- Key Laboratory of Animal Genetics and Breeding and Molecular Design of Jiangsu Province, Yangzhou University, Yangzhou, China
Linseed oil, an important source of dietary α-linolenic acid, is used to provide meat enriched in n-3 PUFA. We investigated the effects of dietary linseed oil (0, 0.5, 1, and 2%) on growth performance, meat quality, tissue fatty acid (FA), and transcriptome profiles in ducks. The result showed that dietary linseed oil had no effect on growth performance. Increasing dietary linseed oil enrichment raised n-3 PUFA and linoleic acid (LA) levels in both the liver and breast muscle, but decreased dihomo-gamma-linolenic acid (DGLA) and arachidonic acid (ARA) levels in the liver. The liver n-3 PUFA content was negatively correlated with duck body weight. Transcriptome analysis showed that dietary linseed oil caused hepatic changes in genes (SCD, FADS1, FADS2, and ACOT6) related to the biosynthesis of unsaturated fatty acids. Besides, dietary linseed oil also affected the expression of genes related to PUFAs and downstream metabolites (such as linoleic acid, steroid hormone, progesterone, etc.) metabolic pathways in both liver and breast muscle. Key genes involved in PUFA synthesis and transport pathways were examined by RT-qPCR, and the results verified that hepatic expression levels of FADS1 and FADS2 decreased, and those of FABP4 and FABP5 increased when 2% linseed oil was added. CD36 expression level increased in breast muscle when 2% linseed oil was added. Thus, 2% dietary linseed oil supplementation produces n-3 PUFA-enriched duck products by regulating the PUFA metabolic pathways, which could be advantageous for health-conscious consumers.
Introduction
Polyunsaturated fatty acids (PUFAs), classified into n-3 and n-6 PUFAs, influence growth, development, reproduction, and vulnerability to diseases. Linoleic acid (LA), dihomo-gamma-linolenic acid (DGLA), and arachidonic acid (ARA) are n-6 PUFAs, whereas α-linolenic acid (ALA), eicosapentaenoic acid (EPA), and docosahexaenoic acid (DHA) belong to n-3 PUFAs (1). Both types of fatty acids (FAs) are precursors of signaling molecules with opposing effects that regulate membrane microdomain composition, receptor signaling, and gene expression (2). LA and ALA are derived from lipids of vegetable origin and are considered essential because they cannot be synthesized de novo in vertebrates. The conversion of the n-6 fatty acid LA to ARA plays a role in inducing inflammation, adipogenesis, and regulation of the endocannabinoid system (3). n-3 PUFAs decrease inflammatory signaling and lipid synthesis and stimulate fatty acid degradation (4). In humans, the optimal n-6:n-3 PUFA ratio is 5–10, whereas in popular modern western diets, the ratio is 15–16.7 (5). Excessive intake of n-6 PUFA and a very high n-6/n-3 PUFA ratio in the food increase the risk of obesity, cardiovascular diseases, and cancer (3, 6). It is necessary to improve human nutrition by supplying n-3 PUFA enriched foods.
Duck meat is popular in both Asian and European countries. Compared to chicken meat, ducks have a higher content of red muscle fibers in breast muscles (7). While the n-6: n-3 PUFA ratio in duck is about 15–30 under a regular diet pattern, which does not meet human optimal health requirements. ALA, the plant omega-3, is enriched in flaxseed, perilla, echium, walnut, and algal oil (8), and fish oils that contain high contents of EPA and DHA are typically the most abundantly available (9). Therefore, it is important to consider the quality of added fats in duck diet, and duck meat could be a viable source of n-3 PUFAs for humans. On the other hand, ducks have a high ability to efficiently convert dietary ALA to DHA (10). The conversion occurs through fatty acid desaturases (encoded by FADS1 and FADS2) and elongases (encoded by ELOVL5 and ELOVL2) in the liver (11) and is subsequently supplied (via blood circulation) to other tissues (12). Long-chain fatty acid (LCFA) uptake occurs not only through free diffusion across the plasma membrane, but also by a protein-mediated mechanism (13). Their translocation depends on fatty acid transporters, such as fatty acid transport proteins/solute carrier 27A (FATP/SLC27A), fatty acid translocase/cluster of differentiation 36 (FAT/CD36), caveolin-1 (CAV1), and fatty acid binding protein (FABP) (13). Although PUFA composition in ducks has been studied, the mechanism of PUFA synthesis and transport in ducks has not been fully elucidated.
Recently, linseed oil has been incorporated into foods to improve the cardiovascular health of humans (14). Considering the high cost of fish oil, linseed oil is also an ideal source of n-3 PUFA in animal diets. In poultry, linseed oil or flaxseed-supplemented diets increase n-3 PUFA levels and decrease n-6:n-3 PUFA ratios in both eggs and meat, providing products enriched in n-3 PUFA, for which many positive health effects have been described (15–17). However, little attention has been paid to the combined effect of diets formulated with linseed oil on gene transcription in ducks, as well as to their effects on polyunsaturated fatty acid metabolism in ducks. Therefore, in this study, Runzhou white-crested ducks, a popular duck breed with a small body size, were used to assess the effect of a linseed-oil-enriched diet on the performance, meat quality, genes transcriptome and lipid metabolism. These insights might provide feasible method in producing n-3 PUFA enriched ducks, and key genes involved in PUFA synthesis and transport pathways were evaluated in linseed oil-administered ducks.
Materials and methods
Experimental design and diets
A total of 224 28-day old Runzhou white-crested ducks of the commodity generation (average weight: 753.35 ± 12.94 g) were randomly divided into four treatment groups: control (basal diet + 2% duck oil), low linseed oil (basal diet + 1.5% duck oil + 0.5% linseed oil), medium linseed oil (basal diet + 1% duck oil + 1% linseed oil), and high linseed oil (basal diet + 2% linseed oil). Each treatment group had four replicates, each consisting of 14 ducks (half male and half female). The ducks from one batch were fed the same diet at 1–28 days of age. The four treatment diets were formulated by adding different proportions of duck oil and linseed oil (Table 1). Duck oil was gradually replaced with linseed oil to achieve a higher ALA content and lower n-6/n-3 PUFA ratio. The fatty acid composition of the experimental diets and oils are presented in Table 2. The ALA content in linseed oil was 35.21%, and with an increase in linseed oil, the ALA level increased gradually from 0.0766 to 0.6816 g/100 g, and the n-6/n-3 PUFA ratio decreased gradually from 17.37 to 1.97, as expected. The birds were raised in deep litter comprising sawdust and paddy husks. All ducks had ad libitum access to food and water. Considering the previous research (17) and the characteristics of small size meat ducks, the feeding trial lasted for 28 days. None of the ducks died during the 28 days experiment. All ducks were provided by Zhenjiang Tiancheng Agricultural Technology, Jiangsu Province, where the experiments were also conducted.
Production performance and sample collection
Following a 12-h fast, the duck body weight (BW), and feed intake (28–56 days of age) were recorded, and average daily feed intake (ADFI), average daily weight gain (ADG), and average feed/gain (F/G) were calculated. Six ducks from each replicate (three male and three female) were randomly selected and killed by stunning at the end of the experiment. Four small portions of the right breast muscle and right liver were collected, immediately placed in liquid N2, and stored at –80°C for fatty acid and gene expression analyses. A large portion of the left breast muscle was collected and stored at –80°C.
Meat quality indexes
At the time of debonding (24 h post-mortem), the whole left breast muscle was used to determine physical properties (meat color, pH values, water loss rate, and shearing force values) analysis of meat. The rest of the left breast muscle was frozen at –20°C.
Meat color was measured by the optical reflection method using the CIELAB system (CIE, 1978) with L* (lightness), a* (redness), and b* (yellowness) measured using a colorimeter (CR-400, Konica Minolta, Japan). Color measurements were taken on the medial surface of each left breast or thigh and averaged. The pH of the upper, middle, and lower portions of the left breast muscles was determined in triplicate using a glass electronic pH meter (DELTA 320; Mettler Toledo, Switzerland) inserted 10 mm into the breast muscles.
After cooking in a water bath (20 min at 80°C), the breast filets were cooled down, and at least three cubes of approximately 30 g were cut from the breast filets. The water-holding capacity was determined using a hydraulic machine (M10, Bulader, Beijing, China) equipped with a 35 kg load for 5 min. Water loss rate was calculated by one minus the water-holding capacity. The water-holding capacity. At least three cylinders (3 cm in length, 1 cm in width, and 1 cm in height) were cut from the samples. The cylinders were then sheared perpendicular to the longitudinal route of the fibers loaded with a maximum 500-N load cell and a crosshead speed of 200 mm/min using a tenderness meter (C-LM3, Tenovo, Beijing, China). Subsequently, the shear forces of the cylinders were recorded. A FoodScan Meat Product Analyzer (Foss, Denmark) was used for chemical composition detection (18). After the removing of membranes, tendons, and visible fat were removed, the meat was homogenized and prepared for moisture, protein, intramuscular fat (IMF), and collagen analyses. Measurements were taken using a mixture of three individual ducks from the same replicate group, and the analysis was repeated three times.
