, zinc, and iodine) (1, 2).
Mineral requirements increase during pregnancy to support the physiological changes in the mother and the growing fetus. For example, an ~18–36% additional zinc is required during pregnancy (3), the daily recommended intake being 7–15 mg (4–6). Zinc deficiency in pregnancy is prevalent worldwide, especially in countries with poor nutrition (7, 8). In New Zealand, 18.8% of women aged 19–30 years were estimated to have inadequate zinc intake (9). In Singapore, 19% of pregnant women (at 26–28 weeks' gestation) were estimated to be zinc deficient (10). This has been associated with adverse complications such as preterm birth, intrauterine growth restriction, and low infant birth weight (7, 11). In women with a risk of zinc deficiency, supplementation may be beneficial (12–14).
Human milk (HM) is the major source of zinc for newborn infants, who require 2–4 mg/day (aged 0–6 months) and 3–5 mg/day (aged 7–12 months) (4, 6). As such, physiological mechanisms to maintain adequate zinc status of the mother and the infant, via HM, continues during lactation. This is associated with mobilization of maternal zinc pools from involuting tissues as well as the trabecular bone (3, 14). In HM, zinc has been identified to be bound to protein ligands, casein and serum albumin associated with 14% and 28% of total HM zinc, respectively (15). Zinc is involved in various cellular processes, enzyme functions, and immune functions (16). Zinc has been associated with growth (17, 18), the immune system (19, 20), and cognitive development (21) in infants. Therefore, ensuring optimal maternal zinc status during lactation is crucial for supporting infant growth.
The Nutritional Intervention Preconception and During Pregnancy to Maintain Healthy Glucose Metabolism and Offspring Health (NiPPeR) study was designed to investigate the effects of an enhanced micronutrient supplement during preconception and pregnancy on maternal pregnancy outcomes and infant growth (22). The control and intervention supplements both contained micronutrients (i.e., calcium, iron, and iodine) that are part of common pregnancy supplements. For example, calcium plays a role in fetal bone formation, and enzyme and hormone functioning, and supplementation during pregnancy has been associated with reduced risks of preeclampsia and preterm birth (23). Iodine is essential for maternal and fetal thyroid hormone production, and its deficiency increases the risks of infant mortality and intellectual impairment (24, 25). Iron deficiency during pregnancy may lead to anemia in mothers, which has been associated with low birth weight and preterm birth (26). The intervention supplement contained additional micronutrients, including zinc. Overall, understanding the factors that influence HM mineral concentrations, such as maternal micronutrient supplement use and lactation stage, is essential to ensure optimal nutrition status in breastfeeding mothers and breastfed infants.
The aim of the present study was to assess the effects of the intervention supplement taken during preconception and pregnancy on subsequent HM zinc and other mineral concentrations. Moreover, the longitudinal changes in HM minerals (zinc, calcium, copper, iodine, iron, magnesium, manganese, phosphorus, potassium, selenium, and sodium) in the first year of lactation were analyzed. We hypothesized that (i) zinc supplementation during preconception/pregnancy would influence HM zinc concentrations during lactation, and (ii) HM concentrations of zinc and other minerals would have differing patterns of change over 12 months of lactation.
2. Materials and methods
2.1. Study design
The detailed protocol for the NiPPeR study (ClinicalTrials.gov, identifier: NCT02509988, Universal Trial Number U1111-1171-8056; registered on 16 July 2015) has been published previously (22). In brief, the NiPPeR study was a double-blind, randomized controlled trial investigating the effects of a nutritional supplement taken from preconception and during pregnancy on maternal pregnancy and infant outcomes. The control supplement comprised of standard amounts of micronutrients that are present in supplements commonly used during pregnancy including calcium, iron, iodine, folic acid, and β-carotene (Table 1). In addition to these nutrients, the NiPPeR intervention supplement contained vitamins B2, B6, B12, and D, as well as zinc, myo-inositol, and probiotics (Table 1). Importantly, zinc was the only mineral present in the intervention supplement, but not in the control supplement. The study supplements were packaged as a powder in sachets, and were taken twice daily as a drink reconstituted with water. Adherence to the study drinks was ascertained by sachet counting, with good adherence defined as at least 60% of the sachets taken (27). The study was conducted in Southampton (UK), Singapore, and Auckland (New Zealand), with ethics approval obtained at each site [Southampton—Health Research Authority National Research Ethics Service Committee South Central Research Ethics Committee (15/SC/0142); Singapore—the National Healthcare Group Domain Specific Review Board (2015/00205); and New Zealand—Northern A Health and Disability Ethics Committee (15/NTA/21)]. All participants provided written informed consent.
2.2. Study participants
Participants were recruited by self-referral after study information was disseminated through local and social media advertisements. The full inclusion, exclusion, and withdrawal criteria have been reported previously (22), and are provided in Supplementary Table 1. Briefly, women aged 18–38 years who were planning to conceive within 6 months were eligible for the study and withdrawn if they had not conceived within 12 months. Eligible participants were randomized in a 1:1 ratio to either the control or the intervention group through the electronic study database (22), and stratified by site and ethnicity to ensure balanced allocation of participants.
2.3. Human milk sample collection
HM samples were collected only in Singapore for 3 months of lactation (from July 2016 to March 2019) and New Zealand for up to 12 months of lactation (from May 2017 to November 2019) (Figure 1). Samples were collected at 1 week ± 3 days, 3 weeks ± 5 days, 6 weeks ± 5 days, and 3 months ± 10 days (4 time points); in New Zealand, there were additional HM collections at 6 months ± 14 days, 9 months ± 14 days, and 12 months ± 14 days (7 time points overall). In Singapore, samples could only be collected until 3 months due to logistical constraints. HM samples were collected in the morning, and mothers were asked to refrain from breastfeeding for 2 h prior to collection from the unilateral breast from where samples would be collected. Whole HM samples were collected from a single breast using an Ameda Lactaline breast pump (Ameda, Inc, Murarrie, Australia). The breast was pumped for 15 min or until fully emptied, under the supervision of a trained staff. Soon after collection, HM samples were vortexed for homogenization, divided into aliquots and then stored at −80°C until analysis. HM samples were not collected if the mother refused, had ceased breastfeeding, her milk supply was low, or there were complications with milk expression. The total number of samples collected at each time point are outlined in Figure 1. The number of participants with longitudinal samples to 3 months of lactation is summarized in Table 2, and to 12 months of lactation in New Zealand in Table 3.
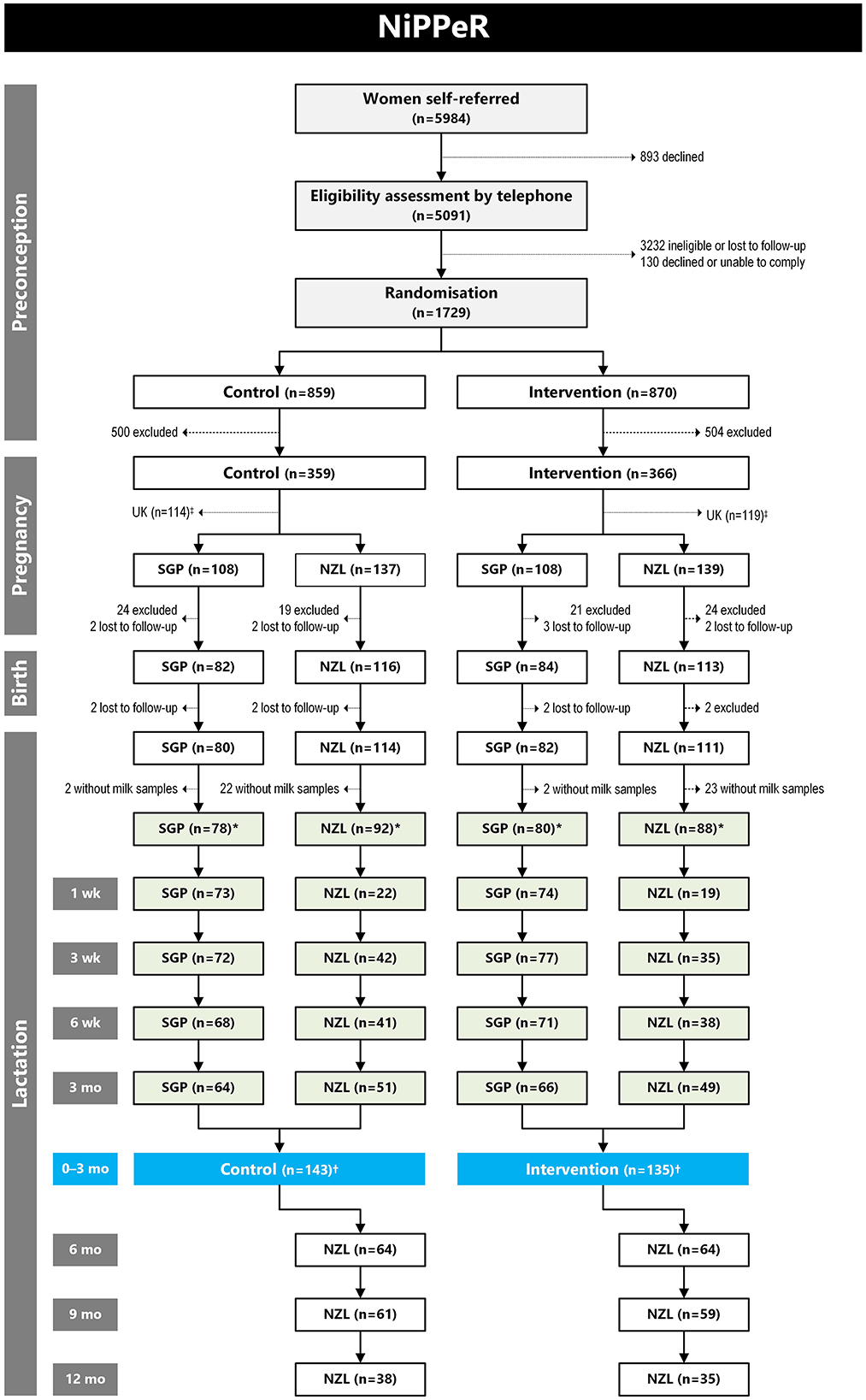
Figure 1. CONSORT diagram for number of human milk (HM) samples analyzed for mineral concentrations in the NiPPeR study. Reasons for exclusion during the preconception phase have been published previously (27), while reasons for exclusion during pregnancy and birth in Singapore (SGP) and New Zealand (NZL) are provided in Supplementary Table 2. There were no HM samples collected in the United Kingdom (UK), so all participants from that site were excluded from this diagram. *Number of participants who provided at least one HM sample during 12 months of lactation. †Number of participants who provided at least one HM sample during the first 3 months of lactation.
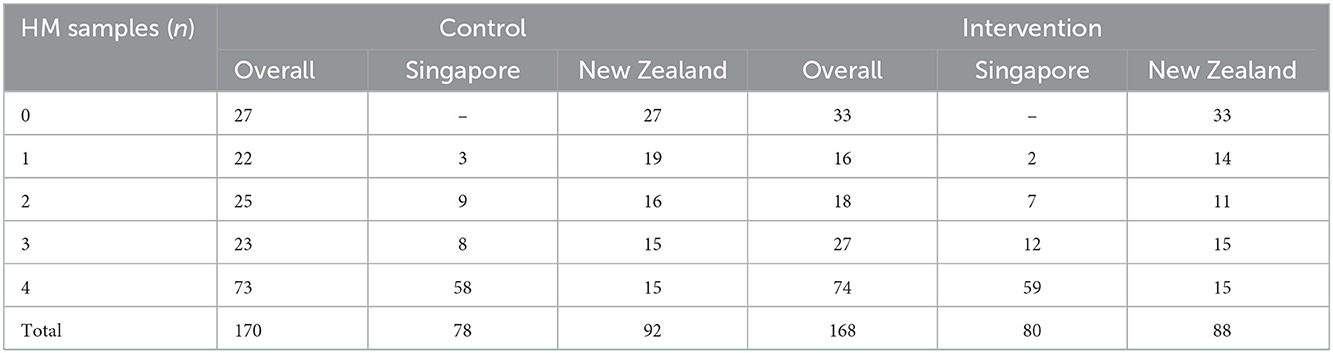
Table 2. Number of longitudinal human milk (HM) samples collected per participant in the first 3 months of lactation in Singapore and New Zealand.
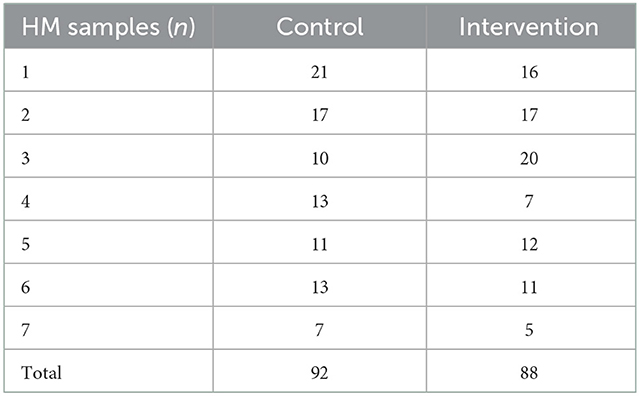
Table 3. Number of longitudinal human milk (HM) samples collected in New Zealand during 12 months of lactation.
2.4. Human milk mineral quantification
HM mineral quantification was carried out by ALS Scandinavia AB (Luleå, Sweden). HM calcium, cobalt, copper, iron, potassium, magnesium, manganese, sodium, nickel, phosphorus, selenium, and zinc were quantified using sector field inductively coupled plasma mass spectrometry (SF-ICP-MS), ELEMENT 2 (Thermo, Bremen, Germany) equipped with an ASX 500 sample changer (CETAC Technologies Inc., Omaha, USA), using a modified version of methods described by Rodushkin et al. (28, 29), with the sample intake for the microwave-assisted acidic decomposition reduced to 0.2 mL. Briefly, HM samples (0.2 mL), were transferred in perfluoroalkoxy polymer lined vessels and mineralized with a microwave oven (MDS-2000, CEM Corporation, Matthews, USA) using analytical grade nitric acid (Merck, Darmstadt, Germany) after additional purification by sub-boiling distillation in a quartz still. After mineralization, the resulting solutions were diluted with Milli-Q water (Millipore Milli-Q, Bedford, USA) and spiked with internal standard solution containing scandium, indium, and lutetium. Iodine was also quantified using SF-ICP-MS ELEMENT 2 with an ASX 500 sample changer, but following the instrumental method by Engström et al. (30). Sample preparation was slightly modified from Krachler et al. (31) using the alkaline reagent composition given by Engström et al. (30) and a dilution factor of 1:50. Briefly, prior to ICP-MS analysis, HM samples were diluted (with a dilution factor of 1:50) with alkaline diluent containing 0.01 M ammonia (Suprapur, Merck), 0.2 mM (NH4)2EDTA (Fluka) and 0.07% Triton X-100 (Merck). All intra- and inter-assay coefficients of variation were < 10%.
2.5. Statistical analyses
Mineral concentration measurements below the lower level of quantification (LLoQ) were assigned a value of 0.5 × LLoQ (Supplementary Table 3). To minimize the removal of values from the data set, we adopted a conservative approach defining extreme values (i.e., outliers) as measurements outside the mean ± 5 standard deviations (SD) range. There were no values below mean – 5 SD range, but for some minerals there were a few values greater than the mean + 5 SD classified as extreme values (i.e., >99.99997th percentile) (Supplementary Table 3) and removed from analyses. It was not possible to undertake reliable statistical analyses on cobalt and nickel as a large proportion of values were below the LLoQ (41.4 and 83.0%, respectively). For all other minerals, data were log-transformed to approximate a normal distribution, then back-transformed for reporting.
Potential intervention effects on HM mineral concentrations were only examined on the samples collected in the first 3 months of lactation, which were collected in both Singapore and New Zealand. In a sensitivity analysis this was also assessed in a subgroup of participants who provided consecutive samples across the 4 time points in the first 3 months. Data were analyzed using linear mixed models with a repeated measures design. Parameters included were randomization group, visit, their interaction term (group*visit), and study site, as well as adherence to the study protocol, maternal pre-pregnancy body mass index (BMI), and gestational age at birth as covariates. The participant's study ID was also included as a random factor to account for the multiple measurements on the same individual (non-independence). If the interaction term was statistically significant, between-group comparisons were only reported on a per-visit basis.
The time-weighted area-under-the-curve (TwAUC) was also calculated for each participant who had at least 3 valid HM measurements within the first 3 months of lactation, using the following formula:
where age0 and aget were the infant's ages when the first and last measurements used in the AUC were collected, respectively. TwAUC data were analyzed using general linear models adjusted for study site, adherence, maternal pre-pregnancy BMI, and gestational age at birth.
Subgroup analyses were also performed to examine potential treatment effects over the first 3 months of lactation separately for Singapore and New Zealand. Temporal changes in HM minerals from 1 week to 12 months of lactation were plotted and reported for the New Zealand site only. These were also examined in a subgroup of New Zealand participants who provided HM samples for at least five out of six time points between 3 weeks and 12 months.