Fatty acid analysis
For FA analysis, approximately 100 mg of feed, liver, or breast muscle was weighed and hydrolyzed, extracted, saponified, and methylated. The FA methyl esters were subjected to Trace1310-ISQ gas chromatography and mass spectrometry (Thermos, Waltham, MA, USA) using an HP-88 column (100 m × 0.25 mm × 0.20 μm, Agilent, Santa Clara, CA, USA) for fatty acid separation. The column oven temperature was maintained at 100°C for 15 min after sample injection and was programmed to increase from 100 to 190°C at 15°C/min and maintained at 190°C for 25 min. Then the temperature was increased to 235°C at a rate of 2.5°C/min and held for 4 min. The injector and detector temperatures were 240 and 280°C, respectively. Helium was used as the carrier gas at a flow rate of 1.0 ml/min. Fatty acids were identified and quantified by comparing the retention times and peak areas with those of standard components (Sigma, Louis, MO, USA). The results were recorded as a percentage of fresh feed or breast muscle.
Transcriptome analysis
Total RNA of liver and breast muscle samples was extracted by a Trizol reagent (Invitrogen, Carlsbad, CA, USA) according to the manufacturer’s protocol. mRNA was purified from total RNA using poly-T oligo-attached magnetic beads. And then the mRNA was randomly segmented into small fragments by divalent cations in a fragmentation buffer. First strand cDNA was synthesized using random hexamer primer. Second strand cDNA synthesis was subsequently performed using DNA Polymerase I and RNase H. The cDNA fragments were purified with AMPure XP system (Beckman Coulter, Beverly, MA, USA), and then connected with sequencing adapters according to an Illumina protocol (San Diego, CA, USA). After quality assessment, the target fragments of 250–300 bp were selected for PCR amplification to create the final cDNA library. The sequencing and analysis were performed by the Novogene Bioinformatics Technology Co., Ltd.
Clean reads were obtained by removing reads containing adapter, reads containing ploy-N and low quality reads from raw data. All samples had a Q20 (bases of Q ≥ 20/all bases of sequencing) of >96%. Clean data were mapped to the duck reference genome1 by Hisat2 (version: 2.0.5). mRNA levels was quantified by the value of fragments per kilobase of exon per million mapped reads (FPKM). Differential expression analysis of the high linseed oil group vs. control group was performed using the DESeq2 R package (version 1.24.0) (19). Genes with FDR (adjusted p-value) less than 0.05 and absolute log2-fold change more than 1 were assigned as DEGs.
Gene Ontology (GO) enrichment analysis of differentially expressed genes was implemented by the clusterProfiler R package. GO terms with P < 0.05 were considered significantly enriched by differential expressed genes. Kyoto Encyclopedia of Genes and Genomes (KEGG)2 is a database resource for pathway analysis. ClusterProfiler R package is used to test the statistical enrichment of differential expression genes in KEGG pathways.
Gene expression validation using RT-qPCR
Total RNA was extracted from breast muscle using TRIzol reagent (Takara, Osaka, Janpan). Extracted RNA was quantified and reverse-transcribed into cDNA using PrimeScript RT-PCR Kit (TaKaRa, Osaka, Japan). mRNA expression of genes was assessed using the QuantStudio 5 Real-Time PCR detection system (Applied Biosystems, Foster, CA, USA) in a final volume of 20 μl using the SYBR Premix Ex Taq II kit (Takara, Osaka, Japan). The relative mRNA abundance of nine PUFA-synthesis-related genes was assayed. The primers used are listed in Supplementary Table 1. Glyceraldehyde-3-phosphatedehydrogenase (GAPDH) and Beta-actin (β-ACTIN) were used as internal controls, and all assays were performed in triplicates. Relative transcriptional alterations were evaluated using 2–ΔΔCt.
Statistical analysis
The data were analyzed by one-way ANOVA using SPSS (version 17.0) in a completely randomized design with a model containing treatment as the main effect. The pen was used as the experimental unit to measure live weight, feed intake, and FCR. For the other indices, individual ducks were considered as experimental units. Data are expressed as mean ± standard deviation. Differences among the groups were considered significant at P < 0.05. When the treatment effect was significant, differences among the treatment means were detected using Duncan’s test.
Results
Growth performance and meat quality
The data presented in Table 3 show that dietary linseed oil had no effect on the BW of ducks at 56 days of age. Additionally, dietary linseed oil had no effect on the ADFI, ADG, or F/G of ducks at 28–56 days of age (P > 0.05). Physical properties (color, pH, water loss rate, and shear force) and chemical composition (moisture, protein, IMF, and collagen contents) of breast muscle in the linseed oil-supplemented diet groups are shown in Table 4. There were also no significant differences in these breast muscle indexes among groups fed different percentages of linseed oil diets (P > 0.05).
Fatty acid profile in the liver
The effects of dietary linseed oil on the FA composition in the liver are presented in Table 5. Different levels of dietary linseed oil had no effect (P > 0.05) on total saturated FA (SFA) or monounsaturated fatty acid (MUFA) concentrations in the liver. With the increase in dietary linseed oil supplement, the concentration of PUFA tended (P < 0.001) to increase significantly, and the contents of total n-3 PUFA, ALA, EPA, and DHA were significantly higher (P < 0.001) in the low, medium, and high groups than in the control group. There were no significant differences (P > 0.05) in total n-6 PUFA content among the groups. However, the DGLA and ARA contents were significantly lower (P < 0.01) in the medium and high groups than in the control group, whereas the LA content was significantly higher (P < 0.001) in the medium and high groups than in the low and control groups. Therefore, in the liver, the n-6 to n-3 PUFA ratio increased (P < 0.001) by 13.98, 8.70, 6.55, and 4.38 for ducks fed the control, low, medium, and high linseed oil diets, respectively.
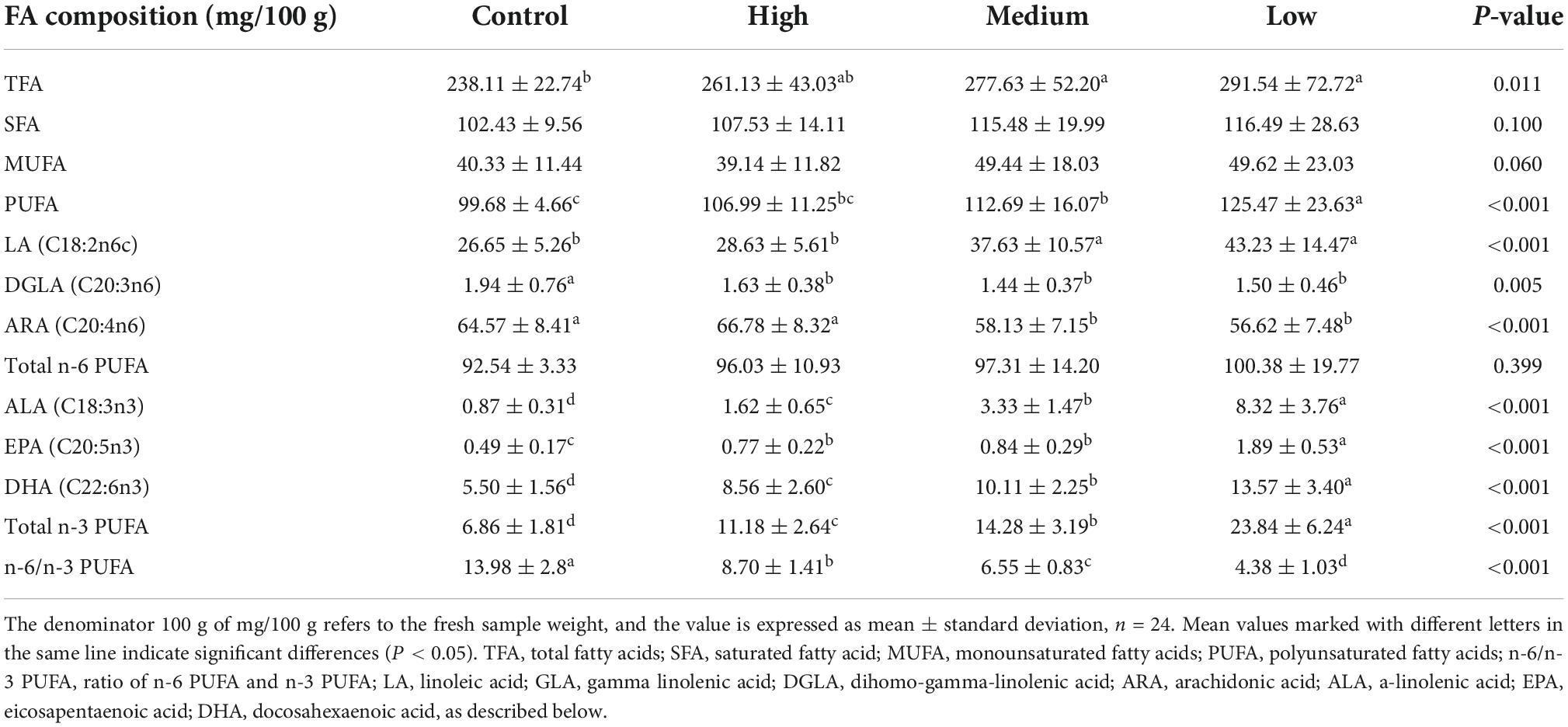
Table 5. Effects of different linseed oil levels on the polyunsaturated fatty acid (PUFA) profile of duck liver.
Fatty acid profile in the breast muscle
In the breast muscle, different levels of linseed oil diets had no effect (P > 0.05) on the concentrations of TFA, SFA, MUFA, n-6 PUFA, or ARA (Table 6). However, the contents of PUFA, LA, and DGLA increased (P < 0.05) with the increase in dietary linseed oil level. The concentrations of n-3 PUFA, ALA, and DHA were significantly (P < 0.001) higher in the control vs. low, low vs. medium, and medium vs. high dietary linseed oil. EPA was not detected in breast muscle in the control or low linseed oil groups, and it increased significantly (P < 0.001) in the medium vs. high linseed oil groups. In contrast, the proportion of n-6/n-3 PUFA ratios in the breast muscle decreased (P < 0.001) from 32.94 to 8.54 with increasing dietary linseed oil ratios.
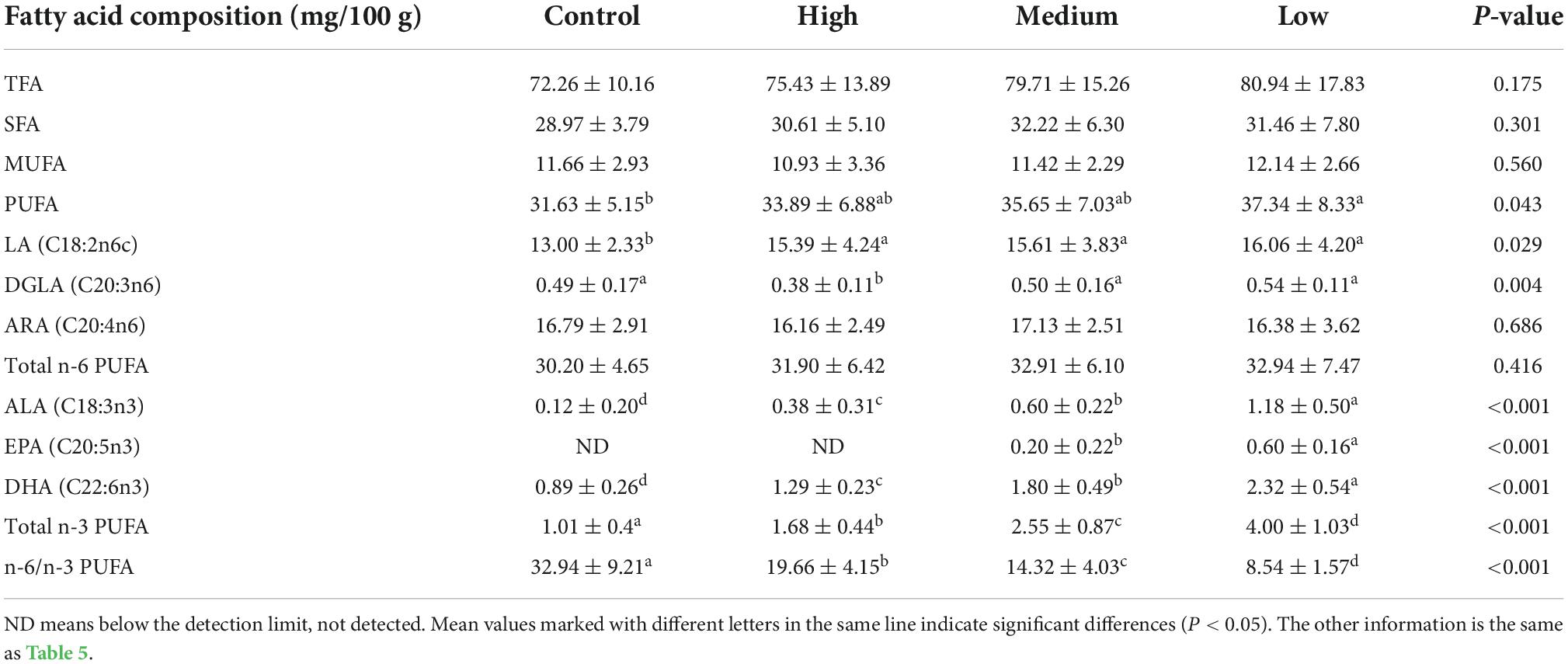
Table 6. Effects of different dietary linseed oil levels on the polyunsaturated fatty acid (PUFA) profile of duck breast muscle.
Correlation analysis
Group, sex, BW, and FA composition of the liver and breast muscle were analyzed for correlation analysis, and the results are shown in Figure 1. Linseed oil supplementation was positively (P < 0.001) correlated with n-3 PUFA levels in the liver and breast muscle and negatively (P < 0.001) correlated with the proportion of n-6: n-3 PUFA. Sex was not correlated with most indexes (P > 0.05) but was positively (P < 0.05) correlated with liver DGLA levels. BW was negatively correlated (P < 0.05) with n-3 PUFA levels, especially DHA levels, in the liver (P < 0.001). Total fatty acids, saturated fatty acids, monounsaturated fatty acids, and n-6 PUFAs in the liver and breast muscle showed little correlation; however, only DGLA in the liver and breast muscle showed a positive correlation (P < 0.01). n-3 PUFA, ALA, EPA, and DHA in the liver were positively (P < 0.001) correlated, and other n-3 PUFAs in the breast muscle were also significantly positively correlated (P < 0.001), except for EPA (with was undetected). There was a significant positive (P < 0.001) correlation between n-3 PUFAs in the liver and breast muscle (except for breast muscle EPA). These results indicate that dietary n-3 PUFAs had a great influence on the fatty acid composition of the body, and there was a correlation between the n-3 PUFA composition of the liver and breast muscle.
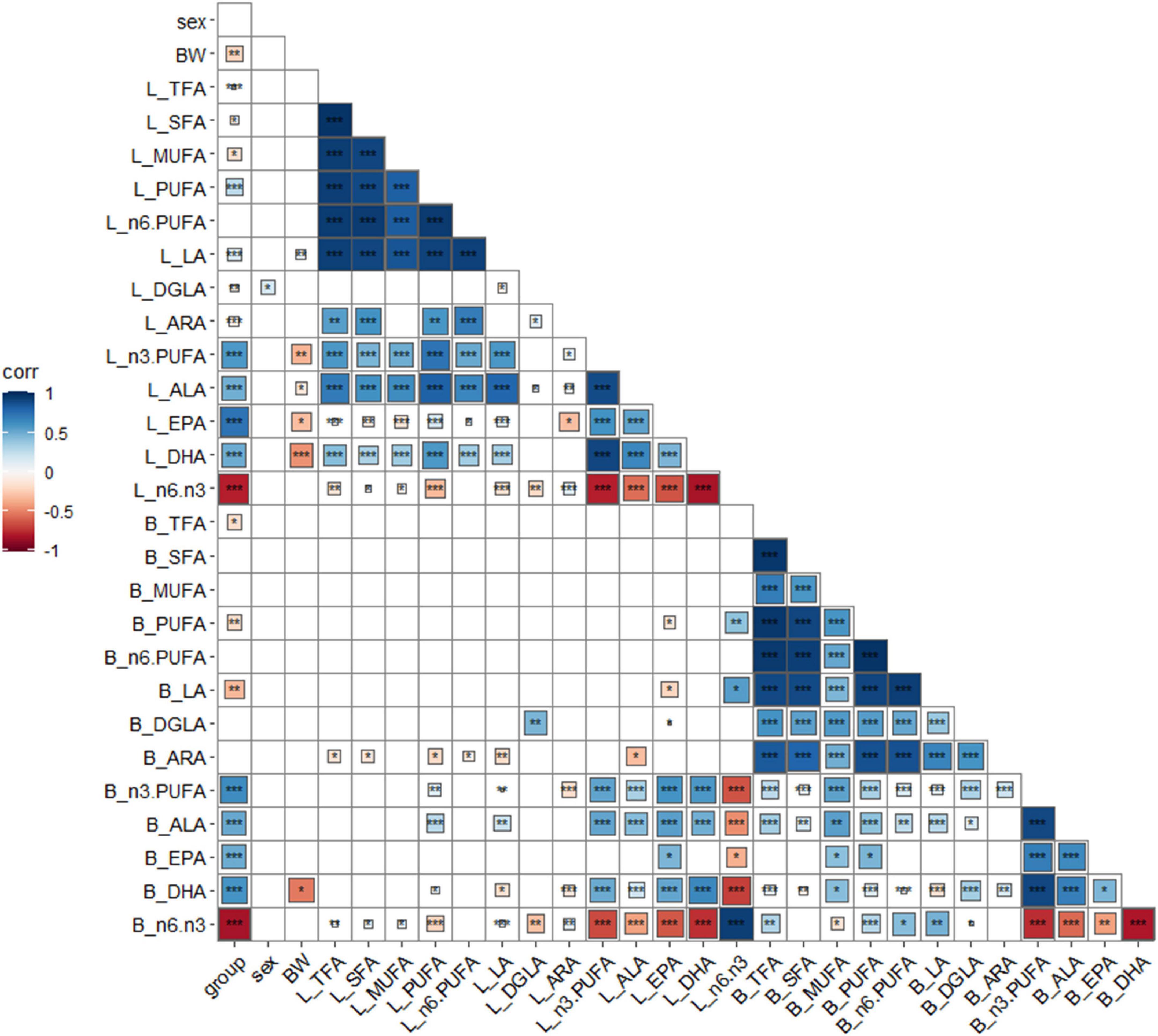
Figure 1. Correlation analysis of key indexes. *P < 0.05, **P < 0.01, ***P < 0.001, blue positive correlation, red negative correlation, darker color, higher correlation, lighter color, lower correlation. Group: different diet group; sex, sex; BW, body weight; B, breast muscle; L, liver.