Study outcomes are reported as the back-transformed least-squares means (i.e., adjusted means) for each group or the adjusted mean differences (aMD) between groups, and their respective 95% confidence intervals (CI). Note that the aMD for back-transformed values represent proportional differences between groups. Statistical analyses were carried using SAS version 9.4 (SAS Institute Inc., Cary, NC, USA) and graphs created with GraphPad Prism version 8.2.1 (GraphPad Software, San Diego, California USA). All statistical tests were two-sided with significance maintained at p < 0.05, without adjustments for multiple comparisons or imputation of missing values.
3. Results
3.1. Study population
At Singapore and New Zealand sites combined, 387 participants continued to postpartum stage of the study, of which 338 participants (87.3%) provided at least one HM sample during the study period (Figure 1). Maternal demographic and pre-pregnancy BMI characteristics were similar in control and intervention groups (Table 4), noting that participants were mostly Chinese in Singapore and Caucasian in New Zealand (Supplementary Table 4). Adherence to the study drinks was high and averaged at about 87% consumption for both groups. The mean (±SD) duration of supplementation was 405 ± 105 days in the control group and 393 ± 98 days in the intervention group. Passive smoking during pregnancy was more common among controls than in the Intervention group (19.4 vs. 9.5%, respectively; p = 0.013) [comparison made with a Fisher's exact test]. Other pregnancy and birth outcomes were also similar between the two groups overall (Table 4) and within sites (Supplementary Table 4). The characteristics for the subgroup who provided HM samples were similar to the total group of participants from Singapore and New Zealand sites who continued to the postpartum stage of the study (Supplementary Table 5).
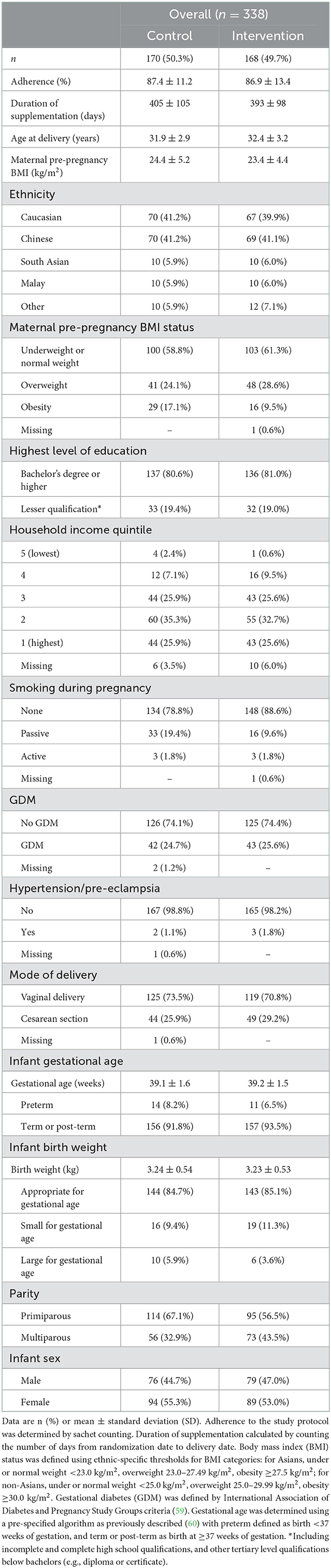
Table 4. Baseline and perinatal characteristics of participants in the NiPPeR study who provided at least one human milk sample in 12 months of lactation.
3.2. Impact of intervention on zinc and other minerals
The mean HM zinc concentrations over the first 3 months of lactation were 11% higher in the intervention than in the control group (p = 0.021; Table 5), with a similar difference observed for the TwAUC (p = 0.022;
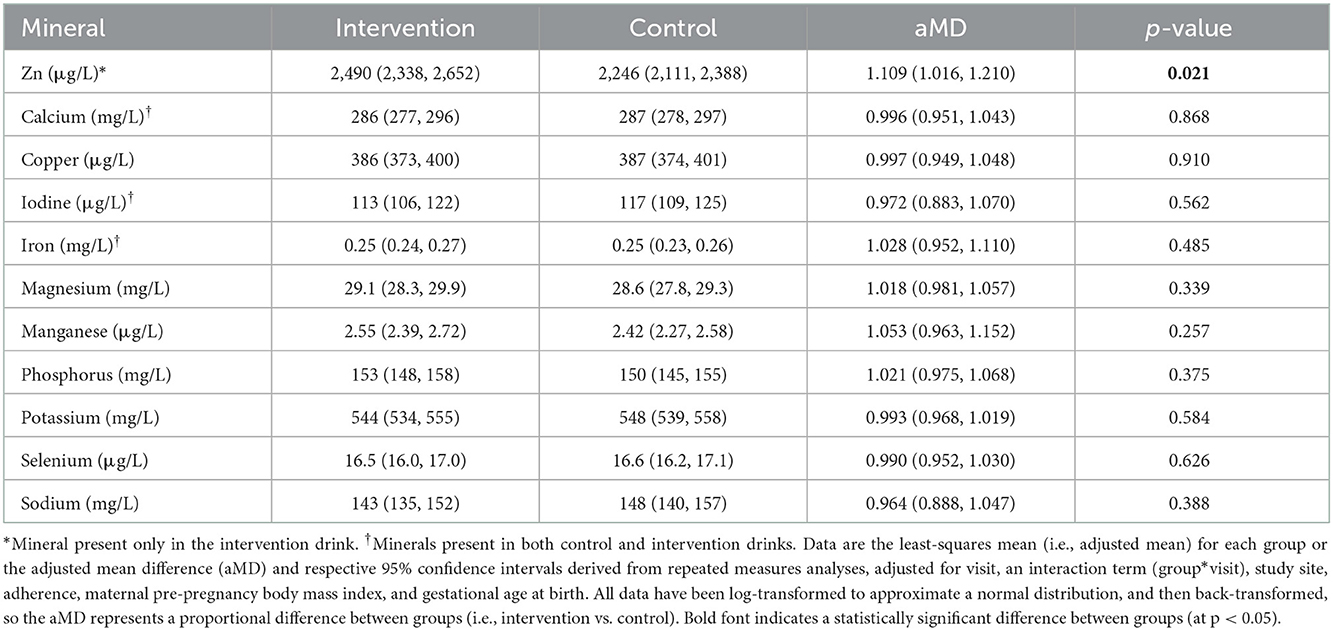
Table 5. Mineral concentrations in human milk (HM) over the first 3 months of lactation in the intervention and control groups.
Table 6). When zinc concentrations at individual visits were examined, the difference between groups was most evident at 6 weeks: 2,126 μg/L (95% CI 1,962, 2,304) and 1,790 μg/L (95% CI 1,654, 1,938) in the intervention and control groups, respectively (p = 0.003; Figure 2A). The intervention effect on HM zinc was also present in the subgroup of mothers who had all four consecutive samples examined in the first 3 months (n = 147, data not shown).

Table 6. Time-weighted area-under-the-curve for zinc concentrations (μg/mL/day) in human milk samples collected in the NiPPeR study between birth and 3 months of age.
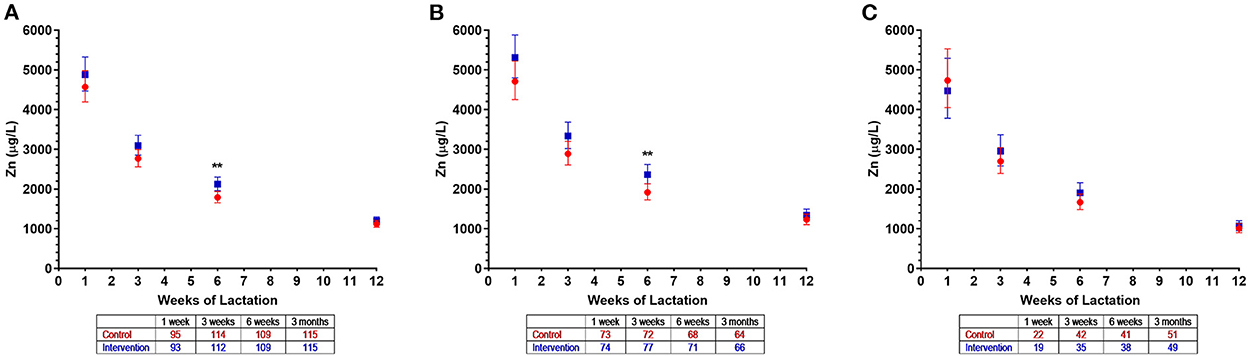
Figure 2. Zinc concentrations in human milk of control () and intervention (
) groups in the NiPPeR study during the first 3 months of lactation: (A) Overall, (B) Singapore, and (C) New Zealand. Data are the least-squares means (i.e., adjusted means) for each group, adjusted for visit, an interaction term (group*visit), study site, adherence, maternal pre-pregnancy body mass index, and gestational age at birth; error bars represent the respective 95% confidence intervals. *p < 0.01 for the difference between intervention and control groups at a given time point. The number of HM samples per group analyzed at a given time point are provided in the tables below the x axes.