Overview of transcriptome data
RNA-seq was performed on 10 male individuals: five duck livers or breast muscles with the lowest n-3 PUFA ratios (CL1, CL2, CL3, CL4, CL5 and CM1, CM2, CM3, CM4, CM5) and five duck livers with the highest n-3 PUFA ratios (HL1, HL2, HL3, HL4, HL5 and HM1, HM2, HM3, HM4, HM5). The PUFA compositions are shown in Supplementary Table 2. The read numbers and quality are shown in Supplementary Table 3. Clean reads were obtained after removing the low-quality sequences. The results indicated that more than 81% of the total clean reads of the 20 liver and breast muscle samples matched the reference sequences and more than 66% of the total clean reads were uniquely mapped to the reference sequences. The results demonstrated that all samples met the requirements for subsequent analysis. To assess intergroup differences and intragroup sample duplication, we performed PCA analysis on FPKM and read count of all samples, and the results showed same tissue aggregation and intergroup isolation (Figure 2A). The distribution of gene expression levels in different samples was shown in Figure 2B. And such data could produce a reliable basis for subsequent analysis.
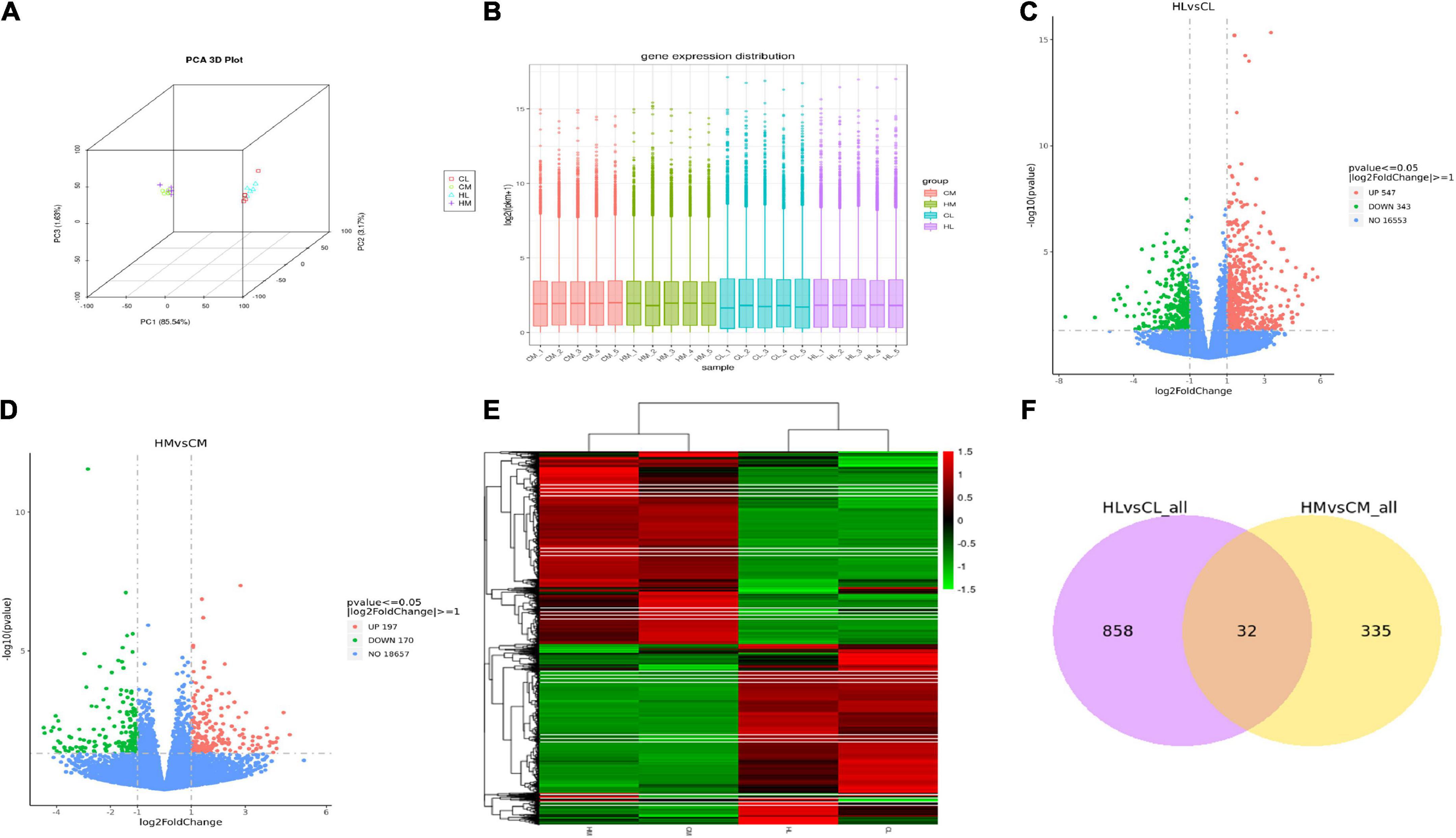
Figure 2. Transcriptomic analysis of liver and breast muscle tissues in the high linseed oil and control groups. (A) PCA 3D Plot of samples. (B) Box plot of sample gene expression distribution. (C) Volcano plots of the DEGs between HL and CL. (D) Volcano plots of the DEGs between HM and CM. (E) Cluster heatmap of the DEGs, red: upregulated genes; green: downregulated genes. (F) Venn diagram indicated unique and shared DEGs between HL vs. CL and HM vs. CM.
Identification of differentially expressed genes and functional enrichment analysis
A total of 9,859 genes were annotated in the duck livers and 10,426 genes were annotated in the duck breast muscles. With FDR < 0.05 and | log2-fold change| > 1 as the threshold, a total of 890 hepatic DEGs were identified including 547 upregulated genes and 343 downregulated genes (Figure 2C), and a total of 367 breast muscle DEGs were identified including 197 upregulated genes and 170 downregulated genes (Figure 2D). Other compassions were performed in Supplementary Figures 1A,B. There were 7876 DEGs between HL and HM, and there were 8003 DEGs between CL and CM. A hierarchical cluster analysis was performed by all DEGs, as shown in Figure 2E, there was considerable variation between livers and breast muscles. There were 32 common DEGs between HL vs. CL and HM vs. CM (Figure 2F). While there were 6785 common DEGs between HL vs. HM and CL vs. CM (Supplementary Figure 1C).
The KEGG analysis showed that the top 20 KEGG terms were showed in Figure 3. And there were 7 common pathways (cytokine-cytokine receptor interaction, calcium signaling pathway, progesterone-mediated oocyte maturation, linoleic acid metabolism, steroid hormone biosynthesis, toll-like receptor signaling pathway, and neuroactive ligand-receptor interaction) enriched by DEGs from both HL vs. CL and HM vs. CM. Differential genes in livers were mainly enriched in biosynthesis of unsaturated fatty acids (downregulated SCD, FADS1, FADS2, upregulated ACOT6), PUFAs (LA, ARA) metabolism (upregulated PTGS2, TBXAS1, PLA2G4A, and CYP3A40, downregulated GPX2, CYP2C16), PPAR signaling pathway (upregulated ANGPTL4, CPT1B, ACSL5, downregulated SCD, FABP2), steroid hormone biosynthesis (upregulated CYP3A40, ST2B1, downregulated COMT, CYP7A1, and CYP2C16). Differential genes in breast muscles were mainly enriched in LA or ALA acid metabolism (upregulated CYP3A1, PLA2), cytokine-cytokine receptor interaction (upregulated IL16, INHBA, CX3CL1, TNFRSF17, CCL20, downregulated CNTFR, BMPR1B, CXCL14), calcium signaling pathway (upregulated HRH1, MCOLN2, P2RX5, OXTR, MCOLN3, BDKRB1, downregulated CAMK1G, ADCY8), and steroid hormone biosynthesis (upregulated CYP3A1, ST2B1, downregulated CYPC21). Of course, because of the difference in tissue function between the liver and breast muscles, there were 12 common pathways enriched by DEGs from both HL vs. HM and CL vs. CM included by biosynthesis of unsaturated fatty acids, PPAR signaling pathway, primary bile acid biosynthesis, cardiac muscle contraction, calcium signaling pathway (Supplementary Figure 2).