As expected, for other HM minerals not differently supplemented between the groups, there were no observed differences between the intervention and control groups in the first 3 months of lactation (Figures 3A–J). The exceptions were isolated (and likely random) findings on magnesium at 1 week (Figure 3E) and sodium at 3 weeks (Figure 3J).
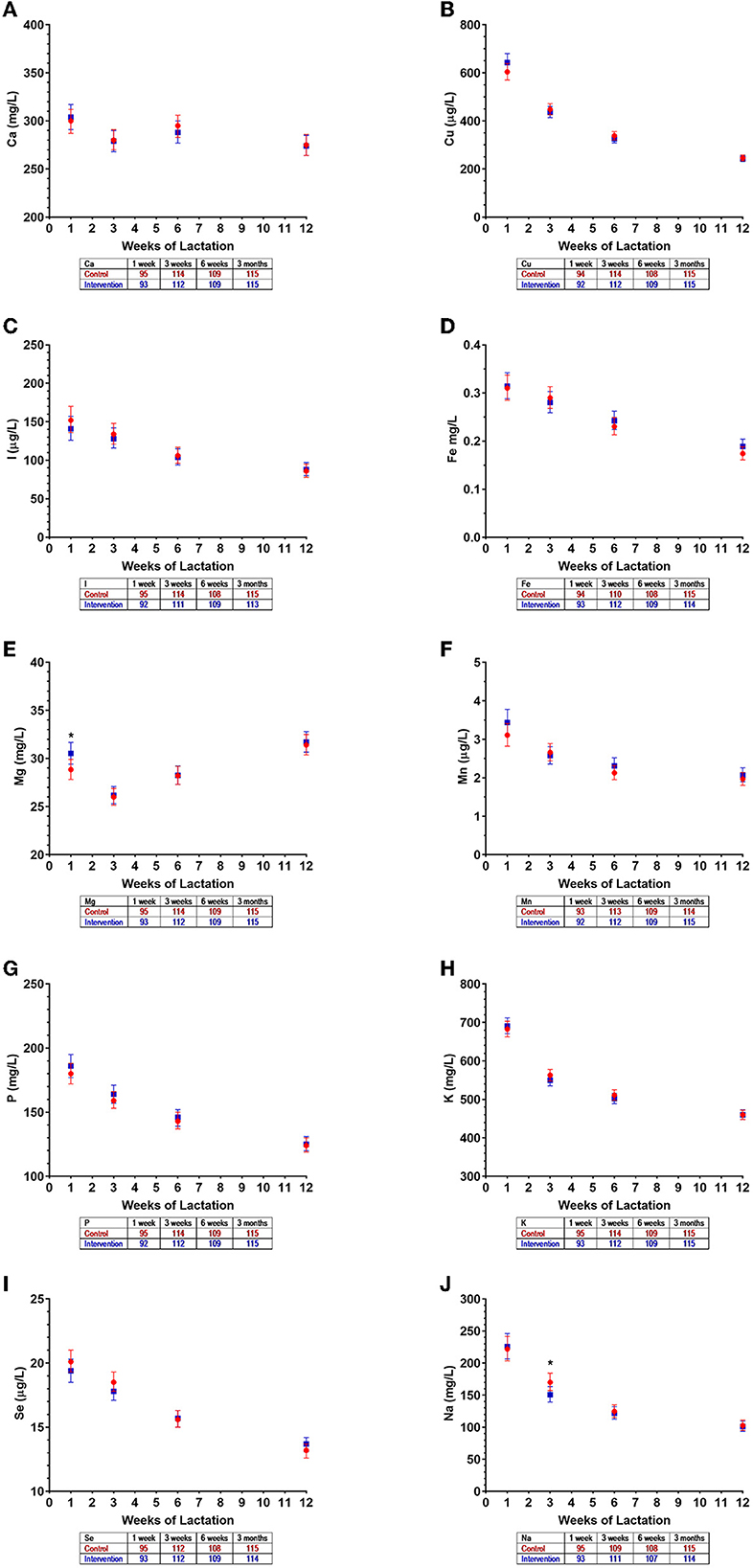
Figure 3. Mineral concentrations in human milk of control () and intervention (
) groups in the NiPPeR study during the first 3 months of lactation: (A) calcium, (B) copper, (C) iodine, (D) iron, (E) magnesium, (F) manganese, (G) phosphorus, (H) potassium, (I) selenium, and (J) sodium. Data are the least-squares means (i.e., adjusted means) for each group, adjusted for visit, an interaction term (group*visit), study site, adherence, maternal pre-pregnancy body mass index, and gestational age at birth; error bars represent the respective 95% confidence intervals. *p < 0.05 for a difference between intervention and control groups at a given time point. The number of HM samples per group analyzed at a given time point are provided in the tables below the x axes.
In analyses stratified by site, among the 158 Singapore mothers, average zinc concentrations over the first 3 months were 15% higher in the intervention group compared to controls (p = 0.015), as also observed for the TwAUC (p = 0.027; Table 6). This difference was also most evident at 6 weeks [2,364 μg/L (95% CI 2,132, 2,621) vs. 1,919 μg/L (95% CI 1,729, 2,131), respectively; p = 0.006] (Figure 2B). No differences in zinc concentrations were detected in the 180 New Zealand mothers, likely due to a smaller number of participants in the first 3 months, although a similar pattern overall was observed (Figure 2C).
3.3. Changes in minerals over time in New Zealand (0–12 months)
Zinc concentrations in HM in New Zealand decreased markedly over the first 3 months of lactation and continued to decline until 12 months (Figure 4). In both control and intervention groups, zinc concentration peaked at 1 week [4,452 μg/L (95% CI 3,659, 5,416) and 4,781 μg/L (95% CI 3,987, 5,734), respectively], with an ~4.5-fold reduction by 3 months in each group [1,057 μg/L (95% CI 930, 1,201) and 1,020 μg/L (95% CI 901, 1,156), respectively] (Figure 4). Zinc concentrations continued to decline and reached a nadir at 12 months at the end of our HM collection period [340 μg/L (95% CI 392, 295) and 365 μg/L (95% CI 423, 315), respectively] (Figure 4).
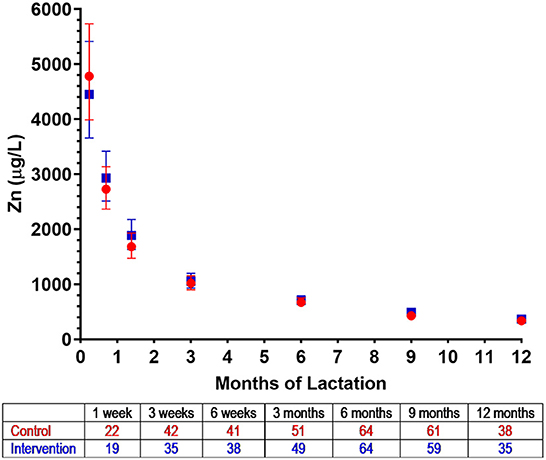
Figure 4. Zinc concentrations in human milk from control () and intervention (
) groups in New Zealand in the NiPPeR study during 12 months of lactation. Data are the least-squares means (i.e., adjusted means) for each group adjusted for visit, a group*visit interaction term, adherence, maternal pre-pregnancy body mass index, and gestational age at birth; error bars represent the respective 95% confidence intervals. The number of HM samples per group analyzed at a given time point are provided in the tables below the x axes.
For other minerals, different patterns of change were observed over time (Figure 5; Supplementary Table 6). Calcium and copper concentrations progressively decreased throughout 12 months of lactation, while iodine remained stable throughout the study period (Figure 5). Concentrations of iron, potassium, sodium, and phosphate gradually declined until 6 months, but then remained relatively constant until 12 months (Figure 5). In contrast, magnesium concentrations increased during the first 3 months of lactation but were largely unchanged thereafter (Figure 5). The concentrations of manganese and selenium fell over the initial months of lactation, with a nadir observed at 6 months, and increasing concentrations thereafter (Figure 5). The patterns of temporal changes in HM minerals were unchanged across all groups when assessed in a subset of New Zealand participants from whom HM samples were collected in at least five of the six visits between 3 weeks and 12 months (data not shown).
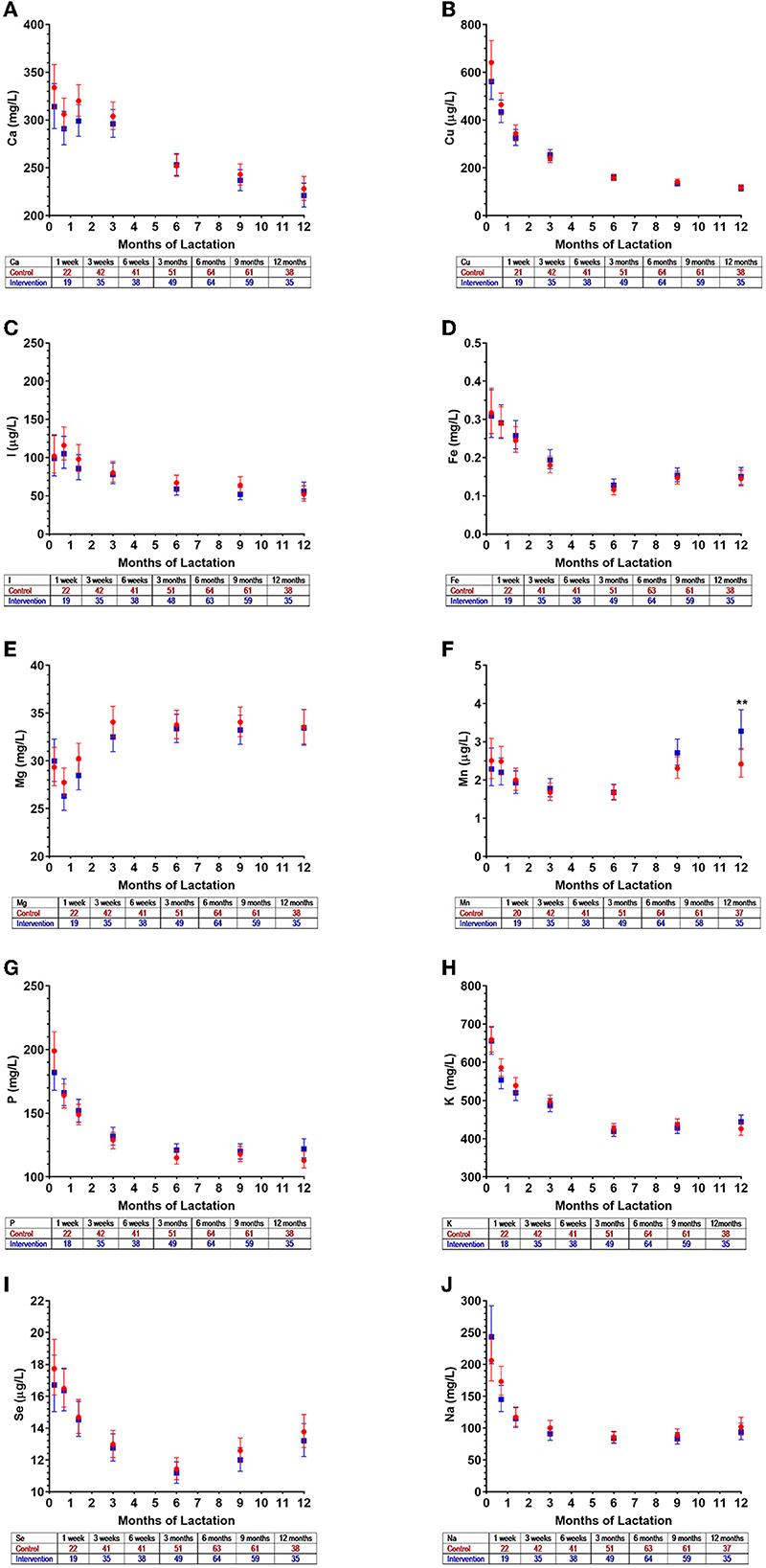
Figure 5. Mineral concentrations in human milk from control () and intervention (
) groups in New Zealand in the NiPPeR study during 12 months of lactation: (A) calcium, (B) copper, (C) iodine, (D) iron, (E) magnesium, (F) manganese, (G) phosphorus, (H) potassium, (I) selenium, and (J) sodium. Data are the least-squares means (i.e., adjusted means) for each group adjusted for visit, a group*visit interaction term, adherence, maternal pre-pregnancy body mass index, and gestational age at birth; error bars represent the respective 95% confidence intervals. **p < 0.01 for the difference between intervention and control at a given time point. The number of HM samples per group analyzed at a given time point are provided in the tables below the x axes.
4. Discussion
The present study showed that the NiPPeR intervention supplement containing zinc starting before conception and taken throughout pregnancy increased subsequent HM zinc concentrations compared to a control supplement without zinc. Overall, the effect was most evident at 6 weeks and persisted throughout the first 3 months of lactation. This effect was more evident in Singapore than in New Zealand, perhaps due to a smaller sample size early in lactation at the latter site.
To the best of our knowledge, this is the first study examining the impact of zinc supplementation prior to as well as during pregnancy on HM zinc concentrations, with most previous intervention studies beginning well into the first trimester of pregnancy. A study in Indonesian women observed no effects of zinc supplementation (30 mg/day) during pregnancy (from before 20 weeks gestation until delivery) on HM zinc concentrations at the first and sixth months of lactation, although the direction of change was similar to that observed in the present study (32). In contrast, in the current study, we demonstrated a 19% increase in HM zinc concentrations at 6 weeks of lactation as a result of zinc supplementation (10 mg/day) taken before and during pregnancy. Such effects of supplementation may not have been detected in the previous study due to lack of intervention during preconception, a smaller sample size as compared to the present study, and lack of a HM collection time point at 6 weeks where we observed the peak difference. The authors of the Indonesian study acknowledged that wide variation in the single HM sample collected at any time across the first month of lactation could have masked the intervention effect (32), as zinc concentrations dynamically change during this time as we have observed (Figure 2). Nevertheless, both studies indicate that pregnancy zinc supplementation does not influence HM concentrations at 6 months of lactation, as the pregnancy intervention effect would be expected to decline postnatally over time.
Previous studies reported no associations between maternal zinc intake or plasma zinc at the time of lactation and HM zinc concentrations (2, 33–36). It has been suggested that HM zinc concentrations are maintained through mobilization of maternal zinc pools during lactation (3). For example, previous studies have observed increased intestinal zinc absorption during lactation compared to preconception (37) or early pregnancy (38). Also, active zinc transport has been suggested to be tightly regulated in the mammary gland (3, 39). Zinc transporters (ZnTs) are localized throughout the body including the intestines, bone, and the mammary gland (40). ZnT2 and ZnT4 are predominantly found in the mammary glands. Studies have identified increased gene expression of ZnT4 in mammary glands of rodents during lactation (41–43) and ZnT2 in human breast cell lines (39, 44). In the NiPPeR intervention group, the estimated cumulative exposure of zinc was 3,416 mg, considering the prescribed dose (10 mg/day), average duration, and average adherence rate (86.9%). Zinc acquired and stored in the body during preconception and pregnancy through supplementation could have contributed to HM zinc concentrations in the intervention group. For example, ~30% of total body zinc is found in bone (11) and over the first 6 months of full lactation, 4–6% of bone mass is lost, enabling maternal bone to contribute ~20% of HM zinc (45). Hence, it can be speculated that in the NiPPeR study, zinc supplementation during preconception and pregnancy led to greater storage in bone and other maternal tissues that contributed to increased HM zinc concentrations.
In addition, we have observed higher HM zinc concentrations in the Singapore site compared to the New Zealand site. We speculate this could be due to differences in dietary patterns during pregnancy, potentially resulting in different amounts of dietary zinc stored in the body that can contribute to HM zinc during lactation.
It is unknown if other factors such as smoking during pregnancy influence HM composition. In the current study, passive smoking rates during pregnancy were higher in the control group than in the intervention group. When smoking was adjusted for in our model assessing intervention effects on HM mineral concentrations (including zinc), smoking (predominantly passive) during pregnancy was not associated with HM mineral concentrations and did not alter the overall intervention effect on HM zinc (data not shown). However, mother's smoking behavior might change after delivery and unavailability of smoking rates during lactation in the current study limits our understanding of potential effects of smoking on HM minerals. Nonetheless, previous studies reported no difference in HM zinc concentrations between smoking and non-smoking mothers (46, 47). Therefore, this is expected to have limited impact on the observed intervention effect on increased HM zinc concentrations in the current study.
HM zinc concentrations progressively decline throughout lactation, which may be due to changes in transport activity or maternal dietary intake, although the latter is less likely. Previously, Silvestre et al. (48) showed that zinc concentration in unsupplemented women was the highest in colostrum at 7,990 ± 3,230 μg/L, which decreased to 1,050 ± 710 μg/L by day 90. Similarly, Djurovic et al. (45) reported a decrease in zinc concentration from 4,700 ± 1,740 μg/L at day 1 to 460 ± 360 μg/L at 6 months. These concentrations are comparable to those in our study at the respective time points. Here, beyond 6 months, HM zinc concentrations continued to steadily decrease until 12 months of lactation, which has also been described previously (49).