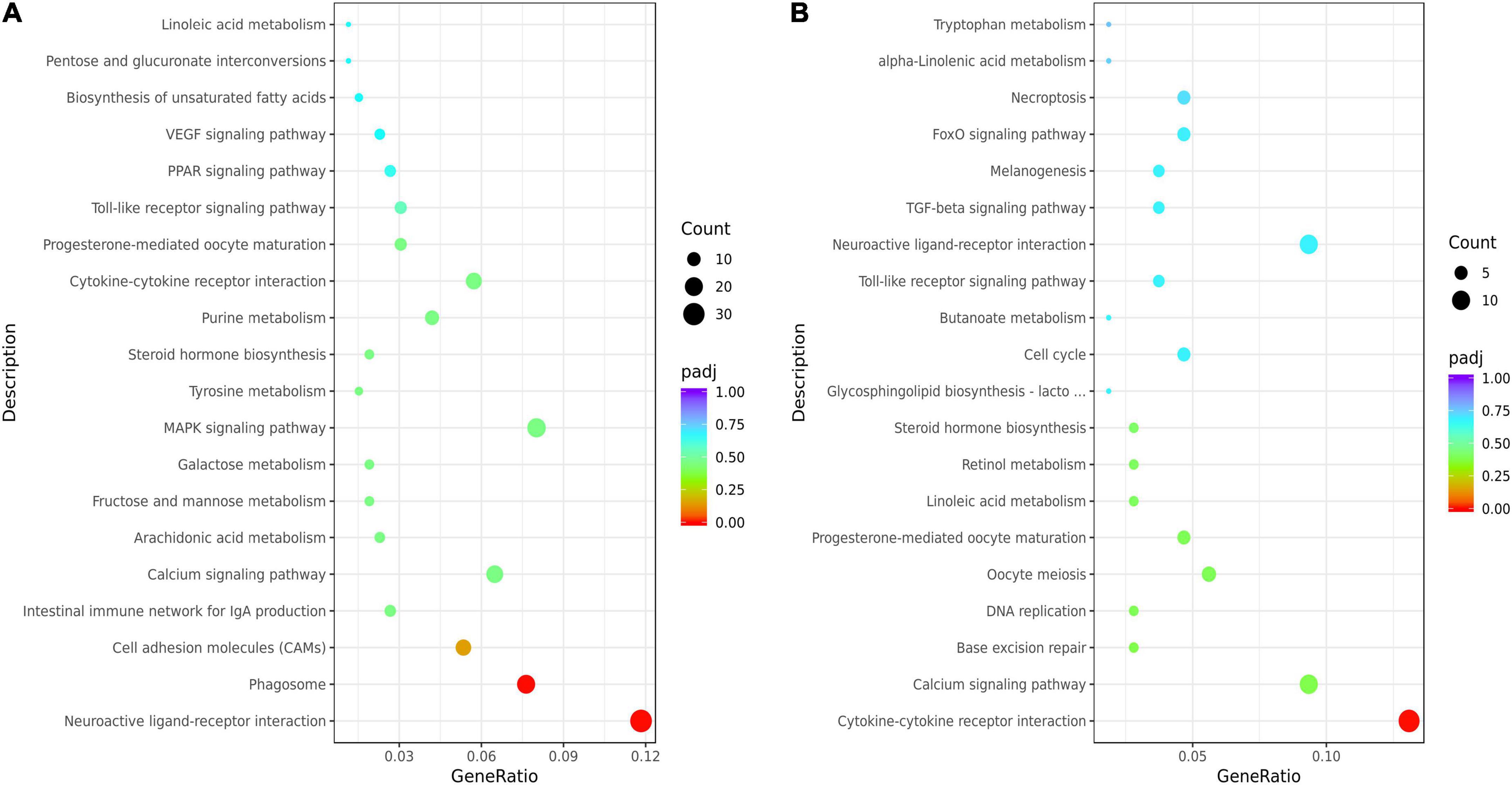
Figure 3. Kyoto Encyclopedia of Genes and Genomes (KEGG) enrichment analysis of the liver or breast muscle samples affected by linseed oil. (A) Bubble diagram of the top 20 KEGG pathway enrichments for the HL vs. CL comparisons. (B) Bubble diagram of the top 20 KEGG pathway enrichments for the HM vs. CM comparisons. The ordinate indicates the pathways.
RT-qPCR validation of key genes
Since the PUFA metabolic pathways were involved by the DEGs, We further selected the key genes (ELOVL2, ELOVL5, FADS1, FADS2, CPT1B, CPT2 and CAV1, CD36, FABP4, FABP5, FATP1, FATP6) related to n-3 polyunsaturated FA synthesis and transportation for quantitative verification. The expression levels of genes in the liver and breast muscle of ducks were shown in Figures 4A,B. The result was coincide with that of transcriptome result (Supplementary Figure 3). Dietary linseed oil supplementation had significant effects (P < 0.05) on the expression levels of liver desaturase and carbon chain extension enzyme genes. With the increase in linseed oil level, the expression levels of most genes first increased and then decreased. ELOVL2 and ELOVL5 mRNA expression levels were higher (P < 0.05) in the low linseed oil group than in the control, medium, and high linseed oil groups. Hepatic FADS1 and FADS2 mRNA expression levels were higher (P < 0.05) in the control and low-linseed-oil groups than in the medium and high-linseed-oil groups. Although there were changes in these genes in breast muscle, the differences were not statistically significant (P > 0.05). Hepatic FABP4 and FABP5 mRNA expression levels increased significantly (P < 0.05) in the medium and high linseed oil groups compared to that in the control and low linseed oil groups. Breast muscle CD36 mRNA expression increased (P < 0.05) in all linseed oil groups, whereas the expression levels of FATP6 were lower (P < 0.05) in the medium and high linseed oil groups than in the control and low linseed oil groups.
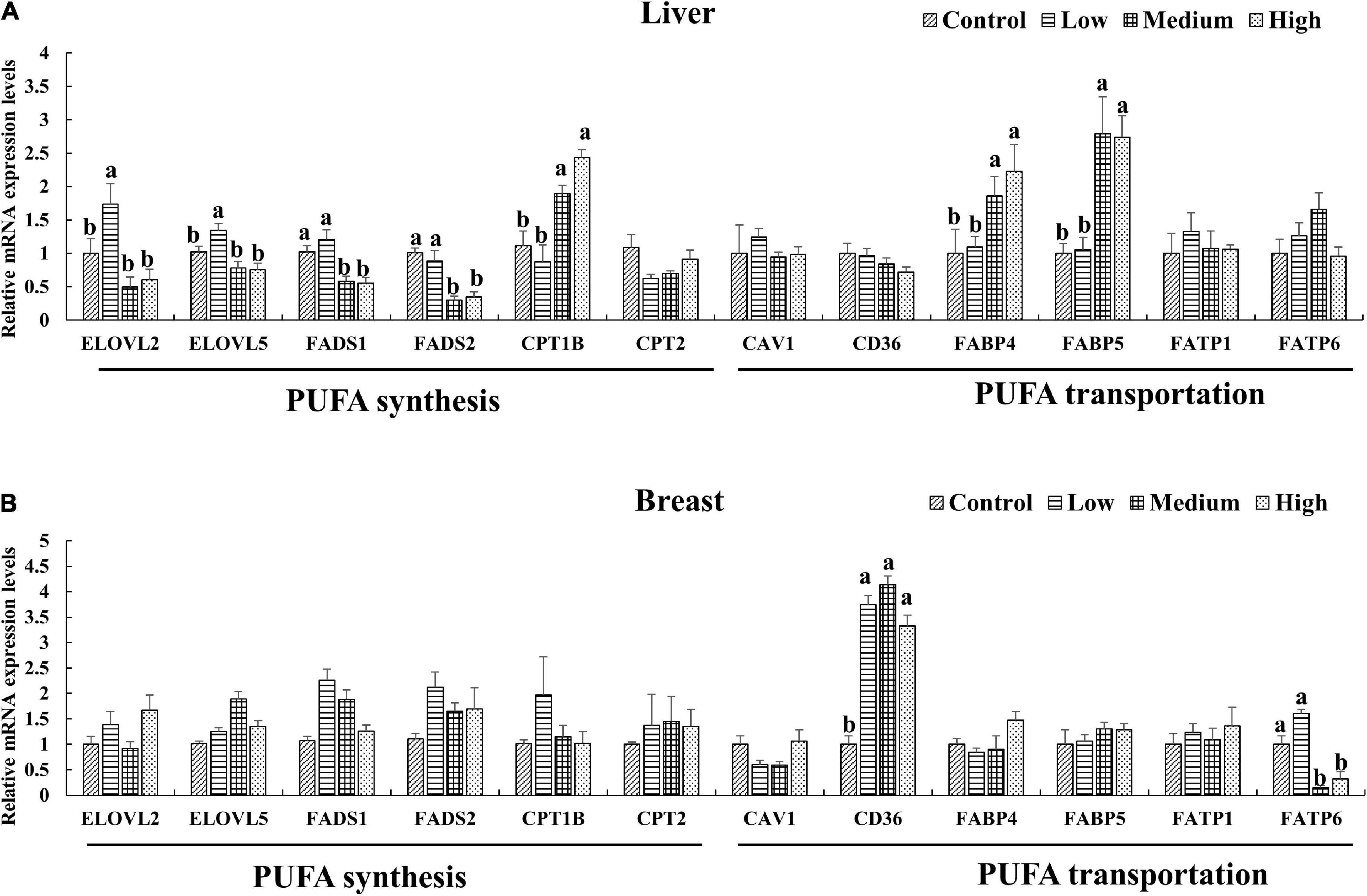
Figure 4. Expression of key genes verified by RT-qPCR in liver (A) and breast muscle (B). Mean values marked with different letters in the same column indicate significant differences (P < 0.05, n = 8).
Integrative analysis the effects of linseed oil on the polyunsaturated fatty acid transport and synthesis pathways in the liver and breast muscle
Combined with the results of the FAs profile and genes expression analyses, we constructed a model diagram of the effect of linseed oil on the liver and breast muscle of ducks (Figure 5). Supplementation with linseed oil increased the synthesis of n-3 PUFA and the content of LA in the liver and breast muscle and decreased the synthesis of n-6 PUFA downstream of LA in the liver. Moreover, linseed oil had a significant influence on PUFA synthesis in the liver. Based on the shared enzyme system of n-6 and n-3 PUFA synthesis, the different distribution of enzymes caused by the substrate ALA may be the main reason for the change in the liver PUFA profile. FABP4 and FABP5, which play an important role in liver PUFA transport, were upregulated in this organ. Linseed oil had little effect on PUFA synthesis genes in breast muscle, but the expression levels of CD36 increased significantly with the increase in linseed oil, suggesting that PUFA in breast muscle may be partly derived from the transport of other tissues such as the liver, and PUFA transporters CD36 play an important role in this process.
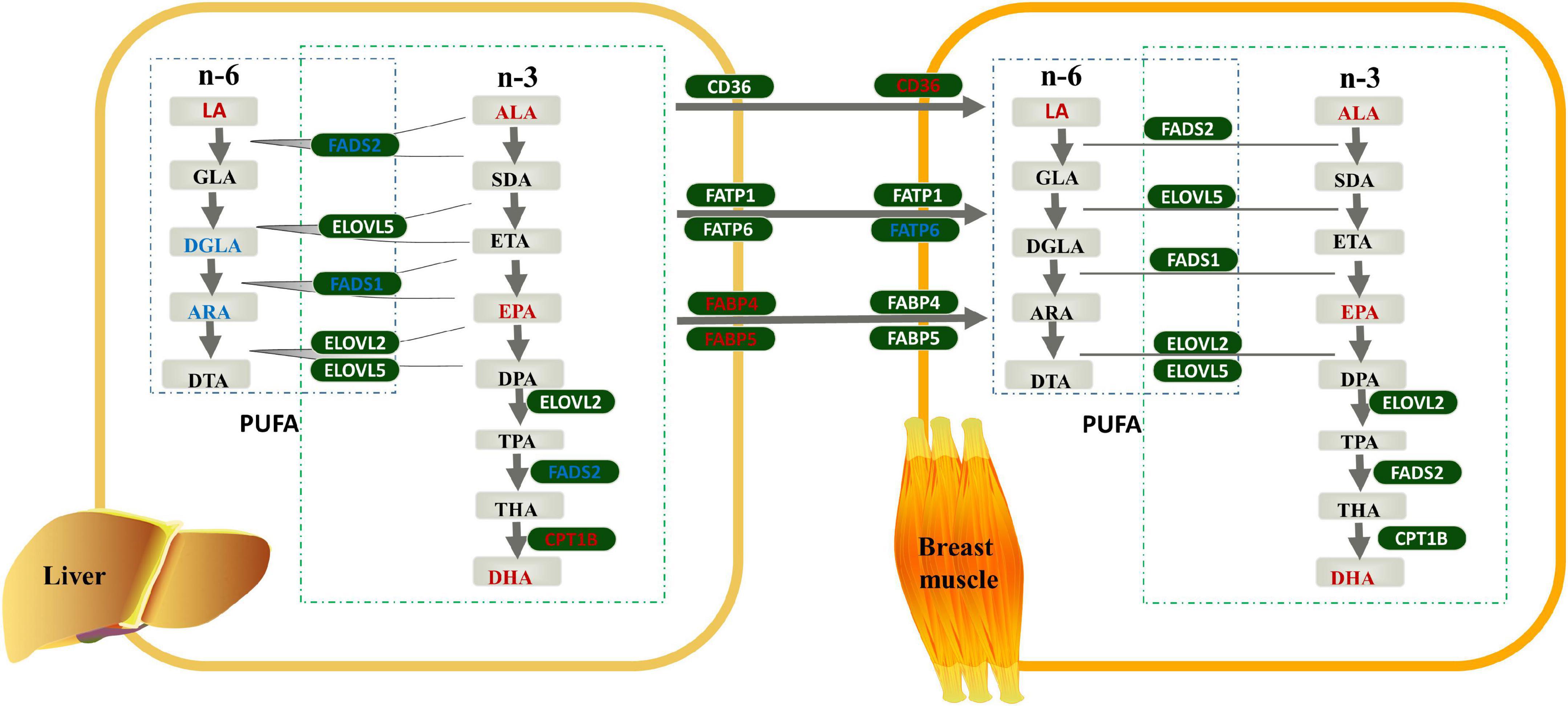
Figure 5. Effects of linseed oil on the polyunsaturated fatty acid (PUFA) synthesis and transport pathways in duck liver and breast muscle. Green boxes indicate genes, gray boxes indicate metabolites, red font indicate upregulated, blue font indicate downregulated.
Discussion
Linseed oil is the richest terrestrial source of n-3 fatty acids; it contains more than 35% ALA. n-3 PUFA have several health benefits, and high levels of such FAs in meat products would be desirable. Runzhou white-crested ducks were small sized meat ducks, with a marketing weight of about 1.4 kg. We evaluated the effects of dietary linseed oil on the liver and breast muscle of ducks. The results of this study reveal that diets with different amounts of linseed oil levels did not influence the body weight, ADFI, or FCR in ducks during the 28 d phase when dietary oil was supplemented at 0–2%. Similar findings have been reported in poultry fed diets supplemented with linseed oil (4, 20). This result could be attributed to the similar fat content in the experimental diets or the similar feed intake during the trial. Dietary linseed oil ratios had no effects on physical properties or chemical composition in duck breast muscle, which is consistent with corresponding results of both chickens and ducks (4, 21). While the study in pigs showed that high PUFA supplementation concentration improved IMF content and reduced meat color L* and pH 24 h, whereas low concentration decreased meat color b* and increased ADFI (22). Further, breast-meat sensory quality is not affected in broilers fed 100 g/kg flaxseed for 14 days (23). However, significant fishy odor and taste were recorded when chickens are fed a diet containing 7% linseed oil, whereas no significant differences in texture, tenderness, or juiciness of breast meat were reported (24).
In contrast to mammals, fat synthesis in birds is greater in the hepatic tissue and very limited in the adipose tissue. Fats that are metabolized in the liver are derived from three main sources: dietary fat, depot fat, and fat from de novo fatty acid synthesis (25). Dietary fatty acid composition and animal synthesis determine the fatty acid composition of the liver. Linseed oil is a valuable source of ALA in poultry diets and is effectively incorporated from the feed to the bird’s tissues (26). The n-3 PUFA synthesis pathway involves FADS1, FADS2, ELOVL2, and ELOVL5, which are affected by the nutritional status of the organism (11, 27, 28). Poultry have been shown to have strong elongating and desaturating activity during PUFA synthesis (10, 29, 30). Dietary linseed oil increases n-3 PUFA (ALA) concentrations and decreases n-6: n-3 PUFA ratios in birds fed diets. In this study, the contents of n-3 PUFAs (ALA, EPA, and DHA) increased in duck liver and breast muscle with increasing dietary linseed oil ratios, which is consistent with previous reports (31, 32). Correlation analysis results show that sex had no effect on most PUFA levels in breast muscles or livers, and previous studies have shown that sex had little effect on fatty acid composition in breast muscle and liver (33). In addition, most existing studies have focused on the detection of FA composition, and few studies have conducted correlation analysis. The results show that different linseed oil supplementation levels caused changes in fatty acids in the liver and breast muscle, and the content of n-3 fatty acids was significantly increased. There was no significant correlation between total fatty acids, SFA, MUFA, and n-6 PUFAs in the liver and breast muscle, whereas n-3 PUFA, ALA, EPA, and DHA levels in the liver and breast muscle were positively correlated, indicating that the change in n-3 PUFA induced by daily grain intake was systemic. The status of n-3 PUFA in other tissues can be roughly inferred from the level of n-3 PUFA in known tissues. In addition, BW was negatively correlated with n-3 PUFA levels, especially DHA levels, in the liver. Animal and human studies have also revealed that supplementation with EPA and DHA can prevent obesity and possibly improve BW in obese animals and humans (34).
Compared with the control group, the expression of genes related to polyunsaturated fatty acid synthesis, such as SCD, FADS1, and FADS2, were downregulated, while the expression of genes related to polyunsaturated fatty acid metabolism, such as PTGS2, TBXAS1, and PLA2G4A, was upregulated in the liver of ducks in the high linseed oil group. Cytosolic phospholipase A2 (cPLA2, PLA2G4A) catalyzes the release of arachidonic acid for prostaglandin synthesis by cyclooxygenase 1 (PTGS1), cyclooxygenase 2 (PTGS2), and thromboxane synthase (TBXAS1) (35). The DEGs, PTGS2, TBXAS1, and PLA2G4A, play important roles in downstream metabolites synthesis. n-6 PUFA (ARA) and n-3 PUFA (EPA, DHA) are two kinds of competitive substrates for the enzymes and products of PUFA metabolism (6). DHA- and EPA-derived eicosanoids antagonize the pro-inflammatory effects of ARA is as the substrate converted to prostaglandins, leukotrienes and other lipoxygenase or cyclooxygenase products. These products are important regulators of cellular functions with inflammatory, atherogenic and prothrombotic effects (2). Dietary linseed oil increased the synthesis of n-3 PUFAs in liver and promoted the expression of genes related to downstream anti-inflammatory metabolites synthesis, which is speculated to maintain the healthy state of ducks. Previous studies mainly focused on quantitative detection of genes related to PUFA synthesis, but little attention has been paid to the effect of dietary linseed oil on muscle transcriptome level. And we found that linseed oil mainly induced changes in the expression levels of genes in breast muscles, such as CYP3A1, PLA2, ST2B1, IL16, INHBA, TNFRSF17, which related to PUFA (linoleic acid or alpha-linolenic acid) and its metabolites (glycosphingolipid, steroid hormone, progesterone) metabolic pathways.