While previous studies have examined HM mineral concentration only for a short period of time, ranging from few weeks to 6 months of lactation, we were able to describe patterns in HM zinc and other mineral concentrations for a longer lactation period, until 12 months. Changes in HM mineral concentrations over lactation may reflect different roles of these minerals at different stages of infancy.
It was reported previously that HM calcium concentrations increase in the first 6 weeks of lactation (50, 51). We observed that not only calcium but also magnesium increases within the first 3 months then steadily decreases until 12 months of lactation. Such higher concentrations of HM calcium and magnesium may promote bone formation in early infancy (50).
As reported previously, we also observed decreases in HM copper (49, 52, 53), iron (54, 55), phosphorus (50), potassium and sodium (55–57) over the first 6 months of lactation. Beyond this time, copper concentrations steadily decreased while iron, phosphorus, potassium, and sodium concentrations remained relatively stable until 12 months of lactation. On the other hand, iodine concentrations were reported to remain constant in the first 8 weeks of lactation (58); similarly, we observed that beyond this time point, it continued to remain unchanged throughout 12 months of lactation.
There are limited studies that have investigated changes in HM manganese and selenium concentrations over time. Both were reported to decrease in the first 4 months for manganese (49) and in the first month for selenium (52). We demonstrated similar initial falls in manganese and selenium over the initial months of lactation, with a nadir at 6 months, and increasing concentrations thereafter. How these changes in HM manganese and selenium relate to the developmental stage of the infant and their implications for infant outcomes requires further investigation.
5. Strengths and limitations
This study investigated the impact of nutritional supplementation during preconception and pregnancy on HM mineral composition during lactation. An international, multicenter design allowed the investigation of HM mineral composition in a large population of diverse ethnic groups. The Singapore and New Zealand study sites used standardized sample collection, processing, storage and mineral quantification methods, minimizing any potential variations that might have occurred during these processes. In addition, as we have tightly controlled the visit windows, so each HM collection time point was at a distinctive stage of lactation, making it possible to describe the changes in HM mineral concentration between the different stages of lactation. However, due to logistical constraints, longitudinal samples could not be collected from every participant. To address the imbalance in the number of samples at each time point, a repeated measures design was used for statistical analyses. While maternal diet and use of other supplements during lactation was not considered in this study, previous studies reported that HM zinc concentrations are not associated with dietary zinc intake (33). Therefore, other dietary sources of zinc are expected to have limited impact on HM concentrations in the current study.
6. Conclusions
This study showed that maternal supplementation of zinc from as early as preconception and pregnancy influences HM zinc concentrations during lactation. This ensures not only adequate maternal zinc status during these times but also adequate zinc transfer to the infants via HM. In the future, ongoing evaluation of offspring from this cohort will help to understand the associations between HM zinc concentrations and both short- and long-term offspring outcomes.
Data availability statement
The datasets presented in this article are not readily available because public sharing of the data was not part of the original participant informed consent. Requests to access the datasets should be directed to WC, dy5jdXRmaWVsZEBhdWNrbGFuZC5hYy5ueg==.
Ethics statement
The studies involving human participants were reviewed and approved by the study was conducted in Southampton (UK), Singapore, and Auckland (New Zealand), with ethics approval obtained at each site [Southampton—Health Research Authority National Research Ethics Service Committee South Central Research Ethics Committee (15/SC/0142); Singapore—the National Healthcare Group Domain Specific Review Board (2015/00205); and New Zealand—Northern A Health and Disability Ethics Committee (15/NTA/21)]. All participants provided written informed consent. The patients/participants provided their written informed consent to participate in this study.
NiPPeR Study Group
The NiPPeR Study Group authors for the Frontiers in Nutrition citation comprises:
• Benjamin B Albert (Yi5hbGJlcnRAYXVja2xhbmQuYWMubno=),
• Shelia J Barton (Uy5KLkJhcnRvbkBzb3Rvbi5hYy51aw==),
• Mary Cavanagh (bS5jYXZhbmFnaEBhdWNrbGFuZC5hYy5ueg==),
• Hsin Fang Chang (aHNpbl9GYW5nX0NoYW5nQG51aHMuZWR1LnNn),
• Yap Seng Chong (eWFwX3NlbmdfY2hvbmdAbnVocy5lZHUuc2c=),
• Mary F Chong (bWFyeV9jaG9uZ0BudXMuZWR1LnNn),
• Cathryn Conlon (Qy5Db25sb25AbWFzc2V5LmFjLm56),
• Cyrus Cooper (Y2NAbXJjLnNvdG9uLmFjLnVr),
• Paula Costello (cGNAbXJjLnNvdG9uLmFjLnVr),
• Vanessa Cox (dmFjQG1yYy5zb3Rvbi5hYy51aw==),
• Christine Creagh (Y2hyaXN0aW5lLmNyZWFnaEBhdWNrbGFuZC5hYy5ueg==),
• Marysia Depczynski (bS5kZXBjenluc2tpQGF1Y2tsYW5kLmFjLm56),
• Sarah El-Heis (c2VAbXJjLnNvdG9uLmFjLnVr),
• Judith Hammond (ai5oYW1tb25kQGF1Y2tsYW5kLmFjLm56),
• Nicholas C Harvey (bmNoQG1yYy5zb3Rvbi5hYy51aw==),
• Mrunalini Jagtap (bXJ1bmFsaW5pX2phZ3RhcEBzaWNzLmEtc3Rhci5lZHUuc2c=),
• Timothy Kenealy (dC5rZW5lYWx5QGF1Y2tsYW5kLmFjLm56),
• Heidi Nield (aG5AbXJjLnNvdG9uLmFjLnVr),
• Justin M O'Sullivan (anVzdGluLm9zdWxsaXZhbkBhdWNrbGFuZC5hYy5ueg==),
• Gernalia Satianegara (Z2VybmFsaWFfc2F0aWFuZWdhcmFAc2ljcy5hLXN0YXIuZWR1LnNn),
• Irma Silva-Zolezzi (SXJtYS5TaWx2YVpvbGV6emlAcmRscy5uZXN0bGUuY29t),
• Shu E Soh (c2h1X2Vfc29oQG51aHMuZWR1LnNn),
• Vicky Tay (Vmlja3lfdGF5QHNpY3MuYS1zdGFyLmVkdS5zZw==),
• Rachael Taylor (cmFjaGFlbC50YXlsb3JAb3RhZ28uYWMubno=),
• Elizabeth Tham (ZWxpemFiZXRoX3RoYW1AbnVocy5lZHUuc2c=),
• Philip Titcombe (cHQ2ZzEzQHNvdG9uLmFjLnVr),
• Clare Wall (Yy53YWxsQGF1Y2tsYW5kLmFjLm56),
• Ray Wong (cmF5X3dvbmdAc2ljcy5hLXN0YXIuZWR1LnNn),
• Gladys Woon (Z2xhZHlzX3dvb25AbnVocy5lZHUuc2c=).
Author contributions
KMG, S-YC, and WSC led the design of the original study. The present sub-study was developed and undertaken by SMH, SDe, JGBD, MHV, FH, SKT, and WSC. SDu supervised the laboratory analyses. SMH and JGBD performed the statistical analyses. SMH led the manuscript writing. SDe contributed to sections of the manuscript. SKT and WSC supervised all aspects of the present study. All authors contributed to interpretation, manuscript revision, read, and approved the final version.
Funding
Public good funding for this investigator-led study is through the Medical Research Council (UK) (MRC) as part of an MRC award to the MRC Lifecourse Epidemiology Unit (MC_UU_12011/4), the Singapore National Research Foundation, the National Medical Research Council (SG) (NMRC) (NMRC/TCR/012-NUHS/2014), the National University of Singapore (NUS), the Agency for Science, Technology and Research (SG) as part of the Growth, Development and Metabolism Programme of the Singapore Institute for Clinical Sciences (H17/01/a0/005), and as part of Gravida, a New Zealand Government Center of Research Excellence. Funding for provision of the intervention and control drinks and to cover aspects of the fieldwork for the study was provided by Société des Produits Nestlé SA under a research agreement with the University of Southampton, Auckland UniServices Ltd., Singapore Institute for Clinical Sciences, National University Hospital Singapore PTE Ltd., and NUS. KMG is supported by the UK Medical Research Council (MC_UU_12011/4), the National Institute for Health Research [NIHR Senior Investigator (NF-SI-0515-10042), NIHR Southampton 1000DaysPlus Global Nutrition Research Group (17/63/154) and NIHR Southampton Biomedical Research Center (IS-BRC-1215-20004)], the European Union (Erasmus+ Programme ImpENSA 598488-EPP-1-2018-1-DE-EPPKA2-CBHE-JP) and the British Heart Foundation (RG/15/17/3174, SP/F/21/150013). For the purpose of Open Access, the author has applied a Creative Commons Attribution (CC BY) license to any Author Accepted Manuscript version arising from this submission.