Genes related to n-3 PUFA synthesis and transportation were further verified by RT-qPCR. Most of the hepatic genes involved in PUFA synthesis showed the highest expression level in the low linseed oil group, and, as the proportion of linseed oil further increased, the gene expression levels decreased. This finding is consistent with that described by Li et al. (4). And the expression of FADS2 is proved to be changed over time according to the dietary n-6: n-3 ratio, supporting that metabolic enzymes of long-chain PUFA are regulated by dietary fatty acid composition (36). Besides, researchers have suggested that the consumption of an n-3 enriched diet stimulates mRNA expression of FADS2 owing to the higher affinity of FADS2 for n-3 than for n-6 (33, 37). Interestingly, the conversion of n-3 and n-6 fatty acids shares the same desaturase and elongase series. There is competition between the n-3 and n-6 fatty acid families; an excess of one leads to a significant reduction in the conversion of the other (24). We also found that hepatic LA increased, whereas the downstream n-6 PUFA (DGLA and ARA) decreased with increasing dietary linseed oil ratios. This may reflect the possibility that the increase in n-3 PUFA synthesis does not depend on the increase in related enzymes but on the increase in substrates (ALA) and the allotted enzyme proportion. The increased synthesis of EPA and DHA using ALA as a substrate competitively inhibits the transformation of LA to downstream DGLA and ARA.
The fatty acid binding protein (FABP) family comprises a series of small proteins that act as lipid uptake and intracellular transporters, including FABP4 and FABP5 (38). Both play an important role in lipid-related metabolic processes and are associated with liver fat, independent of obesity (39). FABP4/5-deficient mice do not exhibit any extension in their lifespan (40). In duck livers, linseed oil caused high expression levels of FABP4 and FABP5, and the supplement level of linseed oil was negatively correlated with the body weight. These results indicate that the increased expression levels of FABP4 and FABP5 in linseed oil-supplemented duck liver is related to the transport of PUFA, they but do not lead to body weight gain. CD36 is a high-affinity receptor that functions in muscle and adipose tissues uptake of long-chain fatty acids (41). Furthermore, the increasing CD36 protein of the muscle or the translocation of CD36 from an intracellular pool to the plasma membrane can promote LCFA transport (42). Our results show that CD36 was upregulated by linseed oil in the duck breast muscles. This is consistent with the results of previous studies (43, 44). FATPs are a group of transport proteins that mediate the uptake of long-chain fatty acids (45). The study in both human and mouse showed that sustained skin barrier disruption gives rise to increases in FATP1 and –6 levels, as well as a robust increase in the levels of CD36 protein (46). We found that linseed oil decreased the expression level of FATP6; however, whether this affects barrier changes in ducks is unknown, and there are few relevant studies on FATP6. The metabolic mechanism of PUFA in the duck body induced by dietary linseed oil is currently unclear and needs to be further investigated.
Conclusion
Dietary linseed oil supplementation for 28 days had little adverse effect on the performance and breast meat quality of ducks, but it enriched the meat with ALA, EPA, and DHA and decreased the n-6-to-n-3 ratio. Therefore, it is appropriate for health-conscious consumers. The levels of n-3 PUFA, especially DHA, in the liver were negatively correlated with the BW of ducks. The liver is an important tissue for PUFA synthesis. Members of the n-6 and n-3 FUFAs compete for the corresponding desaturase and elongase enzymes. The increase in ALA reduces the expression of FADS1 and FADS2, increases the expression of PTGS2, TBXAS1, and PLA2G4A in the liver, and the substrate-dependent PUFA metabolic pattern promotes the synthesis and metabolic pathways of n-3 PUFA, while inhibiting the synthesis and metabolic pathways of n-6 PUFA. n-3 PUFA in breast muscle is not only synthesized by itself but also derived from transport from other tissues, such as the liver. The expression levels of PUFA transport-related genes, such as FABP4 and FABP5 in the liver, and CD36 in the breast muscle are increased. This study provides nutrition- and gene-regulated perspectives for n-3 PUFA-enriched poultry production and reference for the effects of linseed oil intake on lipid metabolism in human. Further studies are needed to explore the effect of ALA on the relationship between cells (liver or breast muscle) and PUFA metabolism.
Data availability statement
The datasets presented in this study can be found in online repositories. The names of the repository/repositories and accession number(s) can be found below: https://www.ncbi.nlm.nih.gov/geo/, GSE214632.
Ethics statement
The animal study was reviewed and approved by the Institutional Animal Care and Use Committee of Yangzhou University.
Author contributions
GBC and LW conceived and designed the study. XH and AZ collected the samples. BD, LW, and ZW performed the experiments and analyzed the data. LW wrote the manuscript. GHC and ZW contributed to revisions of the manuscript. All authors read and approved the manuscript.
Funding
This work was supported by the China Agriculture Research System of MOF and MARA (grant: CARS-42) and the Jiangsu Agricultural Industry Technology System [grant: JATS(2021)326].
Conflict of interest
The authors declare that the research was conducted in the absence of any commercial or financial relationships that could be construed as a potential conflict of interest.
Publisher’s note
All claims expressed in this article are solely those of the authors and do not necessarily represent those of their affiliated organizations, or those of the publisher, the editors and the reviewers. Any product that may be evaluated in this article, or claim that may be made by its manufacturer, is not guaranteed or endorsed by the publisher.
Supplementary material
The Supplementary Material for this article can be found online at: https://www.frontiersin.org/articles/10.3389/fnut.2022.1030712/full#supplementary-material
Footnotes
- ^ https://ftp.ncbi.nlm.nih.gov/genomes/all/GCF/015/476/345/GCF_015476345.1_ZJU1.0/GCF_015476345.1_ZJU1.0_genomic.fna.gz
- ^ http://www.genome.jp/kegg/
References
1. Du X, Liu Y, Lu L, Wang W, Zeng T, Tian Y, et al. Effects of dietary fats on egg quality and lipid parameters in serum and yolks of Shan partridge duck. Poult Sci. (2017) 96:1184–90. doi: 10.3382/ps/pew348
2. Schmitz G, Ecker J. The opposing effects of n-3 and n-6 fatty acids. Prog Lipid Res. (2008) 47:147–55.
3. Naughton SS, Mathai ML, Hryciw DH, McAinch AJ. Linoleic acid and the pathogenesis of obesity. Prostaglandins Other Lipid Mediat. (2016) 125:90–9.
4. Li M, Zhai S, Xie Q, Tian L, Li X, Zhang J, et al. Effects of dietary n-6:n-3 PUFA ratios on lipid levels and fatty acid profile of cherry valley ducks at 15-42 days of age. J Agric Food Chem. (2017) 65:9995–10002. doi: 10.1021/acs.jafc.7b02918
5. Simopoulos AP. The importance of the ratio of omega-6/omega-3 essential fatty acids. Biomed Pharmacother. (2002) 56:365–79.
6. Marventano S, Kolacz P, Castellano S, Galvano F, Buscemi S, Mistretta A, et al. A review of recent evidence in human studies of n-3 and n-6 PUFA intake on cardiovascular disease, cancer, and depressive disorders: does the ratio really matter? Int J Food Sci Nutr. (2015) 66:611–22. doi: 10.3109/09637486.2015.1077790
7. Frahm HD, Rehkämper G. Brain size, brain composition and intracranial fat bodies in a population of free-living crested ducks (‘Hochbrutflugenten’). Br Poult Sci. (2004) 45:590–7. doi: 10.1080/00071660400006297
8. Lane K, Derbyshire E, Li W, Brennan C. Bioavailability and potential uses of vegetarian sources of omega-3 fatty acids: a review of the literature. Crit Rev Food Sci Nutr. (2014) 54:572–9. doi: 10.1080/10408398.2011.596292
9. Ghasemi Fard S, Wang F, Sinclair AJ, Elliott G, Turchini GM. How does high DHA fish oil affect health? A systematic review of evidence. Crit Rev Food Sci Nutr. (2019) 59:1684–727. doi: 10.1080/10408398.2018.1425978
10. Gregory MK, James MJ. Functional characterization of the duck and turkey fatty acyl elongase enzymes ELOVL5 and ELOVL2. J Nutr. (2014) 144:1234–9. doi: 10.3945/jn.114.194159
11. Cormier H, Rudkowska I, Lemieux S, Couture P, Julien P, Vohl MC. Effects of FADS and ELOVL polymorphisms on indexes of desaturase and elongase activities: results from a pre-post fish oil supplementation. Genes Nutr. (2014) 9:437. doi: 10.1007/s12263-014-0437-z
13. Canbay A, Bechmann L, Gerken G. Lipid metabolism in the liver. Z Gastroenterol. (2007) 45:35–41.
15. Kalakuntla S, Nagireddy NK, Panda AK, Jatoth N, Thirunahari R, Vangoor RR. Effect of dietary incorporation of n-3 polyunsaturated fatty acids rich oil sources on fatty acid profile, keeping quality and sensory attributes of broiler chicken meat. Anim Nutr. (2017) 3:386–91. doi: 10.1016/j.aninu.2017.08.001
16. Chen W, Jiang YY, Wang JP, Yan BX, Huang YQ, Wang ZX. Effect of flaxseed on the fatty acid profile of egg yolk and antioxidant status of their neonatal offspring in Huoyan geese. Animal. (2015) 9:1749–55. doi: 10.1017/S1751731115001287
17. Shahid MS, Wu Y, Xiao Z, Raza T, Dong X, Yuan J. Duration of the flaxseed diet promotes deposition of n-3 fatty acids in the meat and skin of Peking ducks. Adv Food Nutr Res. (2019) 63:3590. doi: 10.29219/fnr.v63.3590
18. Anderson S. Determination of fat, moisture, and protein in meat and meat products by using the FOSS FoodScan near-infrared spectrophotometer with FOSS artificial neural network calibration model and associated database: collaborative study. J AOAC Int. (2007) 90:1073–83.