Acknowledgments
SMH is currently receiving University of Auckland Doctoral Scholarship. The authors thank the participants and their families for their enthusiastic involvement in the study, the study research staff and hospital clinical staff at participating centers, and operational support staff for contributions to the trial, and the members of the independent data monitoring and safety committee for invaluable contributions and for overseeing the conduct of the trial.
Conflict of interest
KMG, S-YC, and WSC are part of an academic consortium that has received grants from Société des Produits Nestlé SA relating to the submitted work, and from Abbott Nutrition, Danone, and BenevolentAI Bio Ltd. outside the submitted work, and are co-inventors on patent filings by Société des Produits Nestlé SA relating to the NiPPeR intervention or its components. FH, SDe, SDu, and SKT are employees of Société des Produits Nestlé SA.
The remaining authors declare that the research was conducted in the absence of any commercial or financial relationships that could be construed as a potential conflict of interest.
Publisher's note
All claims expressed in this article are solely those of the authors and do not necessarily represent those of their affiliated organizations, or those of the publisher, the editors and the reviewers. Any product that may be evaluated in this article, or claim that may be made by its manufacturer, is not guaranteed or endorsed by the publisher.
Supplementary material
The Supplementary Material for this article can be found online at: https://www.frontiersin.org/articles/10.3389/fnut.2022.1034828/full#supplementary-material
References
1. Khayat S, Fanaei H, Ghanbarzehi A. Minerals in pregnancy and lactation: a review article. J Clin Diagn Res. (2017) 11:QE01–5. doi: 10.7860/JCDR/2017/28485.10626
2. Dror DK, Allen LH. Overview of nutrients in humanmilk. Adv Nutr. (2018) 9:278S–94S. doi: 10.1093/advances/nmy022
3. Donangelo CM, King JC. Maternal zinc intakes and homeostatic adjustments during pregnancy and lactation. Nutrients. (2012) 4:782–98. doi: 10.3390/nu4070782
4. Department of Health. Dietary Reference Values: A Guide. London: HMSO Publications Centre (1991).
5. Dietetics Department NUH. Vitamins and Minerals Chart. Singapore: National University Hospital (2006).
6. National Health and Medical Research Council Australian Australian Government Department of Health and Ageing New New Zealand Ministry of Health. Nutrient Reference Values for Australia and New Zealand Including Recommended Dietary Intakes. Canberra: National Health and Medical Research Council (2006).
7. Caulfield LE, Zavaleta N, Shankar AH, Merialdi M. Potential contribution of maternal zinc supplementation during pregnancy to maternal and child survival. Am J Clin Nutr. (1998) 68(2 SUPPL):499S−508S.
8. Berhe K, Kidanemariam A, Gebremariam G, Gebremariam A. Prevalence and associated factors of adolescent undernutrition in Ethiopia: a systematic review and meta-analysis. BMC Nutr. (2019) 5:49. doi: 10.1186/s40795-019-0309-4
9. University of Otago and Ministry of Health. A Focus on Nutrition: Key Findings of the 2008/09 New Zealand Adult Nutrition Survey. Otago: University of Otago and Ministry of Health (2011). p. 360.
10. Lai JS, Cai S, Feng L, Shek LP, Yap F, Tan KH, et al. Associations of maternal zinc and magnesium with offspring learning abilities and cognitive development at 4 years in GUSTO. Nutr Neurosci. (2021) 24:467–76. doi: 10.1080/1028415X.2019.1643624
11. King JC. Determinants of maternal zinc status during pregnancy. Am J Clin Nutr. (2000) 71:1334S–43S. doi: 10.1093/ajcn/71.5.1334s
12. Ota E, Mori R, Middleton P, Tobe-Gai R, Mahomed K, Miyazaki C, et al. Zinc supplementation for improving pregnancy and infant outcome. Cochrane Database Syst Rev. (2015) 2015:2. doi: 10.1002/14651858.CD000230.pub5
13. Goldenberg RL, Tamura T, Neggers Y, Copper RL, Johnston KE, DuBard MB, et al. The effect of zinc supplementation on pregnancy outcome. JAMA. (1995) 274:463–8.
14. Hess SY, King JC. Effects of maternal zinc supplementation on pregnancy and lactation outcomes. Food Nutr Bull. (2009) 30(1 SUPPL):60–78. doi: 10.1177/15648265090301S105
15. Lönnerdal B, Hoffman B, Hurley LS. Zinc and copper binding proteins in human milk. Am J Clin Nutr. (1982) 36:1170–6.
16. Ackland ML, Michalczyk AA. Zinc and infant nutrition. Arch Biochem Biophys. (2016) 611:51–7. doi: 10.1016/j.abb.2016.06.011
17. Friel JK, Andrews WL, Matthew JD, Long DR, Cornel AM, Cox M, et al. Zinc supplementation in very-low-birth-weight infants. J Pediatr Gastroenterol Nutr. (1993) 17:97–104.
18. Umeta M, West CE, Haidar J, Deurenberg P, Hautvast JGAJ. Zinc supplementation and stunted infants in Ethiopia: a randomised controlled trial. Lancet. (2000) 355:2021–6. doi: 10.1016/S0140-6736(00)02348-5
19. Black RE. Trace element undernutrition: biology to interventions. Zinc deficiency, infectious disease and mortality in the developing world. Am Soc Nutr Sci. (2003) 3:1485–9. doi: 10.1093/jn/133.5.1485S
20. Terrin G, Canani RB, Passariello A, Messina F, Conti MG, Caoci S, et al. Zinc supplementation reduces morbidity and mortality in very-lowbirth-weight preterm neonates: a hospital-based randomized, placebo-controlled trial in an industrialized country. Am J Clin Nutr. (2013) 98:1468–74. doi: 10.3945/ajcn.112.054478
21. Bhatnagar S, Taneja S. Zinc and cognitive development. Br J Nutr. (2001) 85:S139–45. doi: 10.1079/BJN2000306
22. Godfrey KM, Cutfield W, Chan SY, Baker PN, Chong YS, Aris IBM, et al. Nutritional intervention preconception and during pregnancy to maintain healthy glucose metabolism and offspring health (“NiPPeR”): study protocol for a randomised controlled trial. Trials. (2017) 18:131. doi: 10.1186/s13063-017-1875-x
23. Kumar A, Kaur S. Calcium: a nutrient in pregnancy. J Obstet Gynecol India. (2017) 67:313–8. doi: 10.1007/s13224-017-1007-2
24. Harding KB, Peña-Rosas JP, Webster AC, Yap CMY, Payne BA, Ota E, et al. Iodine supplementation for women during the preconception, pregnancy and postpartum period. Cochrane Database Syst Rev. (2017) 2017:3. doi: 10.1002/14651858.CD011761.pub2
25. Zimmermann MB. The effects of iodine deficiency in pregnancy and infancy. Paediatr Perinat Epidemiol. (2012) 26(SUPPL 1):108–17. doi: 10.1111/j.1365-3016.2012.01275.x
26. Scholl TO, Reilly T. Symposium: improving adolescent iron status anemia, iron and pregnancy outcome. J Nutr. (2000) 1991:443–7. doi: 10.1093/jn/130.2.443S
27. Godfrey KM, Barton SJ, El-Heis S, Kenealy T, Nield H, Baker PN, et al. Myo-inositol, probiotics, and micronutrient supplementation from preconception for glycemia in pregnancy: NiPPeR international multicenter double-blind randomized controlled trial. Diabetes Care. (2021) 44:1091–9. doi: 10.2337/figshare.13874705.v1
28. Rodushkin I, Ödman F, Branth S. Multielement analysis of whole blood by high resolution inductively coupled plasma mass spectrometry. Fresenius J Anal Chem. (1999) 364:338–46.
29. Rodushkin I, Odman OF, Olofsson R, Axelsson MD. Determination of 60 elements in whole blood by sector field inductively coupled plasma mass spectrometry. J Anal At Spectrom. (2000) 15:937–44. doi: 10.1039/B003561K
30. Engström E, Stenberg A, Senioukh S, Edelbro R, Baxter DC, Rodushkin I. Multi-elemental characterization of soft biological tissues by inductively coupled plasma-sector field mass spectrometry. Anal Chim Acta. (2004) 521:123–35. doi: 10.1016/j.aca.2004.06.030
31. Krachler M, Heisel C, Philippe Kretzer J. Validation of ultratrace analysis of Co, Cr, Mo, and Ni in whole blood, serum and urine using ICP-SMS. J Anal At Spectrom. (2009) 24:605–10. doi: 10.1039/b821913c
32. Dijkhuizen MA, Wieringa FT, West CE. Zinc plus β-carotene supplementation of pregnant women is superior to β-carotene supplementation alone in improving vitamin A status in both mothers and infants. Am J Clin Nutr. (2004) 80:1299–307. doi: 10.1093/ajcn/80.5.1299
33. Aumeistere L, Ciproviča I, Zavadska D, Bavrins K, Borisova A. Zinc content in breast milk and its association with maternal diet. Nutrients. (2018) 10:1438. doi: 10.3390/nu10101438
34. Hannan MA, Faraji B, Tanguma J, Longoria N, Rodriguez RC. Maternal milk concentration of zinc, iron, selenium, and iodine and its relationship to dietary intakes. Biol Trace Elem Res. (2009) 127:6–15. doi: 10.1007/s12011-008-8221-9
35. Domellöf M, Lönnerdal B, Dewey KG, Cohen RJ, Hernell O. Iron, zinc, and copper concentrations in breast milk are independent of maternal mineral status. Am J Clin Nutr. (2004) 79:111–5. doi: 10.1093/ajcn/79.1.111
36. Moser PB, Reynolds RD. Dietary zinc intake and zinc concentrations of plasma, erythrocytes, and breast milk in antepartum and postpartum lactating and nonlactating women: a longitudinal study. Am J Clin Nutr. (1983) 38:101–8.
37. Fung EB, Ritchie LD, Woodhouse LR, Roehl R, King JC. Zinc absorption in women during pregnancy and lactation: a longitudinal study. Am J Clin Nutr. (1997) 66:80–8.
38. Donangelo CM, Zapata CLV, Woodhouse LR, Shames DM, Mukherjea R, King JC. Zinc absorption and kinetics during pregnancy and lactation in Brazilian women. Am J Clin Nutr. (2005) 82:118–24. doi: 10.1093/ajcn/82.1.118
39. Kelleher SL, Seo YA, Lopez V. Mammary gland zinc metabolism: regulation and dysregulation. Genes Nutr. (2009) 4:83–94. doi: 10.1007/s12263-009-0119-4
40. Huang L, Tepaamorndech S. The SLC30 family of zinc transporters: a review of current understanding of their biological and pathophysiological roles. Mol Aspects Med. (2013) 34:548–60. doi: 10.1016/j.mam.2012.05.008
41. Liuzzi JP, Bobo JA, Cui L, McMahon RJ, Cousins RJ. Zinc transporters 1, 2 and 4 are differentially expressed and localized in rats during pregnancy and lactation. J Nutr. (2003) 133:342–51. doi: 10.1093/jn/133.2.342
42. Kelleher SL, Lönnerdal B. Zn transporter levels and localization change throughout lactation in rat mammary gland and are regulated by Zn in mammary cells. J Nutr. (2003) 133:3378–85. doi: 10.1093/jn/133.11.3378
43. Huang L, Gitschier J. A novel gene involved in zinc transport is deficient in the lethal milk mouse. Nat Genet. (1997) 17:292–7. doi: 10.1038/ng1197-292
44. Chowanadisai W, Lönnerdal B, Kelleher SL. Identification of a mutation in SLC30A2 (ZnT-2) in women with low milk zinc concentration that results in transient neonatal zinc deficiency. J Biol Chem. (2006) 281:39699–707. doi: 10.1074/jbc.M605821200
45. Moser-Veillon PB. Zinc needs and homeostasis during lactation. Analyst. (1995) 120:895–7. doi: 10.1039/an9952000895
46. Szukalska M, Merritt TA, Lorenc W, Sroczyńska K, Miechowicz I, Komorowicz I, et al. Toxic metals in human milk in relation to tobacco smoke exposure. Environ Res. (2021) 197:111090. doi: 10.1016/j.envres.2021.111090
47. Berlanga M del R, Salazar G, Garcia C, Hernandez J. Maternal smoking effects on infant growth. Food Nutr Bull. (2002) 23(3 Suppl):142–5.
48. Silvestre D, Martìnez-Costa C, Lagarda MJ, Brines J, Farré R, Clemente G. Copper, iron, and zinc contents in human milk during the first 3 months of lactation: a longitudinal study. Biol Trace Elem Res. (2001) 80:1–11. doi: 10.1385/BTER:80:1:01
49. Casey CE, Neville MC, Hambidge KM. Studies in human lactation: secretion of zinc, copper, and manganese in human milk. Am J Clin Nutr. (1989) 49:773–85. doi: 10.1093/ajcn/49.5.773
50. Greer FR, Tsang RC, Levin RS, Searcy JE, Wu R, Steichen JJ. Increasing serum calcium and magnesium concentrations in breast-fed infants: longitudinal studies of minerals in human milk and in sera of nursing mothers and their infants. J Pediatr. (1982) 100:59–64. doi: 10.1016/s0022-3476(82)80235-7
51. Kent JC, Arthur PG, Retallack RW, Hartmann PE. Calcium, phosphate and citrate in human milk at initiation of lactation. J Dairy Res. (1992) 59:161–7. doi: 10.1017/s0022029900030405
52. Wasowicz W, Gromadzinska J, Szram K, Rydzynski K, Cieslak J, Pietrzak Z. Selenium, zinc, and copper concentrations in the blood and milk of lactating women. Biol Trace Elem Res. (2001) 79:221–33. doi: 10.1385/BTER:79:3:221
53. Nagra SA. Longitudinal study in biochemical composition of human milk during first year of lactation. J Trop Pediatr. (1989) 35:126–8. doi: 10.1093/tropej/35.3.126
54. Shashiraj F, Singh O, Rusia U. Mother's iron status, breastmilk iron and lactoferrin: are they related? Eur J Clin Nutr. (2006) 60:903–8. doi: 10.1038/sj.ejcn.1602398
55. Lemons JA, Moye L, Hall D, Simmons M. Differences in the composition of preterm and term human milk during early lactation. Pediatr Res. (1982) 16:113–7. doi: 10.1203/00006450-198202000-00007
56. Wack RP, Lien EL, Taft D, Roscelli JD. Electrolyte composition of human breast milk beyond the early postpartum period. Nutrition. (1997) 13:774–7. doi: 10.1016/s0899-9007(97)00187-1
57. Mastroeni SSBS, Okada IA, Rondó PHC, Duran MC, Paiva AA, Neto JM. Concentrations of Fe, K, Na, Ca, P, Zn, and Mg in maternal colostrum and mature milk. J Trop Pediatr. (2006) 52:272–5. doi: 10.1093/tropej/fmk004
58. Sabatier M, Garcia-Rodenas CL, De Castro CA, Kastenmayer P, Vigo M, Dubascoux S, et al. Longitudinal changes of mineral concentrations in preterm and term human milk from lactating swiss women. Nutrients. (2019) 11:1855. doi: 10.3390/nu11081855
59. International International Association of Diabetes and Pregnancy Study Groups Consensus Panel, Metzger BE, Gabbe SG, Persson B, Buchanan TA, Catalano PA, et al. International Association of Diabetes and Pregnancy Study Groups recommendations on the diagnosis and classification of hyperglycemia in pregnancy. Diabetes Care. (2010) 33:676–82. doi: 10.2337/dc09-1848
Keywords: human milk, minerals, pregnancy, supplement, zinc
Citation: Han SM, Devaraj S, Derraik JGB, Vickers MH, Huang F, Dubascoux S, Godfrey KM, Chan S-Y, Pang WW, Thakkar SK, Cutfield WS and NiPPeR Study Group (2023) A nutritional supplement containing zinc during preconception and pregnancy increases human milk zinc concentrations. Front. Nutr. 9:1034828. doi: 10.3389/fnut.2022.1034828
Received: 02 September 2022; Accepted: 12 December 2022;
Published: 10 January 2023.
Edited by:
Hector Gonzalez-Iglesias, Spanish National Research Council (IPLA-CSIC), SpainReviewed by:
María Luisa Fernández-Sanchez, University of Oviedo, SpainHiten D. Mistry, King's College London, United Kingdom
Copyright © 2023 Han, Devaraj, Derraik, Vickers, Huang, Dubascoux, Godfrey, Chan, Pang, Thakkar, Cutfield and NiPPeR Study Group. This is an open-access article distributed under the terms of the Creative Commons Attribution License (CC BY). The use, distribution or reproduction in other forums is permitted, provided the original author(s) and the copyright owner(s) are credited and that the original publication in this journal is cited, in accordance with accepted academic practice. No use, distribution or reproduction is permitted which does not comply with these terms.
*Correspondence: Wayne S. Cutfield, dy5jdXRmaWVsZEBhdWNrbGFuZC5hYy5ueg==
†These authors share first authorship
‡These authors share last authorship