19. Love MI, Huber W, Anders S. Moderated estimation of fold change and dispersion for RNA-seq data with DESeq2. Genome Biol. (2014) 15:550. doi: 10.1186/s13059-014-0550-8
20. Olomu JM, Baracos VE. Influence of dietary flaxseed oil on the performance, muscle protein deposition, and fatty acid composition of broiler chicks. Poult Sci. (1991) 70:1403–11. doi: 10.3382/ps.0701403
21. Zhao D, Li X, Yao T, Yang X, Shi Y, Li J, et al. Effects of extruded flaxseed on growth performance, slaughter performance, plasma biochemical indexes, antioxidant capacity and muscle n-3 polyunsaturated fatty acid deposition of Beijing Fatty Chicken. Chin J Anim Nutr. (2022) 34:274–84.
22. Wang L, Huang Y, Wang Y, Shan T. Effects of polyunsaturated fatty acids supplementation on the meat quality of pigs: a meta-analysis. Front Nutr. (2021) 8:746765. doi: 10.3389/fnut.2021.746765
23. Gonzalez-Esquerra R, Leeson S. Effects of menhaden oil and flaxseed in broiler diets on sensory quality and lipid composition of poultry meat. Br Poult Sci. (2000) 41:481–8. doi: 10.1080/713654967
24. Kartikasari LR, Hughes RJ, Geier MS, Makrides M, Gibson RA. Dietary alpha-linolenic acid enhances omega-3 long chain polyunsaturated fatty acid levels in chicken tissues. Prostaglandins Leukot Essent Fatty Acids. (2012) 87:103–9.
25. Zaefarian F, Abdollahi MR, Cowieson A, Ravindran V. Avian liver: the forgotten organ. Animals. (2019) 9:63. doi: 10.3390/ani9020063
26. Abbasi F, Samadi F, Jafari SM, Ramezanpour S, Shams Shargh M. Ultrasound-assisted preparation of flaxseed oil nanoemulsions coated with alginate-whey protein for targeted delivery of omega-3 fatty acids into the lower sections of gastrointestinal tract to enrich broiler meat. Ultrason Sonochem. (2019) 50:208–17. doi: 10.1016/j.ultsonch.2018.09.014
27. Nakamura MT, Nara TY. Structure, function, and dietary regulation of delta6, delta5, and delta9 desaturases. Annu Rev Nutr. (2004) 24:345–76. doi: 10.1146/annurev.nutr.24.121803.063211
28. Castro LF, Tocher DR, Monroig O. Long-chain polyunsaturated fatty acid biosynthesis in chordates: insights into the evolution of Fads and Elovl gene repertoire. Prog Lipid Res. (2016) 62:25–40.
29. Osman RH, Liu L, Xia L, Zhao X, Wang Q, Sun X, et al. Fads1 and 2 are promoted to meet instant need for long-chain polyunsaturated fatty acids in goose fatty liver. Mol Cell Biochem. (2016) 418:103–17. doi: 10.1007/s11010-016-2737-7
30. Gregory MK, Geier MS, Gibson RA, James MJ. Functional characterization of the chicken fatty acid elongases. J Nutr. (2013) 143:12–6.
31. Mandal GP, Ghosh TK, Patra AK. Effect of different dietary n-6 to n-3 fatty acid ratios on the performance and fatty acid composition in muscles of broiler chickens. Asian Australas J Anim Sci. (2014) 27:1608–14.
32. Talahalli RR, Vallikannan B, Sambaiah K, Lokesh BR. Lower efficacy in the utilization of dietary ALA as compared to preformed EPA + DHA on long chain n-3 PUFA levels in rats. Lipids. (2010) 45:799–808. doi: 10.1007/s11745-010-3464-6
33. Poureslami R, Raes K, Huyghebaert G, De Smet S. Effects of diet, age and gender on the polyunsaturated fatty acid composition of broiler anatomical compartments. Br Poult Sci. (2010) 51:81–91. doi: 10.1080/00071660903419518
34. Buckley JD, Howe PR. Anti-obesity effects of long-chain omega-3 polyunsaturated fatty acids. Obes Rev. (2009) 10:648–59.
35. Barroeta AC. Nutritive value of poultry meat: relationship between vitamin E and PUFA. Worlds Poultry Sci J. (2007) 63:277–84.
36. Jing M, Gakhar N, Gibson RA, House JD. Dietary and ontogenic regulation of fatty acid desaturase and elongase expression in broiler chickens. Prostaglandins Leukot Essent Fatty Acids. (2013) 89:107–13. doi: 10.1016/j.plefa.2013.05.006
37. Gómez Candela C, Bermejo López LM, Loria Kohen V. Importance of a balanced omega 6/omega 3 ratio for the maintenance of health: nutritional recommendations. Nutr Hosp. (2011) 26:323–9. doi: 10.1590/S0212-16112011000200013
38. Guaita-Esteruelas S, Gumà J, Masana L, Borràs J. The peritumoural adipose tissue microenvironment and cancer. The roles of fatty acid binding protein 4 and fatty acid binding protein 5. Mol Cell Endocrinol. (2018) 462:107–18. doi: 10.1016/j.mce.2017.02.002
39. Westerbacka J, Kolak M, Kiviluoto T, Arkkila P, Sirén J, Hamsten A, et al. Genes involved in fatty acid partitioning and binding, lipolysis, monocyte/macrophage recruitment, and inflammation are overexpressed in the human fatty liver of insulin-resistant subjects. Diabetes. (2007) 56:2759–65. doi: 10.2337/db07-0156
40. Charles KN, Li MD, Engin F, Arruda AP, Inouye K, Hotamisligil GS. Uncoupling of metabolic health from longevity through genetic alteration of adipose tissue lipid-binding proteins. Cell Rep. (2017) 21:393–402. doi: 10.1016/j.celrep.2017.09.051
41. Pepino MY, Kuda O, Samovski D, Abumrad NA. Structure-function of CD36 and importance of fatty acid signal transduction in fat metabolism. Annu Rev Nutr. (2014) 34:281–303. doi: 10.1146/annurev-nutr-071812-161220
42. Luiken JJ, Miskovic D, Arumugam Y, Glatz JF, Bonen A. Skeletal muscle fatty acid transport and transporters. Int J Sport Nutr Exerc Metab. (2001) 11(Suppl.):S92–6.
43. Chorner Z, Barbeau PA, Castellani L, Wright DC, Chabowski A, Holloway GP. Dietary α-linolenic acid supplementation alters skeletal muscle plasma membrane lipid composition, sarcolemmal FAT/CD36 abundance, and palmitate transport rates. Am J Physiol Regul Integr Comp Physiol. (2016) 311:R1234–42. doi: 10.1152/ajpregu.00346.2016
44. DeMoranville KJ, Carter WA, Pierce BJ, McWilliams SR. Flight training in a migratory bird drives metabolic gene expression in the flight muscle but not liver, and dietary fat quality influences select genes. Am J Physiol Regul Integr Comp Physiol. (2020) 319:R637–52. doi: 10.1152/ajpregu.00163.2020
45. Huang J, Zhu R, Shi D. The role of FATP1 in lipid accumulation: a review. Mol Cell Biochem. (2021) 476:1897–903.
Keywords: n-3 PUFA, n-6 PUFA, FADS, CD36, duck
Citation: Wang L, Dong B, Yang T, Zhang A, Hu X, Wang Z, Chang G and Chen G (2022) Dietary linseed oil affects the polyunsaturated fatty acid and transcriptome profiles in the livers and breast muscles of ducks. Front. Nutr. 9:1030712. doi: 10.3389/fnut.2022.1030712
Received: 29 August 2022; Accepted: 07 October 2022;
Published: 28 October 2022.
Edited by:
Eugenio Aprea, University of Trento, ItalyReviewed by:
Tao Zeng, Zhejiang Academy of Agricultural Sciences, ChinaFrancesco Gai, Institute of Sciences of Food Production (CNR), Italy
Copyright © 2022 Wang, Dong, Yang, Zhang, Hu, Wang, Chang and Chen. This is an open-access article distributed under the terms of the Creative Commons Attribution License (CC BY). The use, distribution or reproduction in other forums is permitted, provided the original author(s) and the copyright owner(s) are credited and that the original publication in this journal is cited, in accordance with accepted academic practice. No use, distribution or reproduction is permitted which does not comply with these terms.
*Correspondence: Guobin Chang, Z2JjaGFuZzE5NzVAeXp1LmVkdS5jbg==; Guohong Chen, Z2hjaGVuQHl6dS5lZHUuY24=